- 1College of Animal Science and Technology, Nanjing Agricultural University, Nanjing, China
- 2Wuxi Sanzhi Bio-Tech Co., Ltd., Wuxi, China
- 3College of Food Science and Technology, Jiangnan University, Wuxi, China
Weaning stress decreases the growth performance of piglets and is one of the main concerns of pig industries. Traditional Chinese herbal medicines have been used to reduce the adverse effects of weaning stress as both nutritional supplements and antibiotic substitutes. This study aimed to evaluate the effects of a Chinese herbal mixture (Kangtaile, which contained Paeonia lactiflora, licorice, dandelion, and tea polyphenols) on the growth performances, immune response, antioxidant capacity, and intestinal microbiota of weaned pigs. A total of 400 weaned pigs [Duroc × (Landrace × Yorkshire)] were randomly allocated into one of four treatments: the CON group, fed with basic diet; the HM1 group, fed with basal diet supplemented with 0.5 g herbal mixture/kg diet; the HM2 group, fed with basal diet supplemented with 1.0 g herbal mixture/kg diet; or the HM3 group, fed with basal diet supplemented with 1.5 g herbal mixture/kg diet. The results revealed that dietary supplementation with the herbal mixture for 28 days improved average daily gain and feed conversion ratio, while decreased the diarrhea rate of weaned pigs. Moreover, dietary supple-mentation with the herbal mixture improved the antioxidant capacity through increasing the activity of catalase (CAT) and the total antioxidant capacity (T-AOC) level, while decreasing the concentration of malondialdehyde (MDA) in the serum. Pigs supplemented with herbal mixture presented an increased serum immunoglobulin (Ig)M level on day 14 compared with control pigs. The herbal mixture altered the composition of intestinal microbiota by influencing the relative abundances of Firmicutes and Bacteroidetes at the phylum level. The relative abundances of the Firmicutes and Bacteroidetes were significantly related to the body weight gain of pigs. In conclusion, supplementation of herbal mixture to the diet improved growth performance, immunity, and antioxidant capacity and modified the composition of intestinal microbiota in weaning pigs. This study provided new insights into the nutritional regulation effects of the herbal mixtures on weaned pigs.
Introduction
Weaning stress often leads to diarrhea, respiratory diseases, and loss of weight gain in weaned pigs (1). Antibiotic additives were thus used to reduce weaning stress in the feed of weaned pigs (2). However, the abuse of antibiotic drugs led to antibiotic residues in animal derived foods, while antibiotic-resistant bacteria were transmitted to humans through the food chain, which led to serious health hazards for humans (3). Therefore, with the ban on antibiotics as feed additives, it is urgent to identify the safe alternatives to antibiotics to enhance the protection of both animal derived foods and human health.
In livestock production, Chinese herbal medicines have been considered as safe feed additives to substitute antibiotics in animal feed (4, 5). Because Chinese herbal medicines are natural products, they contain a variety of beneficial ingredients that maintain the overall health of animals and prevent diseases in animals (6). A previous study found that Paeoniflorin is an effective active ingredient in Paeoniae Radix Alba (PRA) (dried root of Paeonia lactiflora Pall) and had anti-inflammatory and immune regulation functions (7). Dietary supplementation with peony pollen improved the growth performance, digestive capacity, catalase, and total antioxidant capacity and reduced malondialdehyde level in carps (8). Licorice has anti-inflammatory and antiviral properties, and its main effective active ingredient is glycyrrhizin (9). Previous studies have revealed that the supplementation of seaweed and licorice increased the concentration of IgA and decreased the mRNA expression level of tumor necrosis factor-α in the saliva of pigs (10). The main active ingredients of dandelion are sesquiterpene lactone and phenylpropanoid polysaccharide, which have anti-inflammatory and immune regulating effects (11). A combination of 5 g/kg garlic and 50 g/kg dandelion improved fattening pig growth and carcass quality (12). Tea polyphenols (TP) are the main active ingredients in tea and have the functions of antioxidants, obesity reduction, and disease risk reduction (13). In addition, a previous study indicated that dietary supplementation of 0.2% TP increased the relative abundance of Lactobacillus, also decreased the abundance of Bacteroidacease and the total bacteria count in pigs (14).
However, previous studies have not mentioned the use of combinations of herbs to reduce the negative effects of weaning stress in pigs. Furthermore, the mechanism of herbal combination formulation to improve the health and physiological condition of pigs has not been clarified. In general, multiple active ingredients in herbal mixture composed of several herbs have synergistic effects, and their biological efficiency is greater than that of a single herbal extract (15, 16). Furthermore, the factors influencing the production performance of weaning pigs are complex, so the effect of a single herbal medicine is often limited in the treatment of these complicated problems caused by weaning stress (17). Previous studies have revealed that the synergistic effects of herbal mixture are achieved by applying a pair of herbs with similar therapeutic functions (18). For example, the coexisting constituents in a herbal mixture promoted the intestinal absorption of active constituents by improving solubility and increasing the membrane permeability of enterocytes (19). The synergistic effect of herbal mixture also indicated that the combined preparation reduces the toxicity of one of the herbs through the selective metabolism of the main active components, which achieved the therapeutic effect through metabolic detoxification (20). Shaoyao-Gancao Decoction (SGD), a classical formulated Chinese herbal medicine, consists of Paeoniae Radix Alba and licorice (21), which has pharmacological functions of antioxidation and immune regulation (22, 23). Similarly, the main ingredients of 24 flavor tea are dandelion and licorice, which have protective effects on immune rejection during organ transplantation (24). However, the combined effects of these herbal mixture (R. paeoniae alba, Licorice, Dandelion and Tea polyphenols) have not been investigated in weaned pigs. Therefore, this study aimed to investigate the effects of herbal mixture on the growth performance, immunity, antioxidant capacity, and intestinal microorganisms of weaned pigs. The present study provides experimental data for supplementation of the herbal mixture as an alternative to reduce post-weaning stress in pigs.
Materials and methods
Preparation of the herbal mixture (Kangtaile)
A type of herbal mixture called Kangtaile was prepared by Wuxi Sanzhi Biotechnology Co., Ltd (Wuxi, China). The herbal mixture is a mixed combination of five commonly used herbal medicines with different proportions including: Paeoniae Radix Alba (42%), licorice (28%), dandelion (28%), and tea polyphenols (2%). The ratio of Paeoniae Radix Alba and licorice was revised on the basis of the traditional Chinese medicine prescription “Shaoyao Gancao Decoction” (25). In addition, the combination of licorice and dandelion has the functions of antioxidant, anti-inflammatory and antibacterial (26). They exist simultaneously as the main components in tea drinks (27). The herbs are dried, broken and crushed into powder, as well as screened through an 800-mesh sieve before use, and then mixed by proportion to obtain herbal mixture (dark brown powder), which was stored in a sealed container at room temperature before use. Total polysaccharides, total saponins, flavonoids, and polyphenols are the main antioxidant compounds (28). The compositions of total polysaccharide and total flavonoids in herbal mixtures mainly come from Paeoniae Radix Alba, licorice and dandelion. The compositions of total saponins in herbal mixtures mainly come from licorice. The compositions of total polyphenols in herbal mixtures mainly come from Paeoniae Radix Alba, dandelion, and tea polyphenols. The principal active antioxidant components (total polysaccharide, and total saponins) of herbal mixture were determined by ultra-performance liquid chromatography (UPLC) method based on a previous study (29). In brief, the UPLC analysis was performed using a UPLC-Xevo TQ-S mass spectrometer (Waters Xevo TQ MS, Waters Corp., Milford, MA, USA). The following UPLC condition was used: the chromatographic column is a Kinetex C18 column (2.1 × 100 mm, 1.7 μm); Mobile phase A was acetonitrile and mobile phase B was 0.1% formic acid and injection volume 1 μL. A flow rate of 0.15 mL/min; column temperature: 45°C; total running time 12 min. Total polyphenols content was determined according to the Folin-Ciocalteu chromatometry method (30). Absorbance of the sample was measured with the microplate reader (Tecan, Austria GmbH, Grödig, Austria) at 760 nm. The total phenolic content was expressed as milligram of gallic acid equivalent per gram of herbal mixture. The determination of total flavonoids was performed by NaNO2-Al(NO3)3-NaOH colorimetric method with rutin as a standard for estimating the content of total flavonoids (31). After determination, the concentration of total polysaccharide, total saponins, total polyphenols and total flavonoids in the herbal mixture were 70.5, and 24.8, 13.0, and 26.2 mg/g, respectively.
Experimental design, animals, and housing
This study was approved by the Animal Care and Use Committee of Nanjing Agricultural University (SYXK2017-0027, Nanjing, China). The experiments were conducted at Jiangsu Huaduo Animal Husbandry Technology Co., Ltd. (Xuzhou, China). A total of 400 post-weaned pigs [Duroc × (Landrace × Yorkshire)] (equal number of males and females) with body weight (BW) about 8.37 ± 0.11 kg and age of 28 days were blocked according to BW and sex and then randomly allocated into one of four treatments: the CON group, fed with the basal diet (Supplementary Table 1); the HM1 group, fed with the basal diet supplemented with 0.5 g herbal mixture/kg diet; the HM2 group, fed with the basal diet supplemented with 1.0 g herbal mixture/kg diet; or the HM3 group, fed with the basal diet supplemented with 1.5 g herbal mixture/kg diet. There were 4 replicates (containing 2 male and 2 female pig pens) per treatment and 25 pigs per pen. The detailed feed formula and the nutritional levels were presented in Supplementary Table 1. All experimental pigs were raised in the same environment for 28 days. All pens were thoroughly cleaned and disinfected to reduce viral and bacterial contamination before the pigs moved in. During the trial, air-exhaust fans and hot-blast heaters were equipped to automatically control the room temperature between 21 and 25°C. The pens (3.5 × 4.5 m) were equipped with slatted plastic floors, an adjustable stainless-steel feeder, and two nipple drinkers for ad libitum access to feed and water.
The growth performance of weaned pigs
The weaned pigs and feed consumed were weighed on d 0, 14, and 28 of the experiment period to calculate the growth performances: average daily gain (ADG), average daily feed intake (ADFI), and feed to gain ratio (F: G). The diarrhea rates were determined based on the diarrhea score of pigs at the end of the experiment. The feces of weaned pigs were assessed regularly from 08:00 to 09:00 every day according to the criteria: 1-solid, well-formed feces; 2-loose and shapeless feces; 3-runny feces; and 4-watery diarrhea (32). Pigs with a fecal score ≥ 3 were considered to have diarrhea; pigs with a fecal score of <3 were considered normal. Diarrhea symptoms and mortality, if any, were recorded daily for each pig during the trial. The diarrhea rate was calculated as the (number of pigs with diarrhea)/(number of pigs tested × total experiment days) × 100%.
Complete blood count examination
A total of 64 pigs (8 male and 8 female pigs per treatment) were randomly selected to collect 5 mL blood via jugular venipuncture into anticoagulant (heparin) tubes on day 14 and 28 of the experimental period. The blood was then divided equally into two parts: one for complete blood count (CBC) test and the other for determination of antioxidant indicators. A total of 16 CBC indicators were measured by an automatic biochemical analyzer (Mindray BC-5000, Mindray Medical International Co. LTD, Shenzhen, China). These CBC indicators include white blood cell number (WBC), Neutrophil number (NEU), Lymphocyte number (LYM), monocyte number (MON), eosinophils number (EOS), basophils number (BAS), Red blood cell number (RBC), Hemoglobin (HGB), Erythrocyte specific Volume (HCT), Mean Erythrocyte Volume (MCV), Mean Erythrocyte Hemoglobin content (MCH), Mean Red blood cell hemoglobin concentration (MCHC), platelet count (PLT), mean platelet volume (MPV), platelet distribution width (PDW), and platelet hematocrit (PCT).
Antioxidant capacity and immunoglobulin M determination
The serum samples (n = 64) were obtained by centrifuging (4,000 × g for 10 min) at 4°C, and stored at −80°C until analysis. Six oxidative stress indicators, such as malondialdehyde (MDA), total superoxide dismutase (SOD), glutathione (GSH), glutathione peroxidase (GSH-Px), catalase (CAT), and total antioxidant capacity (T-AOC) were determined using ELISA kits (Nanjing Jiancheng Institute of Biological Engineering, Nanjing, China) according to the manufacturer's instructions. The sensitivities of the kits are as follows: MDA: 0.1 nmol/mL; SOD: 0.1 U/mL; GSH: 10 μmol/L; GSH-Px: 0.2 mg/mL; CAT: 0.2 U/mL; T-AOC: 0.1 mmol/gprot. The intra- and inter-assay coefficients of variation were <10 and 15%, respectively. Concentration of immunoglobulin M (IgM) was determined using an ELISA kit (Nanjing Jiancheng Institute of Biological Engineering, Nanjing, China) with an assay sensitivity of 0.1 mg/ml according to the manufacturer's instruction. A microplate reader (Tecan, Austria GmbH, Grödig, Austria) was used to determine all indicators. The intra- and inter-assay of the coefficient of variation were <10 and 15%, respectively.
Fecal sample collection, DNA extraction, and 16S rRNA gene sequencing
On day 14 and 28 of the experimental period, a total of 96 weaned pigs (6 male and 6 female pigs per treatment) were randomly selected to collect fresh feces using clean plastic bags into a 1.5 mL centrifugation tube and stored at −80°C until analysis. Genomic DNA of fecal samples was extracted by the hexadecyltrimethylammonium bromide (CTAB) method (33), and DNA purity was detected by agarose gel electrophoresis. The genomic DNA was used as the template. The V3–V4 region of the bacterial 16S rRNA gene was amplified with the specific primers (F: 5′-GTGYCAGCMGCCGCGGTAA-3′, R: 5′-GGACTACHVGGGTWTCTAAT-3′). The PCR products were confirmed with 2% agarose gel electrophoresis, purified with AMPure XT beads (Beckman Coulter Genomics, Danvers, MA, USA), and then quantified by an Invitrogen Qubit 4.0 fluorometer (Invitrogen, Thermo Fisher Scientific, Waltham, MA, USA). For library construction, the NEBNext® Ultra™ DNA Library Prep Kit (Illumina, United States) was used. After the library was qualified, the library was sequenced on an Illumina next-generation sequencing platform NovaSeq 6000 to generate the 250 bp paired-end reads. The original sequencing data were spliced and filtered to obtain clean reads. Then, through Divisive Amplicon Denoising Algorithm 2 (DADA2), the sequences with an abundance <5 were filtered out to obtain the final Amplicon Sequence Variants (ASVs) (34). Each de-weighting sequence generated after DADA2 is called ASVs, which corresponds to the Operational Taxonomic Units (OTUs) representative sequence. The available data were then annotated and abundances were analyzed to reveal the species composition, and further Alpha and Beta diversity analyses were performed to explore differences in community structure.
Statistical analysis
Data on growth performances (body weight, average daily gain, average daily feed intake, feed to gain ratio), CBC test, antioxidant indicators, and relative abundance of microbiota were analyzed using the GLIMMIX procedure of SAS® software 9.4 (SAS Institute, Inc., Cary, NC, USA). The model included dietary treatment, gender, and their interaction as fixed effects and pen as random effect. The experimental units for the growth performance parameters (average daily gain, average daily feed intake, and feed to gain ratio) and diarrhea rate were the pen, while the experimental units for the antioxidant indicators, blood hematology parameters and gut microbiota was the individual pig. A partial correlation analysis between the gut microbiota and growth performance indicators, antioxidant indicators was carried out using R statistical software. Graphs were performed using Graphpad Prism 8.0 (GraphPad Software Inc., San Diego, CA, USA). The results were expressed as the mean ± standard error of the mean (SEM). All tests were considered statistically significant at p < 0.05 and tendencies at 0.05 < p < 0.10. P-values were rounded to three digits after the decimal point: p-values < 0.001 are shown as < 0.001.
Results
Growth performance and diarrhea rate
The effects of the herbal mixture on the growth performance and the diarrhea rate of weaned pigs were presented in Table 1. There was no significant effect of dietary treatment, sex or treatment × sex interaction on initial body weight and body weight on day 14 (p > 0.05). On day 28 of the experiment period, the body weight of pigs in the HM1, HM2, and HM3 groups were greater than that of pigs in the CON group (19.17, 19.73, and 19.26 vs. 18.70 kg, p < 0.001). During the first period (day 1–14), dietary supplementation with herbal mixture tended to increase ADG compare to the CON group (325.52, 340.16, and 322.77 vs. 307.99 g/day, p = 0.064). ADFI in the HM3 group was less than that in the CON group (464.50 vs. 479.84 g/day, p = 0.002). Female pigs had a higher ADFI compared with male pigs (478.84 vs. 472.33 g/day, p = 0.018). Dietary supplementation with herbal mixture had a lower F: G compared with pigs offered the basal diets (1.48, 1.40, and 1.44 vs. 1.56, p = 0.004). During the second period (day 15–28), ADFI in the HM1 and HM2 groups were greater than that in the CON group (673.93 and 676.62 vs. 659.89 g/day, p = 0.009). Female pigs had a higher ADFI compared with male pigs (674.41 vs. 664.88 g/day, p = 0.007). In the male pigs, dietary supplementation with herbal mixture had a greater ADFI compared with pigs offered the basal diets (674.57, 675.10, and 664.50 vs. 645.36 g/day, p = 0.015). Pigs offered herbal mixture had a lower F: G compared with the pigs offered the basal diets (1.49, 1.41, and 1.49 vs. 1.56, p < 0.001). Male pigs had a lower F: G compared with female pigs (1.46 vs. 1.51, p = 0.005). In the male pigs, F: G in the HM2 group was less than that of pigs in the CON group (1.42 vs. 1.49, p = 0.024). In the female pigs, dietary supplementation with herbal mixture had a lower F: G compared with pigs offered the basal diets (1.51, 1.40, and 1.52 vs. 1.62, p = 0.024). During the whole experiment period, dietary supplementation with herbal mixture had a greater ADG compared with pigs offered the basal diets (388.99, 409.78, and 386.22 vs. 365.74 g/day, p = 0.002). ADFI in the HM3 group was less than that in the CON group (566.32 vs. 576.59 g/day, p < 0.001). Dietary supplementation with herbal mixture had a lower F: G compared with pigs offered the basal diets (1.49, 1.41, and 1.47 vs. 1.58, p < 0.001). During the whole 28-day experimental period, dietary supplementation with herbal mixture had a lower diarrhea rate compared with pigs offered the basal diets (4.86, 4.46, and 3.79 vs. 6.30, p < 0.001).
Serum antioxidant indicators and IgM concentration
The effects of the herbal mixture on the antioxidant capacity of weaned pigs were presented in Table 2. There were evident tendencies of dietary treatment × sex interaction effects on the level of SOD (p = 0.060) and GSH (p = 0.052) in pigs on day 14. In the male pigs, the activity of SOD in the HM1 group was greater than that in the CON group (166.68 vs. 155.14 U/mL, p = 0.046). In the male pigs, the activity of GSH in the HM2 and HM3 were greater than that in the CON group (64.67 vs. 58.47 μmol/L, p = 0.036, 64.92 vs. 58.47 μmol/L, p = 0.037, respectively). On day 14, male pigs had a higher activity of GSH-Px compared with female pigs (671.54 vs. 620.64 mg/mL, p = 0.016). On day 14, the level of T-AOC in the HM3 group was significantly greater than that in the CON group (5.73 vs. 5.62 U/mL, p = 0.001). The concentration of MDA in the female pigs had a tendency to decrease compare with the male pigs (2.97 vs. 3.14 nmol/mL, p = 0.075). Dietary supplementation with herbal mixture had a greater concentration of IgM compared with the pigs offered the basal diets (1.91, 1.91, and 1.91 vs. 1.86 mg/mL, p = 0.026). On day 28, the dietary supplementation with herbal mixture significantly increased the activity of CAT in weaned pigs (6.77, 6.89, and 6.80 vs. 5.97 U/mL, p = 0.022) compared with the CON group. The level of T-AOC in the HM groups was significantly greater than that in the CON group (4.68, 4.67, and 4.58 vs. 4.36 U/mL, p = 0.018). The concentration of MDA in the HM2 and HM3 group was significantly less than that in the CON group (3.12 and 2.97 vs. 3.50 nmol/mL, p = 0.015). There was no effect of treatment, sex or treatment × sex interaction on the activity of other antioxidant indicators in the serum of pigs (p > 0.05).
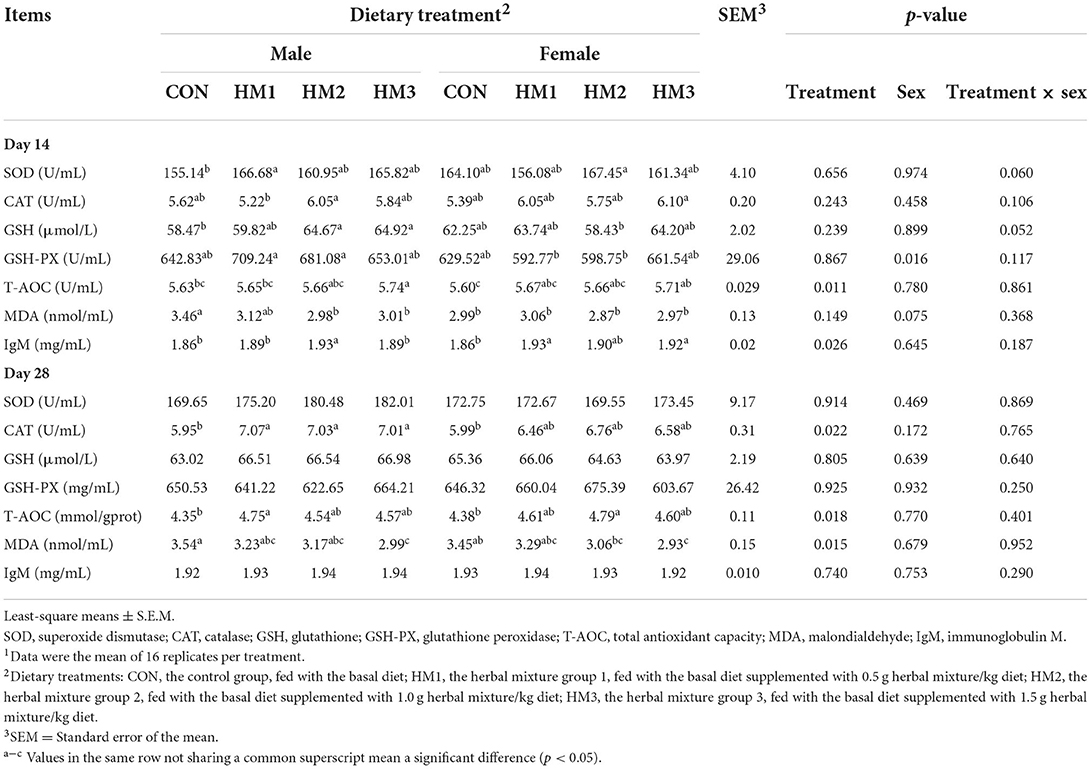
Table 2. Effects of herbal mixture on antioxidant indicators and IgM concentrations in serum of weaned pigs1.
Complete blood count test
The effects of the herbal mixture on the complete blood count of weaned pigs were presented in Supplementary Table 2. On day 14, female pigs had a higher concentration of MCHC compared with male pigs (317.91 vs. 309.97 g/L, p = 0.008). On day 28 of the trial, dietary supplementation with herbal mixture had greater number of WBC compared with pigs offered the basal diets (23.13, 24.32, and 23.98 vs. 22.60 × 109/L, p < 0.001). The number of NEU in the HM2 and HM3 groups were significantly greater than that in the CON group (12.36 and 11.98 vs. 10.17 × 109/L, p = 0.001). Similarly, the number of MON in the HM2 and HM3 groups were significantly greater than that in the CON group (1.97 and 2.04 vs. 1.70 × 109/L, p < 0.001). Dietary supplementation with herbal mixture had greater concentration of MCHC compared with pigs offered the basal diets (328.81, 319.69, and 323.81 vs. 313.31 g/L, p < 0.001). The number of PCT in the HM1 group was significantly less than that in the CON group (0.31 vs. 0.45%, p = 0.025).
Effect of herbal mixture on intestinal microbiota
To evaluate the effects of the herbal mixture on intestinal microbiota diversity, 16s rRNA gene sequencing was performed using the fresh feces of weaned pigs. A total of 7,205,863 clean reads were obtained from 96 fecal samples after quality control of the original raw reads with an average of 75,061 ± 1,233 reads per sample (Supplementary Table 3). These results indicated that the sequencing data met the criteria for further analysis.
Effect of herbal mixture on intestinal microbiota diversity of weaned pigs
As shown in Figure 1, chao 1, observed_OTUs, shannon, and simpson indexes were used to analyze the differences in Alpha diversity between groups. On day 14, chao1 and observed_OTUs indexes of the HM1, HM2, and HM3 groups were greater than those of the CON group (p < 0.05). The Shannon index of the HM1 group was greater than that of the CON group (p < 0.05). The Simpson index in the HM2 group was less than that in the CON group (p < 0.05). On day 28, chao1, observed_ OTUs, and Shannon index of the HM1 and HM2 groups were less than those of the CON group (p < 0.05). The Simpson index of the HM1 group was less than that of the CON group (p < 0.05).
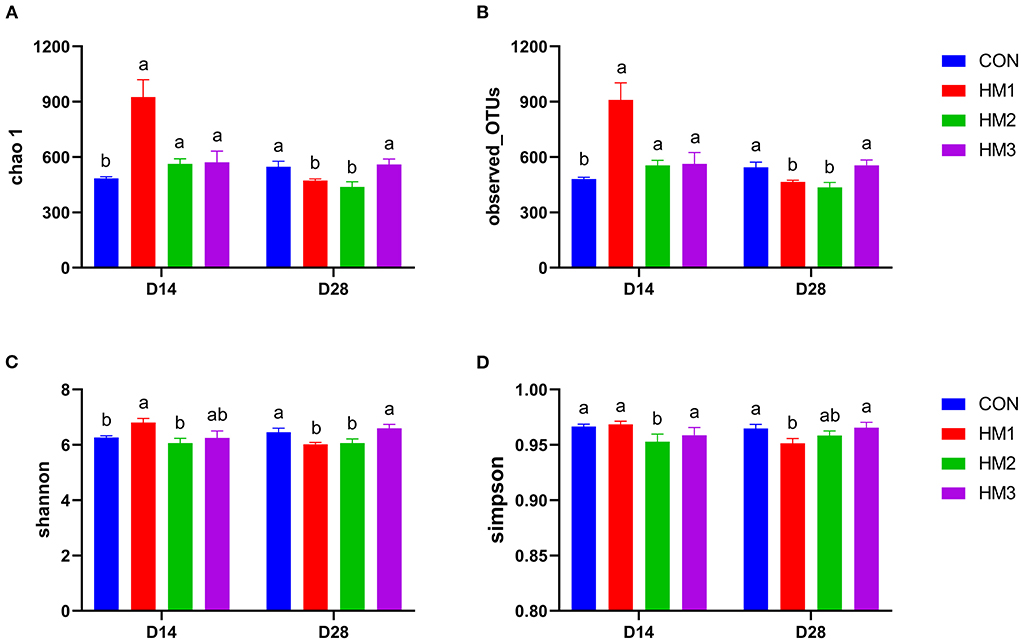
Figure 1. Effects of herbal mixture on the intestinal microbiota diversity in weaned pigs on day 14 and day 28 based on alpha diversity parameters of chao1 index (A), observed OTUs (B), shannon index (C) and simpson index (D). The X-axis is the experimental period, and the Y-axis is the diversity indexes. CON, basic diet; HM1, basic diet with 0.5 g/kg of herbal mixture; HM2, basic diet with 1.0 g/kg of herbal mixture; HM3, basic diet with 1.5 g/kg of herbal mixture. Different lowercase letters in the figure indicate statistically significant differences (p < 0.05).
Effect of herbal mixture on intestinal microbiota composition and abundance in weaned pigs
The overall microbiota composition at the phylum and genus levels were presented in Figure 2. At the phylum level, the top 10 dominant bacterial species in the feces of weaned pigs were Firmicutes, Bacteroidata, Actinobacteriota, Proteobacteria, Euryarchaeota, Spirochaetoa, Acidobacteriota, Chloroflexi, Verrucomicrobiota, and Desulfobacterota. The fecal flora composition of the pigs was dominated by Firmicutes, accounting for 78.29%, and 75.44% on day 14, and 28, respectively. Bacteroidata accounted for 13.96%, and 15.79% on day 14 and 28, respectively (Figure 2A). At the genus level, the top 10 dominant bacterial species in the feces of weaned pigs were Lactobacillus, Bifidobacterium, Clostridium_sensu_stricto_1, Prevotella, Muribaculaceae, Blautia, Clostridia_UCG-014, Catenisphaera, Holdemanella, and Faecalibacterium. The fecal flora composition of the pigs was dominated by Lactobacillus, accounting for 9.79%, and 4.70% on day 14, and 28, respectively (Figure 2B).
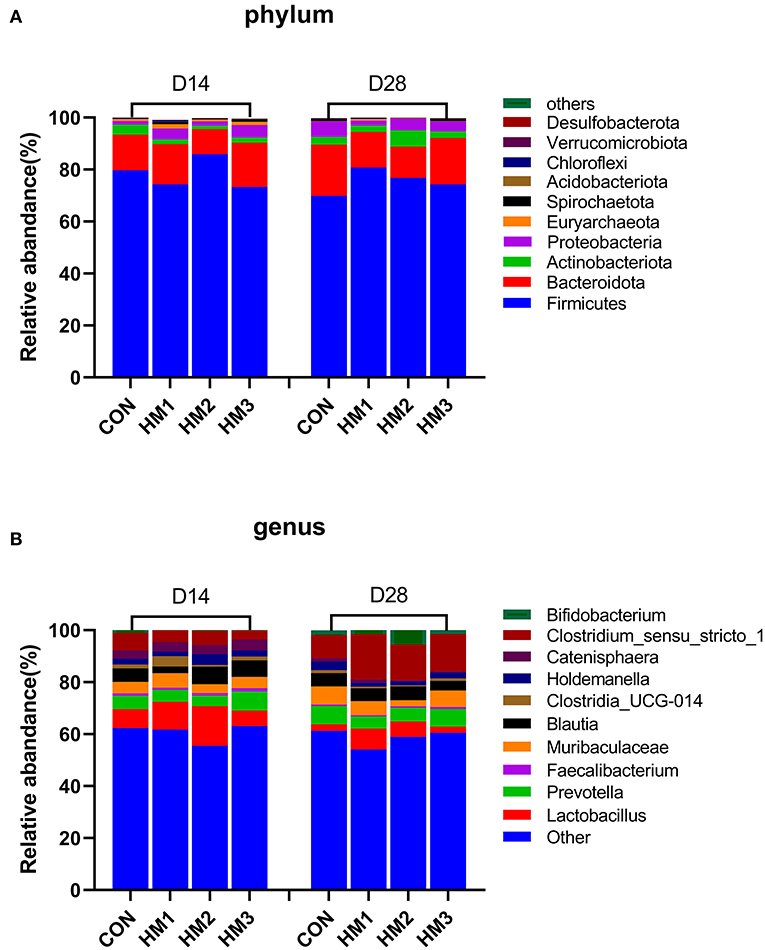
Figure 2. Intestinal microbiota compositions of different groups at phylum (A) and genus (B) levels on days 14 and 28. CON, basic diet; HM1, basic diet with 0.5 g/kg of herbal mixture; HM2, basic diet with 1.0 g/kg of herbal mixture; HM3, basic diet with 1.5 g/kg of herbal mixture.
When comparing the relative abundance of the four dominant phyla of the intestinal microbiota between groups (Figure 3), we found that there were significant differences between the experimental groups and the control group. On day 14, the relative abundance of Firmicutes in the HM2 group was greater than that in the HM1 and HM3 groups (p < 0.05) (Figure 3A). The relative abundance of Bacteroidata in the HM2 group was less than that in the CON group (p < 0.05) (Figure 3B). The relative abundance of Actinobacteriota in the HM1 group was greater than that in the HM2 and HM3 groups (p < 0.05) (Figure 3C). The relative abundance of Proteobacteria in the HM3 group was greater than that in the CON group (p < 0.05) (Figure 3D). Male pigs had a lower relative abundance of Firmicutes compared with female pigs (74.59 vs. 81.98%, p = 0.009), while male pigs had a higher relative abundance of Bacteroidata (16.80 vs. 10.80%, p = 0.019) and Proteobacteria (2.41 vs. 1.33%, p = 0.006) compared with female pigs (Supplementary Figure 1). There was a significant effect of treatment × sex interaction on the relative abundance of Actinobacteriota and Proteobacteria on day 14 (p < 0.05). Specifically, the relative abundance of Actinobacteriota in the HM2 group was increased compared with the HM3 group in the male pigs (1.80 vs. 1.13%, p = 0.002). In the female pigs, the relative abundance of Actinobacteriota in the HM2 group was decreased compared with the HM1 group (0.70 vs. 1.70%, p = 0.002) and CON group (0.70 vs. 1.45%, p = 0.002) (Supplementary Figure 2A). In the male pigs, the relative abundance of Proteobacteria in the HM1 and HM3 groups were greater than that in the CON group (3.70 vs. 0.76%, 3.79 vs. 0.76%, p = 0.001, respectively). In the female pigs, the relative abundance of Proteobacteria in the HM2 group was greater than that in the CON group (1.98 vs. 0.83%, p = 0.001) (Supplementary Figure 2B). On day 28, the relative abundance of Firmicutes in the HM1 and HM2 groups was greater than that in the CON group (p < 0.05) (Figure 3A). The relative abundance of Bacteroidata in the HM2 group was less than that in the CON group (p < 0.05) (Figure 3B). The relative abundance of Proteobacteria in the HM1 group was less than that in the CON group (p < 0.05) (Figure 3D). Male pigs had a higher relative abundance of Firmicutes (80.79 vs. 72.35%, p = 0.009), while had a lower relative abundance of Bacteroidata (12.36 vs. 19.22%, p = 0.009) compared with female pigs (Supplementary Figure 1). In the male pigs, the relative abundance of Proteobacteria in the HM1 group was less than that in the CON group (1.35 vs. 4.63%, p = 0.025) (Supplementary Figure 2B).
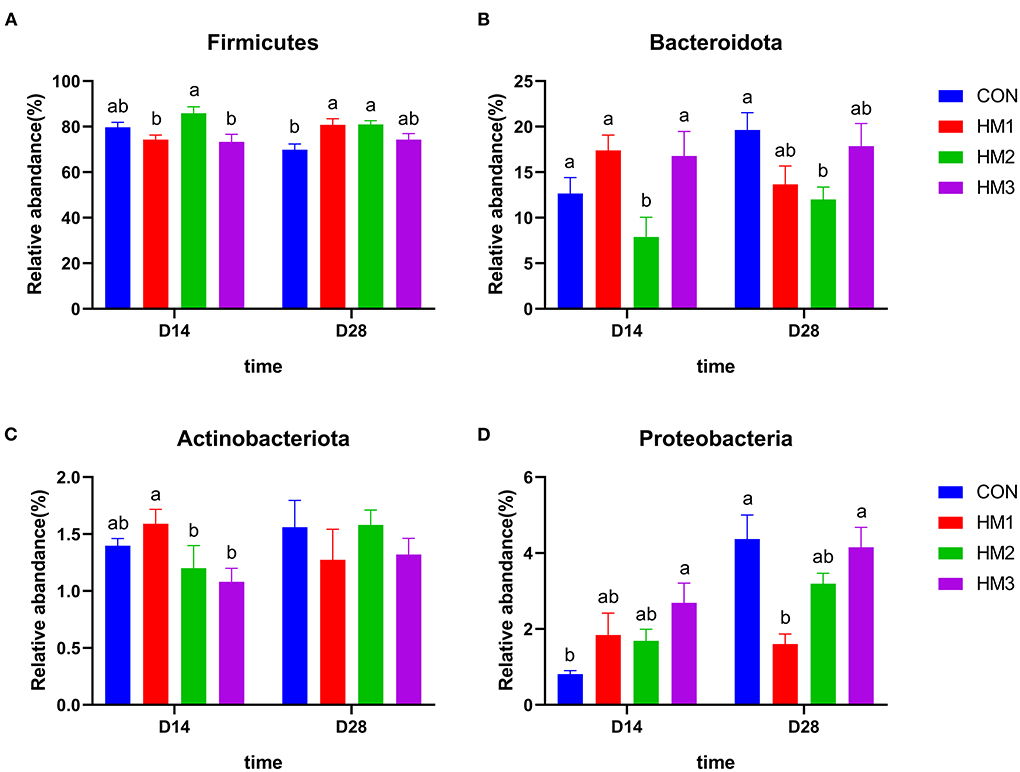
Figure 3. Comparison of dominant intestinal microbiota at phylum level. Relative abundances of Firmicutes (A), Bacteroidetes (B), Actinobacteria (C), and Proteobacteria (D) among the four groups on d 18 and d 35. CON, basic diet; HM1, basic diet with 0.5 g/kg of herbal mixture; HM2, basic diet with 1.0 g/kg of herbal mixture; HM3, basic diet with 1.5 g/kg of herbal mixture. Different lowercase letters in the figure indicate statistically significant differences (p < 0.05).
The relative abundance of the dominant genus of the intestinal microbiota between groups were presented in Figure 4. On day 14, the relative abundance of Lactobacillus and Blautia in the HM2 group was greater than that in the other groups (p < 0.05) (Figures 4A,B). The relative abundance of Clostridium and Prevotella in the HM groups was less than that in the CON groups (p < 0.05) (Figures 4C,D); The relative abundance of Bifidobacterium in the HM1 and HM3 groups were less than that in the CON group (p < 0.05) (Figure 4E); The relative abundance of Clostridia_UCG-014 in the HM1 group was greater than that in the CON group (p < 0.05) (Figure 4F). In the male pigs, the relative abundance of Bifidobacterium in the HM2 group was greater than that in the CON group (0.29 vs. 0.11%, p = 0.008) (Supplementary Figure 3). On day 28, the relative abundance of Lactobacillus in the HM1 and HM2 groups was greater than that in the CON group (p < 0.05) (Figure 4A). The relative abundance of Clostridium in the HM1 group was greater than that in the CON group (p < 0.05) (Figure 4C). The relative abundance of Prevotella in the HM1 group was less than that in the CON group (p < 0.05) (Figure 4D). Female pigs had a higher relative abundance of Prevotella (6.36 vs. 4.38%, p = 0.014) compared with female pigs (Supplementary Figure 4).
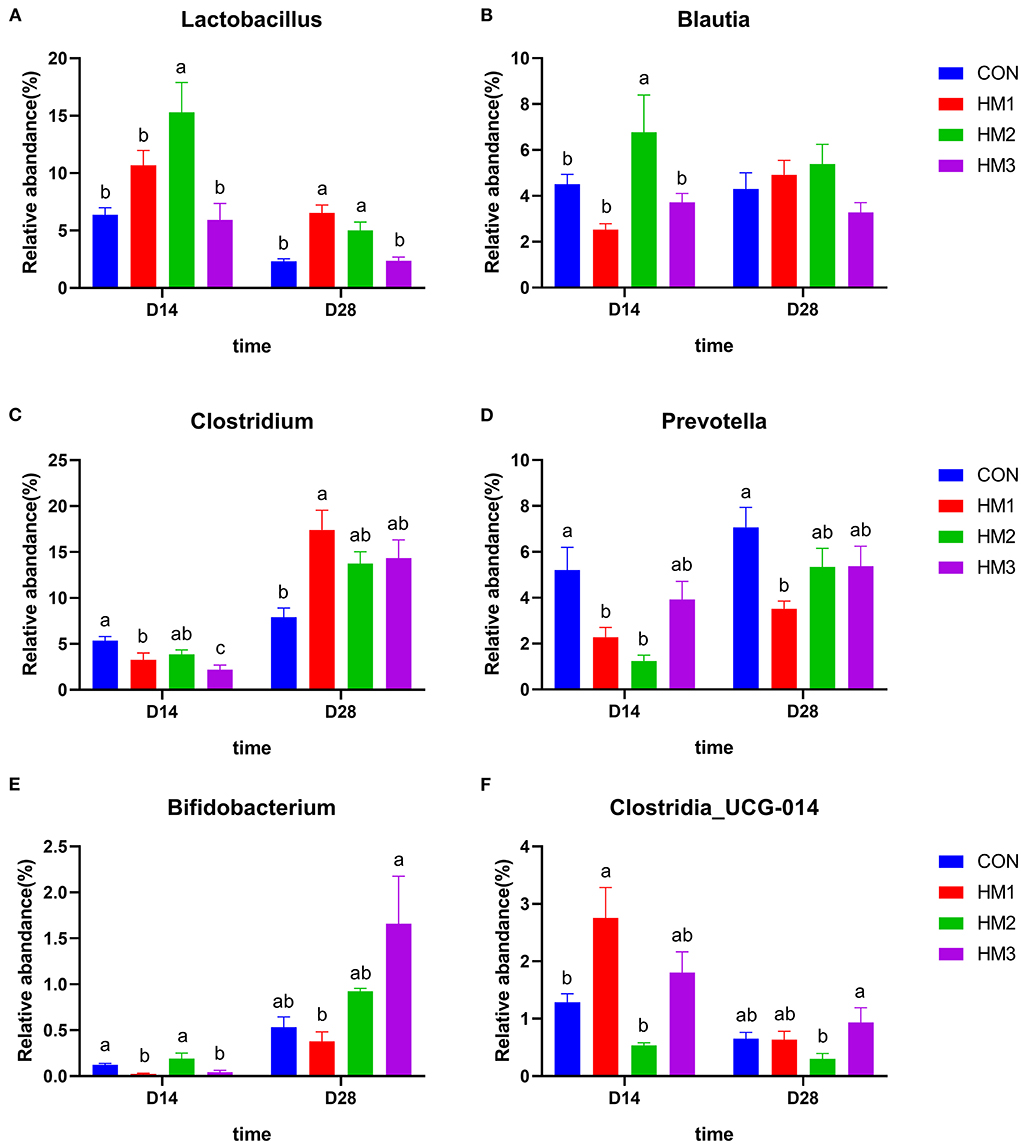
Figure 4. Comparison of dominant intestinal microbiota at genus level. Relative abundances of Lactobacillus (A), Clostridium (B), Blautia (C), Clostridia_UCG-014 (D), Prevotella (E), and Bifidobacterium (F) among the four groups on d 18 and d 35. CON, basic diet; HM1, basic diet with 0.5 g/kg of herbal mixture; HM2, basic diet with 1.0 g/kg of herbal mixture; HM3, basic diet with 1.5 g/kg of herbal mixture. Different lowercase letters in the figure indicate statistically significant differences (p < 0.05).
To identify bacterial taxa that significantly differentiated among HM and CON groups, a linear discriminant analysis effect size (LEfSe) analysis (LDA score > 4) was performed (Figure 5). On day 14, Clostridia_UCG-014 (order), Clostridia_UCG-014 (genus), and Clostridia_UCG-014 (family) were more abundant in the HM1 group; Firmicutes (phylum), Lactobacillales (order), and Blautia (genus) were more abundant in the HM2 group; Lachnospiraceae (family), and Lachnospirales (order) were more abundant in HM3 group; Streptococcus (genus), Streptococcaceae (family), Olsenella (genus), Atopobiaceae (family), and erysipelotrichaceae_UCG-006 (genus) were enriched in the CON group (Figure 5A). The cladogram reports the taxa showing different abundance values according to LEfSe. On day 14, 5 and 2 different taxonomic levels of microorganisms were identified as potential biomarkers between the HM and CON groups, respectively (Figure 5B). On day 28, the relative abundances of Clostridiaceae (family), Clostridiales (order), and Clostridium_sensu_stricto_1 (family) increased significantly in the HM1 group, whereas Proteobacteria (phylum) was more prevalent in the CON group (Figure 5C). However, there were no significant differences in microbiota abundance between the HM2, HM3, and CON groups. On day 28, only 2 different taxonomic levels of microorganisms were identified as potential biomarkers in the HM1 group (Figure 5D).
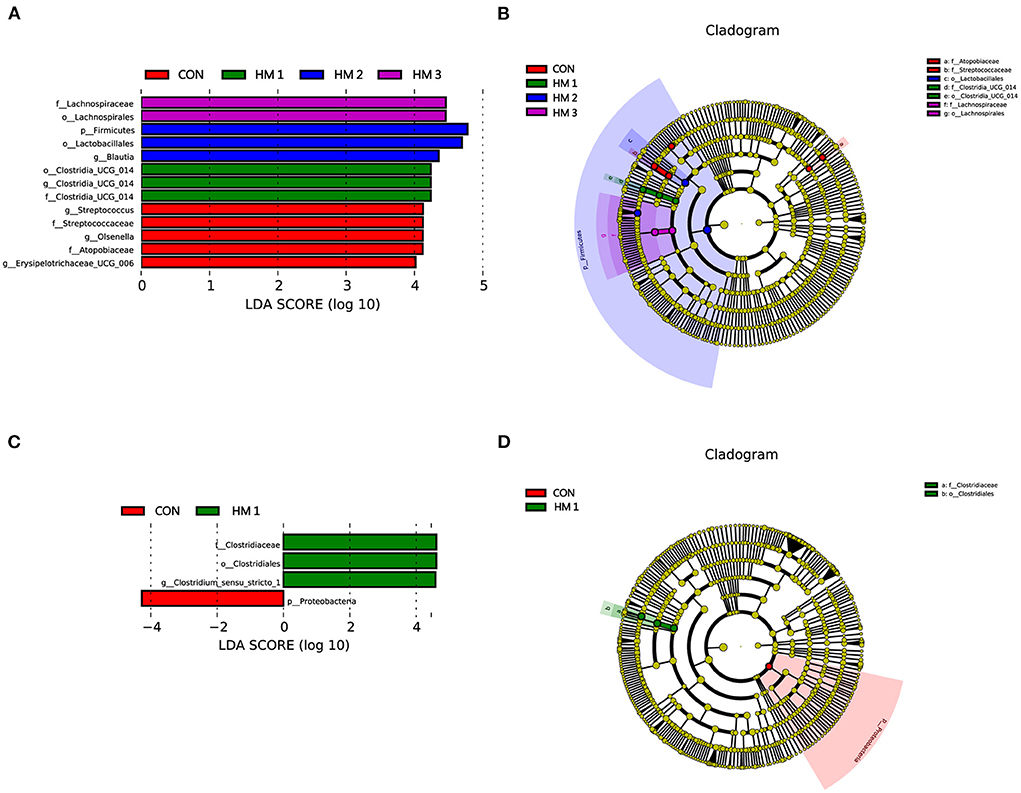
Figure 5. Linear discriminant analysis coupled with effect size (LEFse). The LEfSe analysis identified the differentially abundant (LDA score > 4) bacterial taxa between the HM group and the CON group on day 14 (A) or on day 28 (C). A linear discriminant analysis (LDA) score higher than 4 indicates that the relative abundance of the corresponding group is higher than that of the other groups. The abscissa of the bar chart represents the LDA value, and the ordinate is the different species of the selected treatments. Cladogram showing the most discriminative bacterial clades identified by LEfSe between the HM group and CON group on day 14 (B) or on day 28 (D). LEfSe taxonomic clade: Different colors indicate that some taxa are enriched in different groups. The size of a circle is based on relative abundance. CON, basic diet; HM1, basic diet with 0.5 g/kg of herbal mixture; HM2, basic diet with 1.0 g/kg of herbal mixture; HM3, basic diet with 1.5 g/kg of herbal mixture.
Effect of herbal mixture on intestinal microbiota function of weaned pigs
The Kyoto Encyclopedia of Genes and Genomes (KEGG) pathway enrichment analysis were presented in Supplementary Figure 5. The top 35 KEGG pathways between the HM and the CON groups were selected from the results of species function annotation and abundance information, and then clustered by their function annotation information and the inter-group differences, to obtain a KEGG pathway enrichment analysis heatmap. Predicted KEGG pathways were mainly concentrated in ABC-2 type transport system ATP-Binding protein, ATP-binding cassette, subfamily B, bacterial, sucrose-6-phosphatase, putative ABC transport system permease protein, ABC-2 type transport system permease protein, RNA polymerase sigma-70 factor, ECF subfamily, and K02003 putative ABC transport system ATP-binding protein pathways.
Correlation analysis between the differential microbial species and measured parameters
The partial correlation analyses between the relative abundance of dominant bacteria and growth performance, serum antioxidant parameters were presented in Figure 6. These results indicated that ADG and F: G were positively associated with phyla Firmicutes (R = 0.610, R = 0.674, respectively, p < 0.05) (Figure 6A). Whereas, ADG was negatively associated with phyla Bacteroidota (R = −0.609, p < 0.05) (Figure 6A). Meanwhile, the abundance of Firmicutes was negatively associated with the diarrhea rate of pigs (R = −0.600, p < 0.05). Interestingly, the serum T-AOC were positively associated with genus Lactobacillus, Catenisphaera, and Faecalibacterium (R = 0.256, R = 0.247, R = 0.244, respectively, p < 0.05), while negatively associated with phyla Proteobacteria and genus Clostridium (R = −0.239, R = −0.399, respectively, p < 0.05) (Figure 6B). Furthermore, Clostridia and Catenisphaera had significant negative correlation with the concentration of serum IgM (R = −0.251, R = −0.222, respectively, p < 0.05) (Figure 6B).
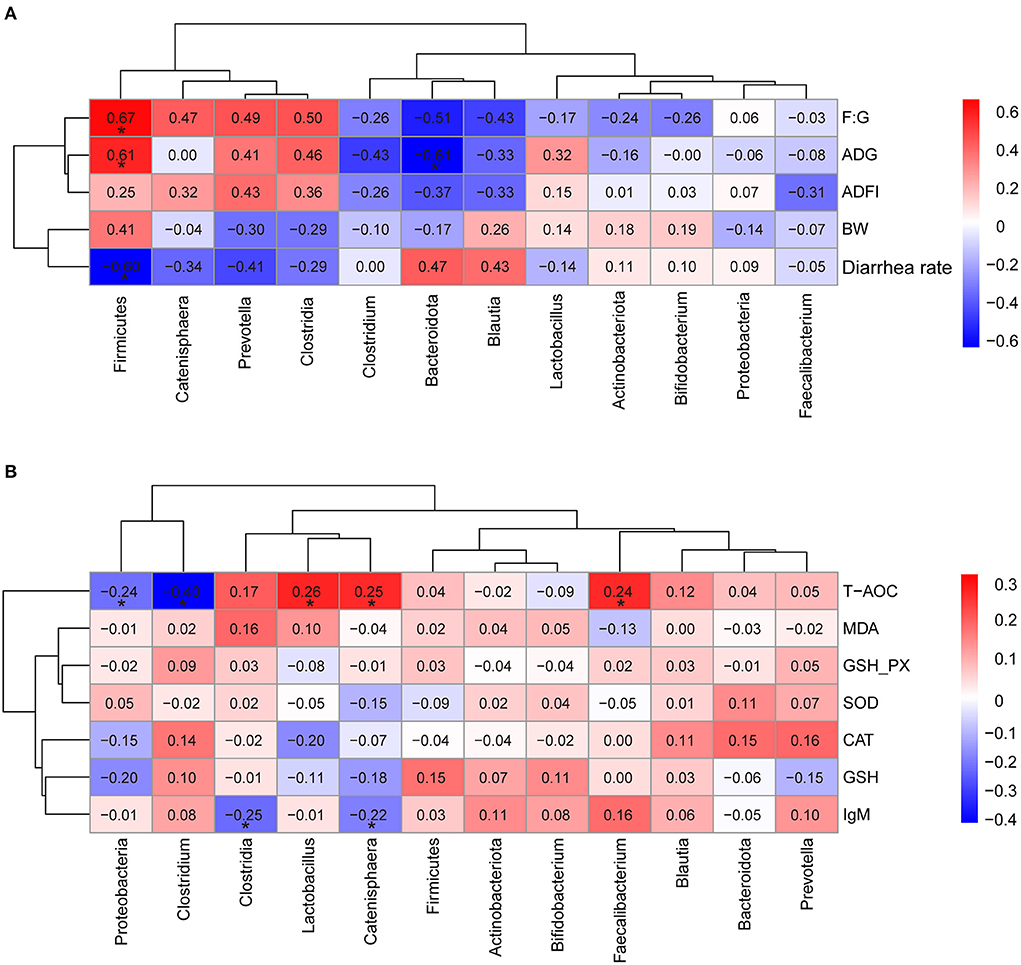
Figure 6. Heatmap of the partial correlation analysis between the dominant microbial species and growth performance (A), serum antioxidant parameters (B). ADG, the average daily gain; ADFI, average daily feed intake; F: G, feed to gain ratio; T-AOC, total antioxidant capacity; MDA, malondialdehyde; GSH-PX, glutathione peroxidase; SOD, superoxide dismutase; CAT, catalase; GSH, glutathione; IgM, immunoglobulin M. The red represents positive correlation and the blue represents negative correlation, respectively (*p < 0.05).
Discussion
Herbal medicine and their extracts are widely used as health promoters and novel approaches to disease treatment (35). Meanwhile, herbal medicine feed additives have also been receiving increasing attention due to their ability to improve the nutrition and wellbeing of farm animals (36). Furthermore, herbal medicine as an alternative to antibiotic feed additives improved the growth performance, meat quality, and nutrient digestibility parameters of pigs, which contributed to reducing the cost of raising pigs and produced antibiotic-free pork products (37). Combined herbal mixture have been shown to be more effective in improving animal health than single herbal extracts (38). Herbal mixtures are also regarded as one of the most economical and effective additives due to their ease of preparation and low cost, so their use has increased in livestock production (39). However, the effect of the herbal mixture containing Paeoniae Radix Alba, licorice, dandelion, and tea polyphenols on weaned pig production is not yet known. The present study aims to investigate the effects of these herbal mixtures on the growth performance, antioxidant capacity, immune response, and intestinal microbiota of weaned pigs.
In the swine industry, newly weaned pigs simultaneously encounter psychological, physiological, and nutritional pressures, resulting in impaired the digestive and absorptive capacity, which has a negative impact on feed intake and daily gain of weaned pigs (40). It is reported that adding feed additives to the diet reduces the negative effects of weaning stress (41). We first investigated the effect of herbal mixtures as promising feed additives that are able to substitute antibiotics on the growth performance of weaned pigs. Our findings indicated that the dietary supplementation of herbal mixture significantly improved the growth performance of weaned pigs as demonstrated by several indicators, including the BW, ADG, ADFI, and F: G, which suggested that herbal mixture effectively helped pigs overcome weaning stress. Specifically, the body weight at the end of the experiment period and the average daily gain during the whole experiment period of the pigs supplemented with herbal mixture were significantly greater than those of the CON group. Among them, the HM2 group (basic diet with 1.0 g/kg of herbal mixture) had the highest BW and ADG at the end of the trial. Previous studies found that weaned pigs fed herbal mixtures (including Codonopsis pilosula, Radix astragalus, R. isatidis, R. paeoniae alba, and Atractylodes macrocephalae) supplementation diets had positive effects on BW and ADG, which was in agreement with our results (42). We speculated that the beneficial effects of herbal mixtures on the growth performance of pigs due to a variety of nutrients and active ingredients in herbal medicines, which improved digestive tract function in pigs (43). In addition, we also observed that the feed intake of pigs in the HM1 and HM2 group were greater than that of CON group during the second experiment period, while the feed intake of pigs in the HM3 group was the lowest among the four groups during the whole experiment period. Due to herbal mixture ingredients including Paeoniae Radix Alba, dandelion, and tea polyphenols tasting bitter to mammals (44, 45), we speculated that the bitter taste of a high dose herbal mixture added to the diet might affect the appetite of pigs, resulting in a decrease in feed intake. Feed conversion efficiency is a very important indicator in pig production that determines the economic benefits of breeding. In the present study, the F: G of pigs supplemented with herbal mixture was significantly less than those in the CON group, which suggested that adding the herbal mixture to the diet was helpful to improve economic benefits. A previous study indicated that supplementation of plant extract mixtures in the drinking water increased ADG and decreased F: G in weaned pigs (46). Supplementation of 1.0 g dandelion/kg improved ADG, feed efficiency, and nutrient digestibility of weaned pigs (47). These were basically consistent with the experimental results presented in our study. The present study demonstrated that adding herbal mixture to the diets of pigs improved the growth performance of pigs, including improving average daily gain and reducing feed to weight ratio. Among them, dietary supplemented with 1.0 g/kg herbal mixture had the best effect.
Early weaned pigs are vulnerable to diarrhea and even death due to weaning stress and immature intestinal development (48). In the present study, the diarrhea rate of pigs supplemented with herbal mixture was significantly lower than that of the CON group, and the frequency of diarrhea decreased with the increase of the herbal mixture supplementation. A previous study reported that plant derived polyphenol extracts reduced post-weaning diarrhea caused by E. coli in pigs (49). A traditional herbal medicine called “Tongxie Yaofang” containing paeoniae lactiflora has been widely used to treat diarrhea-predominant irritable bowel syndrome (50). A herbal medicine compound containing licorice extract reduced diarrhea rates and improved the nutrient apparent digestibility of piglets (51). These observations were consistent with our results in the present study. The herbal mixture contains both Paeoniae Radix Alba, licorice and polyphenol extracts in this study, and their combination may be the reason for the significant reduction in diarrhea rate in weaned pigs.
Weaning stress disrupts free-radical metabolism and the antioxidative system, causing severe oxidative stress and mitochondrial dysfunction in pigs (52, 53). The serum antioxidant indicators like GSH, T-AOC, SOD, GSH-Px, and CAT were commonly used to assess pig antioxidant stress (54). The content of MDA reflects the degree of lipid peroxidation and impaired antioxidative capacity in animals (55). Next, we investigated the effects of the dietary supplementation of herbal mixture on antioxidant capacity of weaned pigs. In the present study, the concentrations of serum T-AOC and CAT in the herbal mixture groups were greater than that those in the CON group, whereas the concentration of serum MDA in herbal mixture group was lower. Single herbal medicine or herbal mixture extracts with antioxidant capacity have been widely used in both pharmaceutical raw materials and animal husbandry (56, 57). For example, a previous study demonstrated that an optimized mixture of plant polyphenols (apples, grape seeds, green tea, and olive leaves) increased serum GSH-Px and T-AOC activity of piglets (58). Similarly, supplementation of dandelion leaf powder increased the concentration of serum GSH-Px but decreased the concentration of MDA in broilers (59). Licorice extract, as the other ingredient in the mixture of herbal medicine, increased serum T-AOC level, and 50 mg/kg increased serum T-SOD activity and decreased serum MDA concentration in weaned pigs (60). It had been reported that paeoniflorin inhibits cell apoptosis, improves cell viability, and increases the concentration of SOD and CAT (61). These results demonstrated that the supplementation of herbal mixture to the diet decreased the oxidative stress level of weaned pigs, improved the antioxidant capacity of weaned pigs. This is probably due to the fact that the five herbs used in the herbal mixture in our study contain a variety of active ingredients with antioxidant effects.
Weaning stress causes immune system dysfunction and results in a negative impact on performance and health in pigs (62). IgM is the major serum immunoglobulin (63). The level of serum IgM was associated with the level of the autoimmune ability of piglets (64). White blood cells participate in the immune response of animals, and their abnormal increase indicates the presence of inflammation, so they are important clinical indicators for health status (65, 66). Both neutrophils and monocytes are immune-related white blood cells that aid in the host's defense against invading pathogens (67). We next further investigated the effect of adding herbal medicine mixture to the diet on the immune index of weaned pigs. In the present study, the serum IgM concentrations of pigs fed with the herbal mixture increased, indicating that the antibody produced by B lymphocytes was increased, which was conducive to improving the humoral immunity of pigs. Similarly, previous research has shown that supplementation with herbal mixture (include dandelion and licorice) increased serum IgG and IgM concentrations in piglets (68). This is basically consistent with our research results. It had been reported previously that herbs, such as Radix Alba (69), licorice (70), dandelion (71), and tea polyphenols (72), have functions of immunomodulatory and resisting inflammation, which could be used as a new-type immunoenhancer. The synergistic effect of Radix Alba and licorice improved immunity and reduced inflammation (73). Furthermore, our results indicated that the treatment of the herbal mixture with 1.0 and 1.5 g/kg concentrations significantly increased the WBC, NEU, and MON counts compared with the CON group. As immune biomarkers, abnormal levels of white blood cell (WBC) counts indicate abnormal immune processes (74). Neutrophils are the most abundant white blood cells and an important part of the innate immune system (75). Monocytes are innate immune cells and play an important defensive role in the primary innate immune response (76). A previous study had demonstrated that green tea extract increased the WBC counts in finishing pigs (77). WBC concentration was greater in pigs fed an herbal mixture (including: Astragalus membranaceus, Codonopsis pilosula and allicin) than in the CON group (16). This indicated that the herbal mixture had the potential to attenuate inflammation and enhance immunity in weaned pigs. Mean corpuscular hemoglobin concentration (MCHC) is a parameter of risk for anemia in weaning pigs (78). In the present study, the mean corpuscular hemoglobin concentration in the HM groups was greater than that of in the CON group, indicating that herbal mixture could reduce the incidence of anemia in weaned pigs by increasing corpuscular hemoglobin concentration. Based on the improvement of immunity indicators that accompanied supplementation with herbal mixture, we speculated that adding the herbal mixture to the feed could improve the swine immunity and also their general health status.
The gut microbiota is essential for gut homeostasis and host health (79). When people investigated the microbiota associated with health and diseases, they found that microbial diversity ensures the consistent immune regulation ability of microbiota, so it is more important to understand microbial diversity (80). Microbial diversity was assessed using the Chao1, observed_OTUs, Shannon, and Simpson index (81). In the present study, chao 1 and observed_OTUs in the HM groups were greater than those in the CON group on day 14 of the experiment. In contrast, chao 1, observed_OTUs, and Shannon index in the HM groups were less than those in the CON group on day 28 of the trial period. This indicates that the herbal mixture significantly improved the diversity of bacterial community in the early stage of the experiment. As animals aged, the number of microbial species in the feces of weaned pigs decreased after adding an herbal mixture to the diet. A previous study reported that the alpha diversity indices of intestinal microbiota in pigs changed with age, and they decreased at 35 days after weaning compared with 21 days after weaning (42). We speculated that the addition of herbal mixtures to the diet might inhibit pathogenic bacteria colonization in the intestine over time, and then reduce the diversity of intestinal flora.
In addition to its role in microbial diversity, our study also indicated that the addition of herbal mixtures to the diet had an impact on the abundance of intestinal flora in piglets. In this experiment, Firmicutes and Bacteroidetes were the dominant phyla in the gut microbiome of piglets, which was basically consistent with previous report (82). We also found that the HM2 group (basic diet with 1.0 g/kg of herbal mixture) significantly increased the relative abundance of Firmicutes, while decreasing the relative abundance of Bacteroidetes compared to the CON group. Meanwhile, the HM2 group had the highest BW and ADG at the end of the trial. This indicated obese pigs had a higher abundance of Firmicutes and a lower abundance of Bacteroidetes than lean pigs during the nursery period. Human and mouse studies yielded similar results (83, 84). In addition, the abundance of Firmicutes and Bacteroidetes was related to fat deposition in animals (85, 86). These results suggested that the intestinal microbiota (i.e., Firmicutes, Bacteroides) affected by the herbal mixture might affect the fat metabolism of the host, leading to fat deposition and body weight gain. Furthermore, the pathogenic bacteria decreased in the herbal mixture groups. For example, the relative abundance of Proteobacteria was lower in the HM2 group than in the control group on day 28. Studies had revealed that plant-derived polyphenols reduce the relative abundance of Proteobacteria in pigs, attenuating diarrhea and intestinal damage (87). Our study also found that the diarrhea rate of HM2 group was significantly lower than that of the control group. These results suggested that changes in the abundance of Proteobacteria in the intestine might disrupt the balance of microbial community structure and the health state of the host. Supplementing the diet with herbal mixtures was able to reduce the risk of intestinal diseases. Lactobacillus, as a beneficial bacteria, maintains the balance of intestinal microflora and enhances the human immune function (88). In the present study, the relative abundance of Lactobacillus in the HM1 and HM2 groups were greater than that in the CON group. Therefore, there was reason to believe that supplementing an appropriate dose of herbal mixture improved the relative abundance of Lactobacillus, which was conducive to improving host health. Interestingly, we found that Lactobacillus decreased but Bifidobacterium increased in the group with 1.5 g/kg herbal mixture. Previous studies showed that Lactobacillus and Bifidobacterium strains had different effects on host inflammation and intestinal microbial fermentation and composition (89). Lactic acid inhibits the proliferation of Lactobacillus during the fermentation process (90). Therefore, we speculate that the addition of high-dose herbal mixtures to the feed produce more lactic acid in the intestine, which inhibits the abundance of Lactobacillus in the intestine. Since Lactobacillus and Bifidobacterium are two major probiotics in the intestine (91), the abundance of Bifidobacterium could increase to maintain the balance of probiotics when the abundance of Lactobacillus decreases. The function prediction of the microbiota analysis suggested that the main pathways were protein transport, ATP binding, and sucrase, which indirectly reflected the microorganisms participate in the digestion and absorption of nutrients in the pig intestine. Our findings indicated that supplementing with herbal mixture to the diet had an inhibitory effect on pathogenic bacteria like Proteobacteria while increasing the relative abundance of beneficial bacteria like Lactobacillus and ultimately helping pigs cope with weaning stress.
When there are multiple variables, the correlation analysis between the two variables is often affected by other variables (92). In order to exclude the possible influence of controlling variables on other related variables, partial correlation analysis was used to assess the correlation between the relative abundance of dominant bacteria and measured parameters. Partial correlation analysis indicated that ADG was significantly positively correlated with the abundance of Firmicutes and negatively correlated with the abundance of Bacteroides. Previous reports indicated that the ADG of meat goats was linearly associated with the abundance of Firmicutes (93), which was consistent with our experimental observations. In this study, we did not find a significant correlation between body weight and the abundance of Firmicutes, but there was a positive correlation (R = 0.41). We speculated that the selection of samples and the differences between individuals mask this correlation due to limited sample sizes. The abundance of Firmicutes and Bacteroides has the potential to be considered as a biomarker to evaluate the body weight gain performance of pigs. Due to pigs being closer to humans in genetics, anatomy, and physiology and often used as biomedical models (94), our findings also support the view of Firmicutes and Bacteroidetes to evaluate human obesity (95). Our study also found that the abundance of Lactobacillus was positively associated with the T-AOC activity in the serum. Lactobacillus has been shown to have an antioxidant effect and to reduce oxidative stress injury by lowering ROS level (96). This indicated that supplementing herbal mixtures to the diet was capable of enhancing the abundance of Lactobacillus and then improving the antioxidant capacity of pigs.
Conclusions
The current findings indicated that supplementation of the herbal mixture (including: Paeoniae Radix Alba, licorice, dandelion, and tea polyphenols) to the diet significantly improved the ADG, while reduced feed to gain ratio (F: G) of weaned pigs. Our results also demonstrated that the herbal mixture significantly improved the total antioxidant capacity of weaned pigs. In addition, we also found that the relative abundance of beneficial intestinal microbiota such as Lactobacillus was increased in the pigs supplemented with 1.0 g/kg herbal mixture to the diet. These results also demonstrated the potential effect of the herbal mixture as an alternative to antibiotics in promoting the growth of weaned pigs, improving the ability of antioxidant capacity, and maintaining the intestinal microflora balance and improving the overall health of the host.
Data availability statement
The data presented in the study are deposited in the NCBI repository, accession number PRJNA851026. We have release our data, the data can be found at: https://www.ncbi.nlm.nih.gov/bioproject/PRJNA851026.
Ethics statement
The animal study was reviewed and approved by the Animal Care and Use Committee of Nanjing Agricultural University (SYXK2017-0027, Nanjing, China). Written informed consent was obtained from the owners for the participation of their animals in this study.
Author contributions
QX and MC analyzed the data. QX wrote—review and editing and visualization. MC wrote—original draft and investigation. RJ provided the resources. MC, XZ, and ML conducted the animal experiments. JZ contributed to formal analysis. XC and CZ contributed to software and visualization. BZ contributed to the project administration, and funding acquisition, project supervision. All authors have read and approved the final version of the manuscript.
Funding
This work was supported by the JBGS Project in Jiangsu Province, China (No. JBGS [2021]101).
Acknowledgments
We gratefully acknowledge Jiangsu Huado Co., Ltd. (Xuzhou, China) for the conditions provided during the animal feeding trial. In addition, we thank Wuxi Sanzhi Co., Ltd. (Wuxi, China) for providing the supplies and reagents for this trial.
Conflict of interest
Author RJ was employed by Wuxi Sanzhi Bio-Tech Co., Ltd.
The remaining authors declare that the research was conducted in the absence of any commercial or financial relationships that could be construed as a potential conflict of interest.
Publisher's note
All claims expressed in this article are solely those of the authors and do not necessarily represent those of their affiliated organizations, or those of the publisher, the editors and the reviewers. Any product that may be evaluated in this article, or claim that may be made by its manufacturer, is not guaranteed or endorsed by the publisher.
Supplementary material
The Supplementary Material for this article can be found online at: https://www.frontiersin.org/articles/10.3389/fvets.2022.971647/full#supplementary-material
Abbreviations
ADG, average daily gain; ADFI, average daily feed intake; F: G, feed to gain ratio; BW, body weight; CAT, catalase; T-AOC, total antioxidant capacity; MDA, malondialdehyde; SOD, superoxide dismutase; GSH, glutathione; GSH-Px, glutathione peroxidase; HM, herbal mixture; CBC, complete blood count; IgM, immunoglobulin M; OTUs, Operational Taxonomic Units; SEM, standard error of the mean; CON, control; KEGG, Kyoto Encyclopedia of Genes and Genomes.
References
1. Campbell JM, Crenshaw JD, Polo J. The biological stress of early weaned piglets. J Anim Sci Biotechnol. (2013) 4:19. doi: 10.1186/2049-1891-4-19
2. Barton MD. Antibiotic use in animal feed and its impact on human healt. Nutr Res Rev. (2000) 13:279–99. doi: 10.1079/095442200108729106
3. Khan MZH. Recent biosensors for detection of antibiotics in animal derived food. Crit Rev Anal Chem. (2022) 52:780–90. doi: 10.1080/10408347.2020.1828027
4. Guo S, Liu L, Lei J, Qu X, He C, Tang S, et al. Modulation of intestinal morphology and microbiota by dietary Macleaya cordata extract supplementation in Xuefeng Black-boned Chicken. Animal. (2021) 15:100399. doi: 10.1016/j.animal.2021.100399
5. Gong J, Yin F, Hou Y, Yin Y. Review: Chinese herbs as alternatives to antibiotics in feed for swine and poultry production: potential and challenges in application. Can J Anim Sci. (2014) 94:223–41. doi: 10.4141/cjas2013-144
6. Chen JS, Kang BJ, Yao K, Fu CX, Zhao YR. Effects of dietary Macleaya cordata extract on growth performance, immune responses, antioxidant capacity, and intestinal development in weaned piglets. J Appl Anim Res. (2019) 47:349–56. doi: 10.1080/09712119.2019.1636800
7. Ji Y, Wang T, Wei ZF, Lu GX, Jiang SD, Xia YF, et al. Paeoniflorin, the main active constituent of Paeonia lactiflora roots, attenuates bleomycin-induced pulmonary fibrosis in mice by suppressing the synthesis of type I collagen. J Ethnopharmacol. (2013) 149:825–32. doi: 10.1016/j.jep.2013.08.017
8. Ren HT, Huang Y, Lin L. Effects of dietary supplementation with peony pollen on growth, intestinal function, fillet quality and fatty acids profiles of common carp. Aquacult Nutr. (2021) 27:908–17. doi: 10.1111/anu.13235
9. Kitagawa I. Licorice root. A-natural sweetener and an important ingredient in Chinese medicine. Pure Appl Chem. (2002) 74:1189–98. doi: 10.1351/pac200274071189
10. Katayama M, Fukuda T, Okamura T, Suzuki E, Tamura K, Shimizu Y, et al. Effect of dietary addition of seaweed and licorice on the immune performance of pigs. Anim Sci J. (2011) 82:274–81. doi: 10.1111/j.1740-0929.2010.00826.x
11. Gonzalez-Castejon M, Visioli F, Rodriguez-Casado A. Diverse biological activities of dandelion. Nutr Rev. (2012) 70:534–47. doi: 10.1111/j.1753-4887.2012.00509.x
12. Samolińska W, Grela ER, Kowalczuk-Vasilev E, Kiczorowska B, Klebaniuk R, Hanczakowska E. Evaluation of garlic and dandelion supplementation on the growth performance, carcass traits, and fatty acid composition of growing-finishing pigs. Anim Feed Sci Technol. (2020) 259:114316. doi: 10.1016/j.anifeedsci.2019.114316
13. Khan N, Mukhtar H. Tea polyphenols for health promotion. Life Sci. (2007) 81:519–33. doi: 10.1016/j.lfs.2007.06.011
14. Hara H, Orita N, Hatano S, Ichikawa H, Hara Y, Matsumoto N, et al. Effect of tea polyphenols on fecal flora and fecal metabolic products of pigs. J Vet Med Sci. (1995) 57:45–9. doi: 10.1292/jvms.57.45
15. Wang L, Zhou GB, Liu P, Song JH, Liang Y, Yan XJ, et al. Dissection of mechanisms of Chinese medicinal formula Realgar-Indigo naturalis as an effective treatment for promyelocytic leukemia. Proc Natl Acad Sci USA. (2008) 105:4826–31. doi: 10.1073/pnas.0712365105
16. Lan RX, Park JW, Lee DW, Kim IH. Effects of Astragalus membranaceus, Codonopsis pilosula and allicin mixture on growth performance, nutrient digestibility, faecal microbial shedding, immune response and meat quality in finishing pigs. J Anim Physiol Anim Nutr. (2017) 101:1122–29. doi: 10.1111/jpn.12625
17. Keith CT, Borisy AA, Stockwell BR. Multicomponent therapeutics for networked systems. Nat Rev Drug Discov. (2005) 4:71–8. doi: 10.1038/nrd1609
18. Zhou X, Seto SW, Chang D, Kiat H, Razmovski-Naumovski V, Chan K, et al. Synergistic effects of Chinese herbal medicine: a comprehensive review of methodology and current research. Front Pharmacol. (2016) 7:201. doi: 10.3389/fphar.2016.00201
19. Zhao Q, Luan X, Zheng M, Tian XH, Zhao J, Zhang WD, et al. Synergistic mechanisms of constituents in herbal extracts during intestinal absorption: focus on natural occurring nanoparticles. Pharmaceutics. (2020) 12:128. doi: 10.3390/pharmaceutics12020128
20. Qin Y, Wang S, Wen Q, Xia Q, Wang S, Chen G, et al. Interactions between Ephedra sinica and Prunus armeniaca: from stereoselectivity to deamination as a metabolic detoxification mechanism of amygdalin. Front Pharmacol. (2021) 12:744624. doi: 10.3389/fphar.2021.744624
21. Chiu YJ, Lin CH, Lee MC, Hsieh-Li HM, Chen CM, Wu YR, et al. Formulated Chinese medicine Shaoyao Gancao Tang reduces NLRP1 and NLRP3 in Alzheimer's disease cell and mouse models for neuroprotection and cognitive improvement. Aging. (2021) 13:15620–37. doi: 10.18632/aging.203125
22. Chen CM, Chen WL, Hung CT, Lin TH, Lee MC, Chen IC, et al. Shaoyao Gancao Tang (SG-Tang), a formulated Chinese medicine, reduces aggregation and exerts neuroprotection in spinocerebellar ataxia type 17 (SCA17) cell and mouse models. Aging. (2019) 11:986–1007. doi: 10.18632/aging.101804
23. Zhu N, Hou J, Ma G, Liu J. Network pharmacology identifies the mechanisms of action of Shaoyao Gancao decoction in the treatment of osteoarthritis. Med Sci Monit. (2019) 25:6051–73. doi: 10.12659/MSM.915821
24. Dufay S, Worsley A, Monteillier A, Avanzi C, Sy J, Ng TF, et al. Herbal tea extracts inhibit Cytochrome P450 3A4 in vitro. J Pharmacy Pharmacol. (2014) 66:1478–90. doi: 10.1111/jphp.12270
25. Feng D, Tang T, Fan R, Luo J, Cui H, Wang Y, et al. Gancao (Glycyrrhizae Radix) provides the main contribution to Shaoyao-Gancao decoction on enhancements of CYP3A4 and MDR1 expression via pregnane X receptor pathway in vitro. BMC Complement Altern Med. (2018) 18:345. doi: 10.1186/s12906-018-2402-7
26. Lau D, Plotkin BJ. Antimicrobial and biofilm effects of herbs used in traditional chinese medicine. Nat Product Commun. (2013) 8:1617–20. doi: 10.1177/1934578X1300801129
27. Deng J, Fan C, Yang Y. Identification and determination of the major constituents in Deng's herbal tea granules by rapid resolution liquid chromatography coupled with mass spectrometry. J Pharm Biomed Anal. (2011) 56:928–36. doi: 10.1016/j.jpba.2011.08.005
28. Liu Z, Xie HL, Chen L, Huang JH. An improved weighted partial least squares method coupled with near infrared spectroscopy for rapid determination of multiple components and anti-oxidant activity of Pu-Erh tea. Molecules. (2018) 23:1058. doi: 10.3390/molecules23051058
29. Liu B, Piao X, Niu W, Zhang Q, Ma C, Wu T, et al. Kuijieyuan decoction improved intestinal barrier injury of ulcerative colitis by affecting TLR4-dependent PI3K/AKT/NF-kappaB oxidative and inflammatory signaling and gut microbiota. Front Pharmacol. (2020) 11:1036. doi: 10.3389/fphar.2020.01036
30. Maupetit A, Larbat R, Pernaci M, Andrieux A, Guinet C, Boutigny AL, et al. Defense compounds rather than nutrient availability shape aggressiveness trait variation along a leaf maturity gradient in a biotrophic plant pathogen. Front Plant Sci. (2018) 9:1396. doi: 10.3389/fpls.2018.01396
31. Xiang Q, Hu S, Ligaba-Osena A, Yang J, Tong F, Guo W. Seasonal variation in transcriptomic profiling of Tetrastigma hemsleyanum fully developed tuberous roots enriches candidate genes in essential metabolic pathways and phytohormone signaling. Front Plant Sci. (2021) 12:659645. doi: 10.3389/fpls.2021.659645
32. Pedersen KS, Toft N. Intra- and inter-observer agreement when using a descriptive classification scale for clinical assessment of faecal consistency in growing pigs. Prev Vet Med. (2011) 98:288–91. doi: 10.1016/j.prevetmed.2010.11.016
33. Ahrens U. Detection of DNA of plant pathogenic mycoplasmalike organisms by a polymerase chain reaction that amplifies a sequence of the 16S rRNA gene. Phytopathology. (1992) 82:828–32. doi: 10.1094/Phyto-82-828
34. Li M, Shao D, Zhou J, Gu J, Qin J, Chen W, et al. Signatures within esophageal microbiota with progression of esophageal squamous cell carcinoma. Chin J Cancer Res. (2020) 32:755–67. doi: 10.21147/j.issn.1000-9604.2020.06.09
35. Li FS, Weng JK. Demystifying traditional herbal medicine with modern approach. Nat Plants. (2017) 3:17109. doi: 10.1038/nplants.2017.109
36. Abdallah A, Zhang P, Zhong Q, Sun Z. Application of traditional Chinese herbal medicine by-products as dietary feed supplements and antibiotic replacements in animal production. Curr Drug Metabolism. (2019) 20:54–64. doi: 10.2174/1389200219666180523102920
37. Lin ZN, Ye L, Li ZW, Huang XS, Lu Z, Yang YQ, et al. Chinese herb feed additives improved the growth performance, meat quality, and nutrient digestibility parameters of pigs. Anim Model Exp Med. (2020) 3:47–54. doi: 10.1002/ame2.12104
38. Cho IJ, Kim SE, Choi BR, Park HR, Park JE, Hong SH, et al. Lemon balm and corn silk extracts mitigate high-fat diet-induced obesity in mice. Antioxidants. (2021) 10:2015. doi: 10.3390/antiox10122015
39. Kuralkar P, Kuralkar SV. Role of herbal products in animal production - an updated review. J Ethnopharmacol. (2021) 278:114246. doi: 10.1016/j.jep.2021.114246
40. Upadhaya SD, Kim IH. The impact of weaning stress on gut health and the mechanistic aspects of several feed additives contributing to improved gut health function in weanling piglets-A review. Animals. (2021) 11:2418. doi: 10.3390/ani11082418
41. Martinez G, Dieguez SN, Fernandez Paggi MB, Riccio MB, Perez Gaudio DS, Rodriguez E, et al. Effect of fosfomycin, Cynara scolymus extract, deoxynivalenol and their combinations on intestinal health of weaned piglets. Anim Nutr. (2019) 5:386–95. doi: 10.1016/j.aninu.2019.08.001
42. Li Y, Sun T, Hong Y, Qiao T, Wang Y, Li W, et al. Mixture of five fermented herbs (Zhihuasi Tk) alters the intestinal microbiota and promotes the growth performance in piglets. Front Microbiol. (2021) 12:725196. doi: 10.3389/fmicb.2021.725196
43. Wenk C. Herbs and botanicals as feed additives in monogastric animals. Asian Austr J Anim Sci. (2003) 16:282–89. doi: 10.5713/ajas.2003.282
44. Bandyopadhyay P, Ghosh AK, Ghosh C. Recent developments on polyphenol-protein interactions: effects on tea and coffee taste, antioxidant properties and the digestive system. Food Funct. (2012) 3:592–605. doi: 10.1039/c2fo00006g
45. Rolnik A, Olas B. The plants of the asteraceae family as agents in the protection of human health. Int J Mol Sci. (2021) 22:3009. doi: 10.3390/ijms22063009
46. Bontempo V, Jiang XR, Cheli F, Lo Verso L, Mantovani G, Vitari F, et al. Administration of a novel plant extract product via drinking water to post-weaning piglets: effects on performance and gut health. Animal. (2014) 8:721–30. doi: 10.1017/S175173111400041X
47. Yan L, Zhang ZF, Park JC, Kim IH. Evaluation of Houttuynia cordata and Taraxacum officinale on growth performance, nutrient digestibility, blood characteristics, and fecal microbial shedding in diet for weaning pigs. Asian Australas J Anim Sci. (2012) 25:1439–44. doi: 10.5713/ajas.2012.12215
48. Chen L, Xu Y, Chen X, Fang C, Zhao L, Chen F. The maturing development of gut microbiota in commercial piglets during the weaning transition. Front Microbiol. (2017) 8:1688. doi: 10.3389/fmicb.2017.01688
49. Verhelst R, Schroyen M, Buys N, Niewold T. Dietary polyphenols reduce diarrhea in enterotoxigenic Escherichia coli (ETEC) infected post-weaning piglets. Livestock Sci. (2014) 160:138–40. doi: 10.1016/j.livsci.2013.11.026
50. Li J, Cui H, Cai Y, Lin J, Song X, Zhou Z, et al. Tong-Xie-Yao-Fang regulates 5-HT level in diarrhea predominant irritable bowel syndrome through gut microbiota modulation. Front Pharmacol. (2018) 9:1110. doi: 10.3389/fphar.2018.01110
51. Chen J, Mao Y, Xing C, Hu R, Xu Z, Cao H, et al. Traditional Chinese medicine prescriptions decrease diarrhea rate by relieving colonic inflammation and ameliorating caecum microbiota in piglets. Evid Based Complement Alternat Med. (2020) 2020:3647525. doi: 10.1155/2020/3647525
52. Cao ST, Wang CC, Wu H, Zhang QH, Jiao LF, Hu CH. Weaning disrupts intestinal antioxidant status, impairs intestinal barrier and mitochondrial function, and triggers mitophagy in piglets. J Anim Sci. (2018) 96:1073–83. doi: 10.1093/jas/skx062
53. Novais AK, Deschene K, Martel-Kennes Y, Roy C, Laforest JP, Lessard M, et al. Weaning differentially affects mitochondrial function, oxidative stress, inflammation and apoptosis in normal and low birth weight piglets. PLoS ONE. (2021) 16:e0247188. doi: 10.1371/journal.pone.0247188
54. Pardo Z, Fernandez-Figares I, Lachica M, Lara L, Nieto R, Seiquer I. Impact of heat stress on meat quality and antioxidant markers in Iberian pigs. Antioxidants. (2021) 10:1911. doi: 10.3390/antiox10121911
55. Bednarz-Misa I, Berdowska I, Zboch M, Misiak B, Zielinski B, Placzkowska S, et al. Paraoxonase 1 decline and lipid peroxidation rise reflect a degree of brain atrophy and vascular impairment in dementia. Adv Clin Exp Med. (2020) 29:71–78. doi: 10.17219/acem/111377
56. Liang X, Yamazaki K, Kamruzzaman M, Bi X, Panthee A, Sano H. Effects of Chinese herbal medicine on plasma glucose, protein and energy metabolism in sheep. J Anim Sci Biotechnol. (2013) 4:51. doi: 10.1186/2049-1891-4-51
57. Huang P, Wang P, Xu J, Sun M, Liu X, Lin Q, et al. Fermented traditional Chinese medicine alters the intestinal microbiota composition of broiler chickens. Res Vet Sci. (2021) 135:8–14. doi: 10.1016/j.rvsc.2020.12.021
58. Jiang XR, Zhang HJ, Mantovani G, Alborali GL, Caputo JM, Savoini G, et al. The effect of plant polyphenols on the antioxidant defence system of weaned piglets subjected to an Escherichia coli challenge. J Anim Feed Sci. (2014) 23:324–30. doi: 10.22358/jafs/65668/2014
59. Noor AS, Kadhim AH, Ali MA. The effect of feeding different levels of dandelion leaf powder (Taraxacum officinale) in the diet on the productive and physiological performance of broiler chickens, strain Ross-308. IOP Conf Series Earth Environ Sci. (2021) 722:012002. doi: 10.1088/1755-1315/722/1/012002
60. Ting Y, Yong TJ, Gang J, Mang LG, Gang T, Ling CX, et al. Effects of Dietary Licorice Extract on Serum Biochemical Index, Tissues Antioxidant Capacity and Immunity Function of Weaned Piglets. Chengdu: Research Square (2020).
61. Yuan J, Lu Y, Wang H, Feng Y, Jiang S, Gao XH, et al. Paeoniflorin resists H2O2-induced oxidative stress in melanocytes by JNK/Nrf2/HO-1 pathway. Front Pharmacol. (2020) 11:536. doi: 10.3389/fphar.2020.00536
62. Su Y, Li X, Li D, Sun J. Fecal microbiota transplantation shows marked shifts in the multi-omic profiles of porcine post-weaning diarrhea. Front Microbiol. (2021) 12:619460. doi: 10.3389/fmicb.2021.619460
63. Khan SR, van der Burgh AC, Peeters RP, van Hagen PM, Dalm V, Chaker L. Determinants of serum immunoglobulin levels: a systematic review and meta-analysis. Front Immunol. (2021) 12:664526. doi: 10.3389/fimmu.2021.664526
64. Wang K, Cao G, Zhang H, Li Q, Yang C. Effects of Clostridium butyricum and Enterococcus faecalis on growth performance, immune function, intestinal morphology, volatile fatty acids, and intestinal flora in a piglet model. Food Funct. (2019) 10:7844–54. doi: 10.1039/C9FO01650C
65. Xu P, Cui L, Huang T, Zhang Z, Yang B, Chen C, et al. Genome-wide identification of quantitative trait transcripts for blood traits in the liver samples of a White Duroc x Erhualian F2 pig resource population. Physiol Genomics. (2016) 48:573–9. doi: 10.1152/physiolgenomics.00123.2015
66. Chen Z, Huo X, Zhang S, Cheng Z, Huang Y, Xu X. Relations of blood lead levels to echocardiographic left ventricular structure and function in preschool children. Chemosphere. (2021) 268:128793. doi: 10.1016/j.chemosphere.2020.128793
67. Mok AC, Mody CH, Li SS. Immune cell degranulation in fungal host defence. J Fungi. (2021) 7:484. doi: 10.3390/jof7060484
68. Yan C, Wang K, Chen L, He YM, Tang ZX. Effects of feeding an herbal preparation to sows on immunological performance of offspring. J Anim Sci. (2012) 90:3778–82. doi: 10.2527/jas.2011-4946
69. Wang J, Li G, Zhong W, Zhang H, Yang Q, Chen L, et al. Effect of dietary paeoniae radix alba extract on the growth performance, nutrient digestibility and metabolism, serum biochemistry, and small intestine histomorphology of raccoon dog during the growing period. Front Vet Sci. (2022) 9:839450. doi: 10.3389/fvets.2022.839450
70. Sabbadin C, Bordin L, Dona G, Manso J, Avruscio G, Armanini D. Licorice: from pseudohyperaldosteronism to therapeutic uses. Front Endocrinol. (2019) 10:484. doi: 10.3389/fendo.2019.00484
71. Wang L, Li L, Gao J, Huang J, Yang Y, Xu Y, et al. Characterization, antioxidant and immunomodulatory effects of selenized polysaccharides from dandelion roots. Carbohydr Polym. (2021) 260:117796. doi: 10.1016/j.carbpol.2021.117796
72. Wang S, Li Z, Ma Y, Liu Y, Lin CC, Li S, et al. Immunomodulatory effects of green tea polyphenols. Molecules. (2021) 26. doi: 10.3390/molecules26123755
73. Chen L, Hu C, Hood M, Kan J, Gan X, Zhang X, et al. An integrated approach exploring the synergistic mechanism of herbal pairs in a botanical dietary supplement: a case study of a liver protection health food. Int J Genom. (2020) 2020:9054192. doi: 10.1155/2020/9054192
74. Sealock JM, Lee YH, Moscati A, Venkatesh S, Voloudakis G, Straub P, et al. Use of the PsycheMERGE network to investigate the association between depression polygenic scores and white blood cell count. JAMA Psychiatry. (2021) 78:1365–74. doi: 10.1001/jamapsychiatry.2021.2959
75. Lakschevitz FS, Hassanpour S, Rubin A, Fine N, Sun C, Glogauer M. Identification of neutrophil surface marker changes in health and inflammation using high-throughput screening flow cytometry. Exp Cell Res. (2016) 342:200–9. doi: 10.1016/j.yexcr.2016.03.007
76. Sampath P, Moideen K, Ranganathan UD, Bethunaickan R. Monocyte subsets: phenotypes and function in tuberculosis infection. Front Immunol. (2018) 9:1726. doi: 10.3389/fimmu.2018.01726
77. Md. Elias H. Dietary supplementation of green tea by-products on growth performance, meat quality, blood parameters and immunity in finishing pigs. J Med Plants Res. (2012) 6:2458–67. doi: 10.5897/JMPR11.1643
78. Antileo R, Figueroa J, Valenzuela C. Characterization of a novel encapsulated oral iron supplement to prevent iron deficiency anemia in neonatal piglets. J Anim Sci. (2016) 94:157–60. doi: 10.2527/jas.2015-9698
79. Vital M, Gao J, Rizzo M, Harrison T, Tiedje JM. Diet is a major factor governing the fecal butyrate-producing community structure across Mammalia, Aves and Reptilia. ISME J. (2015) 9:832–43. doi: 10.1038/ismej.2014.179
80. Geva-Zatorsky N, Sefik E, Kua L, Pasman L, Tan TG, Ortiz-Lopez A, et al. Mining the human gut microbiota for immunomodulatory organisms. Cell. (2017) 168:928–43.e11. doi: 10.1016/j.cell.2017.01.022
81. Miller GE, Engen PA, Gillevet PM, Shaikh M, Sikaroodi M, Forsyth CB, et al. Lower neighborhood socioeconomic status associated with reduced diversity of the colonic microbiota in healthy adults. PLoS ONE. (2016) 11:e0148952. doi: 10.1371/journal.pone.0148952
82. Isaacson R, Kim HB. The intestinal microbiome of the pig. Anim Health Res Rev. (2012) 13:100–9. doi: 10.1017/S1466252312000084
83. Turnbaugh PJ, Ley RE, Mahowald MA, Magrini V, Mardis ER, Gordon JI. An obesity-associated gut microbiome with increased capacity for energy harvest. Nature. (2006) 444:1027–31. doi: 10.1038/nature05414
84. Magne F, Gotteland M, Gauthier L, Zazueta A, Pesoa S, Navarrete P, et al. The firmicutes/bacteroidetes ratio: a relevant marker of gut dysbiosis in obese patients? Nutrients. (2020) 12:1474. doi: 10.3390/nu12051474
85. Turnbaugh PJ, Backhed F, Fulton L, Gordon JI. Diet-induced obesity is linked to marked but reversible alterations in the mouse distal gut microbiome. Cell Host Microbe. (2008) 3:213–23. doi: 10.1016/j.chom.2008.02.015
86. Wang H, Ni X, Qing X, Zeng D, Luo M, Liu L, et al. Live probiotic Lactobacillus johnsonii BS15 promotes growth performance and lowers fat deposition by improving lipid metabolism, intestinal development, and gut microflora in broilers. Front Microbiol. (2017) 8:1073. doi: 10.3389/fmicb.2017.01073
87. Xu B, Qin W, Xu Y, Yang W, Chen Y, Huang J, et al. Dietary quercetin supplementation attenuates diarrhea and intestinal damage by regulating gut microbiota in weanling piglets. Oxid Med Cell Longev. (2021) 2021:6221012. doi: 10.1155/2021/6221012
88. Hevia A, Delgado S, Sanchez B, Margolles A. Molecular players involved in the interaction between beneficial bacteria and the immune system. Front Microbiol. (2015) 6:1285. doi: 10.3389/fmicb.2015.01285
89. Wang J, Tang H, Zhang C, Zhao Y, Derrien M, Rocher E, et al. Modulation of gut microbiota during probiotic-mediated attenuation of metabolic syndrome in high fat diet-fed mice. ISME J. (2015) 9:1–15. doi: 10.1038/ismej.2014.99
90. Chen S, Niu H, Wu Y, Sun J, Han X, Zhang L. Influence of lactic acid on cell cycle progressions in Lactobacillus bulgaricus during batch culture. Appl Biochem Biotechnol. (2021) 193:912–24. doi: 10.1007/s12010-020-03459-8
91. Tankou SK, Regev K, Healy BC, Tjon E, Laghi L, Cox LM, et al. A probiotic modulates the microbiome and immunity in multiple sclerosis. Ann Neurol. (2018) 83:1147–61. doi: 10.1002/ana.25244
92. Luo ZC, Delvin E, Fraser WD, Audibert F, Deal CI, Julien P, et al. Maternal glucose tolerance in pregnancy affects fetal insulin sensitivity. Diabetes Care. (2010) 33:2055–61. doi: 10.2337/dc10-0819
93. Min BR, Gurung N, Shange R, Solaiman S. Potential role of rumen microbiota in altering average daily gain and feed efficiency in meat goats fed simple and mixed pastures using bacterial tag-encoded FLX amplicon pyrosequencing1. J Anim Sci. (2019) 97:3523–34. doi: 10.1093/jas/skz193
94. Yan S, Tu Z, Liu Z, Fan N, Yang H, Yang S, et al. A huntingtin knockin pig model recapitulates features of selective neurodegeneration in Huntington's disease. Cell. (2018) 173:989–1002 e13. doi: 10.1016/j.cell.2018.03.005
95. Sookoian S, Salatino A, Castano GO, Landa MS, Fijalkowky C, Garaycoechea M, et al. Intrahepatic bacterial metataxonomic signature in non-alcoholic fatty liver disease. Gut. (2020) 69:1483–91. doi: 10.1136/gutjnl-2019-318811
Keywords: weaned pigs, herbal mixture, growth performance, antioxidant capacity, gut microbiota
Citation: Xu Q, Cheng M, Jiang R, Zhao X, Zhu J, Liu M, Chao X, Zhang C and Zhou B (2022) Effects of dietary supplement with a Chinese herbal mixture on growth performance, antioxidant capacity, and gut microbiota in weaned pigs. Front. Vet. Sci. 9:971647. doi: 10.3389/fvets.2022.971647
Received: 17 June 2022; Accepted: 03 August 2022;
Published: 22 August 2022.
Edited by:
Asghar Kamboh, Sindh Agriculture University, PakistanReviewed by:
Liang Chen, Institute of Animal Sciences (CAAS), ChinaDandan Han, China Agricultural University, China
Copyright © 2022 Xu, Cheng, Jiang, Zhao, Zhu, Liu, Chao, Zhang and Zhou. This is an open-access article distributed under the terms of the Creative Commons Attribution License (CC BY). The use, distribution or reproduction in other forums is permitted, provided the original author(s) and the copyright owner(s) are credited and that the original publication in this journal is cited, in accordance with accepted academic practice. No use, distribution or reproduction is permitted which does not comply with these terms.
*Correspondence: Bo Zhou, emhvdWJvJiN4MDAwNDA7bmphdS5lZHUuY24=
†These authors have contributed equally to this work