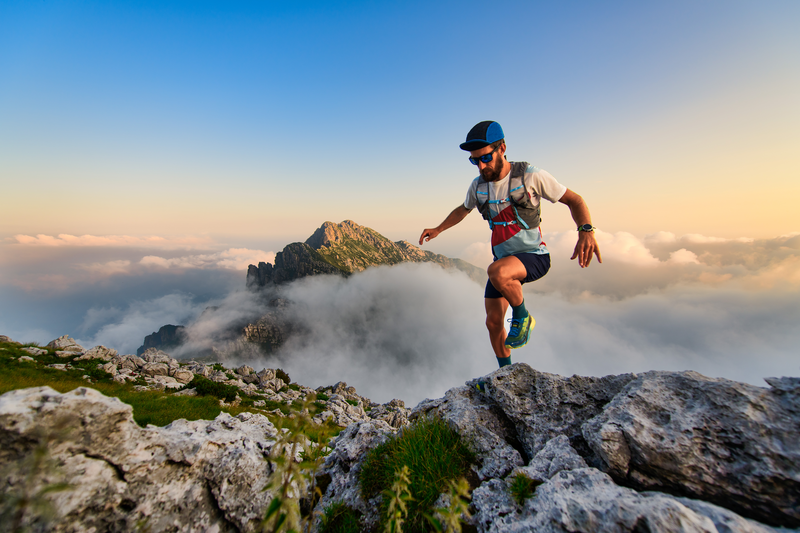
94% of researchers rate our articles as excellent or good
Learn more about the work of our research integrity team to safeguard the quality of each article we publish.
Find out more
ORIGINAL RESEARCH article
Front. Vet. Sci. , 29 August 2022
Sec. Animal Nutrition and Metabolism
Volume 9 - 2022 | https://doi.org/10.3389/fvets.2022.971783
The aim of this study was to compare the influence of different compositions of creep compound feed (CCF) (C-I – control group; TG-II – a CCF containing wheat bran extruded and fermented with L. paracasei; TG-III – a creep compound feed containing sugar beet pulp) on the piglets' growth performance, blood parameters, fecal microbial profile and physicochemical characteristics. Moreover, the fecal volatile compound (VC) profile was analyzed as a possible chemical marker related to changes in the fecal microbial profile and physicochemical characteristics. A 21-day experiment was conducted using 1-day-old 300 Large White/Norwegian Landrace piglets. The highest body weight (at the 21st day) was found in piglets of the TG-III group, and both treated groups showed lower feed conversion ratios. At the end of the experiment, significantly higher lactobacillus counts in the feces of both treated groups were found, and a correlation between fecal textural hardness and the lactobacillus count was established (r = 0.475). Significant correlations of piglets' individual fecal VC with microbiological parameters and fecal pH were established [lactobacilli with 3-n-nonadecanol-1; enterobacteria with butyric acid <2-methyl->; pentanoic acid, 4-methyl-; eicosene(E)-, etc.]. It can be concluded that local material could be successfully incorporated into CCF preparation without impairing animal metabolism.
An effective method of increasing the sustainability of the livestock chain is to include more local material for animal nutrition, with the aim of reducing exports and increasing the stability of the local industry by reducing its dependence on the import of raw materials. Feed production and the feed base are the bases for the sustainable development of highly productive animal husbandry In addition, feed production is especially important for the sustainable use of local agricultural resources, the reduction of problems related to undesirable climate changes and the overall public satisfaction of having an opportunity to choose local products prepared by using local stock.
The food industry generates large amounts of different valuable by-products, which have been undervalued until now. As an example, to date, wheat processing by-products, have been used without any pre-treatment as a feed stock of low nutritional value; however, their functional value could be increased and their safety characteristics improved (1). It was reported that, in Europe, more than 45 million tons/year of wheat and oats are processed, generating more than 6.5 million tons of bran that are further used for the livestock industry (2). Cereal brans are an abundant source of valuable compounds; in addition, their pre-treatment could increase their functional value and safety characteristics (1). Our previous studies showed that the nutritional and functional value of wheat bran (WB) as well as its safety parameters could be improved by using combined extrusion and fermentation technology under optimized conditions [selected extrusion parameters and the lactic acid bacteria (LAB) strain used for fermentation] (1). Also, during the extrusion and fermentation processes, the mycotoxin concentration in WB is reduced, and this parameter is key, especially when further material is used for piglet nutrition, as piglets are very sensitive to low mycotoxin concentrations in feed. Our previous studies also showed that feed fermented with selected LAB strains could positively affect the piglets' microbiota and lead to better detoxification of mycotoxins in vivo (3). Also, it was reported, that the abundance of bifidobacteria in fecal microbiota was significantly higher for weaned piglets fed the WB diet than the piglets fed with other dietary fiber rich sources, because of WB arabinoxylans (4).
In this study, we hypothesized that extruded and fermented WB, when included in the composition of creep compound feed (CCF), could lead to desirable changes in the microbiota of piglet feces, which could lead to better health parameters and growth performance.
Another raw material that could be obtained locally is sugar beet pulp. The latter could be a valuable ingredient in CCF for piglets. It was reported that sugar beet fiber consists, on average, of 29% hemicelluloses, 23% cellulose, 22.3% uronic acids, ~1–2% lignin, ~7–8% protein, 7.5–12% ash and ~0.5% residual sucrose. Previous studies showed that the inclusion of 12% sugar beet pulp in weaned piglets' diets can improve their health status and growth performance (5). However, the abovementioned study does not provide clear evidence of these positive changes. It was reported, that the sugar beet pulp supplementation increased Lactobacillus counts in piglets feces (6). These desirable changes were explained, by the influence of sugar beet pulp soluble dietary fiber compounds, which increasing the number and activity of microbes in the large intestine, and even in the ileum, as well as a reduction in pH, which accelerating growth of beneficial bacteria, i.e., Lactobacillus (7). In this study we hypothesized that the positive influence on piglet health could be related to desirable changes in the microbial composition of the digestive tract, which has a further positive influence on the main health parameters and growth performance.
Finally, piglet weaning under industrial conditions involves many stressors, including a change in diet from sow's milk to solid feed. Reducing the nutritional stressors at weaning may help to mitigate weaning-related problems by habituating piglets to solid feed as an alternative energy source and may reduce neophobia for the weaner diet. The early-life microbial colonization is the most important time for shaping intestinal and immune development (8). Piglets face many early-life events that shape the acquisition and development of their intestinal microbiota, as well as CCF could be one from the factors, which can be involved in this process.
The aim of this study was to compare the influences of different compositions of CCF on the piglets' growth performance, blood parameters, fecal microbial profile and physicochemical characteristics. In addition, fecal volatile compounds were identified as potential chemical markers related to changes in the fecal microbial profile and physicochemical characteristics.
Processed (extruded and fermented) WB as obtained from the SME “Ustukiu malunas” (Pasvalys, Lithuania). Physico-chemical and microbiological characteristics of the extruded and fermented WB were reported by Zokaityte et al. (1). For piglet feeding (to minimize the mycotoxin content (Wex130/screwspeed25Lpa), were selected. Physicochemical, antimicrobial, and antifungal characteristics of the Wex130/screwspeed25Lpa are given in Supplementary material 1.
Sugar beet pulp (dried) was obtained from the company Imlitex Agro (Kaunas, Lithuania). The composition of sugar beet pulp was moisture – 12.0%, crude protein – 10.4%, crude fat – 0.850%, crude fiber – 17.8%, calcium – 1.34% and phosphorus – 0.120%.
The study was conducted on a pig farm in the Silales district (Misuciai, Lithuania) and at the Institute of Animal Rearing Technologies, Lithuanian University of Health Sciences (Kaunas, Lithuania). A 21-day experiment was conducted using 300 1-day-old Large White / Norwegian Landrace (LW/NL) piglets (100 piglets in each group). The farrowing crates (containing a heated creep mat for piglets) were 4.32 m2 (1.8 × 2.4 m), of which 0.15 m2 per piglet and 1.76 m2 per sow. The control and trial groups contained 10 replications. In one box was one sow with 10 suckling piglets. The groups were formed on an analog basis. The sows were fed with compound feed [in accordance with NRC (2012) requirements]. Drinking water was available ad libitum throughout the trial. The trial started with piglets at an initial body weight of 1.76–1.81 kg in all (control and both treatment) groups. The piglet's diet before the trial was composed of 20.0% crude protein, 3.01% crude fiber, 6.26% crude fats, 1.50% av. lysine, 0.610% av. methionine, 0.320% av. tryptophan, 0.940% av. threonine, 0.800% Ca and 0.400% av. P. Antibiotic treatment was not applied.
The piglets were distributed into three groups. Three dietary treatments were compared: (i) control CCF (C-I – control group), (ii) CCF containing Wex130/screwspeed25Lpa (TG-II) and (iii) CCF containing sugar beet pulp (TG-III). All animal groups were fed with wet feed (water and feed in a 3:1 ratio), and the equipment used for feeding was WEDA (Dammann & Westerkamp GmbH, Germany). The amount of CCF in the feed trough was checked at least twice daily to provide the CCF ad libitum, and all CCF was replaced at each feed weigh-back to maintain the freshness of the feed.
The piglets' growth performance was evaluated by testing all 100 piglets from each group; microbiological feces parameters were analyzed by testing 40 piglets from each group, and other piglet parameters were evaluated by testing 10 piglets from each group. The basal feed was formulated according to the nutritional requirements described in (9). The feed composition and nutritional values are shown in Table 1. Dietary contents were analyzed according to the AOAC recommendations (10).
Group body weight (BW) gain was recorded on days 1, 10, 17, and 21 of age using an electronic weighing system (model type: IT1000, SysTec GmbH Bergheim, Germany). The feed conversion ratio (FCR) was calculated from feed intake (87% of dry matter) and BW gain, which was recorded on the same days as BW gain using a WEDA (Dammann & Westerkamp GmbH, Germany) automated feeding system that has an electronic flowmeter and weighing system.
Piglets were bled from the jugular vein into vacuum blood tubes (BD Vacutainer, United Kingdom) before the morning feeding. Tubes with clot activator were used for biochemical examination. To avoid stressing new-born piglets, blood biochemical variables were evaluated after the experiment on day 18 of the piglet's life. The parameters included aspartate aminotransferase (AST), alanine aminotransferase (ALT), cholesterol (CHOL) (mmol), high-density lipoprotein cholesterol (HDL-C), low-density lipoprotein (LDL-C) cholesterol, triglycerides (TG), total protein (TP), albumin (ALB), triiodothyronine (T3), thyroxine (T4), immunoglobulins IgA, IgM and IgG, glucose (GLU), creatinine analyzed by the Jaffe method (CREA), alkaline phosphatase (AP), thyroid-stimulating hormone (TSH), total bilirubin and urea. Blood parameters were analyzed with an automatic biochemistry analyser in the accredited laboratory “Anteja” (Klaipeda, Lithuania).
The fecal pH was analyzed with a pH meter (Inolab 3, Hanna Instruments, Italy). The dry matter (DM) of the feces was evaluated after drying the samples at 103 ± 2°C to a constant weight. The color coordinates were measured at three different points on the fecal sample surface using the CIE L*a*b* system (CromaMeter CR-400, Konica Minolta, Tokyo, Japan).
Feces were prepared for gas chromatography (GC) analysis by using solid-phase microextraction (SPME). An SPME device with Stableflex (TM) fiber, coated with a 50-μm DVB-PDMS-Carboxen™ layer (Supelco, USA), was used for sample preparation. For gas chromatography–mass spectrometry (GC-MS), a GCMS-QP2010 (Shimadzu, Japan) was used. The gas chromatograph was equipped with an AOC-5000 Plus Shimadzu autosampler, upgraded with an SPME analysis kit. Analysis was performed according to the procedure described by Vadopalas et al. (11).
Fecal samples were collected from 40 piglets from each group before (at day 1) and after the experiment (at day 21), stored in vials (+4°C) with transport medium (Faecal Enteric Plus, Oxoid, Basingstoke, UK) and analyzed on the same day. Evaluation of microbiological parameters [LAB, total bacteria count (TBC), total enterobacteria count (TEC), Enterococcus faecalis count and yeast and mold counts (Y/M)] was performed according to methods described by Zavistanaviciute et al. (12).
Before the piglets were divided into groups, feces from 10 healthy piglets (from each group) were taken, and a single pooled sample was prepared for microbiome profiling using next generation targeted sequencing of 16S rRNA. After the experiment, feces from 10 piglets from the control and both treated groups were collected (30 samples in total), and thereafter, three pooled samples representing each of the groups were prepared. Samples were stored in DNA/RNA Shield (1:10 dilution; R1100-250, Zymo Research, USA) at −70°C until DNA extraction. DNA was extracted with a fecal DNA MiniPrep kit (D6010, Zymo Research, USA). Metagenomic libraries were prepared, sequenced, quality controlled, and assembled in an independent service laboratory (Baseclear, the Netherlands). Short paired sequence reads were generated using the Illumina MiSeq system (Illumina, USA) and converted into FASTQ files using the BCL2FASTQ pipeline software, version 1.8.3 (Illumina). Quality trimming was applied based on Phred quality scores. Subsequently, the Illumina paired reads were merged into single reads (so-called “pseudoreads”) through sequence overlap. Chimeric pseudoreads were removed, and the remaining reads were aligned to a combination of the GreenGenes and RDP 16S gene databases. Based on the alignment scores of the pseudoreads, the taxonomic classes were assigned by associating each pseudoread to the best matching Operational Taxonomic Unit (OTU). ZymoBIOMICS Microbial Community Standard (D6300, Zymo Research, Murphy Ave. Irvine, CA, USA) was used as a microbiome profiling quality control. The results of taxonomic classification were visualized using the interactive online platform (https://genome-explorer.com).
All animal procedures were conducted according to the EU Directive of the European Parliament and of the Council from September 22, 2010 (13) on the protection of animals used for scientific purposes and Requirements for the Keeping, Maintenance, and Use of Animals Intended for Science and Education Purposes, approved by the order of the Lithuanian Director of the State Food and Veterinary Service (14). Research was carried out in accordance with the Republic of Lithuania Act (15).
In order to compare the influence of different compositions of creep compound on the fecal microbial profile and physicochemical characteristics, blood parameters and growth performance in piglets, multivariate general linear model (GLM) was used for data analysis (SPSS for Windows, Ver.15.0, SPSS, Chicago, Illinois, USA). The p-values of factors interaction (experiment day × treatment type) were determined by tests of between-subjects effects in multivariate GLM. Baseline measurements were used as covariates to account for the experimental conditions. The mean values were compared using Duncan's multiple range post-hoc test with the significance level defined at p ≤ 0.05. In the tables, the results are presented as mean values with pooled standard errors. Pearson correlations were also calculated; correlations were considered significant when p ≤ 0.05. Differences in bacterial genera between the groups were assessed using the Z-test calculator for two population proportions (Social Science Statistics, socscistatistics.com, 2019). The number of reads of each genera from the total number of reads in samples was counted. Relative abundance of the genera in samples then were compared between the groups. Two-tailed hypothesis was counted. For the quality control of taxonomical identification, standard (D6300, Zymo Research, Murphy Ave. Irvine, CA, USA) of mixed known bacterial cultures were sequenced together with sample sequencing. Statistical comparisons were considered significant when p ≤ 0.05.
In a comparison of body weight (BW) gain in piglets, significantly higher BW in piglets of the TG-III group at 21 days was established (Figure 1A). By comparison, the feed conversion ratio (FCR) after 21 days in both treated groups was lower, in comparison with the C-I group (Figure 1B). Piglet mortality was 7.02%, in the control (CI) group, 5.19% in the TG-II group and 2.92% in the TG-III group. Beet pulp has high levels of crude fiber (CF) (including a large amount of the polymer l-arabinose), nitrogen-free leachate and crude protein (CP) (16). CF serves as the main fermentation substrate, particularly for microorganisms in the hindgut of piglets (17). The beneficial effect of sugar beet addition on BW and the FCR could be mainly attributed to alteration of the bacterial composition in the digestive tract, especially Lactobacillus sp., and the short-chain fatty-acid (SCFA) acetate, propionate and butyrate contents. Moreover, the intestinal morphology is also affected by dietary fiber, and increased intestinal villus height and the ratio of villus height to crypt depth influences intestinal nutrient absorption capacity in pigs, thus leading to increased BW.
Figure 1. (A) Piglet weight; (B) Feed conversion ratio (FCR) (a, bdifferent letters indicate differences among treatments (p < 0.05); (i) basal diet (C-I – control group), (ii) basal diet with extruded-fermented wheat bran added (TG-II), (iii) basal diet with dried sugar beet pulp (TG-III). The data are presented as the mean ± standard error (n = 100/group).
Variable findings are presented in the literature regarding the impact of dietary sugar beet on productivity in piglets. In contrast, Lizardo et al. reported that addition of sugar beet in the diet had a positive effect on growth performance in weaning pigs (18).
The blood parameters of 18-day-old piglets are given in Table 2. Significant differences in the immunoglobulins IgA and IgM concentrations between blood samples of different groups of piglets were not found. However, the lowest IgG concentration showed TG-III group samples and the treatment was not significant factor on all the tested immunoglobulins concentration. Blood serum characteristics are related to the animal's health and nutritional status and could reveal the possible interaction between bacteria and the host's metabolism. Wheat bran is rich in fiber, minerals, B vitamins and lot of bioactive and volatile compounds with health benefits (19). Chemical composition of sugar beet pulp consist mainly of cellulose, hemicellulose and pectin (20). It is known that non-digested fibers may serve as substrate for beneficial bacteria, altering the gut microbiota and increasing the proportion of lactic bacteria (21). The antibodies IgA, IgG, and IgM antibody play a crucial role in immune response of piglets to disease infection (22). Silva et al. reported, that the concentrations of blood IgA values were lower in piglets consuming cellulose (23). This can be explained by the lower incidence of diarrhea, considering that the production of immunoglobulins is stimulated by enteric infections of the gut mucosa (23).
A significantly lower (on average, by 2 times) TSH concentration in blood of the TG-III group was found, and the treatment was significant factor on TSH concentration in piglets' blood (p = 0.002). In animals, the blood concentrations of thyroid hormones are extremely variable (24). Thyroid hormones may be affected by other nutritional and metabolic facts like levels of zinc, selenium and/or iodine, their supplementation. Also in pigs, there seems to be relationship between energy metabolism and thyroid hormones (24). In TG-II group, higher concentration of ALT was found, in comparison with the C-I group (on average, 15.9% higher). The opposite tendencies were found for the AP concentration, and the highest concentration was detected in C-I group blood samples (on average, 15.8% higher than that in TG-II group). Although the ALT results were in line with the normal range for pigs (25, 26), ALT is a serum marker of liver damage, and its concentration could increase in response to cell membrane damage (27). The high concentrations of AST and ALP in serum during the first week after birth indicated the neonatal pig liver and gut were not fully formed and mature, exhibited by higher membrane permeability, whereas the levels of AST and AP in serum were reduced with the development of piglets and their organs. The highest CHOL concentration was found in TG-II group blood samples. The total serum cholesterol level is an index of lipometabolic status, which includes the free and bound forms of HDL. However, higher concentration of LDL-C in the blood of both treated groups was found (on average, 33.0% higher, in comparison with the C-I group). The TG concentrations were similar in blood samples of the C-I and TG-II groups and 32.1% lower, on average, in blood of the TG-III group, in comparison with C-I and TG-II group samples. Evidence suggests that dietary fiber (DF) binds to bile acids in the small intestine, which makes them less likely to enter the body, decreases intestinal lipid absorption and subsequently reduces the level of cholesterol in the blood (28). Other suggested mechanisms include synthesis by products of bacteria fermentation [production of short-chain fatty acids (SCFAs) such as acetate, butyrate, propionate] (29). SCFAs prevent from intestinal diseases, promote proliferation of intestinal tissue, the absorption of minerals and reduce the level of cholesterol in the blood (30). The hypocholesterolemic effects of DF vary depending on the type and level of fiber fed and it differentially modifies dietary energy absorption, animal's gender also alters the hypocholesterolemic responses to DF. The lowest T4 concentration was found in blood samples of the TG-II and TG-III groups. T4 plays a role in lipid metabolism. This suggests that the increased cholesterol concentrations in piglets might have been caused at least partially by decreased plasma concentrations of T4. The inclusion of dietary fiber (WB and sugar beet pulp are sources of different fiber compounds) can alter the gut microbiota and induce changes in plasma hormone and metabolite concentrations. It can be concluded from the results that all tested creep compound feed compositions were safe and did not impair animal metabolism, since all the values are in the normal range for pigs.
Fecal pH, dry matter, and color coordinates are shown in Table 3. In a comparison of fecal pH before the experiment, the mean pH was 6.78 in all tested groups. However, at the end of the experiment, feces of the TG-II group showed a significantly higher pH, in comparison with that of the C-I and TG-III groups (on average, 6.92% higher). However, piglets blood LDL showed a strong negative correlation with fecal pH (r = −0.722, p = 0.028). Significant differences in fecal dry matter at the beginning of the experiment were not found; but, at the end of the experiment, the highest dry matter content was in feces of the TG-II group (48.72%), and in feces of the C-I and TG-III groups, dry matter was lower by 55.8 and 12.1%, respectively. ANOVA indicated a significant interaction effect of treatment duration and creep compound feed composition on fecal dry matter in piglets (p = 0.009). Although, at the beginning of the experiment, TG-III group fecal samples showed the lowest textural hardness, at the end of the experiment, significant differences were not found. Correlations between fecal microbiological and physicochemical parameters and their significance are given in Supplementary material 2. A moderate negative correlation between pH and TCM was established (r = 0.501, p = 0.034) (Supplementary material 2). Positive moderate correlations between fecal textural hardness and LAB as well as Y/F were found (r = 0.475, p = 0.046; r = 0.567, p = 0.014, respectively); furthermore, a strong moderate correlation between fecal textural hardness and TCM was established (r = 0.710, p = 0.001). These correlations could be explained by the predominance of organic acid producers in TCM; for this reason, a higher TCM was related to a reduction in fecal pH. Also, increasing the LAB and Y/F counts in piglets' feces increased the fecal textural hardness. Usually, an increased LAB count in the digestive tract is associated with a decreased risk of diarrhea. In addition, due to the symbiotic relationship between Y/F and LAB in the same substrate, the avoidance of Y/F growth in feces is very complicated.
In a comparison of fecal color coordinates, at the beginning of the experiment, significant differences between the lightness (L*) coordinates were not found; however, at the end of the experiment, C-I and TG-II group samples showed the highest lightness (on average, 41.01 NBS). At the beginning of the experiment, significant differences between the redness and (or) greenness (a* and -a*, respectively) coordinates were found, whereas at the end of the experiment, the feces of both treated 'showed similar greenness values (on average, −1.49 NBS), and the C-I group samples showed significantly higher redness values (1.27 NBS). In a comparison of yellowness (b*), at the beginning of the experiment, significantly higher b* values were found in C-I group samples, and TG-II and TG-III group samples showed, on average, 25.8% lower b* values. In a comparison of b* values at the end of the experiment, different tendencies were established: TG-III group feces showed the lowest b* (7.85 NBS), and the b* values in C-I and TG-II group feces were, on average, 56.5 and 43.4% higher, respectively.
The consistency, color, odor and volume of the stools are characteristics of certain disease agents. Usually, colibacillosis is associated with alkaline pH (8 and higher), because the feces are rich in bicarbonate. A fecal pH 7 and lower could be associated with atrophic enteritis and malabsorptive diarrhea. Color characteristics are seldom used; however, they could be associated with blood residues in the feces of piglets (<5 days old) suffering from C. perfringens type-C enteritis.
Also, it should be pointed out, that there were no cases of diarrhea in any group at the end of the experiment. From this point of view, the differences in fecal parameters could be associated with dietary component variability, that lead to products of varying digestibility and variations in color and pH.
Volatile compounds (VC) of which the content was higher than 1% in at least one piglet fecal sample are shown in Figure 2. The whole VC profile is given in Supplementary material 3. Volatile compound profile of piglet feces. The predominant VC in piglet feces were p-cresol; indole and 1H-indole, 3-methyl. Butanoic acid and butanoic acid, 2-methyl- were found only in TG-III group feces at the end of the experiment. Although significant differences in the immunoglobulin concentration between blood samples of different groups of piglets were not found, blood IgA and IgM concentrations showed a strong positive correlation with the fecal volatile compound butanoic acid (r = 0.673, p = 0.47, and r = 0.670, p = 0.048, respectively). Pentanoic acid was detected in the feces of both treated groups (TG-II and TG-III) at the end of the experiment. In all the tested groups (at the beginning and end of the experiment), phenethyl alcohol; dodecane; 2-undecanone and n-nonadecanol-1 were found. Pentanoic acid, 4-methyl- and 3-eicosene, (E)- were detected only in the fecal VC profile of the C-I group at the beginning of the experiment, whereas phosphonic acid, (p-hydroxyphenyl)-; 5-hepten-2-one, 6-methyl- and cyclopentane, 1-pentyl-2-propyl- were detected only in the fecal VC profile of the TG-II group at the beginning of the experiment. Decane < n-> was found in the fecal VC profiles of all the groups at the beginning of the experiment. At the beginning of the experiment, 2-nonanone was found in the fecal VC profiles of all the tested groups; however, at the end of the experiment, 2-nonanone did not remain in C-I group feces, and in TG-II and TG-III group feces, its content was reduced by, on average, 15 and 21 times, respectively. Nonanoic acid was detected only in the TG-III group (at the beginning and end of the experiment). Cyclopentadecane was found only at the beginning of the experiment and only in the two treated groups. Butylated hydroxytoluene was found in lower quantities at the beginning of the experiment and was not detected in the C-I group; however, at the end of the experiment, this VC was found in samples from all tested piglets. At the beginning of the experiment, benzene, 1,3-bis(1,1-dimethylethyl)-; n-pentadecanol and 2-pentadecanone were found in all the tested groups; however, at the end of the experiment, their content was reduced and (or) they were not identified in the whole VC profile. The opposite tendencies were found for benzaldehyde: at the beginning of the experiment, this VC was detected only in C-I group feces but was found in the feces of all tested groups at the end of the experiment. Trisulfide < dimethyl-> was found at the beginning of the experiment in feces of the C-I and TG-II groups; however, at the end of the experiment, it was found in feces of the C-I and TG-III groups but not in the TG-II group. Phenol was found in feces of the C-I and TG-III groups at the beginning and end of the experiment. However, different tendencies were found for changes in the phenol content: at the end of the experiment, the quantity of this VC was increased in feces of the C-I group and reduced in that of the TG-III group.
Figure 2. Volatile compound (VC) profile of piglet feces [(i) basal diet (C-I – control group), (ii) basal diet with extruded-fermented wheat bran added (TG-II), (iii) basal diet with dried sugar beet pulp (TG-III)].
Data on the fecal VC profile of new-born piglets are scarce. Pentanoic acid, 3-methylbutanoic acid, 4-methylphenol, 3-methylindole and indole have been reported in growing pig feces and in manure, slurry, air and dust taken from piggeries (31). Changes in the parameters of new-born piglet feces, including the VC profile, could also be related to their coprophagy, which also helps to prevent anemia in piglets (32).
Microbiological parameters of the piglets' fecal samples are given in Table 4. In a comparison of the total counts of enterobacteria (TCE) at the beginning of the experiment, the lowest TCE was found in feces of the C-I group; however, at the end of the experiment, the lowest TCE was established in feces of the TG-II group. In feces of the C-I and TG-III groups, TCE was on average 7.2% higher. Usually, E. coli lives in symbiosis with its host (33); however, virulent strains can cause diarrhea (33). It was reported that high levels of contamination with enteric bacteria could be a risk factor for the vectorization of these strains (34). Also, T4 concentration in piglets' blood showed very strong positive correlations with TCE and Y/F counts in feces (r = 0.991, p = 0.0001 and r = 0.929, p = 0.0001, respectively). In a comparison of the LAB count in piglet feces, significant differences were not found at the beginning of the experiment; however, at the end of the experiment, significantly higher LAB counts were found in both treated groups, in comparison with the C-I group (on average, 22.4 and 10.8% higher in TG-II and TG-III, respectively). ANOVA indicated that the interaction of the duration of treatment and differences in the diet is a significant factor in the LAB count in piglets' feces (p = 0.014). An increased population of LAB strains along with the Bacteroides genus might contribute to improvements in digestive tract health. More intensive growth of beneficial microorganisms could lead to suppression of pathogen growth (35). In this study, a correlation between LAB and TCE counts in piglets' feces was not found; however, between TCE and TCM, a moderate positive correlation was established (r = 0.723, p = 0.001). At the beginning of the experiment, a higher total microorganism count (TCM) in the feces of both treated groups was found; however, after 21 days, TCM was the lowest in feces of the TG-III group (on average, 7.8% lower). Piglets blood LDL showed a strong positive correlation with TCM in piglet feces (r = 0.750, p = 0.020). Significant differences in Enterococcus faecalis counts at the end of the experiment in piglets' feces were not found. However, strong positive correlations were established between the piglets' blood TG concentration and Enterococcus faecalis as well as Y/F in feces (r = 0.746, p = 0.021 and r = 0.796, p = 0.037, respectively). Enterococcus faecalis is described as a gut commensal and usually does not cause clinical issues (36). However, this strain can form biofilms and can be 1,000-fold more resistant to antibiotics than planktonic cells (37).
The differences between yeast/fungi (Y/F) counts were established, and, at the beginning and end of the experiment, the highest count of Y/F was found in piglet feces of the TG-II group (on average, 6.05 log10 CFU/g). Also, a strong positive correlation between the TSH concentration in blood and the Y/M count in feces was found (r = 0.742, p = 0.022). In addition, piglets blood ALT showed a very strong positive correlation with TCE in the feces of piglets (r = 0.976, p = 0.0001), and both blood ALT and ALP showed very strong positive correlations with the Y/F count in fecal samples (r = 0.936, p = 0.0001 and r = 0.831, p = 0.006, respectively). The CHOL concentration in blood samples showed a strong positive correlation with the TCE and Y/F counts in piglet feces (r = 0.769, p = 0.015 and r = 0.730, p = 0.026, respectively). Synergistic interactions between LAB and yeast have been reported (38); however, most molds are suppressed by LAB action (39). Fungi could produce allergenic spores as well as fungal metabolites (mycotoxins), which pose essential health hazards. For this reason, it is very important to avoid their high numbers in farm environments and reduce fungal counts in the digestive tracts of farm animals.
Also, in our study, some correlations between the VC in feces and microbiological parameters were found. Negative moderate correlations of butyric acid <2-methyl-> and pentanoic acid, 4-methyl- with TCE were established (r = −0.476, p = 0.046 and r = −0.479, p = 0.044, respectively) (Supplementary material 2). Trisulfide < dimethyl-> showed a moderate negative correlation with fecal pH (r = −0.527, p = 0.025). By contrast, p-cresol and butylated hydroxytoluene showed moderate positive correlations with fecal pH (r = 0.560, p = 0.016 and r = 0.547, p = 0.019, respectively). 3-Eicosene, (E)- showed a moderate negative correlation with TCE in piglet feces (r = −0.477, p = 0.046). n-Nonadecanol-1 showed a positive moderate correlation with the LAB count in piglet feces (r = 0.534, p = 0.022).
Finally, broader research should be performed to indicate individual compounds that could be related to specific health conditions; however, the results obtained are very promising, because parameters with which correlations were established are well known, and the influence of the latter on piglet health could be interpreted.
Microbial profiles in piglet feces before the experiment are presented in Figure 3. The predominant genus was Escherichia, for which the prevalence among all detected bacteria was 28.7%. Other prevalent genera included Bacteroides (16.1%), Fusobacterium (15.1%), Clostridium (7.1%) and Prevotella (5.8%). The less abundant genera were Lactobacillus, Lachnoclostridium, Enterococcus, Alloprevotella and Peptostreptococcus. Although microbial profiles in piglets can differ depending on the circumstances, the abovementioned genera are frequently found as the normal microbiota in the early life stages of piglets (40, 41). Although Escherichia are known as pathogens which can cause diseases in new-born piglets, their high prevalence in pigs does not indicate infection, as E. coli, which is the most prevalent species among Escherichia is highly variable and usually has no enterotoxigenic properties. This study also demonstrates that Escherichia in new-born piglets usually is not associated with disease but is part of the transient microbiota in pigs at a very young age.
Bacterial profiles in pig feces of all the groups at the end of the experiment are presented in Figure 4, 5. At the end of the experiment Firmicutes was the most predominant phylum with the prevalence of more than 60% in all groups of piglets (Figure 4). The second most prevalent phylum was Bacteroidetes which amount varied from 17.6% in TG-II to 30.5% in TH-III. Proteobacteria and other phyla amounted a small part of microbiome. As phyla contain different bacterial genera and species it is complicated to determine the influence of differences in microbial profiles at phylum level between the groups. Figure 5 contains the data about bacterial genera prevalence in different groups of piglets. More detail prevalence of bacterial diversity are presented in Supplementary materials 1–4. There is an evidence that intestinal microbiota impact host's biological activities, including digestion of indigestible feed components, energy harvest, and immunity (42). In this study the highest indices of weight gain were observed in TG-III and the lowest in C-I. The most prevalent genera in TC-III were Lactobacillus, Ruminococcus, and Barnesiella. There are many studies which demonstrated that different species of Lactobacillus were associated with the growth improvement of piglets and promoting the development of small intestinal villi (43). Reduced numbers of Ruminococcus can be associated with diarrhea in suckling piglets therefore, this genus also may have association with animal body weight. The resent study also detected significant relation between Barnesiella and Lactobacillus to the growth performance in Yorkshire pigs (44). Lactobacillus also induces porcine host defense peptides and therefore improving immunity (45).
Figure 4. Bacterial composition differences (%) between the animal groups at the end of the experiment [The most prevalent genera (prevalence of at least 1% out of the total amount of bacteria) are presented. (i) Basal diet (C-I – control group), (ii) basal diet with extruded-fermented wheat bran added (TG-II), (iii) basal diet with dried sugar beet pulp added (TG-III)] (* insignificant results between TG-II and TG-III, **insignificant results between C-I and TG-II, ***insignificant results between C-I and TG-III).
At the end of the experiment, a significant reduction of Escherichia in the feces of pigs was detected, compared with that of new-born piglets. This may be explained by microbiota composition changes during different growth periods as Escherichia usually presents in high numbers only at early age and normally decreases after weaning (46). The most prevalent genus in all groups was Lactobacillus, although the number of species of this genus differed significantly between C-I and the experimental groups (p < 0.05). The amount of Lactobacillus was higher in the experimental animal groups, whereas there were no statistical differences between groups TG-II and TG-III (p = 0.14). The proportions of bacteria of other genera depended on the group. In the C-I group, the most prevalent genera were Rikenella, Clostridium, Lachnosclostridium, and Parabacteroides, with a total prevalence of 25.7%. In TG-II, the most prevalent were Oscillibacter, Bacteroides, Clostridium, and Lachnoclostridium, with a total prevalence of 36.4%, and in the TG-III group, Prevotella, Ruminococcus, Barnesiella, and Oscillibacter, with a total prevalence of 28.8%. The data on these and other bacterial genera detected in separate groups of pigs are presented in Figure 5. The rest of the genera included bacteria often mentioned as the core microbiota in vertebrate animals. Although to date there are no clear data on the role of all microorganisms detected in this study, some knowledge about certain genera exists. For example, Prevotella is known to be associated with improvements in the average daily gain and feed conversion ratio in weaned-off pigs (47), while Ruminococcus spp. are known as preventive bacteria that reduce the possibility of postweaning diarrhea by producing butyrate, which regulates jejunal homeostasis (48). The amount of Prevotella in this study was much higher in the TG-III group, compared with the C-I and TG-II (p < 0.05) groups, and it is probably associated with the high carbohydrate content of sugar beet pulp. Piglet feces of this group also contained higher numbers of Ruminococcus than the C-I and TG-II groups (p < 0.05). Oscillibacter, which was the second most prevalent genus after Lactobacillus spp. in TG-II, belongs to the order Clostridiales and was previously isolated from cows, humans and clams. Regarding a possible biological function of this genus in the gastrointestinal tract, Oscillibacter was reported as a probiotic because of the production of anti-inflammatory metabolites (49). Recent results demonstrated that Oscillibacter in pigs was associated with feed efficiency (50). Pigs of the TG-II group also had large numbers of Bacteroides and Clostridium, which are members of the core microbiome in the porcine gut. Pigs in the C-I group had a high prevalence of Rikenella—a bacterial genus that is poorly described in association with pigs. It is known as a producer of short-chain fatty acids (51), which are the main energy source of colonocytes and therefore have a positive effect on gastrointestinal health. Other prevalent genera in this group were Parabacteroides, Clostridium, and Lachnoclostridium, i.e., the normal core microbiota of the porcine gastrointestinal tract. Taking into account the microbial profiles, an increase in beneficial microbiota is visible, particularly of Lactobacillus in both experimental groups, whereas different supplementation schemes resulted in different microbial compositions, although both of them had a positive influence: addition of sugar beet pulp resulted in higher numbers of Prevotella and Ruminococcus, while addition of extruded and fermented WB resulted in increased numbers of Oscillibacter, Bacteroides, and Clostridium.
All the tested CCF formulations were safe and did not impair animal metabolism. In addition, the used treatment reduced FCR, increased viable LAB counts in piglet feces, and resulted in better desirable microbial compositions in pigs' gut. Finally, the data suggest that appropriate feed composition can be used for bacterial profile regulation with the aim of supporting animal health and feed efficiency. Also, significant correlations between individual fecal VC and microbiological parameters, and between VC and pH found in this study shed new light on the search for possible chemical markers, as the latter could be used for non-invasive evaluation of piglet health status.
The original contributions presented in the study are included in the article/Supplementary material, further inquiries can be directed to the corresponding author.
All animal procedures were conducted according to the EU Directive of the European Parliament and of Council from September 22, 2010 (13) on the protection of animals used for scientific purposes and Requirements for the Keeping, Maintenance, and Use of Animals Intended for Science and Education Purposes, approved by the order of the Lithuanian Director of the State Food and Veterinary Service (14). The research was carried out in accordance with both the Republic of Lithuania Act (November 6, 1997) regulating animal care and maintenance and its subsequent legal amendment (Act 8-500) (15).
The LSMU group conceived the study, participated in its design and coordination, participated in interpretation of the findings, drafted, and reviewed the manuscript. The KTU group participated in the animal studies and interpretation of the findings in the manuscript. The BIOR group developed the methods for feed and biological samples physico-chemical analysis. All authors read and approved the final manuscript.
The authors gratefully acknowledge the COST Action CA18101 SOURDOugh biotechnology network toward novel, healthier, and sustainable food and bIoproCesseS, the EUREKA Network Project E!13309 SUSFEETECH (Nr. 01.2.2-MITA-K-702-05-0001), and EIP project approved by the Chancellor of the Ministry of Agriculture of the Republic of Lithuania in 27-11-2019 protocol No. 4D178(1.8 E).
The authors declare that the research was conducted in the absence of any commercial or financial relationships that could be construed as a potential conflict of interest.
All claims expressed in this article are solely those of the authors and do not necessarily represent those of their affiliated organizations, or those of the publisher, the editors and the reviewers. Any product that may be evaluated in this article, or claim that may be made by its manufacturer, is not guaranteed or endorsed by the publisher.
The Supplementary Material for this article can be found online at: https://www.frontiersin.org/articles/10.3389/fvets.2022.971783/full#supplementary-material
Supplementary material 1. Physicochemical, antimicrobial, and antifungal characteristics of the Wex130/screwspeed25Lpa.
Supplementary material 2. Correlations between fecal microbiological and physicochemical parameters.
Supplementary material 3. Correlations of fecal microbiological and physicochemical parameters with volatile compounds and their significance.
1. Zokaityte E, Lele V, Starkute V, Zavistanaviciute P, Klupsaite D, Bartkevics V, et al. The influence of combined extrusion and fermentation processes on the chemical and biosafety parameters of wheat bran. LWT. (2021) 146:111498. doi: 10.1016/j.lwt.2021.111498
2. European Flour Millers. Available online at: http://www.flourmillers.eu/ (accessed February 26, 2022).
3. Vadopalas L, Ruzauskas M, Lele V, Starkute V, Zavistanaviciute P, Zokaityte E, et al. Combination of antimicrobial starters for feed fermentation: influence on piglet feces microbiota and health and growth performance, including mycotoxin biotransformation in vivo. Front Vet Sci. (2020) 7:528990. doi: 10.3389/fvets.2020.528990
4. Yu C, Zhang S, Yang Q, Peng Q, Zhu J, Zeng X, et al. Effect of high fibre diets formulated with different fibrous ingredients on performance, nutrient digestibility and faecal microbiota of weaned piglets. Arch Anim Nutr. (2016) 70:263–77. doi: 10.1080/1745039X.2016.1183364
5. Schiavon S, Tagliapietra F, Bailoni L, Bortolozzo A. Effects of sugar beet pulp on growth and health status of weaned piglets. Ital J Anim Sci. (2004) 3:337–51. doi: 10.4081/ijas.2004.337
6. Yan CL, Kim HS, Hong JS, Lee JH, Han YG, Jin YH, et al. Effect of dietary sugar beet pulp supplementation on growth performance, nutrient digestibility, fecal microflora, blood profiles and diarrhea incidence in weaning pigs. J Anim Sci Technol. (2017) 59:18. doi: 10.1186/s40781-017-0142-8
7. Williams BA, Verstegen MW, Tamminga S. Fermentation in the large intestine of single-stomached animals and its relationship to animal health. Nutr Res Rev. (2001) 14:207–28. doi: 10.1079/NRR200127
8. Nowland TL, Kirkwood RN, Pluske JR. Review: can early-life establishment of the piglet intestinal microbiota influence production outcomes? Animal. (2022) 16:100368. doi: 10.1016/j.animal.2021.100368
9. National Research Council. Nutrient Requirements of Swine. 11th revised ed. Washington, DC: National Academic Press (2012).
10. Association of Official Analytical Chemists. Official Methods of Analysis. Arlington, VA: AOAC (1990).
11. Vadopalas L, Zokaityte E, Zavistanaviciute P, Gruzauskas R, Starkute V, Mockus E, et al. Supplement based on fermented milk permeate for feeding newborn calves: influence on blood, growth performance, and faecal parameters, including microbiota, volatile compounds, and fatty and organic acid profiles. Animals. (2021) 11:2544. doi: 10.3390/ani11092544
12. Zavistanaviciute P, Lele V, Antanaitis R, Televičius M, Ruzauskas M, Zebeli Q, et al. Separate and synergic effects of lactobacillus uvarum luhss245 and arabinogalactan on the in vitro antimicrobial properties as well as on the fecal and metabolic profile of newborn calves. Animals. (2020) 10:593. doi: 10.3390/ani10040593
13. Directive 2010/63/EU of the European Parliament and of the Council of 22 September 2010 on the protection of animals used for scientific PurposesText with EEA relevance. Eur Parliam Counc. (2010) 47:82–128.
14. Lithuanian Director of the State Food and Veterinary Service. B1-866 Del Mokslo Ir Mokymo Tikslais Naudojamu Gyvunu Laikymo, PrieŽiuros Ir Naudojimo Reikalavimu Patvirtinimo. Available online at: https://e-seimas.lrs.lt/portal/legalAct/lt/TAD/TAIS.437081?positionInSearchResults=0&searchModelUUID=64a1f51f-6356-4b60-a21e-3a67ac3b1107 (accessed February 9, 2022).
15. Law of the Republic of Lithuania: Amending the Law on the Care, Keeping and Use of Animals. Available online at: https://e-seimas.lrs.lt/portal/legalAct/lt/TAD/TAIS.434660 (accessed February 3, 2022).
16. Mohsen MK, Ali MF, Gaafar HM, Al-Sakka TS, Aboelenin SM, Soliman MM, et al. Impact of dry sugar beet pulp on milk production, digestibility traits, and blood constituents of dairy Holstein cows. Animals. (2021) 11:3496. doi: 10.3390/ani11123496
17. Lindberg JE. Fiber effects in nutrition and gut health in pigs. J Anim SciBiotechnol. (2014) 5:15. doi: 10.1186/2049-1891-5-15
18. Lizardo R, Peiniau J, Aumaitre A. Inclusion of sugar-beet pulp and change of protein source in the diet of the weaned piglet and their effects on digestive performance and enzymatic activities. Anim Feed Sci Technol. (1997) 66:1–14. doi: 10.1016/S0377-8401(96)01144-3
19. He W, Gao Y, Guo Z, Yang Z, Wang X, Liu H, et al. Effects of fermented wheat bran and yeast culture on growth performance, immunity, and intestinal microflora in growing-finishing pigs. J Anim Sci. (2021) 99, skab308. doi: 10.1093/jas/skab308
20. Arce ON, Alagón G, Ródenas L, Martínez-Paredes E, Moya VJ, Pascual JJ, et al. Efecto de La Época de Cosecha En Las Características Químicas de La Pulpa de Remolacha Azucarera (Beta Vulgaris) Granulada. Nota Técnica. Cuban J Agric Sci. (2019) 53:51–7.
21. Batson KL, Calderón HI, Tokach MD, Woodworth JC, Goodband RD, Dritz SS, et al. Effects of feeding diets containing low crude protein and coarse wheat bran as alternatives to zinc oxide in nursery pig diets. J AnimSci. (2021) 99:skab090. doi: 10.1093/jas/skab090
22. Che D, Adams S, Wei C, Gui-Xin Q, Atiba EM, Hailong J. Effects of astragalus membranaceus fiber on growth performance, nutrient digestibility, microbial composition, VFA production, gut PH, and immunity of weaned pigs. MicrobiologyOpen. (2019) 8:e00712. doi: 10.1002/mbo3.712
23. Silva RO, Lopes AD, Faria RM. Eletroforese de Proteínas Séricas: Interpretação e Correlação Clínica, Vol. 18 (2008). p. 116–22. Available online at: https://pesquisa.bvsalud.org/portal/resource/pt/lil-510393?lang=en
24. Lossow K, Renko K, Schwarz M, Schomburg L, Schwerdtle T, Kipp AP. The nutritional supply of iodine and selenium affects thyroid hormone axis related endpoints in mice. Nutrients. (2021) 13:3773. doi: 10.3390/nu13113773
25. Caprarulo V, Hejna M, Giromini C, Liu Y, Dell'Anno M, Sotira S, et al. Evaluation of dietary administration of chestnut and quebracho tannins on growth, serum metabolites and fecal parameters of weaned piglets. Anim Open Access J MDPI. (2020) 10:E1945. doi: 10.3390/ani10111945
26. IZSLER Istituto Zooprofilattico Sperimentale “Bruno Ubertini” - Test Analitici Available online at: https://archive.izsler.it/pls/izs_bs/v3_s2ew_consultazione.mostra_pagina?id_pagina=1494 (accessed February 26, 2022).
27. Chen H, Wang C, Wang Y, Chen Y, Wan M, Zhu J, et al. Effects of soft pellet creep feed on pre-weaning and post-weaning performance and intestinal development in piglets. Anim Biosci. (2021) 34:714. doi: 10.5713/ajas.20.0034
28. Cho JH, Lee SI, Kim IH. Effects of different levels of fibre and benzoic acid on growth performance, nutrient digestibility, reduction of noxious gases, serum metabolites and meat quality in finishing pigs. J Appl Anim Res. (2015) 43:336–44. doi: 10.1080/09712119.2014.978772
29. Brown L, Rosner B, Willett WW, Sacks FM. Cholesterol-lowering effects of dietary fiber: a meta-analysis. Am J Clin Nutr. (1999) 69:30–42. doi: 10.1093/ajcn/69.1.30
30. Tian M, Chen J, Liu J, Chen F, Guan W, Zhang S. Dietary fiber and microbiota interaction regulates sow metabolism and reproductive performance. Anim Nutr Zhongguo Xu Mu Shou Yi Xue Hui. (2020) 6:397–403. doi: 10.1016/j.aninu.2020.10.001
31. Ni J.-Q., Robarge WP, Xiao C, Heber AJ. Volatile organic compounds at swine facilities: a critical review. Chemosphere. (2012) 89:769–88. doi: 10.1016/j.chemosphere.2012.04.061
32. Gleed PT, Sansom BF. Ingestion of iron in Sow's Faeces by piglets reared in farrowing crates with slotted floors. Br J Nutr. (1982) 47:113–7. doi: 10.1079/BJN19820016
33. Shtawa A. E. coli as a gauge of bacteriological quality of water: an overview. Glob J Res Anal. (2016) 5:144–7. doi: 10.4081/mr.2013.e2
34. Cervelin V, Fongaro G, Pastore JB, Engel F, Reimers MA, Viancelli A. Enterobacteria associated with houseflies (Musca domestica) as an infection risk indicator in swine production farms. Acta Trop. (2018) 185:13–7. doi: 10.1016/j.actatropica.2018.04.024
35. Metzler-Zebeli BU, Trevisi P, Prates JAM, Tanghe S, Bosi P, Canibe N, et al. Assessing the effect of dietary inulin supplementation on gastrointestinal fermentation, digestibility and growth in pigs. Anim Feed Sci Technol. (2017) 233:120. doi: 10.1016/j.anifeedsci.2017.05.010
36. Bolocan AS, Upadrasta A, de Almeida Bettio PH, Clooney AG, Draper LA, Ross RP, et al. Evaluation of phage therapy in the context of Enterococcus faecalis and its associated diseases. Viruses. (2019) 11:366. doi: 10.3390/v11040366
37. Kim M-A, Rosa V, Min K-S. Characterization of Enterococcus faecalis in different culture conditions. Sci Rep. (2020) 10:21867. doi: 10.1038/s41598-020-78998-5
38. Adesulu-Dahunsi AT, Dahunsi SO, Olayanju A. Synergistic microbial interactions between lactic acid bacteria and yeasts during production of Nigerian indigenous fermented foods and beverages. Food Control. (2020) 110:106963. doi: 10.1016/j.foodcont.2019.106963
39. Matevosyan LA, Bazukyan IL, Trchounian AH. Antifungal activity of lactic acid bacteria isolates and their associations: the effects of Ca and Mg divalent cations. Curr Microbiol. (2020) 77:959–966. doi: 10.1007/s00284-020-01897-5
40. Yang J, Wang C, Huang K, Zhang M, Wang J, Pan X. Compound lactobacillus sp. administration ameliorates stress and body growth through gut microbiota optimization on weaning piglets. Appl Microbiol Biotechnol. (2020) 104:6749–65. doi: 10.1007/s00253-020-10727-4
41. Gryaznova MV, Dvoretskaya YD, Syromyatnikov MY, Shabunin SV, Parshin PA, Mikhaylov EV, et al. Changes in the microbiome profile in different parts of the intestine in piglets with diarrhea. Animals. (2022) 12:320. doi: 10.3390/ani12030320
42. Oh JK, Chae JP, Pajarillo EAB, Kim SH, Kwak M-J, Eun J-S, et al. Association between the body weight of growing pigs and the functional capacity of their gut microbiota. Anim Sci J Nihon Chikusan Gakkaiho. (2020) 91:e13418. doi: 10.1111/asj.13418
43. Wang T, Teng K, Liu Y, Shi W, Zhang J, Dong E, et al. Lactobacillus plantarum PFM 105 promotes intestinal development through modulation of gut microbiota in weaning piglets. Front Microbiol. (2019) 10:90. doi: 10.3389/fmicb.2019.00090
44. Li X.-J., Wang M, Xue Y, Duan D, Li C, et al. Identification of microflora related to growth performance in pigs based on 16S RRNA sequence analyses. AMB Express. (2020) 10:192. doi: 10.1186/s13568-020-01130-3
45. Wang J, Zhang W, Wang S, Liu H, Zhang D, Wang Y, et al. Swine-derived probiotic lactobacillus plantarum modulates porcine intestinal endogenous host defense peptide synthesis through TLR2/MAPK/AP-1 signaling pathway. Front Immunol. (2019) 10:2691. doi: 10.3389/fimmu.2019.02691
46. Knecht D, Cholewińska P, Jankowska-Makosa A, Czyz K. Development of swine's digestive tract microbiota and its relation to production indices—a review. Animals. (2020) 10:527. doi: 10.3390/ani10030527
47. Karasova D, Crhanova M, Babak V, Jerabek M, Brzobohaty L, Matesova Z, et al. Development of piglet gut microbiota at the time of weaning influences development of postweaning diarrhea - a field study. Res Vet Sci. (2021) 135:59–65. doi: 10.1016/j.rvsc.2020.12.022
48. Zhong X, Zhang Z, Wang S, Cao L, Zhou L, Sun A, et al. Microbial-driven butyrate regulates Jejunal homeostasis in piglets during the weaning stage. Front Microbiol. (2019) 9:3335. doi: 10.3389/fmicb.2018.03335
49. Li J, Sung CYJ, Lee N, Ni Y, Pihlajamäki J, Panagiotou G, et al. Probiotics modulated gut microbiota suppresses hepatocellular carcinoma growth in mice. Proc Natl Acad Sci USA. (2016) 113:E1306–15. doi: 10.1073/pnas.1518189113
50. Bergamaschi M, Tiezzi F, Howard J, Huang YJ, Gray KA, Schillebeeckx C, et al. Gut microbiome composition differences among breeds impact feed efficiency in swine. Microbiome. (2020) 8:110. doi: 10.1186/s40168-020-00888-9
Keywords: creep compound feed, piglets, wheat bran, sugar beet pulp, microbial profile, health, growth performance
Citation: Badaras S, Ruzauskas M, Gruzauskas R, Zokaityte E, Starkute V, Klupsaite D, Mockus E, Klementaviciute J, Vadopalas L, Zokaityte G, Dauksiene A, Bartkevics V and Bartkiene E (2022) Different creep compound feed formulations for new born piglets: influence on growth performance and health parameters. Front. Vet. Sci. 9:971783. doi: 10.3389/fvets.2022.971783
Received: 17 June 2022; Accepted: 11 August 2022;
Published: 29 August 2022.
Edited by:
Haoyu Liu, Yangzhou University, ChinaReviewed by:
Jiajun Yang, China Agricultural University, ChinaCopyright © 2022 Badaras, Ruzauskas, Gruzauskas, Zokaityte, Starkute, Klupsaite, Mockus, Klementaviciute, Vadopalas, Zokaityte, Dauksiene, Bartkevics and Bartkiene. This is an open-access article distributed under the terms of the Creative Commons Attribution License (CC BY). The use, distribution or reproduction in other forums is permitted, provided the original author(s) and the copyright owner(s) are credited and that the original publication in this journal is cited, in accordance with accepted academic practice. No use, distribution or reproduction is permitted which does not comply with these terms.
*Correspondence: Elena Bartkiene, ZWxlbmEuYmFydGtpZW5lQGxzbXVuaS5sdA==
Disclaimer: All claims expressed in this article are solely those of the authors and do not necessarily represent those of their affiliated organizations, or those of the publisher, the editors and the reviewers. Any product that may be evaluated in this article or claim that may be made by its manufacturer is not guaranteed or endorsed by the publisher.
Research integrity at Frontiers
Learn more about the work of our research integrity team to safeguard the quality of each article we publish.