- 1College of Chemistry and Life Sciences, Chengdu Normal University, Chengdu, China
- 2Sichuan Provincial Key Laboratory for Development and Utilization of Characteristic Horticultural Biological Resources, Chengdu Normal University, Chengdu, China
Natural edible fungal polysaccharides are of research and application value for the prevention of diseases by improving the microenvironment within the intestine. Inonotus obliquus polysaccharide (IOP) extracts have strong antioxidant, anti-inflammatory, and other biological activities, and as such, it could be used as prebiotics to improve the viability of intestinal microbes, maintain intestinal homeostasis and improve intestinal immunity. The effects of sex on intestinal microbiota after IOP absorption was determined. In this study, IOP had different effects on the intestinal flora of male and female rats, with the diversity and richness showing opposite changes. At the same time, after IOP intervention, changes in the dominant intestinal flora of female rats was less compared with that of males. In addition, while Clostridia, Lactobacillus and Roseburia were the dominant intestinal microbes in female rats, males had mainly Bacteroidota from different families and genera, along with an increasing proportion of Muribaculaceae from different families and genera. IOP could further regulate the intestinal microenvironment of male and female SD rats by enhancing the vitality of their dominant microorganisms, and for both sexes, this enabled the screening of dominant microflora that were conducive to the balance of the intestinal flora. These results help to understand the effects of sex-related differences on the composition of the intestinal microbiota as well as on diseases.
Introduction
Gut microbes are considered to be a dynamic organ that plays a vital role in the digestion of food components as well as in host immune responses (1). Clear individual differences in the composition of human gut microbes have been identified by genome-based assessments (2), and simultaneously, conclusive evidence have shown that diversification of the gut microbiome can lead to diseases such as asthma (3) and cancer (4). Nevertheless, correlational research of intestinal microbes between individuals still has knowledge gaps. It is particularly important to understand how the composition of the gut microbiota vary between individuals as this would have significant implications for the prevention of sex-related diseases.
Sex hormones are associated with the composition of human gut microbes (5). Sex-related differences in intestinal microbiome composition in animals have been reported and the studies indicated that sex-related differences in gut microbes do not appear until after puberty (6, 7). The gut microbiota is sex-dependent and may be directly affected by sex hormones. In addition, these hormones interact with environmental factors such as dietary habits and antibiotic treatment to influence the microbiome (8). The body's dynamic changes in response to estrogen or other hormones, is also potentially linked to disease. In this context, some studies have shown that the microbiome is related to sex dimorphism and sex-specific rhythm of the liver, and this could be caused by the secretion of growth hormone (GH) and the regulation of sexual maturity (9). The findings of these studies provide a basis that explains sex-related differences in susceptibility to certain diseases. The occurrence and development of many diseases are linked to immunity and metabolism. Given that the intestinal flora plays an important role in maintaining an internal environmental balance that regulates immunity and metabolism and defends against pathogens (10, 11), it is likely that prebiotics may help to understand the impact of the gut microbiome on disease (12).
Inonotus obliquus is a natural edible and medicinal fungus which grows mainly in the colder regions of Asia and Europe. Its metabolites have been shown to possess a wide range of biological properties such as anti-inflammatory, antioxidant and antiviral (13–15), and as such, they have the potential to be used for preventing cancer, diabetes, cerebrovascular or other diseases (13, 16, 17). However, of these metabolites, most researchers have been paying greater attention to the biological effects of Inonotus obliquus polysaccharides on the body where they form a biological barrier that protects hosts from pathogens (18, 19). The composition of polysaccharides as well as their effects on gut health vary greatly, and consequently, differences in their effects on gut microbiome between individuals need to be considered.
To determine how the effects of Inonotus obliquus polysaccharide consumption vary between sexes, the composition of intestinal microbiota in SD rats was assessed, after they had been fed a normal diet or a rich Inonotus obliquus polysaccharide one. The relationship between sex and the composition of intestinal microbiota as well as the distribution of specific taxa was studied after consuming the polysaccharides. It is expected that the results of this study will help to better understand the effects of polysaccharides on gut microbiota in order to reduce risk of diseases and mitigate specific health risks.
Materials and methods
Animals and reagents
All laboratory procedures, including those related to animal handling, welfare and euthanasia, were carried out in accordance with the guidelines and regulations of Animal Research Reporting of In Vivo Experiments (ARRIVE) and approved by the Animal Care Office of Chengdu Normal University, Chengdu, China (No: CDNU-2021092614M). SD rats, free from pathogens, were purchased from the Chengdu Dossy Experimental Animals Co., Ltd while Inonotus obliquus was obtained from Suifenhe Market in the Heilongjiang Province.
The extraction of Inonotus obliquus
Traditional extraction methods based on hot water extraction, followed by centrifugation, were applied (20, 21). For this purpose, Inonotus obliquus (IO) was first crushed before being degreased with petroleum ether. The polysaccharide residue was then dried at low temperature, heated and boiled with distilled water and subsequently filtered. After repeating the above process three times, all resulting filtrates were mixed, the solvent was recovered under pressure, and 1% of trichloroacetic acid was added to precipitate proteins. The mixture was then centrifuged and concentrated into a liquid extract before overnight precipitation at 0°C with anhydrous ethanol. The alcohol-containing solution was centrifuged at high speed to obtain a crude extract which was subsequently washed 2–3 times with a small amount of anhydrous ethanol to obtain crude polysaccharide. DEAE- cellulose anionic column chromatography was used to specifically isolate acidic and neutral mucopolysaccharides. After removing the pigment, 2% sodium nitrite solution was added in a ratio of 1:2 before heating the mixture in a water bath at 80°C. This was followed by the addition of activated carbon and after stirring, the solution was heated again for 1 h before being left overnight. It was eventually centrifuged and freeze-dried to obtain the decolorized polysaccharides.
Monosaccharide and uronic acid compositional analysis
The monosaccharide and uronic acid composition of IOP was analyzed by HPLC (Agilent 1200, USA). For this purpose, 3.41 mg of IOP was carefully taken into an ampoule before adding 0.5 ml of 4 M trifluoroacetic acid. Nitrogen was then pumped into the ampoule for 5 min before sealing it at 121°C for 2 h. The trifluoroacetic acid was blown dry with nitrogen, and 0.5 ml of 0.5 M PMP as well as 0.5 ml of 0.3 M NaOH were added. The mixture was then kept in a water bath at 70°C for 1 h, and after allowing it to cool, 0.5 ml of 0.3 M HCl was added. This was followed by chloroform extraction three times, with the supernatant subsequently centrifuged after the third extraction. The final sample was passed through a 0.22 μm membrane before eventually injecting 20 μl into the injection bottle for analysis at 245 nm. Standard monosaccharides and uronic acids were also analyzed, and their standard curves are shown in Table 1.
Experimental treatment
Forty-eight SD rats (female: 150 ± 10 g, male: 180 ± 10 g) were placed in an environment with a 12-h light/dark cycle, a temperature of 25 ± 3°C and a relative humidity of 75 ± 5%. The animals were also provided adequate food and water. Rats of each sex were then randomly divided into a control group and an IOP group after 7 days of adaptation. The IOP group was given IOP (60 mg/kg) by timing intragastric administration, while the control group was simultaneously given an equal proportion of Stroke-Physiological Saline Solution. The rats were eventually stunned with ether before being killed by neck dislocation after 4 weeks of treatment. The colorectal content was then collected under sterile conditions and stored at −80°C after rapid-freezing with liquid nitrogen (Figure 1).
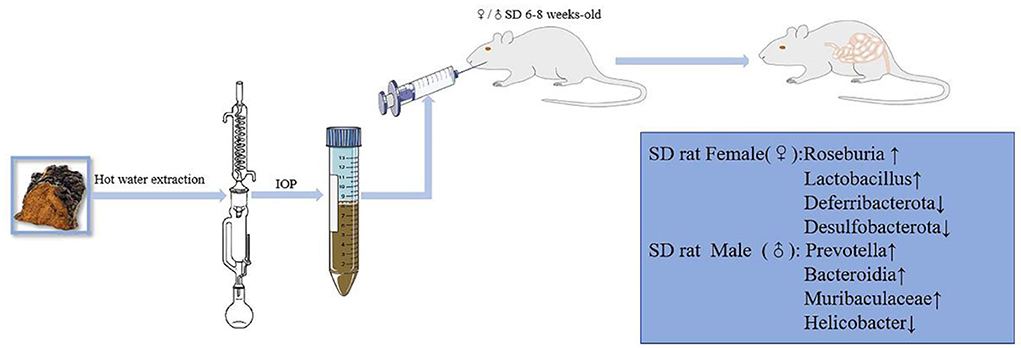
Figure 1. Animal experiment process. IOP increased the diversity and richness of intestinal microbes in male and female SD rats, while also affecting the abundance of specific microbes differently in each sex.
DNA extraction and amplification
Bacterial DNA was first isolated from colorectal content by using the MagPure Soil DNA LQ kit (Guangdong Magen, China) as per the manufacturer's instructions. DNA concentration and integrity were then determined with a NanoDrop 2000 spectrophotometer (Thermo Fisher Scientific, Waltham, MA, USA) and agarose gel electrophoresis. Using TksGflflex DNA polymerase (Takara, R060B) as well as the universal primer pairs 343f (5′-TACGGRAGGCAGCAG-3′) and 798r (5′-AGGGTATCTAATCT-3′), PCR amplification of the V3–V4 hypervariable region of bacterial 16S rRNA gene was subsequently performed in 25 μl reaction mixtures. The reverse primers contained sample barcodes and both were also connected to Illumina sequencing adapters.
Library construction and sequencing
PCR products were purified with Agencourt AMPure XP beads (Beckman Coulter Co., USA) and quantified with Qubit dsDNA detection kits. Sequencing was then performed on an Illumina NovaSeq6000 platform to generate 250 bp paired-end reads (Illumina Inc., San Diego, California; Shanghai OE Biotech Co., Shanghai, China).
Bioinformatics analysis
Paired-end reads were preprocessed using Trimmomatic software (22) to detect and cut off ambiguous bases (N). The sliding window pruning method was used to cut off low-quality sequences with an average mass score below 20. After trimming, FLASH software was used to assemble paired-end reads (23) according to the following parameters: a minimum overlap of 10 bp, a maximum overlap of 200 bp and a maximum mismatch rate of 20%. Further denoising of the sequences involved discarding readings with ambiguous or homologous sequences as well as those below 200 bp. QIIME software (version 1.8.0) (24) was then used to retain 75% of bases above Q20, while VSEARCH was applied to detect and remove both chimeras and primer sequences. Clean reads were clustered at a 97% similarity cutoff value to generate operational classification units (OTUs) (25), with the QIIME package subsequently used to select representative reads for each OTU. All representative reads were annotated against the Silva database (version 132) using the RDP classifier (confidence threshold 70%) (26). Alpha-diversity indices, including the Chao1 index (27) and the Shannon index (28) were then used to estimate microbial diversity in samples of colon content before comparing the results based on Wilcoxon rank sum test. The Unifrac distance matrix, as estimated by QIIME software, was eventually used for unweighted Unifrac principal coordinate analysis (PCoA) as well as for constructing phylogenetic trees.
Results
Monosaccharide and uronic acid composition of IOP
In order to further understand the structure-activity relationship of polysaccharides from Inonotus obliquus, the compound was purified. During the purification process, protein, pigments and acidic sugars were removed to obtain neutral sugars. Finally, the chromatogram of the polysaccharides was obtained by HPLC (Figure 2). It was observed that the purified polysaccharides were composed of Man, Glu A, Glu, Gal, Xyl, Ara and Fuc.
The effects of IOP on the overall abundance of intestinal microbes
Between 71,485 and 75,214 clean tags were obtained after quality control and the removal of chimeras from the original sequences. After Operational taxonomic units (OTU) classification, the number of OTUs in 48 samples ranged from 1,673 to 2,342. Results for the Shannon index and Chao1 index indicated that the diversity and richness of the IOP group were significantly changed compared with the control group (Figure 3). Beta-diversity, combined with adonis analysis (unweighted R = 0.45, P = 0.001; weighted R = 0.75, P = 0.001), further showed that the differences between groups were greater than for within groups, with significant differences being noted between the IOP group and the control group. Based on the unweighted (qualitative) and weighted (quantitative) UniFrac distance measures in PCoA analysis, significant differences in weighted and unweighted UniFrac distances between male and female rats of the control group were also found (Figure 4).
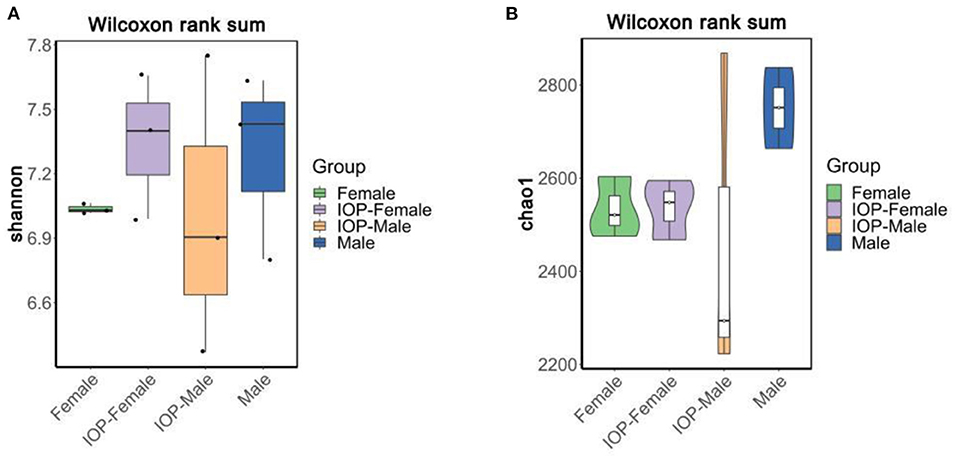
Figure 3. Alpha-diversity compared based on Wilcoxon rank sum test. (A) Shannon boxplots of all SD rats. (B) Chao1 violin plots of all SD rats.
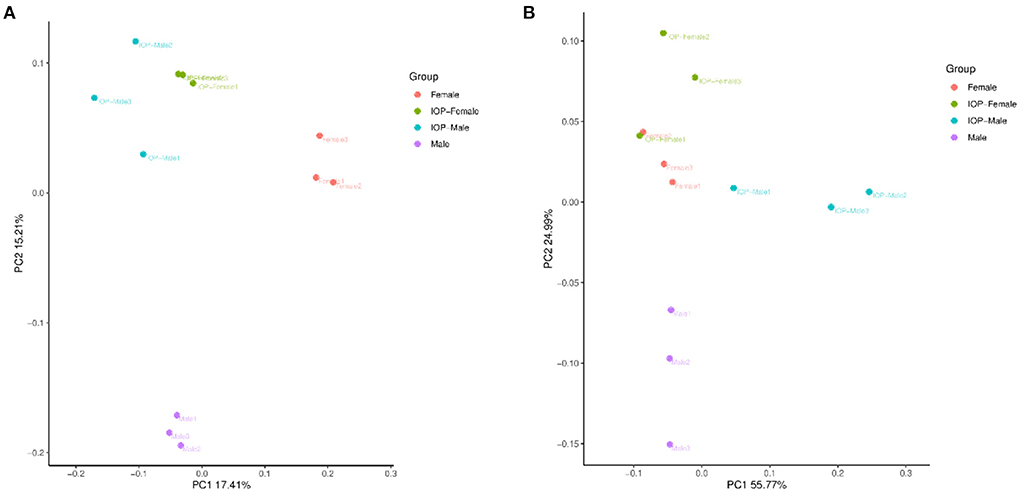
Figure 4. Beta diversity of samples based on Adonis test. (A) PCoA plots based on unweighted unifrac distances for all SD rats. (B) PCoA plots based on weighted unifrac distances for all SD rats.
The community structure of colorectal intestinal microbes of rats in the IOP group, as presented in the bar chart (Figure 5), showed a total of seven phyla with high abundance at phylum level. Firmicutes and Bacteroidota were the most dominant, accounting for 45.57 and 47.13% of the abundance respectively. There were, however, no significant differences in the F/B ratio for females, although that of males decreased. Desulfobacterota (3.28%), Campilobacterota (1.1%), Spirochaetota (1.6%), Proteobacteria (1.1%) and Actinobacteriota (0.2%) were some of the additional phyla that were also detected. At the genus level, the dominant genera mostly included Muribaculaceae (22.3%), Prevotella (12.2%), Lachnospiraceae_NK4A136_group (8.7%) and Alloprevotella (5.5%). In addition, the abundance of different species in each group was compared by Kruskal Wallis and it was found that, at the genus level, Prevotellaceae_NK3B31_group, Lactobacillus and Roseburia had significantly different abundances between groups.
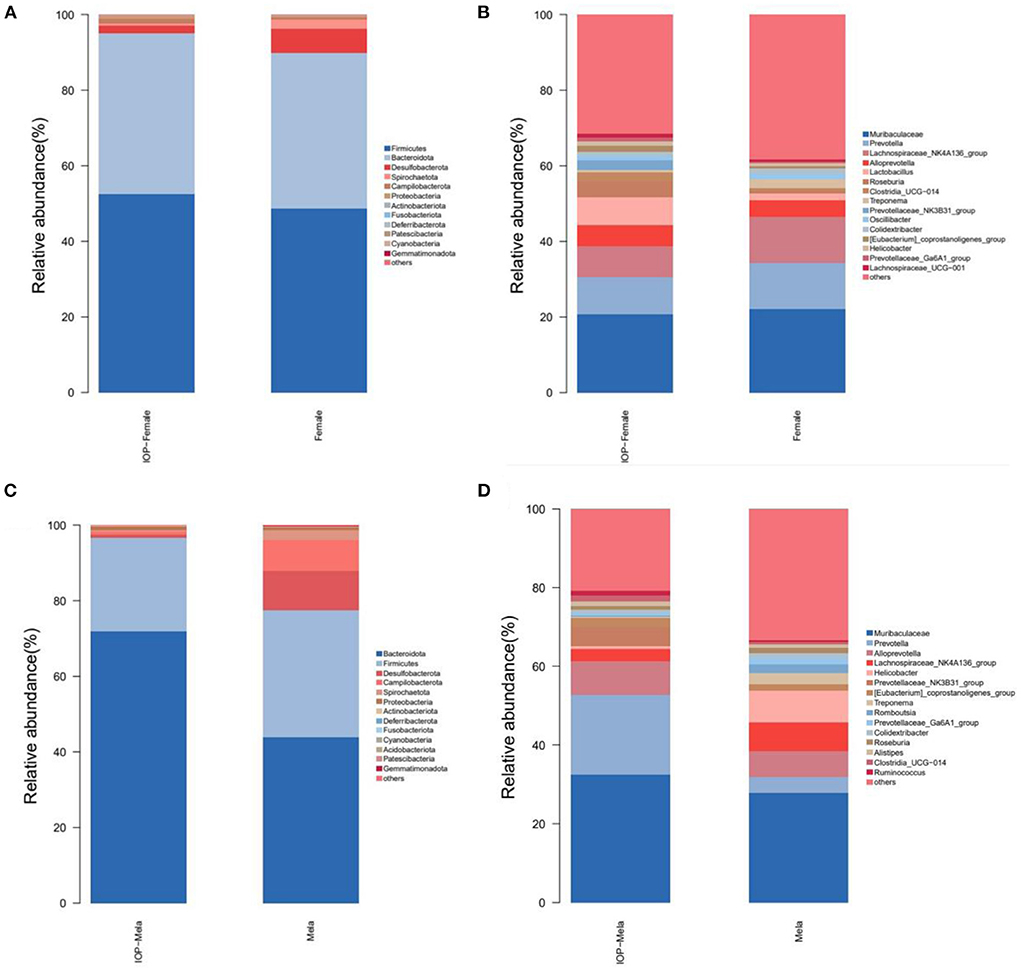
Figure 5. Histogram showing changes in the composition of intestinal microbes. (A,B) The first 15 different bacteria in female SD rats at phylum and genus levels. (C,D) The first 15 different microorganisms in male SD rats at phylum and genus levels.
A bar chart of the community structure of colorectal intestinal flora for SD rats within the control group is shown in Figure 5. At the phylum level, there were five phyla of relatively high abundance, and these included Bacteroidota (40.38%), Firmicutes (45.26%), Desulfobacterota (8.1%), Campilobacterota (2.9%) and Spirochaetota (2.0%). In particular, the abundance of Firmicutes in female rats was higher than for males, while that of Campilobacterota was lower compared with males. At the genus level, the five most abundant genera consisted of Muribaculaceae (22.3%), Lachnospiraceae_NK4A136_group (10.9%), Prevotella (8.25%), Alloprevotella (6.0%%) and Helicobacter (2.8%%). In this case, the beneficial bacteria Prevotella was higher in abundance in female rats than in male ones, while the harmful bacteria Helicobacter was more abundant in male rats. However, after IOP intervention, the diversity of female rats' microbiota increased, such as Roseburia and other beneficial bacteria. The F/B ratio and the proportion of Helicobacter and other harmful bacteria decreased in male rats.
Differential analysis of intestinal microflora between male and female SD rats affected by IOP
To evaluate whether differences in intestinal microbes after IOP treatment were influenced by the sex of the rats, Welch's two sample t-tests were used to explore differences between male and female SD rats at phylum level (Figure 6). The results, in this case, showed that, for the control group (without IOP treatment), Firmicutes (P = 0.3), Lactobacillus (P = 0.03) and Roseburia (P = 0.05) were higher in females than in males, while Bacteroidota (P = 1), Campilobacterota (P = 0.18) Prevotella (P = 0.04) and [Eubacterium]siraeum_group were lower when compared with males. Similarly, for the experimental group (treated with IOP), among the highly abundant species, Firmicutes and Lactobacillus were significantly higher in females than in males, while Bacteroidota (P = 0.36) was significantly lower compared with males. In contrast, among species of low abundance, Desulfobacterota (P = 1), Deferribacterota (P = 1) and Roseburia (P = 0.669) were significantly higher in females, while Prevotella (P = 1).
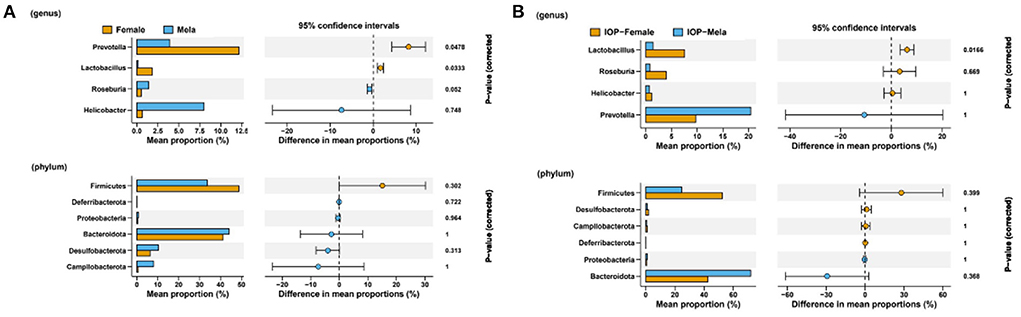
Figure 6. Comparison of intestinal microflora between male and female SD rats. Welch's two sample t-tests were performed to compare the control group (A) and the experimental group (B) with IOP intervention.
Analysis of differences in dominant species between sexes
Linear discriminant analysis Effect Size (LEFSE) was performed to evaluate the impact of sex-related differences on intestinal dominant microbes (Figure 7). It was found that, for the control, Prevotella and Firmicutes were the dominant intestinal microbes in female rats, while the dominant ones in males were Helicobacter, Muribaculaceae, Campilobacterota and Desulfobacterota. In contrast, several genera of Firmicutes were dominant in female rats after IOP intervention, and, in particular, the proportion of Clostridia, Lactobacillus and Roseburia increased. As far as male rats were concerned, several Bacteroidota families and genera were dominant, with the proportion of Muribaculaceae families and genera particularly increasing. At the same time, the proportion of some pathogenic bacteria also decreased. Therefore, it was found that the changes in dominant bacteria occurring in females were smaller than those of males after IOP intervention. On the other hand, female intestinal microbes showed better diversity than that of males.
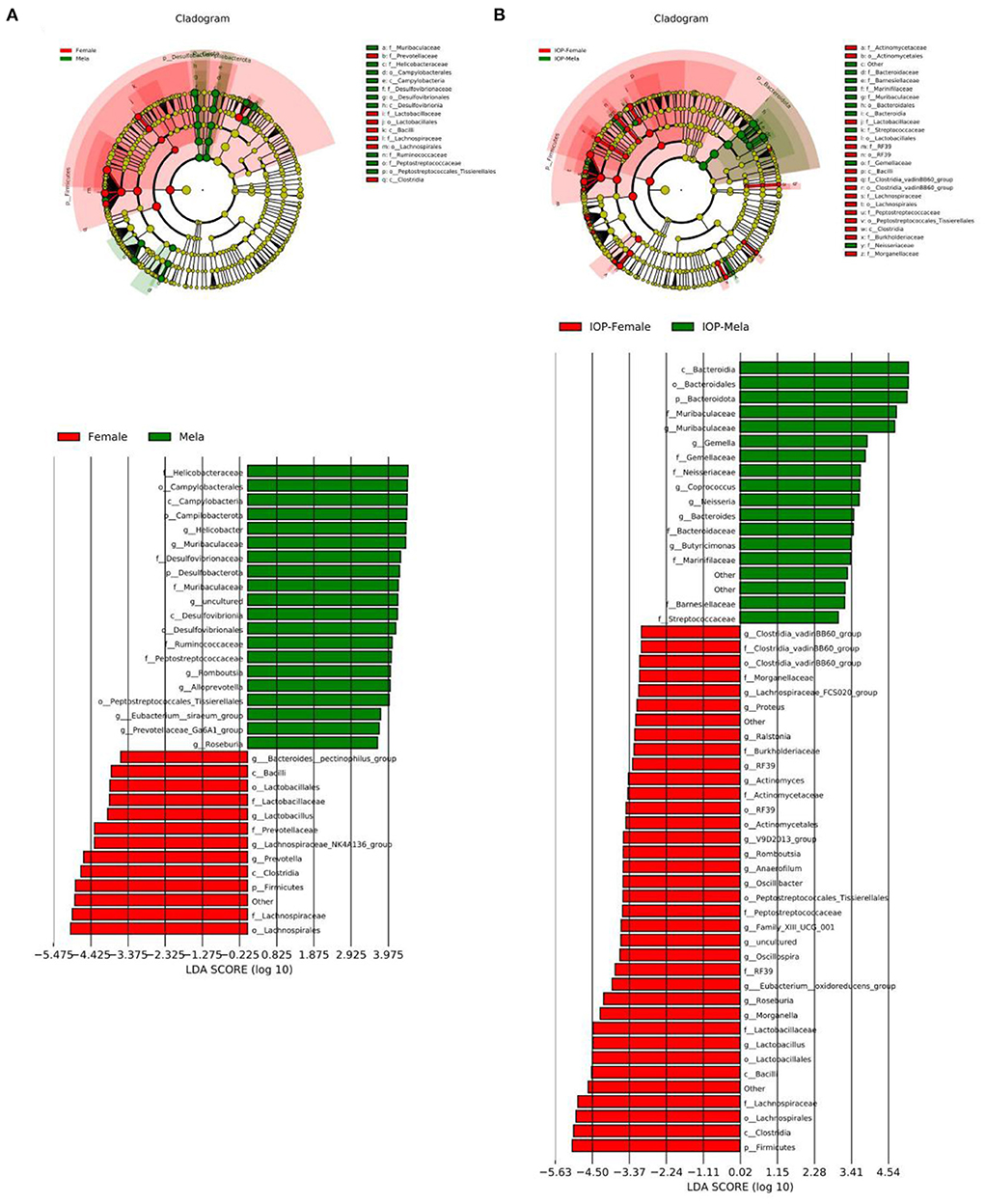
Figure 7. The control group (A) and the experimental group (B) with IOP interference were compared. Results of linear discriminant analysis were used to determine the dominant species after IOP interference.
Discussion
The diversity of gut microbes is associated with humans or animals health (29–31), and disruption of this relationship can have adverse effects (20, 32–34). For example, an imbalance in the normal gut flora may contribute to the development of type 2 diabetes mellitus, non-alcoholic fatty liver disease and other related metabolic diseases (35, 36). Similarly, sex hormones may induce changes in gut microbiota during ontogeny (37). In this case, too much androgen can cause dysregulation of the intestinal flora in females with polyovarian syndrome (38), while estrogen can mediate irritable bowel syndrome (IBS) by altering the permeability of the intestinal barrier (37). Interestingly, the polysaccharides from Inonotus obliquus exert certain biological activities, thereby allowing it to be used as a prebiotic to fight diseases such as diabetes, cancer and cardiovascular diseases (16). Previous research has already found that IOP could help alleviate acute endometritis (20) and improve the severity of both obesity (39) and chronic pancreatitis (40) by converting the diversity of intestinal flora to a healthier state and this, in turn, improves the antioxidant status, thereby reducing inflammation levels, and improving host metabolism (41).
The richness and diversity of intestinal microflora in male and female rats fed with IOP were analyzed by 16S rRNA sequencing. The results showed that there were differences in the regulation of intestinal microflora between male and female SD rats after Inonotus obliquus consumption, with these observations being consistent with a previous study which reported opposite changes in the correlation of α-diversity in the intestinal microflora of male and female SD rats (42). Similarly, as far as humans are concerned, sex-related differences such as total estradiol levels were shown to be negatively correlated with α diversity in healthy men, non-hyperandrogenic women as well as women with polycystic ovary syndrome (PCOS) (43).
Moreover, based on the weighted and unweighted UniFrac PCoA index, significant differences in both quantitative and qualitative indexes (weighted and unweighted UniFrac distance) were noted between male and female SD rats in the control group, while for the experimental group, differences between sexes were only visible for the qualitative index (unweighted UniFrac distance) (44).These findings could have been due to differences in the types of hormones secreted by female and male individuals, resulting in variations in the diversity of intestinal flora between mice of different sexes. Estrogen is an inhibitor of gastric acid production while testosterone is an inducer, estrogen may be more effective at repairing damage to the stomach wall as it reduces gastric blood flow in males (45). Estrogen has also been shown to influence the composition of intestinal microbes, with the reverse (i.e., the intestinal flora affecting the levels of estrogen) also being true (46).
Nevertheless, differences in the composition of intestinal microbes between male and female rats before and after being fed with IOP were compared and analyzed, with the results showing significant differences between male and female rats at phylum and genus level. The intestinal microbe for the IOP group was also significantly different compared with the control and at the same time, significant differences in the type of bacteria between sexes were also observed. Roseburia was significantly more abundant in females than in males. This genus is able to utilize fructan and increase glucagon-like peptide-1, thereby reducing the risk of obesity (43), with this function being important as obesity has been reported to promote high androgen production, which could lead to the development of polycystic ovarian syndrome (47). Based on the results, it was further speculated that Roseburia could reduce the secretion of sex hormones. In male rats that were given IOP, Muribaculaceae (48), which can regulate testosterone induced benign prostatic hyperplasia (43, 49). However, Prevotella, which was positively correlated with testosterone, was higher in abundance than in females while the F/B ratio was lower compared with that of females. Previous studies have also shown that the F/B ratio is related to intestinal environment stability and body health status (50). In this context, the results of this study showed that IOP could reduce the ratio of F/B in the intestinal flora of male and female rats, and this can promote the stability of the intestinal environment and lower the Body Mass Index. Therefore, altogether, the findings suggest that changes in intestinal microbes could be dependent on sex and sex hormones, as reported by previous research results (51).
The relative abundance of harmful bacteria like Desulfobacterota and Campilobacterota decreased after using IOP. The level of Desulfobacterota was reported to be positively correlated with body weight and lipid level (52). The current results showed that IOP reduced the relative abundance of Desulfobacterota in both male and female rats and therefore, it was hypothesized that IOP could intervene with the body health by regulating intestinal microbes. It has been reported that the abundance of Campilobacterota was significantly increased in the NLRP6 inflammatory body regulating chronic alcoholic liver disease in mouse models (53). In the results of this study, IOP reduced the abundance of harmful bacteria such as Campilobacterota in male rats, and hence, it was shown that IOP could reduce inflammation by improving intestinal flora. Additionally, changes in intestinal microbe between male and female rats may also affect the digestion of polysaccharide compounds (54), vitamin synthesis (55), and the expulsion of pathogenic microorganisms (56) etc. Hence, these results may further explain differences in the incidence of intestinal metabolic syndrome (44), and obesity (57) between sexes as they are all associated with changes in gut flora.
Differences in dominant bacterial species in the intestinal flora of male and female rats were also analyzed after IOP consumption. It was found that Lactobacillus, Roseburia and Clostridia were dominant in females of the IOP group as previously reported (19, 57). For instance, in the case of females from the experimental group, Lactobacillus mainly consumed polysaccharides, and lactate, the end product of its fermentation, could be used as an energy source by Roseburia to produce butyrate. Additionally, bacterial fermentation of polysaccharides leads to the production of acidic products such as lactic acid and short chain fatty acid (SCFAs) which reduce the pH of the colorectum to influence the microbial community composition of the gastrointestinal tract. In this context, it has been reported that higher acidic conditions are conducive to the growth of Firmicutes such as Roseburia, which produce butyric acid, thereby reducing the proliferation of acid-sensitive Bacteroidia species (58). The presence of Lactobacillus can not only reduce intestinal permeability and increase the release of anti-inflammatory factors in steroid deficient mice (59), but also prevent the adhesion of Neisseria gonorrhoeae, thus protecting the host from infections (60). In addition, Clostridia not only participates in bile acid (BA) biotransformation (61), but also converts glucocorticoids to androgens (62). In studies of the potential role of gender-dependent autoimmunity in gut microbiota, it was suggested that hormones could interact with gut microbiota to mediate sexual dimorphism (63). In male rats, polysaccharide-degrading bacteria were mainly dominated by Bacteroidia, such as Muribaculaceae (64), which could increase the expression of AhRR in intestinal immune cells as well as Bacteroides which could synthesize conjugated linoleic acid (CLA). The latter can regulate immune functions, lower blood lipids and exert anti-diabetic as well as anti-inflammatory effects (65, 66).Through comparative analysis examining how dominant bacteria varied between sexes after consuming IOP, it was found that IOP could improve the activity of dominant bacteria in intestinal microbes, and these results could form the basis of a future approach whereby bacterial species are selected based on sex-related differences to reshape the intestinal microenvironment, while providing ideas for metabolic analysis.
Conclusion
The results of this study suggest that there are indeed sex-related differences in the diversity of intestinal microbes after IOP interference and that these differences could be associated with the incidence of metabolic diseases. IOP could not only produce different effects on the intestinal microbiota based on the sex of the animals, but also improve the activity of dominant microbes. The current findings could also provide a basis for designing new interventions aimed at preventing metabolic diseases by regulating differences in the intestinal microbes of different sexes through IOP, improving the microenvironment of intestinal microbes and enhancing the vitality of dominant species.
Data availability statement
The datasets presented in this study can be found in online repositories. The names of the repository/repositories and accession number(s) can be found below: Sequence Read Archive of the National Center for Biotechnology Information repository; PRJNA862723.
Ethics statement
The animal study was reviewed and approved by Animal Care Office of Chengdu Normal University.
Author contributions
Conceptualization: BH, XH, and JT. Methodology: BH, WZ, and XD. Software: XL. Validation: BH and GW. Formal analysis: XH, JT, and ML. Investigation: YG, MS, RS, and XD. Resources, supervision, and funding acquisition: BH and SL. Data curation: BH, XH, JT, and SL. Writing—original draft preparation and visualization: BH and YM. Writing—review and editing: YM, BH, and SL. Project administration: GW. All authors have read and agreed to the published version of the manuscript.
Funding
This work was supported by the Chengdu Normal University scientific research project [No.111-153701] (Acceptor: BH), Public welfare project of pet chaperoning [No.107-261277] (Acceptor: BH), and Chengdu Normal University agricultural ecology and green food development project [CSCXTD2020B11] (Acceptor: SL).
Acknowledgments
We thank the investigators and staff of the Shanghai LUMING Biotechnology Co., Ltd for technical support.
Conflict of interest
The authors declare that the research was conducted in the absence of any commercial or financial relationships that could be construed as a potential conflict of interest.
Publisher's note
All claims expressed in this article are solely those of the authors and do not necessarily represent those of their affiliated organizations, or those of the publisher, the editors and the reviewers. Any product that may be evaluated in this article, or claim that may be made by its manufacturer, is not guaranteed or endorsed by the publisher.
References
1. Zhang T, Yang Y, Liang Y, Jiao X, Zhao C. Beneficial effect of intestinal fermentation of natural polysaccharides. Nutrients. (2018) 10:1055. doi: 10.3390/nu10081055
2. Costello EK, Lauber CL, Hamady M, Fierer N, Gordon JI, Knight R, et al. Bacterial community variation in human body habitats across space and time. Science. (2009) 326:1694–7. doi: 10.1126/science.1177486
3. Tashiro H, Kasahara DI, Osgood RS, Brown T, Cardoso A, Cho Y, et al. Sex differences in the impact of dietary fiber on pulmonary responses to ozone. Am J Respir Cell Mol Biol. (2020) 62:503–12. doi: 10.1165/rcmb.2019-0124OC
4. Sobhani I, Tap J, Roudot-Thoraval F, Roperch JP, Letulle S, Langella P, et al. Microbial dysbiosis in colorectal cancer (CRC) patients. PLoS ONE. (2011) 6:e16393. doi: 10.1371/journal.pone.0016393
5. Flores R, Shi J, Fuhrman B, Xu X, Veenstra TD, Gail MH, et al. Fecal microbial determinants of fecal and systemic estrogens and estrogen metabolites: a cross-sectional study. J Transl Med. (2012) 10:253. doi: 10.1186/1479-5876-10-253
6. Markle JG, Frank DN, Mortin-Toth S, Robertson CE, Feazel LM, Rolle-Kampczyk U, et al. Sex differences in the gut microbiome drive hormone-dependent regulation of autoimmunity. Science. (2013) 339:1084–8. doi: 10.1126/science.1233521
7. Yurkovetskiy L, Burrows M, Khan AA, Graham L, Volchkov P, Becker L, et al. Gender bias in autoimmunity is influenced by microbiota. Immunity. (2013) 39:400–12. doi: 10.1016/j.immuni.2013.08.013
8. Valeri F, Endres K. How biological sex of the host shapes its gut microbiota. Front Neuroendocrinol. (2021) 61:100912. doi: 10.1016/j.yfrne.2021.100912
9. Weger BD, Gobet C, Yeung J, Martin E, Jimenez S, Betrisey B, et al. The mouse microbiome is required for sex-specific diurnal rhythms of gene expression and metabolism. Cell Metab. (2019) 29:362–82.e8. doi: 10.1016/j.cmet.2018.09.023
10. Gensollen T, Iyer SS, Kasper DL, Blumberg RS. How colonization by microbiota in early life shapes the immune system. Science. (2016) 352:539–44. doi: 10.1126/science.aad9378
11. Bäumler AJ, Sperandio V. Interactions between the microbiota and pathogenic bacteria in the gut. Nature. (2016) 535:85–93. doi: 10.1038/nature18849
12. Ericsson AC, Franklin CL. Manipulating the gut microbiota: methods and challenges. ILAR J. (2015) 56:205–17. doi: 10.1093/ilar/ilv021
13. Szychowski KA, Skóra B, Pomianek T, Gmiński J. Inonotus obliquus - from folk medicine to clinical use. J Tradit Complement Med. (2021) 11:293–302. doi: 10.1016/j.jtcme.2020.08.003
14. Tuohy KM, Conterno L, Gasperotti M, Viola R. Up-regulating the human intestinal microbiome using whole plant foods, polyphenols, and/or fiber. J Agric Food Chem. (2012) 60:8776–82. doi: 10.1021/jf2053959
15. Enzi G, Gasparo M, Biondetti PR, Fiore D, Semisa M, Zurlo F, et al. Subcutaneous and visceral fat distribution according to sex, age, and overweight, evaluated by computed tomography. Am J Clin Nutr. (1986) 44:739–46. doi: 10.1093/ajcn/44.6.739
16. Lu Y, Jia Y, Xue Z, Li N, Liu J, Chen H, et al. Recent developments in Inonotus obliquus (Chaga mushroom) polysaccharides: isolation, structural characteristics. Biological activities and application. Polymers. (2021) 13:1441. doi: 10.3390/polym13091441
17. Wang Y, Ouyang F, Teng C, Qu J. Optimization for the extraction of polyphenols from Inonotus obliquus and its antioxidation activity. Prep Biochem Biotechnol. (2021) 51:852–9. doi: 10.1080/10826068.2020.1864642
18. Sibai M, Altuntaş E, Yildirim B, Öztürk G, Yildirim S, Demircan T. Microbiome and longevity: high abundance of longevity-linked muribaculaceae in the gut of the long-living rodent Spalax leucodon. Omics. (2020) 24:592–601. doi: 10.1089/omi.2020.0116
19. Nie K, Ma K, Luo W, Shen Z, Yang Z, Xiao M, et al. Roseburia intestinalis: a beneficial gut organism from the discoveries in genus and species. Front Cell Infect Microbiol. (2021) 11:757718. doi: 10.3389/fcimb.2021.757718
20. Hu B, Dong Y, Zhou W, Ma Y, Li L, Fu X, et al. Effect of Inonotus obliquus polysaccharide on composition of the intestinal flora in mice with acute endometritis. PLoS ONE. (2021) 16:e0259570. doi: 10.1371/journal.pone.0259570
21. Tang Y, Xiao Y, Tang Z, Jin W, Wang Y, Chen H, et al. Extraction of polysaccharides from Amaranthus hybridus L. by hot water and analysis of their antioxidant activity. PeerJ. (2019) 7:e7149. doi: 10.7717/peerj.7149
22. Bolger AM, Lohse M, Usadel B. Trimmomatic: a flexible trimmer for Illumina sequence data. Bioinformatics. (2014) 30:2114–20. doi: 10.1093/bioinformatics/btu170
23. Reyon D, Tsai SQ, Khayter C, Foden JA, Sander JD, Joung JK, et al. FLASH assembly of TALENs for high-throughput genome editing. Nat Biotechnol. (2012) 30:460–5. doi: 10.1038/nbt.2170
24. Caporaso JG, Kuczynski J, Stombaugh J, Bittinger K, Bushman FD, Costello EK, et al. QIIME allows analysis of high-throughput community sequencing data. Nat Methods. (2010) 7:335–6. doi: 10.1038/nmeth.f.303
25. Rognes T, Flouri T, Nichols B, Quince C, Mahé F. VSEARCH: a versatile open source tool for metagenomics. PeerJ. (2016) 4:e2584. doi: 10.7717/peerj.2584
26. Wang Q, Garrity GM, Tiedje JM, Cole JR. Naive Bayesian classifier for rapid assignment of rRNA sequences into the new bacterial taxonomy. Appl Environ Microbiol. (2007) 73:5261–7. doi: 10.1128/AEM.00062-07
27. Chao A, Bunge J. Estimating the number of species in a stochastic abundance model. Biometrics. (2002) 58:531–9. doi: 10.1111/j.0006-341X.2002.00531.x
28. Hill TC, Walsh KA, Harris JA, Moffett BF. Using ecological diversity measures with bacterial communities. FEMS Microbiol Ecol. (2003) 43:1–11. doi: 10.1111/j.1574-6941.2003.tb01040.x
29. Álvarez J, Fernández Real JM, Guarner F, Gueimonde M, Rodríguez JM, Saenz M, et al. Gut microbes and health. Gastroenterol Hepatol. (2021) 44:519–35. doi: 10.1016/j.gastre.2021.01.002
30. Wang X, Zhang P, Zhang X. Probiotics regulate gut microbiota: an effective method to improve immunity. Molecules. (2021) 26:6076. doi: 10.3390/molecules26196076
31. Xia QY, Lu D, Zhang JM, Wei YC, Yang MM, Yang ZY, et al. [Intestinal flora polymorphisms with different lesional stages in an animal model of MAFLD]. Zhonghua Gan Zang Bing Za Zhi. (2021) 29:1069–76. doi: 10.3760/cma.j.cn501113-20200826-00478
32. Sommer F, Rühlemann MC, Bang C, Höppner M, Rehman A, Kaleta C, et al. Microbiomarkers in inflammatory bowel diseases: caveats come with caviar. Gut. (2017) 66:1734–8. doi: 10.1136/gutjnl-2016-313678
33. Sommer F, Anderson JM, Bharti R, Raes J, Rosenstiel P. The resilience of the intestinal microbiota influences health and disease. Nat Rev Microbiol. (2017) 15:630–8. doi: 10.1038/nrmicro.2017.58
34. Gomaa EZ. Human gut microbiota/microbiome in health and diseases: a review. Antonie Van Leeuwenhoek. (2020) 113:2019–40. doi: 10.1007/s10482-020-01474-7
35. Pushpanathan P, Mathew GS, Selvarajan S, Seshadri KG, Srikanth P. Gut microbiota and its mysteries. Indian J Med Microbiol. (2019) 37:268–77. doi: 10.4103/ijmm.IJMM_19_373
36. Aron-Wisnewsky J, Warmbrunn MV, Nieuwdorp M, Clément K. Nonalcoholic fatty liver disease: modulating gut microbiota to improve severity? Gastroenterology. (2020) 158:1881–98. doi: 10.1053/j.gastro.2020.01.049
37. Yoon K, Kim N. Roles of Sex Hormones and Gender in the Gut Microbiota. J Neurogastroenterol Motil. (2021) 27:314–25. doi: 10.5056/jnm20208
38. Barrea L, Frias-Toral E, Verde L, Ceriani F, Cucalón G, Garcia-Velasquez E, et al. PCOS and nutritional approaches: differences between lean and obese phenotype. Metabol Open. (2021) 12:100123. doi: 10.1016/j.metop.2021.100123
39. Yu J, Xiang H, Xie Q. The difference of regulatory effect of two Inonotus obliquus extracts on high-fat diet mice in relation to the fatty acid elongation function of gut microbiota. Food Sci Nutr. (2021) 9:449–58. doi: 10.1002/fsn3.2012
40. Li XY, He C, Zhu Y, Lu NH. Role of gut microbiota on intestinal barrier function in acute pancreatitis. World J Gastroenterol. (2020) 26:2187–93. doi: 10.3748/wjg.v26.i18.2187
41. Hu Y, Teng C, Yu S, Wang X, Liang J, Bai X, et al. Inonotus obliquus polysaccharide regulates gut microbiota of chronic pancreatitis in mice. AMB Express. (2017) 7:39. doi: 10.1186/s13568-017-0341-1
42. Ma J, Hong Y, Zheng N, Xie G, Lyu Y, Gu Y, et al. microbiota remodeling reverses aging-associated inflammation and dysregulation of systemic bile acid homeostasis in mice sex-specifically. Gut Microbes. (2020) 11:1450–74. doi: 10.1080/19490976.2020.1763770
43. d'Afflitto M, Upadhyaya A, Green A, Peiris M. Association between sex hormone levels and gut microbiota composition and diversity-a systematic review. J Clin Gastroenterol. (2022) 56:384–92. doi: 10.1097/MCG.0000000000001676
44. Santos-Marcos JA, Haro C, Vega-Rojas A, Alcala-Diaz JF, Molina-Abril H, Leon-Acuña A, et al. Sex differences in the gut microbiota as potential determinants of gender predisposition to disease. Mol Nutr Food Res. (2019) 63:e1800870. doi: 10.1002/mnfr.201800870
45. Afonso-Pereira F, Dou L, Trenfield SJ, Madla CM, Murdan S, Sousa J, et al. Sex differences in the gastrointestinal tract of rats and the implications for oral drug delivery. Eur J Pharm Sci. (2018) 115:339–44. doi: 10.1016/j.ejps.2018.01.043
46. Pace F, Watnick PI. The interplay of sex steroids, the immune response, and the intestinal microbiota. Trends Microbiol. (2021) 29:849–59. doi: 10.1016/j.tim.2020.11.001
47. Thackray VG. Sex, microbes, and polycystic ovary syndrome. Trends Endocrinol Metab. (2019) 30:54–65. doi: 10.1016/j.tem.2018.11.001
48. Li LY, Han J, Wu L, Fang C, Li WG, Gu JM, et al. Alterations of gut microbiota diversity, composition and metabonomics in testosterone-induced benign prostatic hyperplasia rats. Mil Med Res. (2022) 9:12. doi: 10.1186/s40779-022-00373-4
49. Kovatcheva-Datchary P, Nilsson A, Akrami R, Lee YS, Vadder FD, Arora T, et al. Dietary fiber-induced improvement in glucose metabolism is associated with increased abundance of prevotella. Cell Metab. (2015) 22:971–82. doi: 10.1016/j.cmet.2015.10.001
50. Turnbaugh PJ, Ley RE, Mahowald MA, Magrini V, Mardis ER, Gordon JI, et al. An obesity-associated gut microbiome with increased capacity for energy harvest. Nature. (2006) 444:1027–31. doi: 10.1038/nature05414
51. Kim YS, Unno T, Kim BY, Park MS. Sex differences in gut microbiota. World J Mens Health. (2020) 38:48–60. doi: 10.5534/wjmh.190009
52. Wei J, Zhao Y, Zhou C, Zhao Q, Zhong H, Zhu X, et al. Dietary polysaccharide from Enteromorpha clathrata attenuates obesity and increases the intestinal abundance of butyrate-producing bacterium, Eubacterium xylanophilum, in mice fed a high-fat diet. Polymers. (2021) 13:3286. doi: 10.3390/polym13193286
53. Mainz RE, Albers S, Haque M, Sonntag R, Treichel NS, Clavel T, et al. NLRP6 inflammasome modulates disease progression in a chronic-plus-binge mouse model of alcoholic liver disease. Cells. (2022) 11:182. doi: 10.3390/cells11020182
54. Zhang Z, Hyun JE, Thiesen A, Park H, Hotte N, Watanabe H, et al. Sex-specific differences in the gut microbiome in response to dietary fiber supplementation in IL-10-deficient mice. Nutrients. (2020) 12:182. doi: 10.3390/nu12072088
55. Brown JB, Langley SA, Snijders AM, Wan KH, Morris SNS, Booth BW, et al. An integrated host-microbiome response to atrazine exposure mediates toxicity in Drosophila. Commun Biol. (2021) 4:1324. doi: 10.1038/s42003-021-02847-y
56. Khan I, Ullah N, Zha L, Bai Y, Khan A, Zhao T, et al. Alteration of gut microbiota in inflammatory bowel disease (IBD): cause or consequence? IBD treatment targeting the gut microbiome. Pathogens. (2019) 8:126. doi: 10.3390/pathogens8030126
57. Min Y, Ma X, Sankaran K, Ru Y, Chen L, Baiocchi M, et al. Sex-specific association between gut microbiome and fat distribution. Nat Commun. (2019) 10:2408. doi: 10.1038/s41467-019-10440-5
58. Holscher HD. Dietary fiber and prebiotics and the gastrointestinal microbiota. Gut Microbes. (2017) 8:172–84. doi: 10.1080/19490976.2017.1290756
59. Li JY, Chassaing B, Tyagi AM, Vaccaro C, Luo T, Adams J, et al. Sex steroid deficiency-associated bone loss is microbiota dependent and prevented by probiotics. J Clin Invest. (2016) 126:2049–63. doi: 10.1172/JCI86062
60. Spurbeck RR, Arvidson CG. Inhibition of Neisseria gonorrhoeae epithelial cell interactions by vaginal Lactobacillus species. Infect Immun. (2008) 76:3124–30. doi: 10.1128/IAI.00101-08
61. Zhao L, Yang W, Chen Y, Huang F, Lu L, Lin C, et al. A Clostridia-rich microbiota enhances bile acid excretion in diarrhea-predominant irritable bowel syndrome. J Clin Invest. (2020) 130:438–50. doi: 10.1172/JCI130976
62. Ridlon JM, Ikegawa S, Alves JM, Zhou B, Kobayashi A, Iida T, et al. Clostridium scindens: a human gut microbe with a high potential to convert glucocorticoids into androgens. J Lipid Res. (2013) 54:2437–49. doi: 10.1194/jlr.M038869
63. Rizzetto L, Fava F, Tuohy KM, Selmi C. Connecting the immune system, systemic chronic inflammation and the gut microbiome: the role of sex. J Autoimmun. (2018) 92:12–34. doi: 10.1016/j.jaut.2018.05.008
64. Schanz O, Chijiiwa R, Cengiz SC, Majlesain Y, Weighardt H, Takeyama H, et al. Dietary AhR ligands regulate AhRR expression in intestinal immune cells and intestinal microbiota composition. Int J Mol Sci. (2020) 21:3189. doi: 10.3390/ijms21093189
65. de Jesús-Gil C, Sans-de San Nicolàs L, García-Jiménez I, Ferran M, Pujol RM, Santamaria-Babí LF. Human CLA(+) memory T cell and cytokines in psoriasis. Front Med. (2021) 8:731911. doi: 10.3389/fmed.2021.731911
Keywords: Inonotus obliquus, polysaccharide, intestinal microflora, 16S rRNA, SD rats
Citation: Hu B, He X, Tan J, Ma Y, Wang G, Liu S, Li M, Guo Y, Sun R, Sun M, Deng X, Zhou W and Lv X (2022) Gender-related differences in the effects of Inonotus obliquus polysaccharide on intestinal microorganisms in SD rats model. Front. Vet. Sci. 9:957053. doi: 10.3389/fvets.2022.957053
Received: 30 May 2022; Accepted: 24 August 2022;
Published: 20 September 2022.
Edited by:
Asghar Kamboh, Sindh Agriculture University, PakistanCopyright © 2022 Hu, He, Tan, Ma, Wang, Liu, Li, Guo, Sun, Sun, Deng, Zhou and Lv. This is an open-access article distributed under the terms of the Creative Commons Attribution License (CC BY). The use, distribution or reproduction in other forums is permitted, provided the original author(s) and the copyright owner(s) are credited and that the original publication in this journal is cited, in accordance with accepted academic practice. No use, distribution or reproduction is permitted which does not comply with these terms.
*Correspondence: Binhong Hu, YmluaG9uZy5odTg2JiN4MDAwNDA7bWFpbC5ydQ==; Songqing Liu, c29uZ3FpbmdsaXUmI3gwMDA0MDtjZG51LmVkdS5jbg==