Corrigendum: Epidemiology, pathology, prevention, and control strategies of inclusion body hepatitis and hepatitis-hydropericardium syndrome in poultry: A comprehensive review
- 1Poultry and Fish Diseases Department, Faculty of Veterinary Medicine, Alexandria University, Alexandria, Egypt
- 2Poultry and Fish Diseases Department, Faculty of Veterinary Medicine, Damanhour University, Damanhour, Egypt
- 3Poultry Disease Department, Faculty of Veterinary Medicine, Beni-Suef University, Beni-Suef, Egypt
- 4Bioinformatic Department, Genetic Engineering and Biotechnology Research Institute, University of Sadat City, El Sadat City, Egypt
- 5Smart-Health Initiative and Red Sea Research Center, Division of Biological and Environmental Sciences and Engineering, King Abdullah University of Science and Technology, Thuwal, Saudi Arabia
- 6Department of Clinical Laboratory Sciences, College of Applied Medical Sciences, Jouf University, Sakaka, Saudi Arabia
- 7Department of Biology, College of Science, United Arab Emirates University, Al-Ain, United Arab Emirates
- 8Khalifa Center for Genetic Engineering and Biotechnology, United Arab Emirates University, Al-Ain, United Arab Emirates
- 9Harry Butler Institute, Murdoch University, Murdoch, WA, Australia
- 10Poultry Department, Faculty of Agriculture, Zagazig University, Zagazig, Egypt
Infection with fowl adenoviruses (FAdVs) can result in a number of syndromes in the production of chicken, including inclusion body hepatitis (IBH), hepatitis-hydropericardium syndrome (HHS), and others, causing enormous economic losses around the globe. FAdVs are divided into 12 serotypes and five species (A–E; 1–8a and 8b−11). Most avian species are prone to infection due to the widespread distribution of FAdV strains. The genus aviadenovirus, which is a member of the adenoviridae family, is responsible for both IBH and HHS. The most popular types of transmission are mechanical, vertical, and horizontal. Hepatitis with basophilic intranuclear inclusion bodies distinguishes IBH, but the buildup of translucent or straw-colored fluid in the pericardial sac distinguishes HHS. IBH and HHS require a confirmatory diagnosis because their clinical symptoms and postmortem abnormalities are not unique to those conditions. Under a microscope, the presence of particular lesions and inclusion bodies may provide clues. Traditional virus isolation in avian tissue culture is more delicate than in avian embryonated eggs. Additionally, aviadenovirus may now be quickly and precisely detected using molecular diagnostic tools. Preventive techniques should rely on efficient biosecurity controls and immunize breeders prior to production in order to protect progeny. This current review gives a general overview of the current local and global scenario of IBH, and HHS brought on by FAdVs and covers both their issues and preventative vaccination methods.
Introduction
Avian adenoviruses of the family adenoviridae are found all over the world, infecting a wide variety of poultry hosts and ages, including falcons, raptors, ostriches, psittacine, and parrots (1). Chickens are the primary host for some avian adenovirus diseases such as inclusion body hepatitis (IBH), hepatitis-hydropericardium syndrome (HHS), adenoviral gizzard erosion (AGE), avian adenoviral splenomegaly (AAS), and egg drop syndrome (EDS). All of these diseases are caused by different virus types belonging to different adenovirus genera (Table 1). Recently, IBH and HHS have been widely distributed in broiler flocks in several countries (2–4), and there has been a trend toward more epidemic breakouts rather than sporadic epidemics. Furthermore, the significant mortality and growth retardation associated with IBH, and HHS have resulted in enormous economic losses (2–4).
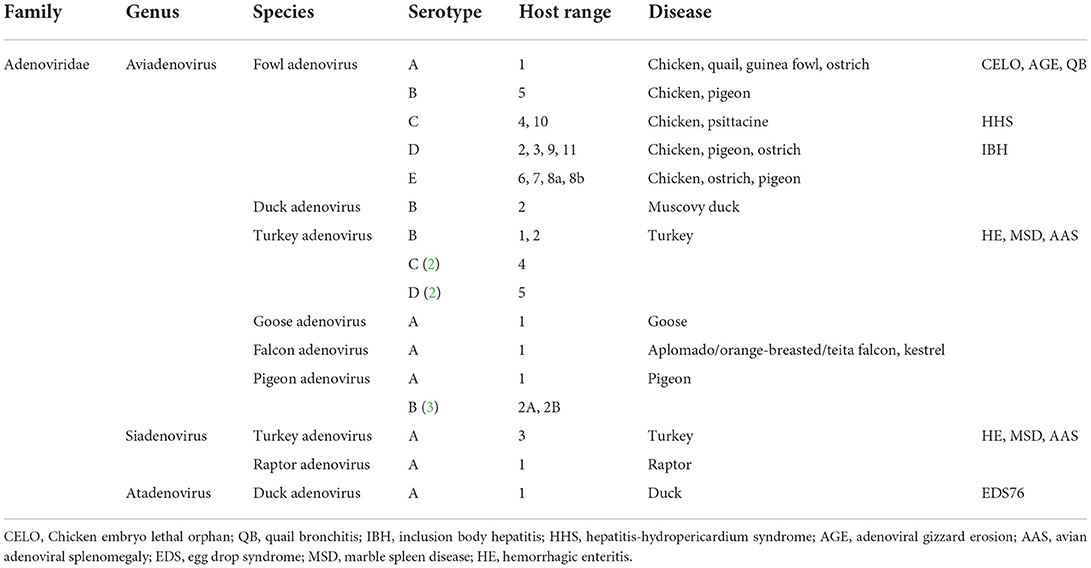
Table 1. Classification of adenoviruses affecting poultry [modified from (1)].
In Egypt, the poultry industry, particularly the broiler chicken sector, is currently experiencing high losses as a result of a high mortality rate, a poor feed conversion ratio, and low weight gain. By diagnosis, it was revealed that they are mostly viral outbreaks such as avian influenza (AI-H5Nx and H9N2), Newcastle disease (ND), infectious bronchitis, and infectious bursal disease (IBD) (5–8), despite extensive vaccination. Vaccination failure may be one of a variety of factors contributing to these losses and another common factor is the immune suppression. As a result, the question here is whether the adenovirus infection is to be blamed for vaccination failure because of its immune suppressive effect (indirect effect) or is it possible that the adenovirus itself is to be blamed for the death and economic losses (direct effect).
The purpose of this current review is to provide an overview of the current global and local situation of IBH, and HHS caused by fowl adenoviruses (FAdVs) and explains their problems and vaccination strategies, in addition to underly the desired procedures that are urgently required to investigate FAdV infections and how to control the losses in poultry industry.
A brief history
Figure 1 summarizes the history of adenovirus emergence and identification in poultry. The first aviadenovirus was discovered by chance in the 1950's from embryonated chicken eggs used for diagnosing or preparing vaccines for other non-avian pathogens (9). It was later isolated from Bobwhite quail in West Virginia, USA suffering from respiratory manifestations (10) and named quail bronchitis (QB) disease (11).
Adenoviruses were found in both healthy and diseased birds, and they were all characterized by being replicated in the host cell nucleus and vertically transmitted (11). Several adenovirus-associated outbreaks have emerged in the years since IBH was first detected in two broiler chicken flocks with high mortalities in the USA in 1963 (12). Marble spleen disease (MSD) infiltrated the lungs and spleens of ring-necked pheasants in 1966 (13). Hemorrhagic enteritis (HE), a turkey disease, was discovered to be caused by an adenovirus in the mid-1970's (14), despite outbreaks occurring since the 1930's (15). EDS outbreaks were first reported in laying hens with a soft shell and shell-less egg in the Netherlands in 1976 (16), and were later thought to be initiated from ducks as a natural host via contaminated vaccines (17).
AAS was described nearly 3 years later in broiler breeder chickens with enlarged spleens and pulmonary edema (18), but the disease was sporadic and subclinical. Sudden and high mortality in broiler chickens aged 3–6 weeks were first reported in Pakistan in 1987 (Angara Disease; HHS) (19). Tanimura et al. (20) discovered AGE associated with adenovirus intranuclear inclusion bodies (INIB) in layer chickens in Asia, but likes HE, it has been present since the 1930's. IBH and HHS outbreaks brought on by various FAdV serotypes have been reported globally in recent years. With serotypes 2, 8a, 8b, 11, and 4 being the most frequently involved, and the genotypes FAdV-D, -E, or -C have been related to the bulk of these outbreaks (4, 21).
Due to the variety in illness associations, it is currently difficult to determine the overall economic significance of aviadenoviruses (1). However, the high mortality observed in flocks with HHS or IBH, as well as the growth limitation caused by IBH and AGE, result in significant economic losses (4). In rare cases, IBH has been reported in layers and broiler breeders in addition to broilers. Mortality peaks during IBH outbreaks occurs in 3–4 days and can reach 10%, and on rare occasions, 30% (4), especially when concomitant infections are present. This may help to explain why some elements of field outbreaks are difficult to replicate in an experiment.
HHS is clinically similar to IBH, but the mortality in HHS may reach 80% (4, 22, 23) and is characterized by poor development, apathy, prostration, ruffled feathers, and huddling behavior which are some of the clinical indications that may be observed in affected chickens (4, 24). In addition, Bertran et al. (25) mentioned that the dramatic increase in IBH cases caused major economic losses in the Spanish poultry industry, which prompted the use of vaccination in broiler breeders.
Current status of IBH and/or HHS associated problems
In recent years, regional and global attention has been focused on the occurrence and economic significance of IBH and HHS and there is an increase in the number of IBH and/or HHS epidemics caused by FAdV over the past 20 years. For instance, FAdV-4 was the dominant serotype in China. Chen et al. (21) isolated 155 FAdV strains from diseased chickens during 3 years (2015–2018) from which FAdV-4 was the dominant serotype representing 79.4% (123/155), while FAdV-8a and FAdV-8b were identified by 13.5 and 3.9%, respectively through the phylogenetic analysis of hexon sequences (21).
In another study by Cui et al. (26) conducted also in China between 2015 and 2018, FAdV-4 was identified in 48 out of 73 hexon-sequencing isolates, whereas 24 of them belonged to serotype 10 (FAdV-10), and one to serotype 2 (FAdV-2). In the USA, IBH was isolated from 15 broiler flocks of age 18–35 days suffering from classic hepatic pathology and swollen kidney (27). Mete et al. (28) reported FAdV4 for the first time in California in a mixed chicken of different breeds and ages (6 months to 2 years old) with a mortality rate of 27% and hydropericardium. In Canada, Philippe et al. (29) isolated serotype 2 from 10-day old broiler breeder pullets experienced a sudden increase in mortality (2.1%). FAdV 8b serotype has been isolated as well by Dar et al. (30) from liver of a 17-day-old broiler chicken flock.
In addition, several reports of IBH and/or HHS were documented in Japan (31), Korea (32), India (33), Pakistan (34), Spain (25), Poland (35), Iran (36), Mexico (37), Brazil (38), Croatia (39), Australia (40), Greece (41), Iraq (42), Palastine (43), United Arab Emirates (44), Saudi Arabia (45), Morocco (46), and South Africa (47).
In Egypt, Mousa et al. (48) detected IBH during the late last century, then El-Shamy (49) detected the IBH histopathologically in the liver and pancreas samples of three broiler chicken flocks suffering from high morbidity (28%) and low mortality rate (2%). There were no subsequent records of its isolation or detection, either experimentally or in the field, until 2015. The detection of IBH (FAdV-E serotype 8a) in 2015 was from two out of nine surveyed (2/9) commercial broiler flocks aged 35 days in El-Beheira province, Egypt by Radwan et al. (50). The commercial flocks had mortality rates ranging from 7 to 17%, a European performance index of 143–212, as well as observable ascites and an enlarged fibrotic liver. However, when the pathogenicity of the isolated and molecularly identified virus was tested in 4-day-old specific-pathogen-free (SPF) layer chicks via oral and nasopharyngeal inoculation with 104 tissue culture infective dose50 ml−1 (TCID50 ml−1), only severe hepatitis and INIB were observed without mortality (50).
El-Tholoth and Abou El-Azm (51) conducted a case-control study in Kafr EL-Sheikh province, Egypt in the Spring of 2017 to assess IBH incidence. The flocks under investigation were 10 IBH suspected Ross and Sasso broiler chicken flocks aged 7–21 days with a mortality rate of 2.7–15% in comparison to an equal number of control flocks clinically appearing normal. FAdV was examined by polymerase chain reaction (PCR) in 100 liver and spleen samples (10 pooled samples flock−1), as well as IBD and chicken infectious anemia virus (CIAV) by PCR for bursa of Fabricius and ELISA, respectively. Not only was FAdV-D detected in seven of 10 flocks examined, but CIAV antibodies were also detected in all IBH positive flocks, despite the absence of IBD infection. Unfortunately, the CIAV status in Egyptian poultry flocks is unknown, so the alarm should sound not only for IBH, but also for CIAV, either as a single or co-infection (51).
Elbestawy et al. (52) detected and characterized FAdV species D (serotype 2 and 11) in 17 out of 37 broiler chicken flocks in northern Egypt during 2017–2018 (Kafr El-Sheikh, Beheira, and Alexandria governorate, Egypt). Sixteen positive flocks died at rates ranging from 0.4 to 16.6%, with one flock dying at a rate of 20% due to co-infection with a highly virulent IBD virus. Furthermore, ecchymotic hemorrhages on enlarged friable livers with hydropericardium were observed, as well as histopathological INIB in hepatocytes (52).
El-Basrey et al. (53) detected IBH in the livers of three out of 40 samples (7.5%) broiler flocks aged 3–5 weeks in Sharkia governorate, Egypt, based on hexon loop-1 gene detection using PCR. The only observed signs with varying mortality were depression and ruffled feathers (8–14%). The three positive samples (EG101/2018, EG102/2018, and EG103/2018) had nucleotide sequence identities of >97.7% and were genetically more similar to reference strains serotype 2 (FAdV-2). Two isolates out of 15 samples from broilers aged 19–40 days suffering from deaths of 5–10% were positive to FAdV-E 8a in Sharkia governorate, Egypt in the period 2019–2020 (54).
In terms of HHS, Sultan et al. (55) reported for the first time a pathogenic fowl aviadenovirus-4 (FAdV-4) in a Cobb-500 broiler flock aged 32 days in Alexandria governorate, Egypt in 2021. This flock had a high mortality rate (15%), depression, greenish diarrhea, a flabby heart with a pericardial sac filled with straw-colored fluid, a pale enlarged friable liver with hemorrhage, necrotic foci, and an edematous kidney. This FAdV-4 genotype was 98% identical to the Israeli strain IS/1905/2019. All of the recent studies were focused primarily on a region of northern Egypt (Figure 2).
As a result, additional research on the prevalence of HHS and other IBH serotypes should be conducted, including all Egyptian governorates and a wide range of poultry sectors. Furthermore, research into their pathogenicity, impact on immunity, interaction with other pathogens in the field, and association with severe economic losses in Egyptian poultry production became necessary.
Etiology and classification
The causative agent, adenovirus, is a member of the family adenoviridae and consists of a non-enveloped capsid with pseudo-icosahedral symmetry (Figure 3) composed of 720 hexons arranged in 240 trimers and 12 vertex pentons each with two fiber proteins protruding to the surface that are transcribed from a single fiber gene except for FAdV-A and FAdV-C which have two genes (56). Its genome is composed of 35–36 kb of linear, monopartite, double-stranded DNA that encodes ~40 proteins. The DNA contains terminally redundant sequences with inverted terminal repeats and a terminal protein attached to each 5′ end of the ds-DNA (56–58).
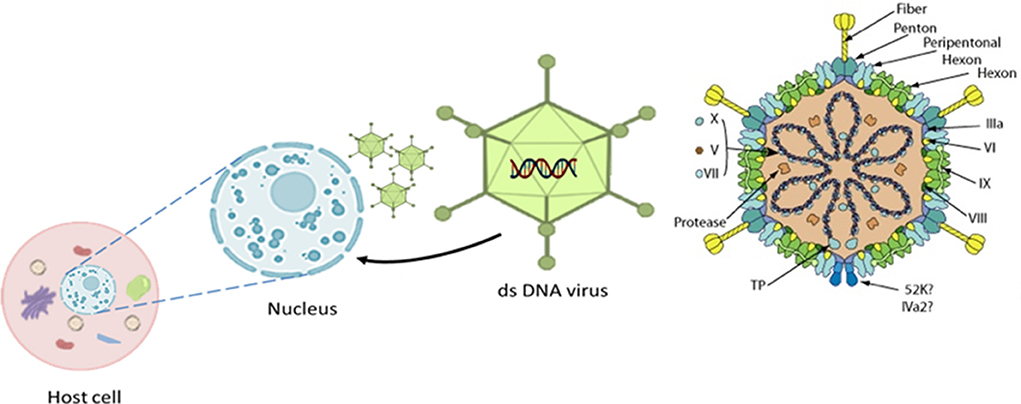
Figure 3. The 3D icosahedral structure of aviadenovirus with its pseudo-icosahedral capsid, genome, associated structural protein, and the fiber (252 capsomers, 240 hexons, and 12 penton bases carrying fiber protein projections). Replication takes place in the host cell nucleus. Modified after
Hexon protein has group and type-specific epitopes (59), and it is involved in the production of neutralizing antibodies, which could provide protection. Aside from neutralization, this protein is linked to hemagglutination, virus infectivity, and pathogenicity, making it a virulence factor (60). Sequencing of variable regions (right and left flank) of hexons, DNA polymerase genes, or the entire genome has recently been used not only to assign adenoviruses to species levels but also to further subdivide them into genotypes or serotypes (61–63). Based on fiber 2 phylogenetic analysis, Liu and his co-authors (64) distinguished pathogenic and non-pathogenic FAdV-4 strains inducing HHS from various geographical regions. It can also be used to investigate amino acid substitutions to study FAdV-4 strain genetic variation and molecular evolution (64).
Historically, avian adenoviruses were divided into three groups: group I (fowl adenovirus), group II (HE, MSD, and AAS), and group III (EDS76). The family adenoviridae has recently been divided into six confirmed genera: mastadenovirus, aviadenovirus, atadenovirus, siadenovirus, and ichtadenovirus, as well as the proposed testadenovirus of turtles and tortoises (Figure 4). Three of them can infect birds (aviadenovirus, siadenovirus, and atadenovirus), and viruses within each were classified into species, which can be further classified into serotypes (64). This classification was based on the most important criterion, phylogenetic distance, along with at least one of the following: cross-neutralization, genome organization, guanidine and cytosine (G+C) content, and host range (65). Within the aviadenovirus genus, FAdVs are classified into five different species (FAdV-A to FAdV-E) based on their molecular structure, and 12 serotypes (FAdV-1 to−8a and−8b to−11), based on cross-neutralization tests (Table 1).
FAdVs isolated from IBH cases are most commonly FAdV-D and FAdV-E. FAdV strains associated with HHS are FAdV-4 (FAdV-C) and are highly pathogenic to chickens (66). FAdV-1 (FAdV-A) has been isolated from the majority of gizzard erosion (65). Although individual adenoviruses are usually hosting species-specific, adenoviruses from multiple genera can infect a single animal species. FAdV-D (serotype 2) and –E (serotype 6) causing IBH outbreaks were molecularly identified in diseased broiler chickens and falcons, in Saudi Arabia, respectively and these viruses were distinct from falcon adenovirus species A (67). As a result, they reported that cross-species transmission between chickens and falcons was possible. This phenomenon will result in recombination events that will result in the emergence of a new adenovirus with new host affinity and pathogenicity characteristics.
Das et al. (68) discovered novel recombination of the fiber-2 gene from poicephalus adenovirus (PoAdV) affecting parrots to FAdV-C, the latter is causing the most recent outbreaks of HHS in broiler chicken flocks around the world (68). Furthermore, Marek et al. (69) concluded that possible recombination events within different hosts (domesticated, wild, and psittacine birds) may explain the frequent rapid updates of adenoviruses incidence in poultry in recent years, and that future results cannot be predicted (69).
Epidemiology, pathogenicity, and clinical signs
In the middle of the twentieth century, embryonic eggs became useful for producing vaccines as well as for isolating filterable chemicals from various sources. It was occasionally found that such procedures could isolate a virus unrelated to the original material (10, 70). As a result, vertical transmission was recognized as one of the first important biological characteristics of chicken adenoviruses, particularly at the height of egg production.
Losses in healthy chicks without co-infections are possible with vertically transmitted FAdVs (71). On the other hand, they may go unnoticed for a while and then reactivate in young birds with immunosuppression (72, 73). FAdVs are also easily horizontally transferred via the oral-fecal route because of the high shedding titers in the feces (74). Later, experimental infections and epidemiological studies were conducted to determine the role of FAdVs in IBH (70). Table 2 shows the induced IBH and/or HHS in birds infected with different FAdV serotypes experimentally or naturally.
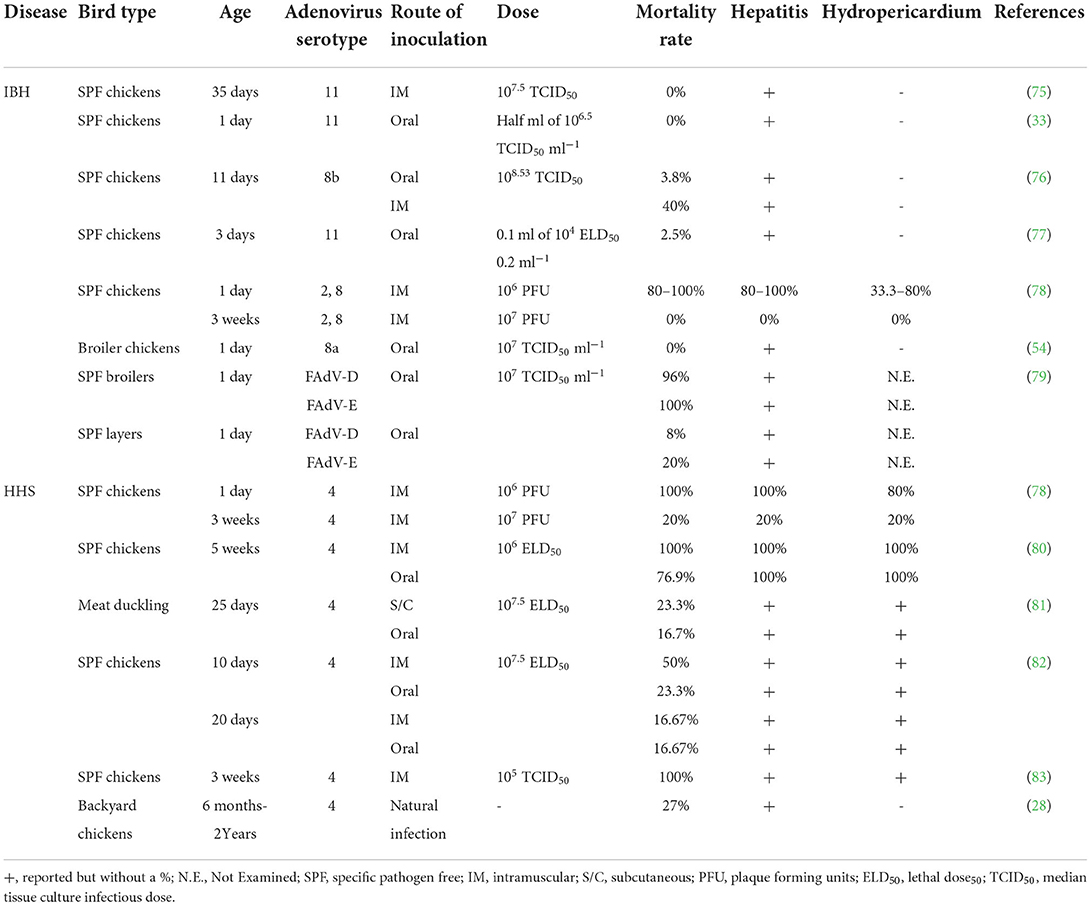
Table 2. Induced inclusion body hepatitis (IBH) and/or hepatitis-hydropericardium syndrome (HHS) upon experimental or natural infection of birds with different fowl adenoviruses (FAdVs) serotypes.
It was shown that the virus colonizes the intestinal epithelium at 12 h post-infection (hpi) and can be found in the blood as early as 24 hpi after oral inoculation of chickens (74). As early as 2 and 3 days after infection, the virus is detectable in the liver and pancreas, which are its target organs. The incubation period is during which there are no clinical symptoms or obvious abnormalities (84). The fast viraemia that results from the virus multiplication after the incubation period and causing pathological lesions in its target organs occurs at the same time as the disease's clinical manifestation (74, 79, 84). Beginning around 79% recovery in birds is marked by a lessening of clinical symptoms, cellular regeneration, and a decline in viral load in the affected organs (79, 84).
The virus, however, is persistently secreted in feces and dormant in the caecal tonsils. Twelve weeks after SPF hens were orally infected with an FAdV-1 strain when they were just 1 day old, the virus was still detectable in their intestines (85). Additionally, rather than being related to the pathogenicity of a particular strain, the quantity and duration of virus shedding in the feces coincide with the viral dose supplied (86, 87). Due to the prevalence of the viruses vs. sporadic outbreaks and variation between experimental experiments, the pathogenicity of FAdVs as a single infection (primary pathogen) has long been debated (40, 78, 88–91). However, the importance of FAdVs as primary pathogens is still debatable because various strains of the same serotype may have varying levels of pathogenicity (86, 92, 93). Recent research has shown that FAdVs have been found in both healthy and ill chickens (94, 95).
IBH
Historically, IBH was known to occur as a secondary or concurrent pathogen (opportunistic agents), particularly in the presence of immunosuppressive agents such as IBD, CIAV, avian reticuloendotheliosis virus (ARV), avian leukosis virus (ALV), and mycotoxins (96–100). Nonetheless, it was observed in recent outbreaks as an independent primary pathogen in various parts of the world, causing hepatitis and sudden death of up to 30% of broiler chickens aged 3–5 weeks, which usually peaked at the 4th days post infection (dpi) and may terminate by the fifth dpi, but deaths may extend for another 2–3 weeks (4).
IBH has been documented in chickens as young as 7 and 10 days old, as well as in 1-day-old turkeys, even though it typically affects poultry between the ages of 3–5 weeks (29, 101–103). Before passing away, the sick birds may exhibit despondency, ruffled feathers, and decreased meal intake (104). IBH can impact breeder, broiler, and layer hens. The route of inoculation, virus dose, age of the bird, serotype or even strain pathogenicity, and bird type are just a few of the variables that are considered when determining the disease result during various in vivo experiments (78, 105–107).
To cause a clinical manifestation of IBH in chickens older than 1 week old, an invasive route of infection, such as intramuscular or intraperitoneal, is typically necessary (91). Up till now, no study has conclusively defined this phenomenon, and the mechanisms causing it are not entirely understood. IBH affects different breeds of hens, which has a significant impact on how the disease progresses. Cook used a variety of chicken breeds of SPF and non-SPF origin to study the effects of host age and the manner of inoculation on the results of FAdV infection. Upon intraperitoneal inoculation, he found that SPF Light Sussex hens were more vulnerable to infection than SPF Rhode Island red chicks, with fatality rates of 67 and 33%, respectively (86).
More recently, FAdV-D or -E oral infection of SPF broilers resulted in a mortality rate of 96–100% with severe clinical signs, compared to 8–20% mortality in SPF layers inoculated under the same conditions (79), which was consistent with the situation reported from the field and the higher susceptibility of broilers. Similar to this, 20% of SPF layers and 40% of SPF broilers died after subcutaneous FAdV-E strain infection (108).
Furthermore, there is an age-associated resistance to IBH (over 3–5 weeks). Dar et al. (30) observed that the infection susceptibility increases in SPF leghorn chicks at 1 day old and declines as they get older. In addition, FAdV strain or serotype affects the virus's pathogenicity. However, no conclusive molecular data exists to distinguish FAdVs according to their pathogenicity (30). Animal challenge and evaluation of morbidity, mortality, and the severity of the lesions are the most reliable ways to assess the pathogenicity of FAdVs (109). On the other hand, co-infection with other pathogens such as IBD, CIAV, ND, and Escherichia coli, as well as the bird's immune status, viral dose, and route of infection, can all influence the outcome of IBH (109).
Furthermore, no statistically significant correlation between flocks exposed to FAdV and co-infected with CIAV or IBDV was discovered in a recent investigation (110). On the other hand, reports from Australia, New Zealand, Canada, and Japan reported IBH epidemics without the presence of risk factors, supporting the idea that FAdVs are a primary pathogen (36, 40, 78, 88–90). All 12 FAdV serotypes have been connected to IBH epidemics (111). However, serotypes 2 and 11 of FAdV-D, as well as 8a and 8b of FAdV- Serotypes 9 and 3 of FAdV-D were found in South Korea (107), serotype 7 of FAdV-E was found in Iraq (42), Poland (112), and China (43). Other serotypes have also been reported to be included in IBH (100). FAdV-E was found to be the most prevalent in different geographical regions (4).
Mittal et al. (113) identified FAdV serotypes 2, 4, 5, 6, 7, 8, and 12 in broiler chickens affected with HHS or IBH in India. Furthermore, an outbreak can include at least two serotypes; due to the lack of serotypes cross-protection. For example, the predominant combinations indicating mixed infection in broiler chickens in India were FAdV-8 and FAdV-5, FAdV-8 and FAdV-7, FAdV-8 and FAdV-6, FAdV-8 and FAdV-12 (113). Therefore, an autogenous vaccine covering all serotypes involved in such outbreaks should be used.
HHS or Angara Disease
In Pakistan, sudden and high mortality in broiler chickens aged 3–5 weeks was reported as a new disease (Angara Disease) in 1987, and it was later reported globally with a mortality rate of 20–80%. It is caused by the highly pathogenic FAdV-C serotype 4 virus and is characterized by an enlarged, friable and hemorrhagic liver with INIB, pulmonary edema, and nephritis (114).
All these symptoms are similar to IBH, but the main difference between the two diseases is the presence of a typical hydropericardium caused by HHS. In India, HHS was dubbed “Lychee” disease because the affected birds' hearts were surrounded by hydropericardium which resembled a peeled Indian lychee fruit (115). It was suggested that testing the virus pathogenicity for chickens older than 3 weeks is an easy way to differentiate between IBH and HHS (78). HHS outbreaks have also been reported in Kuwait, Iraq, Russia, India, Korea, Japan, North, and South America, and Egypt (55, 66, 115–119).
The FAdV causing HHS was identified as serotype 4 by a serum-neutralization test, PCR assay, and restriction enzyme analysis. Inoculating isolated FAdV−4 subcutaneously and orally in 28–day-old broilers, resulted in disease reproduction (120, 121). The virus's affiliation with avian adenovirus serotype 4 was established through sequence analysis of the variable region hexon gene. India and Pakistan isolates were 94–98% homologous (122).
According to phylogenetic research using the entire nucleotide sequences of the short fiber genes, the FAdV-4 strains from HHS in Japan, India, and Pakistan formed a distinct cluster from FAdV-4 strains not originating from HHS (123). The phylogeny of the hexon gene sequences of two FAdV isolates from Anand, Gujarat in India revealed that both viruses clustered with chicken adenovirus 12 (strain 380) and fowl adenovirus 11 (strain C2B) and formed a small branch of the upper group, indicating the establishment of a novel fowl adenovirus genotype (124).
Later, the HHS was formed, which highlighted the significance of FAdV infections in young chickens, especially broilers, and elevated awareness of FAdVs as primary causative agents (91). The signs of an ill bird include lethargy, huddling with ruffled feathers, appetite loss, and mucoid yellow droppings. Infected flocks' lower weight gain has led to a low feed conversion ratio (125, 126). In the later stages of the infection, the chicks were dull, sad, with ruffled feathers, and reluctant to move, collecting in corners, according to Asrani et al. (126). The birds continued to be active until they died. Birds with HHS had severe anemia and a considerable decline in all hematological values, according to clinical pathology characteristics (127).
Additionally, albumin levels were altered in serum, whereas b-globulin levels rose (128). When the osmotic pressure of colloidal plasma is decreased, fluid leaks into the pericardial sac. While serum levels of uric acid, potassium, calcium, and triglycerides were significantly greater, blood glucose and plasma protein levels were decreased. This could be because of fluid buildup in the pericardial sac and the abdomen (129). Normal birds have low serum enzyme activities for aspartate aminotransferase, alanine aminotransferase, and creatine phosphokinase, whereas HHS-infected birds have high serum enzyme activities (130, 131). All these changes suggested that the liver, kidney, and heart were all involved in HHS.
Pathology: Gross and microscopic lesions
Gross lesions
The major liver abnormalities in IBH are pale, friable, enlarged livers with minute white foci and, in certain cases, petechial or ecchymotic hemorrhages. Glomerulonephritis is commonly accompanied by swollen kidneys (27). In both spontaneous and artificial infections, atrophied bursa and thymus, aplastic bone marrow, and hepatitis have all been observed (4).
Gross lesions peaked 4–10 days following infection in SPF hens with ocular or oral illness (84, 87). Broilers are more vulnerable to greater mortality because of the severe metabolic imbalance and the substantial damage of the pancreas in addition to the liver (79). In HHS compared to IBH, there are more severe gross lesions in the liver and kidneys. Pathognomonic for HHS is hydropericardium, which is described as a buildup of clear, straw-colored fluid in the pericardial sac (78). Pulmonary edema, a larger, discolored liver, and enlarged kidneys with dilated tubules are typical abnormalities (118, 132, 133).
Some cases of spontaneous infections were recorded with petechial hemorrhages, focal necrosis in the liver, severe congestion, and hemorrhaging with myocardium fracture (23, 134–137). Ascites and widespread necrosis of the pancreas have been documented in HHS severe epidemics (138). Different FAdV serotypes causes HHS and IBH, and HHS has a substantially greater fatality rate than IBH does (1). The main pathognomonic lesion in HHS, the hydropericardium, has not been reported in IBH (78). Accordingly, abrupt high mortality in broiler hens aged 3–6 weeks with hydropericardium, nephritis, and hepatitis is what constitutes suspected field cases (125, 139, 140).
Microscopic lesions
INIB in the liver is a hallmark of FAdV infection, which has also been previously documented, in both IBH and HHS (30, 84, 133). The pancreas, kidneys, gizzard, and small and large intestines all found to have INIB (1). They are primarily big, spherical, irregularly shaped, and basophilic with a clear, pale halo with virus particles in those intranuclear bodies.
Additionally, the hepatic parenchyma displayed congestion, hemorrhages, centrilobular or diffuse hepatocyte degeneration, cloudy swelling, fatty alterations, multifocal areas of coagulative necrosis, and sinusoidal space enlargement (141, 142). The presence of lymphocytes, plasma cells, macrophages, and heterophils can also be seen in multi-focal coagulative necrosis (141). Steer et al. (84) classified the disease into three stages based on the presence and severity of hepatic lesions: incubation (1–3 dpi), degeneration (4–7 dpi), and convalescence (14 dpi).
FAdV-8b and FAdV-11 were found in the liver, kidney, and gizzard of most birds during the degenerative stages, and remained in the gizzard until convalescence. The kidneys displayed subcapsular hemorrhages, hyperemia, denuded tubular epithelium, tubular epithelium degeneration, necrosis, and moderate interstitial lymphoplasmacytic nephritis (141, 142). Glomerulonephritis is characterized by an increase in the glomerular area and the average glomerular cell count, which was observed during a severe outbreak of IBH (27). Glomerulonephritis was the prominent renal pathology recorded in a broiler flock infected with IBH and complicated with citrinin mycotoxicosis (142).
Pancreatic necrosis is possible (4) and myocarditis as well as heart hemorrhages have been observed in chickens and ducks that died from HHS (23, 136, 143) with focal accumulation of mononuclear cells (141, 142). Furthermore, depletion of B and T cells in lymphoid organs was significantly induced after virulent FAdV-4 experimental infection in SPF birds leading to a significant immunosuppression (144, 145). Therefore, lymphocyte depletion in spleen, vascular congestion and hemorrhagic focal areas was noticed (141) with associated reticular endothelial cell hyperplasia (142).
Similarly, lymphocyte depletion with hemorrhages and follicular atrophy in the bursa of Fabricius can be seen (1). Moreover, congestion, hemorrhages, edema, and mononuclear cell infiltration were present in the lungs of chickens infected with HHS (141). Sharma et al. (146) and Ahamad et al. (147) suggested that the persistent intravascular pulmonary pressure may be the cause of the edema and injury to the pulmonary vessels.
Immunosuppressive effect of aviadenovirus
HHS caused by aviadenovirus is a relatively new humoral and cellular immunosuppressive disorder (145, 148). Although layer and breeder flocks of <20 weeks of age were affected as frequently as broiler chickens, the disease manifested in a milder form with a < 10% mortality rate (113). However, there have recently been reports in China of aviadenovirus serotype 4 strains evolving to be hypervirulent (having a deletion of ORF19) and causing recent emergent outbreaks in layers with a 50% mortality in the absence of other pathogen co-infection (80, 133, 149). Furthermore, in HHS outbreaks in China, high mortalities in commercial duck farms with pericardial effusion and stunted growth have occurred (81, 143).
After experimental FAdV4 infection of SPF birds, atrophy of the bursa of Fabricius follicles, lymphocyte loss with hemorrhages, and significant immunosuppression in the form of B and T cell depletion in lymphoid organs were detected (144, 145). Steer et al. (84) discovered FAdV-8b and FAdV-11 in the bursa and thymus of the majority of birds throughout the degenerative stage. The depletion of the mucosal immune system in the respiratory tract and the FAdV-induced degenerative alterations in the spleen and bursa, organs crucial for both cellular and humoral immune response, increased the risk of infection with other avian diseases (50). The virus was also discovered in the bursa and/or gizzard of some birds between 2 and 7 dpi, despite the FAdV-1 isolate being determined to be a pathogenic (50).
The effects on lymphatic organs in the thymus and bursa atrophy in the case of HHS and IBH, particularly caused by FAdV-4, FAdV-8b, and FAdV-11 as primary pathogens, may indicate that these strains are involved in immunosuppression; however, possible coinfections should be considered (23, 84, 136).
Diagnosis of aviadenovirus
Isolation and identification of aviadenoviruses
The preferred specimens for viral isolation are feces, kidney, pharynx, and afflicted organs (e.g., liver in IBH). For FAdV, liver, kidney, or hepatocellular cancer cell lines from chick embryos were infected with a 10% suspension of sample material. Although chicken cells can be used, it is better to use cells from the same species when trying to isolate aviadenoviruses from other avian species (24).
Most aviadenoviruses cannot be successfully isolated from embryonated eggs, although some isolates of FAdV have been shown to infect the yolk sac. An adenovirus isolate's identification can be verified using electron microscopy. Immunocytochemistry is a technique for the detection of adenoviruses that involves staining infected cells with an avian adenovirus antiserum tagged with a fluorescent dye. Virus neutralization testing using the isolate against common reference antisera to each known serotype are required to identify the serotype of an isolated virus (24).
Molecular techniques
FAdVs were genetically divided into five distinct genotypes, A through E, which included all 12 serotypes (150). Since over two decades, FAdV has been detected using PCR. Primers are mostly based on the hexon gene, and the sequencing of its variable sections is used to classify viruses into species A through E and to determine genotypes within species (24, 56, 61, 151–154). High resolution melting curve analysis or pyrosequencing can also be used to differentiate viruses based on variation within loop one of the hexon gene (155, 156).
For a small number of strains, applying the hexon and fiber gene sequences for typing reveals some inconsistencies (61). The PCR can be extended beyond aviadenoviruses by creating primers of additional conserved areas, but without further subtyping of FAdV genotypes (24, 154, 157). In comparison to virus isolation, nested and real-time PCRs have been reported to increase sensitivity and can be employed for quantification (158, 159). FAdV have been isolated and found in birds without lesions using the cecal tonsils. The liver and pancreas are the two internal organs that were attacked (74, 160).
Recent studies of the whole genome sequences of both pathogenic and non-pathogenic FAdV-4 isolates revealed probable genomic changes related to virulence. The two most significant structural proteins, fiber 2 and hexon, as well as nucleic acid insertions and deletions in ORFs 19, 27, 48, and 19 A, are the main areas of variation (64). After the initial whole-genome sequencing projects and the adoption of hexon-based molecular typing (151, 161, 162), FAdV sequence data started to quickly increase in the early 2000's (4).
The molecular studies and sequencing of both the fiber and hexon proteins increase our understanding of how FAdV strains have evolved over time, which in turn helps us develop methods to stop disease outbreaks in poultry farms. The fiber and hexon proteins are essential for FAdV infectivity because they encode virulence factors. While the fiber-1 protein is crucial for viral replication and assembly independent of virulence potential as opposed to infectivity, and the fiber-2 protein is more crucial for FAdV pathogenicity than the hexon protein. In tissue tropism, the hexon protein is crucial (163).
In situ hybridization (ISH) has been shown to be able to identify viral DNA in tissue samples (24). If these procedures are not accessible, the presence of DNA-containing viruses can be inferred from the staining of infected cell monolayers or tissue slices as well as from the appearance of intranuclear basophilic inclusions. Finally, current developments in the diagnosis of diseases were documented (122, 158, 164–167).
Serology
Hemagglutination inhibition is not applicable since hemagglutination is a distinguishing characteristic of FAdV1 strains, some of which hemagglutinate rat and/or sheep erythrocytes (168). The results of the ELISA, or indirect immunofluorescence assay, rely on the antigen employed to identify group-specific antibodies (24). Although the existence of numerous serotypes makes interpretation more difficult, ELISA can be used for this purpose. Type-specific antibodies are typically discovered using the serum-neutralization test. First detected in serum on day 14, virus-specific antibodies peaked at statistically significant levels at days 21, 28, 35, and 42 (137).
It is possible to discriminate between infected and vaccine-exposed mice using the recombinant non-structural proteins 33 and 100 K (169). The incomplete hexon protein has a high sensitivity for the detection of homologous antibodies (170, 171). The level of local immunity at mucosal surfaces is not indicated by the presence of humoral antibodies (171).
Intervention strategies to control IBH and HHS
Management procedures to prevent or minimize FAdV disease
Preventing aviadenovirus infection is primarily based on biosecurity practices. Strict managemental practices, as well as cleaning and disinfecting of premises and equipment; restriction of entry and/or personal protection of visitors and vaccination crews into poultry house, all play an important role in IBH and HHS prevention (1, 172).
The resistance of aviadenoviruses to inactivation by heat (up to 70°C) as well as high resistance to common disinfectants (particularly lipid solvents such as ether, chloroform, and phenol) (173) resembles a significant challenge, particularly in poultry houses with impervious floors and walls.
Application of glutaraldehyde and calcium hydroxide liquid combination under ambient temperature of 21°C during the downtime inside and outside the house was successful in preventing the persistence of gizzard erosion caused by FAdV-1 infection (174). On the other hand, it has been reported that the susceptibility of human adenovirus to disinfectants varies depending on the strain, and some strains are less susceptible to disinfectants like liposomal povidone-iodine, peracetic acid, and formaldehyde (175). It would be tough to get rid of aviadenoviruses once they have infected chicken farms. Overall, the viruses are regarded as latent across the country (11).
Yamaguchi et al. (11) isolated and identified serotypes (FAdV-A) causing gizzard erosion, and 5 (FAdV-B) as well as 8b (FAdV-E) from apparently healthy broiler and layer breeder chickens (1–20 weeks of age) in Japan. Similarly, Meng et al. (100) isolated FAdV serotype 7 from parental and offspring generations of chickens, in addition to co-infection with REV, ALV, and CIAV. Therefore, effective FAdV control begins at the primary breeder level with optimum disinfection and vaccination as two parallel lines that could prevent infection and, as a result, protect against vertical transmission. However, the horizontal spread is a significant issue that should not be overlooked, and it takes some effort to keep a commercial flock free of FAdV infection. Furthermore, controlling and/or eliminating immunosuppressive diseases such as IBD virus and CIAV is also critical in reducing FAdV disease because they increase the pathogenicity of FAdV (176).
Vaccination
In recent years, outbreaks of IBH and HHS have resulted in higher mortality in birds as compared to early milder outbreaks, resulting in severe economic losses to the poultry sector. As a result, it is possible that the virus's virulence has changed, causing HHS as well as IBH, resulting in high mortality (177). As several evidences indicated the primary pathogenicity of adenoviruses, vaccination with certain genotypes/serotypes may be becomes more appealing. Many countries employ both live and inactivated vaccines to combat IBH and HHS. The FAdV serotypes 4 and 8 are most commonly used in commercial vaccines preparation (177). However, for disease prevention and control in endemic areas, it is recommended that autogenous inactivated vaccines prepared from the prevalent serotype of FAdV be administered. Primary breeders with strict biosecurity practices used autogenous inactivated vaccines to prevent vertical transmission and ensure the transfer of maternal immunity from breeding flocks to their progeny (177).
In Pakistan, broiler chicks are routinely immunized against HHS using an avian adenovirus of serotype 4, with apparent efficacy in containing the illness (4). Broilers are occasionally immunized in Pakistan at 10 days of age if their mothers lack serotype-specific adenovirus antibodies or if maternal antibody transmission is irregular because of poor vaccination practices, leading to a high proportion of unprotected birds. Historically, numerous trials have been developed to prepare inactivated vaccines driven from embryos and cell culture (178).
Ahmad et al. (179) demonstrated that the formalized vaccine of aqua base liver organ has a faster but lower immune response than oil base tissue culture vaccine; however, both vaccines are effective against HHS. According to Reddy et al. (180), the HHS vaccine was not only effective against HHS but also influenced immune responses against the ND virus. In addition, prophylactic vaccination of broilers aged 10–15 days with a formalin-inactivated liver homogenate vaccine provided excellent protection in the field in Pakistan (179, 181–183). However, many efficacies and safety factors such as batch–to–batch variation in viral antigen quantum, incomplete inactivation, and contamination by adventitious avian viruses have been overlooked in this successful type of autogenous vaccine, which are major disadvantages that may jeopardize biosecurity (184).
In India, only inactivated oil emulsion vaccinations are used to prevent HHS, but only in suspected epidemic situations (185). A few weeks after vaccination, Kataria et al. (186) found that an inactivated oil emulsified anti-IBH-HHS vaccine made from fowl adenovirus produced in cell culture gave protection. Similarly, Gupta et al. (187) has created an inactivated vaccine against the HHS virus using chicken embryo kidney cell culture. When challenged with virulent FAdV−4, the vaccine provided 100% protection in broiler chickens.
In the USA, autogenous inactivated vaccines are commonly used in primary breeder flocks where biosecurity measures are strictly enforced. Full protection against IBH was achieved in breeders up to the age of 50 weeks by immunizing a grandparent stock at 10 and 17 weeks of age with an inactivated vaccine containing isolates of species FAdVD and FAdVE (108). Using a polyphosphazene and avian beta-defensin 2 as an adjuvant induced an antibody response and upregulated certain cytokines in the spleen even after in ovo vaccination with a dead FAdV8b vaccine (188, 189).
Breeders and broilers are both immunized with inactivated vaccinations in other nations like Mexico and Peru. If the right vaccination is carried out, the progeny of breeders acquires the maternal antibodies, protecting them from both an infection in the field and a clinical form of the disease. A large proportion of unvaccinated birds died in the age range of birds under 10 days old, either because they did not get serotype-specific anti-adenovirus antibodies or because their transmission was irregular as a result of poor vaccination (190). One study in Chile found that vaccinating broiler breeders against both CIAV and FAdV-4 provided better protection for progenies against HHS than vaccination against either disease alone (191).
In Australia, breeders were given a live vaccine (FAdV8b) 1-3 times between the ages of 9 and 18 weeks by drinking water; yet, IBH outbreaks in broilers persist. A heterologous strain was infrequently identified from ill birds in addition to an FAdV similar to the vaccine, indicating a lack of cross-protection (84). Contrarily, an inactivated oil-emulsion FAdV-4 vaccine generated high levels of protection against different serotypes in both vaccinated/challenged SPF chickens and broilers descended from vaccinated breeders (192).
In Egypt, an inactivated FAdV-C serotype 4 vaccine has been commercially available and used for vaccination of breeder chickens since late 2020; however, the cross-protection of this vaccine with the isolated FAdVs D serotype 2 and 11 and E serotype 8 a,b remains unknown and requires additional research (data under investigation).
Inactivated vaccines, subunit vaccinations, and genetically modified vaccines have been the focus of recent research on FAdV-4 vaccines (193–196). However, neither low pathogenic nor non-pathogenic live vaccines have been the subject of in-depth research. Live FAdV-4 has been attenuated by passing virulent FAdV-4 strains through chicken embryos or QT35 cells (145, 197), however it is unknown whether these attenuated vaccines have any protective effects on the developing FAdV-4.
Zhang et al. (198) discovered that the hexon gene is the key gene responsible for FAdV-4's high pathogenicity and created a non-pathogenic chimeric virus rHN20 strain based on the newly discovered FAdV-4. The immunogenicity of artificially rescued rHN20 as a live vaccine was tested in chickens using various routes and doses (198). The live rHN20 vaccine induced high titers of neutralizing antibodies against FAdV-4 and fully protected immunized chickens against a lethal dose of FAdV-4 without any clinical symptoms or histopathological lesions in the liver, and significantly decreased viral load in immunized chicken tissues, leading to consideration of this live vaccine candidate for preventing HHS in the poultry industry (198).
Regarding the innovative recombinant vaccines that have recently been introduced into poultry production, several research studies have been conducted in order to obtain a recombinant vaccine for FAdVs-IBH and -HHS. The structural proteins fiber 2 or penton base of FAdV-4 expressed as recombinant proteins induced a high level of protection against HHS (199, 200), compared to only 40% protection with the 100 K non-structural protein (201). Furthermore, the VP2 gene of IBDV incorporated into FAdV serotype 10 genomes demonstrated efficient expression and protection of challenged birds (202).
Recent studies showed that FAdV-E field isolates from both homotypic (FAdV-8a) and heterotypic (-8b) serotypes causing IBH were resistant to the recombinant FAdV fiber protein, which was generated from an FAdV-8a strain. The liver viral load was significantly reduced after the birds had the homologous challenge, but they were not clinically protected from infection by heterologous serotypes. Additionally, fiber immunization promoted the proliferation of CD4+ T lymphocytes while moderating the CD8+ T cell response and prevented challenge-induced changes in systemic monocytes/macrophages and T cell subpopulations, suggesting that FAdV-E, recombinant fiber represents a vaccine candidate to only control the harmful effects of homotypic infection (203).
Additionally, recombinant viruses were created using unique genotype FAdV-4 strains in which the fiber-2 gene's N-terminus was either knocked off or fused to enhance green fluorescent protein in order to prevent pathogenicity in SPF hens. However, the primary cause for worry was the fact that in vitro recombinant virus titers were much lower than those of wild-type FAdV-4 (204). These advancements, which range from severe primary pathogens to non-virulent vectors, show the diversity of FAdV (204).
Recently, Wang et al. (75) detected for the first time fiber-1 which directly mediates the infection of FAdV-4 through its shaft and knob domains, through superinfection resistance assay, interfering assay, and serum neutralizing assay. The chicken coxsackie and adenovirus receptor (CAR) homology recently known as a cell receptor for fiber-1 was identified by Pan et al. (205). The efficient protection against the lethal challenge of FAdV-4 in chickens conferred by the vaccination of the knob domain containing fusion protein not only confirms the significant roles of the fiber-1 and its knob domain in mediating the infection of FAdV-4 but also highlight a promising application of knob domain-based subunit vaccines against FAdV-4 in the future (205).
De Luca et al. (206) created a unique chimeric combination epitope from two different FAdV serotypes, FAdV-4 and−11, and tested its effectiveness to shield hens from both HHS and IBH at the same time. In comparison to the corresponding challenge controls, the vaccinated/challenged birds showed fewer clinical signs, limited hepatomegaly, lower levels of AST, prevented the atrophy of HHS-affected lymphoid organs like the thymus and bursa of Fabricius, and significantly decreased viral loads in the target organs. By using CRISPR-Cas9 and homologous recombinant procedures, Lu et al. (207) created a novel recombinant FAdV-4 that expresses the fiber of FAdV-8b, known as FA4-F8b, which can effectively guard against both FAdV-4 and FAdV-8b challenge in 2-week-old SPF hens. Their work has laid a foundation for development of vaccines against multi serotypes of FAdV in the future (207).
To demonstrate their full potential, recent developments are required in the creation of efficient vaccines (172, 203, 208–216), novel therapeutics (217–220), and effective prevention and control strategies (172).
Conclusion and future perspectives
IBH and HHS are currently considered emerging poultry diseases; however, the pathogenicity of most isolates is still debatable. The severity of infection in the field is considerably worsened by co-infections with other immunosuppressive viruses as CIAV, IBDV, ARV, ALV, and Marek's disease virus (MDV). The best approaches for identifying both diseases in birds are clinical diagnosis and molecular detection using PCR. Breeders need to follow tight biosecurity procedures, appropriate management methods, and immunization programs to control these diseases. As a result, preserving the expansion of chicken sector depends on expanding research into diseases linked to adenoviruses.
Author contributions
All authors equally contributed to writing this review article. All authors reviewed and approved the final version of the manuscript.
Acknowledgments
Authors thank their respective institutions for their support. KE-T would also like to thank the library at Murdoch University, Australia, for the valuable online resources and comprehensive databases.
Conflict of interest
The authors declare that the research was conducted in the absence of any commercial or financial relationships that could be construed as a potential conflict of interest.
Publisher's note
All claims expressed in this article are solely those of the authors and do not necessarily represent those of their affiliated organizations, or those of the publisher, the editors and the reviewers. Any product that may be evaluated in this article, or claim that may be made by its manufacturer, is not guaranteed or endorsed by the publisher.
References
1. Hess M. Adenovirus infections. In:DE Swayne, M Boulianne, CM Logue, LR McDougald, V Nair, , editors, Diseases of Poultry, 14th ed. Hoboken, NJ: Wiley-Blackwell (2020). p. 322.
2. Marek A, Ballmann MZ, Kosiol C, Harrach B, Schlotterer C, Hess M. Whole-genome sequences of two turkey adenovirus types reveal the existence of two unknown lineages that merit the establishment of novel species within the genus Aviadenovirus. J Gen Virol. (2014) 95:156–70. doi: 10.1099/vir.0.057711-0
3. Teske L, Rubbenstroth D, Meixner M, Liere K, Bartels H, Rautenschlein S. Identification of a novel aviadenovirus, designated pigeon adenovirus 2 in domestic pigeons (Columba livia). Virus Res. (2017) 227:15–22. doi: 10.1016/j.virusres.2016.09.024
4. Schachner A, Matos M, Grafl B, Hess M. Fowl aviadenovirus (FAdV) induced diseases and strategies for their control–a review on the current global situation. Avian Pathol. (2018) 47:111–26. doi: 10.1080/03079457.2017.1385724
5. Awad AM, Ali AB, Abd El-Hamid HS, El-Naggar AL, Sediek ME, El-Shall NA, et al. Epidemiological studies on H5N1 and H9N2 avian influenza viruses during late 2013 and 2015 in Egypt. Alex J Vet Sci. (2016) 51:164–73. doi: 10.5455/ajvs.221099
6. Artois J, Ippoliti C, Conte A, Dhingra MS, Alfonso P, El Tahawy A, et al. Avian influenza A (H5N1) outbreaks in different poultry farm types in Egypt: the effect of vaccination, closing status and farm size. BMC Vet Res. (2018) 14:187. doi: 10.1186/s12917-018-1519-8
7. El-Shall NA, Sedeek ME, El-badawy MM, Hussein EG, Awad AM. Phylogenetic characterization of infectious bursal disease (IBD) viruses isolated from field outbreaks in chickens from Behera and Alexandria governorates, Egypt. Alex J Vet Sci. (2018) 56:153–61. doi: 10.5455/ajvs.284794
8. Sedeik ME, El-Shall NA, Awad AM, Saif MA, Elsayed HD. Surveillance and molecular characterization of Newcastle disease virus from chickens in North Egypt during 2015-2017. Alex J Vet Sci. (2019) 62:82–90. doi: 10.5455/ajvs.55709
9. Van den Ende M, Don PA, Kipps A. The isolation in eggs of a new filterable agent which may be the cause of bovine lumpy skin disease. J Gen Microbiol. (1949) 3:174–83. doi: 10.1099/00221287-3-2-174
10. Olson NO. A respiratory disease (bronchitis) of quail caused by a virus. in Proceedings of the 54th Annual Meeting of US Livestock Sanitation Association. Baltimore, MD: Waverly Press (1950). p. 171–4.
11. Yamaguchi M, Miyaoka Y, Hasan MA, Kabir MH, Shoham D, Murakami H, et al. Isolation and molecular characterization of fowl adenovirus and avian reovirus from Breeder chickens in Japan in 2019-2021. J Vet Med Sci. (2022) 84:238–43. doi: 10.1292/jvms.21-0616
12. Helmboldt CF, Frazier MN. Avian hepatic inclusion bodies of unknown significance. Avian Dis. (1963) 7:446–50. doi: 10.2307/1587881
13. Mandelli G, Rinaldi A, Cervio G. A disease involving the spleen and lungs in pheasants: epidemiology, symptoms, and lesions. Clin Vet. (1966) 89:129–38.
14. Carlson HC, Al-Sheikhly F, Pettit JR, Seawright GL. Virus particles in spleens and intestines of turkeys with hemorrhagicenteritis. Avian Dis. (1974) 18:67–73. doi: 10.2307/1589243
15. Pomeroy BS, Fenstermacher R. Hemorrhagic enteritis in turkeys. Poult Sci. (1937) 16:378–82. doi: 10.3382/ps.0160378
16. Van Eck JH, Davelaar FG, Heuvel-Plesman TA, Van Kol N, Kouwenhoven B, Guldie FH. Dropped egg production, soft shelled and shell-less eggs associated with appearance of precipitins to adenovirus in flocks of laying fowls. Avian Pathol. (1976) 5:261–72. doi: 10.1080/03079457608418195
17. McFerran JB, Rowley HM, McNulty MS, Montgomery LJ. Serological studies on flocks showing depressed egg production. Avian Pathol. (1977) 6:405-13. doi: 10.1080/03079457708418249
18. Domermuth CH, Harris JR, Gross WB, DuBose RT. A naturally occurring infection of chickens with a hemorrhagic enteritis/marble spleen disease type of virus. Avian Dis. (1979) 23:479–84. doi: 10.2307/1589578
19. Khawaja DA, Ahmad S, Racuf MA, Zulfiqar MZ, Mahmood SMI, Hassan M. Isolation of an adenovirus from hydropericardium syndrome in broiler chicks. Pak J Vet Res. (1988) 1:2–17.
20. Tanimura N, Nakamura K, Imai K, Maeda M, Gobo T, Nitta S, et al. Necrotizing pancreatitis and gizzard erosion associated with adenovirus infection in chickens. Avian Dis. (1993) 37:606–11. doi: 10.2307/1591697
21. Chen L, Yin L, Zhou Q, Peng P, Du Y, Liu L, et al. Epidemiological investigation of fowl adenovirus infections in poultry in China during 2015–2018. BMC Vet Res. (2019) 15:271. doi: 10.1186/s12917-019-1969-7
22. Li PH, Zheng PP, Zhang TF, Wen GY, Shao HB, Luo QP. Fowl adenovirus serotype 4: epidemiology, pathogenesis, diagnostic detection, and vaccine strategies. Poult Sci. (2017) 96:2630–40. doi: 10.3382/ps/pex087
23. Shah MS, Ashraf A, Khan MI, Rahman M, Habib M, Chughtai MI, et al. Fowl adenovirus: history, emergence, biology and development of a vaccine against hydropericardium syndrome. Arch Virol. (2017) 162:1833–43. doi: 10.1007/s00705-017-3313-5
24. Hess M. Detection and differentiation of avian adenoviruses: a review. Avian Pathol. (2000) 29:195–206. doi: 10.1080/03079450050045440
25. Bertran K, Blanco A, Antilles N, Nofrarías M, Valle RM, Cobos À, et al. A 10-year retrospective study of inclusion body hepatitis in meat-type chickens in Spain (2011-2021). Viruses. (2021) 28:2170. doi: 10.3390/v13112170
26. Cui J, Xu Y, Zhou Z, Xu Q, Wang J, Xiao Y, et al. Pathogenicity and molecular typing of fowl adenovirus-associated with hepatitis/hydropericardium syndrome in Central China (2015-2018). Front Vet Sci. (2020) 7:190. doi: 10.3389/fvets.2020.00190
27. Wilson FD, Wills RW, Senties-Cue CG, Maslin WR, Stayer PA, Magee DL. High incidence of glomerulonephritis associated with inclusion body hepatitis in broiler chickens: routine histopathology and histomorphometric studies. Avian Dis. (2010) 54:975–80. doi: 10.1637/9050-090709-Reg.1
28. Mete A, Armien AG, Rejmanek D, Mott M, Crossley BM. Emergence of fowl aviadenovirus C-4 in a backyard chicken flock in California. J Vet Diagn Invest. (2021) 33:806–9. doi: 10.1177/10406387211019962
29. Philippe C, Grgic H, Nagy É. Inclusion body hepatitis in young broiler breeders associated with a serotype 2 adenovirus in Ontario, Canada. J Appl Poult Res. (2005) 14:588–93. doi: 10.1093/japr/14.3.588
30. Dar A, Gomis S, Shirley I, Mutwiri G, Brownlie R, Potter A, et al. Pathotypic and molecular characterization of a fowl adenovirus associated with inclusion body hepatitis in Saskatchewan chickens. Avian Dis. (2012) 56:73–81. doi: 10.1637/9764-041911-Reg.1
31. Mase M, Hiramatsu K, Nishijima N, Iguchi H, Honda S, Hanyu S, et al. Fowl adenoviruses type 8b isolated from chickens with inclusion body hepatitis in Japan. Avian Dis. (2020) 64:330–4. doi: 10.1637/aviandiseases-D-20-00028
32. Choi KS, Kye SJ, Kim JY, Jeon WJ, Lee EK, Park KY, et al. Epidemiological investigation of outbreaks of fowl adenovirus infection in commercial chickens in Korea. Poult Sci. (2012) 91:2502–6. doi: 10.3382/ps.2012-02296
33. Chitradevi S, Sukumar K, Suresh P, Balasubramaniam GA, Kannan D. Molecular typing and pathogenicity assessment of fowl adenovirus associated with inclusion body hepatitis in chicken from India. Trop Anim Health Prod. (2021) 53:412. doi: 10.1007/s11250-021-02851-8
34. Sharif N, Mehmood MD, Naqvi SZH, Ul-Haq HA, Ahmed SS, Ghani MU, et al. PCR based detection and phylogenetic analysis of fowl adenovirus strains isolated from 2019 epidemic from Punjab and Sindh, Pakistan. Am J Mol Biol. (2020) 10:246–58. doi: 10.4236/ajmb.2020.103016
35. Niczyporuk JS, Kozdrun W, Czekaj H, Piekarska K, Stys-Fijol N. Characterisation of adenovirus strains represented species B and E isolated from broiler chicken flocks in eastern Poland. Heliyon. (2021) 7:e06225. doi: 10.1016/j.heliyon.2021.e06225
36. Mirzazadeh A, Asasi K, Mosleh N, Abbasnia M, Abdi Hachesoo B. A primary occurrence of inclusion body hepatitis in absence of predisposing agents in commercial broilers in Iran: a case report. Iran J Vet Res. (2020) 21:314–8.
37. Vera-Hernández PF, Morales-Garzón A, Cortés-Espinosa DV, Galiote-Flores A, García-Barrera LJ, Rodríguez-Galindo ET, et al. Clinicopathological characterization and genomic sequence differences observed in a highly virulent fowl aviadenovirus serotype 4. Avian Pathol. (2016) 45:73–81. doi: 10.1080/03079457.2015.1125443
38. De la Torre D, Nuñez LFN, Parra SHS, Astolfi-Ferreira CS, Ferreira AJP. Molecular characterization of fowl adenovirus group I in commercial broiler chickens in Brazil. Virus Dis. (2018) 29:83–8. doi: 10.1007/s13337-018-0430-z
39. Zadravec M, Slavec B, Krape, Ž, U., Kaján GL, Račnik J, Cizerl RJ, et al. Inclusion body hepatitis (IBH) outbreaks in broiler chickens in Slovenia. In: IX Simpozij Peradarski dani, S medunarodnim sudjelovanjem; 2011 May 11-14; Sibenik, Croatia. Zagreb: Centar za Peradarstvo (2011). p. 36–9.
40. Steer PA, O'Rourke D, Ghorashi SA, Noormohammadi AH. Application of high resolution melting curve analysis for typing of fowl adenoviruses in field cases of inclusion body hepatitis. Aust Vet J. (2011) 89:184–92. doi: 10.1111/j.1751-0813.2011.00695.x
41. Franzo G, Prentza Z, Paparounis T, Tsiouris V, Centonze G, Legnardi M, et al. Molecular epidemiology of fowl adenoviruses in Greece. Poult Sci. (2020) 99:5983–90. doi: 10.1016/j.psj.2020.07.019
42. Abdulrahman NR, Saeed NM, Dyary HO, Mohamad SF, Sulaiman RR, Rashid PMA, et al. Outbreaks of inclusion body hepatitis caused by fowl adenovirus in commercial broiler farms in the Kurdistan region, North Iraq from 2013 to 2021. Pak Vet J Vol. (2022) 42:201–7. doi: 10.1637/9813-052511-case.1
43. Alzuheir IM, Jalboush NH, Fayyad AF, Daibes RA. Phylogenetic analysis and pathological characterization of fowl adenovirus isolated during inclusion body hepatitis outbreak in Tubas, Palestine. Vet Res Forum. (2021) 12:511–4. doi: 10.30466/vrf.2020.116932.2778
44. Ishag HZA, Terab AMA, El Tigani-Asil ETA, Bensalah OK, Khalil NAH, Khalafalla AI, et al. Pathology and molecular epidemiology of fowl adenovirus serotype 4 outbreaks in broiler chicken in Abu Dhabi Emirate, UAE. Vet Sci. (2022) 9:154. doi: 10.3390/vetsci9040154
45. Hemida MG, Al-Hammadi M. Prevalence and molecular characteristics of fowl adenovirus serotype 4 in eastern Saudi Arabia. Turk J Vet Anim Sci. (2017) 41:506–13. doi: 10.3906/vet-1612-19
46. Abghour S, Zro K, Mouahid M, Tahiri F, Tarta M, Berrada J, et al. Isolation and characterization of fowl aviadenovirus serotype 11 from chickens with inclusion body hepatitis in Morocco. PLoS One. (2019) 14:e0227004. doi: 10.1371/journal.pone.0227004
47. Joubert HW, Aitchison H, Maartens LH, Venter EH. Molecular differentiation and pathogenicity of aviadenoviruses isolated during an outbreak of inclusion body hepatitis in South Africa. J S Afr Vet Assoc. (2014) 85:1058. doi: 10.4102/jsava.v85i1.1058
48. Mousa S, Bayoumi A, Gad N, El-Demerdach M. Inclusion body hepatitis complex in chicken at Elwaady-Elgadeed, Egypt. Assiut Vet Med J. (1984) 13:83–94.
49. El-Shamy SA. Clinicopathological study on an outbreak of inclusion body hepatitis in broiler chickens. Assiut Vet Med J. (2003) 49:239–49. doi: 10.21608/avmj.2003.178322
50. Radwan MM, El-Deeb AH, Mousa MR, El-Sanousi AA, Shalaby MA. First report of fowl adenovirus 8a from commercial broiler chickens in Egypt: molecular characterization and pathogenicity. Poult Sci. (2019) 98:97–104. doi: 10.3382/ps/pey314
51. El-Tholoth M, Abou El-Azm KI. Molecular detection and characterization of fowl adenovirus associated with inclusion body hepatitis from broiler chickens in Egypt. Trop Anim Health Prod. (2019) 51:1065–71. doi: 10.1007/s11250-018-01783-0
52. Elbestawy AR, Ibrahim M, Hammam H, Noreldin AE, El Bahrawy A, Ellakany HF. Molecular characterization of fowl adenovirus D species in broiler chickens with inclusion body hepatitis in Egypt. Alex J Vet Sci. (2020) 64:110–7. doi: 10.5455/ajvs.74411
53. El-Basrey YF, Hamed RI, Mohamed M, Lebdah MA. Detection of inclusion body hepatitis virus in broilers at Sharkia province, Egypt. J Anim Health Prod. (2020) 9:84–9. doi: 10.17582/journal.jahp/2020/9.s1.84.89
54. Lebdah M, Alshaya DS, Jalal AS, Mousa MR, Radwan MM, Samir M, et al. Molecular characterization of aviadenovirus serotypes and pathogenicity of the identified adenovirus in broiler chickens. Poult Sci. (2022) 2022:101918. doi: 10.1016/j.psj.2022.101918
55. Sultan H, Arafa AE, Adel A, Selim K, Hossiny M, Talaat S. Molecular detection of a novel fowl adenovirus serotype-4 (FadV-4) from an outbreak of hepatitis hydropericardium syndrome in commercial Broiler chickens in Egypt. Avian Dis. (2021) 65:385–90. doi: 10.1637/0005-2086-65.3.385
56. Marek A, Nolte V, Schachner A, Berger E, Schlotterer C, Hess M. Two fiber genes of nearly equal lengths are a common and distinctive feature of fowl adenovirus C members. Vet Microbiol. (2012) 156:411–7. doi: 10.1016/j.vetmic.2011.11.003
57. Rowe WP, Huebner RJ, Gilmore LK, Parrott RH, Ward TG. Isolation of a cytopathogenic agent from human adenoids undergoing spontaneous degeneration in tissue culture. Proc Soc Exp Biol Med. (1953) 84:570–3. doi: 10.3181/00379727-84-20714
58. Harrach B, Kaján GL. Aviadenovirus. In:C Tidona, G Darai, , editors, The Springer Index of Viruses. New York, NY: Springer (2011). p. 13–28. doi: 10.1007/978-0-387-95919-1_2
59. McFerran JB, Adair BM. Avian adenoviruses—a review. Avian Pathol. (1977) 6:189–217. doi: 10.1080/03079457708418228
60. Tan PK, Michou AI, Bergelson JM, Cotton M. Defining CAR as a cellular receptor for the avian adenovirus CELO using a genetic analysis of the two viral fiber proteins. J Gen Virol. (2001) 82:1465–72. doi: 10.1099/0022-1317-82-6-1465
61. Schachner A, Marek A, Grafl B, Hess M. Detailed molecular analyses of the hexon loop-1 and fibers of fowl aviadenoviruses reveal new insights into the antigenic relationship and confirm that specific genotypes are involved in field outbreaks of inclusion body hepatitis. Vet Microbiol. (2016) 186:13–20. doi: 10.1016/j.vetmic.2016.02.008
62. Kajan GL, Affranio I, Bistyak AT, Kecskemeti S, Benko M. An emerging new fowl adenovirus genotype. Heliyon. (2019) 5:e01732. doi: 10.1016/j.heliyon.2019.e01732
63. Salzmann E, Müller E, Marschang RE. Detection of testadenoviruses and atadenoviruses in tortoises and turtles in Europe. J Zoo Wildl Med. (2021) 52:223–31. doi: 10.1638/2020-0078
64. Liu Y, Wan W, Gao D, Li Y, Yang X, Liu H, et al. Genetic characterization of novel fowl aviadenovirus 4 isolates from outbreaks of hepatitis-hydropericardium syndrome in broiler chickens in China. Emerg Microbes Infect. (2016) 5:e117. doi: 10.1038/emi.2016.115
65. Ono M, Okuda Y, Yazawa S, Shibata I, Sato S, Okada K. Outbreaks of adenoviral gizzard erosion in slaughtered broiler chickens in Japan. Vet Rec. (2003) 153:775–9. doi: 10.1136/vr.153.25.775
66. Hess M, Raue R, Prusas C. Epidemiological studies on fowl adenoviruses isolated from cases of infectious hydropericardium. Avian Pathol. (1999) 28:433–9. doi: 10.1080/03079459994443
67. Mohamed MHA, El-Sabagh IM, Abdelaziz AM, Al-Ali AM, Alramadan M, Lebdah MA, et al. Molecular characterization of fowl aviadenoviruses species D and E associated with inclusion body hepatitis in chickens and falcons indicates possible cross-species transmission. Avian Pathol. (2018) 47:384–90. doi: 10.1080/03079457.2018.1457769
68. Das S, Fearnside K, Sarker S, Forwood JK, Raidal SR. A novel pathogenic aviadenovirus from red-bellied parrots (Poicephalus rufiventris) unveils deep recombination events among avian host lineages. Virology. (2017) 502:188–97. doi: 10.1016/j.virol.2016.12.031
69. Marek A, Schulz E, Hess C, Hess M. Comparison of the fibers of fowl adenovirus A serotype 1 isolates from chickens with gizzard erosions in Europe and apathogenic reference strains. J Vet Diagn Invest. (2010) 22:937–41. doi: 10.1177/104063871002200613
70. Yates VJ, Fry DE. Observations on a chicken embryo lethal orphan (CELO) virus. Am J Vet Res. (1957) 18:657–60.
71. Reece RL, Grix DC, Barr DA. An unusual case of inclusion body hepatitis in a cockerel. Avian Dis. (1986) 30:224–7. doi: 10.2307/1590640
72. Fadly AM, Riegle BJ, Nazerian K, Stephens EA. Some observations on an adenovirus isolated from specific pathogen free chickens. Poult Sci. (1980) 59:21–7. doi: 10.3382/ps.0590021
73. Girshick T, Crary CK, Luginbuhl RE. Serologic detection of adenovirus infections in specific-pathogen-free chickens. Avian Dis. (1980) 24:527–31. doi: 10.2307/1589725
74. Saifuddin M, Wilks CR. Pathogenesis of an acute viral hepatitis: inclusion body hepatitis in the chicken. Arch Virol. (1991) 116:33–43. doi: 10.1007/BF01319229
75. Wang J, Zaheer I, Saleemi MK, Qi X, Gao Y, Cui H, et al. The first complete genome sequence and pathogenicity characterization of fowl adenovirus 11 from chickens with inclusion body hepatitis in Pakistan. Vet Microbiol. (2020) 244:108670. doi: 10.1016/j.vetmic.2020.108670
76. Sabarudin NS, Tan SW, Phang YF, Omar AR. Molecular characterization of Malaysian fowl adenovirus (FAdV) serotype 8b species E and pathogenicity of the virus in specific-pathogen-free chicken. J Vet Sci. (2021) 22:e42. doi: 10.4142/jvs.2021.22.e42
77. Abghour S, Mouahid M, Darkaoui S, Berrada J, Zro K, Kichou F. Pathogenicity of field strain of fowl aviadenovirus serotype 11 isolated from chickens with inclusion body hepatitis in Morocco. PLoS One. (2021) 16:e0261284. doi: 10.1371/journal.pone.0261284
78. Nakamura K, Mase M, Yamaguchi S, Yuasa N. Induction of hydropericardium in one-day-old specific-pathogen-free chicks by adenoviruses from inclusion body hepatitis. Avian Dis. (2000) 44:192–6. doi: 10.2307/1592524
79. Matos M, Grafl B, Liebhart D, Hess M. The outcome of experimentally induced inclusion body hepatitis (IBH) by fowl aviadenoviruses (FAdVs) is crucially influenced by the genetic background of the host. Vet Res. (2016) 47:69. doi: 10.1186/s13567-016-0350-0
80. Pan Q, Liu L, Gao Y, Liu C, Qi X, Zhang Y, et al. Characterization of a hypervirulent fowl adenovirus 4 with the novel genotype newly prevalent in China and establishment of reproduction infection model of hydropericardium syndrome in chickens. Poult Sci. (2017) 96:1581–8. doi: 10.3382/ps/pew431
81. Yu X, Wang Z, Chen H, Niu X, Dou Y, Yang J, et al. Serological and pathogenic analyses of fowl adenovirus serotype 4 (FAdV-4) strain in muscovy ducks. Front Microbiol. (2018) 9:1163. doi: 10.3389/fmicb.2018.01163
82. Sun J, Zhang Y, Gao S, Yang J, Tang Y, Diao Y. Pathogenicity of fowl adenovirus serotype 4 (FAdV-4) in chickens. Infect Genet Evol. (2019) 75:104017. doi: 10.1016/j.meegid.2019.104017
83. Yin D, Xue M, Yang K, Xiong X, Geng S, Tu J, et al. Molecular characterization and pathogenicity of highly pathogenic fowl adenovirus serotype 4 isolated from laying flock with hydropericardium-hepatitis syndrome. Microb Pathog. (2020) 147:104381. doi: 10.1016/j.micpath.2020.104381
84. Steer PA, Sandy JR, O'Rourke D, Scott PC, Browning GF, Noormohammadi AH. Chronological analysis of gross and histological lesions induced by field strains of fowl adenovirus serotypes 1, 8b and 11 in one-day-old chickens. Avian Pathol. (2015) 44:106–13. doi: 10.1080/03079457.2015.1007919
85. Jones RC, Georgiou K. Experimental infection of chickens with adenoviruses isolated from tenosynovitis. Avian Pathol. (1984) 13:13–23. doi: 10.1080/03079458408418504
86. Cook JK. Fowl adenoviruses: studies on aspects of the pathogenicity of six strains for 1-day-old chicks. Avian Pathol. (1983) 12:35–43. doi: 10.1080/03079458308436147
87. Matos M, Grafl B, Liebhart D, Schwendenwein I, Hess M. Selected clinical chemistry analytes correlate with the pathogenesis of inclusion body hepatitis experimentally induced by fowl aviadenoviruses. Avian Pathol. (2016) 45:520–9. doi: 10.1080/03079457.2016.1168513
88. Reece RL, Barr DA, Grix DC. Pathogenicity studies with a strain of fowl adenovirus serotype 8 (VRI-33) in chickens. Aust Vet J. (1987) 64:365–7. doi: 10.1111/j.1751-0813.1987.tb09604.x
89. Christensen NH, Saifuddin M. A primary epidemic of inclusion body hepatitis in broilers. Avian Dis. (1989) 33:622–30. doi: 10.2307/1591135
90. Gomis S, Goodhope AR, Ojkic AD, Willson P. Inclusion body hepatitis as a primary disease in broilers in Saskatchewan, Canada. Avian Dis. (2006) 50:550–5. doi: 10.1637/7577-040106R.1
91. Hess M. Commensal or pathogen–a challenge to fulfil Koch's postulates. Br Poult Sci. (2017) 58:1–12. doi: 10.1080/00071668.2016.1245849
92. Erny KM, Barr DA, Fahey KJ. Molecular characterization of highly virulent fowl adenoviruses associated with outbreaks of inclusion body hepatitis. Avian Pathol. (1991) 20:597–606. doi: 10.1080/03079459108418799
93. Slaine PD, Ackford JG, Kropinski AM, Kozak RA, Krell PJ, Nagy E. Molecular characterization of pathogenic and nonpathogenic fowl aviadenovirus serotype 11 isolates. Can J Microbiol. (2016) 62:993–1002. doi: 10.1139/cjm-2016-0297
94. Kaján GL, Kecskemeti S, Harrach B, Benko M. Molecular typing of fowl adenoviruses, isolated in Hungary recently, reveals high diversity. Vet Microbiol. (2013) 167:357–63. doi: 10.1016/j.vetmic.2013.09.025
95. Niczyporuk JS. Phylogenetic and geographic analysis of fowl adenovirus field strains isolated from poultry in Poland. Arch Virol. (2016) 161:33–42. doi: 10.1007/s00705-015-2635-4
96. Fadly AM, Winterfield RW. Isolation and some characteristics of an agent associated with inclusion body hepatitis, hemorrhages, and aplastic anemia in chickens. Avian Dis. (1973) 17:182–93. doi: 10.2307/1588936
97. Hoffmann R, Wessling E, Dorn P, Dangschat H. Lesions in chickens with spontaneous or experimental infectious hepato-myelopoietic disease (inclusion body hepatitis) in Germany. Avian Dis. (1975) 19:224–36. doi: 10.2307/1588976
98. Rosenberger J, Klopp S, Eckroade RJ, Krauss WC. The role of the infectious bursal agent and several avian adenoviruses in the hemorrhagic-aplastic-anemia syndrome and gangrenous dermatitis. Avian Dis. (1975) 19:717–29. doi: 10.2307/1589185
99. Sandhu BS, Singh H, Singh B. Pathological studies in broiler chicks fed aflatoxin or ochratoxin and inoculated with inclusion body hepatitis virus singly and in concurrence. Vet Res Commun. (1995) 19:27–37. doi: 10.1007/BF01839249
100. Meng F, Dong G, Zhang Y, Tian S, Cui Z, Chang S, et al. Co-infection of fowl adenovirus with different immunosuppressive viruses in a chicken flock. Poult Sci. (2018) 97:1699–705. doi: 10.3382/ps/pex414
101. Goodwin MA, Hill DL, Dekich MA, Putman MR. Multisystemic adenovirus infection in broiler chicks with hypoglycemia and spiking mortality. Avian Dis. (1993) 37:625–27. doi: 10.2307/1591701
102. Guy JS, Barnes HJ. Characterization of an avian adenovirus associated with inclusion body hepatitis in day-old turkeys. Avian Dis. (1997) 41:726–31. doi: 10.2307/1592167
103. Pilkington P, Brown T, Villegas P, McMurray B, Page RK, Rowland GN, et al. Adenovirus-induced inclusion body hepatitis in four-day-old broiler breeders. Avian Dis. (1997) 41:472–4. doi: 10.2307/1592208
104. Hafez HM. Avian adenovirus infections with special attention to inclusion body hepatitis/ hydropericardium syndrome and egg drop syndrome. Pak Vet J. (2011) 31:85–92. doi: 10.17169/refubium-18486
105. Clemmer D. Age-associated changes in fecal excretion patterns of strain 93 chick embryo lethal orphan virus in chicks. Infect Immun. (1972) 5:60–4. doi: 10.1128/iai.5.1.60-64.1972
106. Cook JK. Pathogenicity of avian adenoviruses for day-old chicks. J Comp Pathol. (1974) 84:505–15. doi: 10.1016/0021-9975(74)90043-7
107. Lim TH, Lee HJ, Lee DH, Lee YN, Park JK, Youn HN, et al. Identification and virulence characterization of fowl adenoviruses in Korea. Avian Dis. (2011) 55:554–60. doi: 10.1637/9730-032011-Reg.1
108. Alvarado IR, Villegas P, El-Attrache J, Jensen E, Rosales G, Perozo F, et al. Genetic characterization, pathogenicity, and protection studies with an avian adenovirus isolate associated with inclusion body hepatitis. Avian Dis. (2007) 51:27–32. doi: 10.1637/0005-2086(2007)051[0027:GCPAPS]2.0.CO;2
109. Gupta A. Pathogenesis and Control of Inclusion Body Hepatitis in Broiler Chickens. (Ph.D. thesis), University of Saskatchewan, Saskatoon, SK, Canada (2018).
110. Eregae ME, Dewey CE, McEwen SA, Ouckama R, Ojkic D, Guerin MT. Flock prevalence of exposure to avian adeno-associated virus, chicken anemia virus, fowl adenovirus, and infectious bursal disease virus among Ontario broiler chicken flocks. Avian Dis. (2014) 58:71–7. doi: 10.1637/10612-071113-Reg.1
111. Ruan SF, Zhao J, Ren YC, Feng JL, Zhang GZ. Phylogenetic analyses of fowl adenoviruses (FAdV) isolated in China and pathogenicity of a FAdV-8 isolate. Avian Dis. (2017) 61:353–7. doi: 10.1637/11671-050817-RegR
112. Niczyporuk JS. Molecular characterization of fowl adenovirus type 7 isolated from poultry associated with inclusion body hepatitis in Poland. Arch Virol. (2017) 162:1325–33. doi: 10.1007/s00705-017-3240-5
113. Mittal D, Jindal N, Tiwari AK, Khokhar RS. Characterization of fowl adenoviruses associated with hydropericardium syndrome and inclusion body hepatitis in broiler chickens. Virus Dis. (2014) 25:114–9. doi: 10.1007/s13337-013-0183-7
114. Li L, Luo L, Luo Q, Zhang T, Zhao K, Wang H, et al. Genome sequence of a fowl adenovirus serotype 4 strain lethal to chickens, isolated from China. Genome Announc. (2016) 4:e00140–e00116. doi: 10.1128/genomeA.00140-16
115. Gowda RNS, Satyanarayana ML. Hydropericardium syndrome in poultry. Indian J Vet Pathol. (1994) 18:159–61.
116. Abdul-Aziz TA, Al-Attar MA. New syndrome in Iraqi chicks. Vet Rec. (1991) 129:272. doi: 10.1136/vr.129.12.272
117. Borisov VV, Borisov AV, Gusev AA. Hydropericardium syndrome in chickens in Russia. In: Proceedings of 10th International Congress of the WVPA; 1997 August 18-22; Budapest, Hungary. (1997). p. 258.
118. Kim JN, Byun SH, Kim MJ, Kim JJ, Sung HW, Mo IP. Outbreaks of hydropericardium syndrome and molecular characterization of Korean fowl adenoviral isolates. Avian Dis. (2008) 52:526–30. doi: 10.1637/8178-112207-Case
119. Nakamura K, Mase M, Yamamoto Y, Takizawa K, Kabeya M, Wakuda T, et al. Inclusion body hepatitis caused by fowl adenovirus in broiler chickens in Japan, 2009–2010. Avian Dis. (2011) 55:719–23. doi: 10.1637/9813-052511-Case.1
120. Shukla SK, Chandra R, Kumar M. Outbreaks of hydropericardium syndrome in layer flocks of poultry in India. Ind J Vet Med. (1997) 17:61–4.
121. Dahiya S, Srivastava RN, Hess M, Gulati BR. Fowl adenovirus serotype 4 associated with outbreaks of infectious hydropericardium in Haryana, India. Avian Dis. (2002) 46:230–3. doi: 10.1637/0005-2086(2002)046[0230:FASAWO]2.0.CO;2
122. Mansoor MK, Hussain I, Arshad M, Muhammad G, Hussain MH, Mehmood MS. Molecular characterization of fowl adenovirus serotype 4 (FAV-4) isolate associated with fowl hydropericardium-hepatitis syndrome in Pakistan. Pak J Zool. (2009) 41:269–76.
123. Mase M, Nakamura K, Imada T. Characterization of Fowl adenovirus serotype 4 isolated from chickens with hydropericardium syndrome based on analysis of the short fiber protein gene. J Vet Diagn Invest. (2010) 22:218–23. doi: 10.1177/104063871002200207
124. Thakor KB, Dave CJ, Prajapati KS, Fefar DT, Jivani BM. Molecular characterization of avian adeno virus causing inclusion body hepatitis–hydropericardium syndrome in broiler chickens of Anand, Gujarat, India. Vet World. (2012) 5:178–82. doi: 10.5455/vetworld.2012.178-182
125. Anjum AD, Sabri MA, Iqbal Z. Hydropericarditis syndrome in broiler chickens in Pakistan. Vet Rec. (1989) 124:247–8. doi: 10.1136/vr.124.10.247
126. Asrani RK, Gupta VK, Sharma SK. Hydropericardium-hepatopathy syndrome in Asian poultry. Vet Rec. (1997) 141:271–3. doi: 10.1136/vr.141.11.271
127. Niazi AK, Khan MZ, Siddique M. Hematological studies on naturally occurring hydropericardium syndrome in broiler chicks. Vet Rec. (1989) 125:400. doi: 10.1136/vr.125.15.400
128. Mahmood T, Hussain Z. Propagation of angara disease virus in duck's embryonated eggs. Pak J Livestock and Poultry. (1995) 1:104–5.
129. Bhatti BM, Qureshi MS, And Bajwa TM. Hematology and clinical chemistry values in broiler chickens affected with hydropericardium syndrome prevalent in Pakistan. Vet Arh. (1989) 50:107–11.
130. Zaman T, Khan MZ. Serum enzyme [LDH, PK, AP, SGPT and SGOT] profiles in hydropericardium affected broiler chicks. Pak Vet, J. (1991) 11:50–2.
131. Iqbal MJ, Bhatti BM, Khan KM. Studies on serum enzymes in hydropericardium syndrome (Angara disease) of broilers. Pak Vet J. (1994) 14:83–5.
132. Benko M, Elo P, Ursu K, Ahne W, LaPatra SE, Thomon D, et al. First molecular evidence for the existence of distinct fish and snake adenoviruses. J Virol. (2002) 76:10056–9. doi: 10.1128/JVI.76.19.10056-10059.2002
133. Zhao J, Zhong Q, Zhao Y, Hu YX, Zhang GZ. Pathogenicity and complete genome characterization of fowl adenoviruses isolated from chickens associated with inclusion body hepatitis and hydropericardium syndrome in China. PLoS One. (2015) 10:e0133073. doi: 10.1371/journal.pone.0133073
134. Nakamura K, Mase M, Yamaguchi S, Shibahara T, Yuasa N. Pathologic study of specific pathogen-free chicks and hens inoculated with adenovirus isolated from hydropericardium syndrome. Avian Dis. (1999) 43:414–23. doi: 10.2307/1592638
135. Ivanics E, Palya V, Markos B, Dan A, Ursu K, Harrach B, et al. Hepatitis and hydropericardium syndrome associated with adenovirus infection in goslings. Acta Vet Hung. (2010) 58:47–58. doi: 10.1556/avet.58.2010.1.5
136. Asthana M, Chandra R, Kumar R. Hydropericardium syndrome: current state and future developments. Arch Virol. (2013) 158:921–31. doi: 10.1007/s00705-012-1570-x
137. Ren G, Wang H, Yan Y, Liu F, Huang M, Chen R. Pathogenicity of a fowl adenovirus serotype 4 isolated from chickens associated with hydropericardium-hepatitis syndrome in China. Poult Sci. (2019) 98:2765–71. doi: 10.3382/ps/pez042
138. Niu YJ, Sun W, Zhang GH, Qu YJ, Wang PF, Sun HL, et al. Hydropericardium syndrome outbreak caused by fowl adenovirus serotype 4 in China in 2015. J Gen Virol. (2016) 97:2684–90. doi: 10.1099/jgv.0.000567
139. Afzal M, Muneer R, Stein G. Studies on the aetiology of hydropericardium syndrome (Angara disease) in broilers. Vet Rec. (1991) 128:591–3. doi: 10.1136/vr.128.25.591
140. Kumar R, Chandra R, Shukla SK, Agrawal DK, Kumar M. Hydropericardium syndrome (HPS) in India: a preliminary study on the causative agent and control of the disease by inactivated autogenous vaccine. Trop Anim Health. Prod. (1997) 29:158–64. doi: 10.1007/BF02633014
141. Dutta B, Deka P, Gogoi SM, Sarmah M, Bora MK, Pathak DC. Pathology of inclusion body hepatitis hydropericardium syndrome (IBH-HPS) in broiler chicken. Int J Chem Stud. (2017) 5:458–61.
142. Mariappan AK, Munusamy P, Latheef SK, Singh SD, Dhama K. Hepato nephropathology associated with inclusion body hepatitis complicated with citrinin mycotoxicosis in a broiler farm. Vet World. (2018) 11:112–7. doi: 10.14202/vetworld.2018.112-117
143. Chen H, Dou Y, Zheng X, Tang Y, Zhang M, Zhang Y, et al. Hydropericardium hepatitis syndrome emerged in Cherry Valley ducks in China. Transbound Emerg Dis. (2016) 64:1262–7. doi: 10.1111/tbed.12500
144. Naeem K, Niazi T, Malik SA, Cheema AH. Immunosuppressive potential and pathogenicity of an avian adenovirus isolate involved in hydropericardium syndrome in broilers. Avian Dis. (1995) 39:723–8. doi: 10.2307/1592408
145. Schonewille E, Singh A, Gobel TW, Gerner W, Saalmuller A, Hess M. Fowl adenovirus (FAdV) serotype 4 causes depletion of B and T cells in lymphoid organs in specific pathogen-free chickens following experimental infection. Vet Immunol Immunopathol. (2008) 121:130–9. doi: 10.1016/j.vetimm.2007.09.017
146. Sharma S, Asrani RK, Singh G, Gulathi BR, Patil PK, Gupta VK. Outbreak of hydropericardium syndrome associated with ascites and liver rupture in caged broilers. Vet Res Int. (2014) 2:33–45.
147. Ahamad DB, Selvaraj J, Sasikala M, Prasath NB. Pathological study of an outbreak of hydropericardium syndrome in Giriraja chicken. Indian J Vet Pathol. (2017) 41:53–6. doi: 10.5958/0973-970X.2017.00012.8
148. Singh A, Grewal GS, Maiti NK, Oberoi MS. Effect of fowl adenovirus-1 (IBH isolate) on humoral and cellular immune competency of broiler chicks. Comp Immunol Microbiol Infect Dis. (2006) 29:315–21. doi: 10.1016/j.cimid.2006.08.001
149. Ye J, Liang G, Zhang J, Wang W, Song N, Wang P, et al. Outbreaks of serotype 4 fowl adenovirus with novel genotype, China. Emerg Microbes Infect. (2016) 5:e50. doi: 10.1038/emi.2016.50
150. Harrach B, Benk M, Both GW, Brown M, Davison AJ, Echavarria M, et al. Family adenoviridae. In:AMQ King, MJ Adams, EB Carstens, EJ Lefkowitz, , editors, Virus Taxonomy: Ninth Report of the International Committee on Taxonomy of Viruses. New York, NY: Elsevier Academic Press. (2012). p. 125–41. doi: 10.1016/B978-0-12-384684-6.00009-4
151. Meulemans G, Couvreur B, Decaesstecker M, Boschmans M, van den Berg TP. Phylogenetic analysis of fowl adenoviruses. Avian Pathol. (2004) 33:164–70. doi: 10.1080/03079450310001652086
152. Xie Z, Tang Y, Fan Q, Liu J, Pang Y, Deng X, et al. Rapid detection of group I avian adenoviruses by a loop-mediated isothermal amplification. Avian Dis. (2011) 55:575–9. doi: 10.1637/9719-031611-Reg.1
153. Niczyporuk JS, Wozniakowski G, Samorek-Salamonowicz E. Application of cross-priming amplification (CPA) for detection of fowl adenovirus (FAdV) strains. Arch Virol. (2015) 160:1005–13. doi: 10.1007/s00705-015-2355-9
154. Wang J, Wang J, Chen P, Liu L, Yuan W. Development of a TaqMan-based real-time PCR assay for rapid and specific detection of fowl aviadenovirus serotype 4. Avian Pathol. (2017) 46:338–43. doi: 10.1080/03079457.2016.1278428
155. Steer PA, Kirkpatrick NC, O'Rourke D, Noormohammadi AH. Classification of fowl adenovirus serotypes by use of high-resolution melting-curve analysis of the hexon gene region. J Clin Microbiol. (2009) 47:311–21. doi: 10.1128/JCM.01567-08
156. Pizzuto MS, DeBattisti C, Marciano S, Capua I, Cattoli G. Pyrosequencing analysis for a rapid classification of fowl adenovirus species. Avian Pathol. (2010) 39:391–8. doi: 10.1080/03079457.2010.510499
157. Raue R, Hafez HM, Hess M. A fiber gene-based polymerase chain reaction for specific detection of pigeon adenovirus. Avian Pathol. (2002) 31:95–9. doi: 10.1080/03079450120106660
158. Romanova N, Corredor JC, Nagy E. Detection and quantitation of fowl adenovirus genome by a real-time PCR assay. J Virol Methods. (2009) 159:58–63. doi: 10.1016/j.jviromet.2009.02.026
159. Günes A, Marek A, Grafl B, Berger E, Hess M. Real-time PCR assay for universal detection and quantitation of all five species of fowl adenoviruses (FAdV-A to FAdV-E). J Virol Methods. (2012) 183:147–53. doi: 10.1016/j.jviromet.2012.04.005
160. Grgic H, Yang DH, Nagy E. Pathogenicity and complete genome sequence of a fowl adenovirus serotype 8 isolate. Virus Res. (2011) 156:91–7. doi: 10.1016/j.virusres.2011.01.002
161. Chiocca S, Kurzbauer R, Schaffner G, Baker A, Mautner V, Cotton M. The complete DNA sequence and genomic organization of the avian adenovirus CELO. J Virol. (1996) 70:2939–49. doi: 10.1128/jvi.70.5.2939-2949.1996
162. Raue R, Hess M. Hexon based PCRs combined with restriction enzyme analysis for rapid detection and differentiation of fowl adenoviruses and egg drop syndrome virus. J Virol Methods. (1998) 73:211–7. doi: 10.1016/S0166-0934(98)00065-2
163. Sohaimi NM, Hair-Bejo M. A recent perspective on fiber and hexon genes proteins analyses of fowl adenovirus toward virus infectivity-a review. Open Vet J. (2021) 11:569–80. doi: 10.5455/OVJ.2021.v11.i4.6
164. Dhama K, Sawant PM, Kumar D, Kumar R. Diagnostic applications of molecular tools and techniques for important viral diseases of poultry. Poult World. (2011) 6:32–40. doi: 10.4061/2011/712369
165. Rehman LU, Sultan B, Ali I, Bhatti MA, Khan SU, Zaman KU, et al. Duplex PCR assay for the detection of avian adenovirus and chicken anemia virus prevalent in Pakistan. Virol J. (2011) 8:440. doi: 10.1186/1743-422X-8-440
166. Dhama K, Verma AK, Tiwari R, Chakraborty S, Vora K, Kapoor S, et al. A perspective on applications of geographical information system (GIS): an advanced tracking tool for disease surveillance and monitoring in veterinary epidemiology. Adv Anim Vet Sci. (2013) 1:14–24.
167. De Herdt P, Timmerman T, Defoort P, Lycke K, Jaspers R. Fowl adenovirus infections in Belgian broilers: a ten-year survey. Vlaams Diergeneeskd Tijdschr. (2013) 82:125–32. doi: 10.21825/vdt.v82i3.16704
169. Xie Z, Fadl AA, Girshick T, Khan MI. Detection of avian adenovirus by polymerase chain reaction. Avian Dis. (1999) 43:98–105. doi: 10.2307/1592767
170. Junnu S, Lertwatcharasarakul P, Jala S, Phattanakunanan S, Moonjit P, Songserm T. Developing an indirect ELISA based on recombinant hexon protein for serological detection of inclusion body hepatitis in chickens. J Vet Med Sci. (2014) 76:289–93. doi: 10.1292/jvms.13-0196
171. Rajasekhar R, Roy P. Recombinant hexon antigen based single serum dilution ELISA for rapid serological profiling against fowl adenovirus-4 causing hydropericardium syndrome in chickens. J Virol Methods. (2014) 207:121–7. doi: 10.1016/j.jviromet.2014.06.017
172. Kataria JM, Mohan MC, Dey S, Dash BB, Dhama K. Diagnosis and immunoprophylaxis of economically important poultry diseases: a review. Indian J Anim Sci. (2005) 75:555–67.
173. McFerran JB, Smyth JA. Avian adenoviruses. Rev Sci Tech. (2000) 19:589–601. doi: 10.20506/rst.19.2.1238
174. Inoue D, Hayashima A, Tanaka T, Ninomiya N, Tonogawa T, Nakazato S, et al. Virucidal effect of commercial disinfectants on fowl adenovirus serotype 1 strains causing chicken gizzard erosion in Japan. J Appl Poult Res. (2020) 29:383–90. doi: 10.1016/j.japr.2020.01.001
175. Sauerbrei A, Sehr K, Brandstädt A, Heim A, Reimer K, Wutzler P. Sensitivity of human adenoviruses to different groups of chemical biocides. J Hosp Infect. (2004) 57:59–66. doi: 10.1016/j.jhin.2004.01.022
176. Kataria JM, Dhama K, Nagarajan S, Chakraborty S, Kaushal A, Deb R. Fowl adenovirus causing hydropericardium syndrome in poultry. Adv Anim Vet Sci. (2013) 1:5–13.
177. Saifuddin M, Wilks CR. Reproduction of inclusion body hepatitis in conventionally raised chickens inoculated with a New Zealand isolate of avian adenovirus. N Z Vet J. (1990) 38:62–5. doi: 10.1080/00480169.1990.35618
178. Naeem K, Rabbani M, Hussain M, Cheema AH. Development of cell culture vaccine against HPS in poultry. Pak Vet J. (1995) 15:150–1.
179. Ahmad I, Malik MI, Iqbal K, Ahmad K, Naz S. Efficacy of formalized liver organ vaccine against angara disease in broiler. Vet Arh. (1990) 60:131–8.
180. Reddy YK, Ravikumar G, Manoharan S, Koteswaran A. Comparison of the efficacy of different inactivated infectious hydropericardium vaccines. Ind J Anim Sci. (2004) 74:908–10.
181. Chishti MA, Afzal M, Cheema AH. Preliminary studies of the development of vaccine against the “hydropericardium syndrome” of poultry. Rev Sci Tech. (1989) 8:797–801. doi: 10.20506/rst.8.3.432
182. Ahmad K. In vivo pathogenicity of hydropericadium–hepatitis syndrome (Angara disease) and efficacy of vaccines. Pak Vet J. (1999) 19:200–3.
183. Ahmad K, Hasan S, Siddique B. Efficacy of Angara disease vaccine using different levels of formalinized liver organs. Pak J Vet Res. (2003) 1:40–3.
184. Mughal SAK, Ahmed G, Hussain T, Fazlani SA, Ahmed I, Khan FA. In vivo pathogenicity of hydropericardium hepatitis syndrome (Angara disease). Afr J Biotechnol. (2011) 10:13664–71. doi: 10.5897/AJB10.2506
185. Gowthaman V, Singh SD, Dhama K, Barathidasan R, Kumar MA, Desingu PA, et al. Fowl adenovirus (FAdV) in India: evidence for emerging role as primary respiratory pathogen in chickens. Pak J Biol Sci. (2012) 15:900–3. doi: 10.3923/pjbs.2012.900.903
186. Kataria JM, Verma KC, Jadhao SJ, Deepak JN, Sah RL. Efficacy of an inactivated oil emulsified vaccine against inclusion body hepatitis–hydropericardium syndrome in chickens prepared from cell culture propagated fowl adeno virus. Ind J Comp Microbiol Immunol Infect Dis. (1997) 18:38–42.
187. Gupta RK, Chandra R, Kumar R, Rao VDP. Comparative efficacy of cell culture inactivated vaccines against hydropericardium syndrome in broilers. Indian J Anim Sci. (2005) 75:606–9.
188. Dar A, Tipu M, Townsend H, Potter A, Gerdts V, Tikoo S. Administration of poly[di(sodium carboxylatoethylphenoxy)phosphazene] (PCEP) and avian beta defensin as adjuvants in inactivated inclusion body hepatitis virus and its hexon protein based experimental vaccine formulations in chickens. Avian Dis. (2015) 59:518–24. doi: 10.1637/11202-052815-Reg.1
189. Sarfraz M, Suleman M, Tikoo SK, Wheler C, Potter AA, Gerdts V, et al. Immune responses to in ovo vaccine formulations containing inactivated fowl adenovirus 8b with poly[di(sodium carboxylatoethylphenoxy)]phosphazene (PCEP) and avian beta defensin as adjuvants in chickens. Vaccine. (2017) 35:981–6. doi: 10.1016/j.vaccine.2016.12.023
190. Roy P, Kotteeswaran A, Manickam R. Efficiency of inactivated oil emulsion vaccine against hydropericardium syndrome in broilers. Vet Rec. (1999) 145:458–9. doi: 10.1136/vr.145.16.458
191. Toro H, Gonzalez C, Cerda L, Morales MA, Dooner P, Salamero M. Prevention of inclusion body hepatitis/hydropericardium syndrome in progeny chickens by vaccination of breeders with fowl adenovirus and chicken anemia virus. Avian Dis. (2002) 46:547–54. doi: 10.1637/0005-2086(2002)046[0547:POIBHH]2.0.CO;2
192. Kim MS, Lim TH, Lee DH, Youn HN, Yuk SS, Kim BY, et al. An inactivated oil-emulsion fowl adenovirus serotype 4 vaccine provides broad cross protection against various serotypes of fowl adenovirus. Vaccine. (2014) 32:3564–8. doi: 10.1016/j.vaccine.2014.03.015
193. Pan Q, Yang Y, Gao Y, Qi X, Liu C, Zhang Y, et al. An inactivated novel genotype fowl adenovirus 4 protects chickens against the hydropericardium syndrome that recently emerged in China. Viruses. (2017) 9:216.v9080216. doi: 10.3390/v9080216
194. Ruan S, Zhao J, Yin X, He Z, Zhang G. A subunit vaccine based on fiber-2 protein provides full protection against fowl adenovirus serotype 4 and induces quicker and stronger immune responses than an inactivated oil-emulsion vaccine. Infect Genet Evol. (2018) 61:145–50. doi: 10.1016/j.meegid.2018.03.031
195. Wang X, Tang Q, Qiu L, Yang Z. Penton-dodecahedron of fowl adenovirus serotype 4 as a vaccine candidate for the control of related diseases. Vaccine. (2019) 37:839–47. doi: 10.1016/j.vaccine.2018.12.041
196. Tian KY, Guo HF, Li N, Zhang YH, Wang Z, Wang B, et al. Protection of chickens against hepatitis/hydropericardium syndrome and Newcastle disease with a recombinant Newcastle disease virus vaccine expressing the fowl adenovirus serotype 4 fiber-2 protein. Vaccine. (2020) 38:1989–97. doi: 10.1016/j.vaccine.2020.01.006
197. Mansoor MK, Hussain I, Arshad M, Muhammad G. Preparation and evaluation of chicken embryo-adapted fowl adenovirus serotype 4 vaccine in broiler chickens. Trop Anim Health Prod. (2011) 43:331–8. doi: 10.1007/s11250-010-9694-z
198. Zhang Y, Pan Q, Guo R, Liu A, Xu Z, Gao Y, et al. Immunogenicity of novel live vaccine based on an artificial rHN20 strain against emerging fowl adenovirus 4. Viruses. (2021) 13:2153.v13112153. doi: 10.3390/v13112153
199. Shah MS, Ashraf A, Rahman M, Khan MI, Qureshi JA. A subunit vaccine against hydropericardium syndrome using adenovirus penton capsid protein. Vaccine. (2012) 30:7153–6. doi: 10.1016/j.vaccine.2012.10.013
200. Schachner A, Marek A, Jaskulska B, Bilic I, Hess M. Recombinant FAdV-4 fiber-2 protein protects chickens against hepatitis-hydropericardium syndrome (HHS). Vaccine. (2014) 32:1086–92. doi: 10.1016/j.vaccine.2013.12.056
201. Shah MS, Ashraf A, Khan MI, Rahman M, Habib M, Qureshi JA. Molecular cloning, expression and characterization of 100K gene of fowl adenovirus-4 for prevention and control of hydropericardium syndrome. Biologicals. (2016) 44:19–23. doi: 10.1016/j.biologicals.2015.10.002
202. Sheppard M, Werner W, Tsatas E, McCoy R, Prowse S, Johnson M. Fowl adenovirus recombinant expressing VP2 of infectious bursal disease virus induces protective immunity against bursal disease. Arch Virol. (1998) 143:915–30. 0050342 doi: 10.1007/s007050050342
203. De Luca C, Schachner A, Mitra T, Heidl S, Liebhart D, Hess M. Fowl adenovirus (FAdV) fiber-based vaccine against inclusion body hepatitis (IBH) provides type-specific protection guided by humoral immunity and regulation of B and T cell response. Vet Res. (2020) 51:143. doi: 10.1186/s13567-020-00869-8
204. Xie Q, Wang W, Li L, Kan Q, Fu H, Geng T, et al. Domain in Fiber-2 interacted with KPNA3/4 significantly affects the replication and pathogenicity of the highly pathogenic FAdV-4. Virulence. (2021) 12:754–65. doi: 10.1080/21505594.2021.1888458
205. Pan Q, Wang J, Gao Y, Wang Q, Cui H, Liu C, et al. Identification of chicken CAR homology as a cellular receptor for the emerging highly pathogenic fowl adenovirus 4 via unique binding mechanism. Emerg Microbes Infect. (2020) 9:586–96. doi: 10.1080/22221751.2020.1736954
206. De Luca C, Schachner A, Heidl S, Hess M. Vaccination with a fowl adenovirus chimeric fiber protein (crecFib-4/11) simultaneously protects chickens against hepatitis-hydropericardium syndrome (HHS) and inclusion body hepatitis (IBH). Vaccine. (2022) 40:1837–45. doi: 10.1016/j.vaccine.2022.01.060
207. Lu H, Xie Q, Zhang W, Zhang J, Wang W, Lian M, et al. A novel recombinant FAdV-4 virus with fiber of FAdV-8b provides efficient protection against both FAdV-4 and FAdV-8b. Viruses. (2022) 14:376.v14020376. doi: 10.3390/v14020376
208. Dhama K, Mahendran M, Gupta PK, Rai A. DNA vaccines and their applications in veterinary practice: current perspectives. Vet Res Commun. (2008) 32:341–56. doi: 10.1007/s11259-008-9040-3
209. Al Naguib MG, Morsy EA, Samir M, Khattab MS, Khelfa DG, Abdel-Alim GA. Isolation and molecular characterization of fowl adenoviruses associated with inclusion body hepatitis-hydropericardium syndrome in broiler chickens in Egypt. World Vet J. (2021) 11:333–42. doi: 10.54203/scil.2021.wvj46
210. Dhama K, Wani MY, Deb R, Karthik K, Tiwari R, Barathidasan R, et al. Plant based oral vaccines for human and animal pathogens–a new era of prophylaxis: current and future prospective. J Exp Biol Agric Sci. (2013) 1:1–12.
211. El-Shall NA, Sedeik ME, El-Nahas AF, Abdel-salam RA, Awad AM. Epidemiological surveillance of some avian respiratory viral diseases in broiler chickens. Alex J Vet Sci. (2019) 61:185–94. doi: 10.5455/ajvs.9184
212. Mo J. Historical investigation of fowl adenovirus outbreaks in South Korea from 2007 to 2021: a comprehensive review. Viruses. (2021) 13:2256.v13112256. doi: 10.3390/v13112256
213. Oliver-Ferrando S, Dolz R, Calderon C, Valle R, Rivas R, Perez M, et al. Epidemiological and pathological investigation of fowl aviadenovirus serotypes 8b and 11 isolated from chickens with inclusion body hepatitis in Spain (2011–2013). Avian Pathol. (2017) 46:157–65. doi: 10.1080/03079457.2016.1232477
214. Ono M, Okuda Y, Yazawa S, Imai Y, Shibata I, Sato S, et al. Adenoviral gizzard erosion in commercial broiler chickens. Vet Pathol. (2003) 40:294–303. doi: 10.1354/vp.40-3-294
215. Schonewille E, Jaspers R, Paul G, Hess M. Specific-pathogen-free chickens vaccinated with a live FAdV-4 vaccine are fully protected against a severe challenge even in the absence of neutralizing antibodies. Avian Dis. (2010) 54:905–10. doi: 10.1637/8999-072309-Reg.1
216. Xie Q, Cao S, Zhang W, Wang W, Li L, Kan Q, et al. A novel fiber-2-edited live attenuated vaccine candidate against the highly pathogenic serotype 4 fowl adenovirus. Vet Res. (2021) 52:35. doi: 10.1186/s13567-021-00907-z
217. Mahima A, Rahal A, Deb R, Latheef SK, Samad HA, Tiwari R, et al. Immunomodulatory and therapeutic potentials of herbal, traditional/indigenous and ethnoveterinary medicines. Pak J Biol Sci. (2012) 15:754–74. doi: 10.3923/pjbs.2012.754.774
218. Dhama K, Chakraborty S, Mahima A, Wani MY, Verma AK, Deb R, et al. Novel and emerging therapies safeguarding health of humans and their companion animals: a review. Pak J Biol Sci. (2013) 16:101–11. doi: 10.3923/pjbs.2013.101.111
219. Tiwari R, Chakraborty S, Dhama K, Wani MY, Kumar A, Kapoor S. Wonder world of phages: potential biocontrol agents safeguarding biosphere and health of animals and humans–current scenario and perspectives. Pak J Biol Sci. (2014) 17:316–28. doi: 10.3923/pjbs.2014.316.328
Keywords: aviadenovirus, diagnosis, disease transmission, epidemics, fowl adenoviruses, poultry diseases, vaccines
Citation: El-Shall NA, El-Hamid HSA, Elkady MF, Ellakany HF, Elbestawy AR, Gado AR, Geneedy AM, Hasan ME, Jaremko M, Selim S, El-Tarabily KA and El-Hack MEA (2022) Epidemiology, pathology, prevention, and control strategies of inclusion body hepatitis and hepatitis-hydropericardium syndrome in poultry: A comprehensive review. Front. Vet. Sci. 9:963199. doi: 10.3389/fvets.2022.963199
Received: 07 June 2022; Accepted: 15 August 2022;
Published: 11 October 2022.
Edited by:
Lester J. Perez, Abbott, United StatesReviewed by:
Tuofan Li, Yangzhou University, ChinaJowita Samanta Niczyporuk, National Veterinary Research Institute (NVRI), Poland
Copyright © 2022 El-Shall, El-Hamid, Elkady, Ellakany, Elbestawy, Gado, Geneedy, Hasan, Jaremko, Selim, El-Tarabily and El-Hack. This is an open-access article distributed under the terms of the Creative Commons Attribution License (CC BY). The use, distribution or reproduction in other forums is permitted, provided the original author(s) and the copyright owner(s) are credited and that the original publication in this journal is cited, in accordance with accepted academic practice. No use, distribution or reproduction is permitted which does not comply with these terms.
*Correspondence: Nahed A. El-Shall, nahed.abdelgawad@alexu.edu.eg; Khaled A. El-Tarabily, ktarabily@uaeu.ac.ae