- 1College of Animal Science and Veterinary Medicine, Jinzhou Medical University, Jinzhou, China
- 2Department of Animal Resources Science, Dankook University, Cheonan, South Korea
- 3College of Animal Science and Technology, Jilin Agricultural University, Changchun, China
We investigated the effects of in ovo injection of methionine (Met) and/or disaccharide (DS) on breast muscle and small intestine development, and the aspect of the glycogen contents, digestive enzymes activities, and jejunal antioxidant parameters in geese after incubation. A total of 600 fertilized eggs were used in this study to be employed in a 2 × 2 factorial experiment. Eggs were randomly assigned to 4 groups, 6 replicates per group, and 25 eggs per replicate. Factors in four groups included non-injection, Met injection (5 g/L Met dissolved in 7.5 g/L NaCl), DS injection (25 g/L maltose and 25 g/L sucrose dissolved in 7.5 g/L NaCl), and DS plus Met injection (25 g/L maltose, 25 g/L sucrose, and 5 g/L Met dissolved in 7.5 g/L NaCl). As a result, birth weight, relative weight of breast muscle, diameter of myofiber, glycogen contents, jejunal villus and surface area, and jejunal digestive enzymes activities improved, while liver glucose-6-phosphatase activity decreased, by DS injection. Additionally, DS administration upregulated the expression of myogenic factor-5 (Myf-5) from breast muscle and sodium/glucose cotransporter protein-1 (SGLT-1) from jejunum. In ovo delivery of DS has long-term effects on the improvement of jejunal glucose transporter-2 (GLUT-2) and sucrase-isomaltase expression. In ovo feeding of Met improved the relative weight of breast muscle and small intestine, diameter of myofiber, length of small intestine, jejunal villus width, jejunal sucrase, Na+/K+ATPase and alkaline phosphatase activities, and jejunal glutathione (GSH) concentration, and decreased the jejunal glutathione disulfide (GSSH) and the ratio of GSSG to GSH, in early-life post-hatching. The breast muscle Myf-5 and myostatin expression, jejunal villus height and surface area, jejunal glutathione peroxidase concentration, and the expression of GLUT-2 in jejunum long-term improved by in ovo delivery of Met. Moreover, in ovo feeding of DS plus Met mixture synergistically improved the diameter of myofiber, jejunal villus height and width, jejunal sucrase, and alkaline phosphatase activities in early-life post-hatching, but long-term upregulated the expression of jejunal GLUT-2. Therefore, we concluded that in ovo injection of Met plus DS is an effective way to improve the development of gosling during post-hatching stages.
Introduction
Geese are seasonal egg producers, its eggs are mainly used for eating, reproduction, and research purposes. It becomes more important to improve gosling quality during post-hatching stages in commercial geese farming. In modern gosling hatcheries, birds are always taken out of the incubator at the same time, which means premature birds have to wait for other late-born birds to ensure maximum hatching over the same period. Additionally, commercially hatched gosling faces entirely different post-natal environments in comparison with geese in natural brooding; they could not have immediate access to feed and water. A series of operations such as packaging and shipping also inevitably delays the access to feed and water. Therefore, gosling faces a fasting challenge during its initial crucial period of life.
The vigorous growth and metabolism activities during the fasting periods lead to an insufficient supply of energy and protein and the risk of oxidative stress, resulting in retarded growth, limited gut development, and stunted breast muscle (1–4). The in ovo injection approach provides a method in overcoming these problems and filling the gap between the hatch and first feed access (5).
Amnion has been proven to be an effective site for implementing in ovo injection techniques (6). The embryo orally consumes the amniotic fluid before pipping the air cell under natural conditions, thus consuming the supplemented nutrients which are presented in the enteric tissues (7). This presents an opportunity to inject nutrients into the amnion to uplift the status of growth and development in the embryo (8).
Digestible disaccharides (DS) are possible candidates for exogenous energy provision, it plays an important glucose precursor for late-term bird embryo catabolism and could alleviate energy deficiency by stimulating glucose anabolism (9, 10). Methionine (Met) as the first limiting amino acid for birds has been reported to decrease oxidative stress and uplift the protein supply (11, 12). To our knowledge, studies on the effects of in ovo injection of DS and/or Met on the performance in geese were still limited. In our previous study, in ovo injection of DS and/or Met had positive effects on the development of the embryo of goslings (13). We hypothesized that the administration of DS and/or Met into the amnion of the late-term embryo may serve as a tool to provide energy for small intestine and embryo activity, in turn alleviating energy and protein lack, and improving breast muscle and intestine development in geese during post-hatching periods. To test this hypothesis, we conducted this study to investigate the effects of in ovo injection of DS and/or Met on the post-hatching breast muscle parameters, glycogen reserves, glucose-6-phosphatase (G-6-Pase) activity, myogenic factor-5 (Myf-5) and myostatin (MSTN) expression in breast muscle, small intestine parameters, jejunum morphology, jejunum digestive enzymes activities, jejunum antioxidant indices, jejunum sodium/glucose cotransporter protein-1 (SGLT-1), glucose transporter-2 (GLUT-2), and sucrase-isomaltase (SI) expression.
Materials and methods
Experimental design, animals, and housing
The fertilized eggs (in total 1,000) were purchased from the Dekun Poultry Food Co., Ltd (Meihekou, Jilin). The breed of the egg was Jilin White geese. All eggs were laid on the same day, represented in the same weight class. Fertilized geese eggs were incubated in the standard condition in an incubator (Keyu CFZ microcomputer automatic incubator, Dezhou, Shandong). Before transiting to the incubator, eggs were pre-heated to 30°C for 12 h, disinfected with 37% formaldehyde and potassium permanganate (2:1), and distributed into incubator tray levels. The incubation period included three phases (phase 1, days 1–14; phase 2, days 15–28, and phase 3, days 29–31). During phase 1, the temperature was 38°C and the humidity was 65%; during phase 2, the temperature was 37.5°C and the humidity was 55%; and during phase 3, the temperature was 37.2°C and the humidity was 70%. All eggs were turned once per 2 h for 180 s.
Eggs were candled checking for containing embryonated eggs on day 23 of incubation. A total of 600 fertilized eggs were selected from the embryonated eggs obtained above and randomly assigned into 4 groups with 6 replicates per group and 25 eggs per replicate. This experiment was a two-factor design, which included DS injection or Met injection. Eggs treatment in four groups was divided into non-injection, Met injection (5 g/L Met dissolved in 7.5 g/L NaCl), DS injection (25 g/L maltose and 25 g/L sucrose dissolved in 7.5 g/L NaCl), and DS plus Met injection (25 g/L maltose, 25 g/L sucrose, and 5 g/L Met dissolved in 7.5 g/L NaCl). Injection solutions and paraffin were prepared on the day of injection (day 24 of incubation) and were treated at 121°C for 15 min. The injection was conducted when the solutions and paraffin are cooled down to room temperature.
A 70% of ethanol was used for egg surface sterilization. Cleaned eggs were put onto a holder with the large end on top. The position of amnion was identified by candling. The upper side of the eggs (the air space) was pierced by an egg-shell punch. Injections were performed with a disinfected injector. About 1.5 mL of each solution was injected into the amnion of each egg to a depth of 20 mm, without hurting the embryo. After each inoculation, the needle was routinely disinfected to minimize the risk of infection. All eggs were held outside the incubator for <5 min while injecting, including the non-injected control eggs. Immediately after the injection, the hole was sealed using paraffin, and the eggs were returned to the incubator and incubated in line with the routine procedure until hatched.
After hatching, birds were transported to a temperature-controlled room with continuous lighting and distributed into cages according to the replicates. Feeds were provided immediately after transportation. The raising period was until day 28 of age (Table 1). Uniform management was conducted during this period. The temperature of the room was maintained at 30°C during the first 3 days and then reduced by 2°C per week.
The Animal Care and Use Committee of Jilin Agricultural University (Changchun, China) supervised the procedure of this experiment.
Feed analysis
After homogeneous mixing, feed samples were collected from each dietary group. All feed samples were dried in a 70°C constant temperature oven for 72 h. Subsequently, feed samples were ground and sieved with a 1-mm sieve. The collected feed powder is with a diameter of <1 mm for feed composition analysis. According to the procedure established by the AOAC (14), the dry matter (method 930.15), crude protein (nitrogen × 6.25; method 968.06), and crude fiber (method 991.43) composition in the diet were analyzed. Then, the representative feed samples in each group were hydrolyzed with 6 N HCl for 24 h at 110°C. An amino acid analyzer (2690 Alliance, Waters, Inc., Milford, MA) was used for determining amino acid contents in the diet. In addition, the contents of neutral detergent fiber and acid detergent fiber in the diet were measured according to the method provided by Mertens (15).
Sample collection
On hatching day, day 7 post-hatching, and day 28 post-hatching, three birds were randomly selected from each replicate group, weighed, and slaughtered by cervical dislocation for further analysis.
The liver was removed from the carcass and the adherent material of the liver was carefully removed under ice-cold saline. About 100 mg of liver samples were taken and homogenized in 0.25 mol l−1 sucrose solution for G-6-Pase activity analysis. The remaining sample of the liver was frozen as aliquots in liquid nitrogen, and stored at −80°C for measuring the liver glycogen contents.
Both sides of breast muscle were stripped after being slaughtered. One side of the breast muscle sample was weighed and fixed with 10% neutral-buffered formalin for analyzing myofiber traits. Another side of the breast muscle sample was frozen as aliquots in liquid nitrogen, and stored at −80°C for measuring breast muscle glycogen contents and breast muscle development-related gene expression situation.
The whole small intestine was removed and the adherent material of the small intestine was carefully removed under ice-cold saline, weighed, and separated into the duodenum, jejunum, and ileum. The duodenum was defined as the portion of the small intestine composed of the duodenal loop; the jejunum was considered as the part between the duodenum and ileum; and the ileum as a distal segment before ileo-cecal junction equal to the length of the cecum. The jejunum sample was used for measuring antioxidant parameters first, and then about 1-cm long segment from the middle of the jejunum was taken in duplicate and placed in two separate tubes. One sample was fixed with 10% neutral-buffered formalin solution for histology and the other sample was frozen in liquid nitrogen, and then stored at −80°C for measuring digestive enzyme activities and nutrients transport gene expression.
Experimental parameters measurement
Growth performance parameters analysis
The body weight of goslings was checked after incubation to calculate the birth weight and correspondingly calculated the mortality rate.
Breast muscle and small intestine parameters analysis
The relative weight of breast muscle and small intestine were calculated using the following equation:
The length of the small intestine was measured by a dividing ruler.
Myofiber traits analysis
After slaughtering the geese, the breast muscle samples were cut into small pieces and fixed with 10% neutral buffered formalin for 12 h, followed by dehydration in increasing concentrations of alcohol (70, 80, 90, 95, and 100%) and xylene. Consequently, samples were embedded in paraffin and stored in an oven at 60°C. About 12 h later, samples were removed from the oven and histological cassettes. Fragments were placed in “paper boxes” and covered with paraffin. After the paraffin solidified into blocks, the “papers” were removed and the blocks were kept under refrigeration until the cuts were realized (16).
Serial tissue sections (3 μm thickness) were excised perpendicular to the direction of the myofibers using a cryostat. After sectioning, put the paraffin section ribbon on the coating slide glass. Dried slides were kept in an oven at 60°C for 2 h to eliminate any excess paraffin. The next step consisted of paraffin removal and slide hydration, using xylene, and different concentrations of ethanol. Samples were then stained following the hematoxylin and eosin staining protocol (16).
Samples were then dehydrated again and mounted. In each specimen, the diameters of muscle fibers were measured under a light microscope equipped with a ScopePhpto (LY-WN 300, Hangzhou Scopetek Opto-Eletric Co., Ltd.).
No less than 150 intact, well-oriented muscle fibers cross-sectional area of five fields of vision were measured under 40 times the objective lens. With muscle fibers assumed to be round, the muscle fiber cross-sectional area (A) was converted to diameter (D) by the formula, D = 2√A/π. The average value was calculated to represent the diameter of the muscle fibers (17).
Glycogen reserves analysis
About 0.1 grams sample of liver and breast muscle were stored at 1 ml of 8% HClO4, homogenized (in ice) for 45 s, and centrifuged at 7,700 rpm at 4°C for 16 min. A 10-μl aliquot of the supernatant was transferred to a clean polypropylene tube, along with 0.4 ml of 8% perchloric acid and 2.6 ml of iodine color reagent made of 1.3 ml of solution A (0.26 g iodine + 2.6 g potassium iodide dissolved in 10 ml of distilled water) in 100 ml of 67.8% saturated calcium chloride. All samples were read at a wavelength of 450 nm. The amount of glycogen present in the sample solution was determined by the preparation of a known glycogen standard curve.
G-6-Pase activity analysis
Livers were taken to a total weight of 100 mg and homogenized in 0.25 mol l−1 sucrose solution for analyzing G-6-Pase activity. G-6-Pase was assayed (in 20 mmol l−1 Tris–HCl, pH 7.3 for 10 min at 37°C) by complex formation of inorganic phosphate (Pi) liberated from G-6-Pase (0.1 mol l−1) and subtracting the amount of Pi produced from para-nitrophenylphosphate (20 mmol l−1) under the same conditions of the assay. The protein concentration in liver homogenization was assayed using the method described by Bradford (18). G-6-Pase activity was expressed in micromoles of released inorganic phosphorus per min per milligram protein.
Muscle growth-related gene expression analysis
Total RNA was isolated from muscle samples using RNAiso Reagent (TaKaRa, Dalian, Liaoning, China). The RNA integrity was assessed by electrophoresis on a 1% agarose gel containing formaldehyde. The RNA concentration was measured using a Beckman DU-640 spectrophotometer (Beckman). The sequences of primers for the genes tested were specifically designed according to the sequences located in GenBank (Table 2). The total RNA samples were purified and subjected to reverse transcription using the Takara PrimeScript RT Reagent Kit with gDNA Eraser (Takara, Dalian, China) and processed for cDNA synthesis as per Takara PrimeScript RT instructions (19).
The relative expression levels of Myf-5 and MSTN genes in pectoral muscle were analyzed by RT-PCR, which was performed in a 10-μL reaction mix containing 1 μL 2 × SYBR Premix Ex Taq II (TakaRa, Dalian, China), 3 μL dH2O, 0.5 μL of the upstream and downstream primers, and 1 μL cDNA using a Bio-Rad CFX-96 thermocycler (Bio-Rad, CA). The reaction conditions were as follows: initial denaturation at 95°C for 30 s and 44 cycles of amplification at 72°C for 30 s. The annealing was carried out for 40 s at temperatures specific to each target gene. At the end of the amplification, step-wise melting curves were performed to confirm the product specificity. The cytoskeletal protein, β-actin, was used as the internal reference (20). All the reactions were performed in triplicate. The qRT-PCR data were analyzed using the 2−ΔΔCt method. The relative level of each mRNA normalized to the ΔCt (β-actin) gene was calculated using the following equation:
Jejunum morphology analysis
The jejunum samples were cut into small pieces and further treated by fix and dehydration according to the methods described above to prepare paraffin blocks.
A microtome was used to make five cuts that were 5 μm. The cuts were stained with hematoxylin-eosin. The values were measured using a light microscope. Measurements of villus height and width were determined at a magnification of 10X. A minimum of five measurements per slide were made for each parameter and averaged into one value (21). Villus surface area was calculated from the villus height (from the tip of the villi to the villus crypt junction) and width at half height (22). Values presented means from 10 adjacent villi and only vertically oriented villi were measured.
Digestive enzymes activities analysis
Enzyme activities were assayed in homogenized jejunum tissue. Samples were thawed at 4°C and homogenized in 10 times the volume of cold normal saline. The homogenates were then centrifuged at 20,000 ×g for 20 min at 4°C and the supernatant was collected for enzyme assays. Sucrase [Enzyme Commission (EC) 3.2.1.48] and maltase (EC 3.2.1.20) activity were assayed colorimetrically using sucrose and maltose as substrates, respectively. The activity was expressed as micromoles of glucose released per minute per gram of jejunum wet tissue. Alkaline phosphatase (EC 3.1.3.1) activity was determined by measuring the hydrolysis of p-nitrophenol at 37°C, and the unit of activity was expressed as per min per gram of jejunum wet tissue. Na+/K+ATPase (EC 3.6.1.3) activity was determined by measuring the liberation of phosphate from ATP-Na2 (No. A7699, Sigma-Aldrich) in two media: medium I (all ATPases system) and medium II (Na+/K+ATPase restrained system); and the activity of Na+/K+ATPase was calculated as the difference between phosphates liberated by each homogenate in the two media and was expressed as micromoles of phosphates per milligram homogenate protein or per milliliter of serum per h.
Jejunum antioxidant parameters analysis
The activities of glutathione peroxidase (GPX), glutathione (GSH), and glutathione disulfide (GSSG) in the mucose were determined using commercial kits (Nanjing JianCheng Bioengineering Institute, Nanjing, P. R. China) according to the instructions of the manufacturer. All results were normalized against total protein concentration in each sample for inter-sample comparison. The GSSG/GSH ratio was determined according to the data on GSSG and GSH activities in mucose.
Jejunum nutrients transport gene expression analysis
The abundance of SGLT-1, GLUT-2, and SI mRNA isolated from geese jejunum tissues (approximately 50 mg) was analyzed by the above step. The sequences of primers for the genes tested were specifically designed according to the sequences located in GenBank (Table 2). The cytoskeletal protein, β-actin, was used as the internal reference (20).
Statistical analysis
The data were analyzed as a two-way ANOVA factorial arrangement of treatments using the GLM procedure in SPSS18.0 software, with treatment as the fixed effect and the replicate cage as the experimental unit. Factors involved were in ovo injection of DS and/or Met. The data are presented as the means ± standard deviation (SD). Results were considered significant at P < 0.05.
Results
Growth performance parameter
Birth weight was increased by feeding DS into the embryo (P < 0.05). The in ovo injection strategies had no significant effects on the mortality rate (Table 3).

Table 3. Effects of in ovo injection of methionine (Met) and disaccharide (DS) on growth performance parameters of geese during the early-life post-hatchinga, b.
Breast muscle and myofiber trait parameter
In ovo injection of DS increased the relative weight of breast muscle (P < 0.01) and the diameter of myofiber (P < 0.01) on the day of hatching. Met administration increased the relative weight of breast muscle on the day of hatching (P < 0.01) and day 7 post-hatching (P < 0.05) and increased the diameter of myofiber on day 7 post-hatching (P < 0.01). In addition, in ovo delivery of Met plus DS synergistically increased the diameter of myofiber on the day of hatching (P < 0.01) (Table 4).
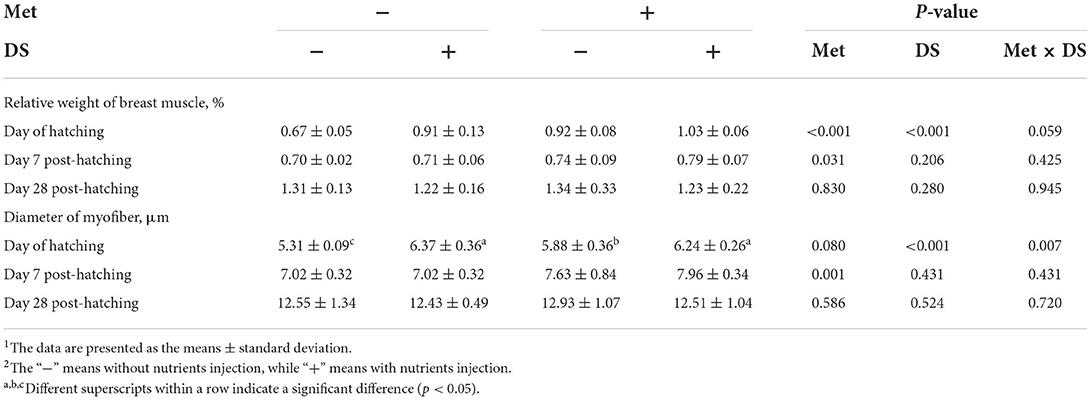
Table 4. Effects of in ovo injection of methionine (Met) and disaccharide (DS) on breast muscle parameters of geese during the early-life post-hatching1, 2.
Glycogen reserves
As shown in Table 5, in ovo injection of Met had no significant effects on the glycogen reserves. However, in ovo feeding of DS increased breast muscle glycogen content on the day of hatching (P < 0.01) and day 7 post-hatching (P < 0.01), liver glycogen content on the day of hatching (P < 0.01), and total glycogen content on the day of hatching (P < 0.01).
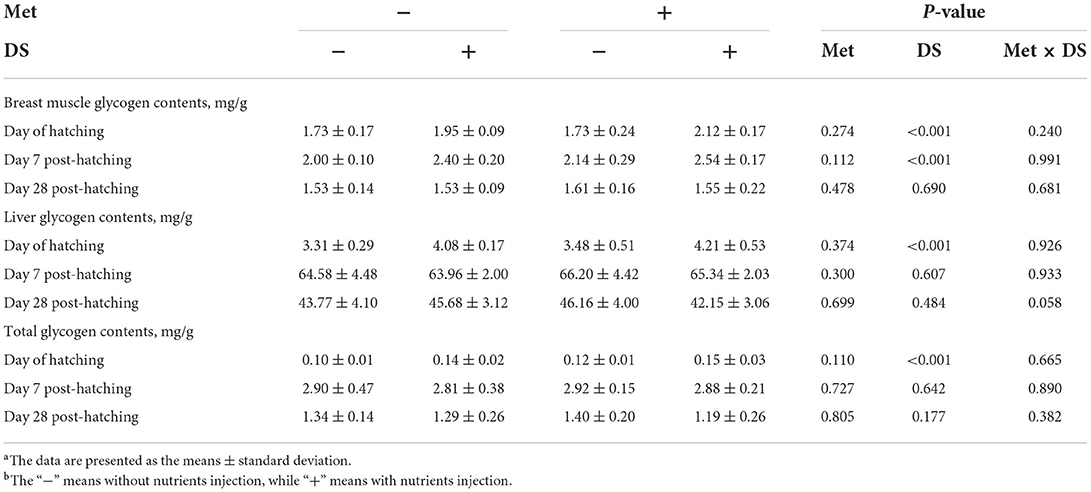
Table 5. Effects of in ovo injection of methionine (Met) and disaccharide (DS) on glycogen reserves of geese during the early-life post-hatchinga, b.
G-6-Pase activity
DS injection decreased the G-6-Pase activity on the day of hatching (P < 0.01). However, Met injection had no significant effects on the G-6-Pase activity (Table 6).
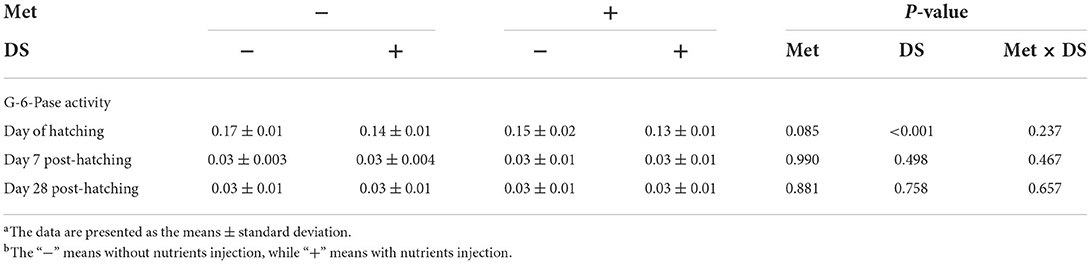
Table 6. Effects of in ovo injection of methionine (Met) and disaccharide (DS) on glucose 6-phosphatase (G-6-Pase) activity of geese during the early-life post-hatchinga, b.
Muscle growth-related gene expression
The expression of Myf-5 from breast muscle was upregulated by DS injection on the day of hatching (P < 0.05). Moreover, Met injection upregulated the Myf-5 and MSTN expression on the day of hatching (P < 0.01), day 7 post-hatching (P < 0.01), and day 28 post-hatching (P < 0.01) (Table 7).
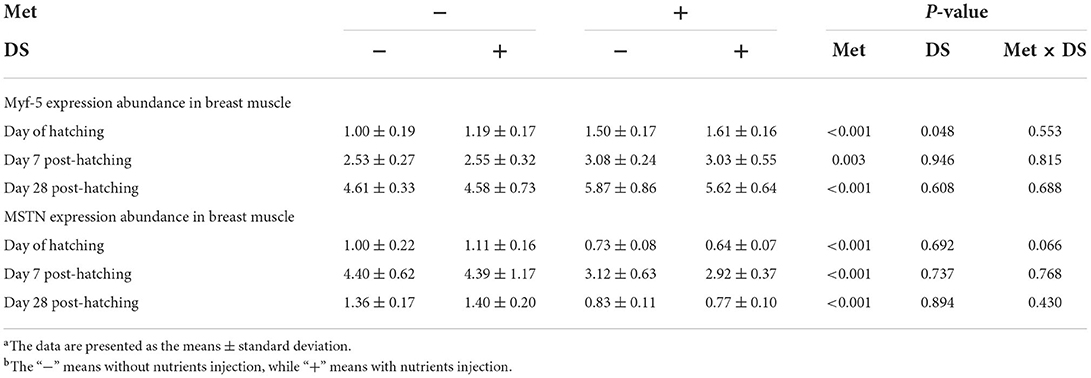
Table 7. Effects of in ovo injection of methionine (Met) and disaccharide (DS) on the expression of myogenic factor-5 (Myf-5) and myostatin (MSTN) from breast muscle of geese during the early-life post-hatchinga, b.
Small intestine parameter
Met injection increased the relative weight of the small intestine on the day of hatching (P < 0.05) and the length of the small intestine on day 7 post-hatching (P < 0.05). However, DS injection had no significant effects on the small intestine parameters (Table 8).
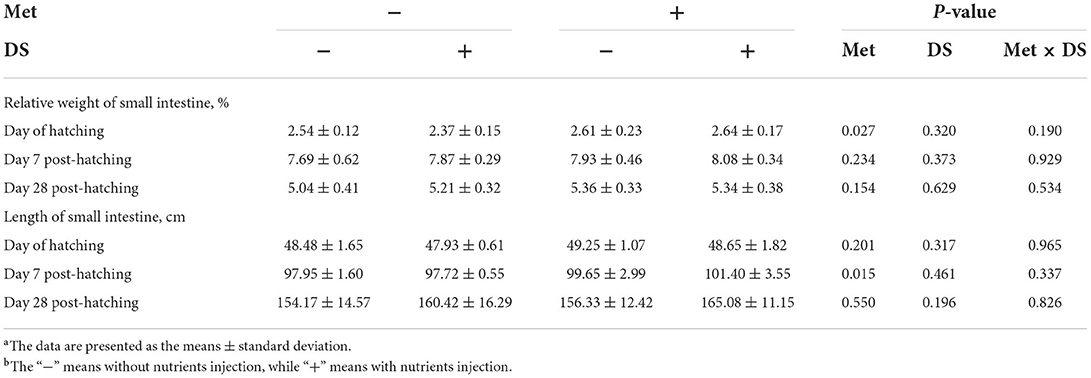
Table 8. Effects of in ovo injection of methionine (Met) and disaccharide (DS) on small intestine parameters of geese during the early-life post-hatchinga, b.
Jejunum morphology
The jejunal villus height (P < 0.01), villus width (P < 0.05), and villus surface area (P < 0.01) on day of hatching, villus height (P < 0.01) on day 7 post-hatching, and villus height (P < 0.01) and villus surface area (P < 0.01) on day 28 post-hatching were increased by in ovo injection of Met. DS injection increased jejunal villus height and villus surface area on the day of hatching (P < 0.01) and day 7 post-hatching (P < 0.01). Additionally, a synergistic effect of DS plus Met injection on the jejunal villus height (P < 0.01) and villus surface area (P < 0.01) was observed on the day of hatching (Table 9; Figures 1–3).
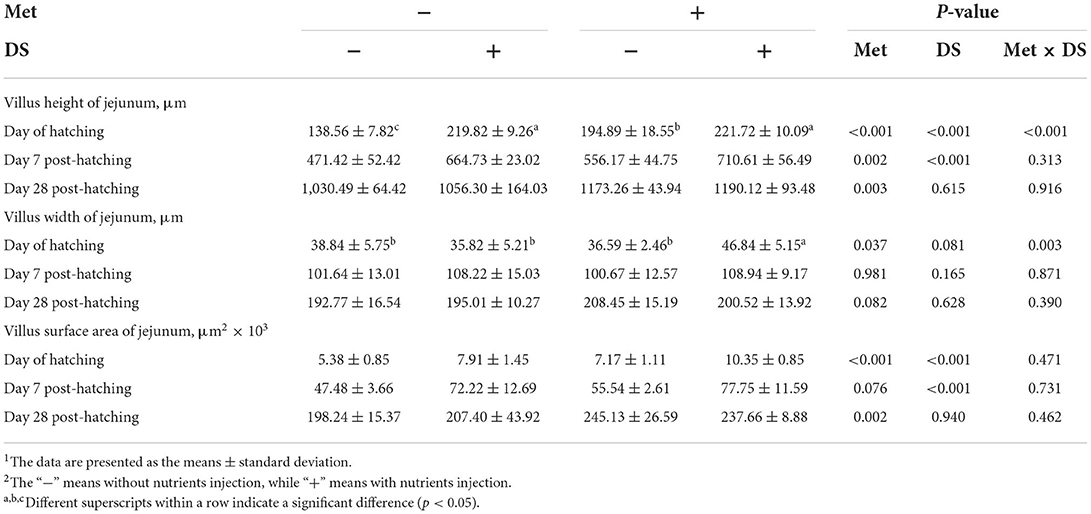
Table 9. Effects of in ovo injection of methionine (Met) and disaccharide (DS) on jejunum parameters of geese during the early-life post-hatching1, 2.
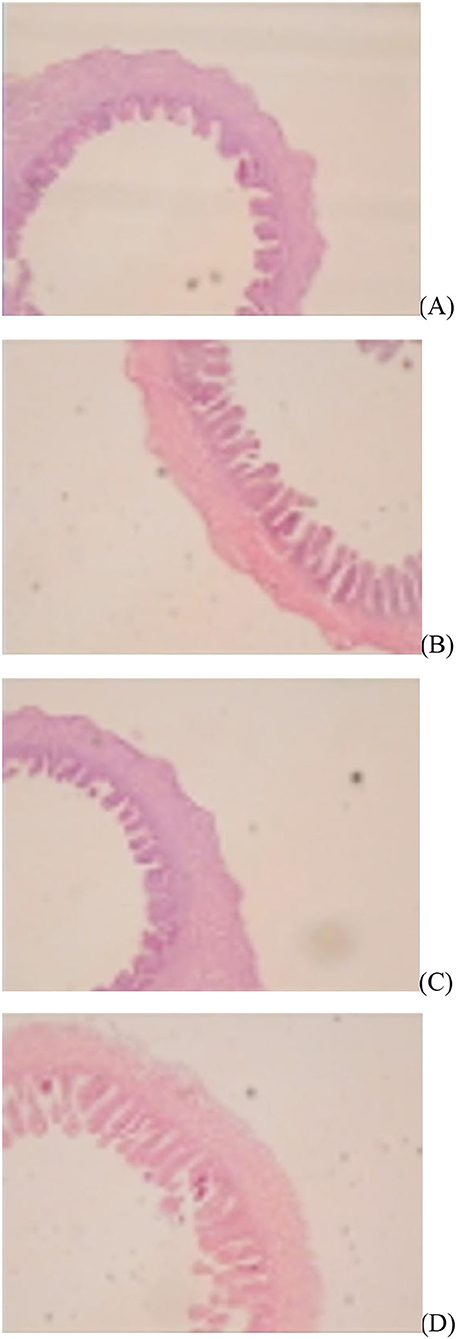
Figure 1. Slice of the jejunum in gosling treated by in ovo injection of methionine (Met) and/or disaccharide (DS) on day of hatching. (A) Non-injection group; (B) DS injection group; (C) Met injection group and (D) DS plus Met injection group.
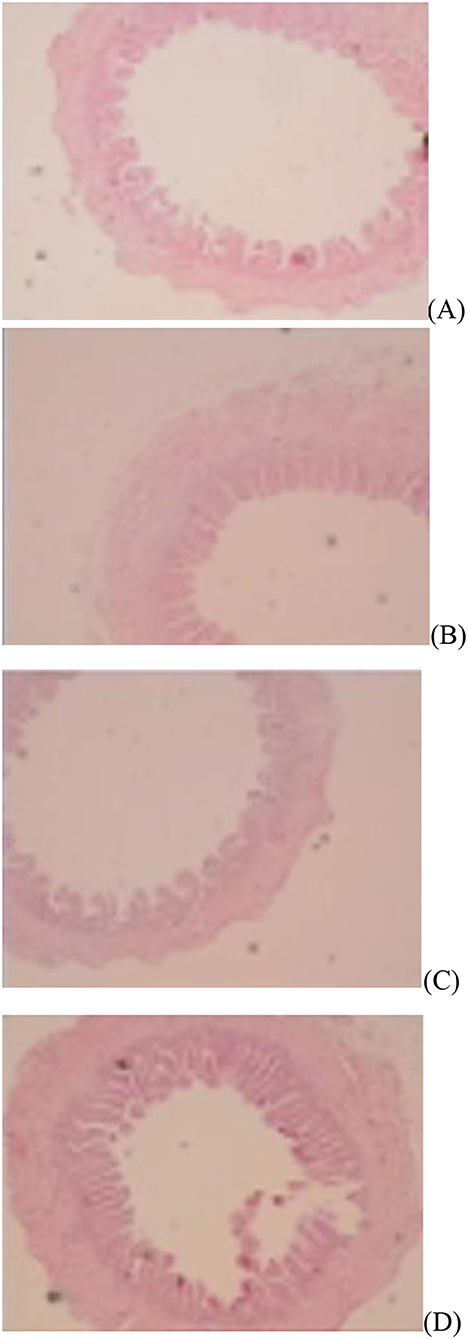
Figure 2. Slice of the jejunum in gosling treated by in ovo injection of methionine (Met) and/or disaccharide (DS) on day 7 post-hatching. (A) Non-injection group; (B) DS injection group; (C) Met injection group and (D) DS plus Met injection group.
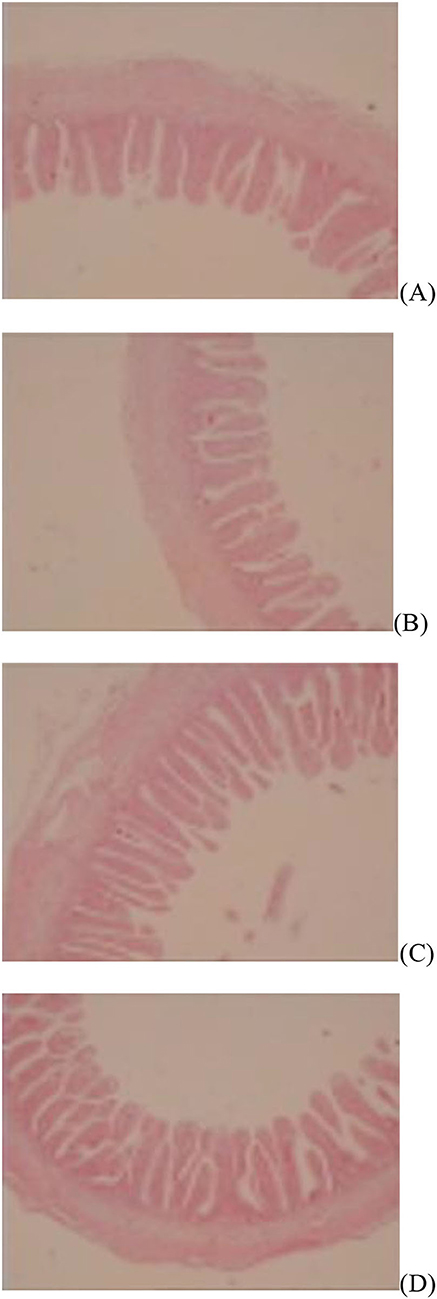
Figure 3. Slice of the jejunum in gosling treated by in ovo injection of methionine (Met) and/or disaccharide (DS) on day 28 post-hatching. (A) Non-injection group; (B) DS injection group; (C) Met injection group and (D) DS plus Met injection group.
Digestive enzymes activities
The Na+/K+ATPase (P < 0.05) and alkaline phosphatase (P < 0.01) activities on the day of hatching and sucrase (P < 0.01) and alkaline phosphatase (P < 0.01) activity on day 7 post-hatching were increased by in ovo Met delivery. In ovo feeding of DS increased maltase (P < 0.01), sucrase (P < 0.01), Na+/K+ATPase (P < 0.05), and alkaline phosphatase (P < 0.01) activities on the day of hatching, also increased sucrase (P < 0.01) and alkaline phosphatase (P < 0.01) activities on day 7 post-hatching. Additionally, in ovo injection of Met plus DS synergistically increased the sucrase activity (P < 0.01) on day 7 post-hatching and the alkaline phosphatase activity (P < 0.01) on the day of hatching (Table 10).
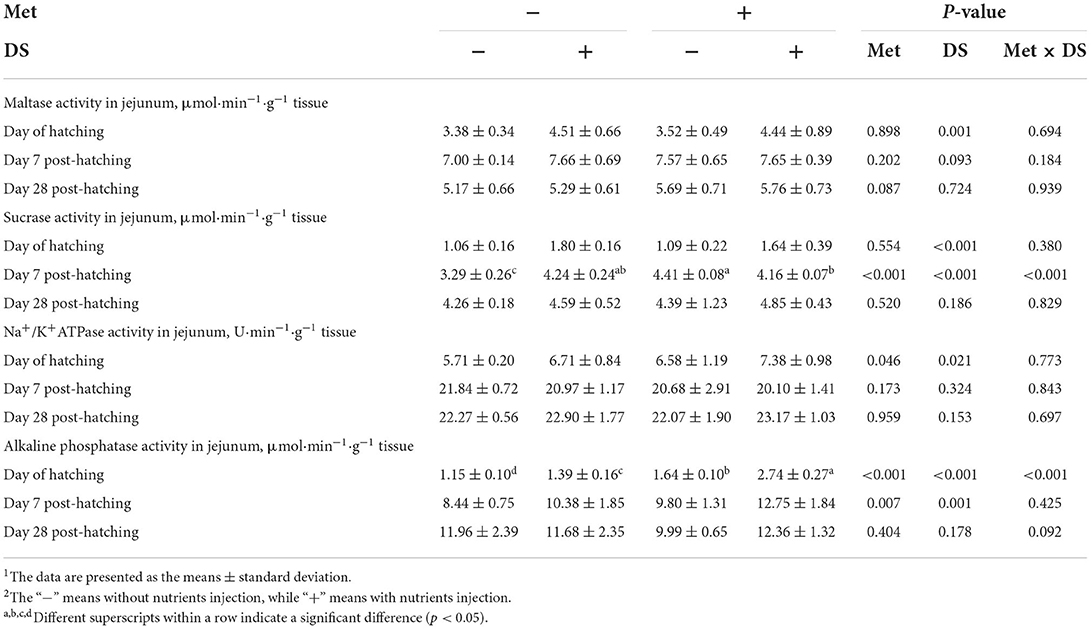
Table 10. Effects of in ovo injection of methionine (Met) and disaccharide (DS) on jejunum digestive enzymes parameters of geese during the early-life post-hatchinga, b.
Jejunum antioxidant parameters
The administration of Met increased GSH (P < 0.01) and GPX (P < .01) levels, whereas decreased GSSG (P < 0.01) levels and GSSG/GSH (P < 0.01) ratio from jejunum on the day of hatching. In addition, the GPX levels on days 7 (P < 0.01) and 28 (P < 0.01) post-hatching was increased by Met injection. However, DS injection had no significant effects on the jejunum antioxidant parameters (Table 11).
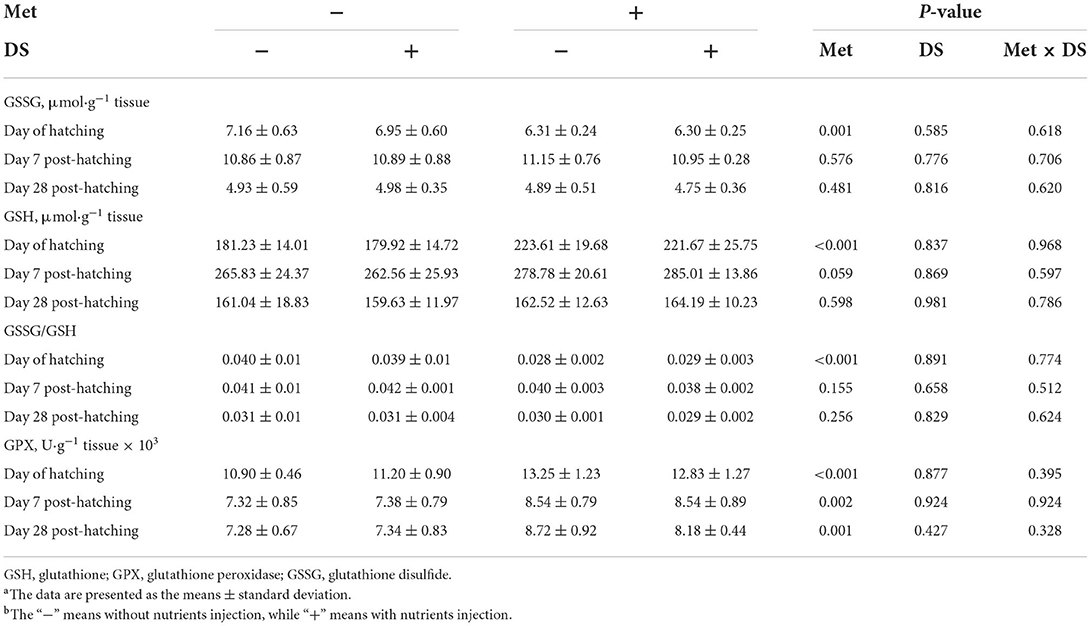
Table 11. Effects of in ovo injection of methionine (Met) and disaccharide (DS) on jejunum antioxidant parameters of geese during the early-life post-hatchinga, b.
Jejunum nutrients transport gene expression
Effects of in ovo injection of Met and/or DS on jejunum nutrients transport gene expression were shown in Table 12. The Met injection upregulated the expression of GLUT-2 on the day of hatching (P < 0.05) and day 28 post-hatching (P < 0.05). DS injection upregulate the SGLT-1 expression on the day of hatching (P < 0.01) and day 7 post-hatching (P < 0.01), GLUT-2 expression on the day of hatching (P < 0.01), day 7 post-hatching (P < 0.01), and day 28 post-hatching (P < 0.01), and SI expression on the day of hatching (P < 0.01), day 7 post-hatching (P < 0.01), and day 28 post-hatching (P < 0.01). Additionally, the expression of GLUT-2 was synergistically upregulated by in ovo injection of Met plus DS on day 28 post-hatching (P < 0.01).
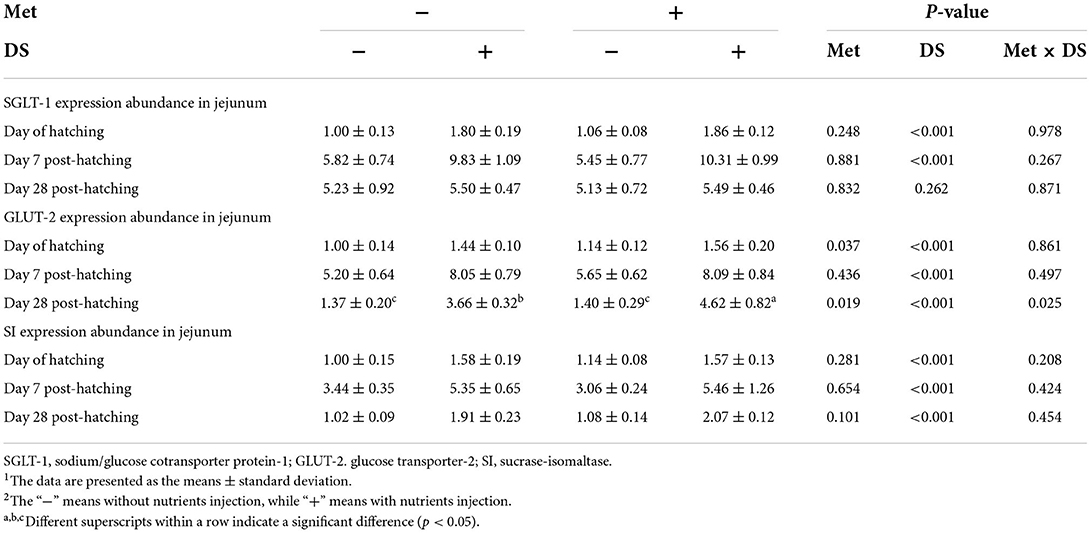
Table 12. Effects of in ovo injection of methionine (Met) and disaccharide (DS) on jejunum nutrients transport gene expression of geese during the early-life post-hatching1, 2.
Discussion
In the present study, the birth weight of goslings was improved by feeding DS into the embryo. Similarly, Foye et al. (23) noted that in ovo injection of carbohydrates (20% dextrin and 3% maltose dissolved in 0.9% saline) improved the growth performance of birds, manifested in high body weight. Indeed, the delivery of carbohydrates into the embryo has been reported to increase the level of available energy, thus avoiding the reduction of body weight induced by the lack of energy (24). We also observed the increase in liver, breast muscle, and total glycogen contents induced by DS delivery on the day of hatching, which indicated an increase in the level of available energy in the embryo. Therefore, we concluded that in ovo injection of DS positively affects the birth weight of goslings, which was attributed to the enhancement of energy storage.
The breast muscle is the largest tissue in the body of poultry, and it plays an important role in metabolic activity because of its relatively large size and glycogen storage. The development of skeletal muscle is a complex process, which comprises the differentiation of myoblast from the mesodermal precursor cells to mature myotubes, thus differentiating to form myofibers during embryogenesis (25–27). Early feeding has been reported to be a suitable strategy to promote the maturation of myofiber, which leads to myofibers with larger diameters (1). Uni et al. (28) injected the DS (25 g/L maltose, 25 g/L sucrose, 200 g/L dextrin, and 1 g/L β-hydroxy-β-methylbutyrate dissolved in 5 g/L NaCl) into the amnion of a chicken egg and found a higher growth of breast muscle. Dong et al. (29) noted that in ovo feeding of DS (25 g/L maltose and 25 g/L sucrose dissolved in 7.5 g/L saline) had stimulating effects on the development of breast muscle in pigeons. In this study, we also observed that in ovo injection of DS increased the relative weight of breast muscle and diameter of myofiber in geese. Additionally, the relative weight of breast muscle and diameter of myofiber were also improved in Met injection. However, studies on the effects of in ovo feeding of Met on the breast muscle parameters were still limited. Mechanisms in Met administration that promote the development of breast muscle may be related to sarcoplasmic hypertrophy (30, 31). Sarcoplasmic hypertrophy would lead to the increase of myofiber cross-sectional area (32). According to Sahebi-Ala et al. (33), Met as a donor of the sulfur group was beneficial to enhancing the diameter of myofiber, resulting in the development of muscle. Therefore, we concluded that in ovo injection of Met or DS had positive effects on the development of breast muscle. However, either Met or DS, the improvement effect of nutrient delivery on breast muscle parameters was only observed in the early-life post-hatching of gosling, while not observed on day 28 post-hatching, which indicated that the injection of DS or Met have short-term effect on the improvement of breast muscle.
Additionally, the growth and development of muscle are usually regulated by specific genes and transcription factors which include Myf-5 and MSTN. Myf-5 is a myogenic regulatory factor and its expression is beneficial to the development of muscle fiber (34, 35). MSTN also known as a growth factor and differentiation factor-8 is mainly expressed in skeletal muscle cells and acts as an essential negative regulator in the growth of skeletal muscle in poultry (36, 37). The downregulation of MSTN expression is contributed to the hypertrophy of myofiber and the development of breast muscle (38). In this study, DS injection upregulated the expression of Myf-5 on the day of hatching, which was matched to the improvement of breast muscle parameters. Additionally, the improvement effects of Met administration on the upregulation of Myf-5 expression and the downregulation of MSTN expression were observed in each measured timepoint. However, higher breast muscle development did not last until day 28 post-hatching. An investigation of the development situation of other muscle parts is needed. As described by Wang et al. (39), the muscle growth-related genes have different expression schedules in different tissues (legs and breast muscle). In brief, we concluded that in ovo delivery of DS or Met positively affects the development of breast muscle by regulating the expression of breast muscle growth-related genes during the early-life post-hatching of goslings.
On the other hand, in ovo injection technique has been reported to support muscle growth via enhancing the liver and muscle glycogen reserves during post-hatching periods (1). Insufficient glycogen reserves will force the embryo to mobilize more muscle proteins toward gluconeogenesis, thus restricting the development of breast muscle (28). A sufficient glycogen store reduces the need for glucose synthesis via gluconeogenesis from muscle protein (3), resulting in the development of breast muscle (28). It has been reported that in ovo injection of carbohydrates improved embryo energy reserves status (10). Uni et al. (28) reported that in ovo injection of DS (25 g/L maltose, 25 g/L sucrose, 200 g/L dextrin, and 1 g/L β-hydroxy-β-methylbutyrate dissolved in 5 g/L NaCl) increased liver and muscular glycogen reserves in chicks. Foye et al. (23) noted that DS (20% dextrin and 3% maltose dissolved in 0.9% saline) injection increased the liver and muscle glycogen levels in turkey. In the present study, we also observed that DS injection increased the liver and muscle glycogen contents, which indicated an enhancement in glycogen store. Additionally, glycogen reserves have been reported to be negatively correlated with G-6-Pase activity (40). G-6-Pase is a membrane-bound enzyme, which is located on the internal membrane of the endoplasmic reticulum. It plays a key role in the terminal step of gluconeogenesis and glycogenolysis, and it can catalyze the conversion of glucose-6-phosphate to glucose, thus releasing glucose from the cell (41, 42). In the present study, DS injection inhibited the G-6-Pase activity. However, Met injection had no significant effects on the glycogen reserves and G-6-Pase activity. We concluded that the promotion effect of DS injection on the development of breast muscle was partially attributed to the increase of glycogen reserves induced by reducing G-6-Pase activity. However, it is worth noticing that the enhancement of glycogen reserves and the reduction of G-6-Pase activity induced by DS administration synchronously occurred, and only presented in the early life of goslings, which indicated the important role of G-6-Pase in regulating the glycogen reserves in the early life and the effect is temporary.
As reported, the increased growth of “demand” tissue such as skeletal muscle should be supported by the development in structure and function of “support” tissue such as gastrointestinal tract (43). Early growth and development of the gastrointestinal tract are critical to optimizing the growth of poultry. The greatest nutrient digestion and absorption happens in the small intestine of poultry, especially the jejunum (44). In the small intestine, the epithelium is thrown into long folds, the villi, which serves to increase the surface area for enzyme secretion and nutrient absorption. Histomorphology is one of the most commonly used parameters to evaluate the status of the gut. Generally, the length and relative weight of the small intestine, as well as the surface area, width, and height of villi from the small intestine, are considered important factors that reflect the development of the small intestine. It has been reported that in ovo feeding of maltose promoted the development of the jejunum villi in poultry (45). Chen et al. (46) noted that DS (25 g/L maltose and 25 g/L sucrose dissolved in 4.5 g/L NaCl) injected into the amnion of duck embryo improved the length and surface area of jejunal villi. In this study, we also observed that in ovo injection of DS increased jejunal villus height and surface area. Additionally, Met also has been reported to be crucial for the maintenance of gut integrity and function (47). Mohammadrezaei et al. (48) reported that in ovo injection of Met enhanced the nutrients absorption ability of chicks by altering the height and width of villi from the small intestine. Chen et al. (49) noted that Met injection increased the relative weight of the duodenum, jejunum, and ileum, as well as the villus height in the small intestine of chicks. Similarly, in the present study, DS injection provisionally improved the development of jejunal villi but did not affect the small intestine parameters. However, Met administration has a long-term effect on the improvement of jejunal villi development and a short-term effect on the improvement of small intestine parameters. Therefore, we concluded that in ovo delivery of Met or DS promoted the development of jejunal villi and small intestine in the early life, which was beneficial to support the absorption of the nutrients.
A mature intestine is often accompanied by abundant digestive enzyme secretion (46). The digestive enzyme plays an important role in the digestion of nutrients into smaller nutrient molecules to facilitate the absorption by the host. It has been reported that a high disaccharidase activity presented in the small intestine ensures rapid carbohydrate digestion, and it breaks down the DS into glucose (50). The alkaline phosphatase involves in digestive processes such as the absorption of glucose (51). Chen et al. (46) reported that DS (25 g/L maltose and 25 g/L sucrose dissolved in 4.5 g/L NaCl) injection increased jejunal sucrase activity in ducks. Similar to the above study, in ovo delivery of Met or DS increased the activities of digestive enzymes in the jejunum; however, this improvement effect was temporary. Further investigation is needed to evaluate the effects of in ovo Met or DS delivery on the digestive enzyme activities. We concluded that in ovo administration of Met or DS had positive effects on the activities of digestive enzymes in jejunum during the early life of goslings.
The nutrient absorption in the intestine is relying on the Na+-dependent kinetics (52). Sodium transportation is achieved by the enterocyte's basolateral Na+/K+ATPase (52). Na+/K+ATPase pumps out sodium to cells whereas it pumps potassium into cells, which is beneficial to maintaining resting potential and regulating nutrient transportation (53–55). In this study, we observed that in ovo injection of Met or DS improved the Na+/K+ATPase activity in the jejunum in early life, with no long-term effect, which indicated that in ovo delivery strategies used in this study had positive effects on the nutrient transportation in the small intestine during the early life.
Moreover, the transport mediators expressed in the apical and basal membrane of enterocytes also play a key role in nutrient absorption. Nutrients are transported into enterocytes by special transporters. The expression of the nutrient transport gene could be used as an indicator of the growth and absorptive capacity of the small intestine (56). Because very small amounts of carbohydrates are presented in the intestine during the late term of incubation, the increase in SI expression at the apical membrane allows for the degradation of carbohydrates into glucose (57). Maintaining high expression levels of SI in the small intestine provides a sufficient supply of substrates for the nutrient transporters such as SGLT-1 and GLUT-2 (57). Glucose is the key fuel and metabolic substrate that could be absorbed via SGLT-1, which is located on the apically of the intestinal epithelium, and transported into the blood via GLUT-2, which is expressed on the basolateral membrane (58, 59). In this study, in ovo injection of Met long-term upregulated the expression of GLUT-2, while DS injection short-term upregulated the expression of SGLT-1, long-term upregulated the expression of GLUT-2, and downregulated the expression of SI. Similarly, Dong et al. (29) reported that the enhancement of the jejunal SGLT-1 and GLUT-2 expression was found during post-hatching periods in pigeon embryos that received carbohydrate solution (2.5% maltose and 2.5% sucrose dissolved in 0.75% saline) into the amniotic fluid. On the other hand, SGLT-1 has been reported to be coupled to the action of the Na+/K+ATPase to mediate glucose transportation (59, 60). GLUT-2 has been reported to be combined with G-6-Pase acted in concert to control the release of glucose from the liver (61). In this study, the increase of Na+/K+ATPase activity was observed by in ovo injection of DS or Met, moreover, in ovo injection of DS decreased G-6-Pase activity. Therefore, we concluded that in ovo injection of DS and/or Met improved nutrient absorption by regulating the nutrient transport gene expression and digestive enzyme activities.
It has been reported that the expression of GLUT-2 was inhibited by the stress response in poultry (62). However, this inhibition effect could be reversed by alleviating oxidative stress (63). Ibrahim et al. (64) reported that feeding broiler chicks with antioxidant herbal extract improved the antioxidant situation of birds, thus upregulating the expression of GLUT-2. The decrease of GSSG and the increase of GSH and GPX concentrations are characteristic of the alleviation of oxidative stress (65). Met is known to decrease oxidative stress and has antioxidant properties (66). Its in ovo delivery has been reported to improve the antioxidative capacity in the embryo (66). In this study, Met injection short-term increased GSH concentration and decreased GSSG and GSSG/GSH levels from jejunum, as well as long-term increased jejunal GPX concentration. Therefore, we concluded that in ovo delivery of Met is beneficial to improve the antioxidative situation of jejunum, thus improving the GLUT-2 expression and further improving intestinal health. Abolfathi et al. (67) reported that feeding broiler chicks with antioxidant herbal extract improved intestinal morphology through increasing intestinal antioxidant capacity. Long et al. (68) noted that dietary supplementation of antioxidant substances improved intestinal antioxidant capacity, thus increasing intestinal digestive enzyme activities. Therefore, we considered that the improvement of jejunum antioxidant parameters by Met injection also benefited the improvement of intestine morphology and digestive enzyme activities.
Conclusion
Our results demonstrated that DS injection increased glycogen reserves and regulated muscle growth-related gene expression, thus improving breast muscle parameters and birth weight during the early-life post-hatching. The short-term effect on the improvement of digestive enzyme activities, nutrient transport enzyme activities, and jejunal villus parameters, as well as the long-term effect on the regulation of nutrient transport-related gene expression, were observed to deliver DS into the embryo, which contributed to promoting the nutrient absorption in post-hatching.
In ovo injection of Met continuously regulated the breast muscle growth-related gene expression, thus improving breast muscle parameters. The temporary improvement effect of Met delivery on the digestive enzymes activities, nutrient transport enzymes activities, small intestine parameters, and nutrient transport-related gene expression, as well as the continuous improvement effect on the jejunal villus parameters and jejunal antioxidative situation, were beneficial to support the nutrient absorption after incubation.
Moreover, in ovo feeding of DS plus Met mixture synergistically improved the diameter of myofiber, jejunal villus height and width, jejunal sucrase, and alkaline phosphatase activities in early-life post-hatching, but continuously upregulated the expression of jejunal GLUT-2. Therefore, we concluded that in ovo delivery of DS plus Met is a suitable strategy to improve the nutrient absorption and breast muscle development of goslings post-hatching.
Data availability statement
The raw data supporting the conclusions of this article will be made available by the authors, without undue reservation.
Ethics statement
The animal study was reviewed and approved by the Animal Care and Use Committee of Jilin Agricultural University (Changchun, China).
Author contributions
DD: writing—original draft, investigation, and writing—review and editing. HZ: formal analysis and investigation. YL: conceptualization and methodology. DL: supervision and writing—review and editing. All authors contributed to the article and approved the submitted version.
Conflict of interest
The authors declare that the research was conducted in the absence of any commercial or financial relationships that could be construed as a potential conflict of interest.
Publisher's note
All claims expressed in this article are solely those of the authors and do not necessarily represent those of their affiliated organizations, or those of the publisher, the editors and the reviewers. Any product that may be evaluated in this article, or claim that may be made by its manufacturer, is not guaranteed or endorsed by the publisher.
References
1. Kornasio R, Halevy O, Kedar O, Uni Z. Effect of in ovo feeding and its interaction with timing of first feed on glycogen reserves, muscle growth, and body weight. Poult Sci. (2011) 90:1467–77. doi: 10.3382/ps.2010-01080
2. Payne JA, Proszkowiec-Weglarz M, Ellestad LE. Delayed access to feed alters expression of genes associated with carbohydrate and amino acid utilization in newly hatched broiler chicks. Am J Physiol Regul Integr Comp Physiol. (2019) 317:R864–78. doi: 10.1152/ajpregu.00117.2019
3. Bertocchi M. Study of the Chicken Physiological Profile in Different Gut Sites and Upon Different Environmental Factors. [Doctor Degree Thesis Dissertation] University of Molise. (2019).
4. Saeed M, Babazadeh D, Naveed M, Alagawany M, Abd El-Hack ME, Arain MA, et al. In ovo delivery of various biological supplements, vaccines and drugs in poultry: current knowledge. J Sci Food Agric. (2019) 99:3727–39. doi: 10.1002/jsfa.9593
5. Kadam MM, Barekatain MR, K Bhanja S, Iji PA. Prospects of in ovo feeding and nutrient supplementation for poultry: The science and commercial applications—A review. J S Food Agric. (2013) 93:3654–61. doi: 10.1002/jsfa.6301
6. Peebles ED. In ovo applications in poultry: a review. Poult Sci. (2018) 97:2322–38. doi: 10.3382/ps/pey081
7. Ashwell C. The effects of intra-amnionic feeding of arginine and/or ß-hyroxy-ß-methylbutyrate on jejunal gene expression in the Turkey embryo and hatchling. Int J Poult Sci. (2009) 8:437–45. doi: 10.3923/ijps.2009.437.445
8. Jha R, Singh AK, Yadav S, Berrocoso JF, Mishra B. Early nutrition programming (in ovo and post-hatch feeding) as a strategy to modulate gut health of poultry. Front Vet Sci. (2019) 6:82. doi: 10.3389/fvets.2019.00082
9. Barbosa RC, Guimarães SB, de Vasconcelos PR, Chaves CR, de Vasconcelos PR. Metabolic effects of l-alanyl-glutamine in burned rats. Burns. (2006) 32:721–7. doi: 10.1016/j.burns.2005.12.014
10. Chen W, Xu J, Tangara M, Peng J. Effects of in ovo injecting disaccharides and alanyl-glutamine dipeptide on the energy status in duck embryos and neonates. Anim Reprod Sci. (2010) 122:29–35. doi: 10.1016/j.anireprosci.2010.06.009
11. Elnesr SS, Elwan HA, Xu QQ, Xie C, Dong XY, Zou XT. Effects of in ovo injection of sulfur-containing amino acids on heat shock protein 70, corticosterone hormone, antioxidant indices, and lipid profile of newly hatched broiler chicks exposed to heat stress during incubation. Poult Sci. (2019) 98:2290–8. doi: 10.3382/ps/pey609
12. Elwan HA, Elnesr SS, Xu Q, Xie C, Dong X, Zou X. Effects of in ovo methionine-cysteine injection on embryonic development, antioxidant status, IGF-I and tlr4 gene expression, and jejunum histomorphometry in newly hatched broiler chicks exposed to heat stress during incubation. Animals. (2019) 9:25. doi: 10.3390/ani9010025
13. Dang DX, Zhou H, Lou Y, Li D. Effects of in ovo feeding of disaccharide and/or methionine on hatchability, growth performance, blood hematology, and serum antioxidant parameters in geese. J Anim Sci. (2022) 100:skac014. doi: 10.1093/jas/skac014
14. AOAC. Official Method of Analysis. 17th ed. Washington, D.C.: Association of Official Analytical Chemists (2000).
15. Mertens DR. Gravimetric determination of amylase-treated neutral detergent fiber in feeds with refluxing in beakers or crucibles: collaborative study. J AOAC Int. (2002) 85:1217–40.
16. Felício AM, Gaya LG, Ferraz JB, Moncau CT, Mattos EC, Santos NP, et al. Heritability and genetic correlation estimates for performance, meat quality and quantitative skeletal muscle fiber traits in broiler. Livest Sci. (2013) 157:81–7. doi: 10.1016/j.livsci.2013.08.005
17. Liu HH, Mao HG, Dong XY, Cao HY, Liu K, Yin ZZ. Expression of MSTN gene and its correlation with pectoralis muscle fiber traits in the domestic pigeons (Columba livia). Poult Sci. (2019) 98:5265–71. doi: 10.3382/ps/pez399
18. Bradford MM. A rapid and sensitive method for the quantitation of microgram quantities of protein utilizing the principle of protein-dye binding. Anal Biochem. (1976) 72:248–54. doi: 10.1016/0003-2697(76)90527-3
19. Zhang M, Liu YL, Fu CY, Wang J, Chen SY, Yao J, et al. Expression of MyHC genes, composition of muscle fiber type and their association with intramuscular fat, tenderness in skeletal muscle of Simmental hybrids. Mol Biol Rep. (2014) 41:833–40. doi: 10.1007/s11033-013-2923-6
20. Wang C, Yue F, Kuang S. Muscle histology characterization using H&E staining and muscle fiber type classification using immunofluorescence staining. Bio protoc. (2017) 7:e2279. doi: 10.21769/BioProtoc.2279
21. Tako E, Ferket PR, Uni Z. Effects of in ovo feeding of carbohydrates and beta-hydroxy-beta-methylbutyrate on the development of chicken intestine. Poult Sci. (2004) 83:2023–8. doi: 10.1093/ps/83.12.2023
22. Uni ZE, Ganot SA, Sklan DA. Posthatch development of mucosal function in the broiler small intestine. Poult Sci. (1998) 77:75–82. doi: 10.1093/ps/77.1.75
23. Foye OT, Uni Z, Ferket PR. Effect of in ovo feeding egg white protein, β-hydroxy-β-methylbutyrate, and carbohydrates on glycogen status and neonatal growth of turkeys. Poult Sci. (2006) 85:1185–92. doi: 10.1093/ps/85.7.1185
24. Kucharska-Gaca J, Kowalska E, Debowska M. In ovo feeding-technology of the future-a review. Ann Anim Sci. (2017) 17:979–92. doi: 10.1515/aoas-2017-0004
25. Velleman SG, Nestor KE, Coy CS, Harford I, Anthony NB. Effect of posthatch feed restriction on broiler breast muscle development and muscle transcriptional regulatory factor gene and heparan sulfate proteoglycan expression. Int J Poult Sci. (2010) 9:417–25. doi: 10.3923/ijps.2010.417.425
26. White RB, Biérinx AS, Gnocchi VF, Zammit PS. Dynamics of muscle fiber growth during postnatal mouse development. BMC Dev Biol. (2010) 10:1–1. doi: 10.1186/1471-213X-10-21
27. Luo W, Nie Q, Zhang X. MicroRNAs involved in skeletal muscle differentiation. J Genet Genomics. (2013) 40:107–16. doi: 10.1016/j.jgg.2013.02.002
28. Uni Z, Ferket PR, Tako E, Kedar O. In ovo feeding improves energy status of late-term chicken embryos. Poult Sci. (2005) 84:764–70. doi: 10.1093/ps/84.5.764
29. Dong XY, Wang YM, Song HH, Zou XT. Effects of in ovo injection of carbohydrate solution on small intestine development in domestic pigeons (Columba livia). J Anim Sci. (2013) 91:3742–9. doi: 10.2527/jas.2013-6400
30. Zhai W, Araujo LF, Burgess SC, Cooksey AM, Pendarvis K, Mercier Y, et al. Protein expression in pectoral skeletal muscle of chickens as influenced by dietary methionine. Poult Sci. (2012) 91:2548–55. doi: 10.3382/ps.2012-02213
31. Zhai W, Peebles ED, Schilling MW, Mercier Y. Effects of dietary lysine and methionine supplementation on Ross 708 male broilers from 21 to 42 d of age (I): Growth performance, meat yield, and cost effectiveness. J Appl Poult Res. (2016) 25:197–211. doi: 10.3382/japr/pfw002
32. Jorgenson KW, Phillips SM, Hornberger TA. Hornberger. Identifying the structural adaptations that drive the mechanical load-induced growth of skeletal muscle: a scoping review. Cells. (2020) 9:1658. doi: 10.3390/cells9071658
33. Sahebi-Ala F, Hassanabadi A, Golian A. Effect of replacement different methionine levels and sources with betaine on blood metabolites, breast muscle morphology and immune response in heat-stressed broiler chickens. Ital J Anim Sci. (2021) 20:33–45. doi: 10.1080/1828051X.2020.1868358
34. Crist CG, Montarras D, Buckingham M. Muscle satellite cells are primed for myogenesis but maintain quiescence with sequestration of Myf5 mRNA targeted by microRNA-31 in mRNP granules. Cell Stem Cell. (2012) 11:118–26. doi: 10.1016/j.stem.2012.07.010
35. Hernández-Hernández JM, García-González EG, Brun CE, Rudnicki MA. The myogenic regulatory factors, determinants of muscle development, cell identity and regeneration. Semin Cell Dev Biol. (2017) 72:10–8. doi: 10.1016/j.semcdb.2017.11.010
36. McPherron AC, Lawler AM, Lee SJ. Regulation of skeletal muscle mass in mice by a new TGF-p superfamily member. Nature. (1997) 387:83–90. doi: 10.1038/387083a0
37. Kim GD, Lee JH, Song S, Kim SW, Han JS, Shin SP, et al. Generation of myostatin-knockout chickens mediated by D10A-Cas9 nickase. FASEB J. (2020) 34:5688–96. doi: 10.1096/fj.201903035R
38. Dou T, Li Z, Wang K, Liu L, Rong H, Xu Z, et al. Regulation of myostatin expression is associated with growth and muscle development in commercial broiler and DMC muscle. Mol Biol Rep. (2018) 45:511–22. doi: 10.1007/s11033-018-4187-7
39. Wang H, Shen Z, Zhou X, Yang S, Yan F, He K, et al. Identification of differentially expressed genes in different types of broiler skeletal muscle fibers using the RNA-seq technique. Biomed Res Int. (2020) 2020:9478949. doi: 10.1155/2020/9478949
40. De Oliveira JE, Druyan S, Uni Z, Ashwell CM, Ferket PR. Metabolic profiling of late-term turkey embryos by microarrays. Poult Sci. (2013) 92:1011–128. doi: 10.3382/ps.2012-02354
41. Vinayagam R, Jayachandran M, Chung SS, Xu B. Guava leaf inhibits hepatic gluconeogenesis and increases glycogen synthesis via AMPK/ACC signaling pathways in streptozotocin-induced diabetic rats. Biomed Pharmacother. (2018) 103:1012–7. doi: 10.1016/j.biopha.2018.04.127
42. Kim MS, Krawczyk SA, Doridot L, Fowler AJ, Wang JX, Trauger SA, et al. ChREBP regulates fructose-induced glucose production independently of insulin signaling. J Clin Invest. (2019) 126:4372–86. doi: 10.1172/JCI81993
43. Rance KA, McEntee GM, McDevitt RM. Genetic and phenotypic relationships between and within support and demand tissues in a single line of broiler chicken. Br Poult Sci. (2002) 43:518–27. doi: 10.1080/0007166022000004426
44. Liu H, Zhang J, Zhang S, Yang F, Thacker PA, Zhang G, et al. Oral administration of Lactobacillus fermentum I5007 favors intestinal development and alters the intestinal microbiota in formula-fed piglets. J Agric Food Chem. (2014) 62:860–6. doi: 10.1021/jf403288r
45. Jia CL, Wei ZH Yu M, Wang XQ Yu F. Effect of in-ovo feeding maltose on the embryo growth and intestine development of broiler chicken. Indian J Anim Sci. (2011) 81:503–6.
46. Chen W, Wang R, Xiong XL, Wan HF, Xu J, Peng J. Influence of in ovo injection of disaccharides, glutamine and β-hydroxy-β-methylbutyrate on the development of small intestine in duck embryos and neonates. Br Poult Sci. (2010) 51:592–601. doi: 10.1080/00071668.2010.520533
47. Nazem MN, Sajjadian SM, Kheirandish R, Mohammadrezaei H. Histomorphometric analysis of the small intestine of broiler chick embryos injected in ovo with methionine. Anim Prod Sci. (2017) 59:133–9. doi: 10.1071/AN17269
48. Mohammadrezaei H, Nazem M, Mohammadrezaei M. Effect of in ovo injection of Methionine on the histomorphometry of Jejunum of chicken embryo. Biol Forum. (2015) 7:23.
49. Chen MJ, Zhou JY, Chen YJ, Wang XQ, Yan HC, Gao CQ. The in ovo injection of methionine improves intestinal cell proliferation and differentiation in chick embryos by activating the JAK2/STAT3 signaling pathway. Anim Nutr. (2021) 7:1031–8. doi: 10.1016/j.aninu.2021.03.009
50. Shibata M, Takahashi T, Kozakai T, Kakudo M, Kasuga S, Azuma Y, et al. Active transport of glucose across the jejunal epithelium decreases with age in broiler chickens. Poult Sci. (2019) 98:2570–6. doi: 10.3382/ps/pez002
51. Bilal T, Atis S, Keser O. The effects of microbial phytase on serum calcium and phosphorus levels and alkaline phosphatase activities in broilers fed diets containing different levels of phosphorus. Acta Scientiae Veterinariae. (2015) 43:1–5.
52. Uni Z. Early development of small intestinal function. In: Perry GC, editors. Avian Gut Function in Health and Disease. United Kingdom Branch: World's Poultry Science Association (2006). p. 28-9. doi: 10.1079/9781845931803.0029
53. Abbasi F, Liu J, Zhang H, Shen X, Luo X. Effects of feeding corn naturally contaminated with aflatoxin on growth performance, apparent ileal digestibility, serum hormones levels and gene expression of Na+, K+-ATPase in ducklings. Asian-Australas J Anim Sci. (2018) 31:91. doi: 10.5713/ajas.17.0383
54. Kiela PR, Ghishan FK. Physiology of intestinal absorption and secretion. Best Pract Res Clin Gastroenterol. (2016) 30:145–59. doi: 10.1016/j.bpg.2016.02.007
55. Revajová V, Herich R, Levkut M, Žitnan R, Albrecht E, Röntgen M, et al. Immunolocalization of Na+/K+-ATPase and proliferative activity of enterocytes after administration of glucan in chickens fed T-2 toxin. Acta Veterinaria Brno. (2019) 87:371–7. doi: 10.2754/avb201887040371
56. Gao CQ, Yang JX, Chen MX, Yan HC, Wang XQ. Growth curves and age-related changes in carcass characteristics, organs, serum parameters, and intestinal transporter gene expression in domestic pigeon (Columba livia). Poult Sci. (2016) 95:867–77. doi: 10.3382/ps/pev443
57. Dong XY, Wang YM, Yuan C, Zou XT. The ontogeny of nutrient transporter and digestive enzyme gene expression in domestic pigeon (Columba livia) intestine and yolk sac membrane during pre-and posthatch development. Poult Sci. (2012) 91:1974–82. doi: 10.3382/ps.2012-02164
58. Mace OJ, Lister N, Morgan E, Shepherd E, Affleck J, Helliwell P, et al. An energy supply network of nutrient absorption coordinated by calcium and T1R taste receptors in rat small intestine. J Physiol. (2009) 587:195–210. doi: 10.1113/jphysiol.2008.159616
59. Wong EA, Gilbert ER, Miska KB. Nutrient transporter gene expression in poultry, livestock and fish. Biol Domest Anim. (2017) 2017:319–44. doi: 10.1201/9781315152080-11
60. Thorsen K, Drengstig T, Ruoff P. Transepithelial glucose transport and Na+/K+ homeostasis in enterocytes: an integrative model. Am J Physiol Cell Physiology. (2014) 307:C320–37. doi: 10.1152/ajpcell.00068.2013
61. Donkin SS, Hammon H. Hepatic gluconeogenesis in developing ruminants. In: Mosenthin R, Zentek J, Zebrowska T, editors. Biology of Growing Animals. Elsevier. 2005. p. 375-390. doi: 10.1016/S1877-1823(09)70022-0
62. Sahin N, Hayirli A, Orhan C, Tuzcu M, Akdemir FA, Komorowski JR, et al. Effects of the supplemental chromium form on performance and oxidative stress in broilers exposed to heat stress. Poult Sci. (2017) 96:4317–24. doi: 10.3382/ps/pex249
63. Al Wakeel RA, Shukry M, Abdel Azeez A, Mahmoud S, Saad MF. Alleviation by gamma amino butyric acid supplementation of chronic heat stress-induced degenerative changes in jejunum in commercial broiler chickens. Stress. (2017) 20:562–72. doi: 10.1080/10253890.2017.1377177
64. Ibrahim D, Moustafa A, Metwally AS, Nassan MA, Abdallah K, Eldemery F, et al. Potential application of Cornelian cherry extract on broiler chickens: Growth, expression of antioxidant biomarker and glucose transport genes, and oxidative stability of frozen meat. Animals. (2021) 11:1038. doi: 10.3390/ani11041038
65. Wang J, Zhu H, Zhang C, Wang H, Yang Z. Baicalein ameliorates cadmium-induced hepatic and renal oxidative damage in rats. Indian J Anim Res. (2019) 53:523–7. doi: 10.18805/ijar.B-853
66. Elwan H, Xie C, Miao LP, Dong X, Zou XT, Mohany M, et al. Methionine alleviates aflatoxinb1-induced broiler chicks embryotoxicity through inhibition of caspase-dependent apoptosis and enhancement of cellular antioxidant status. Poult Sci. (2021) 100:101103. doi: 10.1016/j.psj.2021.101103
67. Abolfathi ME, Tabeidian SA, Shahraki AF, Tabatabaei SN. Habibian M. Effects of ethanol extract of elecampane (Inula helenium L) rhizome on growth performance, diet digestibility, gut health, and antioxidant status in broiler chickens. Livestock Sci. (2019) 223:68–75. doi: 10.1016/j.livsci.2019.03.006
Keywords: goose, in ovo injection, intestinal health, post-hatching development, nutrient absorption
Citation: Dang DX, Zhou H, Lou Y and Li D (2022) Effects of in ovo feeding of methionine and/or disaccharide on post-hatching breast development, glycogen reserves, nutrients absorption parameters, and jejunum antioxidant indices in geese. Front. Vet. Sci. 9:944063. doi: 10.3389/fvets.2022.944063
Received: 14 May 2022; Accepted: 25 July 2022;
Published: 22 August 2022.
Edited by:
Abdelrazeq M. Shehata, Al-Azhar University, EgyptReviewed by:
Sameh A. Abdelnour, Zagazig University, EgyptSubrat Kumar Bhanja, Indian Council of Agricultural Research (ICAR), India
Sharif Hasan Siddiqui, University of Rochester, United States
Mohammadali Alizadeh, University of Guelph, Canada
Copyright © 2022 Dang, Zhou, Lou and Li. This is an open-access article distributed under the terms of the Creative Commons Attribution License (CC BY). The use, distribution or reproduction in other forums is permitted, provided the original author(s) and the copyright owner(s) are credited and that the original publication in this journal is cited, in accordance with accepted academic practice. No use, distribution or reproduction is permitted which does not comply with these terms.
*Correspondence: Desheng Li, bGlkZXNoZW5nMDcyNjUyMSYjeDAwMDQwOzEyNi5jb20=