- 1College of Animal Science and Technology, Yangzhou University, Yangzhou, China
- 2Apiculture Science Institute of Jilin Province, Jilin, China
- 3College of Forestry, Beihua University, Jilin, China
- 4College of Food Science and Technology, Jiangsu Agri-animal Husbandry Vocational College, Taizhou, China
- 5Institute of Agricultural Technology, Suranaree University of Technology, Nakhon Ratchasima, Thailand
The health of the western honeybee, Apis mellifera, the most crucial pollinator, has been challenged globally over the past decades. An ectoparasitic mite, Varroa destructor, together with the viruses it vectored, is generally regarded as the vital pathogenic agent. Although the poor health status of A. mellifera compared to its eastern counterpart, Apis cerana, has been broadly identified, the underlying mechanism remains poorly understood and comparison between susceptible and resistant hosts will potentially ameliorate this predicament. Here, we investigated the impacts of two widespread viruses—deformed wing virus type A (DWV-A) and Israeli acute paralysis virus (IAPV), mediated by V. destructor mite, on the capped developing honeybee brood, in the absence of adult workers, of A. mellifera and A. cerana, with positive and negative controls. Our results demonstrated that the endogenous viruses imposed limited damage on the hosts even if the brood was wounded. In contrast, the exogenous viruses introduced by ectoparasites triggered variable mortality of the infested brood between host species. Intriguingly, death causes of both honeybee species presented a similar trend: the acute IAPV generally causes morbidity and mortality of late larvae, while the chronic DWV-A typically leads to brood mortality during and after pupation. Notably, the susceptible immature A. cerana individuals, supported by higher observed mortality and a lower virus tolerance, serve the interests of the colony and foster the overall survival of a resistant honeybee superorganism. These results improve our understanding of the interactions between viruses carried by ectoparasites and their developing hosts, and the novel insight of weak individuals fostering strong colonies may promote breeding efforts to mitigate the indefensible colony losses globally.
Introduction
Honeybees, the most important managed pollinating insect, provide vital ecosystem services and contribute to agricultural crop production worldwide (1). However, the health of the western honeybee, Apis mellifera, has been challenged globally over the past decades. In contrast, its eastern cousin, Apis cerana, thrives in Asia and remains largely unaffected. This distinct health status has been attributed to lower viral loads in A. cerana colonies and to their ability to resist an ectoparasitic mite, Varroa destructor (2–5). V. destructor mites originally parasitized A. cerana colonies and did not infest A. mellifera until the middle of the last century (6, 7). Since then, an invasive lineage of V. destructor rapidly spread worldwide attributed to the global western honeybee trade (7–9).
The ubiquitous ectoparasite V. destructor, which punctures the host's integument and feeds on the bee's fat bodies, lives on developing and adult honeybee hosts (10, 11) and grievously injures the honeybee's immune system (12, 13). Without treatment against these mites by beekeepers, A. mellifera colonies will usually die within 1–2 years, and as a consequence, most wild and feral A. mellifera populations have succumbed to the parasite since it switched hosts nearly a century ago (14, 15). On top of weakening the individuals of a colony, the main damage caused by V. destructor is facilitating virus transmission to its hosts, which is regarded as the most severe biotic threat to western honeybees (10, 13, 16). Although the correlation between V. destructor and the effects of the viruses it vectors has been intensively studied over the past decade [e.g., (13, 16, 17)], the strategies developed by honeybees to resist and overcome viral diseases remain unclear. Comparing such strategies between susceptible and resistant hosts would therefore allow a better understanding of virus resistance dynamics in honeybee populations.
Although more than 30 honeybee viruses have been identified (18), most of them were of minor importance for A. mellifera until the arrival of V. destructor, which serves as a vector to many of them, thereby affecting the host's immune system and enabling a drastic change in virus prevalence (13, 16). Among them, two widespread picorna-like viruses, the deformed wing virus (DWV) and the Israeli acute paralysis virus (IAPV), which can be transmitted by V. destructor, have triggered much attention worldwide (17, 19, 20). DWV is regarded as a chronic virus with long-term latent infection in the host colonies (21, 22), and IAPV is considered to be an acute virus with extremely virulent pathology, as the affected hosts develop paralysis (23–25). The distinct nature of these two viruses is relevant to investigate their roles, mediated by V. destructor, in the infection of susceptible and resistant hosts. The susceptible A. mellifera colonies are distributed worldwide, whereas the resistant A. cerana colonies can only be found in parts of Asia.
In this study, we took advantage of both host species being sympatric in China to better understand the mechanisms underlying the principal resistance of A. cerana to the ectoparasite-associated viruses. The infestation of V. destructor on the developing honeybee brood has been reported to cause partial death of both A. mellifera and A. cerana hosts, i.e., social apoptosis (26, 27). The DWV and IAPV titers of live and dead honeybee broods were quantitatively evaluated. Although three variants of DWV, i.e., DWV-A, DWV-B, and DWV-C, have been described (28, 29), we focused on DWV-A since it occurs most widespread and plays a key role in honeybee health (28, 30). We aimed to examine if the virus proliferation model varied in the two host species that were equipped with different resistance capacities for diseases.
Materials and methods
Honeybee colonies
The experimental A. mellifera (n = 5) and A. cerana (n = 5) colonies were kept at Wenhui Campus, Yangzhou University, Yangzhou City, southeast China (N 32°23′03″ and E 119°25′35″). The A. mellifera colonies were of European origin, without disease-resistant breeding, imported to China a century ago and raised by local beekeepers (31). The A. cerana colonies were of indigenous origin (i.e., Apis cerana cerana). All the colonies were queenright and kept in Langstroth hives. Two of the A. mellifera colonies were not treated against V. destructor mites for 6 months and were used as V. destructor donor colonies (VDI and VDII).
Experimental treatment and sample collection
V. destructor infestation
V. destructor mites enter the brood cells of honeybees just before the cells are about to be sealed by workers. Here, we experimentally infested each freshly capped cell with one V. destructor mite, previously kept on A. mellifera nurse workers that were collected from an apparent healthy colony, for a 2-day dispersal phase (7), following the method established in the laboratory (9). The introduction of the mite into the experimental cell was completed within 6 h after the honeybee larvae were sealed, which has been demonstrated to be consistent with a natural infestation (32). Each batch of V. destructor mites was simultaneously used to infest two combs obtained from each honeybee species. We sampled 10 mites in each batch before experimental infestation to assess the viral load of mites. Freshly capped cells of A. mellifera and A. cerana were infested by the mites derived from VDI and VDII, respectively. For each colony, 15–30 freshly capped cells were used as treatments, and 10–20 control cells were also employed to assess the effect of cell opening. In total, 97 and 93 broods of A. mellifera and 109 and 110 broods of A. cerana were infested by VDI and VDII, respectively. A total of 65 and 57 broods of A. mellifera, and 58 and 55 broods of A. cerana were left uninfested.
Benign pin-prick
Freshly capped cells were used in this test as well. The wax caps were opened and resealed following the methods of experimental infestation (9). Rather than V. destructor introduction, here, we used a sterile capillary needle (Eppendorf TransferTip®-R, Hamburg, Germany) to benignly prick the larva body to assess the effect of endogenous viruses on the wounded brood. Four A. mellifera and four A. cerana colonies, among the five colonies used in the experimental infestation, were employed. For each colony, 15–30 freshly capped cells were used as treatments, and 10–15 control cells were also used. In A. mellifera, a total of 57 larvae were pin-pricked and 50 were left as controls. In A. cerana, a total of 69 larvae were pin-pricked and 44 were left as controls.
Honeybee sample collection
All the treated brood combs were reared in an incubator at 34.5°C and 70% RH (33). One and a half day (1.5 days) later, just before the start of pupation, we collected 5–8 treatment broods and 4–7 control broods with sterile disposable plastic tweezers. The sampling process was done in our laboratory at a room temperature of 30°C, and the sampling time of each group was performed within 30 min, so as to reduce the influence of sampling on the honeybee brood, as well as the state of V. destructor. Larvae that appeared black, smelly, or deflated were considered dead larvae. The remaining brood cells were opened and collected 1 day before the expected adult emergence (after 11 days and 10 days for A. mellifera and A. cerana, respectively). The developmental status of honeybee broods was noted as larva, prepupa, pupa, and pre-emergence adults. The first three stages showing apparent developmental delay were considered as dead brood. Normally developed honeybee brood with dead V. destructor mite in the cell was regarded as failed infestation and was not considered in the data analyses. All the sampled honeybee broods were stored at −80°C until RNA isolation.
Viral load quantification
We extracted the RNA of each honeybee brood and V. destructor mite sample using an isolation kit (Tiangen, Beijing, China), following the manufacturer's protocol. Synthesis of cDNA was conducted with RNA products, according to the instructions of the ReverTra Ace qPCR RT Master Mix (Tiangen, Beijing, China). The viral load of each sample was quantitatively evaluated by qPCR using the ChamQ Universal SYBR qPCR Master Mix (Vazyme, Nanjing, China) on the QuantStudio™3 Real-Time PCR System (Applied Biosystems, CA, USA). Three replicates for each sample were performed in a 96-well reaction plate with 2 μL cDNA in a 20 μL reaction volume mixture. For each run, 1 μL forward and 1 μL reverse primers of DWV-A (F: CGTGGTGTAGTAAGCGTCGT; R: TCATCCGTAGAAAGCCGAGT) or IAPV (F: TCGCTGAAGGCATGTATTTC; R: ATTACCACTGCTCCGACACA) were used. The amplification efficiency of the two primers was assessed to be 100.0 and 102.4%, respectively, by gradient dilution of the positive cloned plasmid. Negative (water) and positive (cloned sequence) controls were also included in the reaction. The thermal cycling conditions consisted of 1 min at 95°C followed by 40 cycles of 95°C for 15 s and 60°C for 1 min. A sample was considered virus positive if the melting temperature of the PCR product was similar to the positive controls' and its Ct value was ≤34. Virus titers of positive samples were log-transformed to account for the exponential distribution of the data, and the transformed data were used in the statistical analysis.
Statistical analyses
Differences in the honeybee brood virus loads among groups were assessed by a non-parametric test followed by a Tamhane post-hoc test since the data did not meet normal distribution. The survival rate of honeybee brood between species was analyzed by logistic regression. Student's t-test was used to analyze the different virus titers of sampled mites between V. destructor donor colonies and other comparisons between the two groups. All the analyses were performed with SPSS Statistics 25.
Results
Viral loads in V. destructor mites
Five batches of V. destructor mites, respectively, from VDI and VDII, were sampled before each experimental infestation. The DWV-A load of VDI mites was significantly higher than VDII (Student's t-test, p < 0.001; Figure 1), and the IAPV load of the former mites was significantly lower than the latter (Student's t-test, p = 0.007; Figure 1). Meanwhile, for the mites from VDI, DWV-A load was significantly higher than IAPV (Student's t-test, p < 0.001), while there was no significant difference between the two viral loads in the mites of VDII (Student's t-test, p = 0.189). Mites from the two donor colonies thus carried different virus baselines before infestation experiments took place.
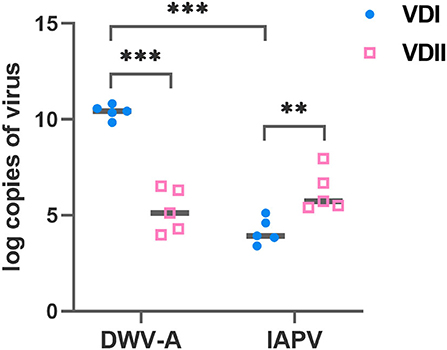
Figure 1. Log-transformed viral loads of Varroa destructor mites used for the experimental infestations. Ten mites were collected as a sample before each experiment and five samples were collected. VDI and VDII, two different V. destructor donor colonies; DWV-A, deformed wing virus type A; IAPV, Israeli acute paralysis virus; **p < 0.01; ***p < 0.001.
Viral loads of honeybee larvae sampled 1.5 days later after capping
A striking difference in brood development was observed. At the first sampling time point-−1.5 days after capping, we collected 37 A. mellifera and 38 A. cerana larvae infested by VDI with, respectively, 83.8 and 68.4% survival rates (logistic regression, p = 0.125; Figure 2A). For VDII, we collected 31 infested larvae of both host species with 80.6 and 58.1% survived brood (logistic regression, p = 0.059; Figure 2A). Twenty A. mellifera and 25 A. cerana pin-pricked larvae were also sampled with 80.0 and 68.0% survival (logistic regression, p = 0.369; Figure 2A). More A. cerana than A. mellifera larvae had died in each treatment type, although the difference between the two species was not significant (Figure 2A). In addition, 31 and 25 for VDI, 27 and 27 for VDII, and 24 and 15 for pin-prick controls of A. mellifera and A. cerana were, respectively, sampled, and no dead larvae were observed (Figure 2A).
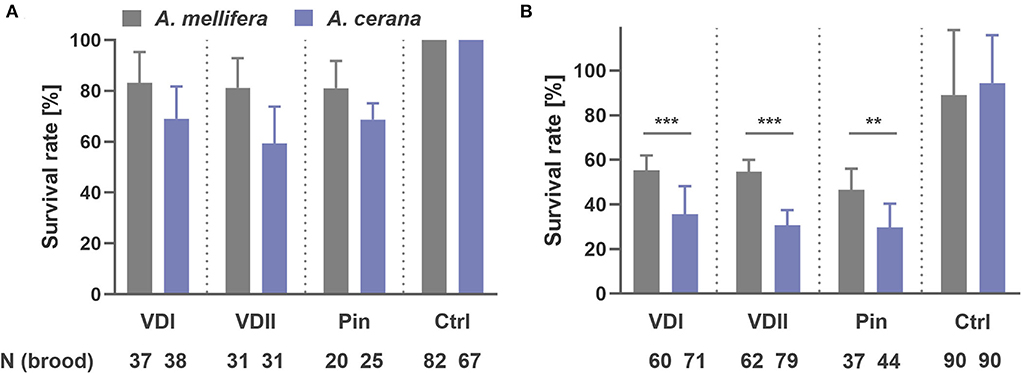
Figure 2. Survival rate of honey bee (Apis mellifera and Apis cerana) brood sampled at 1.5 days after capping (A) and at 1 day before emergence, i.e., 11 and 10 days later after capping, respectively, for A. mellifera and A. cerana (B). VDI and VDII, brood infested by V. destructor mites from two different donor colonies; Pin, the wounded brood pricked by a sterile capillary needle; Ctrl, the pooled control brood with cell opening and resealing operation. **p < 0.01; ***p < 0.001.
Intriguingly, the viral loads of the two host species displayed a similar trend under the same treatment (Supplementary Figure S1). The infested larvae, both live and dead, did not show a high DWV-A infection (Supplementary Figure S1a). In contrast, IAPV in the infested dead larvae presented significantly higher titers than the other groups (Supplementary Figure S1b), indicating a similar lethal mechanism between the two honeybee species. We thus combined the two sister species with the same treatments in the analyses.
The DWV-A loads did not elevate in the dead larvae, while the infested live larvae showed significantly higher DWV-A titers compared to the control (Tamhane, VDI, p = 0.022; VDII, p < 0.001; Figure 3A). The infested dead larvae displayed significantly higher IAPV loads (Tamhane, VDI, and VDII, p < 0.001; Figure 3B), while the infested live ones did not show a higher virus level than the control (Tamhane, VDI, p = 0.963; VDII, p = 0.155; Figure 3B). Meanwhile, the virus levels of all kinds of brood in the pin-prick group were always low (Supplementary Figure S1; Figures 3A,B), suggesting the limited pathogenicity of endogenous viruses in honeybees.
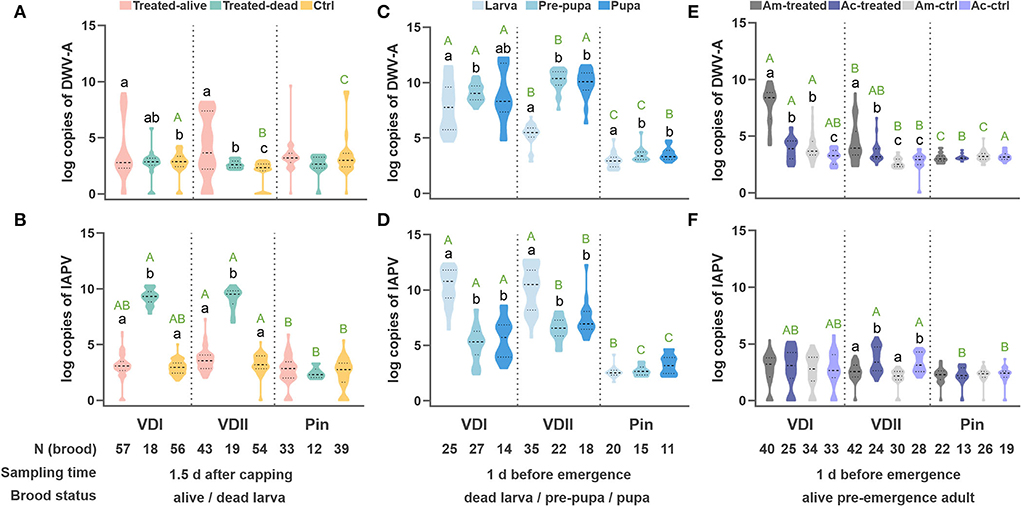
Figure 3. Log-transformed viral loads of the developing honeybee brood. DWV-A load (A) and IAPV load (B) per honeybee (A. mellifera and A. cerana) larva sampled 1.5 days after capping. DWV-A load (C) and IAPV load (D) per dead honeybee brood sampled at 1 day before emergence. DWV-A load (E) and IAPV load (F) per pre-emergence adult. Different black lowercase letters indicate significant differences within a group (p < 0.01) and different green uppercase letters indicate significant differences of DWV-A or IAPV in the samples under the same state (designated as the same color) among VDI, VDII, and Pin groups (p < 0.01). VDI and VDII, brood infested by V. destructor mites from two different donor colonies; Pin, the wounded brood pricked by a sterile capillary needle; Ctrl, the control brood with cell opening and resealing operation corresponding to each treatment; Am, A. mellifera; Ac, A. cerana.
Viral loads of honeybee brood sampled 1 day before the expected emergence date
At the second sampling time point, 11 days and 10 days later after capping, respectively, for A. mellifera and A. cerana, we collected 60 A. mellifera and 71 A. cerana broods infested by VDI with 66.7 and 35.2% surviving pupae, respectively (logistic regression, p < 0.001; Figure 2B). Sixty-two A. mellifera and 79 A. cerana brood were collected in the VDII infestation group with 67.7 and 30.4% survival (logistic regression, p < 0.001; Figure 2B). For the treatment of pin-prick, 37 A. mellifera and 44 A. cerana brood were available with 59.5 and 29.5% survival (logistic regression, p = 0.008; Figure 2B). Significantly more dead brood of the resistant A. cerana were found, compared to A. mellifera, for all the three treatments (Figure 2B). As for the controls, 89.0 ± 29.2% A. mellifera and 94.4 ± 21.5% A. cerana brood survived (logistic regression, p = 0.568).
The virus loads of the dead brood were first evaluated based on different honeybee species. Again, we found a similar trend between the sister species (Supplementary Figures S1c,d). The dead brood of the two honeybee hosts was thus integrated as above. In this case, DWV-A dominated in the dead pre-pupae and pupae for all three groups, particularly for the brood infested by VDII mites (Tamhane, larva vs. pre-pupa, p < 0.001; larva vs. pupa, p < 0.001; Figure 3C). Significantly higher DWV-A loads in the dead larvae infested by VDI compared to VDII (Tamhane, p < 0.001) may be caused by the higher DWV-A copies in the mites (Figure 1). Moreover, generally significantly higher virus loads of the dead brood of mite-infested than of pin-pricked (Figures 3C,D) resulted from the exogenous viruses transmitted by V. destructor as well. Although IAPV load was still high in the dead larva, a significant higher DWV-A level was observed in these samples compared to the dead larvae sampled at 1.5 days (Student's t-test, p < 0.001 for both VDI and VDII), while this is not the case for pin-pricked samples (Student's t-test, p = 0.274), indicating the exogenous DWV-A may proliferate by exploiting dead hosts.
Interestingly, the similar trend of virus loads between honeybee species decreased in the pre-emergence adult samples (Figures 3E,F). As the survived larvae were sampled at 1.5 days after capping, the live pre-emergence adults held relatively low viral loads, specifically IAPV, whether it had been treated or not (Figure 3F). IAPV level was rarely high in the dead pre-pupa or pupa (Figures 3B,D,F), suggesting that this virus predominantly targets the early developmental stages before pupation occurs. DWV-A loads in the infested samples were generally higher than in the controls of corresponding species (Tamhane, VDI, A. mellifera p < 0.001, A. cerana p = 0.026; VDII, A. mellifera p < 0.001, A. cerana p = 0.015; Figure 3E) and DWV-A loads were superior in the infested A. mellifera than in infested A. cerana (Tamhane, VDI, p < 0.001; VDII, p = 0.033; Figure 3E). A. mellifera pre-emergence adults infested by VDI mites exhibited a higher DWV-A load than the ones by VDII mites (Tamhane, p < 0.001), while the difference was not significant in A. cerana individuals (Tamhane, p = 0.611). The pricked honeybees did not show higher viral loads than their counterparts (Tamhane, A. mellifera p = 0.839, A. cerana p = 0.941; Figures 3E,F).
Discussion
We investigated the effect of two common honeybee viruses—DWV and IAPV, mediated by V. destructor mites, on the immature honeybee brood, with positive and negative controls, during the capping period. Consistent with previous findings, the harm of virus infection to the host is limited in absence of V. destructor infestation. However, contrary to our expectations, the two viruses direct the morbidity and mortality of immature honeybees at different developmental stages, and the pathogenesis seems accordant between the sister species A. mellifera and A. cerana. The acute IAPV, vectored by V. destructor, typically targets the early stage during capping and may be the principal cause of dead larvae. Mite-carried exogenous chronic DWV normally targets the developing brood since the initiation of pupation and appears to be the potential leading factor of dead pre-pupa and pupa.
Since the viral loads of the pin-pricked samples were always low with no significant difference compared to the controls (Figure 3), the exogenous viruses vectored by the ectoparasitic V. destructor mites were considered as the potential culprit for the differences in viral loads observed in our experiments. DWV-A did not massively multiply in any of the 1.5 day samples (Figure 3A), suggesting that DWV-A might not be an acute lethal factor for larvae, which is consistent with the chronic virulent attribute (21, 22). Nonetheless, significantly higher IAPV loads were identified in the infested dead larvae (Figures 3B,D). Two hypotheses were thus proposed for the high IAPV in dead larvae: ① IAPV proliferates after or on the verge of the brood death; ② IAPV leads to the death of the host. If the former holds, the endogenous IAPV would have rapidly multiplied in the pin-pricked samples as well, which does not match the facts. Therefore, acute IAPV is very likely to be a potential causative factor in the rapid death of capped larvae.
Following the above conjecture, the high DWV-A loads detected in pre-pupae and pupae may reflect its fatal role (Figure 3C). The distinctly different virus loads between dead larvae and pre-pupae reveal that DWV-A may exponentially multiply during the brood pupation, while the relatively low IAPV loads in the dead pre-pupae and pupae may reflect the different virus replication modes between acute and chronic viruses. On the other hand, an antagonistic interaction between the two viruses (34, 35) may limit the proliferation of IAPV as a result of high DWV-A infection.
The exogenous viruses vectored by V. destructor from two donor colonies led to different viral loads on the infested hosts. In contrast, the viruses of all kinds of brood, including the dead ones, by pin-prick treatment were consistently low. These yields highlight the danger of exogenous viruses mediated by ectoparasitic mites. The corpses of dead larvae, collected 10/11 days after cell capping, had been left in the comb for a few days. Compared to the dead counterparts sampled at 1.5 days, these larva corpses held much higher DWV-A, implying DWV-A may achieve virus proliferation on the dead hosts. However, DWV-A in the pin-pricked dead larvae did not show such differences between the two sampling points. For the dead pre-pupae and pupae, meanwhile, IAPV loads in the infested brood were higher than in the pin-pricked brood (Figure 3D). This divergence between infested and pin-pricked samples may be caused by different virus sources. A finely balanced relationship resides between the endogenous virus and the host (17, 36), while the exogenous viral load may destroy this balance since host immunosuppression induced by V. destructor parasitism has been demonstrated to facilitate virus replication (13). The host immunosuppression induced by V. destructor parasitism facilitates virus replication as well (12). It hence will be of particular interest in future studies to separate the roles of exogenous virus load and ectoparasite presence to investigate their individual effects on the developing honeybee brood.
In accordance with the dead brood after pupation, the IAPV level is low in the pre-emergence adults (Figure 3F), implying a covert infection in the colonies (23). Covert infection of the chronic and hypovirulent DWV-A is well-known in honeybees (16, 37, 38). Furthermore, the higher DWV-A load in the susceptible A. mellifera, compared to the resistant A. cerana, suggests a higher tolerance to this virus in the former individuals, which seems to be in contradiction to the weak disease resistance. Indeed, the death of infested and infected brood can limit the proliferation and spread of pathogens to other individuals in the social colony (26, 39). The susceptible immature A. cerana individuals, supported by higher observed mortality (Figure 2) and a lower virus tolerance (Figure 3) in our experiments, would thus contribute to the overall survival of a resistant honeybee superorganism, which has been defined as a group immunity of social apoptosis (26, 27). Such findings of achieving social immunity at the expense of individuals are not only instructive for researchers but also could be implemented and integrated into A. mellifera breeding programs, which may ultimately alleviate global colony losses and thus contribute to sustain natural and agricultural ecosystems in the long run.
Data availability statement
The original contributions presented in the study are included in the article/Supplementary material, further inquiries can be directed to the corresponding authors.
Author contributions
TJ, QN, and ZL conceived the ideas and designed the study. NZ, MZ, and QW carried out the experimental infestations. NZ and ZW performed the pin-prick experiment. ZL, DN, and KW performed the data analyses. ZL, PP, and DN led the writing of the manuscript. All authors contributed critically to the drafts and gave final approval for publication.
Funding
The financial support was granted by the National Natural Science Foundation of China (32272935 and 31902220 to ZL; 32202743 to DN), the Science and Technology Development Project of Jilin Province (20200402042NC to ZW and QN), the Earmarked Fund for Modern Agro-industry Technology Research System (CARS-44 to TJ, QN, and ZW), the COLOSS Ricola Award for Excellence (ZL), and the Lvyangjinfeng Program of Yangzhou (YZLYJFJH2021YXBS155 to ZL).
Conflict of interest
The authors declare that the research was conducted in the absence of any commercial or financial relationships that could be construed as a potential conflict of interest.
Publisher's note
All claims expressed in this article are solely those of the authors and do not necessarily represent those of their affiliated organizations, or those of the publisher, the editors and the reviewers. Any product that may be evaluated in this article, or claim that may be made by its manufacturer, is not guaranteed or endorsed by the publisher.
Supplementary material
The Supplementary Material for this article can be found online at: https://www.frontiersin.org/articles/10.3389/fvets.2022.951159/full#supplementary-material
References
1. Potts SG, Imperatriz-Fonseca V, Ngo HT, Aizen MA, Biesmeijer JC, et al. Safeguarding pollinators and their values to human well-being. Nature. (2016) 540:220–9. doi: 10.1038/nature20588
2. Ellis JD, Munn PA. The worldwide health status of honey bees. Bee World. (2005) 86:88–101. doi: 10.1080/0005772X.2005.11417323
3. Ai H, Yan X, Han R. Occurrence and prevalence of seven bee viruses in Apis mellifera and Apis cerana apiaries in China. J Invertebr Pathol. (2012) 109:160–4. doi: 10.1016/j.jip.2011.10.006
4. Li J, Qin H, Wu J, Sadd BM, Wang X, Evans JD, et al. The prevalence of parasites and pathogens in Asian honeybees Apis cerana in China. PLoS ONE. (2012) 7:e47955. doi: 10.1371/journal.pone.0047955
5. Lin Z, Page P, Li L, Qin Y, Zhang Y, Hu F, et al. Go East for better honey bee health: Apis cerana is faster at hygienic behavior than A. mellifera PLoS ONE. (2016) 11:e0162647. doi: 10.1371/journal.pone.0162647
6. Rosenkranz P, Aumeier P, Ziegelmann B. Biology and control of Varroa destructor. J Invertebr Pathol. (2010) 103:S96–119. doi: 10.1016/j.jip.2009.07.016
7. Traynor KS, Mondet F, de Miranda JR, Techer M, Kowallik V, Oddie M, et al. Varroa destructor: a complex parasite, crippling honey bees worldwide. Trends Parasitol. (2020) 36:592–606. doi: 10.1016/j.pt.2020.04.004
8. Anderson D, Trueman J. Varroa jacobsoni (Acari: Varroidae) is more than one species. Exp Appl Acarol. (2000) 24:165–89. doi: 10.1023/A:1006456720416
9. Lin Z, Wang S, Neumann P, Chen G, Page P, Li L, et al. Population genetics and host specificity of Varroa destructor mites infesting eastern and western honeybees. J Pest Sci. (2021) 94:1487–504. doi: 10.1007/s10340-020-01322-7
10. Nazzi F, Le Conte Y. Ecology of Varroa destructor, the major ectoparasite of the western honey bee, Apis mellifera. Annu Rev Entomol. (2016) 61:417–32. doi: 10.1146/annurev-ento-010715-023731
11. Ramsey SD, Ochoa R, Bauchan G, Gulbronson C, Mowery JD, Cohen A, et al. Varroa destructor feeds primarily on honey bee fat body tissue and not hemolymph. Proc Natl Acad Sci USA. (2019) 116:1792–801. doi: 10.1073/pnas.1818371116
12. Yang X, Cox-Foster DL. Impact of an ectoparasite on the immunity and pathology of an invertebrate: evidence for host immunosuppression and viral amplification. Proc Natl Acad Sci USA. (2005) 102:7470–5. doi: 10.1073/pnas.0501860102
13. Di Prisco G, Annoscia D, Margiotta M, Ferrara R, Varricchio P, Zanni V, et al. A mutualistic symbiosis between a parasitic mite and a pathogenic virus undermines honey bee immunity and health. Proc Natl Acad Sci USA. (2016) 113:3203–8. doi: 10.1073/pnas.1523515113
14. Kraus B, Page Jr RE. Effect of Varroa jacobsoni (Mesostigmata: Varroidae) on feral Apis mellifera (Hymenoptera: Apidae) in California. Environ Entomol. (1995) 24:1473–80. doi: 10.1093/ee/24.6.1473
15. Oldroyd BP. What's killing American honey bees? PLoS Biol. (2007) 5:e168. doi: 10.1371/journal.pbio.0050168
16. Martin SJ, Highfield AC, Brettell L, Villalobos EM, Budge GE, et al. Global honey bee viral landscape altered by a parasitic mite. Science. (2012) 336:1304–6. doi: 10.1126/science.1220941
17. Wilfert L, Long G, Leggett HC, Schmid-Hempel P, Butlin R, et al. Deformed wing virus is a recent global epidemic in honeybees driven by Varroa mites. Science. (2016) 351:594–7. doi: 10.1126/science.aac9976
18. Beaurepaire A, Piot N, Doublet V, Antunez K, Campbell E, Chantawannakul P, et al. Diversity and global distribution of viruses of the western honey bee, Apis mellifera. Insects. (2020) 11:239. doi: 10.3390/insects11040239
19. Di Prisco G, Pennacchio F, Caprio E, Boncristiani HF Jr, Evans JD, Chen Y. Varroa destructor is an effective vector of Israeli acute paralysis virus in the honeybee, Apis mellifera. J Gen Virol. (2011) 92(Pt 1):151–5. doi: 10.1099/vir.0.023853-0
20. Cox-Foster DL, Conlan S, Holmes EC, Palacios G, Evans JD, Moran NA, et al. A metagenomic survey of microbes in honey bee colony collapse disorder. Science. (2007) 318:283–7. doi: 10.1126/science.1146498
21. McMenamin AJ, Genersch E. Honey bee colony losses and associated viruses. Curr Opin Insect Sci. (2015) 8:121–9. doi: 10.1016/j.cois.2015.01.015
22. Kang Y, Blanco K, Davis T, Wang Y, DeGrandi-Hoffman G. Disease dynamics of honeybees with Varroa destructor as parasite and virus vector. Math Biosci. (2016) 275:71–92. doi: 10.1016/j.mbs.2016.02.012
23. de Miranda JR, Cordoni G, Budge G. The Acute bee paralysis virus-Kashmir bee virus-Israeli acute paralysis virus complex. J Invertebr Pathol. (2010) 103(Suppl. 1):S30–47. doi: 10.1016/j.jip.2009.06.014
24. Chen YP, Pettis JS, Corona M, Chen WP, Li CJ, Spivak M, et al. Israeli acute paralysis virus: epidemiology, pathogenesis and implications for honey bee health. PLoS Pathog. (2014) 10:e1004261. doi: 10.1371/journal.ppat.1004261
25. Tantillo G, Bottaro M, Di Pinto A, Martella V, Di Pinto P, Terio V. Virus Infections of Honeybees Apis Mellifera. Ital J Food Saf. (2015) 4:5364. doi: 10.4081/ijfs.2015.5364
26. Page P, Lin Z, Buawangpong N, Zheng H, Hu F, Neumann P, et al. Social apoptosis in honey bee superorganisms. Sci Rep. (2016) 6:27210. doi: 10.1038/srep27210
27. Lin Z, Qin Y, Page P, Wang S, Li L, Wen Z, et al. Reproduction of parasitic mites Varroa destructor in original and new honeybee hosts. Ecol Evol. (2018) 8:2135–45. doi: 10.1002/ece3.3802
28. Mordecai G, Wilfert L, Martin S, Jones IM, Schroeder DC. Diversity in a honey bee pathogen: first report of a third master variant of the Deformed Wing Virus quasispecies. ISME J. (2016) 10:1264–73. doi: 10.1038/ismej.2015.178
29. Mordecai G, Brettell L, Martin S, Dixon D, Jones IM, Schroeder DC. Superinfection exclusion and the long-term survival of honey bees in Varroa-infested colonies. ISME J. (2016) 10:1182–91. doi: 10.1038/ismej.2015.186
30. Posada-Florez F, Childers AK, Heerman MC, Egekwu NI, Cook SC, Chen Y, et al. Deformed wing virus type A, a major honey bee pathogen, is vectored by the mite Varroa destructor in a non-propagative manner. Sci Rep. (2019) 9:12445. doi: 10.1038/s41598-019-47447-3
32. Frey E, Odemer R, Blum T, Rosenkranz P. Activation and interruption of the reproduction of Varroa destructor is triggered by host signals (Apis mellifera). J Invertebr Pathol. (2013) 113:56–62. doi: 10.1016/j.jip.2013.01.007
33. Crailsheim K, Brodschneider R, Aupinel P, Behrens D, Genersch E, Vollmann J, et al. Standard methods for artificial rearing of Apis mellifera larvae. J Apic Res. (2013) 52:1–16. doi: 10.3896/IBRA.1.52.1.05
34. Mascia T, Gallitelli D. Synergies and antagonisms in virus interactions. Plant Sci. Int. J. Exp. Plant Biol. (2016) 252:176–92. doi: 10.1016/j.plantsci.2016.07.015
35. Syller J, Grupa A. Antagonistic within-host interactions between plant viruses: molecular basis and impact on viral and host fitness. Mol Plant Pathol. (2016) 17:769–82. doi: 10.1111/mpp.12322
36. Varela M, Spencer TE, Palmarini M, Arnaud F. Friendly viruses: the special relationship between endogenous retroviruses and their host. Ann N Y Acad Sci. (2009) 1178:157–72. doi: 10.1111/j.1749-6632.2009.05002.x
37. Mondet F, de Miranda JR, Kretzschmar A, Le Conte Y, Mercer AR. On the front line: quantitative virus dynamics in honeybee (Apis mellifera L.) colonies along a new expansion front of the parasite Varroa destructor. PLoS Pathog. (2014) 10:e1004323. doi: 10.1371/journal.ppat.1004323
38. Kevill JL, de Souza FS, Sharples C, Oliver R, Schroeder DC, Martin SJ. DWV-a lethal to honey bees (Apis mellifera): a colony level survey of DWV variants (A, B, and C) in England, Wales, and 32 States across the US. Viruses. (2019) 11:426. doi: 10.3390/v11050426
Keywords: honeybee, deformed wing virus (DWV), Israeli acute paralysis virus (IAPV), Varroa destructor, social apoptosis
Citation: Lin Z, Zhang N, Wang Z, Zhuang M, Wang Q, Niu D, Page P, Wang K, Niu Q and Ji T (2022) Acute and chronic viruses mediated by an ectoparasite targeting different developmental stages of honeybee (Apis mellifera and Apis cerana) brood. Front. Vet. Sci. 9:951159. doi: 10.3389/fvets.2022.951159
Received: 23 May 2022; Accepted: 19 September 2022;
Published: 06 October 2022.
Edited by:
Levon Abrahamyan, Université de Montréal, CanadaReviewed by:
Marcelo Lisandro Signorini, Consejo Nacional de Investigaciones Científicas y Técnicas (CONICET), ArgentinaBin Han, Chinese Academy of Agricultural Sciences (CAAS), China
Eugene V. Ryabov, University of Maryland, United States
Copyright © 2022 Lin, Zhang, Wang, Zhuang, Wang, Niu, Page, Wang, Niu and Ji. This is an open-access article distributed under the terms of the Creative Commons Attribution License (CC BY). The use, distribution or reproduction in other forums is permitted, provided the original author(s) and the copyright owner(s) are credited and that the original publication in this journal is cited, in accordance with accepted academic practice. No use, distribution or reproduction is permitted which does not comply with these terms.
*Correspondence: Ting Ji, dGppQHl6dS5lZHUuY24=; Qingsheng Niu, MTQ2MzE5OTc3OUBxcS5jb20=