- 1Departamento de Medicina Preventiva Animal, Facultad de Ciencias Veterinarias y Pecuarias, Universidad de Chile, Santiago, Chile
- 2Magister en Ciencias Animales y Veterinarias, Facultad de Ciencias Veterinarias y Pecuarias, Universidad de Chile, Santiago, Chile
- 3Departamento de Ciencias Biológicas, Facultad de Ciencias Veterinarias y Pecuarias, Universidad de Chile, Santiago, Chile
- 4Escuela de Medicina Veterinaria, Facultad de Ciencias de la Vida, Universidad Andrés Bello, Santiago, Chile
Salmonella enterica is a food-borne pathogen with a wide host-range that during decades has been of public health concern in developed and developing countries. In Chile, the poultry and pig industries represent the biggest contribution of meat consumption in the population, and sanitary regulations have been imposed for Salmonella control. The aim of this work was to determine and characterize Salmonella strains isolated from pigs and chicken raised on commercials farms in Chile. For this, isolates belonging to pigs (n = 46) and poultry (n = 57) were genotyped by two multiplex PCR reactions and virulotyped by the PCR detection of virulence-associated genes. In addition, isolates were serotyped and analyzed by the Kirby Bauer assay to determine their antimicrobial resistance phenotypes. From these analyses 52 genotypes, six serotypes and several multidrug resistance phenotypes and different combinations of virulence-associated genes were detected. These results suggest that S. enterica in pigs and poultry in central Chile should be monitored due to potential consequences in public and animal health.
Introduction
Salmonella enterica is a foodborne pathogen that can cause enterocolitis with diarrhea, fever and abdominal pain in humans, as major clinical manifestations (1). Typically, S. enterica causes a self-limited digestive disease, although extra-intestinal infections of medical importance have also been reported, especially in patients of the high-risk group (2–4). Thus, the severity of human salmonellosis varies according to the serotype involved and the health status of the host (4, 5).
The S. enterica subsp. enterica has multiple reservoirs in domestic animals and wildlife, and its transmission to humans is usually by consumption of contaminated foodstuff, such as eggs or meat (6, 7). Colonized chickens and pigs, as well as contaminated products derived from those animals, are major sources of human salmonellosis in most countries. Thus, it is important to investigate and survey the epidemiology of this pathogen in those animal species for public health and poultry and pig husbandry (8). In a high proportion of cases, the meat is contaminated with feces during slaughter or dressing line, which constitutes the main risk factor for Salmonella to enter into the food chain (9). However, the food chain is not the only transmission pathway, being the direct animal-human contact a route that has been increasingly reported worldwide (10–12).
The expression of several virulence genes during the host-pathogen interaction allows the activation of adhesion and invasion mechanisms which determines the course of infection. These factors include flagella, capsule, plasmids, adhesins, and type 3 secretion systems (T3SS), among others. These virulence factors could enhance the adaptive capacity of some serotypes, representing Salmonella pathogenic mechanisms to infect, survive and establish the infection in the host (13, 14).
An additional factor of current concern is the emergence of antimicrobial resistance (AMR) in different S. enterica serotypes. In general, self-limited salmonellosis does not require antibiotic treatment; however, in patients with the invasive disease, it may be necessary to use antibiotics, especially cephalosporins and fluoroquinolones. Thus, AMR represent a risk for patients and for public health because therapies are less effective and the genes encoding resistance are positively selected and disseminated through mobilizable elements between bacterial strains of self and different species and sources (15, 16).
There are several zoonotic Salmonella serotypes that have a wide host range and cause infection and disease in humans, wildlife and domestic animals (4, 5). Thus, reporting the Salmonella serotypes involved in an outbreak turns essential for conducting an outbreak investigation and epidemiological surveillance (17). The traditional method of serotyping Salmonella isolates follow the Kauffmann-White scheme, based on the typification by serological discrimination, through the agglutination of somatic (O) and flagellar (H) antigens, and the capsular Vi antigen; describing more than 2,650 serotypes with diverse epidemiology and geographic distribution (18, 19). Depending on the serotype, this method could be slow, laborious and costly, which justifies the study of other faster and cost-effective S. enterica typing methodologies, such as the multiplex PCR (20, 21).
In South America, there is limited surveillance data on non-typhoidal Salmonella strains in food producing animals. Therefore, the objective of this study was to identify S. enterica serotypes in pigs and chickens from commercials farms located in central Chile and to characterize their AMR phenotypes, serotypes and virulotypes in order to provide relevant data for surveillance programs at the national and international level.
Materials and methods
Sample collection
In Chile, the national industrialized production of poultry and swine is concentrated in the central zone, specifically in three regions: Metropolitan Region, Libertador Bernardo O'Higgins Region and Maule Region, concentrating 79% of the national production (22, 23). A total of 500 stool samples from five pig farms and 300 stool samples from six poultry farms were individually collected during 2017 in central Chile. Animals in the final stage of production just before slaughter were sampled, by using sterile swabs with Cary Blair transport medium (Copan Diagnostics, Murrieta, CA, USA). Sampling was performed by personnel working on the same farms during a period of 12 months. After collection, all samples (n = 800) were immediately refrigerated and transported to the laboratory.
Sample processing
In order to isolate Salmonella strains, samples were processed as previously reported (24). Briefly, swabs were placed into 5 mL of buffered peptone water (BPW, Beckton Dickinson, Franklin Lakes, NJ, USA) supplemented with 20 μg/mL of novobiocin (Sigma, St. Louis, MO, USA), and incubated for 24 h at 37°C. Then, 100 μL of the suspension were inoculated into modified semisolid Rappaport Vassiliadis basal medium (Oxoid, Basingstoke, UK) plates supplemented with 20 μg/mL of novobiocin and incubated for 24 or 48 h at 41°C. Cultures with bacterial growth were replated into xylose lysine deoxycholate agar (XLD, Beckton Dickinson, Franklin Lakes, NJ, USA) plates and incubated for 24 h at 37°C. Two suspicious colonies were initially subjected to traditional morphological and biochemical testing including Gram staining, and the use of triple sugar iron agar slopes and API 20E strips (bioMérieux, Marcy l'Etoile, France). Subsequently, if both colonies were Salmonella, only one of them was randomly selected for further analysis. From one colony, the genomic DNA was extracted using a commercial kit (Thermo Scientific, Waltham, MA, USA), following manufacturer's instructions. Concentration and quality (260/280 absorbance ratio) of the obtained extracted DNA was measured in a NANO-400 micro-spectrophotometer (Hangzhou Allsheng Instruments Co., Hangzhou, China). Samples with an absorbance ratio closest to the optimal range (1.8–2.0) (25) were kept at −20°C for further analyses.
Molecular typing of S. enterica serovars
All identified Salmonella strains were subjected to a multiplex PCR protocol in order to identify genotypes (17, 20, 21). Each combination of amplified sequences was considered a genotype. Briefly, the method consists of two five-plex PCR reactions and one two-plex PCR reaction. The primers for each of the reactions and accession numbers for each of the gene are listed in Supplementary Table S1. The amplified regions of the genome mostly correspond to prophages and fimbrial clusters that are remarkably variable between serovars. These regions are potentially of great interest as they represent gene clusters that may have a serovar-specific association (17, 26–28). Reaction mixtures and amplification conditions were performed under standard conditions (21). Thus, the protocol used to perform the amplifications included a total volume of 50 μL containing 2U Taq Polymerase (Invitrogen®, ON, Canada), 2X Taq buffer (5 mM KCl Tris-HCl, pH 8.5), 1.5 mM MgCl2, 0.1 mM dNTPs (Promega®, Madison, WI, USA), and 5.0 mM of each primer (Promega®, Madison, WI, USA), and 2 μL of DNA. The amplification parameters included a first incubation for 5 min at 94°C, followed by 40 amplification cycles that consisted of a 30 s denaturing step at at 60°C (reactions 1 and 2) or 65°C (reaction 3), and a 1 min elongation step at 72°C, and a final extension step of 5 min. The gels were stained with Gel Red (Invitrogen®, Carlsbad, CA, USA), the amplicons were resolved by 5% agarose gel electrophoresis at 120 V for 1 h, and band visualization was carried out with a UV-transilluminator (Vilber Lourmat, Collegien, France). The concentration of agarose used was suitable for the expected band sizes. The stained gel was captured on a desktop computer using the Infinity® software (Tallahassee, FL, USA). Two control strains were used, S. Typhimurium 14,028 strain and S. Typhi STH 2,370 strain. Nuclease-free water was used as a negative control. For each serotype, the results of the multiplex PCR were analyzed through the construction of a binary matrix using “1” for presence and “0” for absence of amplified bands from each isolate. This matrix was used to calculate the similarity of the isolates by a hierarchical clustering, using the average linkage method and Jaccard's distance, and was represented by a dendrogram. This analysis was carried out using the Infostat software (29).
To determine the diversity of genotypes for each bacterial serotype, the Gini coefficient was calculated, which values range between “0” and “1”. In this coefficient, “0” suggests an equal distribution of isolates across all genotypes, and “1” represents one genotype as the unique source for all isolates (30).
Serotyping
Once bacteria were genotyped by the multiplex PCR, one isolate representative of each genotype was sent to the national reference laboratory Instituto de Salud Pública de Chile (ISP), where they were serotyped following the Kauffman-White scheme (18).
Detection of virulence genes
All isolated S. enterica strains were subjected to PCR for detection of seven virulence genes, including spvC, pagK, sirA, gipA, prot6e, SEN1417 and pefA (31–33). The PCR reactions were performed with primers described in Supplementary Table S2, in a total volume of 25 μL containing 1U Taq Polymerase (Invitrogen®, ON, Canada), 1X Taq buffer (5 mM KCl Tris-HCl, pH 8.5), 1.5 mM MgCl2, 0.1 mM dNTPs (Promega®, Madison, WI, USA), 1 μM of forward and reverse primers (Promega®, Madison, WI, USA), and 1 μL of DNA. An incubation at 95°C for 10 min was used as an initial denaturation step followed by 35 cycles of amplification. Each cycle consisted of a denaturation step at 95°C for 1 min, followed by 1 min of annealing at different temperatures according to the target gene and elongation at 72°C for 1 min. The final elongation step was conducted at 72°C for 10 min. Previously sequenced strains harboring the target genes were used as controls (9). Supplementary Table S2 describes the annealing temperatures of each virulence gene and the GenBank accession number. A Salmonella strain that was negative for all virulence genes was used as a negative control. The amplicons were resolved by agarose gel electrophoresis (1.5 or 2.0% agarose) at 120 V for 1 h, and band visualization was carried out with Gel Red stain (Invitrogen®, Carlsbad, CA, USA) and a UV-transilluminator (Vilber Lourmat, Collegien, France) using the Infinity® software (Tallahassee, FL, USA).
Phenotypic antimicrobial susceptibility testing
All isolated S. enterica strains were analyzed by the Kirby Bauer technique, according to the recommendations of the Clinical and Laboratory Standards Institute (34). The Escherichia coli ATCC 25,922 and Klebsiella pneumoniae ATCC 700,603 strains were used as quality controls. The bacterial inoculum was standardized to 0.5 McFarland units using a nephelometer. The antibiotics analyzed included ampicillin (AMP, 10 μg), amoxicillin + clavulanic acid (AMC, 20/10 μg), cefadroxil (CFR, 30 μg), ceftazidime (CAZ, 30 μg), ceftiofur (EFT, 30 μg), ceftriaxone (CRO, 30 μg), ciprofloxacin (CIP, 5 μg), gentamicin (CN, 10 μg), nalidixic acid (NA, 30 μg), sulfamethoxazole + trimethoprim (SXT, 23.75/1.25 μg), tetracycline (TE, 30 μg), streptomycin (S, 10 μg), azithromycin (AZM, 15 μg), enrofloxacin (ENR, 5 μg), trimethoprim (W, 5 μg), sulfisoxazole (SF, 300 μg), chloramphenicol (C, 30 μg), and fosfomycin (FOS, 200 μg), using commercial disks (Oxoid®, UK). Multidrug resistance (MDR) was defined as the resistance to three or more antimicrobial classes (35). Strains were analyzed for critically important antimicrobials, as defined by the World Health Organization (WHO) (36). For enrofloxacin, ciprofloxacin breakpoints were used; and the values for azithromycin were analyzed based on Parry et al. (37) and Martínez-Cortés et al. (38). The resistant isolates with an inhibition diameter less than or equal to the breakpoints for cefotaxime, ceftazidime, or ceftiofur were also examined to identify extended spectrum β-lactamses (ESBL) production, using the phenotypic confirmatory test with a cefotaxime + clavulanic acid disk (30/10 μg) or a ceftazidime + clavulanic acid disk (30/10 μg) (34). Intermediate strains were classified as resistant (39). The multiple antimicrobial resistance (MAR) index was calculated as “a/b”, where “a” corresponds to the number of antimicrobials for a particular isolate was resistant and “b” the total number of antimicrobials tested (40).
Statistical analysis
Categorical data analyses were made through contingency tables with Infostat (2010v) software (29).
Results
Isolation and serotyping of S. enterica
From the 500 fecal samples collected from pigs, S. enterica strains were isolated in 9.2% of the sampled animals (n = 46). Among those strains, most corresponded to S. Typhimurium (60.9%, n = 28), followed by S. Infantis and S. Derby (15.2% each, n = 7), S. enterica group B (6.5%, n = 3), and S. enterica subsp. enterica rough strain (2.2%, n = 1). On the other hand, S. enterica strains were isolated in 19% (n = 57) from the 300 samples obtained from chickens, which corresponded to S. Infantis (56.1%, n = 32), S. Typhimurium (22.8%, n = 13), and S. Enteritidis (21.1%, n = 12) (Table 1).
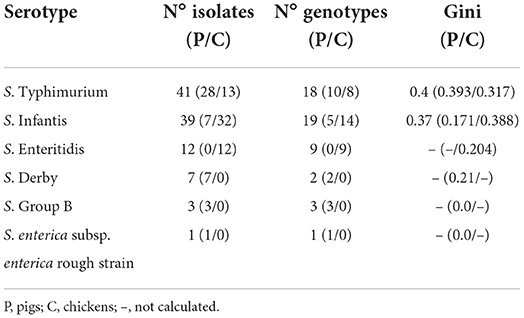
Table 1. Number of isolates, genotypes and Gini coefficients of serotypes detected in pigs (P) and chickens (C).
Typing of S. enterica isolates using the multiplex PCR
The 46 isolates from pigs were typed by PCR and grouped into 21 different genotypes (Table 1 and Supplementary Table S3), and the 57 isolates from chickens were grouped into 31 different genotypes (Table 1 and Supplementary Table S4). Each genotype was always associated to only one serotype, although each serotype grouped several genotypes.
The Gini coefficient varied according to the Salmonella serotype and animal species, ranging from 0.171 to 3.93 (Table 1). When this coefficient is calculated with all isolates by serotype, S. Infantis showed a slightly higher diversity than S. Typhimurium (Gini = 0.37 and 0.4, respectively), grouping 19 and 18 genotypes, respectively (Table 1). Most of them were represented by one or two isolates (Figures 1, 2 and Supplementary Tables S3, S4).
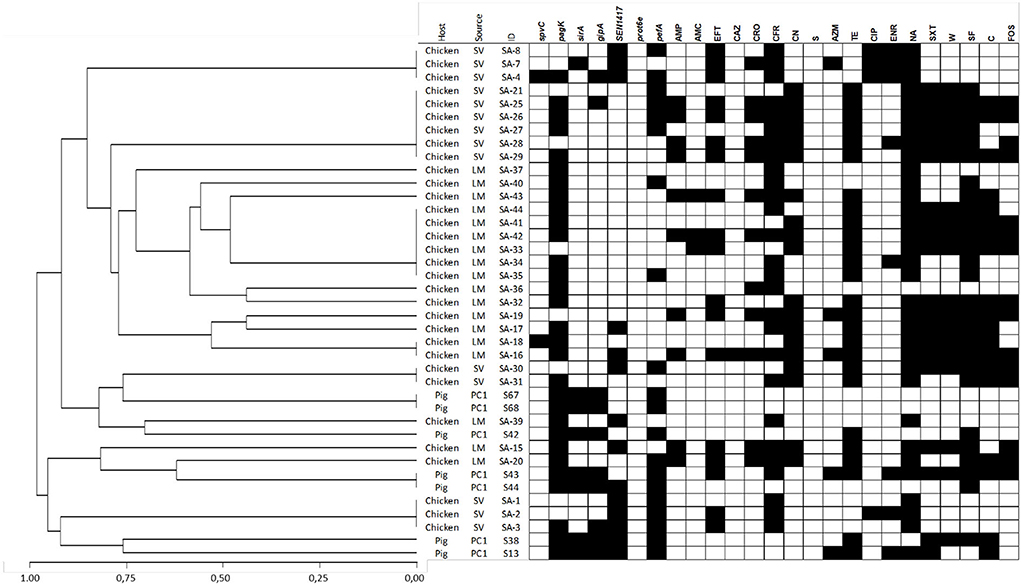
Figure 1. Dendrogram showing genetic similarities (%) among S. Infantis isolates analyzed using multiplex PCR. Detection of virulence-associated genes and antimicrobial resistance phenotypes are depicted as black squares when present. The dendrogram was constructed using a hierarchical clustering, using the average linkage method and Jaccard's distance.
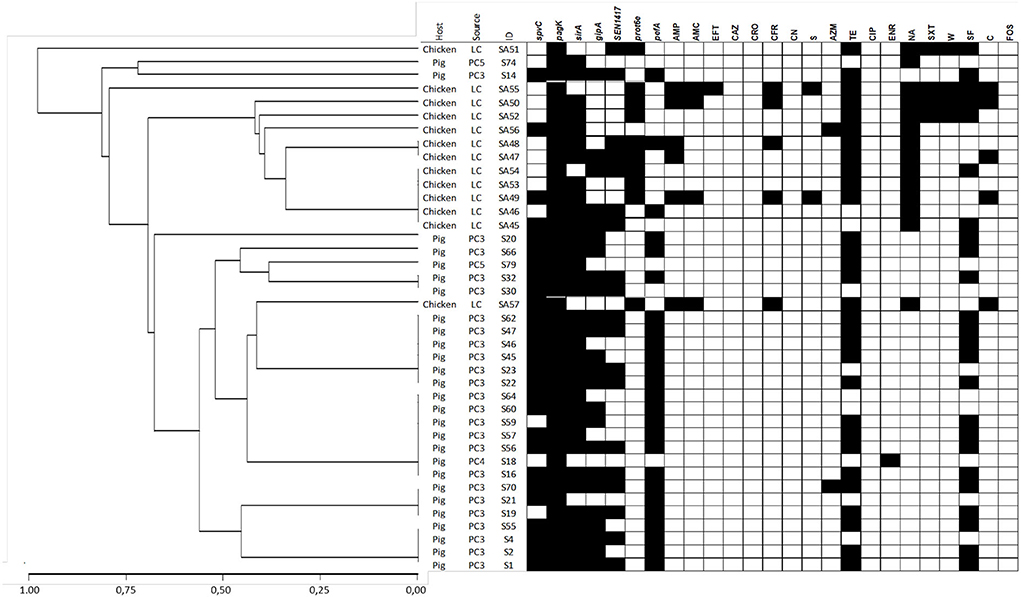
Figure 2. Dendrogram showing genetic similarities (%) among S. Typhimurium isolates analyzed using multiplex PCR. Detection of virulence-associated genes and antimicrobial resistance phenotypes are depicted as black squares when present. The dendrogram was constructed using a hierarchical clustering, using the average linkage method and Jaccard's distance.
Phenotypic antimicrobial resistance characterization of S. enterica strains
Most of the S. enterica strains isolated from pigs were resistant to at least one antibiotic (73.3%), with resistances against tetracycline (53.3%) and sulfisoxazole (51.1%) being the most frequent. Despite 10 phenotypic resistance profiles were detected, only 4 isolates (8.9%) showed MDR (Supplementary Table S5). In contrast, 98.2% of the S. enterica strains isolated from chickens were resistant to at least one drug and 82.5% presented MDR. This high AMR level was mainly observed with nalidixic acid (94.7%), cefadroxil (70.2%) and sulfisoxazole (61.4%) (Figure 3 and Supplementary Table S6), and determined 46 phenotypic resistance profiles (Supplementary Table S6). Within the Salmonella strains resistant to cefotaxime, ceftazidime and ceftiofur isolated from chicken, 32% were ESBL positive; while all strains isolated from pigs were ESBL negative. Furthermore, the highest MAR index value (0.8, a = 14, b = 18) was found in a Salmonella strain isolated from chicken.
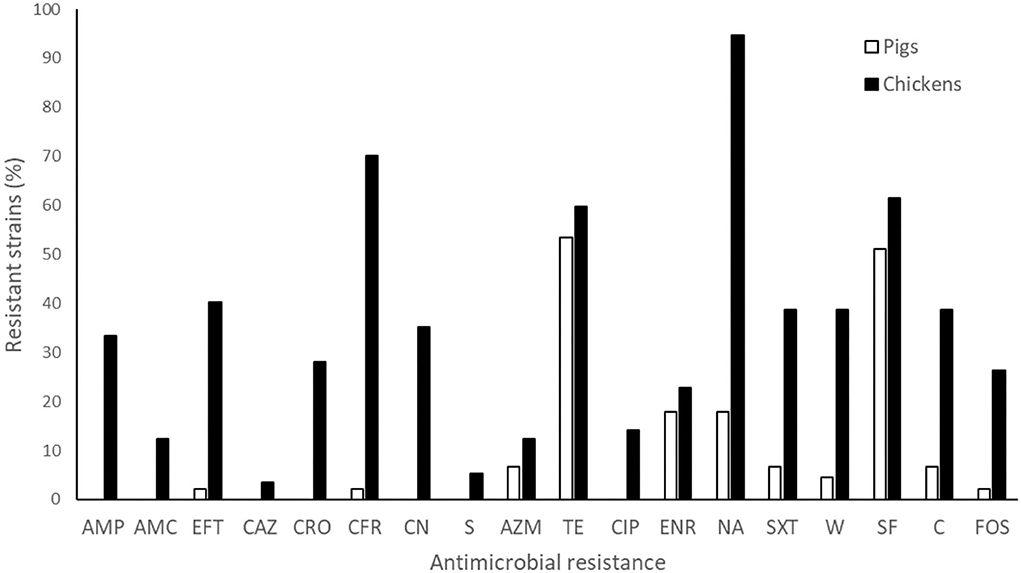
Figure 3. Phenotypic antimicrobial resistance of S. enterica strains isolated from pigs and chickens against 18 classes of antibiotics. AMP, ampicillin; AMC, amoxicillin + clavulanic acid; CFR, cefadroxil; CAZ, ceftazidime; EFT, ceftiofur; CRO, ceftriaxone; CIP, ciprofloxacin; CN, gentamicin; NA, nalidixic acid; SXT, sulfamethoxazole + trimethoprim; TE, tetracycline; S, streptomycin; AZM, azithromycin; ENR, enrofloxacin; W, trimethoprim; SF, sulfisoxazole; C, chloramphenicol; FOS, fosfomycin.
Distribution of virulence genes among S. enterica strains
The most prevalent virulence genes among the pig isolates were pagK and sirA (95.6% each), pefA (80%), gipA (62.2%), spvC (53.3%), and SEN1417 (37.8%). Twelve virulotypes were detected, with spvC-pagK-sirA-gipA-SEN1417-pefA as the most frequent (26.7%) (Supplementary Table S7). No isolates harbored the prot6e gene.
In the case of S. enterica strains isolated from chickens, the genes pagK (68.4%), SEN1417 (42.1%), pefA (28.1%), sirA (26.3%), prot6e (17.5%), gipA (14%), and spvC (12.3%) were detected. In these strains, 25 virulotypes were registered, with pagK alone as more frequently detected (21.1%) (Supplementary Table S8).
Discussion
Understanding the epidemiology of S. enterica in different animal reservoirs is essential to achieve sanitary control of this pathogen and reduce its transmission to humans. Our results show several differences between strains isolated from two food-producing animal species, such as pigs and chickens. First, the rate of S. enterica isolation in feces of pigs was 9% and 19% in chickens. At national level, the isolation rate from pigs is higher than that reported previously by San Martín et al. (41), but lower than that reported by Lapierre et al. (42), with rates of 4.5 and 11.9% respectively. In the case of isolation rates from poultry, and as far as we know, to date there are no published studies. On the other hand, and at international level, our isolation rates differ from those of other authors. In this context, Ishihara et al. (43) reported a prevalence of Salmonella spp. of 2.9% and 36.1% in pigs and broiler chickens, respectively. More recently, Chen et al. (44) in China registered isolation rates of 44 and 9% in pork and chickens, respectively. These differences in detection rates may be due to variations in biosecurity measures for controlling bacterial contamination during the productive cycle of animals and processing of their carcasses. Moreover, the increased use of low-risk and certified feed ingredients in Chile could also contribute to lower residual contamination in animals, facilitating the traceability for detection of other sources of infection. However, differences between studies can also be attributed to specific sample sizes and isolation methods, the local environmental conditions and husbandry practices, among other factors. Regarding methodologies for the isolation of Salmonella from animal feces, the ability of the laboratory method to recover Salmonella from a sample containing a low concentration of this bacterium can be affected by biological factors such as competing microbiota and technical factors such as the culture media used, the volume of the feces and the pre-enrichment of the samples. We performed the isolation based on that described by Jensen et al. (24). These authors propose to add novobiocin to the BPW in the pre-enrichment stage. The authors point out that this method is more sensitive and that it increases the growth of Salmonella in the samples, although they also point out that 13 positive samples were lost. The conclusion of the authors is that the increased growth of Salmonella in novobiocin medium is due to a reduction of competitive microorganisms. So, it is possible that with the addition of novobiocin to BPW, we were not able to isolate Salmonella from all contaminated samples, and probably the actual positivity rate in our samples was higher. However, it is important to note that the animals sampled were healthy animals and therefore could excrete a low concentration of Salmonella, which might not be isolated from a sample with competitive microbiota (45). Thus, there are advantages and disadvantages to the addition of novobiocin in BPW for Salmonella isolation that should be evaluated by laboratories according to their objectives.
On the other hand, the most frequent serotype found in pig farms was S. Typhimurium, while in chicken farms was S. Infantis, in agreement with other reports from United States and the European Union (46–50). In particular, S. Infantis is considered an emerging serotype in the poultry industry across the world, associated with the acquisition of a pESI-like megaplasmid conferring MDR phenotypes (9, 51–54). Furthermore, both S. Infantis and S. Typhimurium have been ranked among the top 10 most frequently Salmonella serotypes involved in human infections worldwide, representing a food safety and public health hazard (55). In Chile, these serotypes occupy the 2nd and 3rd position in frequency, respectively, after S. Enteritidis (56); representing in aggregate around 75% of all clinical cases during last years.
The agglutination techniques for Salmonella serotyping does not have the required discriminative power to describe transmission chains during outbreaks investigations. Therefore, other quick, cost-effective resolution procedures have been studied, such as the multiplex PCR technique. In this work, this assay was performed for genotyping Salmonella isolates, detecting 21 different genotypic patterns in 46 strains isolated from pigs, and 31 distinct genotypic patterns in 57 strains from poultry. This means that genomic polymorphisms harbored by circulating S. enterica serotypes are giving rise to a high genotypic diversity of Salmonella isolates in both hosts, with Gini coefficients lower than 0.4 in all of them (Table 1). Apparently, there are few Salmonella serotypes very well adapted for survival into industrial environments, which infect animals after having been transmitted from various sources. In this context, industry-adapted S. enterica strains show high biofilm production, abiotic stress adaptation and extracellular adhesion; as well as adaptation to their poultry hosts (57–61). This scenario contrasts with the previous results of Kim et al. (17), Beaubrun et al. (20), and Beaubrun et al. (21), which suggested a unique genotype-serotype relationship. The genetic variability observed in our strains might be associated with several contributing factors, such as the origin of the animals and their food (62), the contamination in the premises by direct and/or indirect contact with humans (62), domestic animals or wild birds (63), or the presence of other carriers acting as vehicles of S. enterica strains (64, 65). For this reason, the inclusion of routine testing and categorization of animal herds on its S. enterica infection status are important components of integrated programs focused at reducing its prevalence in the food chain and the risks for animal and public health. Additionally, the observed diversity establishes the need of future analyses to determine the capability of multiplex PCR for supporting studies like that, and its potentiality for complementing the traditional serotyping through its discriminatory ability at a sub-serotype level. In this regard, it is important to highlight that the same genotype was not shared between serotypes, maintaining the specificity of the method. Furthermore, this molecular typing method suggests that pig and chicken industries are not sharing isolates (Figures 1, 2), being affected by independent infection sources which would require specific efforts to characterize Salmonella transmission chains and the implementation of their control methods.
A variety of virulence factors have been shown to play different roles in the pathogenesis of Salmonella infections. To perform virulotyping in Salmonella it is important to choose genes that are not present in 100% of the strains, based on the literature, in order to determine the variability of the isolated strains. Regarding the detection of virulence genes, we found 25 different virulotypes in chicken and 12 different virulotypes in pig. The most detected genes were pagK (68%) and SEN1417 (42.1%). In fact, the pagK gene, as the only amplified gene, was the most frequent virulotype (n = 12; 21%), corresponding to serotypes S. Infantis (n = 10) and S. Enteritidis (n = 2). The high frequency of pagK in pigs (95.6%) and chicken (68.4%) suggests its association with an important function during bacterial colonization of animal hosts, codifying a translocated factor into the cytosol of host cells (66), which also participates in biofilm formation (9).
A striking result is the difference in the number of antibiotic resistant strains in both food animal industries. The strains isolated from pigs showed low AMR levels and no critical drug resistances were detected. On the contrary, S. enterica strains isolated from poultry were much more resistant, including resistance against critical antibiotics, especially to cephalosporins. In Chile, the commercial pig and chicken farms have developed a high technological, biosecurity and sanitary related standards. They are intensive, vertically integrated, with restrictions on the use of antibiotics and homogeneity on their sanitary and productive management. Then, the contrasting findings in the AMR profiles could suggest a serotype effect, especially associated to S. Infantis, or a management effect, related to differences in the antibiotic usage in both type of productions. In this regard, emerging strains of S. Infantis carrying the megaplasmid pESI show a MDR phenotype; and have been described in several countries, including Chile (67–69). It is therefore very likely that the S. Infantis strains, mostly isolated from chickens, contains this megaplasmid and, as a consequence, express a MDR phenotype and develop a higher virulence than non-MDR strains (70). In the case of pigs, four strains were MDR, one of which showed simultaneous resistance against 10 antibiotics. In the case of S. enterica strains isolated from chickens, 33 strains presented a MDR phenotype, with two of them resistant to 10 antibiotics, two to 11 antibiotics, and even two to 12 antibiotics. In this study, a high MAR rate (>0.50) was observed in 9 (15.8%) strains isolated from chickens, indicating that Salmonella strains isolated from chickens express higher levels of multi-resistance compared to Salmonella strains isolated from pigs.
In Chile, despite regulations for the use of antibiotics in food-producing animals have been implemented (71), the most common antimicrobials used in pig or broiler chicken production are unknown. Moreover, ceftiofur, which is a third-generation cephalosporin, is permitted in these species. Consequently, bacteria isolated from chickens expressed resistance against critical antibiotics indicated by the WHO and OIE, such as cephalosporins, especially ceftiofur (39%), and fosfomycin (33%) (36, 72). In this context, Lai et al. (73) in China, also described high (42%) ceftiofur resistance rates in Salmonella strains isolated from chickens, pigs and ducks. The importance of monitoring the resistance to ceftiofur relies on its similar mechanism of action with ceftriaxone, a cephalosporin often used to treat Salmonella infections in children (74). Therefore, the increased rate of ceftiofur resistant Salmonella strains of animal origin may has important public health implications, limiting therapeutic alternatives in human medicine. In South America, there is limited information about AMR associated to food-producing animals. Among the scarce information available, Vinueza-Burgos et al. (75) in Ecuador reported AMR to critical drugs, including 5.8% to ceftazidime, 98% to nalidixic acid, and 94% to ciprofloxacin. In our study, among the Salmonella strains isolated from chickens, we found 15% of enrofloxacin resistant strains, and similarly to Vinueza-Burgos et al. (75), our isolates were also highly resistant to nalidix acid (80%). The high percentage of resistance to this antibiotic could be reflected in the future with higher AMR to fluoroquinolones, such as enrofloxacin, a risk that deserves a close surveillance. Additionally, 33% of isolates from chickens were resistant to fosfomycin, which is a recently reintroduced antibiotic for the treatment of acute urinary tract infections in humans (76). Several studies in Enterobacteriales have reported the simultaneous presence of fosfomycin resistance genes with β-lactamases encoding genes (77, 78). In this work 17.5% of isolates showed both phenotypes of resistance, a situation that can also be considered of concern for public health.
In summary, this study suggests that in pig farms located in central Chile the predominant S. enterica serotype is S. Typhimurium and in chicken farms is S. Infantis, with a genotypic diversity implying different sources and transmission chains. Isolates from poultry showed more resistance phenotypes against antibiotics, with critical importance those associated with cephalosporins, fosfomycin and fluoroquinolones. The presence of genes, related with virulence and phenotypic antibiotic resistance, indicate a convergence of virulence and resistance determinants, highlighting that efforts should be focused on implementing strict farm-to-table surveillance programs for this pathogen in industrial pig and poultry farms, in order to prevent outbreaks in the population.
Data availability statement
The original contributions presented in the study are included in the article/Supplementary material, further inquiries can be directed to the corresponding author/s.
Ethics statement
Ethical review and approval was not required for the animal study because Study was performed with resistant bacteria. Samples where taken by authorized veterinarians working in the farms. Researchers do not perform any animal studies.
Author contributions
PR and LL contributed to the conception, design of the study, and wrote the first draft of the manuscript. PR, LL, LS, and JC contributed with resources to the study. JG, MB, and TA performed the laboratory analyses. PR, LL, and JC performed the statistical analysis. LL, NG, LS, and JC wrote sections of the manuscript. All authors contributed to the article and approved the submitted version.
Funding
This work was self-founded by JC and LL.
Conflict of interest
The authors declare that the research was conducted in the absence of any commercial or financial relationships that could be construed as a potential conflict of interest.
Publisher's note
All claims expressed in this article are solely those of the authors and do not necessarily represent those of their affiliated organizations, or those of the publisher, the editors and the reviewers. Any product that may be evaluated in this article, or claim that may be made by its manufacturer, is not guaranteed or endorsed by the publisher.
Supplementary material
The Supplementary Material for this article can be found online at: https://www.frontiersin.org/articles/10.3389/fvets.2022.971246/full#supplementary-material
References
1. Pui CF, Wong WC, Chai LC, Tunung R, Jeyaletchumi P, Noor Hidayah MS, et al. Salmonella: A foodborne pathogen. Int Food Res J. (2011) 18:465–73. Avialble online at: https://web.p.ebscohost.com/ehost/detail/detail?vid=0&sid=c259bcaf-f4bb-4699-ad0d-5e479cede221%40redis&bdata=Jmxhbmc9ZXMmc2l0ZT1laG9zdC1saXZl#db=fsr&AN=64500041 (accessed March 1, 2022).
2. Fica CA, Alexandre SM, Prat MS, Fernández RA, Fernández OJ, Heitmann GI. Cambios epidemiológicos de las salmonelosis en Chile: Desde Salmonella typhi a Salmonella enteritidis. Rev Chil Infectol. (2001) 18:85–93. doi: 10.4067/S0716-10182001000200002
3. Basler C, Nguyen TA, Anderson TC, Hancock T, Behravesh CB. Outbreaks of human Salmonella infections associated with live poultry, United States, 1990–2014. Emerg Infect Dis. (2016) 22:1705–11. doi: 10.3201/eid2210.150765
4. Crump JA, Sjölund-Karlsson M, Gordon MA, Parry CM. Epidemiology, Clinical Presentation, Laboratory Diagnosis, Antimicrobial Resistance, and Antimicrobial Management of Invasive Salmonella Infections. Clin Microbiol Rev. (2015) 28:901–37. doi: 10.1128/CMR.00002-15
6. Outbreaks Involving Salmonella | CDC. (2022). Available online at: https://www.cdc.gov/salmonella/outbreaks.html (accessed March 1, 2022).
7. Mahmoud DBSM. Salmonella: A Dangerous Foodborne Pathogen. London: IntechOpen. (2012). p. 454. doi: 10.5772/1308
8. Song H, Zhu A. Isolation and Identification of Salmonella in Pork. In: Schatten H, editor. Salmonella: Methods and Protocols (New York, NY: Springer US). (2021). p. 197–203. doi: 10.1007/978-1-0716-0791-6_18
9. Lapierre L, Cornejo J, Zavala S, Galarce N, Sánchez F, Benavides MB, et al. Phenotypic and genotypic characterization of virulence factors and susceptibility to antibiotics in Salmonella infantis strains isolated from chicken meat: first findings in Chile. Animals. (2020) 10:1049. doi: 10.3390/ani10061049
10. Schiellerup P, Abdul-Redha RJ, Baggesen DL, Andersen SL, Sandvang D. Five cases of gastroenteritis with multiresistant Salmonella enterica serovar Typhimurium DT104 related to farm animals in Denmark. Ugeskr Laeger. (2001) 163:5677–8.
11. Hendriksen SWM, Orsel K, Wagenaar JA, Miko A, van Duijkeren E. Animal-to-Human Transmission of Salmonella Typhimurium DT104A Variant. Emerg Infect Dis. (2004) 10:2225–7. doi: 10.3201/eid1012.040286
12. Hale CR, Scallan E, Cronquist AB, Dunn J, Smith K, Robinson T, et al. Estimates of enteric illness attributable to contact with animals and their environments in the United States. Clin Infect Dis. (2012) 54:S472–9. doi: 10.1093/cid/cis051
13. Lan TTQ, Gaucher ML, Nhan NTM, Letellier A, Quessy S. Distribution of virulence genes among salmonella serotypes isolated from pigs in southern Vietnam. J Food Protect. (2018) 81:1459–66. doi: 10.4315/0362-028X.JFP-17-408
14. Nguyen BD, Cuenca V. M, Hartl J, Gül E, Bauer R, Meile S, et al. Import of aspartate and malate by DcuABC drives H2/fumarate respiration to promote initial salmonella gut-lumen colonization in mice. Cell Host Microbe. (2020) 27:922–36.e6. doi: 10.1016/j.chom.2020.04.013
15. Michael GB, Schwarz S. Antimicrobial resistance in zoonotic nontyphoidal Salmonella: an alarming trend? Clin Microbiol Infect. (2016) 22:968–74. doi: 10.1016/j.cmi.2016.07.033
16. Roy PH, Partridge SR. Genetic mechanisms of transfer of drug resistance. In: Mayers DL, Sobel JD, Ouellette M, Kaye KS, Marchaim D, editors. Antimicrobial Drug Resistance: Mechanisms of Drug Resistance (Cham: Springer International Publishing). (2017). p. 61–76. doi: 10.1007/978-3-319-46718-4_5
17. Kim S, Frye JG, Hu J, Fedorka-Cray PJ, Gautom R, Boyle DS. Multiplex PCR-based method for identification of common clinical serotypes of Salmonella enterica subsp. Enterica. J Clin Microbiol. (2006) 44:3608–15. doi: 10.1128/JCM.00701-06
18. Available, online at: Antigenic_Formulae_of_the_Salmonella_ser20160122-19332-1wjmz5c-with-cover-page-v2.pdf. Available online at: https://www.pasteur.fr/sites/default/files/veng_0.pdf (accessed March 1, 2022).
19. Issenhuth-Jeanjean S, Roggentin P, Mikoleit M, Guibourdenche M, de Pinna E, Nair S, et al. Supplement 2008–2010 (no. 48) to the White–Kauffmann–Le Minor scheme. Res Microbiol. (2014) 165:526–30. doi: 10.1016/j.resmic.2014.07.004
20. Jean-Gilles Beaubrun J, Cheng CM, Chen KS, Ewing L, Wang H, Agpaoa MC, et al. The evaluation of a PCR-based method for identification of Salmonella enterica serotypes from environmental samples and various food matrices. Food Microbiol. (2012) 31:199–209. doi: 10.1016/j.fm.2012.03.016
21. Jean-Gilles Beaubrun J, Ewing L, Jarvis K, Dudley K, Grim C, Gopinath G, et al. Comparison of a PCR serotyping assay, Check & Trace assay for Salmonella, and Luminex Salmonella serotyping assay for the characterization of Salmonella enterica identified from fresh and naturally contaminated cilantro. Food Microbiol. (2014) 42:181–7. doi: 10.1016/j.fm.2014.02.008
22. Oficina de estudios y políticas agrarias, Ministerio de Agricultura de Chile. Carne de aves. Available online at: https://www.odepa.gob.cl/wp-content/uploads/2014/05/Carnedeaves201405.pdf (accessed June 7, 2022).
23. Oficina de estudios y políticas agrarias, Ministerio de Agricultura de Chile. Situación del mercado nacional e internacional de la carne de cerdo. Available online at: https://www.odepa.gob.cl/publicaciones/articulos/situacion-del-mercado-nacional-e-internacional-de-la-carne-de-cerdo (accessed June 7, 2022).
24. Jensen AN, Sørensen G, Baggesen DL, Bødker R, Hoorfar J. Addition of Novobiocin in pre-enrichment step can improve Salmonella culture protocol of modified semisolid Rappaport–Vassiliadis. J Microbiol Methods. (2003) 55:249–55. doi: 10.1016/S0167-7012(03)00158-1
25. Oliveira CFD, Paim TGDS, Reiter KC, Rieger A, D'azevedo PA. Evaluation of four different DNA extraction methods in coagulase-negative Staphylococci clinical isolates. Rev Inst Med Trop São Paulo. (2014) 56:29–33. doi: 10.1590/S0036-46652014000100004
26. Chan K, Baker S, Kim CC, Detweiler CS, Dougan G, Falkow S. Genomic Comparison of Salmonella enterica Serovars and Salmonella bongori by Use of an S. enterica Serovar typhimurium DNA microarray. J Bacteriol. (2003) 185:553–63. doi: 10.1128/JB.185.2.553-563.2003
27. Porwollik S, Boyd EF, Choy C, Cheng P, Florea L, Proctor E, et al. Characterization of Salmonella enterica Subspecies I Genovars by Use of Microarrays. J Bacteriol. (2004) 186:5883–98. doi: 10.1128/JB.186.17.5883-5898.2004
28. Porwollik S, McClelland M. Lateral gene transfer in Salmonella. Microbes Infect. (2003) 5:977–89. doi: 10.1016/S1286-4579(03)00186-2
29. Infostat - Software estadístico. Available online at: https://www.infostat.com.ar/ (accessed Mar 15, 2022).
30. Jackson BR, Griffin PM, Cole D, Walsh KA, Chai SJ. Outbreak-associated Salmonella enterica Serotypes and Food Commodities, United States, 1998–2008. Emerg Infect Dis. (2013) 19:1239–44. doi: 10.3201/eid1908.121511
31. Huehn S, Bunge C, Junker E, Helmuth R, Malorny B. Poultry-Associated Salmonella enterica subsp. enterica Serovar 4,12:d:– Reveals High Clonality and a Distinct Pathogenicity Gene Repertoire. Appl Environ Microbiol. (2009) 75:1011–20. doi: 10.1128/AEM.02187-08
32. Huehn S, La Ragione RM, Anjum M, Saunders M, Woodward MJ, Bunge C, et al. Virulotyping and antimicrobial resistance typing of Salmonella enterica serovars relevant to human health in Europe. Foodborne Pathog Dis. (2010) 7:523–35. doi: 10.1089/fpd.2009.0447
33. Pan Z, Carter B, Núñez-García J, AbuOun M, Fookes M, Ivens A, et al. Identification of genetic and phenotypic differences associated with prevalent and non-prevalent Salmonella Enteritidis phage types: analysis of variation in amino acid transport. Microbiology. (2009) 55:3200–13. doi: 10.1099/mic.0.029405-0
34. M100Ed32 | Performance Standards for Antimicrobial Susceptibility Testing 32nd Edition [Internet]. Clinical & Laboratory Standards Institute. Available online at: https://clsi.org/standards/products/microbiology/documents/m100/ (accessed March 15, 2022).
35. Magiorakos AP, Srinivasan A, Carey RB, Carmeli Y, Falagas ME, Giske CG, et al. Multidrug-resistant, extensively drug-resistant and pandrug-resistant bacteria: an international expert proposal for interim standard definitions for acquired resistance. Clin Microbiol Infect. (2012) 18:268–81. doi: 10.1111/j.1469-0691.2011.03570.x
36. World Health Organization. Critically important antimicrobials for human medicine. 6th rev. Geneva: World Health Organization. (2019). p. 45. Available online at: https://apps.who.int/iris/handle/10665/312266 (accessed March 15, 2022).
37. Parry CM, Thieu NTV, Dolecek C, Karkey A, Gupta R, Turner P, et al. Clinically and Microbiologically Derived Azithromycin Susceptibility Breakpoints for Salmonella enterica Serovars Typhi and Paratyphi A. Antimicrob Agents Chemother. (2015) 59:2756–64. doi: 10.1128/AAC.04729-14
38. Martínez-Cortés I, Gutierrez L, Tapia G, Ocampo L, Sumano H. Serum and milk concentrations of enrofloxacin in cows intramammarily treated with a new enrofloxacin-polymorph. Med Weter. (2016) 72:686–92. doi: 10.21521/mw.5587
39. Kahlmeter G. EUCAST proposes to change the definition and usefulness of the susceptibility category ‘Intermediate'. Clin Microbiol Infect. (2017) 23:894–5. doi: 10.1016/j.cmi.2017.08.015
40. Krumperman PH. Multiple antibiotic resistance indexing of Escherichia coli to identify high-risk sources of fecal contamination of foods. Appl Environ Microbiol. (1983) 46:165–70. doi: 10.1128/aem.46.1.165-170.1983
41. San Martín B, Lapierre L, Cornejo J, Bucarey S. Characterization of antibiotic resistance genes linked to class 1 and 2 integrons in strains of Salmonella spp. isolated from swine. Can J Microbiol. (2008) 54:569–76. doi: 10.1139/W08-045
42. Lapierre L, San Martín B, Araya-Jordán C, Borie C. Comparison of integron-linked antibiotic resistance genes in strains of Salmonella spp. isolated from swine in Chile in 2005 and 2008. Can J Microbiol. (2010) 56:515–21. doi: 10.1139/W10-033
43. Ishihara K, Takahashi T, Morioka A, Kojima A, Kijima M, Asai T, et al. National surveillance of Salmonella enterica in food-producing animals in Japan. Acta Vet Scand. (2009) 51:35. doi: 10.1186/1751-0147-51-35
44. Chen T, Jiang J, Ye C, Xie J, Chen X, Xu D, et al. Genotypic characterization and antimicrobial resistance profile of Salmonella isolated from chicken, pork and the environment at abattoirs and supermarkets in Chongqing, China. BMC Vet Res. (2019) 15:456. doi: 10.1186/s12917-019-2202-4
45. Baggesen D, Nielsen L, Sørensen G, Bødker R, Ersbøll A. Growth inhibitory factors in bovine faeces impairs detection of Salmonella Dublin by conventional culture procedure. J Appl Microbiol. (2007) 103:650–6. doi: 10.1111/j.1365-2672.2007.03292.x
46. Nollet N, Maes D, De Zutter L, Duchateau L, Houf K, Huysmans K, et al. Risk factors for the herd-level bacteriologic prevalence of Salmonella in Belgian slaughter pigs. Prev Vet Med. (2004) 65:63–75. doi: 10.1016/j.prevetmed.2004.06.009
47. Merialdi G, Barigazzi G, Bonilauri P, Tittarelli C, Bonci M, D'incau M, et al. Longitudinal study of Salmonella infection in Italian farrow-to-finish swine herds. Zoonoses Public Health. (2008) 55:222–6. doi: 10.1111/j.1863-2378.2008.01111.x
48. Visscher CF, Klein G, Verspohl J, Beyerbach M, Stratmann-Selke J, Kamphues J. Serodiversity and serological as well as cultural distribution of Salmonella on farms and in abattoirs in Lower Saxony, Germany. Int J Food Microbiol. (2011) 146:44–51. doi: 10.1016/j.ijfoodmicro.2011.01.038
49. Denis M, Houard E, Fablet A, Rouxel S, Salvat G. Distribution of serotypes and genotypes of Salmonella enterica species in French pig production. Vet Rec. (2013) 173:370. doi: 10.1136/vr.101901
50. Bonardi S. Salmonella in the pork production chain and its impact on human health in the European Union. Epidemiol Infect. (2017) 145:1513–26. doi: 10.1017/S095026881700036X
51. Rodríguez I, Barownick W, Helmuth R, Mendoza MC, Rodicio MR, Schroeter A, et al. Extended-spectrum {beta}-lactamases and AmpC {beta}-lactamases in ceftiofur-resistant Salmonella enterica isolates from food and livestock obtained in Germany during 2003-07. J Antimicrob Chemother. (2009) 64:301–9. doi: 10.1093/jac/dkp195
52. Noda T, Murakami K, Etoh Y, Okamoto F, Yatsuyanagi J, Sera N, et al. Increase in resistance to extended-spectrum cephalosporins in Salmonella isolated from retail chicken products in Japan. PLoS ONE. (2015) 10:e0116927. doi: 10.1371/journal.pone.0116927
53. Franco A, Leekitcharoenphon P, Feltrin F, Alba P, Cordaro G, Iurescia M, et al. Emergence of a Clonal Lineage of Multidrug-Resistant ESBL-Producing Salmonella Infantis Transmitted from Broilers and Broiler Meat to Humans in Italy between 2011 and 2014. PLoS ONE. (2015) 10:e0144802. doi: 10.1371/journal.pone.0144802
54. Tate H, Folster JP, Hsu CH, Chen J, Hoffmann M, Li C, et al. Comparative Analysis of Extended-Spectrum-β-Lactamase CTX-M-65-Producing Salmonella enterica Serovar Infantis Isolates from Humans, Food Animals, and Retail Chickens in the United States. Antimicrob Agents Chemother. (2017) 61:e00488–17. doi: 10.1128/AAC.00488-17
55. Antunes P, Mourão J, Campos J, Peixe L. Salmonellosis: the role of poultry meat. Clin Microbiol Infect. (2016) 22:110–21. doi: 10.1016/j.cmi.2015.12.004
56. BoletínSalmonella-12052020A.pdf. Available online at: https://www.ispch.cl/sites/default/files/Bolet%C3%ADnSalmonella-12052020A.pdf (accessed June 7, 2022).
57. Schonewille E, Nesse LL, Hauck R, Windhorst D, Hafez HM, Vestby LK. Biofilm building capacity of Salmonella enterica strains from the poultry farm environment. FEMS Immunol Med Microbiol. (2012) 65:360–5. doi: 10.1111/j.1574-695X.2012.00966.x
58. Knudsen GM, Nielsen MB, Grassby T, Danino-Appleton V, Thomsen LE, Colquhoun IJ, et al. third mode of surface-associated growth: immobilization of Salmonella enterica serovar Typhimurium modulates the RpoS-directed transcriptional programme. Environ Microbiol. (2012) 14:1855–75. doi: 10.1111/j.1462-2920.2012.02703.x
59. Rajan K, Shi Z, Ricke SC. Current aspects of Salmonella contamination in the US poultry production chain and the potential application of risk strategies in understanding emerging hazards. Crit Rev Microbiol. (2017) 43:370–92. doi: 10.1080/1040841X.2016.1223600
60. Pardo-Esté C, Lorca D, Castro-Severyn J, Krüger G, Alvarez-Thon L, Zepeda P, et al. Genetic characterization of salmonella infantis with multiple drug resistance profiles isolated from a poultry-farm in Chile. Microorganisms. (2021) 9:2370. doi: 10.3390/microorganisms9112370
61. Dunkley KD, Callaway TR, Chalova VI, McReynolds JL, Hume ME, Dunkley CS, et al. Foodborne Salmonella ecology in the avian gastrointestinal tract. Anaerobe. (2009) 15:26–35. doi: 10.1016/j.anaerobe.2008.05.007
62. Jones FT. A review of practical Salmonella control measures in animal feed. J Appl Poultry Res. (2011) 20:102–13. doi: 10.3382/japr.2010-00281
63. Hilbert F, Smulders FJM, Chopra-Dewasthaly R, Paulsen P. Salmonella in the wildlife-human interface. Food Res Int. (2012) 45:603–8. doi: 10.1016/j.foodres.2011.08.015
64. Xu Y, Tao S, Hinkle N, Harrison M, Chen J. Salmonella, including antibiotic-resistant Salmonella, from flies captured from cattle farms in Georgia, U.S.A. Sci Total Environ. (2018) 616:90–96. doi: 10.1016/j.scitotenv.2017.10.324
65. Donoso A, Paredes N, Retamal P. Detection of Antimicrobial Resistant Salmonella enterica Strains in Larval and Adult Forms of Lesser Mealworm (Alphitobius diaperinus) From Industrial Poultry Farms. Front Vet Sci. (2020) 7:577848. doi: 10.3389/fvets.2020.577848
66. Yoon H, Ansong C, Adkins JN, Heffron F. Discovery of Salmonella virulence factors translocated via outer membrane vesicles to murine macrophages. Infect Immun. (2011) 79:2182–92. doi: 10.1128/IAI.01277-10
67. Alba P, Leekitcharoenphon P, Carfora V, Amoruso R, Cordaro G, Di Matteo P, et al. Molecular epidemiology of Salmonella Infantis in Europe: insights into the success of the bacterial host and its parasitic pESI-like megaplasmid. Microb Genom. (2020) 6:e000365. doi: 10.1099/mgen.0.000365
68. Bogomazova AN, Gordeeva VD, Krylova EV, Soltynskaya IV, Davydova EE, Ivanova OE, et al. Mega-plasmid found worldwide confers multiple antimicrobial resistance in Salmonella Infantis of broiler origin in Russia. Int J Food Microbiol. (2020) 319:108497. doi: 10.1016/j.ijfoodmicro.2019.108497
69. Mejía L, Medina JL, Bayas R, Salazar CS, Villavicencio F, Zapata S, et al. Genomic Epidemiology of Salmonella Infantis in Ecuador: From Poultry Farms to Human Infections. Front Vet Sci. (2020) 7:547891. doi: 10.3389/fvets.2020.547891
70. García-Soto S, Abdel-Glil MY, Tomaso H, Linde J, Methner U. Emergence of multidrug-resistant Salmonella enterica subspecies enterica serovar infantis of multilocus sequence type 2283 in German broiler farms. Front Microbiol. (2020) 11:1741. doi: 10.3389/fmicb.2020.01741
71. SAG Servicio Agrícola y Ganadero de Chile. Resolución Exenta N?:4579/2018. (2018). Available online at: https://www.sag.gob.cl/sites/default/files/resol_4.579-2018.pdf (accessed May 19, 2022).
72. OIE_List_antimicrobials_July2019.pdf. Available online at: https://www.oie.int/fileadmin/Home/eng/Our_scientific_expertise/docs/pdf/AMR/A_OIE_List_antimicrobials_July2019.pdf (accessed May 19, 2022).
73. Lai J, Wu C, Wu C, Qi J, Wang Y, Wang H, et al. Serotype distribution and antibiotic resistance of Salmonella in food-producing animals in Shandong province of China, 2009 and 2012. Int J Food Microbiol. (2014) 180:30–8. doi: 10.1016/j.ijfoodmicro.2014.03.030
74. Douris A, Fedorka-Cray PJ, Jackson CR. Characterization of Salmonella enterica serovar Agona slaughter isolates from the animal arm of the National Antimicrobial Resistance Monitoring System-Enteric Bacteria (NARMS): 1997 through 2003. Microb Drug Resist. (2008) 14:55–63. doi: 10.1089/mdr.2008.0787
75. Vinueza-Burgos C, Cevallos M, Ron-Garrido L, Bertrand S, De Zutter L. Prevalence and Diversity of Salmonella Serotypes in Ecuadorian Broilers at Slaughter Age. PLoS ONE. (2016) 11:e0159567. doi: 10.1371/journal.pone.0159567
76. Fajfr M, Balik M, Cermakova E, Bostik P. Effective treatment for uncomplicated urinary tract infections with oral fosfomycin, single center four year retrospective study. Antibiotics. (2020) 9:511. doi: 10.3390/antibiotics9080511
77. Falagas ME, Kastoris AC, Kapaskelis AM, Karageorgopoulos DE. Fosfomycin for the treatment of multidrug-resistant, including extended-spectrum beta-lactamase producing, Enterobacteriaceae infections: a systematic review. Lancet Infect Dis. (2010) 10:43–50. doi: 10.1016/S1473-3099(09)70325-1
78. Hou J, Huang X, Deng Y, He L, Yang T, Zeng Z, et al. Dissemination of the fosfomycin resistance gene fosA3 with CTX-M β-lactamase genes and rmtB carried on IncFII plasmids among Escherichia coli isolates from pets in China. Antimicrob Agents Chemother. (2012) 56:2135–8. doi: 10.1128/AAC.05104-11
Keywords: Salmonella, virulence, antimicrobial resistance, pigs, chicken, Chile
Citation: Retamal P, Gaspar J, Benavides MB, Saenz L, Galarce N, Aravena T, Cornejo J and Lapierre L (2022) Virulence and antimicrobial resistance factors in Salmonella enterica serotypes isolated from pigs and chickens in central Chile. Front. Vet. Sci. 9:971246. doi: 10.3389/fvets.2022.971246
Received: 16 June 2022; Accepted: 31 August 2022;
Published: 20 September 2022.
Edited by:
Rafael Vignoli, Universidad de la República., UruguayReviewed by:
Yujie Hu, China National Center for Food Safety Risk Assessment, ChinaAna Umpiérrez, Instituto de Investigaciones Biológicas Clemente Estable (IIBCE), Uruguay
Copyright © 2022 Retamal, Gaspar, Benavides, Saenz, Galarce, Aravena, Cornejo and Lapierre. This is an open-access article distributed under the terms of the Creative Commons Attribution License (CC BY). The use, distribution or reproduction in other forums is permitted, provided the original author(s) and the copyright owner(s) are credited and that the original publication in this journal is cited, in accordance with accepted academic practice. No use, distribution or reproduction is permitted which does not comply with these terms.
*Correspondence: Lisette Lapierre, bGxhcGllcnJlQHVjaGlsZS5jbA==; Javiera Cornejo, amFjb3JuZWpAdWNoaWxlLmNs