- Heilongjiang Key Laboratory for Animal and Comparative Medicine, College of Veterinary Medicine, Northeast Agricultural University, Harbin, China
The growth of poultry farming has enabled higher spread of infectious diseases and their pathogens among different kinds of birds, such as avian infectious bronchitis virus (IBV) and avian influenza virus (AIV). IBV and AIV are a potential source of poultry mortality and economic losses. Furthermore, some pathogens have the ability to cause zoonotic diseases and impart human health problems. Antiviral treatments that are used often lead to virus resistance along with the problems of side effects, recurrence, and latency of viruses. Though target hosts are being vaccinated, the constant emergence and re-emergence of strains of these viruses cause disease outbreaks. The pharmaceutical industry is gradually focusing on plant extracts to develop novel herbal drugs to have proper antiviral capabilities. Natural therapeutic agents developed from herbs, essential oils (EO), and distillation processes deliver a rich source of amalgams to discover and produce new antiviral drugs. The mechanisms involved have elaborated how these natural therapeutics agents play a major role during virus entry and replication in the host and cause inhibition of viral pathogenesis. Nanotechnology is one of the advanced techniques that can be very useful in diagnosing and controlling infectious diseases in poultry. In general, this review covers the issue of the poultry industry situation, current infectious diseases, mainly IB and AI control measures and, in addition, the setup of novel therapeutics using plant extracts and the use of nanotechnology information that may help to control these diseases.
Introduction
Poultry production plays a vital role in food production and poverty alleviation in the absence of other nutrient-rich food items (1, 2). There are several important factors, such as poultry immunity, health, and production, which contest the future growth of the poultry industry (3). Globally, poultry diseases are continuously emerging to be the main subject in the poultry industry (4). Infectious bronchitis (IB), avian influenza (AI), Newcastle disease, and Gumboro disease are considered the common poultry diseases globally (5, 6). Although abundant consideration has been paid to limiting infectious diseases to avoid losses, these diseases continue to emerge and re-emerge (7). Avian infectious bronchitis virus (IBV), of the genus Gammacoronavirus, is one such kind of virus. In 1931, IBV was the first coronavirus identified in poultry and was considered a very vital pathogenic virus in livestock (8). At present, avian coronavirus IBV is a major economic pathogen of domestic poultry that causes mortality and significant losses in production regardless of vaccination (9). Many researchers have reported worldwide outbreaks caused by IBV in chickens and other birds that are characterized by high morbidity, mortality, and poor egg and meat production (10, 11). Currently, live attenuated vaccines are extensively used for the prevention and control of IB (12, 13). However, due to the higher diversity in viral genetic composition and the emergence of novel strains, the efficacy of vaccination is being greatly compromised (14).
Avian influenza virus (AIV) is another very important virus that belongs to the family Orthomyxoviridae and causes huge losses to the global poultry industry (15, 16). This virus has an eight-segment negative, single-stranded RNA and encodes about 11 proteins (17). In addition, AIV is divided into subtypes based on the hemagglutinin (HA) and neuraminidase (NA) proteins that are present on the surface and are responsible for virus attachment and release, respectively (18, 19). The reassortment of HA and NA with different subtypes might consequence in disastrous pandemics as H1N1, H2N2, and H3N2 in humans (20). Based on its pathogenicity, the virus is categorized into highly pathogenic AIV (HPAIV) and low pathogenic AIV (LPAIV) (21, 22). The co-circulation of many subtypes of AIV, such as H5, H7, and H9, makes vaccination unsuccessful for production (23). Thus, it is very important to develop a vaccine that can be useful for multiple serotypes. Considering these factors, the risk of emergence of novel serotypes and infectious diseases into the human population and livestock increases (24). For instance, the occurrence of the highly pathogenic H7N9 AIV caused huge losses due to the intensification of the poultry production system. The increased density of poultry stocks and upsurges in populations lead to higher transmission between birds and humans (25). Additionally, the increased rate of evolution and pressure on the immune system limit the effect of vaccination (26). Furthermore, the use of antibiotics in feed has raised the issue of drug residues and ultimately pathogen resistance, so that the subtherapeutic use of antibiotics has been totally banned in European countries since January 2006 (27).
Therefore, it is becoming very imperative to develop new strategies to control these types of diseases. For this purpose, consideration is being given to antiviral herbs that have no noticeable side effects on human and poultry health (28, 29). The influence of novel and alternative therapeutic agents on definite immune functions and a decrease in hazards might be very useful to counter viral infection (30). In the past, some derivatives and feed additives, i.e., plant extract, prebiotics, probiotics, enzymes, and yeast, have been reported to have immunomodulatory effects (31, 32). Their effects include improving metabolic status, decreasing physiological stress, inhibiting the expedition of cytokines by macrophages, and antimicrobial activity, thus enhancing immunity (33, 34). Antibodies (Abs) from mammals have been used for diagnostic and for therapeutic purposes against the invasion of pathogens (35), however, these Abs are obtained by invasive techniques. Thus, avian eggs have been considered for alternative production of Abs for therapeutic purposes against pathogen invasion (36, 37). Another important application, such as different nanomaterials that promote interactions between molecules and the virus, and enables researchers to construct a portable electroanalytical biosensing analyzer that effectively detects the virus (38, 39) along with the development of a nano-based viral vaccine. Generally, the development of first-generation vaccines has been produced by inactivation/killing or live attenuation of living organisms and second- and third-generation vaccines have been developed using RNA/DNA subunits (40, 41). Though subunit-based vaccines have many advantages, such as lower cost and the proficiency to produce an immune response against a specific pathogen as compared to conventionally developed vaccines (42), there are some disadvantages, such as poor immunogenicity, toxic effect, and are in vivo intrinsically instable along with multiple boosters. Hence, the development of a novel vaccine that can perform as an immunogen along with adjuvants to produce protection against the pathogen with enhanced immune response (43) and certainly nanotechnology is an advance technology that deals with the shortcomings of conventional vaccines.
Genomic assortment and outbreaks of such infectious diseases can turn into an epidemic and cause a widespread adverse effect on the global trade of poultry products along with human health (20, 44). The development of novel antiviral treatment strategies to control IBV and AIV is urgently required as existing inimitable tasks concerning its control in commercial poultry farming. Therefore, in the present review, we discuss possible new strategies and plant derivatives along with the use of nanotechnology that might be helpful to better control IB and AI.
Medicinal Plant Derivatives Against IBV Infection
Research on plant-derived antiviral substances is inadequate in comparison with the search for antimicrobial properties. However, a number of investigations have publicized the positive role of plant extracts in relation to antiviral effect (Figure 1). Assessment of different plant extracts was conducted, and Thymus vulgaris, Mentha piperita, and Desmodium canadense showed an antiviral effect against IBV preinfection and postinfection (53). Hypericum perforatum L, which is also recognized as Saint John's Wort, has been well studied for its biochemical composition and pharmacological activities (54). The extracts of H. perforatum, such as hypericin (HY), quercetin, pseudohypericin, and quercitrin, were assessed as antiviral against IBV. It was proved that a reduction in mRNA expression of pro-inflammatory cytokines [interleukin-6 (IL-6) and tumor necrosis factor-α (TNF-α)] via the nuclear factor kappa B (NF-κB) signaling pathway and upregulation of type I interferon via the MDA5 signaling pathway (55). Similarly, in another study, the natural compound of HY polycyclic quinone (56) has been proven to be antiviral against IBV infection in chicken embryonic kidney cells (CEK). IBV-infected CEK cells were treated with HY, and were found to upregulate anti-apoptosis genes such as Bcl-2 and to downregulate apoptosis-associated genes such as Caspase 3, Caspase 8, Bax and Fas, FasL, and JNK (57). The extract of Sambucus nigra was reported to have an inhibitory effect against IBV infection. When IB occurs at the early age of chicken,. nigra extract has shown inhibition of IBV infection in the early phase of disease infestation (58). Thus, it can be used for the inhibition of IBV and other CoVs. Garlic from the genus Allium of the family Amaryllidaceae was used as an antitherapeutic use in early times (59). The antiviral potential of garlic against IBV has been reported when exposed to various IBV strains and it showed an inhibitory effect (60). Allium (garlic) have been found to be a very effective therapeutic agent against corona viruses including COVID-19 as far as the immune system is concerned (61).
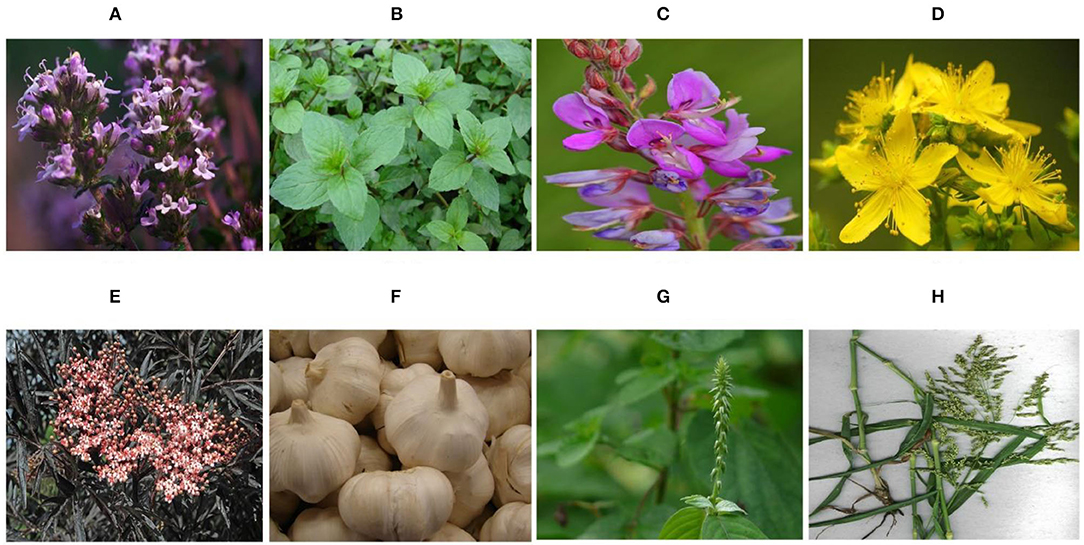
Figure 1. Plants in therapeutics to control infectious bronchitis (IB). (A) Thymus vulgaris (45). (B) Mentha piperita (46). (C) Desmodium canadense (47). (D) Hypericum perforatum (48). (E) Sambucus nigra (49). (F) Alium sativum (50). (G) Achyranthes aspera (51), and (H) Panicum antidotale (52).
Similarly, the immune system of chicken against IBV has shown to be resistant during the supplementation of extract of sweet orange peel (62). Shahzad et al. (63) reported that various plants, such as Achyranthes aspera, Neuroda procumbens, Panicum antidotale, Ochthochloa compressa, and Suaeda fruticose, were very effective against all poultry viruses through their extracts, hence their use can be beneficial in future IBV infections. These plants extracts are useful in controlling the viral growth and have antiviral effect. The optimum antiviral activity and lowest viral growth were observed with the extracts of S. icolados and O. compressa, in terms of HA/HI as HA titer = 0 showed complete control over viral growth (64). The essential oils (EO) have been used for different viruses, such as dengue and herpesvirus, to assess virucidal activities (65, 66). Jackwood et al. (67) have carried out a research using two different hosts, i.e., Embryonated eggs (ECE) and Vero cells (E6). The EO designated as QR448a and botanical oleoresins in liquid emulsion was administered to the chicken infected with IBV. Following the aforementioned treatment, the clinical manifestation, pathological lesion, and RNA of IBV decreased, confirming the positive effect of EO and oleoresins.
Use of Nanotechnology to Control IBV
Nanotechnology is one of the inventive skills that have an inordinate possibility of uses along with a socio-economic prospective in the global poultry industry (68). The origin of nano is a word from the Latin “nanus” that mean a lesser, dwarf, or minute unit approximately 1 nm equals 10−9 m (69). Nanotechnology has developed the area of biomedical sciences, with a vast variety of NPs and capability in regard to diagnosis to therapeutics for viral infectious diseases (Figure 2) (70, 71).
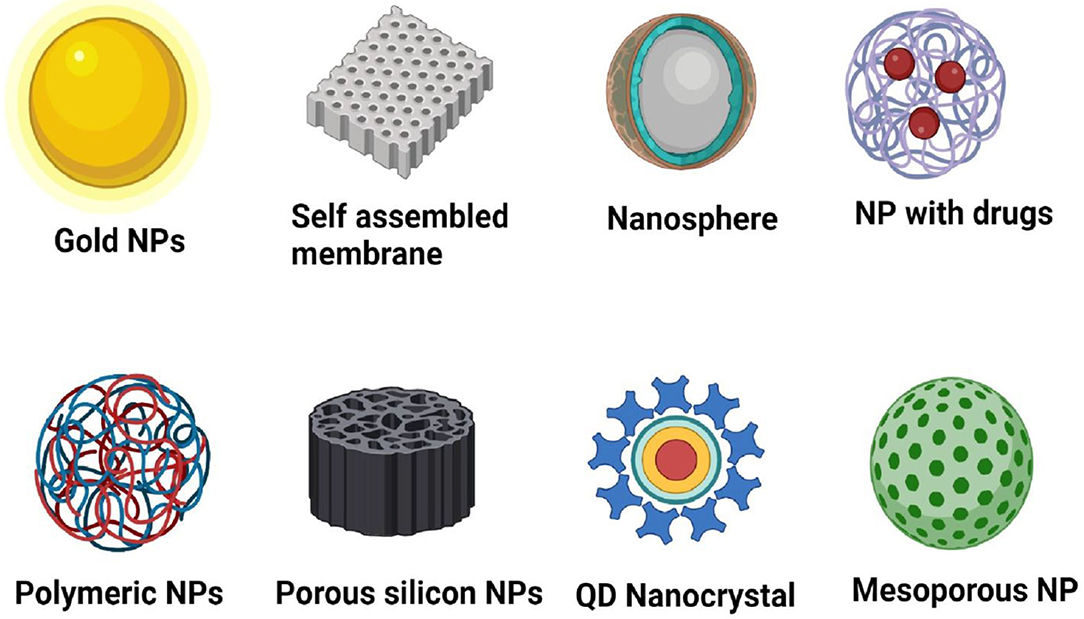
Figure 2. Different types of nanoparticles used in biomedicine for therapeutics of viral diseases. NP, Nanoparticles created with Biorender.com.
The antiviral effects of G-Ag nanocomposites against IBV and Feline CoV (72) were investigated. Li et al. (73) have prepared a vaccine based on IBV-flagellin self-assembled protein nanoparticles (SAPNs) against IBV by incorporating spike protein as an adjuvant with the flagellin. IBV-challenged chicken with the aforementioned nano-vaccine showed a higher Ab response confirming its protective role. In another research, adjuvant-based nano-carriers of Quil-A and chitosan (QAC) having a size <100 nm were developed by Chandrasekar et al. (74). Furthermore, encapsulation with plasmid DNA (pQAC-N) vaccine and coding nucleocapsid (N) protein was done. When this vaccine was administered intranasally, improved immunogenicity and protection in terms of humoral and cellular immunity against IBV infection were observed. In addition, a decrease in viral load and reduced severity of clinical symptoms were observed. Polymeric carbonized nano-gels (CNGs) are also very effective in terms of IBV therapeutic agents as CNGs are very adsorbent on virus particles that may obstruct S1 and S2 glycoproteins to interact with host cells during infection. The development of CNGs is carried out at higher temperatures that possess a greater positive charge, which might cause the neutralization on the surface charge of IBV and weaken the pathogenicity of virus (75). Chou et al. (76) demonstrated the amalgamation of CNGs from lysine hydrochloride by a simple pyrolysis method, which resulted in the inhibition of virus against IBV. At a very lower concentration of 30 μg/ml, its efficiency was demonstrated by showing an inhibitory effect >98% in IBV-infected chicken embryos.
Several uses of full NP based on magnetic and gold quantum dots (QDs) for virus detection and tracing have been described (77). Ahmed et al. (78) reported a novel method by linking anti-IBV Abs with QDs for the production of an immune-link of chiral-QDs as a chiro-immuno-sensor for IBV from blood samples of chicken. Furthermore, a self-assembled nanostructure was established for the limit need to be used for detection and 47.91 egg infection dose (EID)/50 ml, was quite efficient in examination of the target virus (79). Virus-like particles (VLPs) have been extensively studied and developed to transport a range of compounds, including medicines, peptides/proteins, RNA/DNA, Abs, and vaccines, for use as antigen nanocarriers and adjuvants to immune cells in an attempt to elicit a protective humoral immune response (80). Cell surface protein S that binds to the receptor can induce the body to produce an immune response (81). Chen et al. (82) have described a classic approach to the use of avian CoVs VLPs-based S protein using 100-nm gold np incubation with an optimized concentration of viral proteins (Figure 3). The aforementioned study resulted in the impulsive development of proteins with the induction of the assembly of virus-like nanostructures with viral antigens coating the fundamental particulate. Furthermore, it was concluded that the results of VLPs from the present study validate the successful preparation of synthetic VLPs (sVLPs) through NP, innate inclination to persuade protein coating (83, 84).
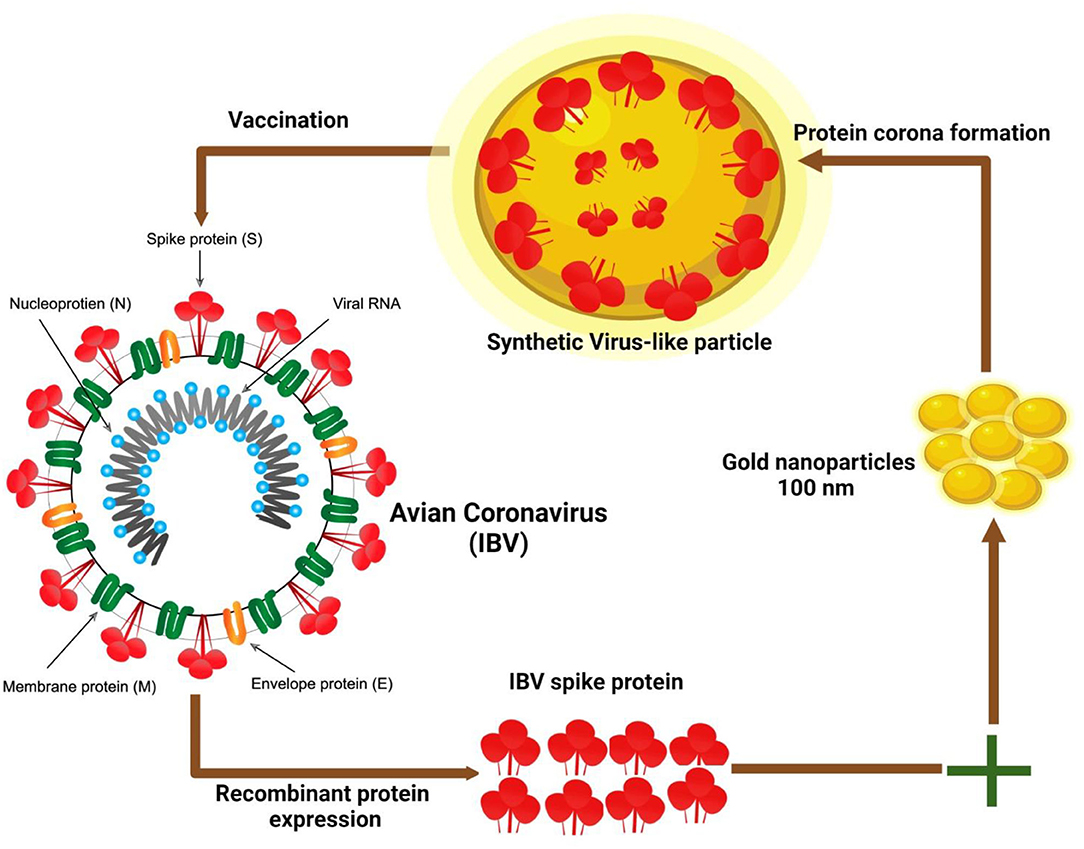
Figure 3. Schematic illustration of the preparation of synthetic virus-like particles (sVLPs) of avian corona virus. sVLPs were prepared from optimized mix enclosing spike (S) protein of infectious bronchitis virus (IBV) and 100 nm gold nanoparticles through impulsive development of corona protein (72).
Use of Virucidal Drugs Against IBV Infection
Advanced studies in the molecular biology of viruses have highlighted many potential targets for antiviral drugs (85). The efficacy of virus activity limiting drugs, such as Argovit, Triviron, Ecocid, and lauric acid monoglycerides, has been tested in chickens by inoculation of the vaccine strain of IBV H120 against IB of chickens. These virucidal drugs were shown to possess potential virucidal activity in the small intestine against IBV (86). Traditional medicines, mainly from China, have been very effective against respiratory viruses (87). A combination effect of traditional Chinese medicines (TCMs), such as Shegandilong (SGDL) and doxycycline, was examined by Feng et al. (88) to prevent viral infection, and the outcomes indicated an increased level of tracheal immunoglobulin A (IgA). Additionally, SGDL granule and doxycycline efficiently subdued the replication of IBV and inhibited the propagation of IBV tropism between the trachea and lung. Furthermore, they controlled the expression of mRNA of IL-6, IL-1β, IFN-γ, and TNF-α, and reduced histopathological lesions of respiratory organs such as trachea and lung. In other similar studies, based on the combination of glycyrrhizin diammonium (GD) and lithium chloride (LiCl) conducted by Li et al. (89), IBV was used to infect cells. The effect of the drugs to inhibit the virus was established by using CEK. Additionally, the apoptotic effect was positively associated with the cytopathic effect and might be repressed in effect of the drug treatment. The replication of IBV and the effect of LiCl were examined in two different cells types, i.e., Vero cells derived from African Green monkey kidney-epithelial cells and DF-1 cells derived from chicken embryo fibroblast cells. The concentration of viral RNA and proteins was reduced after the aforementioned cells lined were treated with LiCl (90).
Amino acid derivatives are known for their antiviral ability against different poultry, animal, plant, and human viruses (91). The modification of amino acids with respect to antimicrobial peptides activities plays an important role in antiviral activities (92). The evaluation of antimicrobial peptides was carried out in terms of swine intestinal antimicrobial peptides (SIAMP) against IBV in chicken embryos. Pre-treatment of embryos with SIAMP and infected with IBV showed a remarkable reduction in mortality. Though the authors of this study did not present the characterization of the antiviral mechanism, they suggested that SIAMP might play a role during virus attachment to the cell surface, thus limiting IBV infection (93). Mannose binding lectin (MBL) is well known as an antiviral agent (94). In terms of IBV, recombinant chicken MBL showed antiviral activity during the direct interaction with virus particles that subsequently inhibited IBV infection (95). Thiazolidines are reported as antiviral inhibitors (96). The substitution of 2-aryl thiazolidine-4-carboxylic acids 1a-h was better antiviral than their N-acylated derivatives 2a and 3a, which would be promising antiviral agents against AIV H9N2 and IBV infections in the near future (97). Avian eggs can produce Abs that protect against pathogen entry by non-invasive techniques. Similarly, when Tsukamoto et al. (98) produced IgY (Ab) from ostrich eggs and used against IBV-infected chickens, infection was remarkably inhibited.
Medicinal Plant Derivatives Against AIV Infection
Novel anti-influenza therapeutic techniques are a prerequisite for its control in the present era, and these developments can be achieved through the search for new ways to modulate the viral mechanism and immune system (Figure 4) (105–107). Numerous herbal species with potential inhibitory effects on the replication of influenza viruses were frequently described using in vitro cell culture methods and embryonated eggs or in vivo mouse models (108, 109). Many phytochemicals of relatively low molecular weight, such as polyphenols, flavonoids, terpenes, glucosides, and alkaloids extracted from different plants have been shown to be endowed with an antiviral activity against AIV (110, 111). In this regard, polyphenol-enriched extract of Rumex acetosa employs an inhibitory effect against AIV replication by weakening the attachment of viral particles to target cells (112).
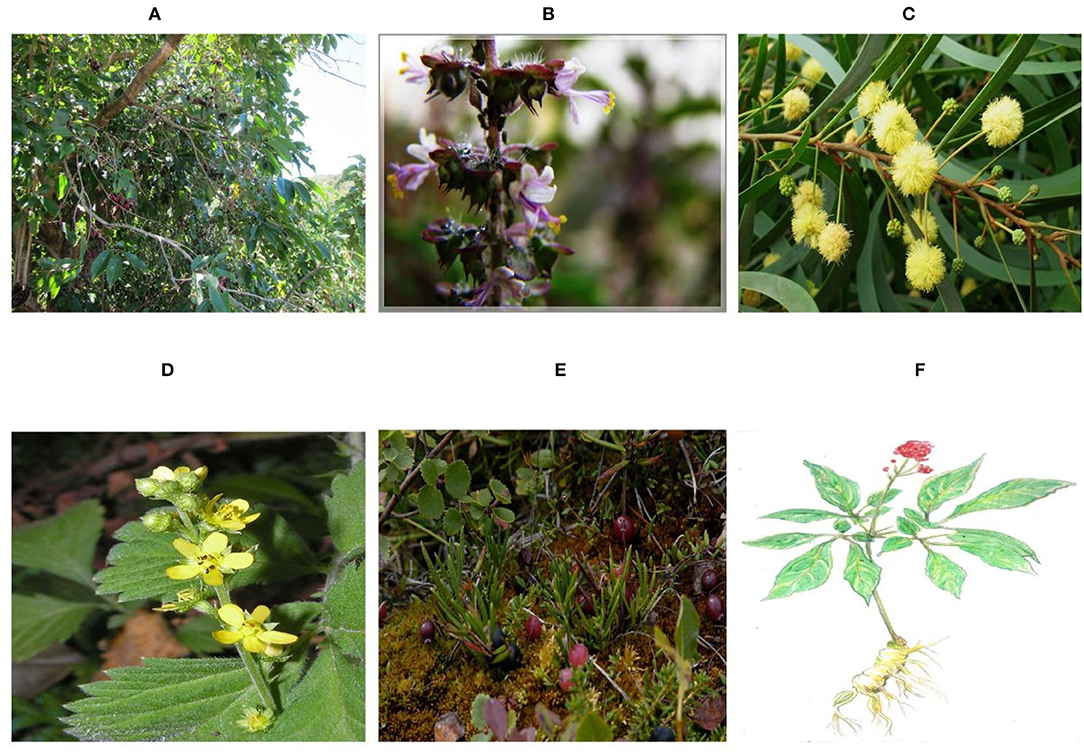
Figure 4. Plants in therapeutics to control avian infectious influenza. (A) Eugenia jambolana (99). (B) Ocimum sanctum (100). (C) Acacia Arabica (101). (D) Agrimonia pilosa (102). (E) cranberry (103), and (F) Ginseng (104).
The antiviral effect of the water extract of Psoraleae semen (WPS) has an auspicious role of novel anti-influenza. Choi et al. (113) have conducted a comprehensive study using RAW 264.7 and MDCK cells to assess the inhibitory effect of virus using 100 μg/ml in WPS. It was proven that WPS served as an immunomodulator and inhibitor of influenza HA and NA. Furthermore, they suggested that WPS can be a substantial alternativee as an antiviral therapeutic agent due to the disruption of infection via type I IFN-mediated signaling pathway involving RAW 264.7 cells. Similar findings were reported in which the anti-influenza effects of WPS produce direct inhibition of HA and NA mediation. The predominant role of Psoralen and bakuchiol in influenza and herpes simplex viruses was reported in WPS antiviral activity against many (114, 115). Recently, bakuchiol was found to exert anti-influenza viral activity via the activation of erythroid 2-related factor 2 (Nrf2) (116).
Oxidative stress tempted by infections of RNA virus may contribute to numerous features of viral disease pathogenicity including apoptosis, impairment of immune system, inflammation response, and body weight loss (117). One of these compounds, Yi-Zhi-Hao pellet (CYZH), is a known TCM that is used as an antiviral. Further, it induced the activation of Nrf2 and NF-κB, which subsequently upregulated heme oxygenase-1 (HO-1) expression. Also, CYZH protected cells from oxidative damage induced by reactive oxygen series. In conclusion, CYZH inhibits IAV replication in vitro, at least partly by activating the expression of the Nrf2/HO-1 pathway (118). Considering multiple components in CYZH, the other relevant mechanisms of anti-IAV are still needed to be considered in future study. Activation of the Nrf2/ARE pathway induces the expression of anti-inflammatory and anti-oxidative genes, such as HO-1, which is known to play a role in alleviating oxidative stress and tissue protection (119, 120). For example, previous studies have indicated that Nrf2 protect cells from the cytopathic effects of AIV, most likely by increasing the expression of antioxidant genes in human alveolar epithelial cells and modifies AIV entry and replication in nasal epithelial cells (121, 122). This mechanism further need to be studied in avian species in the future.
Sood et al. (123) found that extracts of Eugenia jambolana had a 100% virucidal effect against HPAIV H5N1 in tissue culture and ECE in ovo inoculation. In another study (124), Ocimum sanctum and Acacia Arabica crude extract and terpenoid isolated from the leaves of O. sanctum and polyphenol from A. Arabica have shown promising antiviral properties against H9N2 virus, showing significant virucidal activity. Future investigations are necessary to formulate combinations of these compounds for a broader antiviral activity against H9N2 viruses and evaluate them in chickens. The extracts of these plants were used on tissues in ovo, however; further study is needed in avian species. The extract of Agrimonia pilosa also exhibited a virucidal effect at a concentration of 160–570 ng/ml against influenza A and B viruses when the viruses were treated with the extract prior to plaque assay (125).
Luganini et al. (126) conducted research considering the direct action of cranberry extract against influenza virus and showed that the novel Oximacro of cranberry extract impedes two subtypes (A and B) of influenza viruses. During in vitro studies, they elaborated on the mechanism of Oximacro that prevented virus entry and attachment, leading to virucidal activities. In the past, the immune response, mainly humoral, in chickens during the inactivation of the vaccine for AIV has been induced by the addition of immunoadjuvants and extracts of plants in feed. Similarly, when the stems and leaves of Ginseng saponins were added to the drinking water, the humoral immune response increased to a significant level. In another such instance, serum Abs level enhancement was observed significantly when H. perforatum L. was administered orally and in the drinking water (127, 128). When the extract of ginseng after fermentation was administered to mice, the protection level against different subtypes (H1N1, H3N2, H5N1, and H7N9) of the influenza virus. Additionally, components of the adoptive immune system such as B cell, CD4, CD8, and major histocompatibility complex II were observed. However, detailed studies are needed in the future to explore the anti-influenza mechanism of ginseng in fermented form (129).
The efficacy of Sargassum pallidum polysaccharides (SPP) as adjuvant in inactivated vaccines of NDV, AIV, and IBV in chickens was tested by Li et al. (130). In that study, the vaccines containing 10, 30, and 50 mg SPP/ml were compared with the traditional oil adjuvant vaccines. Serum Ab titers against the three viruses significantly increased at the dose 30 mg/ml. Moreover, the CD4 content and T lymphocyte multiplication were enhanced in all treated groups.
Use of Nanotechnology to Control AIV
The application of various nanomaterials endorses the interactions between the materials and the virus, enabling other researchers to build a biosensing analyzer that should work effectively based on the portable electroanalysis, and perform exact detection of influenza virus (131). The use of nano-based vaccines has many advantages that include higher storage time, and the encapsulation of vaccines in NP that are polymers in solid form might assist to stabilize at room temperature, consideration of an alternate route of administration, and enabling precise discharge. Nano-vaccines might release soluble antigens that induce both types of immunity, i.e., humoral and cellular (132). In a similar way, NPs based on a chitosan derivative are used to deliver an immune response when administered into mucosal sites of poultry (Figure 5). Furthermore, a decrease in morbidity, mortality, and viral load is observed in chickens infected with IBV and AIV (133). Magnetic beads are also considered as nano beads and are used to identify signals at amplifion along with quartz crystal microbalance (QCM) apta sensors, the magnetic nano bead-amplified QCM immune-sensors have been used for the detection of the H5N1 protein (134). Silver nanoparticles (AgNPs) magnetic particles, and carbon-based materials are commonly used to analyze and identify the different subtypes of influenza viruses. The preparation of these particles is carried out based on the methodology already available in the literature (135, 136). Additionally, there are well-known techniques such as electrode-based well array, electrochemical quantitative systems, and on-chip nanomembrane tubular sensors of based on full integration (137, 138). Furthermore, mesoporous silica NPs performing functions with the amino group and naturally loaded with prodrugs of quercetin and shikimic acid discovered a novel antiviral nanoformulation that targets the detection of highly pathogenic avian influenza H5N1 virus. They induced also strong immunomodulatory effects: they limited the production of cytokines (IL-1β and TNF-α) and nitric oxide (NO) by 50%. Furthermore, it played an extraordinary role in the critical carrageenan-induced rat model to induce the antiinflammatory influence carried in vivo (38, 139). Thus, nanotechnology through the use of a variety of NPs and in the form of nano-vaccines, nanobodies and nanomedicine, along with the use of adjuvants, has a remarkable future perspective in the field of biomedicine to control avian infectious diseases.
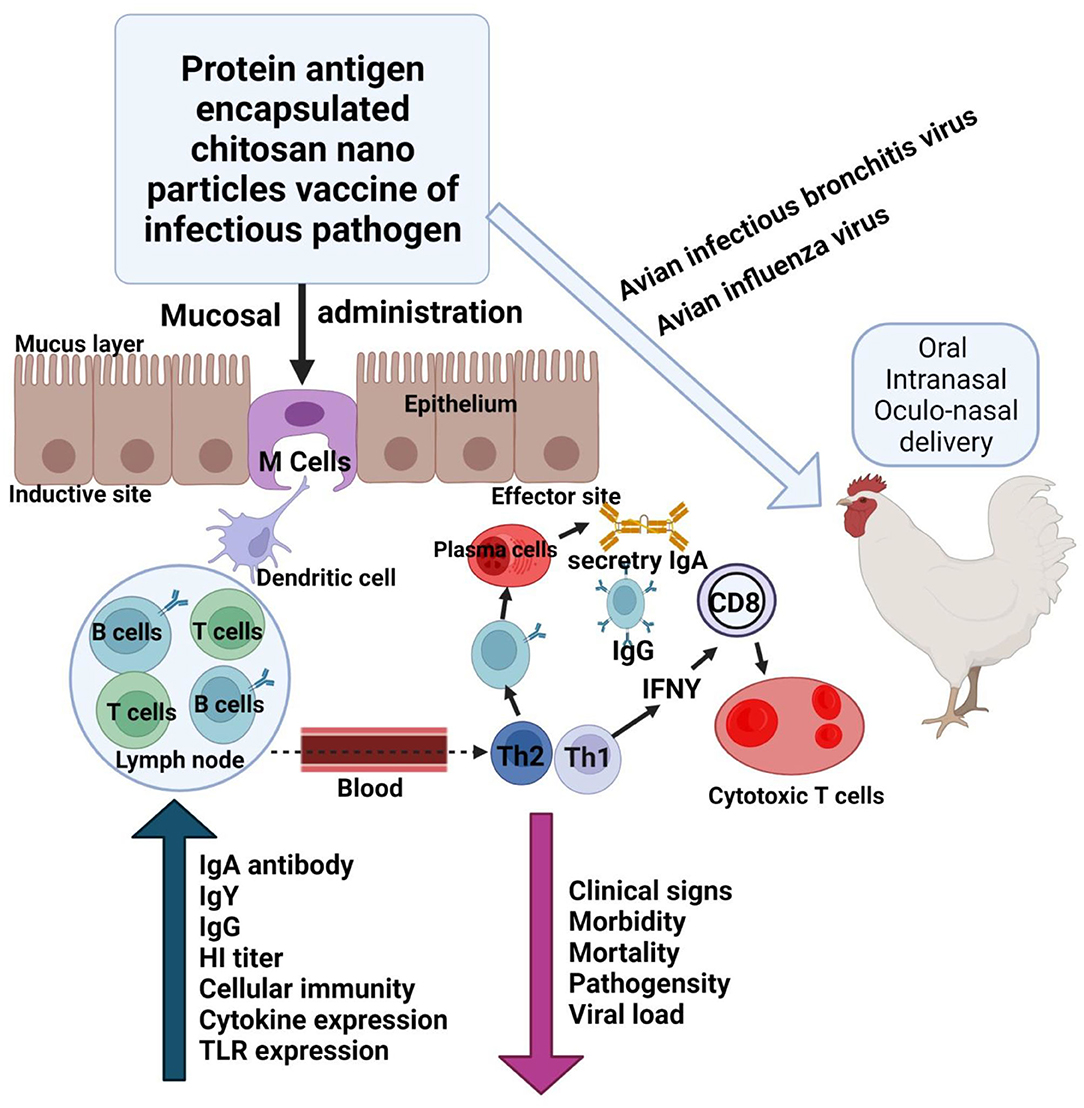
Figure 5. Schematic illustration of the use of nanoparticles (chitosan) to deliver vaccine during IB and avian influenza to mucosal sites to induce immune response. The modified figure was redrawn with permission from Renu and Renukaradhya (133). Image created with Biorender.com.
The control of IBV and AIV has faced many troubles due to several factors such as drug resistance, emergence of novel viral strains, and cross-species infections. Plant extracts can be a potential source to develop novel therapeutic medicine. The use of nanotechnology in terms of nano-vaccines, nanobodies, and nanomedicine might be very helpful to diagnose and control these poultry infectious diseases. In the future, the exact mechanism of action to counteract poultry infectious diseases caused by novel viral strains needs to be studied to develop control strategies.
Author Contributions
Conceptualization and writing—original draft: GA. Writing—review and editing: GA, GL, and JY. Supervision, project administration, and funding acquisition: GL. Validation: GL and JY. All authors agreed to the final version.
Funding
The National Natural Science Foundation of China under Grant (31172295 and 31272569) supported this research.
Conflict of Interest
The authors declare that the research was conducted in the absence of any commercial or financial relationships that could be construed as a potential conflict of interest.
Publisher's Note
All claims expressed in this article are solely those of the authors and do not necessarily represent those of their affiliated organizations, or those of the publisher, the editors and the reviewers. Any product that may be evaluated in this article, or claim that may be made by its manufacturer, is not guaranteed or endorsed by the publisher.
Acknowledgments
The China Scholarship Council is highly thanked for funding the stay of GA for conduction of his doctoral research. Images Credit to Biorender.com.
References
1. Hedman HD, Vasco KA, Zhang L. A. Review of antimicrobial resistance in poultry farming within low-resource settings. Animals (Basel). (2020) 10:1264. doi: 10.3390/ani10081264
2. Desta TT. Indigenous village chicken production: a tool for poverty alleviation, the empowerment of women, and rural development. Trop Anim Health Prod. (2021) 53:1–16. doi: 10.1007/s11250-020-02433-0
3. Hafez HM, Attia YA. Challenges to the poultry industry: current perspectives and strategic future after the COVID-19 outbreak. Front Vet Sci. (2020) 7:516. doi: 10.3389/fvets.2020.00516
4. De Boeck C, Kalmar I, Dumont A, Vanrompay D. Longitudinal monitoring for respiratory pathogens in broiler chickens reveals co-infection of Chlamydia psittaci and Ornithobacterium rhinotracheale. J Med Microbiol. (2015) 64:565–74. doi: 10.1099/jmm.0.000047
5. Nkukwana TT. Global poultry production: current impact and future outlook on the South African poultry industry. S Afr J Anim Sci. (2018) 48:869–84. doi: 10.4314/sajas.v48i5.7
6. Yadav MP, Singh RK, Malik YS. Epidemiological perspective in managing viral diseases in animals. In: Recent Advances in Animal Virology. Singapore: Springer (2019). p. 381–407.
7. Ali RN, Rubin H, Sarkar S. Countering the potential re-emergence of a deadly infectious disease—information warfare, identifying strategic threats, launching countermeasures. PLoS ONE. (2021) 16:e0256014. doi: 10.1371/journal.pone.0256014
8. Schalk AF, Hawn MC. An apparently new respiratory disease in baby chicks. J Am Vet Med Assoc. (1931) 78:413–22.
9. Cavanagh D. Coronavirus avian infectious bronchitis virus. Vet Res. (2007) 38:281–97. doi: 10.1051/vetres:2006055
10. Parvin R, Begum JA, Nooruzzaman M, Kabiraj CK, Chowdhury EH. Circulation of three genotypes and identification of unique mutations in neutralizing epitopes of infectious bronchitis virus in chickens in Bangladesh. Arch Virol. (2021) 166:3093–103. doi: 10.1007/s00705-021-05227-3
11. Mansour S, ElBakrey RM, Mohamed FF, Hamouda EE, Abdallah MS, Elbestawy AR, et al. Avian paramyxovirus type 1 in Egypt: epidemiology, evolutionary perspective, and vaccine approach. Front Vet Sci. (2021) 8:647462. doi: 10.3389/fvets.2021.647462
12. Toro H. Global control of infectious bronchitis requires replacing live attenuated vaccines by alternative technologies. Avian Dis. (2021) 65:635–40. doi: 10.1637/aviandiseases-D-21-00105
13. Sultan HA, Ali A, El Feil WK, Bazid AHI, Zain El-Abideen MA, et al. Protective efficacy of different live attenuated infectious bronchitis virus vaccination regimes against challenge with IBV variant-2 circulating in the Middle East. Front Vet Sci. (2019) 6:341. doi: 10.3389/fvets.2019.00341
14. Franzo G, Tucciarone CM, Blanco A, Nofrarías M, Biarnés M. Cortey Met, et al. Effect of different vaccination strategies on IBV QX population dynamics and clinical outbreaks. Vaccine. (2016) 34:5670–6. doi: 10.1016/j.vaccine.2016.09.014
15. Parvin R, Begum JA, Nooruzzaman M, Chowdhury EH, Islam MR, Vahlenkamp TW. Review analysis and impact of co-circulating H5N1 and H9N2 avian influenza viruses in Bangladesh. Epidemiol Infect. (2018) 146:1259–66. doi: 10.1017/S0950268818001292
16. Dhama K, Chauhan RS, Kataria JM, Mahendran M, Tomar S. Avian influenza: the current perspectives. Vet Immunol Immunopathol. (2005) 7:1–33.
17. Chen J, Deng YM. Influenza virus antigenic variation, host antibody production and new approach to control epidemics. Virol J. (2009) 6:1–3. doi: 10.1186/1743-422X-6-30
18. Ducatez MF, Webster RG, Webby RJ. Animal influenza epidemiology. Vaccine. (2008) 26:D67–9. doi: 10.1016/j.vaccine.2008.07.064
19. Yang H, Carney PJ, Mishin VP, Guo Z, Chang JC, Wentworth DE, et al. Molecular characterizations of surface proteins hemagglutinin and neuraminidase from recent H5Nx avian influenza viruses. Virol J. (2016) 90:5770–84. doi: 10.1128/JVI.00180-16
20. Blagodatski A, Trutneva K, Glazova O, Mityaeva O, Shevkova L, Kegeles E et al. Avian influenza in wild birds and poultry: Dissemination pathways, monitoring methods, and virus ecology. Pathogens. (2021) 10:630. doi: 10.3390/pathogens10050630
21. Soda K, Yamane M., Hidaka C, Miura K, Ung TT, Nguyen HL, et al. Prior infection with antigenically heterologous low pathogenic avian influenza viruses interferes with the lethality of the H5 highly pathogenic strain in domestic ducks. J Vet Med Sci. (2021) 83:1899–1906. doi: 10.1292/jvms.21-0515
22. Luo S, Xie Z, Li M, Li D, Xie L, Huang J, et al. Survey of low pathogenic avian influenza viruses in live poultry markets in Guangxi Province, Southern China, 2016–2019. Sci Rep. (2021) 11:1–0. doi: 10.1038/s41598-021-02639-8
23. Mansour SM, Mohamed FF, Eid AA, Mor SK, Goyal SM. Co-circulation of paramyxo-and influenza viruses in pigeons in Egypt. Avian Pathol. (2017) 46:367–75. doi: 10.1080/03079457.2017.1285391
24. Mohamed MEM, Ahmed HA, Erfan AM, Abdelkarim L, Awadallah MAI. Endemic status and zoonotic potential of avian influenza viruses in Egypt, 2006-2019. Adv Anim Vet Sci. (2019) 7:154–62.
25. Astill J, Dara RA, Fraser ED, Sharif S. Detecting and predicting emerging disease in poultry with the implementation of new technologies and big data: a focus on avian influenza virus. Front Vet Sci. (2018) 5:263. doi: 10.3389/fvets.2018.00263
26. Gilbert M, Xiao X, Robinson TP. Intensifying poultry production systems and the emergence of avian influenza in China: a “One Health/Ecohealth” epitome. Arch Public Health. (2017) 75:1–7. doi: 10.1186/s13690-017-0218-4
27. Manyi-Loh C, Mamphweli S, Meyer E. Okoh, A. Antibiotic use in agriculture and its consequential resistance in environmental sources: potential public health implications . Molecules. (2018) 23:795. doi: 10.3390/molecules23040795
28. Fuzimoto Andréa D, Ciro Isidoro. The antiviral and coronavirus-host protein pathways inhibiting properties of herbs and natural compounds-Additional weapons in the fight against the COVID-19 pandemic?. J Tradit Complement Med. (2020) 10:405–19. doi: 10.1016/j.jtcme.2020.05.003
29. Tagde P, Tagde S, Tagde P, Bhattacharya T, Monzur SM. Rahman et al. Nutraceuticals and herbs in reducing the risk and improving the treatment of COVID-19 by targeting SARS-CoV-2. Biomedicines. (2021) 9:1266. doi: 10.3390/biomedicines9091266
30. Hoang BX, Shaw G, Fang W, Han B. Possible application of high-dose vitamin C in the prevention and therapy of coronavirus infection. J Glob Antimicrob Resist. (2020) 23:256–62. doi: 10.1016/j.jgar.2020.09.025
31. Soccol CR, de Souza Vandenberghe LP, Spier MR, Medeiros AP, Yamaguishi CT, De Dea Lindner J, et al. The potential of probiotics: a review. Food Technol Biotechnol. (2010) 48:413–34.
32. Gadde U, Kim WH. Oh ST, Lillehoj HS. Alternatives to antibiotics for maximizing growth performance and feed efficiency in poultry: a review. Anim Health Res Rev. (2017) 18:26–45. doi: 10.1017/S1466252316000207
33. Patra AK, Amasheh S, Aschenbach JR. Modulation of gastrointestinal barrier and nutrient transport function in farm animals by natural plant bioactive compounds–a comprehensive review. Crit Rev Food Sci Nutr. (2019) 59:3237–66. doi: 10.1080/10408398.2018.1486284
34. Attia YA, Al-Harthi MA. Nigella seed oil as an alternative to antibiotic growth promoters for broiler Chickens. Europ Poult Sci. (2015) 79:1–13. doi: 10.1399/eps.2015.84
35. Wang W, Xu R, Li J. Production of native bispecific antibodies in rabbits. PLoS ONE. (2010) 5:e10879. doi: 10.1371/journal.pone.0010879
36. Pereira EP, Van Tilburg MF, Florean EO, Guedes MI. Egg yolk antibodies (IgY) and their applications in human and veterinary health: a review. Int Immunopharmacol. (2019) 1:293–303. doi: 10.1016/j.intimp.2019.05.015
37. El-Kaawy SA, Abbas AT, Sohrab SS, Tabll AA, Hassan AM, Iwata-Yoshikawa N, et al. Immunotherapeutic efficacy of IgY antibodies targeting the full-length spike protein in an animal model of middle east respiratory syndrome coronavirus infection. Pharmaceuticals. (2021) 14:511. doi: 10.3390/ph14060511
38. Neethirajan S. Recent advances in wearable sensors for animal health management. Sens Bio-Sens Res. (2017) 12:15–29. doi: 10.1016/j.sbsr.2016.11.004
39. Power AC, Gorey B, Chandra S, Chapman J. Carbon nanomaterials and their application to electrochemical sensors: a review. Nanotechnol Rev. (2018) 7:19–41. doi: 10.1515/ntrev-2017-0160
40. Saha A, Chowdhury MI, Nazim M, Alam MM, Ahmed T, Hossain MB, et al. Vaccine specific immune response to an inactivated oral cholera vaccine and EPI vaccines in a high and low arsenic area in Bangladeshi children. Vaccine. (2013) 31:647–52. doi: 10.1016/j.vaccine.2012.11.049
41. Scallan CD. Tingley DW, Lindbloom JD, Toomey JS, Tucker SN. An adenovirus-based vaccine with a double-stranded RNA adjuvant protects mice and ferrets against H5N1 avian influenza in oral delivery models. Clin Vaccine Immunol. (2013) 20:85–94. doi: 10.1128/CVI.00552-12
42. Brisse M, Vrba SM, Kirk N, Liang Y, Ly H. Emerging concepts and technologies in vaccine development. Front Immunol. (2020) 11:2578. doi: 10.3389/fimmu.2020.583077
43. Yan X, Zhou M, Yu S, Jin Z, Zhao K. An overview of biodegradable nanomaterials and applications in vaccines. Vaccine. (2020) 38:1096–104. doi: 10.1016/j.vaccine.2019.11.031
44. Shao W, Li X, Goraya MU, Wang S, Chen JL. Evolution of influenza a virus by mutation and re-assortment. Int J Mol Sci. (2017) 18:1650. doi: 10.3390/ijms18081650
45. Wimmer R,. Thymus Vulgaris. (2016). Available online at: https://www.flickr.com/photos/ralf_wimmer/32777148505 (accessed June 3, 2022).
46. Star K, Star, F,. Mentha Piperita. (2006). Available online at: https://www.flickr.com/photos/ralf_wimmer/32777148505 (accessed June 3, 2022).
47. Mayer J,. Desmodium Canadense. (2013). Available online at: https://www.flickr.com/photos/wackybadger/9382889455 (accessed June 3, 2022).
48. Tanne A,. Hypericum Perforatum. (2007). Available online at: https://www.flickr.com/photos/annetanne/538464711 (accessed June 3, 2022).
49. Hodgkins R,. Sambucus Nigra. (2020). Available online at: https://www.flickr.com/photos/131806380@N05/50097507842 (accessed June 3, 2022).
50. Star K, Star, F,. Allium Sativum (Garlic). (2007). Available online at: https://www.flickr.com/photos/starr-environmental/24522811579 (accessed June 3, 2022).
51. Valke D,. Achyranthes Aspera. (2008). Available online at: https://www.flickr.com/photos/dinesh_valke/2891857287 (accessed June 3, 2022).
52. Star K, Star, F,. Panicum Antidotale. (2000). Available online at: https://www.flickr.com/photos/starr-environmental/23903761613 (accessed June 3, 2022).
53. Lelešius R, Karpovaite A, Mickiene R, Drevinskas T, Tiso N, RagaŽinskiene O, et al. In vitro antiviral activity of fifteen plant extracts against avian infectious bronchitis virus. BMC Vet Res. (2019) 15:1–10. doi: 10.1186/s12917-019-1925-6
54. Zhou SF, Lai X. An update on clinical drug interactions with the herbal antidepressant St. John's wort Curr Drug Metab. (2008) 9:394–409. doi: 10.2174/138920008784746391
55. Chen H, Feng R, Muhammad I, Abbas G, Zhang Y, Ren Y, et al. Protective effects of hypericin against infectious bronchitis virus induced apoptosis and reactive oxygen species in chicken embryo kidney cells. Poult Sci. (2019) 98:6367–77. doi: 10.3382/ps/pez465
56. Barnes J, Arnason JT. Roufogalis BD. St John's wort (Hypericum perforatum L): botanical, chemical, pharmacological and clinical advances. J Pharm Pharmacol. (2019) 71:1–3. doi: 10.1111/jphp.13053
57. Chen H, Muhammad I, Zhang Y, Ren Y, Zhang R, Huang X, et al. Antiviral activity against infectious bronchitis virus and bioactive components of hypericum perforatum L. Front Pharmacol. (2019) 10:1272. doi: 10.3389/fphar.2019.01272
58. Chen C, Zuckerman DM, Brantley S, Sharpe M, Childress K, Hoiczy E, et al. Sambucus nigra extracts inhibit infectious bronchitis virus at an early point during replication. BMC Vet Res. (2014) 10:1–12. doi: 10.1186/1746-6148-10-24
59. Kothari D, Lee WD, Niu KM, Kim SK. The genus Allium as poultry feed additive: a review. Animals. (2019) 9:1032. doi: 10.3390/ani9121032
60. Shojai TM, Langeroudi AG, Karimi V, Barin A. and Sadri, N. The effect of Allium sativum (Garlic) extract on infectious bronchitis virus in specific pathogen free embryonic egg Avicenna. J Phytomed. (2016) 6:458.
61. Donma MM, Donma O. The effects of allium sativum on immunity within the scope of COVID-19 infection. Med hypotheses. (2020) 144:109934. doi: 10.1016/j.mehy.2020.109934
62. Pourhossein Z, Qotbi AAA, Seidavi A, Laudadio V, Centoducati G, Tufarelli V. Effect of different levels of dietary sweet orange (Citrus sinensis) peel extract on humoral immune system responses in broiler chickens. Anim Sci J. (2015) 86:105–10. doi: 10.1111/asj.12250
63. Shahzad MI, Anwar S, Ashraf H, Manzoor A, Naseer M, Rani U, et al. Antiviral activities of Cholistani plants against common poultry viruses. Trop Biomed. (2020) 37:129–40. doi: 10.47665/tb.37.4.1129
64. Aslam A, Shahzad MI, Parveen S, Ashraf H, Naz N. Zehra SS, et al. Evaluation of antiviral potential of different Cholistani plants against infectious bursal disease and infectious bronchitis virus. Pak Vet J. (2016) 36:302–6. Available online at: http://www.pvj.com.pk
65. Duschatzky CB, Possetto ML, Talarico LB, Garcia CC, Michis F, Almeida NV, et al. Evaluation of chemical and antiviral properties of essential oils from South American plants. Antivir Chem Chemother. (2005) 16:247–51. doi: 10.1177/095632020501600404
66. Koch C, Reichling J, Kehm R, Sharaf MM, Zentgraf H, Schneele J, et al. Efficacy of anise oil, dwarf-pine oil and chamomile oil against thymidine-kinase-positive and thymidine-kinase-negative herpesviruses. J Pharm Pharmacol. (2008) 60:1545–50. doi: 10.1211/jpp.60.11.0017
67. Jackwood MW, Rosenbloom R, Petteruti M, Hilt DA, McCall AW, Williams SM. Avian coronavirus infectious bronchitis virus susceptibility to botanical oleoresins and essential oils in vitro and in vivo. Virus Res. (2010) 149:86–94. doi: 10.1016/j.virusres.2010.01.006
68. Abd El-Ghany WA, Shaalan M, Salem HM. Nanoparticles applications in poultry production: an updated review. Poult Sci J. (2021) 77:1001–25. doi: 10.1080/00439339.2021.1960235
69. Youssef FS, El-Banna HA, Elzorba HY, Galal AM. Application of some nanoparticles in the field of veterinary medicine. Int J Vet Sci. (2019) 7:78–93. doi: 10.1080/23144599.2019.1691379
70. Krishnan S, Dusane A, Morajkar R, Venkat A, Vernekar A. Deciphering the role of nanostructured materials in the point-of-care diagnostics for COVID-19: a compre- hensive review. J Mater Chem B. (2021) 9:5967–81. doi: 10.1039/D1TB01182K
71. Ramos AP, Cruz MA, Tovani CB, Ciancaglini P. Bio- medical applications of nanotechnology. Biophys Rev. (2017) 9:79–89. doi: 10.1007/s12551-016-0246-2
72. Chen YN, Hsueh YH, Hsieh CT, Tzou DY, Chang PL. Antiviral activity of graphene–silver nanocomposites against non-enveloped and enveloped viruses. Int J Environ Res Public Health. (2016) 13:430. doi: 10.3390/ijerph13040430
73. Li J, Helal ZH, Karch CP, Mishra N, Girshick T, Garmendia A, et al. A self-adjuvanted nano-particle-based vaccine against infectious bronchitis virus. PLoS ONE. (2018) 13:e0203771. doi: 10.1371/journal.pone.0203771
74. Chandrasekar SS, Kingstad-Bakke B, Wu CW, Suresh M, Talaat AM. A novel mucosal adjuvant system for immunization against avian coronavirus causing infectious bronchitis. J Virol. (2020) 94:e01016–e1020. doi: 10.1128/JVI.01016-20
75. Liu J, Li R, Yang B. Carbon dots: a new type of carbon-based nanomaterial with wide applications. ACS Cent Sci. (2020) 6:2179–95. doi: 10.1021/acscentsci.0c01306
76. Chou DL, Mao JY, Anand A, Lin HJ, Lin JHY, Tseng CP, et al. Carbonized lysine-nanogels protect against infectious bronchitis virus. Int J Mol Sci. (2021) 22:5415. doi: 10.3390/ijms22115415
77. Kang J, Tahir A, Wang H. Chang J. Applications of nanotechnology in virus detection, tracking, and infection mechanisms Wiley Interdiscip. Rev Nanomed. (2021) 13:1700. doi: 10.1002/wnan.1700
78. Ahmed SR, Kang SW, Oh S, Lee J, Neethirajan S. Chiral zirconium quantum dots: a new class of nanocrystals for optical detection of coronavirus. Heliyon. (2018) 4:e00766. doi: 10.1016/j.heliyon.2018.e00766
79. Ahmed SR, Nagy É, Neethirajan S. Self-assembled star-shaped chiroplasmonic gold nanoparticles for an ultrasensitive chiro-immunosensor for viruses. RSC Adv. (2017) 7:40849–57. doi: 10.1039/C7RA07175B
80. Nasrollahzadeh M, Sajjadi M, Soufi GJ, Iravani S, Varma RS. Nanomaterials and nanotechnology-associated innovations against viral infections with a focus on coronaviruses. Nanomaterials. (2020) 10:1072. doi: 10.3390/nano10061072
81. Kato T, Takami Y, Deo VK, Park EY. Preparation of virus-like particle mimetic nanovesicles displaying the S protein of Middle East respiratory syndrome coronavirus using insect cells. J Biotechnol. (2019) 306:177–84. doi: 10.1016/j.jbiotec.2019.10.007
82. Chen HW, Huang CY, Lin SY, Fang ZS, Hsu CH, Lin JC, et al. Synthetic virus-like particles prepared via protein corona formation enable effective vaccination in an avian model of coronavirus infection. Biomaterials. (2016) 106:111–8. doi: 10.1016/j.biomaterials.2016.08.018
83. Liu G, Lv L, Yin L, Li X, Luo D, Liu K, et al. Assembly and immunogenicity of coronavirus-like particles carrying infectious bronchitis virus M and S proteins. Vaccine. (2013) 31:5524–30. doi: 10.1016/j.vaccine.2013.09.024
84. Wang C, Zheng X, Gai W, Zhao Y, Wang H, Wang H, et al. MERS-CoV virus-like particles produced in insect cells induces specific humoural and cellular imminity in rhesus macaques. Oncotarget. (2017) 8:12686. doi: 10.18632/oncotarget.8475
85. García R, Hussain A, Koduru P, Atis M, Wilson K, Park JY, et al. Identification of potential antiviral compounds against SARS-CoV-2 structural and non-structural protein targets: a pharmacoinformatics study of the CAS COVID-19 dataset. Comput Biol Med. (2021) 133:104364. doi: 10.1016/j.compbiomed.2021.104364
86. Nefedova E, Koptev V, Bobikova AS, Cherepushkina V, Mironova T, Afonyushkin V et al. the infectious bronchitis coronavirus pneumonia model presenting a novel insight for the SARS-CoV-2 dissemination route. Vet Sci. (2021) 8:239. doi: 10.3390/vetsci8100239
87. Li B-H, Li ZY, Liu MM, Tian JZ, Cui QH. Progress in traditional chinese medicine against respiratory viruses: a review. Front Pharmacol. (2021) 12:743623. doi: 10.3389/fphar.2021.743623
88. Feng H, Wang X, Zhang J, Zhang K, Zou W, Zhang K, et al. Combined effect of Shegandilong granule and Doxycycline on immune responses and protection against Avian Coronavirus in broilers. Front Vet Sci. (2021) 1:1407. doi: 10.3389/fvets.2021.756629
89. Li J, Yin J, Sui X, Li G, Ren X. Comparative analysis of the effect of glycyrrhizin diammonium and lithium chloride on infectious bronchitis virus infection in vitro. Avian Pathol. (2009) 38:215–21. doi: 10.1080/03079450902912184
90. Harrison SM, Tarpey I, Rothwell L, Kaiser P. and Hiscox, JA. Lithium chloride inhibits the coronavirus infectious bronchitis virus in cell culture. Avian Pathol. (2007) 36:109–14. doi: 10.1080/03079450601156083
91. Song JM, Park KD, Lee KH, Byun YH, Park JH, Kim SH, et al. Biological evaluation of anti-influenza viral activity of semi-synthetic catechin derivatives. Antiviral Res. (2007) 76:178–85. doi: 10.1016/j.antiviral.2007.07.001
92. Tsukamoto Y, Nakano Y, Adachi K. Protection against infectious bronchitis virus, a corona virus infection, using ostrich antibodies. Health. (2018) 10:1294. doi: 10.4236/health.2018.1010100
93. Dong G, Zheng L, Huang SH, Gao J, Zuo Y. Amino acid reduction can help to improve the identification of antimicrobial peptides and their functional activities. Front in Genet. (2021) 12:549. doi: 10.3389/fgene.2021.669328
94. Sun Q, Wang K, She R, Ma W, Peng F, Jin H. Swine intestine antimicrobial peptides inhibit infectious bronchitis virus infectivity in chick embryos. Poult Sci. (2010) 89:464–9. doi: 10.3382/ps.2009-00461
95. Gupta A, Gupta GS. Status of mannose-binding lectin (MBL) and complement system in COVID-19 patients and therapeutic applications of antiviral plant MBLs. Mol Cell Biochem. (2021) 476:2917–42. doi: 10.1007/s11010-021-04107-3
96. Zhang W, Bouwman KM, van Beurden SJ, Ordonez SR, van Eijk M, Haagsman HP, et al. Chicken mannose binding lectin has antiviral activity towards infectious bronchitis virus. Virol. (2017) 509:252–9. doi: 10.1016/j.virol.2017.06.028
97. Abid DS, Shihab NL, Kamounah FS. Synthesis, characterization and structure activity relationship analysis of N-acetyl-2-substituted phenyl thiazolidine4-carboxylic acids derivatives as neuraminidase inhibitors. J Chem Pharm Res. (2014) 6:845–54.
98. Musaddiq S, Shahzad MI, Firdous F, Iqbal A, Tanveer M, Ashraf A, et al. Thiazolidines: Potential anti-viral agents against avian influenza and infectious bronchitis viruses. Vet Res Forum. (2020) 11:415–21. doi: 10.30466/vrf.2018.91264.2211
99. Vorontsova M,. Eugenia Jambolana. (2012). Available online at: https://www.flickr.com/photos/36803481@N06/7064471011 (accessed June 3, 2022).
100. Yaddanapudi P,. Ocimum Sanctum. (2008). Available online at: https://www.flickr.com/photos/neychurluvr/2844691743 (accessed June 3, 2022).
101. Eickhoff D,. Acacia Arabica/ Accacia koaia. (2006). Available online at: https://www.flickr.com/photos/dweickhoff/4765979829 (accessed June 3, 2022).
102. Aqiao HQ,. Agrimonia Pilosa. (2011). Available online at: https://www.flickr.com/photos/nhq9801/9216086004 (accessed June 3, 2022).
103. Pokrzywinski A,. Bog Cranberrys. (2006). Available online at: https://www.flickr.com/photos/andreagp/1516652119 (accessed June 3, 2022).
104. Waller W,. Ginseng. (2009). Available online at: https://www.flickr.com/photos/whitneywaller/3162085912 (accessed June 3, 2022).
105. Pizzorno A, Padey B, Terrier O, Rosa-Calatrava M. Drug repurposing approaches for the treatment of influenza viral infection: reviving old drugs to fight against a long-lived enemy. Front Immunol. (2019) 10:531. doi: 10.3389/fimmu.2019.00531
106. Yuan S. Drugs to cure avian influenza infection–multiple ways to prevent cell death. Cell Death Dis. (2013) 4:e835–e835. doi: 10.1038/cddis.2013.367
107. Loregian A, Mercorelli B, Nannetti G, Compagnin C. Palù, G. Antiviral strategies against influenza virus: towards new therapeutic approaches. Cell Mol Life Sci. (2014) 71:3659–83. doi: 10.1007/s00018-014-1615-2
108. Luo Z, Liu LF, Wang XH Li W, Jie C, Chen H, et al. Epigoitrin, an alkaloid from Isatis indigotica, reduces H1N1 infection in stress-induced susceptible model in vivo and in vitro. Front in pharm. (2019) 10:78. doi: 10.3389/fphar.2019.00078
109. Kurokawa M, Kumeda CA, Yamamura J, Kamiyama T, Shiraki K. Antipyretic activity of cinnamyl derivatives and related compounds in influenza virus-infected mice. Eur J Pharmacol. (1998) 348:45–51. doi: 10.1016/S0014-2999(98)00121-6
110. Grienke U, Richter M, Walther E, Hoffmann A, Kirchmair J, Makarov V, et al. Discovery of prenylated flavonoids with dual activity against influenza virus and Streptococcus pneumoniae. Sci Rep. (2016) 6:27156. doi: 10.1038/srep27156
111. Gangehei L, Ali M, Zhang W, Chen Z, Wakame K, Haidari M. Oligonol a low molecular weight polyphenol of lychee fruit extract inhibits proliferation of influenza virus by blocking reactive oxygen species-dependent ERK phosphorylation. Phytomedicine. (2010) 17:1047–56. doi: 10.1016/j.phymed.2010.03.016
112. Derksen A, Hensel A, Hafezi W, Herrmann F, Schmidt TJ, Ehrhardt C, et al. 3-O-Galloylated procyanidins from Rumex acetosa L. inhibit the attachment of influenza A virus. PLoS ONE. (2014) 9:e110089. doi: 10.1371/journal.pone.0110089
113. Choi JG, Jin YH, Kim JH, Oh TW, Yim NH, Cho WK, et al. In vitro anti-viral activity of psoraleae semen water extract against influenza a viruses. Front Pharmacol. (2016) 7:460. doi: 10.3389/fphar.2016.00460
114. Nakashima K, Chanda PK, Deutsch V, Banerjee AK, Shatkin AJ. Inactivation of influenza and vesicular stomatitis virion RNA polymerase activities by photoreaction with 4 0 -substituted psoralens. J Virol. (1979) 32:838–44. doi: 10.1128/jvi.32.3.838-844.1979
115. Redfield DC, Richman DD, Oxman MN, Kronenberg LH. Psoralen inactivation of influenza and herpes simplex viruses and of virus- infected cells. Infect Immun. (1981) 32:1216–26. doi: 10.1128/iai.32.3.1216-1226.1981
116. Shoji M, Arakaki Y, Esumi T, Kohnomi S, Yamamoto C, Suzuki Y, et al. Bakuchiol Is a phenolic isoprenoid with novel enantiomer-selective anti-influenza A virus activity involving Nrf2 activation. J Biol Chem. (2015) 290:28001–17. doi: 10.1074/jbc.M115.669465
117. He YW, Dong CZ, Zhao JY, Ma LL Li YH, Aisa HA. 1,2,3-Triazole- containing derivatives of rupestonic acid: click-chemical synthesis and antiviral activities against influenza viruses. Eur J Med Chem. (2014) 76:245–55. doi: 10.1016/j.ejmech.2014.02.029
118. Loboda A, Damulewicz M, Pyza E, Jozkowicz A, Dulak J. Role of Nrf2/HO-1 system in development, oxidative stress response and diseases: an evolutionarily conserved mechanism. Cell Mol Life Sci. (2016) 73:3221–47. doi: 10.1007/s00018-016-2223-0
119. Wegiel B, Nemeth Z, Correa-Costa M, Bulmer AC, Otterbein LE. Heme oxygenase-1: a metabolic nike. Antioxid Redox Signal. (2014) 20:1709–22. doi: 10.1089/ars.2013.5667
120. Kosmider B, Messier EM, Janssen WJ, Nahreini P, Wang J, Hartshorn KL, et al. Nrf2 protects human alveolar epithelial cells against injury induced by influenza A virus. Respir Res. (2012) 13:43. doi: 10.1186/1465-9921-13-43
121. Kesic MJ, Simmons SO, Bauer R, Jaspers I. Nrf2 expression modifies influenza A entry and replication in nasal epithelial cells. Free Radic Biol Med. (2011) 51:444–53. doi: 10.1016/j.freeradbiomed.2011.04.027
122. Yin J, Ma L, Wang H, Yan H, Hu J, Jiang W, et al. Chinese herbal medicine compound Yi-Zhi-Hao pellet inhibits replication of influenza virus infection through activation of heme oxygenase-1. Acta Pharmaceutica Sinica B. (2017) 7:630–7. doi: 10.1016/j.apsb.2017.05.006
123. Sood R. Swarup D, Bhatia S, Kulkarni DD, Dey S, Saini M, et al. Antiviral activity of crude extracts of Eugenia jambolana Lam against highly pathogenic avian influenza (H5N1) virus. Indian J Exp Biol. (2012) 50:179–86.
124. Ghoke SS, Sood R, Kumar N, Pateriya AK, Bhatia S, Mishra A. et al. “Evaluation of antiviral activity of Ocimum sanctum and Acacia arabica leaves extracts against H9N2 virus using embryonated chicken egg model” BMC Complement Altern Med. (2018) 18:1–10. doi: 10.1186/s12906-018-2238-1
125. Shin WJ, Lee KH, Park MH, Seong BL. Broad-spectrum antiviral effect of Agrimonia pilosa extract on influenza viruses. Microbiol Immunol. (2010) 54:11–9. doi: 10.1111/j.1348-0421.2009.00173.x
126. Luganini A, Terlizzi ME, Catucci G, Gilardi G, Maffei ME, Gribaudo G. The cranberry extract oximacro® exerts in vitro virucidal activity against influenza virus by interfering with hemagglutinin. Front Microbiol. (2018) 9:1826. doi: 10.3389/fmicb.2018.01826
127. Zhai L, Li Y, Wang W, Hu S. Enhancement of humoral immune responses to inactivated Newcastle disease and avian influenza vaccines by oral administration of ginseng stem-and-leaf saponins in chickens. Poult Sci. (2011) 90:1955–9. doi: 10.3382/ps.2011-01433
128. Jiang W, Liu Y, Zheng H, Zheng Y, Xu H, Lu H. Immune regulation of avian influenza vaccine in hens using Hypericum perforatum L methanol extraction. Plant Omics. (2012) 5:40–5. doi: 10.3316/informit.007871356075970
129. Wang Y, Jung YJ, Kim KH, Kwon Y, Kim YJ. Zhang, Z, et al. Antiviral activity of fermented ginseng extracts against a broad range of influenza viruses. Viruses. (2018) 10:471. doi: 10.3390/v10090471
130. Li LJ, Ming YL Li YT, Feng JJ, Hao FQ, Zhang L. Adjuvant activity of Sargas-sum pallidum polysaccharides against combined Newcastle disease, infectious bronchitis and avian influenza inactivated vaccines. Mar Drugs. (2012) 10:2648–60. doi: 10.3390/md10122648
131. Moulick A, Richtera L, Milosavljevic V, Cernei N, Haddad Y, Zitka O, et al. Advanced nanotechnologies in avian influenza: current status and future trends–a review. Anal Chim Acta. (2017) 983:42–53. doi: 10.1016/j.aca.2017.06.045
132. Chen YC, Cheng HF, Yang YC, Yeh MK. Nanotechnologies applied in biomedical vaccines. Intech Open. (2016) 24:69547. doi: 10.5772/intechopen.69547
133. Renu S. and Renukaradhya GJ. Chitosan nanoparticle based mucosal vaccines delivered against infectious diseases of poultry and pigs. Front Bioeng Biotechnol. (2020) 8:558349. doi: 10.3389/fbioe.2020.558349
134. Brockman L, Wang R, Lum J, Li Y. QCM aptasensor for rapid and specific detection of avian influenza virus. Open J Appl Biosens. (2013) 2:97–103. doi: 10.4236/ojab.2013.24013
135. Mokhtarzadeh A, Eivazzadeh-Keihan R, Pashazadeh P, Hejazi M, Gharaatifar N, Hasanzadeh M, et al. Nanomaterial-based biosensors for detection of pathogenic virus. Trends Anal Chem. (2017) 97:445–57. doi: 10.1016/j.trac.2017.10.005
136. Boroumand H, Badie F, Mazaheri S, Seyedi ZS, Nahand JS, Nejati M, et al. Chitosan-based nanoparticles against viral infections. Front Cell Infect Microbiol. (2021) 11:175. doi: 10.3389/fcimb.2021.643953
137. Krejcova L, Hynek D, Adam V, Hubalek J, Kizek R. Electrochemical sensors and biosensors for influenza detection. Int J Electrochem Sci. (2012) 7:10779–801.
138. Cha MS, Lee JK, Cho SH, Park JG, Lee HY, Lim SH, et al. Quantitative analysis of H5N1 DNA hybridization on nanowell array electrode. J Nanosci Nanotechnol. (2013) 13:5245–9. doi: 10.1166/jnn.2013.7531
139. AbouAitah K, Swiderska-Sroda A, Kandeil A, Salman AM, Wojnarowicz J. Ali, MA, et al., Virucidal action against avian influenza H5N1 virus and immunomodulatory effects of nanoformulations consisting of mesoporous silica nanoparticles loaded with natural prodrugs. Int J Nanomedicine. (2020) 15:518. doi: 10.2147/IJN.S247692
Keywords: therapeutic agents, infectious bronchitis, avian influenza, herbal medicine, nanotechnology, infectious diseases control
Citation: Abbas G, Yu J and Li G (2022) Novel and Alternative Therapeutic Strategies for Controlling Avian Viral Infectious Diseases: Focus on Infectious Bronchitis and Avian Influenza. Front. Vet. Sci. 9:933274. doi: 10.3389/fvets.2022.933274
Received: 30 April 2022; Accepted: 08 June 2022;
Published: 22 July 2022.
Edited by:
Asghar Abbas, Muhammad Nawaz Shareef University of Agriculture, PakistanReviewed by:
Yasir Waqas, Cholistan University of Veterinary and Animal Sciences, PakistanMuhammad Mahboob Ali Hamid, University of Agriculture, Faisalabad, Pakistan
Muhammad Ameen Jamal, Kunming Institute of Zoology (CAS), China
Copyright © 2022 Abbas, Yu and Li. This is an open-access article distributed under the terms of the Creative Commons Attribution License (CC BY). The use, distribution or reproduction in other forums is permitted, provided the original author(s) and the copyright owner(s) are credited and that the original publication in this journal is cited, in accordance with accepted academic practice. No use, distribution or reproduction is permitted which does not comply with these terms.
*Correspondence: Guangxing Li, ligx@neau.edu.cn