- 1College of Animal Science and Technology, Hebei Agricultural University, Baoding, China
- 2Hebei Provincial Animal Husbandry Station, Shijiazhuang, China
- 3Mountainous Area Research Institute of Hebei Province, Hebei Agricultural University, Baoding, China
- 4Agricultural Technology Innovation Center in Mountainous Areas of Hebei Province, Baoding, China
This experiment aimed to establish the effects of zearalenone (ZEN) on ovarian development in prepubertal gilts through the growth hormone axis [growth hormone-releasing hormone (GHRH) / growth hormone (GH) / growth hormone receptor (GHR)]. In a 40-day experiment, 48 Landrace × Yorkshire crossbred prepubertal gilts were randomly allocated to four dietary treatments, including a basal diet supplemented with 0 (control), 400 (T1), 800 (T2), and 1,600 (T3) μg/kg ZEN. The ovary index of T2 (P = 0.058) and T3 (P = 0.065) increased compared to the control group. Besides, histopathological examination revealed that ZEN promoted the development of ovaries and follicles. The GHR content, relative expression levels of GHR, janus activated kinase 2 (JAK2) mRNA, and mean optical density of GHR in the ovaries of prepubertal gilts in the T2 experimental group increased significantly at P < 0.05 compared to the control group. The T3 group had significantly higher GHR content, relative JAK2 expression levels, and signal transducer and activator of transcriptions 3 (STAT3) mRNA. In conclusion, ZEN enhances the biological effect of GH, promotes the development of the ovary (follicle), and exerts reproductive toxicity by increasing the expression level of GHR, JAK2, and STAT3 mRNA ovary and immune intensity of GHR protein.
Introduction
Zearalenone (ZEN) is a mycotoxin mainly produced by Fusarium and has estrogenic activity and various toxic effects (1). This mycotoxin is widely found in feedstuff and compound feeds of pigs and ranks among the most common and harmful mycotoxins in pig production (2, 3). Besides, ZEN affects the reproductive and immune performance of pigs in different growth stages. In a previous study done by Gruber-Dorninger et al. (4) from 2008 to 2017, 61,413 feedstuff and feed samples were collected worldwide. The test results showed a ZEN contamination rate of 88%, a median of 55 μg/kg, and the highest pollution value of 105 mg/kg.
Ovary is an important reproductive organ of sows, and its growth, development, and functional status directly affects the reproductive performance and breeding value of sows (5). Numerous studies have shown that ZEN affects the development of sows' ovaries and exert reproductive toxicity. According to Dai et al. (6), 1.04 mg/kg of ZEN promotes ghrelin gene expression in a piglet ovary and accelerates ovarian follicular depletion via massive follicular activation. In addition, Yang et al. (7) reported that ZEN activates estrogen receptors (ERs)/glycogen synthase kinase (GSK)-3β-dependent Wnt-1/β-catenin signaling pathway and promotes the development of the porcine ovary. Maternal ZEN exposure affects the ovarian development of offspring through breast milk (8). ZEN affects ovarian development in many ways. However, few reports indicate how it affects ovary development through the growth hormone axis (GHRH/GH/GHR). The growth hormone-releasing hormone (GHRH) peptide hormone is secreted by the hypothalamus, which stimulates the pituitary gland to secrete the growth hormone (GH) (9). The growth hormone receptor (GHR) is a single-stranded transmembrane glycoprotein, which regulates the target organs growth by signaling autocrine or paracrine through GH-specific binding (10). According to Zhou et al. (11) GHR is distributed in the ovaries and uterus of pigs, while ZEN increases the relative weight of the uteri and thickness of the myometrium and endometrium of the pig by regulating the GHR expression.
In women, the non-growing population of follicles that constitutes the ovary reserve function is determined at birth (12). This reserve of the dormant, primordial follicle and the mechanism controlling their selective activation is closely related to the women reproductive potential. On the other hand, when the ovary develops too fast, the excessive induction of primordial follicle activation leads to ovarian dysfunction. Previously, ZEN and its derivatives have been detected in various foods such as infant food supplements, bread, and dairy products (13, 14). Besides, ZEN exposure may lead to numerous diseases such as premature thelarche, idiopathic central precocious puberty, hormonal disruption, ovarian cancer and cervical cancer (15, 16).
The ZEN contamination causes economic losses in the pig industry and poses a potential threat to food safety and human health. Currently, the ZEN mechanism on ovary and follicle development has not been fully elucidated. Therefore, this experiment aimed to study the ZEN effects on ovary development of the prepubertal gilts through the growth hormone axis (GHRH/GH/GHR). The findings reported here in will provide a reference for exploring the toxic mechanism of ZEN.
Materials and methods
Experimental diet
ZEN with a guaranteed purity ≥98% was purchased from Triplebond (Guelph, Ontario, Canada). Prepubertal gilts were fed with corn-soybean meal diet with no antibiotics and mildew remover. The basic diet was prepared before the experiment using National Research Council (NRC) (2012) protocol; its composition and nutrition levels are shown in Table 1.
Dietary preparation was completed before the experiments. Based on the enzyme linked immunosorbent assay (ELISA), the ZEN contents in the diets of each group were; 52.37, 241.60, 825.20, and 1 634.46 μg/kg, while other mycotoxins (aflatoxin B1, vomitoxin and fumonisin) were not detected. The ZEN, vomitoxin, and aflatoxin B1 kits were purchased from Fender Biotechnology Co., Ltd. (Shenzhen, China), while the fumonisin kits were purchased from Meilian Biotechnology Co., Ltd. (Shanghai, China).
Experimental design, animal and management
Forty-eight Landrace × Yorkshire crossbred prepubertal gilts (aged 65 ± 3 days, initial body weight 23.20 ± 0.68 kg) were randomly selected and divided into four groups with 12 replicates in each group and one gilt in each replicate. The control group (CON) was fed with a basal diet, while the experimental groups (T1, T2, and T3) were fed with experimental diets obtained by supplementing the basal diet with 200, 800, and 1,600 μg/kg of ZEN. The preliminary trial period was set as 7 days, and the trial period was set as 40 days. Before the experiment, the piggery was cleaned, disinfected, and gilts reared in columns.
The experimental protocols were approved by the Animal Care and Use Committee of Hebei Agriculture University (Baoding, China).
All animal experiments complied with the ARRIVE guidelines and were carried out in accordance with the U.K. Animals (Scientific Procedures) Act, 1986 and associated guidelines, EU Directive 2010/63/EU for animal experiments.
Measurement indicators and methods
Serum and tissue indicators
At the end of the trial period, the test prepubertal gilts were subjected to fasting for 12 h. After that, eight prepubertal gilts were randomly selected from each group, and 15 mL of blood was collected from the anterior vena cava of the sampled prepubertal gilts. The sampled blood was poured into centrifuge tubes, inclined and left to settle for 30 min and then centrifuged at 3000 × g for 10 min to separate the serum. Four prepubertal gilts in each experimental group were randomly selected. The sampled gilts were subjected to euthanasia, and the hypothalamus, pituitary, and ovarian tissues samples were obtained under aseptic conditions, and frozen at−80°C for subsequent tests. Enzyme- ELISA was used to determine the GHRH content in the serum and the GHRH, GH, and GHR contents in the sampled prepubertal gilts tissues. The kit was purchased from Meilian Biotechnology Co., Ltd. (Shanghai, China) and operated according to the instructions.
Growth performance and ovarian index
The live prepubertal gilts were weighed before slaughter, while the ovarian tissue was weighed after slaughter. The obtained weight was used to calculate the organ index based on the formula;
Ovarian index (g/kg) = fresh weight of ovary (g) after slaughter/ live weight before slaughter (kg).
The ovarian tissue morphology
The ovarian tissues were picked under aseptic conditions and fixed using a 10% neutral formaldehyde fixing solution. The fixed tissues were dehydrated using a full-automatic dehydrator (TSJ-II, Zhongshan, Changzhou, China). After embedding and slicing, the following operations were performed: the fixed tissues were subjected to slice dewaxing. After that, it was put in hematoxylin stain for 15 min and rinsed with tap water for 2 min. Thereafter, hydrochloric acid alcohol differentiation was done for 10 s, rinsed with tap water for 2 min, and put in warm water at 50°C until the solution turned blue. It was then rinsed with tap water for 2 min, and put in 85% alcohol for 4 min. The tissues were then stained with eosin for 4 min, washed with distilled water for 5 s. The tissues were then dehydrated using gradient alcohol, made transparent using xylene, and sealed with neutral gum. The trinocular biological microscope camera system (BA200 digital, motic, Xiamen, China) was used for slice observation and image acquisition. All tissues of each section were observed using a microscopic camera system at X40, and the tissue images were taken at X100 and X400. The type and number of follicles were counted according to Schoevers et al. (17). Follicles were counted and classified as primordial follicles, growing follicles and atretic follicles.
Relative gene expression in the tissues
The relative content of mRNA of tissue samples were detected by quantitative real-time PCR (qRT-PCR). The specific primers were designed using primer 6.0 software based on the gene sequences of glyceraldehyde-3-phosphate dehydrogenase (GAPDH), GHRH, growth hormone releasing hormone receptor (GHRHR), GH, GHR, janus activated kinase 2 (JAK2), and signal transducer and activator of transcriptions 3 (STAT3) in the GenBank and were synthesized by Bioengineering Co., Ltd. (Shanghai, China) (Table 2).
The total RNA was extracted using 50–100 mg of the ovarian, hypothalamic and hypophyseal samples, respectively, following the Trizol reagent according to the manufacture's instruction (Invitrogen, Carlsbad, USA). The extracted RNA concentration was detected using an RNA concentration meter (Nanodrop Lite, Thermo Fisher Scientific, Massachusetts, USA). A total of 1 μg RNA were used for cDNA synthesis with the kit of HiScript® III RT SuperMix for qPCR (+gDNA wiper) (Number: R323-01, Vazyme, Nanjing, China) based on the manufacture's instruction. The qRT-PCR was performed with CFX96 touch real-time PCR detection system (Bio-Rad, Hercules, CA, USA) using the primers displayed in Table 2. GAPDH was selected as internal control to compare the amplification efficacy. The ChamQ Universal SYBR qPCR Master Mix (Number: Q711-02, Vazyme, Nanjing, China) was used in qRT-PCR. A total of 20 μL qRT-PCR mixture consisted of 10 μL SYBR Green Master Mix, 0.8 μL of forward and reverse primers mix (stock concentration of 10 μmol/L), 2 μL (200 ng) template cDNA and 7.2 μL DNase/RNase Free H2O. The heat-cycling conditions of qRT-PCR: 95°C for 5 min, followed by 40 cycles at 95°C for 10 s, 60°C for 30 s of melt curve analysis. The experiment was repeated three times and the target gene expression was calculated by the 2−ΔΔCt method.
Immunohistochemical detection
The ovarian tissue was picked under aseptic condition and fixed on using 4% paraformaldehyde fixing solution, and the fixed tissues were dehydrated using a full-automatic dehydrator (TSJ-II, Zhongshan, Changzhou, China). After embedding and slicing, the following operations were carried out: After dewaxing, the sliced tissues were put on a dyeing tank with 3% methanol hydrogen peroxide for 10 min at room temperatures. After that, the tissues were removed and washed three times with PBS, each lasting for 5 min. After washing, the samples slices were immersed in 0.01 M citrate buffer solution (pH = 6.0), heated until it boiled, and allowed to settle. After cooling for 5 min, the same procedure was repeated; washed with PBS twice, each washing step lasting for 5 min. Thereafter, a goat serum was added dropwise to the block solution (Number: zli-9021, ZSBIO, Beijing, China) and allowed to settle at room temperature for 20 min. Besides, primary antibody (GHR, Number: BS-0654R, Bioss, Beijing, China), drops were added and allowed to react overnight at 4°C. After the overnight reaction, a biotinylated secondary antibody (Number: SP-9001, ZSBIO, Beijing, China) was added and allowed to settle at 37°C for 30 min then washed three times with PBS, each step lasting for 5 min. The eighth step was the DAB color development, where the DAB color development kit reagents (Number: K135925C, ZSBIO, Beijing, China) were mixed evenly at room temperature and dropped on the slices to develop the color. The control reaction time under a microscope was set at 2 min, and after that, washed with distilled water. Hematoxylin (Number: LM10N13, J&K Scientific, Beijing, China) was used for light re-dying, followed by dehydration, transparency, and sealing with neutral gum. The microscopic camera system (BA200 digital, motic, Xiamen, China) was used for slice observation and image acquisition. All the tissue slices from each sample were observed at X100, and three visual fields at X400 were selected for microscopic images acquisition. Image-Pro Plus, a 6.0 image analysis system, was used to measure the optical density, area and to calculate the mean optical density (MD) of each image.
Statistical analysis
The statistical data analysis was done using Excel 2016 and SPSS 20.0 software. One-way analysis of variance (ANOVA) was used to test the significant differences between each group data, while the Duncan method was used for multiple comparisons. A p < 0.05 showed a significant difference between the groups, and 0.05 < P < 0.10 was considered a tendency.
Results
Ovarian index
The results of the ovarian index are shown in Figure 1A. There was no significant difference between the ovarian index of the prepubertal gilts groups at P > 0.05. However, when compared with the CON group, T2 (P = 0.058) and T3 (P = 0.065) groups exhibited an increasing trend.
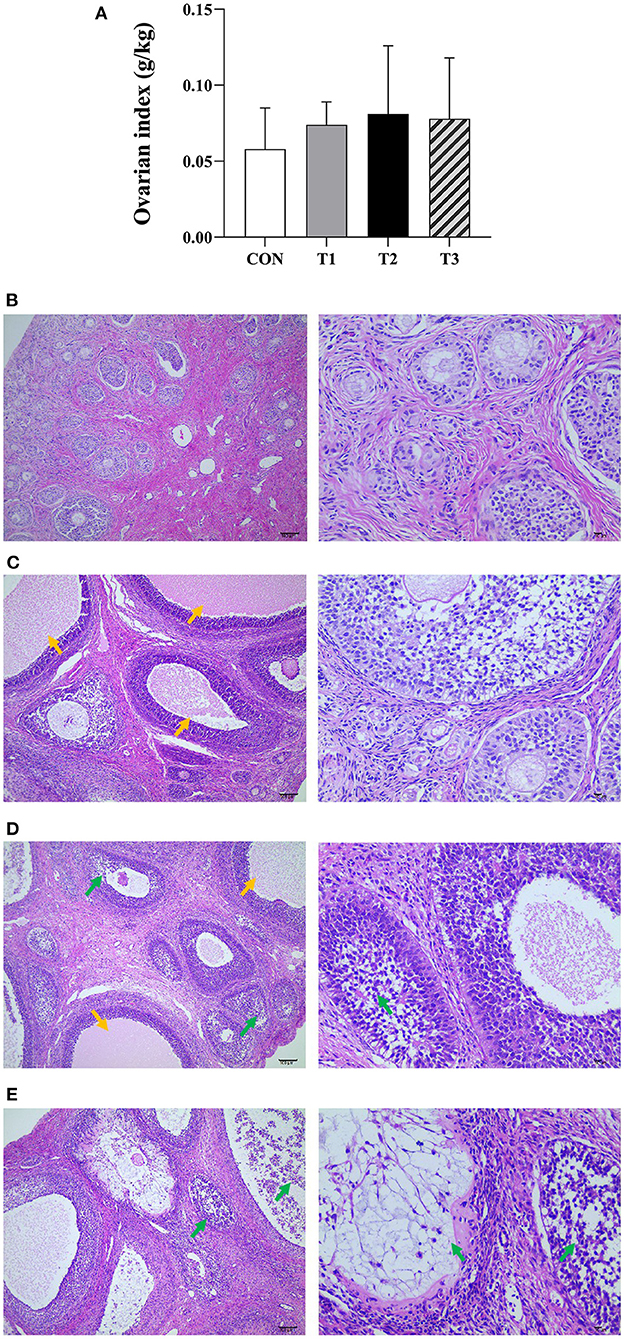
Figure 1. Effects of ZEN on the ovarian index (A), ovarian structure and morphology of prepubertal gilts (B–E). (B–E) Images were taken at X100 (left) and X400 (right) magnification. Key: green- atretic follicle, yellow-secondary follicle simultaneous development. The magnification used was X100 and X400 and scales of 100 μm and 10 μm, respectively. In (A), the letters above the bar are the same, indicating non-significant difference (P > 0.05). N = 4, the data are presented as mean ± standard deviation (SD). The number of primordial follicles in each group is as follows: 84.17 ± 8.42c, 75.67 ± 7.5c, 58.31 ± 11.20ab, 47.50 ± 13.97a, respectively, P < 0.01. The number of growing follicles in each group is as follows: 14.17 ± 2.79a, 16.32 ± 5.13ab, 23.83 ± 7.35c, 20.85 ± 5.78bc, respectively, P = 0.03. The number of atretic follicles in each group is as follows: 5.01 ± 1.79a, 5.67 ± 2.16a, 10.53 ± 3.21b, 14.36 ± 4.77c, respectively, P < 0.01. In the same row, values with different small letter superscripts mean significant difference (P < 0.05).
Ovarian structure and morphology
It can be seen from Figures 1B–E that the ovarian capsule of the prepubertal gilts in the CON group was intact, and the number of follicles was normal at all layers of the cortex. The primordial follicles had a small size and were mainly found on the ovarian cortex superficial layer. Besides, the primary follicle size was larger than that of the primordial follicle, while the epithelium follicle had a multilayered cubic or columnar structure with zona pellucida. The secondary follicles cells proliferated between 6 and 12 layers, and the radiation crowns were formed in the follicular cavities and had a few corpus luteum tissues. The medulla connective tissues were loosely arranged and had abundant blood vessels. Compared with the CON group, the number of primordial follicles decreased in all the experimental groups. However, multiple secondary follicles developed simultaneously with increased follicular volume. The number of atretic follicles increased, the corpus luteum was rarely observed, and the medulla connective tissues were loosely arranged. In T2 and T3 experimental groups, the follicles had necrotic and exfoliated granulosa cells with either contracted or fragmented nuclei. These results show that ZEN promotes follicle development, and an increase in dosage increases the effects on the follicles. In addition, ZEN causes follicular atresia, and an increase in dosage significantly increases the number of atretic follicles. The follicles had necrotic and detached granular cells with either contracted or broken nuclei, resulting in ovarian damage. In this study, ZEN did not cause ovary degeneration, necrosis, and hyperplasia. However, in T2 and T3 experimental groups, inflammatory infiltration was observed.
Serum GHRH content
There was no significant difference between the GHRH content of prepubertal gilts serum in each group at P > 0.05 (Figure 2A).
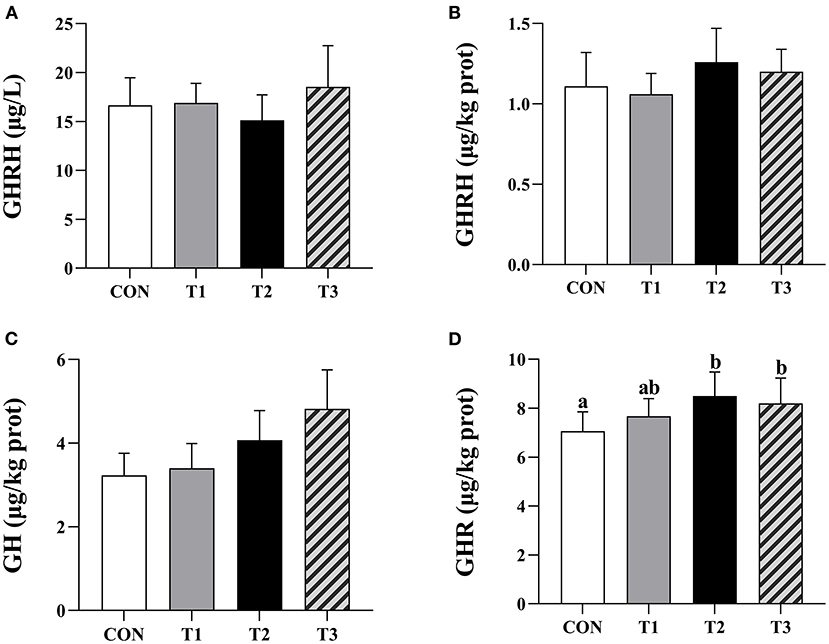
Figure 2. Effects of ZEN on the contents of serum GHRH, hypothalamus GHRH, pituitary GH, and ovarian GHR. Above the bar no letter or the same letter mean no significant (P > 0.05), while with different letter mean significant difference (P < 0.05). In (A), N = 8, in (B–D), N = 4, bars represent the means ± standard deviation (SD). Growth hormone-releasing hormone (GHRH), Growth hormone (GH), Growth hormone receptor (GHR). The content of ovarian GHR in each group: 7.06 ± 0.79a, 7.67 ± 0.72ab, 8.50 ± 0.98b, 8.20 ± 1.03b, respectively, P = 0.03. In the same row, values with different small letter superscripts mean significant difference (P < 0.05).
Contents of hypothalamus GHRH, pituitary GH, and ovarian GHR
There was no significant difference in hypothalamic GHRH and pituitary GH contents in each group of the prepubertal gilts at P > 0.05 (Figures 2B,C). When compared with the CON group, the ovarian GHR content in T2 and T3 experimental groups was significantly high (P < 0.05) (Figure 2D).
Relative expression levels of hypothalamus GHRH, pituitary GHRHR, and GH mRNA
There was no significant difference in the relative expression levels of hypothalamus GHRH, pituitary GHRHR, and GH mRNA in each group of the prepubertal gilts at P > 0.05 (Figures 3A–C).
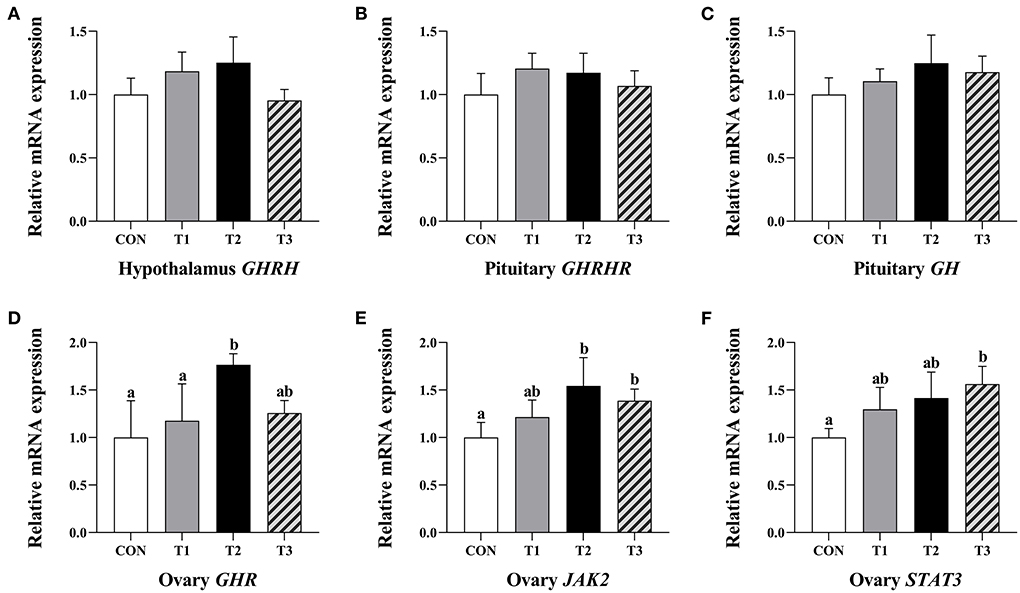
Figure 3. Effects of ZEN on the relative expression level of hypothalamus GHRH gene (A), GHRHR (B) and GH gene (C) in the pituitary and GHR (D), JAK2 (E), and STAT3 genes (F) in the ovary of prepubertal gilts. The mRNA level of genes were determined by qRT-PCR. Bars represent the means ± standard deviation (SD) (n = 12). Above the bar no letter or the same letter mean no significant (P > 0.05), while with different letter mean significant difference (P < 0.05). Growth hormone-releasing hormone (GHRH), Growth hormone-releasing hormone receptor (GHRHR), Growth hormone (GH), Growth hormone receptor (GHR), Janus activated kinase 2 (JAK2), Signal transducer and activator of transcriptions 3 (STAT3).
Relative expression level of GHR, JAK2, and STAT3 mRNA in ovary
The T2 group of prepubertal gilts had significantly higher relative expression levels of GHR mRNA than the CON and T1 groups (P < 0.05). In the T2 group, the relative expression levels of JAK2 mRNA were significantly high at P < 0.05. In the T3 group, the relative expression levels of JAK2 and STAT3 mRNA were significantly high at P < 0.05 (Figures 3D–F).
GHR immunoreactive distribution in the prepubertal gilts ovary
The average optical density of GHR ovarian tissue in T2 experimental group was significantly higher than that of the CON group and T1 experimental group at P < 0.05 (Figure 4A).The immunoreactive substances of GHR were mainly distributed in the ovarian follicles granulosa cells, cytoplasm, and oocytes interstitial cells, while a few were distributed in the theca follicles (Figures 4B–E). The positive cells were either yellow or brownish-yellow. Besides, ZEN had no effect on the GHR distribution and location in the prepubertal gilts ovaries. Compared with the CON group, the T1 experimental group follicle development was accelerated, leading to follicle expansion and increased size. In this study, ZEN increased the ovarian granulosa cell numbers and the immune intensity. The multiple secondary follicles developed simultaneously in the T2 and T3 experimental groups, leading to expansion and increased follicular volume. The number of ovarian granulosa cells further increased, resulting in increased immunoreactive substances. These results show that ZEN promotes ovary and follicle development by increasing the distribution of GHR immunoreactive substances in ovaries and enhances the immunoreactive intensity of the prepubertal gilts.
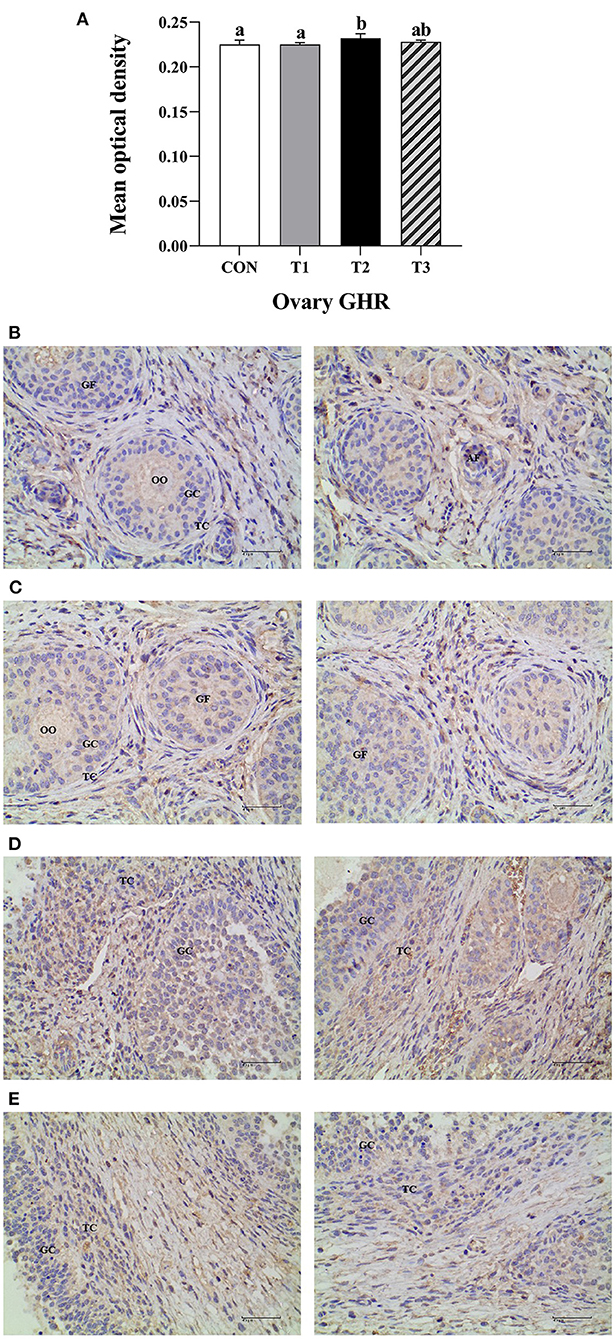
Figure 4. Effects of ZEN on the GHR immunoreactive distribution in the prepubertal gilts ovary. Above the bar no letter or the same letter mean no significant (P > 0.05), while with different letter mean significant difference (P < 0.05) (n = 12) (A). Visual fields of ovarian tissues obtained from the CON (B), T1 (C), T2 (D), and T3 (E) experimental groups. All the images were taken at X400 magnification. Key: Primordial follicle (PF), Growing follicle (GF), Atresia follicle (AF), Oocyte (OO), Granulosa cell (GC), Theca cell (TC). The scale bar represents 40 μm.
Discussion
Comparison of CON group with experimental groups in this experiment showed that the ovarian index in T2 and T3 experimental groups tended to increase while the number of primordial follicles decreased. Besides, multiple secondary follicles developed simultaneously, with an expansion of the follicles and increased follicular volume, indicating that ZEN promotes the development of the ovarian (follicle) in the prepubertal gilts. There have been reports on ZEN ovarian development promoting effects. One of them is Dai et al. (6), who reported that adding 1.04 mg/kg of ZEN into piglet diet promotes ovarian development. In addition, Fu et al. (18), reported that feeding Tibetan piglets with feeds containing 1.13 mg/kg of ZEN promotes the development of the ovary and uterus. Previously, it has been demonstrated that ZEN promotes the development of porcine ovaries and follicles through different ways, such as activating ERs/GSK-3β-dependent Wnt-1/β-catenin signaling pathway Yang et al. (7), promoting autocrine pathway in piglet ovaries, and regulating proliferating cell nuclear antigen (PCNA) gene expression (6). Moreover, offspring exposure to ZEN through breast milk affects the development of offspring ovaries (8). This experiment mainly investigated whether ZEN could affect ovarian development through GHRH, GH, and GHR.
Additionally, GHRH is a peptide hormone mainly synthesized by the hypothalamus arcuate nucleus and ventromedial nucleus. This hormone combines with GHRHR in the anterior pituitary and regulates the synthesis and secretion of GH through the cyclic adenosine monophosphate (cAMP) signaling pathway (9). In all the experimental groups, there was no significant difference in GHRH serum content, hypothalamic GHRH content, pituitary GH content, hypothalamic GHRH relative expression levels, and pituitary GHRHR mRNA. ZEN had no significant effect on the GH content of the gilts serum Wu et al. (19), in line with our previous findings. This implied that ZEN had no significant impact on GH production and secretion in pituitary of prepubertal gilts. Also, Olivares et al. (20) found that zeranol had no significant effect on GH content in lamb serum. However, Thomas et al. (21) reported that zeranol promotes GH secretion through the expression of Pit-1, GHRHR, and GH mRNA in sheep pituitary. The results from this experiment are contrary to those reported by Thomas et al. (21), and this is attributed to the differences in the experimental materials and experimental animals. Different animals have different sensitivity to ZEN and its metabolites (1).
The GH exerts its function at the tissue and cell level, where it binds with GHR on the target cell membrane surface and dimerizes the GHR. After activation of JAK2, the intracellular structure of GHR and its tyrosine residues are phosphorylated. In addition, the STAT3 is activated, and the phosphorylation of protein factor is induced, and it generates a cascade amplification effect. As a result, the biological signals are transmitted to trigger biological effects in the cells and regulate the growth and physiological function of the ovarian tissue (22, 23). In this experiment, there was a significant increase in relative expression levels of GHR and JAK2 mRNA in the T2 experimental group and JAK2 and STAT3 mRNA in the T3 experimental group. Despite the fact that there was no significant difference in the distribution of GHR immunoreactive substances in ovaries of prepubertal gilts in each group, the immunoreactive intensity of GHR in the experimental group presented an increasing trend. In the T2 experimental group, the average optical density of GHR in prepubertal gilts ovaries increased significantly, suggesting that ZEN had no direct effect on the production and secretion of pituitary GH. However, ZEN increases the relative expression levels of JAK2 and STAT3 mRNA by improving the expression levels of GHR mRNA and protein in the ovaries and activating the downstream JAK-STAT signaling pathway, thus enhances the biological effects of GH and promotes the development of the ovaries (follicles). Song et al. (24) reported that adding 1.0 mg/kg of ZEN in the piglet diet up-regulates GHR mRNA and protein expression levels of the piglet ovaries. The content and function of GHR in tissues affects the biological effects of GH. For example, the mutation of the GHR gene reduces the biological effects of GH in boys, which leads to short stature in boys (25). Moreover, Feng et al. (26) reported that GH promotes the proliferation of human endometrial glandular cells through the GHR-JAK2-STAT3 pathway. Application of GHR inhibitor AG490 down-regulates the expression of GHR, JAK2, and STAT3 genes, hence reducing the GH biological effects on cell proliferation rate.
In this experiment, the number of atretic follicles in the experimental group was higher than in the CON group. In T2 and T3 experimental groups, the follicles had necrotic and exfoliated granulosa cells with either contracted or fragmented nuclei, which may be associated with the toxic effects of the oxidative stress Xu et al. (27), inflammatory reaction Zhang et al. (28) and autophagy caused by ZEN (29). Abnormal development of ovaries (follicles) and pathological changes in the ovaries increases risks to ovarian hypoplasia, premature aging, and abnormality in ovary functions hence leads to decreased reproductive performance in gilts (30, 31).
Conclusions
Based on this study, ZEN has no significant effect on the production and secretion of pituitary GH in prepubertal gilts. However, ZEN enhances GH biological effects, promotes the development of the ovary (follicle), and exerts reproductive toxicity by increasing the ovary GHR, JAK2, and STAT3 mRNA expression level and improves the immune intensity of the GHR protein.
Data availability statement
The original contributions presented in the study are included in the article/supplementary material, further inquiries can be directed to the corresponding author.
Ethics statement
The animal study was reviewed and approved by the Animal Care and Use Committee of Hebei Agriculture University (Baoding, China). All animal experiments complied with the ARRIVE guidelines were carried out in accordance with the U.K. Animals (Scientific Procedures) Act, 1986 and associated guide-lines, EU Directive 2010/63/EU for animal experiments.
Author contributions
FW, LG, FL, SC, and BC designed the experiment and wrote the manuscript. FW, LG, and FL performed the statistical analyses. FW, LG, and JC carried out the animal experiment. FW, XY, and YL conducted the sample collection. FW, LG, and FL carried out the sample analysis. All authors contributed to the article and approved the submitted version.
Funding
This study was supported by Key Research and Development Program of Hebei Province (20326613D), Research Development Fund of Hebei Agricultural University (3003003) and Key Research and Development Program of China (S2016G4513).
Conflict of interest
The authors declare that the research was conducted in the absence of any commercial or financial relationships that could be construed as a potential conflict of interest.
Publisher's note
All claims expressed in this article are solely those of the authors and do not necessarily represent those of their affiliated organizations, or those of the publisher, the editors and the reviewers. Any product that may be evaluated in this article, or claim that may be made by its manufacturer, is not guaranteed or endorsed by the publisher.
References
1. Rai A, Das M, Tripathi A. Occurrence and toxicity of a fusarium mycotoxin, zearalenone. Crit Rev Food Sci Nutr. (2020) 60:2710–29. doi: 10.1080/10408398.2019.1655388
2. Cortinovis C, Pizzo F, Spicer LJ, Caloni F. Fusarium mycotoxins: effects on reproductive function in domestic animals–a review. Theriogenology. (2013) 80:557–64. doi: 10.1016/j.theriogenology.2013.06.018
3. Marin DE, Taranu I, Burlacu R, Manda G, Motiu M, Neagoe I, et al. Effects of zearalenone and its derivatives on porcine immune response. Toxicol In Vitro. (2011) 25:1981–8. doi: 10.1016/j.tiv.2011.06.022
4. Gruber-Dorninger C, Jenkins T, Schatzmayr G. Global mycotoxin occurrence in feed: a ten-year survey. Toxins (Basel). (2019) 11:375. doi: 10.3390/toxins11070375
5. Hu HY, Jia Q, Zhou B, Zhang J, Li ZQ, Liu ZW. Comparative analysis of the ovarian transcriptome reveals novel insights into fertility differences in Large White sows. Genes Genomics. (2020) 42:715–25. doi: 10.1007/s13258-020-00926-8
6. Dai ML, Jiang SZ, Yuan XJ, Yang WR, Yang ZB, Huang LB. Effects of zearalenone-diet on expression of ghrelin and PCNA genes in ovaries of post-weaning piglets. Anim Reprod Sci. (2016) 168:126–37. doi: 10.1016/j.anireprosci.2016.03.006
7. Yang LJ, Zhou M, Huang LB, Yang WR, Yang ZB, Jiang SZ, et al. Zearalenone-promoted follicle growth through modulation of wnt-1/β-catenin signaling pathway and expression of estrogen receptor genes in ovaries of postweaning piglets. J Agric Food Chem. (2018) 66:7899–906. doi: 10.1021/acs.jafc.8b02101
8. Kong L, Zhao AH, Wang QW, Feng YQ, Yan ZH, Li MH, et al. Maternal Zearalenone exposure impacted ovarian follicle formation and development of suckled offspring. Sci Total Environ. (2021) 788:147792. doi: 10.1016/j.scitotenv.2021.147792
9. Zhou F, Zhang H, Cong Z, Zhao LH, Zhou Q, Mao C, et al. Structural basis for activation of the growth hormone-releasing hormone receptor. Nat Commun. (2020) 11:5205. doi: 10.1038/s41467-020-18945-0
10. Hrabia A, Paczoska-Eliasiewicz HE, Berghman LR, Harvey S, Rzasa J. Expression and localization of growth hormone and its receptors in the chicken ovary during sexual maturation. Cell Tissue Res. (2008) 332:317–28. doi: 10.1007/s00441-008-0595-7
11. Zhou M, Yang LJ, Yang WR, Huang LB, Zhou XM, Jiang SZ, et al. Effects of zearalenone on the localization and expression of the growth hormone receptor gene in the uteri of post-weaning piglets. Asian-Australas J Anim Sci. (2018) 31:32–9. doi: 10.5713/ajas.17.0526
12. Ford EA, Beckett EL, Roman SD, McLaughlin EA, Sutherland JM. Advances in human primordial follicle activation and premature ovarian insufficiency. Reproduction. (2020) 159:15–29. doi: 10.1530/REP-19-0201
13. Foerster C, Muñoz K, Delgado-Rivera L, Rivera A, Cortés S, Müller A, et al. Occurrence of relevant mycotoxins in food commodities consumed in Chile. Mycotoxin Res. (2020) 36:63–72. doi: 10.1007/s12550-019-00369-5
14. Ojuri OT, Ezekiel CN, Sulyok M, Ezeokoli OT, Oyedele OA, Ayeni KI, et al. Assessing the mycotoxicological risk from consumption of complementary foods by infants and young children in Nigeria. Food Chem Toxicol. (2018) 121:37–50. doi: 10.1016/j.fct.2018.08.025
15. Rogowska A, Pomastowski P, Sagandykova G, Buszewski B. Zearalenone and its metabolites: Effect on human health, metabolism and neutralisation methods. Toxicon. (2019) 162:46–56. doi: 10.1016/j.toxicon.2019.03.004
16. Asci A, Durmaz E, Erkekoglu P, Pasli D, Bircan I, Kocer-Gumusel B. Urinary zearalenone levels in girls with premature thelarche and idiopathic central precocious puberty. Minerva Pediatr. (2014) 66:571–8. doi: 10.1121/1.3073734
17. Schoevers EJ, Santos RR, Colenbrander B, Fink-Gremmels J, Roelen BA. Transgenerational toxicity of Zearalenone in pigs. Reprod Toxicol. (2012) 34:110–9. doi: 10.1016/j.reprotox.2012.03.004
18. Fu GH, Wang LH, Li L, Liu JR, Liu SZ, Zhao X. Bacillus licheniformis CK1 alleviates the toxic effects of zearalenone in feed on weaned female Tibetan piglets. J Anim Sci. (2018) 96:4471–80. doi: 10.1093/jas/sky301
19. Wu FY, Cu J, Yang XY, Chen BJ. Effects of zearalenone on uterus development, growth hormone secretion and its receptor distribution and expression in prepubertal gilts. Acta Zoonutrimenta Sinica. (2021) 33:217–26. doi: 10.3969/j.issn.1006-267x.2021.01.023
20. Olivares VH, Hallford DM. Growth and carcass characteristics and serum growth hormone, prolactin and insulin profiles in Debouillet lambs treated with ovine growth hormone and(or) zeranol. J Anim Sci. (1990) 68:1971–9. doi: 10.2527/1990.6871971x
21. Thomas MG, Carroll JA, Raymond SR, Matteri RL, Keisler DH. Transcriptional regulation of pituitary synthesis and secretion of growth hormone in growing wethers and the influence of zeranol on these mechanisms. Domest Anim Endocrinol. (2000) 18:309–24. doi: 10.1016/S0739-7240(00)00052-7
22. Devesa J, Caicedo D. The role of growth hormone on ovarian functioning and ovarian angiogenesis. Front Endocrinol (Lausanne). (2019) 10:450. doi: 10.3389/fendo.2019.00450
23. Dehkhoda F, Lee CMM, Medina J, Brooks AJ. The growth hormone receptor: mechanism of receptor activation, cell signaling, and physiological aspects. Front Endocrinol (Lausanne). (2018) 9:35. doi: 10.3389/fendo.2018.00035
24. Song TT, Liu XF, Yuan XJ, Yang WR, Liu FX, Hou YM, et al. Dose-effect of zearalenone on the localization and expression of growth hormone, growth hormone receptor, and heat shock protein 70 in the ovaries of post-weaning gilts. Front Vet Sci. (2021) 8:629006. doi: 10.3389/fvets.2021.629006
25. Rughani A, Zhang DS, Vairamani K, Dauber A, Hwa V, Krishnan S. Severe growth failure associated with a novel heterozygous nonsense mutation in the GHR transmembrane domain leading to elevated growth hormone binding protein. Clin Endocrinol (Oxf). (2020) 92:331–7. doi: 10.1111/cen.14148
26. Feng Q, Gao B, Huang H, Zou LX, Zhao XP, Zhu XT, et al. Growth hormone promotes human endometrial glandular cells proliferation and motion through the GHR-STAT3/5 pathway. Ann Transl Med. (2020) 8:53. doi: 10.21037/atm.2019.12.08
27. Xu Y, Sun MH, Li XH, Ju JQ, Chen LY, Sun YR, et al. Modified hydrated sodium calcium aluminosilicate-supplemented diet protects porcine oocyte quality from zearalenone toxicity. Environ Mol Mutagen. (2021) 62:124–32. doi: 10.1002/em.22399
28. Zhang FL, Kong L, Zhao AH, et al. Inflammatory cytokines as key players of apoptosis induced by environmental estrogens in the ovary. Environ Res. (2021) 198:111225. doi: 10.1016/j.envres.2021.111225
29. Zhu YF, Wang H, Wang JP, Han SS, Zhang Y, Ma MG, et al. Zearalenone induces apoptosis and cytoprotective autophagy in chicken granulosa cells by PI3K-AKT-mTOR and MAPK signaling pathways. Toxins (Basel). (2021) 13:199. doi: 10.3390/toxins13030199
30. Wang L, Tang JH, Wang L, Tan F, Song HB, Zhou JW, et al. Oxidative stress in oocyte aging and female reproduction. J Cell Physiol. (2021) 236:7966–83. doi: 10.1002/jcp.30468
Keywords: zearalenone, prepubertal gilts, ovary, growth hormone-releasing hormone, growth hormone, growth hormone receptor
Citation: Wu F, Gao L, Li F, Cui J, Yang X, Liu Y, Chen S and Chen B (2022) Effects of zearalenone on ovarian development of prepubertal gilts through growth hormone axis. Front. Vet. Sci. 9:950063. doi: 10.3389/fvets.2022.950063
Received: 22 May 2022; Accepted: 14 July 2022;
Published: 03 August 2022.
Edited by:
Pierre Comizzoli, Smithsonian Conservation Biology Institute (SI), United StatesReviewed by:
Padet Tummaruk, Chulalongkorn University, ThailandDaniela Bebbere, University of Sassari, Italy
Copyright © 2022 Wu, Gao, Li, Cui, Yang, Liu, Chen and Chen. This is an open-access article distributed under the terms of the Creative Commons Attribution License (CC BY). The use, distribution or reproduction in other forums is permitted, provided the original author(s) and the copyright owner(s) are credited and that the original publication in this journal is cited, in accordance with accepted academic practice. No use, distribution or reproduction is permitted which does not comply with these terms.
*Correspondence: Baojiang Chen, Y2hlbmJhb2ppYW5nJiN4MDAwNDA7dmlwLnNpbmEuY29t; Saijuan Chen, Y2hlbnNhaWp1YW4mI3gwMDA0MDsxMjYuY29t
†These authors have contributed equally to this work