- 1Department of Clinical Pathology, Faculty of Veterinary Medicine, Zagazig University, Zagazig, Egypt
- 2Department of Biochemistry, Animal Health Institute, Mansoura, Egypt
- 3Department of Clinical Pathology, Animal Health Institute, Mansoura, Egypt
- 4Department of Pathology, Faculty of Veterinary Medicine, Kafrelsheikh University, Kafr El-Sheikh, Egypt
- 5Department of Anatomy, Faculty of Medicine, University of Jeddah, Jeddah, Saudi Arabia
- 6Department of Biology, Faculty of Science, Albaha University, Al Aqiq, Saudi Arabia
- 7Department of Zoonoses, Faculty of Veterinary Medicine, Sohag University, Sohag, Egypt
Avian pathogenic Escherichia coli is one of the principal causes of heavy economic losses to the poultry industry. Little is known about the underlying mechanisms, particularly the potential role of immunoglobulin A and the DNA damage, involving the beneficial effects of dietary supplementation of probiotics and prebiotics in avian colibacillosis. The current study investigated the potential effects of probiotic and prebiotic dietary supplementation on E. coli-infected broiler chicks. A total of 120 1-day-old unsexed Hubbard chicks were divided into six groups: Group 1 was considered as a negative control; Group 2 was supplemented with 1 g/kg feed of Lactobacillus plantarum; Group 3 was supplemented with amylase enzyme; Group 4 served as a positive control infected orally by E. coli O78; Group 5 was supplemented with L. plantarum from 1-day-old chicken and then infected orally with E. coli O78; and Group 6 was supplemented with amylase enzyme from 1-day old chicken and then infected orally with E. coli O78. For all examined groups, the experimental period lasted for 42 days. The E. coli-infected group revealed a decrease in body performance parameters with a significant increase in the liver enzymes and renal function tests. The same group recorded a significant decrease in serum total proteins, albumins, and globulins, and the alteration of immunological parameters, antioxidant enzymes, oxidative stress parameters, and comet assay revealed highly damaged DNA in the liver and the intestine. By histopathological examination, a series of histopathological changes in the liver, the kidney, and the intestine were observed. The infected chick pretreated with probiotics or prebiotics demonstrated an improvement in body performance parameters besides a significant decrease in the hepatic enzymes and renal function tests. We noticed that, in treated groups, there was a significant increase in serum total proteins in the serum albumin and globulin levels, immunological parameters, and antioxidant enzymes. Furthermore, DNA damage and histopathological changes within hepatic, renal, and intestinal tissues were markedly diminished in the treated groups compared with the infected group. We concluded that the adverse effects of E. coli could be modulated through the chemopreventive administration of probiotics and prebiotics.
Introduction
Avian colibacillosis is considered one of the most important bacterial diseases that target the poultry industry, resulting in severe economic losses in broilers (Kabir, 2010). The disease is considered one of the principal causes of morbidity and mortality in broilers that reach up to 50%, either as a primary or as a secondary pathogen (Kabir, 2010). Escherichia coli (E. coli) causes avian colibacillosis, which is considered a natural commensal inhabitant of the chicken's intestinal tract and the trachea to a lesser extent. Commonly, 10–15% of intestinal E. coli are avian pathogenic E. coli (APEC) with various virulence factors, which might result in systemic diseases such as pericarditis, perihepatitis, airsaculities, and peritonitis (1). A total of 20 E. coli serotype isolates were recovered in poultry, including O6, O111, O55, O114, O15, O125, and O78 (2). Infection is stimulated when a bird's defense mechanism falls, potentiated by various factors such as bad management, concurrent infections, and immunosuppression (3). No effective vaccine is available against colibacillosis, mainly as a result of the variety of APEC strains existing in the field. Therefore, prophylactic measures and antibiotic treatment are required to control colibacillosis. Antibiotic use is considered the most common way for treating colibacillosis. This strategy might possess major side effects on the bird and consumers combined with the development of drug resistance.
Feed additives are an important strategy. They have been used to advance the efficiency of poultry industry and the performance and health of animals by targeting growth efficiency, disease prevention, and feed utilization improvement (4). Some studies revealed that feed additives have no harmful effects on human and animal health and on the environment (4). Probiotics are live categories of feed additives that consist of one single strain or a combination of several strains of non-pathogenic microorganisms such as bacteria (i.e., Enterococcus, Bacillus, Lactobacillus plantarum, and Pediococcus), yeast, and fungi, which are probiotics, which beneficially improve the gut microflora of the host (5). Among others, L. plantarum is an antimicrobial feed additive probiotic with interesting activity against various poultry pathogens since they inhibit the progress of E. coli, enhance growth performance, reduce Enterobacteriaceae population, and increase Lactobacillus numbers in broilers (6). The probiotic mode of action is mainly based on competitive exclusion, bacterial antagonism, and immune modulation (7). Fermentable sugars (i.e., fructo-oligo-saccharides and galacto-oligo-saccharides) and exogenous enzymes (8) are prebiotics that are non-digestible food ingredients that are used as feed additives to improve host health and protect poultry against pathogens. Amylase, xylanase, and protease are exogenous enzymes, which are a group of proteins that facilitate specific chemical reactions, increase digestion, and are, therefore, supplemented to animal diet (9). It should be highlighted that the amylase enzyme is the exogenous bacterial enzyme commonly added to a bird's diet to match the requirement of birds and improve its performance by increasing nutrient utilization and maintaining health status (10). The mode of action of exogenous enzymes mainly targets lower gut viscosity, leading to complete digestion and absorption of nutrients, reduced microbial proliferation, and improved gut health by selectively stimulating the growth and/or activity of one or a limited number of bacteria (11). From the available literature, several previous studies investigated the potential benefits of dietary probiotic and prebiotic supplementations on animal and poultry diets (12–14). Little information is available about the mechanistic pathways underlying these potential beneficial effects of these supplements in the poultry industry and the potential role of immunoglobulins A and DNA damage using the comet assay test. The present study is aimed at understanding how these feed additives can be used to control E. coli infection and thus improving the efficiency of poultry production.
Materials and methods
Ethical approval
The animal care and experimental protocols were approved by the ZU-IACUC committee, Faculty of Veterinary Medicine, Zagazig University, Egypt. The approval number of the study is ZU-IACUC/2/F/88/2021.
Tested compounds
Lactobacillus plantarum (1.6 Billion CFU) was purchased as a biotechnology grade probiotic bacterial powder from the Egyptian Company for Biotechnological, Cairo, Egypt. Meanwhile, amylase enzyme was also purchased as a biotechnology grade exogenous prebiotic enzyme product produced from natural anaerobic bacteria from New Vet Company, Mansoura, Egypt.
Bacteria (the inoculum's bacteria) (E. Coli strain)
In the present study, a virulent E. coli strain, serotype O78, was kindly supplied, identified, classified, and serotyped by the Microbiology Department, Zagazig University as described in a previous study (15). The used strain of E. coli was first demonstrated to be pathogenic in a preliminary infectivity trial (pilot experiment) (16). The E. coli inoculum was a logarithmic phase culture produced by overnight incubation of E. coli bacteria in a nutrient broth (17). The number of bacteria per milliliter was determined by plating 10-fold serial dilution of the nutrient broth suspension on plate count agar. Titers were expressed as colony-forming units per ml (CFU/ml) as described in a previous study (18). Approximately, 1 ml of inoculum (broth culture) containing 3 × 107 viable CFU of E. coli strain O78/1 ml/chicks were experimentally infected to the chicks orally via intra-crop (19).
Animals and experimental design
The experimental treatments and sampling timeline are presented in Table 1. A total of 120 1-day-old unsexed chicks of Hubbard strain broilers were obtained from a hatchery in Dakahlia Poultry Company, Dakahlia, Egypt. The experiment lasted for 42 days with good ventilation. Birds were randomly divided into six groups (20 each) and raised in an open, well-ventilated house with sawdust. Rearing was initiated in floor pens until 7 days old following all hygienic measures. Chickens were then moved to wire-floored cages until the end of the experiment. Tap water and a balanced commercial ration free from antibiotics and anticoccidial were provided to the chickens ad libitum, and its ingredients and chemical compositions were formulated as presented elsewhere (20). The optimum temperature was adjusted using electric radiators and ventilators, which were set at 34°C during the first week and gradually reduced by 3°C every week until it reached 24°C. In addition, the light program for the first week was 24 h a day and then changed to 16 h of light and 8 h of dark over 7 to 42 days. All birds were vaccinated against Newcastle disease at 7 and 14 days old and 11 and 22 days old for Gumboro disease (21).
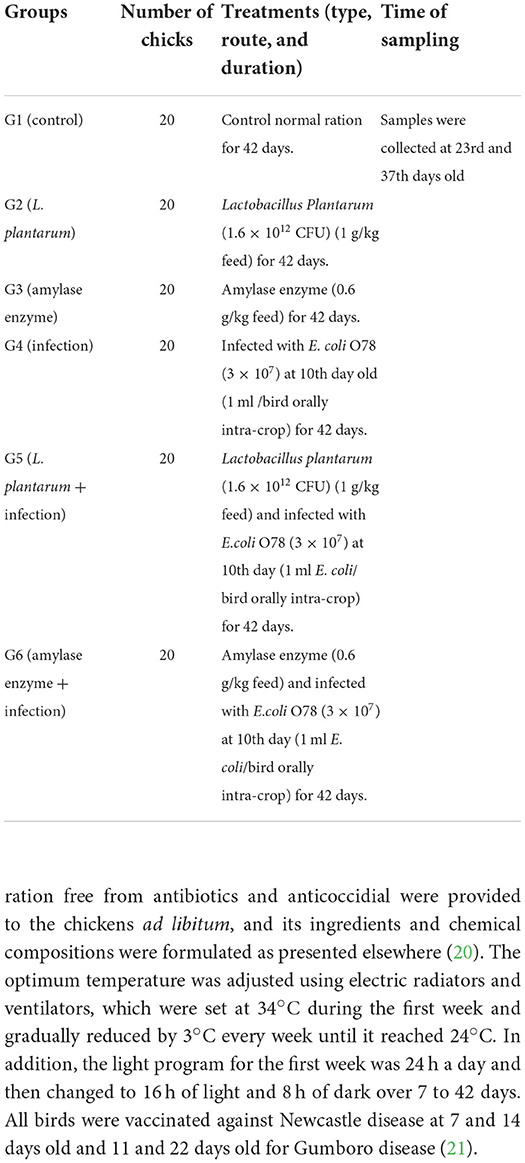
Table 1. The timeline of the experimental protocol explaining the day (D) number, treatments, and sampling.
Growth performance
The average initial body weight (BW) was reported at the beginning of the experiment. BW was then calculated every week as described in a previous study (22), and body weight gain (BWG) was determined (23). The difference between the weight of the provided feed and the feed that remained was used to calculate feed intake (FI) per replicate. The feed conversion ratio (FCR) was estimated according to the following equation (24):
Blood sampling and tissue collection
Blood samples were drawn from the wing vein of each group on the 23rd and 37th day from the start of the experiment. Approximately, 5 ml of blood was used to separate the serum samples, which were then kept at −20°C for further biochemical, immunological, and antioxidant analyses. Meanwhile, 2 ml of blood taken was collected in a heparinized tube for phagocytic activity and phagocytic index test. On the 23rd and 37th days of the experiment, chickens were killed by neck dislocation, specimens from both the liver and the intestine were collected, placed in an ice-cold phosphate buffer saline (BPS), and kept at −20°C until further use for the comet assay. Specimens from the liver, the kidney, and the intestine were collected, fixed in a 10% neutral buffered formalin solution, and trimmed for histopathological examination.
Serum biochemical parameters
The serum activities of alanine aminotransferase (ALT) and aspartate aminotransferase (AST) were determined (25). Serum alkaline phosphatase activity (ALP) and urea were also measured (26), and the serum creatinine levels were determined according to Henry (27). Serum uric acid was estimated according to Fossati et al. (28). The serum total protein and albumin levels were evaluated according to Grant (29) and Doumas et al. (30), respectively. The serum globulin levels were calculated mathematically by subtracting albumin values from total protein values, as described in a previous study (31). The phagocytic activity and phagocytic index test were estimated according to Wilkinson (32), while serum immunoglobulin A (IgA) was estimated according to Bianchi et al. (33). The serum interleukin-6 level was determined according to Chan and Perlstein (34). Furthermore, serum malondialdehyde (MDA), superoxide dismutase (SOD), and catalase (CAT) were determined (35–37).
Comet assay (single cell gel electrophoresis)
The detection of DNA damage was measured in the liver and the intestinal tissues using comet assay (38). Tissues were embedded in 0.6% normal melting point and low melting point agarose gel on microscope slides. The slides were then immersed in lysing solution (2.5 mol NaCl, 100 Mm Na2EDTA, freshly added 1%Triton-x100, and 10% DMSO) for 1 h at 4°C for denaturation and unwinding for DNA. Slides were placed in an electrophoresis buffer (300 mM NaOH, 1 mM Na2EDTA PH. 13.0) at 4°C for 30 min. Neutralization was performed using Tris-Hcl buffer (400 Mm Tris-Hcl, PH 7.4) followed by final staining with a fluorescent dye (20 ug/ml ethidium bromide). Observation of the DNA damage was determined by measuring the tail length and tail moment using X 40 objective on a fluorescent microscope (Nikon Microscope-Eclipse, E600 with Y-FL EPI-Fluorescence attachment, Japan) equipped with an excitation filter of 515–560 nm, barrier filter of 590 nm, and automatic digital imaging system running Comet assay TM software (perceptive Instrument, UK).
Histopathological examination
Specimens from internal organs (mainly the liver, the kidney, and the intestine) were collected on the 23rd and 37th days from all groups and fixed in a 10% neutral buffered formalin solution, dehydrated in graded ethanol (70–100 %), cleared in xylene, and implanted in paraffin. A total of 5 μm thick paraffin sections were prepared and regularly stained with hematoxylin and eosin (HE) dyes and then examined using a standard light microscope (39).
Statistical analysis
Data were analyzed by the analysis of variance method (ANOVA) using SPSS 18.0 software (40). Duncan multiple range tests were used to compare the means and the significance of differences which is considered at p ≤ 0.05.
Results
Clinical Signs and body performance
The experimentally E. coli-infected non-treated chicks exhibited ruffled feathers, inability to stand, dropping wings, sunken eyes, in-appetence, dullness, depression, decreased BW, breathing difficulty and gasping, sneezing and coughing, beak fluid discharge, white to yellowish diarrhea, and high mortality rate, which reached 35% and appeared 5–7 days post infection. Taking this into account, the severity of clinical signs was decreased in the experimentally E. coli-infected chicks treated with L. plantarum and amylase enzyme, whereas some chicks exhibited decreased appetite, decreased BW, respiratory rales, sneezing, and a lower mortality rate of 10–15%. As illustrated in Table 2, E. coli-infected non-treated chicks experienced a significant decrease in BW, BWG, and FI when compared with the control group. However, a highly significant increase in the BW, BWG, and FI were detected in birds treated with L. plantarum and amylase enzyme as compared to the normal control group (p < 0.05). These parameters were improved in the E. coli-infected treated groups with L. plantarum and amylase enzyme as compared to the E. coli-infected non-treated group during the experimental period. The weekly FCR exhibited a highly significant decrease in the groups treated with L. plantarum and amylase enzyme during the experimental period. A significant increase was noticed in the group infected with E. coli, and the non-treated compared with the control group. In addition, the groups infected with E. coli and treated with L. plantarum and amylase enzyme showed a significant improvement in the FCR as compared to the E. coli-infected non-treated group.
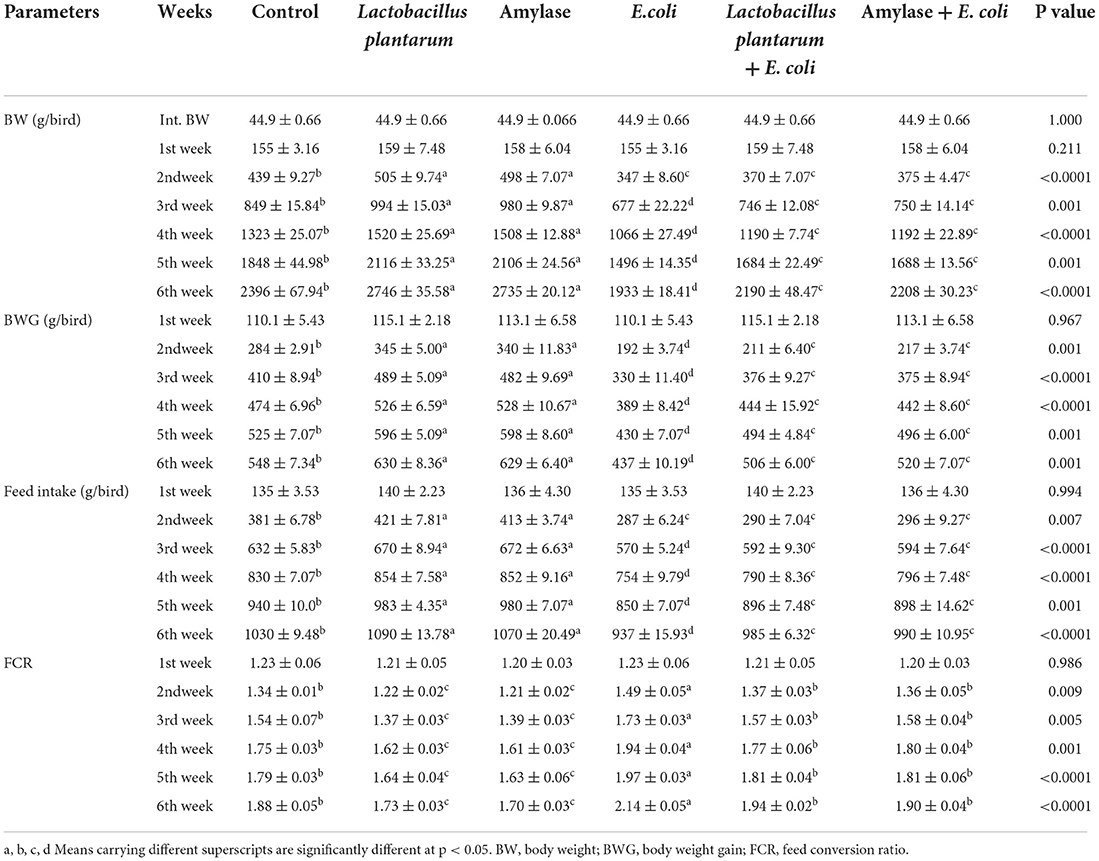
Table 2. Effect of probiotic and prebiotic supplementation on the growth performance in chickens of different groups (mean values ± S.E).
Effects of probiotic and prebiotic supplementation on some biochemical parameters
As shown in Table 3, the administration of L. plantarum or amylase enzyme reported a significant decrease in serum liver enzymes (ALT, AST, and ALP) and in the parameters of the kidney function test (urea, creatinine, and uric acid) at both periods of the experiment (23rd and 37th days of experiment) as compared to the control group. The E. coli infected non-treated chicks revealed a significant increase in the serum liver enzymes level and in the parameters of the kidney function tests when compared with the normal control group. Also, the treatment of infected chicks with either L. plantarum or amylase enzyme revealed an improvement in serum liver enzymes and the kidney function tests, which showed a significant decrease in G4, and the lowest value was reported in G5, which then returned near to normal at the end of the experimental period. Regarding serum total protein and albumin, birds treated with L. plantarum or amylase enzyme showed a significant increase in their values. However, a significant decrease in serum total protein and albumin values was noticed in E. coli-infected non-treated group when compared with the control group. On the contrary, the infected E. coli birds treated with L. plantarum or amylase enzyme recorded an improvement in serum total protein and albumin in comparison with the E. coli-infected non-treated birds over the experimental period. Regarding the serum total globulin, a significant increase was recorded in all groups, and this increase was more pronounced in G4 and less pronounced in G2 and G3 on the 23rd day of the experiment as compared to G1. On the contrary, on the 37th day of the experiment, the treated groups with L. plantarum or amylase enzyme showed a significant increase in serum total globulin. A significant decrease was noticed in the E. coli-infected non-treated group as compared to the control group. Compared with the E. coli-infected non-treated group, G5 and G6 showed an improvement expressed by a significant increase in the serum total globulin and returned to near-normal values at the end of the experiment.
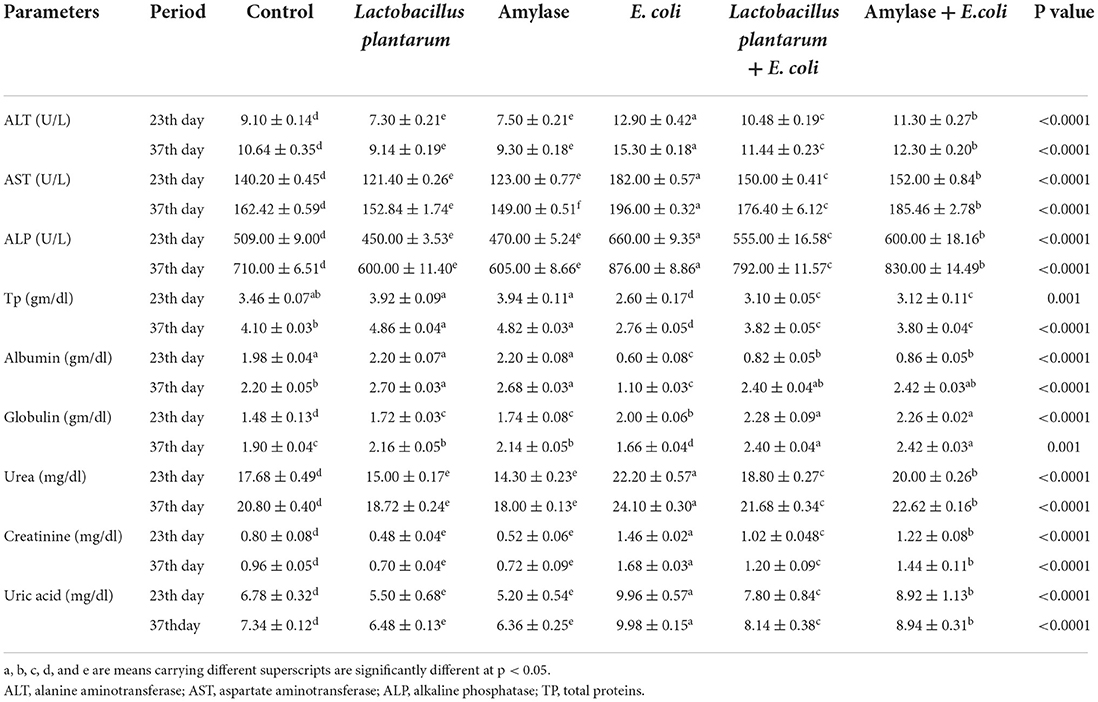
Table 3. Effect of probiotic and prebiotic supplementation on some biochemical parameters in chickens of different groups at 23th and 37th day of the experimental period (mean values ± S.E).
Effects of probiotic and prebiotic supplementation on some serum immunological parameters
Table 4 depicts that, on both the 23rd and 37th days of the experiment, a significant increase in serum IgA was recorded in chicks treated with L. plantarum or amylase enzyme when compared with the control group. E. coli-infected non-treated birds showed a significant increase in serum IgA levels in 23-day-old birds followed by a significant decrease in 37-day-old birds. Moreover, the E. coli-infected treated groups with L. plantarum or amylase enzyme showed a significant increase in serum IgA compared with the infected non-treated group over the experimental period. G6 showed the highest value on both the 23rd and 37th days of the experiment. Moreover, birds treated with L. plantarum or amylase enzyme showed a decrease in serum IL6 during the experimental period, and the lowest value was recorded in G3, while the birds infected with E. coli untreated group recorded a significant increase in serum IL6 as compared with the control group. Compared with the E. coli-infected non-treated group, G5 and G6 revealed a significant increase in serum IL6, and its highest value was recorded in G6. On both the 23rd and 37th days of the experiment, chickens treated with L. plantarum or amylase enzyme recorded a significant increase in phagocytic percent and index, and the highest value was noticed in G3 as compared with the control group (G1). Furthermore, the infected E. coli-non-treated chicks manifested a significant decrease in the aforementioned phagocytic parameters compared with the control group. The E. coli-infected treated chickens with L. plantarum or amylase enzyme revealed a significant increase in phagocytic percentage and index, and the highest value was observed in G6 when compared with the E. coli-infected non-treated group.
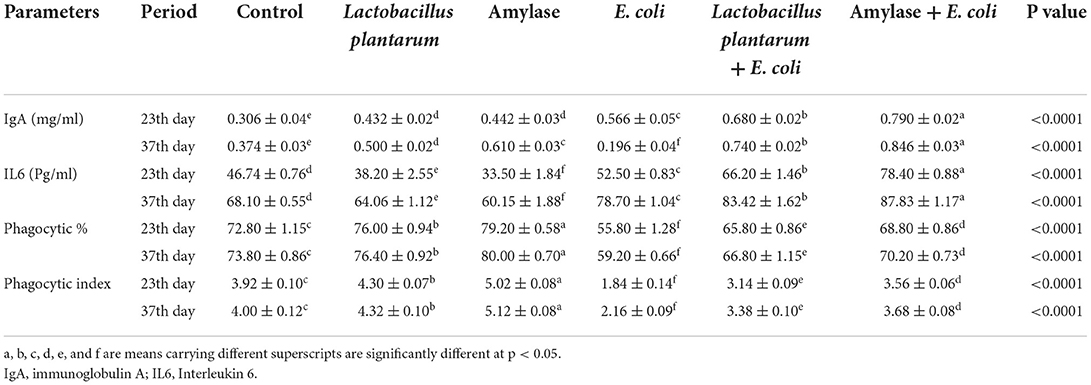
Table 4. Effect of probiotic and prebiotic supplementation on some immunological parameters in chickens of different groups on 23rd and 37th days of the experimental period (mean values ± S.E).
Effects of probiotic and prebiotic supplementation on some serum oxidative stress and antioxidant markers
As illustrated in Table 5, both groups treated with L. plantarum and amylase enzyme showed a significant decrease in serum malondialdehyde (MDA) on the 23rd and 37th days of the experiment. However, there was a significant increase in MDA in the E. coli-infected non-treated group as compared with the control. Compared with the E. coli-infected group, there was a significant decrease in MDA in the groups infected with E. coli and treated with L. plantarum and amylase enzyme over the experimental period, which was still higher than the control values. In addition, serum SOD and catalase activities revealed a significant increase in the birds treated with the L. plantarum and amylase enzyme, but a significant decrease was observed in the E. coli-non-treated infected chicks on 23rd and 37th days. However, birds infected with E. coli and treated with L. plantarum and amylase enzyme exhibited a significant increase in serum SOD and catalase activities as compared with the E. coli-infected non-treated birds.
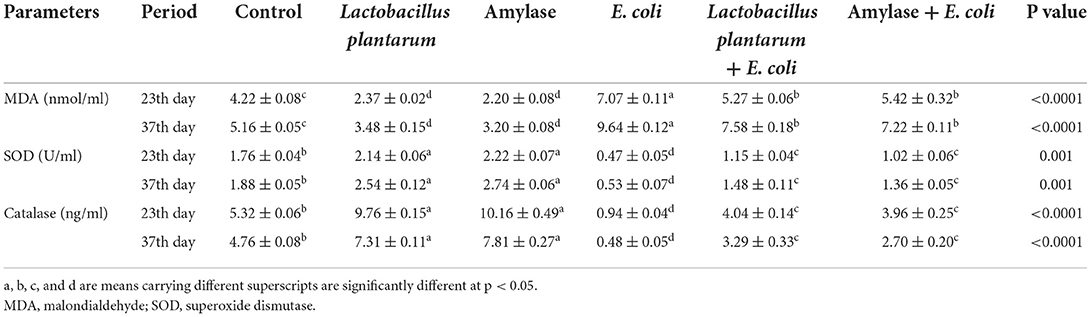
Table 5. Effect of probiotic and prebiotic supplementation on some serum oxidative stress and antioxidant markers in chickens of different groups on 23rd and 37th days of the experimental period (mean values ± S.E).
Effects of probiotic and prebiotic supplementation on DNA damage
The results of the comet assay test in the liver and the intestine are illustrated in Figure 1 and Tables 6, 7. An improvement in the DNA degradation was observed in the group treated with the probiotic L. plantarum, which was represented by a decrease in comet length, tail length, head diameter, DNA percentage in the tail, tail moment, and olive tail moment with a significant increase in DNA percentage in the head, as compared to the control group. Conversely, the group treated with amylase enzyme showed a nonsignificant difference in DNA degradation throughout the experimental period when compared with the control group. The E. coli-infected non-treated group showed high DNA degradation and a highly significant increase in comet length, tail length, head diameter, DNA percentage in the tail, tail moment, and olive tail moment with a significant decrease in head DNA percentage as compared to the control group. Moreover, broilers infected with E. coli and treated with L. plantarum group recorded a significant decrease in DNA degradation also, and a low DNA degradation improvement was reported in the group infected with E. coli and treated with amylase enzyme when compared to the infected non-treated group at both the 23rd and 37th experimental days.
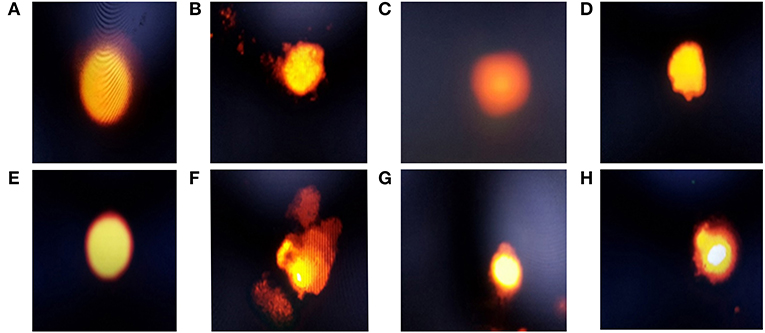
Figure 1. Electrophoresis of hepatic and intestinal nucleus of chickens belonging to different groups, stained with 1.2% ethidium bromide agarose (400x): (A) The hepatic DNA of control (G1), Lactobacillus plantarum (G2), and amylase enzyme (G3) groups showed no DNA damage, which was revealed by no migration of DNA fragment from the nucleus core; (B) The hepatic DNA of E.coli-infected group (G4) showed a high degree of DNA degradation, noticed by large DNA fragments migrating away from the core forming comet tail, with high nucleus core reduction; (C) The hepatic DNA of E.coli-infected group treated with Lactobacillus plantarum showed a minimal degree of DNA damage, with low comet tail; (D) The hepatic DNA of E.coli-infected group and treated with amylase showed a moderate degree of DNA damage with moderate comet tail; (E) Intestinal DNA of control, Lactobacillus plantarum and amylase enzyme groups showed no DNA damage; (F) Intestinal DNA of the E.coli-infected group showed a high degree of DNA damage, with large comet tail and a greatly reduced nucleus core, which showed that the DNA damage in intestinal cells was higher than the hepatic cells; (G) Intestinal DNA of the E.coli-infected group and treated with Lactobacillus plantarum showed a minimal degree of DNA damage with minimal comet tail, but the damage was higher than the hepatic cells; (H) Intestinal DNA of E.coli-infected group and treated with amylase enzyme showed a moderate degree of DNA damage, with moderate comet tail, which showed that these damages were higher in intestinal cells than the hepatic cells.
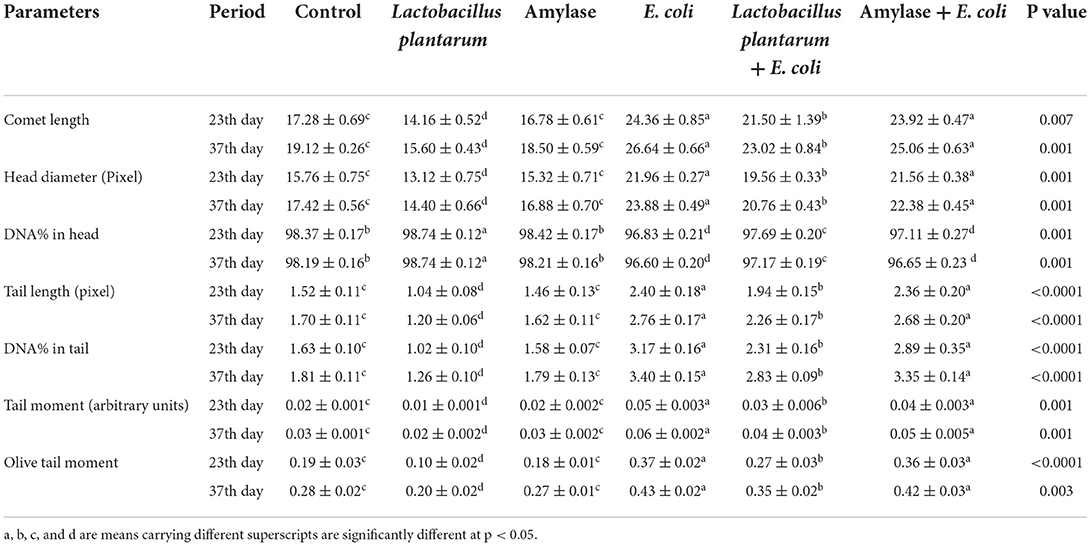
Table 6. Effect of probiotic and prebiotic supplementation on DNA damage indices in the liver tissue of chickens of different groups on 23rd and 37th days of the experimental period (mean values ± S.E).
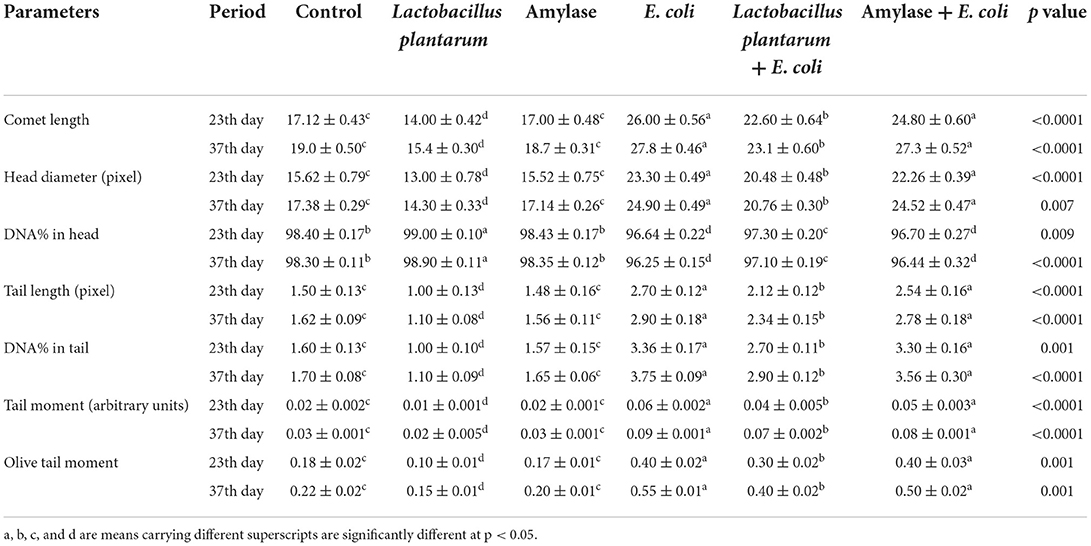
Table 7. Effect of probiotic and prebiotic supplementation on DNA damage indices in the intestine of chickens of different groups on 23rd and 37th days of the experimental period (mean values ± S.E).
Histopathological examination
As shown in Figures 2A–C, the liver of control chicks and those treated with L. plantarum and amylase enzyme revealed normal tissue architecture and cellular details with no significant lesions on the 23rd and 37th days. In contrast, on the 23rd day, the liver of the E. coli-infected group revealed severe dilated and congestion of hepatic blood vessels (central veins and sinusoids) with focal periportal and inflammatory cells (macrophages, lymphocytes, and plasma cells predominantly) infiltration combined with severe degenerative changes as vacuolar degeneration with atrophied hepatocytes (Figure 2D). Moreover, on the 23rd day, the liver of E. coli-infected chicks administered with L. plantarum revealed normal hepatic parenchyma with dilated hepatic sinusoids, mild perivascular inflammatory cells infiltration with congestion of hepatic sinusoids, and mild degeneration in hepatocytes (Figure 2E). In addition, E. coli-infected chicken treated with amylase enzyme exhibited slight congestion of both hepatic blood vessels and sinusoids on the 23rd day. Some chickens of the same group exhibited perivascular infiltration of leucocytic cells and von Kupffer cells (Figure 2F). While, on the 37th day, the liver sections of E. coli-infected chicks showed a disappearance of basic architecture, in addition to multifocal coagulative necrosis represented in the karyoltic nuclei of hepatocytes, perivascular aggregation of leucocytic cells and fibroblast, and hyperemia (Figure 2G). On the 37th day, in most cases, the liver of the E. coli-infected chicks administered with L. plantarum restored their normal histomorphologic picture of tissue architecture and cellular details, while some cases demonstrated mild diffuse atrophied hepatocytes and congestion of both hepatic blood vessels and hepatic sinusoids (Figure 2H), while, on the 37th day, the liver sections of E. coli-infected chicken treated with amylase enzyme showed normal tissue architecture and cellular details, and mild diffuse coagulative necrosis is represented in karyolysis of hepatic cells nuclei with slight lymphocytic cells infiltration (Figure 2I).
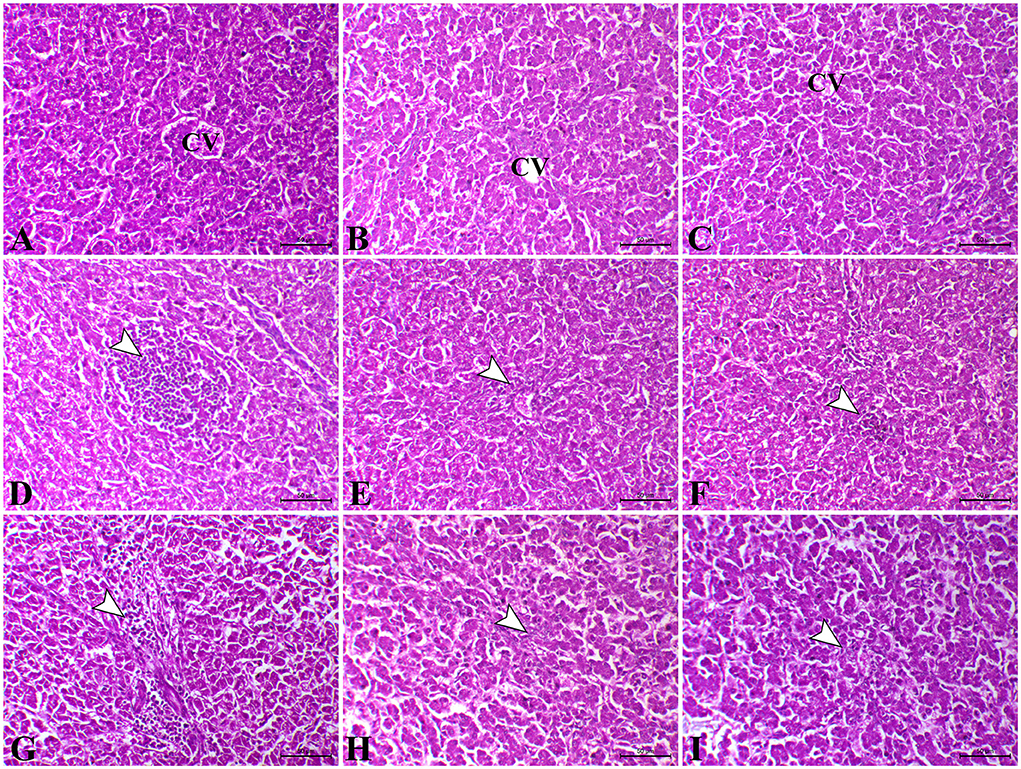
Figure 2. Photomicrograph of H&E stained the liver from control chicken showing normal hepatic parenchyma (A). The liver of L. plantarum chicken showed normal tissue architecture and cellular details (B). The liver of amylase-treated group showing normal hepatic parenchyma (C). The liver of E.coli-infected chicks on the 23rd day showing focal hepatic necrosis associated with infiltration of inflammatory cells (arrowhead) (D). The liver of E.coli-infected chicken treated with L. plantarum on the 23rd day showing slight perivascular inflammatory cells infiltration (arrowhead) (E). The liver of E.coli-infected chickens treated with amylase on the 23rd day showing congestion of sinusoids and tiny necrotic foci (arrowhead) (F). The liver of E.coli-infected chicken on the 37th day showing marked perivascular aggregation of inflammatory cells infiltration (arrowhead) and fibrosis (G). The liver of E.coli-infected chicken treated with L. plantarum on the 37th day showing congestion of hepatic sinusoids (arrowhead) (H). The liver of E.coli-infected chicken treated with amylase on the 37th day showing mild atrophy of some hepatocytes and severe congestion of hepatic blood vessels (arrowhead) (I). H&E, bar = 50 μm.
As shown in Figures 3A–C, on the 23rd and 37th days, the kidney of control, L. plantarum, and amylase enzyme chicks showed normal renal cortex and medulla. On the 23rd day, the kidney of E. coli-infected chicks revealed severe congestion of renal blood vessels with interstitial hemorrhage besides degenerative changes in the epithelial lining of the renal tubule (Figure 3D). Moreover, intrarenal hyaline casts depositing with degeneration of some renal epithelium were also detected in the kidney of E. coli infected chicks. Furthermore, on the 23rd day, the kidney sections of E. coli-infected chicks pre-treated with L. plantarum showed apparently normal renal cortex and medulla with mild focal intertubular hemorrhage (Figure 3E). Also, on the 23rd day, infected-E.coli chicken after amylase enzyme treatment showed normal renal cortex and medulla with cystic dilation of some renal tubules and moderate congestion of blood vessels (Figure 3F). While on the 37th day, the kidney sections of the E. coli infected group showed massive aggregation of leucocytic cells besides focal necrosis of renal tubules and congestion of renal blood vessels (Figure 3G). On the 37th day, the kidney sections E. coli-infected chicks pre-treated with L. plantarum that belong to the same group showed a reduction in renal lesions with few lesions still as degenerations and atrophy of some renal tubules (Figure 3H). While, on the 37th day, the kidney sections of infected-E. coli chicken after amylase enzyme treatment showed improvement of lesions with mild diffuse intertubular hemorrhage and mild congestion combined with degeneration of some renal tubules with hypercellularity of some renal glomeruli (Figure 3I).
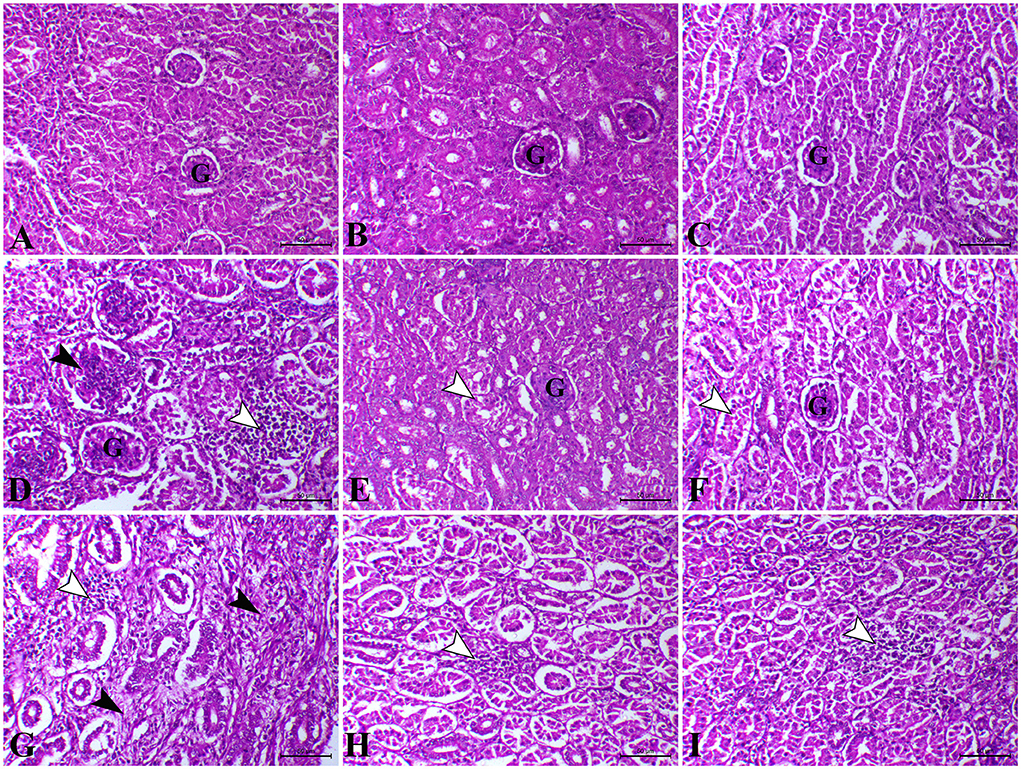
Figure 3. Photomicrograph of H&E stained kidney from control chicken shows the following: (A) Normal renal glomeruli and tubules; (B) The kidney of L. plantarum chicks showing normal renal glomeruli and tubules; (C) The kidney of amylase-treated chicken showing apparently normal renal glomeruli and tubules; (D) The kidney of E.coli-infected chicks at 23rd day showing marked interstitial nephritis (white arrowhead) associated with proliferative glomerulonephritis (black arrow);. (E) The kidney of E.coli-infected chicks treated with L. plantarum at 23rd day showing decreased tubular degeneration (arrowhead); (F) The kidney of E.coli-infected chicks treated with amylase at 23rd day showing a moderate degree of tubular degeneration (arrowhead); (G) The kidney of E.coli-infected chicks at 37th day showing interstitial nephritis associated with focal aggregation of leucocytic cells (white arrowheads) and interstitial fibrosis (black arrowheads); (H) The kidney of E.coli-infected chicks treated with L. plantarum at 37th day showing a mild degree of interstitial nephritis (arrowhead); (I) The kidney of E.coli-infected chicks treated with amylase at 37th day showing a mild degree of interstitial nephritis (arrowhead). H&E, bar = 50 μm.
As illustrated in Figures 4A,B, the intestine of control and L. Plantarum chicks on the 23rd and 37th days recorded normal mucosa and submucosa. Furthermore, chicks treated with the amylase enzyme, at the 23rd and 37th days, recorded normal mucosa and submucosal with elongated villi (Figure 4C). In contrast, the intestine of the E. coli-infected group, on the 23rd day, showed vacuolation of submucosal glandular epithelium besides edema among muscle fiber of muscularis mucosa (Figure 4D). However, on the 23rd day, the intestinal sections of E. coli-infected chicks treated with L. plantarum showed normal mucosa and submucosa combined with congestion of serosa blood vessels (Figure 4E). Diffuse metaplasia of intestinal enterocytes to goblet cells (mucinous degeneration) was noticed in the same later group. Also, the intestinal sections of E. coli-infected chicks administered with amylase enzyme showed elongated villi with or without mild mucinous degeneration (Figure 4F) on the 23rd day besides mild focal submucosal glandular atrophy and leucocytic cells infiltration. While, on the 37th day, the intestinal sections revealed severe inter-glandular and lamina propria inflammatory cells infiltration, mainly lymphocytes, besides atrophy of some intestinal glands (Figure 4G), and atrophy of some submucosal glands was also seen in some cases of E. coli-infected chicks. On the 37th day, the intestinal sections of some chicks belonging to E. coli-infected chicks treated with L. plantarum group exhibited normal intestinal layers with a fusion of some intestinal villi (Figure 4H). However, on the 37th day, the intestinal sections belonging to E. coli-infected chicks administered with amylase enzyme group showed apparently normal mucosal structure with focal infiltration of inflammatory cells in lamina propria (Figure 4I).
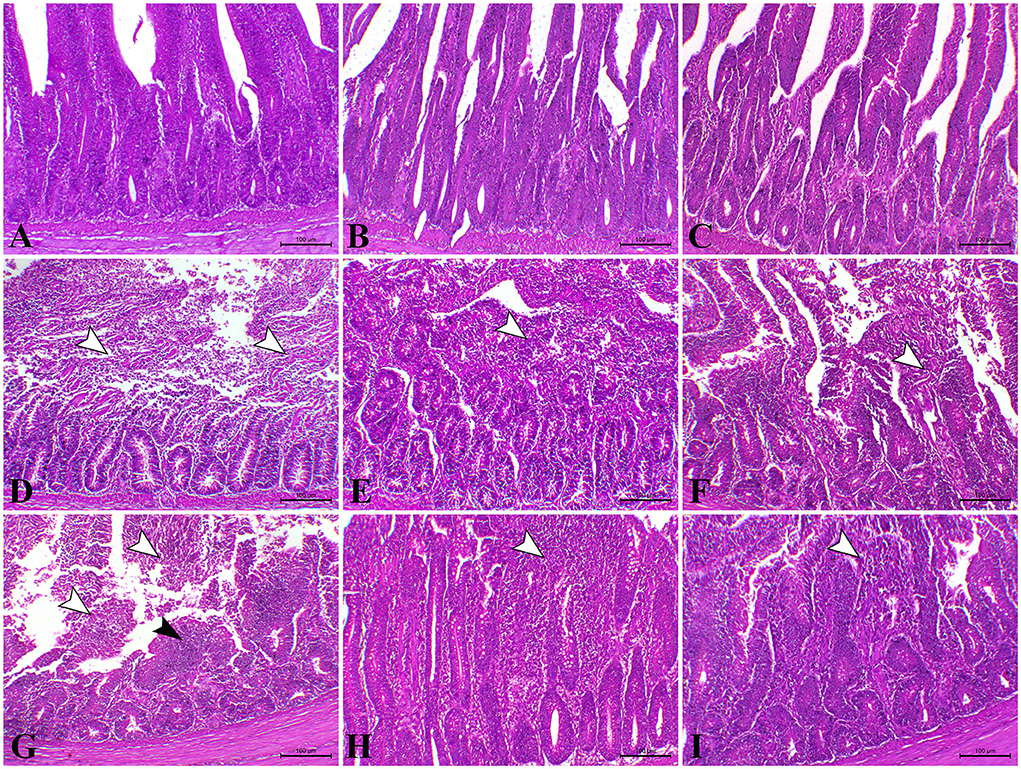
Figure 4. Photomicrograph of H&E stained intestinal section of control chicken shows the following: (A) Normal mucosal lining; (B) The intestine of chicken treated with L. plantarum showing normal mucosa and submucosa; (C) The intestine of chicken treated with amylase showing normal mucosa and submucosa; (D) The intestine of E.coli-infected chicken at 23rd day showing severe degree of necrotic enteritis (arrowheads); (E) The intestine of E.coli-infected chickens treated with L. plantarum at 23rd day showing decreased necrotic enteritis lesions (arrowhead); (F) the intestine of E.coli-infected chickens treated with amylase at 23rd day showing a moderate degree of necrotic enteritis (arrowhead) (G) The intestine of E.coli-infected infected chickens at 37th day showing necrotic enteritis associated with atrophy of intestinal villi (white arrowhead) with regenerative attempts of mucosa (black arrowhead); (H) The intestine of E.coli-infected chickens treated with L. plantarum at 37th day showing mild catarrhal enteritis (arrowhead); (I) The intestine of E.coli-infected chicken treated with amylase at 37th day showing a moderate degree of catarrhal enteritis (arrowhead).
Discussion
To ensure food free of drugs for consumers (41), feed additives have been used as alternatives to in-feed antibiotics to prevent the risk of developing pathogens. Probiotics and prebiotics are used as growth promoters, can be used as non-antibiotic feed additives substitute, and enhance the growth performance of broiler chickens. The present study revealed a series of interesting findings in relation to the modulatory effect of dietary probiotic and prebiotic supplementations on growth, immuno-biochemical alteration, DNA damage, and pathological changes in E. coli-infected broiler chicks, which was revealed in this present study. Consistent with several previous reports (42, 43), supplementation of probiotics (L. plantarum) and prebiotics (amylase enzyme) in diet improved the body performance parameters (BW, BWG, and FI) and decreased the FCR. Weight gain in the probiotic-treated group (G2 and G5) might result from preserving healthy intestinal flora by competitive exclusion and antagonism, increasing digestive enzyme activities, and encouraging the digestion rate of energy nutrients (44). Moreover, treatment of infected birds (G6) with exogenous enzymes (amylase enzyme) might have both direct and indirect actions by providing a suitable environment for the endogenous digestive enzymes to act on the substrate, adjustment of the intestinal microbial populations, speed feed passage rate by increasing the hydrolytic GIT capacity and increasing the availability of macronutrients that are resistant to digestion by endogenous enzymes, leading to enhancement of nutrient solubility, digestibility and availability [40]. The growth performance of E. coli-infected non-treated chicks (G4) revealed a significantly lower BW, BWG, and FI besides an increase in FCR in comparison with control chicks. These present results were consistent with those previously obtained by Liang et al. (45) and Wu et al. (46), who noticed a reduction of FI and growth retardation due to intestinal lesions resulting from the challenges with E. coli, which was associated with villous atrophy and intestinal morphology disorder and consequently reduced nutrients absorption and/or hepatic lesions or kidney dysfunction (42, 47). In addition, the groups infected with E. coli and treated with L. plantarum and amylase enzyme (G5&G6) showed an improvement in body performance and reduction in the FCR as compared to the infected non-treated group. These findings are in agreement with previous studies (48).
It is noteworthy to mention that the liver enzymes (ALT, AST, and ALP) are among the most common indicators of hepatic functions. Our result revealed a significant decrease in ALT, AST, and ALP in treated groups with L. plantarum and amylase enzyme (G2&G3) than in control, which may be attributed to the hepatoprotective effect associated with different xenobiotics (49–51). A significant increase in the liver enzymes was noticed in E. coli-infected non-treated birds all over the experimental period, which may be attributed to hepatocellular damage during the detoxification of E. coli and bacterial toxins and may have led to hepatocyte membrane disruption associated with leakage of hepatocyte contents and, therefore, the targeted elevation of the serum hepatic enzymes (52). On the contrary, the E. coli-infected groups treated with L. plantarum and amylase enzyme (G5 and G6) had a significant decrease in the liver enzymes than the infected non-treated group. The hepatoprotective effect of both probiotics and prebiotics may be due to both antimicrobial and antioxidant effects and enhanced immune status that decreased the pathogenic bacterial population (53). Regarding the renal function parameters, serum creatinine, urea, and uric acid were assessed, and the present study revealed a significant decrease in serum creatinine, urea, and uric acid in G2 and G3 in all experimental periods, which were consistent with several previous reports (54, 55). However, other studies (56, 57) reported that the addition of either probiotics or prebiotics had no significant change in renal enzymes. This contradiction could be attributed to the type, number, and strain of bacteria present in probiotics and the types of exogenous enzymes used in the prebiotic. There is an increase in serum renal function parameters in the E. coli-infected non-treated group compared with the other groups (58), which may be attributed to renal damage, imbalance of protein metabolism, and imbalance of amino acids concentration (53). Both G5 and G6 showed improvement of renal functional tests that may be explained through decreasing urea synthesis, and the improvement of protein metabolism balance (59).
The present study revealed that L. plantarum and amylase enzyme-treated birds demonstrated a significant increase in total protein serum level, albumin, and total globulins when compared with the control group. Probiotics were reported to be associated with an increased anabolic than the catabolic process by increasing the absorptive capacity of the intestine due to histomorphological changes and higher intestinal enzyme activity (60–63). In addition, probiotics increased total globulins, which play a great role in the host's immune system (5). Conversely, the E. coli-infected non-treated birds (G4) showed a significant decrease in serum total protein and albumin over the experiment period as compared with the control group. The total globulins in this group showed a significant increase on the 23rd day and then was followed by a significant decrease on the 37th day. Data were supported by the previously obtained results (64). Damage to the intestinal barrier encourages systemic bacterial population, which in turn results in the liver damage, intestinal malabsorption, and kidney dysfunction associated with excessive degradation of plasma protein by bacterial endotoxins (52). The initial increase of total globulin could be associated with antigenic stimulation of the infecting microorganism, while the later decrease of globulin levels was accompanied by the liver damage (56). Serum IgA is considered one of the most important humoral factors produced by B-cells. Therefore, this acts as the first line of defense against infection (65). Our data revealed a significant increase in serum IgA levels in G2 and G3 as compared with the control, which is consistent with several previous studies (57, 66). The E. coli-infected non-treated birds (G4) revealed an initial increase in serum IgA levels on the 23rd day, which was then decreased on the s37th day. There is some evidence that infection stimulates the immune system to a limited extent, leading to an increase in serum IgA. With time, the infection suppresses the immune system, leading to a decreased serum IgA (46, 67). The infected treated birds (G5 and G6) showed enhanced serum IgA levels as compared to G4. This indicates that the use of probiotics and prebiotics might stimulate the intestinal mucosa to produce more IgA after the E. coli challenge, which blocks the receptors and diminishes the number of pathogenic bacteria in the intestinal lumen (42).
Importantly, cytokines are immunoregulatory peptides that contribute to innate and adaptive immunity, and therefore, they play an essential role in immunoregulation (68). IL-6 is a pleiotropic cytokine produced by T cells and macrophages. This cytokine simulates the immune response, e.g., during infection and any tissue damage causing inflammation, and has proinflammatory and anti-inflammatory roles, which are critical in fighting infection (69).
In the present study, birds treated with L. plantarum and amylase enzyme (G2 and G3) showed a significant decrease in IL6 compared to chickens fed with normal ration. Similarly, a previous study (70) indicated that probiotic supplementation in a piglet diet led to decreased proinflammatory cytokines. In contrast, chicken challenged with E. coli (G4) showed a significant increase in serum IL6, which is consistent with a previous study (71), and the possible explanation could be due to the infection with bacteria, as E. coli enhanced production of extreme levels of IL6 (72, 73). It should be noted that E. coli has molecular patterns on its surface, such as lipopolysaccharides (LPS) and flagellin that bind with toll-like receptors on macrophage surfaces, causing interleukins to be secreted (IL-1, IL-6, and IL-8) (74, 75). Meanwhile, the infected groups supplemented with probiotic and prebiotic (G5 and G6) showed a significant improvement in serum IL6 compared with G4. Present data are supported by previous studies (65, 76), recording the intake of probiotics by E. coli-infected birds which enhanced the production of proinflammatory cytokines such as IL-1, TNF-α, and IL-6. This study also showed a significant increase in phagocytic percentage and phagocytic index in G2 and G3 on the 23rd and 37th days. These findings are in line with a previous study (57) that found dietary supplementation with both multi-enzyme or probiotic preparations either singly or in combination could enhance phagocytic activity and phagocytic index of broiler chicks than control. On the contrary, the control positive group (G4) birds showed a significant decrease in phagocytic percentage and phagocytic index at the 23rd and 37th days of age compared with the control negative group. These findings were consistent with previous study (77), which attributed these findings to bacterial endotoxins that caused pathophysiological effects and induced suppression of antibacterial defense mechanism or/and may be due to exhaustion of immune system by E. coli infection (68). The E. coli-infected birds treated with L. plantarum and amylase enzyme were significantly higher in phagocytic activity and phagocytic index as compared with the E. coli-infected non-treated birds. The improvement in phagocytic activity indicated that probiotics or prebiotics directly promote maturation and activation of macrophages (55).
Malondialdehyde is considered one of the markers for oxidative damage of the lipid peroxidation level (78). Compared to normal control birds, the serum MDA levels significantly decreased in probiotic and prebiotic treated groups (G2 and G3), which may be attributed to the positive modulation of the dynamics of oxidants and antioxidants in the body of chickens. It seems that the enhancement of the intestinal flora would selectively improve the ability to chelate free radicals, capturing reactive oxygen species (ROS) and inhibiting their cytotoxic activities (79). Similar results were previously reported by Wu et al. (51) and Saleh et al. (43). Consistent with results obtained in a previous study (71, 80), serum MDA significantly increased in E. coli-infected chickens (G4). This increase in the serum MDA may be attributed to the E. coli endotoxins, which induced extensive damage to a variety of organs, including the liver, overproduction of ROS, and reactive nitrogen species targeting oxidative stress through cell damage as it can attack protein and nucleic acid of the cell. MDA can also attack the polyunsaturated fatty acids of the membrane lipids, which in turn trigger lipid peroxidation and increase the activity of antioxidant enzymes to minimize the effect of ROS by the production of antioxidants (55). G5 and G6 infected with E. coli and treated with L. plantarum and amylase enzyme showed a significant decrease in serum MDA levels compared with the infected group. This observation could be attributed to the antioxidant effect of the supplemented probiotic and prebiotic that confer sufficient protection against lipid peroxidation, increased glutathione concentrations, and reduced intestinal oxidation (8, 55).
Serum SOD and CAT enzymes play a critical role in the protection of the cell from oxidative damage by ROS. SOD is an enzyme found in all living cells, and it degrades the potentially harmful oxygen molecules in cells through the decomposition of hydrogen peroxide into water and oxygen. This present study showed an increase in serum SOD and catalase activity in probiotic- and prebiotic-treated chickens, suggesting the modulated dynamics of oxidants and antioxidants by improved gut microbes and that microbes, in turn, released some bioactive substances that could potentially prevent oxidative damage (81). The same results were recorded by He et al. (82) and Wang et al. (66). On the other hand, E. coli-infected non-treated chickens (G4) showed a significant decrease in serum SOD and catalase activity, which is nearly similar to the results that were obtained (64). The infection decreased the antioxidant defense system, which could be compensated by the cellular defense systems, or these reactive compounds may inhibit the enzyme activity, leading to a significant decrease in SOD and catalase activities, which catalyze the dismutation of the superoxide anion into hydrogen peroxide. Molecular oxygen is considered one of the most potent antioxidant. As mentioned above, catalase is a widely distributed enzyme that destroys hydrogen peroxide ROS, which is a toxic product of both normal aerobic metabolism and pathogenic ROS production (81). In the present study, the groups infected by E. coli and treated with L. plantarum and amylase enzyme (G5 and G6) showed a significant elevation in serum SOD and catalase activity in comparison with the control positive group (G4). Our present findings are partially in agreement with the results of Dong et al. (83), who illustrated that probiotics could enhance the activity of SOD in chickens infected with E. coli, and treated with probiotic than infected non-treated chickens. It is therefore not surprising to state that the addition of probiotics and prebiotics might play a critical role in the recovery of intestinal linings that are in continuous interaction with the microorganisms and inhibit excess oxidative free radicals that may cause cell damage (84).
From the available literature studies, comet assay is widely recognized as a sensitive technique for studying DNA damage and repair. In this assay, cells with high DNA damage show the migration of chromosomal DNA from the nucleus toward the anode like the shape of a comet. The results of the current study indicated that dietary L. plantarum supplementation reduced DNA damage in the liver and the intestinal tissues than the control. The obtained data are in keeping with some previous reports (85, 86), recording that probiotics can reduce DNA damage caused by any endogenous or external source of stress. The possible explanation was attributed to the role of probiotics in the detoxification and elimination of chemicals and heavy metal toxicity in the body (87). Probiotics act as free radical scavengers and antioxidants by decreasing the oxidative stress that causes DNA damage and genotoxicity, which DNA fragmentations in the comet assay, leading to excision and repair of oxidized bases (88). The group treated with prebiotic (G3) showed non-significant difference in DNA degradation at the 23rd and 37th days of age compared with the control group. These results were in stark contrast with those obtained (89, 90). In contrast, the E. coli-infected non-treated group (G4) showed high DNA damage in the intestine and the liver, which was consistent with several previous reports, revealing that E. coli strain induced DNA double-strand breaks and chromosomal abnormalities in eukaryotic cells (80, 91, 92). Another study (93) reported that the endotoxin shock induced by E. coli infection was responsible for the overexpression of apoptosis-related genes increasing DNA damage to the liver and the brain cells of laying hens. This may result from the activation of the caspase-9- and caspase-3-dependent branch of the apoptotic pathway in cell line during the uptake and digestion of E. coli bacteria. Likewise, the proteolytic action of the caspase family on specific cell substrates led to apoptosis including DNA damage (94). In addition, we should note that oxidative stress induced by E. coli infection induced high ROS production, which resulted in DNA strand breaks (80). The infected birds treated with probiotic were more effective in genoprotective effect against DNA damage compared with G4 in the present study. This concept that L. plantarum supplementation improved DNA damage caused by E. coli infection in broilers was supported by the findings of a previous study (6). The present findings are consistent with the hypothesis that suggests probiotics increase intestinal acidity, which is conducive to reducing the pathogen population in the gut of chickens, and therefore, leading to the reduction of DNA damage caused by infection (95). However, we should note that a partial genoprotective effect against DNA damage was observed in infected birds treated with amylase enzyme compared with G4. The limited genoprotective role of the addition of exogenous enzymes in this study is attributed to the insufficiency of enzyme activity and dosage used. Our findings in this study revealed severe alteration of hepatic, renal, and intestinal tissues in the E. coli-infected non-treated group (G4). The hepatic tissue of this group showed multiple necrotic foci associated with the aggregation of mononuclear cells. Inflammation was extended to renal tissues associated with interstitial nephritis. In addition, the main lesion was noticed as severe necrotic enteritis. This lesion was consistent with several previous works (64, 96). Interestingly, using L. plantarum and amylase enzyme as a prophylactic against E. coli revealed a marked decrease in the inflammatory lesions within the liver, the kidney, and the intestine which is consistent with several previous reports (44, 97).
Conclusion
This study concluded that the experimental infection with E. coli strain O78 in chicks caused severe alterations in the body performance, biochemical, immunological, antioxidant, oxidative stress parameters, and histological structures with extensive DNA degeneration. Interestingly, dietary supplementation with probiotics and prebiotics improved the efficiency of poultry production with body performance and increased immune response in non-infected bird groups. Given the above information, probiotics and prebiotics might have a promising effect as prophylactic supplementation for controlling E. coli infection through the improvement of growth performance and returning the aforementioned parameters to near normal values with histopathological change subsidence and DNA genotoxicity.
Data availability statement
The original contributions presented in the study are included in the article/supplementary material, further inquiries can be directed to the corresponding author.
Ethics statement
The animal study was reviewed and approved by the Institutional Review Board of the faculty of Veterinary Medicine (Local Ethical Approval), Zagazig University, Egypt. The Approval number of the study is ZU-IACUC/2/F/88/2021.
Author contributions
MH, AH, HA-E, WA, and EE conceived the idea and performed the methodology, formal analysis, data curation, and supervision besides revision of the manuscript. WA, ND, AA, and EE participated in the formal analysis, data curation, and contributed their scientific advice. MH, AH, HA-E, WA, ND, AA, and EE drafted the manuscript and prepared the manuscript for publication and revision. All authors have read and agreed to the published version of the manuscript.
Funding
This work was supported by the Faculty of Veterinary Medicine, Zagazig University, Egypt.
Conflict of interest
The authors declare that the research was conducted in the absence of any commercial or financial relationships that could be construed as a potential conflict of interest.
Publisher's note
All claims expressed in this article are solely those of the authors and do not necessarily represent those of their affiliated organizations, or those of the publisher, the editors and the reviewers. Any product that may be evaluated in this article, or claim that may be made by its manufacturer, is not guaranteed or endorsed by the publisher.
References
1. Abo El-magd E, Sallam K, Ramadan H. Prevalence of Escherichia coli in chicken carcasses from Mansoura, Egypt. Mansoura Vet Med J. (2019) 2:41–44. doi: 10.21608/mvmj.2019.01.109
2. Ahmed MA, Youssef FM, Rahman A. Differentiation between E. colis strains causing diarrhea in broiler chicken by using multiplex PCR. In: Proc. 6th Inter. Conf. Vet. Res. Div. Cairo, Egypt: NRC. (2013) p. 33-47.
3. Goswami P, Chakrabarti A, Hui AK, Das R, Ray N. Isolation identification and antibiogram of Escherichia coli from poultry in field conditions. J Interacademia. (2004) 81:680–3.
4. Pirgozliev V, Rose SP, Ivanova S. Feed additives in poultry nutrition. Bulg J Agric Sci. (2019) 25:8–11.
5. Pourakbari M, Seidavi A, Asadpour L, Martínez A. Probiotic level effects on growth performance, carcass traits, blood parameters, cecal microbiota, and immune response of broilers. Anais da Academia Brasileira de Ciências. (2016) 88:1011–21. doi: 10.1590/0001-3765201620150071
6. Ding S, Wang Y, Yan W, Li A, Jiang H, Fang J. Effects of Lactobacillus plantarum 15-1 and fructooligosaccharides on the response of broilers to pathogenic Escherichia coli O78 challenge. PLoS ONE. (2019) 14:e0212079. doi: 10.1371/journal.pone.0222877
7. Patterson J, Burkholder K. Application of prebiotics and probiotics in poultry production. Poult Sci. (2003) 82:627–31. doi: 10.1093/ps/82.4.627
8. Sohail M, Rahman Z, Ijaz A, Yousaf M, Ashraf K, Yaqub T, et al. Single or combined effects of mannan-oligosaccharides and probiotic supplements on the total oxidants, total antioxidants, enzymatic antioxidants, liver enzymes, and serum trace minerals in cyclic heat-stressed broilers. Poult Sci. (2011) 90:2573–7. doi: 10.3382/ps.2011-01502
9. Pirgozliev V, Bedford M. Energy utilisation and growth performance of chicken fed diets containing graded levels of supplementary bacterial phytase. Br J Nutr. (2013) 109:248–53. doi: 10.1017/S0007114512000943
10. Onderci M, Sahin N, Sahin K, Cikim G, Aydin A, Ozercan I, et al. Efficacy of supplementation of α-amylase-producing bacterial culture on the performance, nutrient use, and gut morphology of broiler chickens fed a corn-based diet. Poult Sci. (2006) 85:505–10. doi: 10.1093/ps/85.3.505
11. Abdulla JM, Rose SP, Mackenzie AM, Pirgozliev VR. Feeding value of field beans (Vicia faba L. var minor) with and without enzyme containing tannase, pectinase and xylanase activities for broilers. Arch Anim Nutr. (2017) 71:150–64. doi: 10.1080/1745039X.2017.1283823
12. Dhama K, Mahendran M, Tomar S, Chauhan R. Beneficial effects of probiotics and prebiotics in livestock and poultry: the current perspectives. Intas Polivet. (2008) 9:1–12.
13. Dhama K, Verma V, Sawant P, Tiwari R, Vaid R, Chauhan R. Applications of probiotics in poultry: enhancing immunity and beneficial effects on production performances and health-a review. J Clin Immunol. (2011) 13:1–19. Available online at: https://www.researchgate.net/publication/229596530
14. Al-Khalaifa H, Al-Nasser A, Al-Surayee T, Al-Kandari S, Al-Enzi N, Al-Sharrah T, et al. Effect of dietary probiotics and prebiotics on the performance of broiler chickens. Poult Sci. (2019) 98:4465–79. doi: 10.3382/ps/pez282
15. Quinn PJ, Carte ME, Markeryo B, Carter GR. Clinical Veterinary. Microbial. Year book-wolf publishing- Europe Limited. (1994).
16. Lublin A, Mechani S, Malkinson M, Weisman Y. Efficacy of norfloxacin nicotinate treatment of broiler breeders against Haemophilus paragallinarum. Avian Dis. (1993) 673–679. doi: 10.2307/1592014
17. Sekizaki T, Nonomura L, Imada Y. Loss of virulence associated with plasmid curing of chickens pathogenic Escherichia coli. Avian Dis J. (1989) 51:659–61. doi: 10.1292/jvms1939.51.659
18. Fernandez A, Lara C, Loste A, Marca M. Efficacy of calcium fosfomycin for the treatment of experimental infections of broiler chickens with Escherichia coli O78: K80. Vet Res Commun. (2002) 26:427–36. doi: 10.1023/A:1020582207129
19. El-Dahshan HA. Compartive Clinico-Pathological Studies on some Immunostimulants With Relation to some Poultry Diseases. M.V.Sc Thesis, Faculty of Veterinary Medicine Suez Canal University. (2002).
20. National research Council. Nutrition Requirements of Poultry.9th Ed. Washington,D C: National Academy Press. (1994).
21. Giambrone J, Clay RP. Vaccination of day-old broiler chicks against Newcastle disease and infectious bursal disease using commercial live and/or inactivated vaccines. Avian Diseases. (1986) 30:557–561. doi: 10.2307/1590421
22. Abd El-Rahman M. The prevention of E. coli infection in broilers by using antimicrobial feed additives. Ph. Thesis. D, Fac. of Vet. Med. Zagazig University. (1999).
23. Amer SAA, Nasser A, Al-Khalaifah HS, AlSadek DM, Abdel Fattah DM, Roushdy EM, et al. Effect of dietary medium-chain α-monoglycerides on the growth performance, intestinal histomorphology, amino acid digestibility, and broiler chickens' blood biochemical parameters. Animals. 11:57. doi: 10.3390/ani11010057
24. Wagner D, Furrow R, Bradley B. Subchronic toxicity of monensin in broiler chickens. Vet Pathol. (1983) 20:353–9. doi: 10.1177/030098588302000311
25. Reitman S, Frankel S. A colorimetric method for the determination of serum glutamic oxalacetic and glutamic pyruvic transaminases. Am J Clin Pathol. (1957) 28:56–63. doi: 10.1093/ajcp/28.1.56
26. Tietz NW. Clinical guide to laboratory tests. J Transfus. (1995) 35:972. doi: 10.1111/j.1537-2995.1995.tb03571.x
27. Henry. Clinical Chemistry Principles and Techniques Colorometric Estemiation of Creatinine, 2nd edn. Hagerstown, MD: Harper and Raw (1974).
28. Fossati P, Prencipe L, Berti G. Use of 3, 5-dichloro-2-hydroxybenzenesulfonic acid/4-aminophenazone chromogenic system in direct enzymic assay of uric acid in serum and urine. Clin Chem. (1980) 26:227–31. doi: 10.1093/clinchem/26.2.227
30. Doumas BT, Bayse DD, Carter RJ, Peters T Jr, Schaffer R. A candidate reference method for determination of total protein in serum. I Development and validation. Clini Chemist. (1981) 27:1642–50. doi: 10.1093/clinchem/27.10.1642
32. Wilkinson P. Recognition and response in mononuclear and granular phagocytes. Clinical and Experimental Immunology. Clin Exp Immunol. (1976) 25:3.
33. Bianchi A, Moonen-Leusen H, Van der Heijden P, Bokhout B. The use of a double antibody sandwich ELISA and monoclonal antibodies for the assessment of porcine IgM, IgG and IgA concentrations. Vet Immunol Immunopathol. (1995) 44:309–17. doi: 10.1016/0165-2427(94)05307-E
35. Ohkawa H, Ohishi N, Yagi K. Assay for lipid peroxides in animal tissues by thiobarbituric acid reaction. Anal Biochem. (1979) 95:351–8. doi: 10.1016/0003-2697(79)90738-3
36. Aebi H. Methods enzymol for determination of hydrogen peroxide. Clini Chemist. (1984) 105:121–6. doi: 10.1016/S0076-6879(84)05016-3
37. Martin JP, Dailey M, Sugarman E. Negative positive assays of superoxide dismutase based on hematoxylin autoxidation. Arch Biochem Biophys. (1987) 255:329–36. doi: 10.1016/0003-9861(87)90400-0
38. Singh NP, McCoy MT, Tice RR, Schneider EL. A simple technique for quantitation of low levels of DNA damage in individual cells. Exp Cell Res. (1988) 175:184–91. doi: 10.1016/0014-4827(88)90265-0
39. Suvarna SK, Layton C, Bancroft JD. Bancrofts Theory and Practice of Histological Technique. 7th ed. Churchill Livingstone, England: Elsevier. (2013).
40. Tamhane AF, Dunlop DD. Statistics and Data Analysis from Elementary to intermediate. New Jersey USA: Prentice Hall, Upper Saddle River. (2000).
41. Popova T. Effect of probiotics in poultry for improving meat quality. Curr Opin Food Sci. (2017) 14:72–7. doi: 10.1016/j.cofs.2017.01.008
42. Wang S, Peng Q, Jia H, Zeng X, Zhu J, Hou C, et al. Prevention of Escherichia coli infection in broiler chickens with Lactobacillus plantarum B1. Poult Sci. (2017) 96:2576–86. doi: 10.3382/ps/pex061
43. Saleh AA, Kirrella AA, Abdo SE, Mousa MM, Badwi NA, Ebeid TA, et al. Effects of dietary xylanase and arabinofuranosidase combination on the growth performance, lipid peroxidation, blood constituents, and immune response of broilers fed low-energy diets. Animals. (2019) 9:467. doi: 10.3390/ani9070467
44. Mohamed FM, Thabet MH, Ali MF. The use of probiotics to enhance immunity of broiler chicken against some intestinal infection pathogens. SVU-IJVS. (2019) 2:1–19. doi: 10.21608/svu.2019.23141
45. Liang W, Li H, Zhou H, Wang M, Zhao X, Sun X, et al. Effects of Taraxacum and Astragalus extracts combined with probiotic Bacillus subtilis and Lactobacillus on Escherichia coli–infected broiler chickens. Poult Sci. (2021) 100:101007. doi: 10.1016/j.psj.2021.01.030
46. Wu Z, Yang K, Zhang A, Chang W, Zheng A, Chen Z, et al. Effects of Lactobacillus acidophilus on the growth performance, immune response, and intestinal barrier function of broiler chickens challenged with Escherichia coli O157. Poult Sci. (2021) 100:101323. doi: 10.1016/j.psj.2021.101323
47. Cao G, Zeng X, Chen A, Zhou L, Zhang L, Xiao Y, et al. Effects of a probiotic, Enterococcus faecium, on growth performance, intestinal morphology, immune response, and cecal microflora in broiler chickens challenged with Escherichia coli K88. Poult Sci. (2013) 92:2949–55. doi: 10.3382/ps.2013-03366
48. Azza A, Dahshan AHM, El-Nahass E-S, Abd El-Mawgoud A. Pathogenicity of Escherichia coli O157 in commercial broiler chickens. Beni-Suef Univ J Basic Appl Sci. (2018) 7:620–5. doi: 10.1016/j.bjbas.2018.07.005
49. Safaa H. Influence of dietary enzymes prepared at ensiling (ZADO®) from hatch to 42 days of age on productivity, slaughter traits and blood constituents in broiler chickens. Int J Poult Sci. (2013) 12:529. doi: 10.3923/ijps.2013.529.537
50. Manafi M, Khalaji S, Hedayati M, Pirany N. Efficacy of Bacillus subtilis and bacitracin methylene disalicylate on growth performance, digestibility, blood metabolites, immunity, and intestinal microbiota after intramuscular inoculation with Escherichia coli in broilers. Poult Sci. (2017) 96:1174–83. doi: 10.5772/61986
51. Wu Y, Wang B, Zeng Z, Liu R, Tang L, Gong L, et al. Effects of probiotics Lactobacillus plantarum 16 and Paenibacillus polymyxa 10 on intestinal barrier function, antioxidative capacity, apoptosis, immune response, and biochemical parameters in broilers. Poult Sci. (2019) 98:5028–39. doi: 10.3382/ps/pez226
52. Sonwane S, Ingole R, Hedau M, Rathod P, Hajare S, Ingawale M. Ameliorative effect of Andrographis paniculata on hematobiochemical parameters in Escherichia coli induced broilers. J Pharmacogn Phytochem. (2017) 6:1284–8.
53. Abdelhady DH, El-Abasy MA. Effect of Prebiotic and Probiotic on Growth, Immuno-hematological responses and Biochemical Parameters of infected rabbits with Pasteurella multocida. Benha Vet Med J. (2015) 28:40–51. doi: 10.21608/bvmj.2015.31859
54. Hajati H, Rezaei M, Sayyahzadeh H. The effects of enzyme supplementation on performance, carcass characteristics and some blood parameters of broilers fed on corn-soybean meal-wheat diets. Int J Poult Sci. (2009) 8:1199–205. doi: 10.3923/ijps.2009.1199.1205
55. Abd-El Rhman Shawkat L, Mohammed Fararh K, Samir Fari A. Effect of probiotics and chelated zinc on E. coli infected broilers. Benha Vet Med J. (2018) 35:510–25. doi: 10.21608/bvmj.2018.96446
56. Abd-El-Rahman AH, Kamel H, Ahmed WM, Mogoda O, Mohamed AH. Effect of Bactocell and revitilyte-plus as probiotic food supplements on tm the growth performance, hematological, biochemical parameters and humoral immune response of Broiler Chickens. Sci J. (2012) 3:305–16.
57. Ali RA, El-Shafey AS, El-Kelawy M. Immunophsiological and productive response of broiler chicks to dietary supplementation with multi-enzyme and /or probiotics. Egypt Poultry Sci. (2018) 38:1047–67. doi: 10.21608/epsj.2018.22691
58. Huff G, Huff W, Rath N, Anthony N, Nestor K. Effects of Escherichia coli challenge and transport stress on hematology and serum chemistry values of three genetic lines of turkeys. Poult Sci. (2008) 87:2234–41. doi: 10.3382/ps.2008-00128
59. Luo D, Yang F, Yang X, Yao J, Shi B, Zhou Z. Effects of xylanase on performance, blood parameters, intestinal morphology, microflora and digestive enzyme activities of broilers fed wheat-based diets. Asian-Australas J Anim Sci. (2009) 22:1288–95. doi: 10.5713/ajas.2009.90052
60. Goda AMA, Mabrouk HA-HH, Wafa MAE-H, El-Afifi TM. Effect of using baker's yeast and exogenous digestive enzymes as growth promoters on growth, feed utilization and hematological indices of Nile tilapia, Oreochromis niloticus fingerlings. J Agric Sci Technol. (2012) 11:15–28. doi: 10.1016/0022-1759(86)90354-6
61. El-Sanhoury M, Ahmed A. Broiler performance, enzymes activity and histological observations affected by multi enzymes complex (ZADO®). Egypt J Nutr Feeds. (2017) 20:309–20. doi: 10.21608/ejnf.2017.75216
62. Saleh AA, El-Far AH, Abdel-Latif MA, Emam MA, Ghanem R, Abd El-Hamid HS. Exogenous dietary enzyme formulations improve growth performance of broiler chickens fed a low-energy diet targeting the intestinal nutrient transporter genes. PLoS ONE. (2018) 13:e0198085. doi: 10.1371/journal.pone.0198085
63. Yazhini P, Visha P, Selvaraj P, Vasanthakumar P, Chandran V. Dietary encapsulated probiotic effect on broiler serum biochemical parameters. Vet world. (2018) 11:1344. doi: 10.14202/vetworld.2018.1344-1348
64. Kumari M, Gupta RP, Lather D, Bagri P. Ameliorating effect of Withania somnifera root extract in Escherichia coli–infected broilers. Poult Sci. (2020) 99:1875–87. doi: 10.1016/j.psj.2019.11.022
65. Wold AE. Immune effects of probiotics. Näringsforskning. (2001) 45:76–85. doi: 10.3402/fnr.v45i0.1787
66. Wang Y, Heng C, Zhou X, Cao G, Jiang L, Wang J, et al. Supplemental Bacillus subtilis DSM 29784 and enzymes, alone or in combination, as alternatives for antibiotics to improve growth performance, digestive enzyme activity, anti-oxidative status, immune response and the intestinal barrier of broiler chickens. Br J Nutr. (2021) 125:494–507. doi: 10.1017/S0007114520002755
67. Reid G, Charbonneau D, Erb J, Poehner R, Gonzalez S, Gardiner G, et al. Ability of Lactobacillus GR-1 and RC-14 to stimulate host defences and reduce gut translocation and infectivity of Salmonella typhimurium. Prev Nutr Food Sci. (2002) 7:168–73. doi: 10.3746/jfn.2002.7.2.168
68. Lee K-W, Li G, Lillehoj HS, Lee S-H, Jang SI, Babu US, et al. Bacillus subtilis-based direct-fed microbials augment macrophage function in broiler chickens. Res Vet Sci. (2011) 91:e87–91. doi: 10.1016/j.rvsc.2011.01.018
69. Arango Duque G, Descoteaux A. Macrophage cytokines: involvement in immunity and infectious diseases. Front Immunol. (2014) 5:491. doi: 10.3389/fimmu.2014.00491
70. Li W, Huang Y, Li Y, Huang Q, Cui Z, Yu D, et al. Effect of oral administration of En:terococcus faecium Ef1 on innate immunity of sucking piglets. Pak Vet J. (2012) 33:9−13.
71. Kilany O, Youssef F, Mabrouk M, Fares I. Clinicopathological studies on the effect of some antibacterial medicinal plants in broilers. J Clin Pathol Forecast. (2018) 2018:1003.
72. Nakamura K, Mitarai Y, Yoshioka M, Koizumi N, Shibahara T, Nakajima Y. Serum levels of interleukin-6, alpha1-acid glycoprotein, and corticosterone in two-week-old chickens inoculated with Escherichia coli lipopolysaccharide. Poult Sci. (1998) 77:908–11. doi: 10.1093/ps/77.6.908
73. Aldapa-Vega G, Pastelín-Palacios R, Isibasi A, Moreno-Eutimio MA, López-Macías C. Modulación de la respuesta inmune por los lipopolisacáridos bacterianos. Revista Alergia México. (2016) 63:293–302. doi: 10.29262/ram.v63i3.207
74. Elnagar R, Elkenany R, Younis G. Interleukin gene expression in broiler chickens infected by different Escherichia coli serotypes. Vet World. (2021) 14:2727. doi: 10.14202/vetworld.2021.2727-2734
75. Abou Elazab MF, Nasr NE, Ahmed MS, Alrashdi BM, Dahran N, Alblihed MA, et al. The Effects of Bacterial Lipopolysaccharide (LPS) on Turkey Poults: Assessment of Biochemical Parameters and Histopathological Changes. Vet Sci. (2022) 9:240. doi: 10.3390/vetsci9050240
76. Guo M, Hao G, Wang B, Li N, Li R, Wei L, et al. Dietary administration of Bacillus subtilis enhances growth performance, immune response and disease resistance in Cherry Valley ducks. Front Microbiol. (2016) 7:1975. doi: 10.3389/fmicb.2016.01975
77. Abd El-Tawab AA, El-komy AA, El-Ekhnawy KI, Talaie AT. Effect of fosfomycin on E. coli O78 isolated from broiler chickens in-vitro and in-vivo. Benha Vet Med J. (2015) 28:294–300. doi: 10.21608/bvmj.2015.32501
78. Puvača N, Kostadinović L, Popović S, Lević J, Ljubojević D, Tufarelli V, et al. Proximate composition, cholesterol concentration and lipid oxidation of meat from chickens fed dietary spice addition (Allium sativum, Piper nigrum, Capsicum annuum). Animal Prod Sci. (2015) 56:1920–1927. doi: 10.1071/AN15115
79. Deraz SF, Elkomy AE, Khalil AA. Assessment of probiotic-supplementation on growth performance, lipid peroxidation, antioxidant capacity, and cecal microflora in broiler chickens. J Appl Pharm Sci. (2019) 9:030–9. doi: 10.7324/JAPS.2019.S104
80. Abbas AO, Alaqil AA, El-Beltagi HS, El-Atty A, Hanaa K, Kamel NN. Modulating laying hens productivity and immune performance in response to oxidative stress induced by E. coli challenge using dietary propolis supplementation. Antioxidants. (2020) 9:893. doi: 10.3390/antiox9090893
81. Abudabos A, Alyemni A, Zakaria H. Effect of two strains of probiotics on the antioxidant capacity, oxidative stress, and immune responses of Salmonella-challenged broilers. Brazilian J Poult Sci. (2016) 18:175–80. doi: 10.1590/18069061-2015-0052
82. He T, Long S, Mahfuz S, Wu D, Wang X, Wei X, et al. Effects of probiotics as antibiotics substitutes on growth performance, serum biochemical parameters, intestinal morphology, and barrier function of broilers. Animals. (2019) 9:985. doi: 10.3390/ani9110985
83. Dong Z, Wang Y, Song D, Wang W, Liu K, Wang L, et al. Effects of microencapsulated probiotics and plant extract on antioxidant ability, immune status and caecal microflora in Escherichia coli K88-challenged broiler chickens. Food Agric Immunol. (2019) 30:1123–34. doi: 10.1080/09540105.2019.1664419
84. Rajput IR, Li WF, Li YL, Jian L, Wang MQ. Application of probiotic (Bacillus subtilis) to enhance immunity, antioxidation, digestive enzymes activity and hematological profile of Shaoxing duck. Pak Vet J. (2013) 33:69–72.
85. Abdelrazek H, Yusuf M, Ismail S, Elgawish R. Effect of probiotic strains mixture administration on serum interleukins concentration, lymphocyte proliferation and DNA damage in rams. J Anim Feed Sci. (2015) 24:302–7. doi: 10.22358/jafs/65612/2015
86. Markowiak P, Slizewska K, Nowak A, Chlebicz A., Zbikowski A, Pawłowski K, et al. Probiotic microorganisms detoxify ochratoxin A in both a chicken liver cell line and chickens. J Sci Food Agric. (2019) 99:4309–18. doi: 10.1002/jsfa.9664
87. Rao KS. Free radical induced oxidative damage to DNA: relation to brain aging and neurological disorders. Indian J Biochem Biophys. (2009) 46:9–15. doi: 10.1155/2009/3152324
88. Hashem MA, Abd El Hamied SS, Ahmed E, Amer SA, El-Sharnouby ME. Mitigating the growth, biochemical changes, genotoxic and pathological effects of copper toxicity in broiler chickens by supplementing vitamins C and E. Animals. (2021) 11:1811. doi: 10.3390/ani11061811
89. Cox, J. Evaluation of strategies to improve in vitro mutagenicity assessment: Alternative sources of S9 exogenous metabolic activation and the development of an in vitro assay based on MutaMouse primary hepatocytes. Université d'Ottawa/University of Ottawa. (2019) 31:117–30. doi: 10.20381/ruor-23587
90. Murcia H, Diaz GJ. Dealing with aflatoxin B1 dihydrodiol acute effects: Impact of aflatoxin B1-aldehyde reductase enzyme activity in poultry species tolerant to AFB1 toxic effects. PLoS ONE. (2020) 15:e0235061. doi: 10.1371/journal.pone.0235061
91. Olier M, Marcq I, Salvador-Cartier C, Secher T, Dobrindt U, Boury M, et al. Genotoxicity of Escherichia coli Nissle 1917 strain cannot be dissociated from its probiotic activity. Gut Microbes. (2012) 3:501–9. doi: 10.4161/gmic.21737
92. Aragão AZB, Quel NG, Joazeiro PP, Yano T. Escherichia coli vacuolating factor, involved in avian cellulitis, induces actin contraction and binds to cytoskeleton proteins in fibroblasts. J Venom Anim Toxins Incl Trop Dis. (2021) 27. doi: 10.1590/1678-9199-jvatitd-2020-0106
93. Mehaisen GM, Eshak MG, El Sabry M, Abass AO. Expression of inflammatory and cell death program genes and comet DNA damage assay induced by Escherichia coli in layer hens. PLoS ONE. (2016) 11:e0158314. doi: 10.1371/journal.pone.0158314
94. Häcker H, Fürmann C, Wagner H, Häcker G. Caspase-9/-3 activation and apoptosis are induced in mouse macrophages upon ingestion and digestion of Escherichia coli bacteria. J Immunol. (2002) 169:3172–9. doi: 10.4049/jimmunol.169.6.3172
95. Bian L, Molan A-L, Maddox I, Shu Q. Antimicrobial activity of Lactobacillus reuteri DPC16 supernatants against selected food borne pathogens. World J Microbiol Biotechnol. (2011) 27:991–8. doi: 10.1007/s11274-010-0543-z
96. Taunde PA, Bianchi MV, Mathai VM, Lorenzo CD, Gaspar BD, Correia IMS, et al. Pathological, microbiological and immunohistochemical characterization of avian colibacillosis in broiler chickens of Mozambique. Pesquisa Veterinária Brasileira. (2021) 41. doi: 10.1590/1678-5150-pvb-6831
97. Fadl SE, El-Gammal GA, Sakr OA, Salah AA, Atia AA, Prince AM, et al. Impact of dietary Mannan-oligosaccharide and β-Glucan supplementation on growth, histopathology, E-coli colonization and hepatic transcripts of TNF-α and NF-ϰB of broiler challenged with E. coli O 78. BMC Vet Res. (2020) 16:1–14. doi: 10.1186/s12917-020-02423-2
Keywords: modulatory, probiotic, prebiotic, immuno-biochemical alteration, DNA, histopathological, E. coli, broilers
Citation: Hashem MA, Hassan AEA, Abou-Elnaga HMM, Abdo W, Dahran N, Alghamdi AH and Elmahallawy EK (2022) Modulatory effect of dietary probiotic and prebiotic supplementation on growth, immuno-biochemical alterations, DNA damage, and pathological changes in E. coli-infected broiler chicks. Front. Vet. Sci. 9:964738. doi: 10.3389/fvets.2022.964738
Received: 08 June 2022; Accepted: 10 August 2022;
Published: 20 October 2022.
Edited by:
Asghar Abbas, Muhammad Nawaz Shareef University of Agriculture, PakistanReviewed by:
Mohammed A. E. Naiel, Zagazig University, EgyptTagang Aluwong, Ahmadu Bello University, Nigeria
Copyright © 2022 Hashem, Hassan, Abou-Elnaga, Abdo, Dahran, Alghamdi and Elmahallawy. This is an open-access article distributed under the terms of the Creative Commons Attribution License (CC BY). The use, distribution or reproduction in other forums is permitted, provided the original author(s) and the copyright owner(s) are credited and that the original publication in this journal is cited, in accordance with accepted academic practice. No use, distribution or reproduction is permitted which does not comply with these terms.
*Correspondence: Ehab Kotb Elmahallawy, ZWVoYWFAdW5pbGVvbi5lcw==