- 1Institute of Pharmacology, Toxicology and Pharmacy, Ludwig-Maximilians-University, Munich, Germany
- 2Institute for Laboratory Animal Science, Hannover Medical School, Hanover, Germany
- 3Office for Animal Welfare and 3Rs, University of Zurich, Zurich, Switzerland
Several studies suggested an informative value of behavioral and grimace scale parameters for the detection of pain. However, the robustness and reliability of the parameters as well as the current extent of implementation are still largely unknown. In this study, we aimed to systematically analyze the current evidence-base of grimace scale, burrowing, and nest building for the assessment of post-surgical pain in mice and rats. The following platforms were searched for relevant articles: PubMed, Embase via Ovid, and Web of Science. Only full peer-reviewed studies that describe the grimace scale, burrowing, and/or nest building as pain parameters in the post-surgical phase in mice and/or rats were included. Information about the study design, animal characteristics, intervention characteristics, and outcome measures was extracted from identified publications. In total, 74 papers were included in this review. The majority of studies have been conducted in young adult C57BL/6J mice and Sprague Dawley and Wistar rats. While there is an apparent lack of information about young animals, some studies that analyzed the grimace scale in aged rats were identified. The majority of studies focused on laparotomy-associated pain. Only limited information is available about other types of surgical interventions. While an impact of surgery and an influence of analgesia were rather consistently reported in studies focusing on grimace scales, the number of studies that assessed respective effects was rather low for nest building and burrowing. Moreover, controversial findings were evident for the impact of analgesics on post-surgical nest building activity. Regarding analgesia, a monotherapeutic approach was identified in the vast majority of studies with non-steroidal anti-inflammatory (NSAID) drugs and opioids being most commonly used. In conclusion, most evidence exists for grimace scales, which were more frequently used to assess post-surgical pain in rodents than the other behavioral parameters. However, our findings also point to relevant knowledge gaps concerning the post-surgical application in different strains, age levels, and following different surgical procedures. Future efforts are also necessary to directly compare the sensitivity and robustness of different readout parameters applied for the assessment of nest building and burrowing activities.
Introduction
The detection and scoring of post-surgical pain in laboratory animals are of particular relevance for several reasons. First, they are a prerequisite for severity assessment in studies with surgical interventions, which provides the basis for ethical justification and consideration prior to the conduct of the study and for an evidence-based retrospective evaluation (1, 2). Second, reliable pain assessment is required for decisions about the necessity of analgesia and the choice of an appropriate analgetic regimen. In animals receiving analgesic drugs, scoring pain enables controlling for therapeutic efficacy, thereby providing a basis for additional rescue analgesia in individual animals and for refinement measures including an adjustment of the pain management. In this context, rescue analgesia refers to the treatment of breakthrough pain by administering additional analgesics, either increasing the dose, using a different route of administration, or adding a more potent analgesic.
Moreover, pain assessment is a prerequisite for the application of humane endpoints in various animal models. Finally, it should be considered that different degrees of uncontrolled pain contribute to the variance of data obtained in animal models, thereby increasing the number of animals needed. Resultantly, uncontrolled or insufficiently controlled pain can significantly affect various readout parameters and can therefore restrict study quality (3–5). In this context, manifold effects need to be considered when including effects on the neuroendocrine, immune, cardiovascular, respiratory, autonomous, and central nervous systems.
Thus, the precise detection and scoring of pain are crucial prerequisites for the consequent application of reduction and refinement concepts in laboratory animal science, as defined in the 3R principles (6).
Unfortunately, despite strong efforts to develop and validate methods and techniques for pain detection and scoring, a highly reliable and reproducible pain assessment strategy in daily laboratory practice is yet to be achieved. This is related to various challenges that one has to face when it comes to pain assessment in different animal species. These challenges start with the distinction between nociception and pain. Whereas nociception describes signal transduction from the specialized sensory cellular nociceptors to the central nervous system, pain in animals is considered as an “aversive, sensory experience representing awareness by the animal of damage or threat to the integrity of its tissues (note that there might not be any damage). It changes the animal's physiology and behavior to reduce or avoid the damage, to reduce the likelihood of its recurrence and to promote recovery” (7). When evaluating biochemical and physiological parameters in animals, it needs to be considered that some of the parameters among others, including substance P, cardiovascular, and respiratory parameters, might be modulated by nociception even when the conscious experience of pain is prevented by pharmacological measures.
When it comes to pain assessment, another major challenge is related to the fact that prey animals should rather avoid displaying symptoms of pain and suffering (8–10). In laboratory animals, this prey animal effect has been demonstrated, for example, in mice, rats, rabbits, and sheep (11, 12). Thus, the observer's presence may exert an influence and, consequently, familiarity with the observer is considered advantageous.
Agreement exists that composite measure schemes combining various physiological, endocrine, and behavioral parameters need to be applied to assess pain in laboratory mice and rats as reliably as possible (10). Several reviews summarized and discussed the informative value of various pain assessment methods (10, 13–18). For instance, as highlighted by Turner et al. (10) in their wide-ranging review, different behavioral parameters have been implemented for the analysis of pain in laboratory rodents. Ethograms have, for instance, been described and validated for mice and rats that capture information about the occurrence of pain-associated patterns, such as writhing and back-arching, and about the reduction or loss of normal species-specific behavioral patterns such as grooming and rearing (19–26). Behavioral patterns that can be reduced as a consequence of pain also include the interaction with nesting or burrowing material. Both, nest building and burrowing are evolutionary preserved activities in rodents that are considered non-essential in the laboratory animal facility environment (8, 9). The particular sensitivity of nest building and burrowing activity to pain, including post-surgical pain, has been reported in various studies (8, 27–31). In this context, it should also be considered that several studies provided evidence that mice and rats have various species-specific behavioral needs, and when these are not fulfilled, abnormal behavioral patterns can occur (32). Along this line, pain, sickness, and compromised welfare can exert effects on species-specific behavioral patterns.
As another valuable parameter, facial expression patterns reflecting the experience of pain have been reported across species boundaries (33–39). While early descriptions by Darwin already suggested parallels in facial expressions reflecting emotions in different animal species and humans, Langford et al. (33) were the first group to systematically study facial expressions as a measure of pain. Their groundbreaking work focused on the Mouse Grimace Scale (MGS) and its thorough validation in a variety of pain models (33). Using grimace scales, deviations from the physiological state can be examined based on the facial expressions. The grimace scale for mice comprises five action units (AUs): orbital tightening, nose bulge, cheek bulge, ear position, and whisker change (33). Subsequently, grimace scales have been developed and assessed in different species including rats (34–38, 40).
Regardless of the pain parameter, one needs to consider the pronounced influence of numerous variables such as genetics, age, sex, environmental factors, social interaction, and prior experience that can influence pain assessment (10, 14, 16, 41–44). Despite the fact that extensive narrative reviews have been published, summarizing available information about the value, practical use, and limitations of grimace scales, nest building performance, and burrowing activity as parameters for the assessment of pain (9, 41), there is still a knowledge gap concerning the implementation of these methods for the assessment of post-surgical pain. To our knowledge, more systematic approaches have so far only been used in reviews focused on MGS.
A scoping review by Whittaker et al. examined the MGS in different types of pain (e.g., visceral pain after injection of Freund's adjuvant), indicating a wide application of the MGS in different animal models (45). A recent scoping review focusing on the grimace scales in non-human mammals has already intensely studied the level of evidence for measurement properties of various grimace scales reporting a high level of evidence for MGS and RGS (46). Our analysis is more specifically focused on the application of post-operative pain induced by different types of surgeries under general anesthesia in mice and rats, including the extraction of information about anesthesia and perioperative analgesia.
Taken together, there are remaining knowledge gaps concerning the implementation of grimace scales for post-surgical pain assessment and obvious knowledge gaps concerning the post-surgical implementation of nest building and burrowing assessment.
Therefore, we completed a systematic review exploring the available literature about the application of grimace scales and the assessment of nest building and burrowing activity in the context of surgical interventions and associated pain.
Materials and methods
The systematic review protocol was registered before starting the formal screening of papers with the Systematic Review Facility (SyRF) for preclinical studies on 26 March 2020 and is available from https://syrf.org.uk/protocols and in Supplementary Methods 1. We used the Systematic Review Center for Laboratory animal Experimentation's (SYRCLE) protocol template version 2 (47) to create the protocol. As outlined in the pre-published protocol (https://syrf.org.uk/protocols, 26 March 2020), we extracted information about the strain, sex, age, type of surgical intervention, type of anesthesia and analgesia, time of day, materials used for nest building and burrowing, and video-based analysis of grimace scales vs. direct observation, among other variables. In addition, we obtained information about the respective study quality by application of the SYRCLE risk of bias tool (48), which has been based on the Cochrane risk of bias (49) tool and comprises ten items related to six types of bias to assess the study quality. Reporting was conducted according to the Preferred Reporting Items for Systematic Reviews and Meta-Analyses (PRISMA) guidelines (50). The PRISMA checklist is provided in Supplementary Methods 2. The research question was defined as follows: What is the current evidence base for using the grimace scale, burrowing, and nest building for the assessment of post-surgical pain in mice and rats?
Search string and study selection
A comprehensive search string was developed for PubMed using Entry Terms, keywords, and medical subject headings (MeSH). During search development, “not searches” were performed in which the term being tested and other terms were linked with “not” to evaluate the appropriateness of the term. We combined a search for titles, abstracts, and author-defined keywords with a search for the thesaurus terms. The search comprised relevant synonyms and alternative spellings for the four following components: “tests” (grimace scale, burrowing, nest building), “surgery,” “pain,” and “rodents”.
The following platforms were searched for relevant articles on 16 March 2020: PubMed, Embase via Ovid, and Web of Science. The following databases were searched via the Web of Science platform: Science Citation Index Expanded, Social Sciences Citation Index, Arts & Humanities Citation Index, Conference Proceedings Citation Index—Science, Conference Proceedings Citation Index—Social Science & Humanities, and Emerging Sources Citation Index. The search string was developed for PubMed and translated to Embase and Web of Science. The four search components were combined within the databases with the Boolean operator “AND.” The final search strings are provided in the protocol and in Supplementary Methods 3.
All search results were transferred to EndNote reference management software (Endnote™ X9). Since, to our knowledge, studies that assess the pain parameters of interest related to post-surgical pain were not published before 2005, and only references from 2005 through 2020 were analyzed. Studies published before 2005 and duplicates were manually removed.
Based on a review by Van der Mierden et al. (51), the web application RAYYAN (52) was selected for the two separate screening phases: title and abstract screening followed by full-text screening. Screeners were trained with the SYRCLE's e-learning tool for preclinical systematic reviews and with a prescreened training set of 50 abstracts. Predefined inclusion and exclusion criteria are presented in Table 1.
Screening of titles and abstracts was performed by two independent reviewers (KA and VB). In the title and abstract screening, primary studies in English with mice and/or rats describing surgery were included. Surgery was defined as a procedure involving skin incision (including biopsy) under general anesthesia. Discrepancies were resolved by discussion with a third person (IK). In case of remaining doubt about the decision, in this phase, the study was always included.
Two independent reviewers screened for relevant studies during the full-text phase; KA screened the entire set, whereas the set was divided among four independent people as the second reviewer (HS, HK, MB, and CH). In addition to the criteria mentioned above, only full peer-reviewed studies that describe grimace scale, burrowing, and/or nest building as pain parameters were included. Discrepancies were resolved by discussion with a third person (HP). Reference list screening of included studies was conducted by two independent reviewers (KA and HK) to find relevant studies that were not retrieved from the literature databases. Of the references whose title included a surgical intervention and/or the parameters of interest including synonyms, the full text was retrieved and checked for the previously described inclusion criteria. Only full peer-reviewed studies in English describing grimace scale, burrowing, and/or nest building in the post-surgical phase in mice and/or rats were included (refer to Table 1).
The originally described and validated grimace scale for mice (33) comprises five AUs: orbital tightening, nose bulge, cheek bulge, ear position, and whisker change. For rats, Sotocinal et al. (34) initially described and validated four AUs comprising orbital tightening, nose/cheek flattening, ear changes, and whisker change. The term “grimace scale” is used rather liberally in the current literature, and we identified studies either assessing only the eyes or assessing the AUs within a composite behavioral scale. We thus defined the inclusion criterium for “grimace scale” more precisely; at least two AUs had to be scored and that at least one of the following keywords (grimace scale, facial expression, and pain face) had to be mentioned in the text.
Data extraction
Our unit of analysis was a group of similar animals following the same protocol; if a paper described multiple relevant strains and/ or procedures, data were extracted per group of animals.
Bibliographic details (e.g., first author, year of publication), study design characteristics (e.g., housing, groups), animal model characteristics (e.g., strain, sex), intervention characteristics (e.g., type of surgery, type of analgesia), and outcome measures (e.g., significant alterations of grimace, burrowing or nest building parameters) were extracted for each relevant experimental group/outcome parameter of interest and recorded in an Excel spreadsheet.
For the type of intervention, the following categories were distinguished: biopsy, craniotomy, laparotomy, laparoscopy, (hemi)laminectomy, meniscectomy, neurosurgery, plantar paw incision, subcutaneous implantation, thoracotomy, vascular surgery, and vasectomy. All included procedures grouped under “neurosurgery” were peripheral nervous system interventions.
A subset of 10% of the extracted data, selected by simple randomization with R version 3.6.3. via RStudio version 1.2.1335 (53) was quality checked by a second reviewer (K.Sc.).
Quality assessment—risk of bias
A quality assessment of the studies was performed using SYRCLE's risk of bias (RoB) tool (48). The RoB tool comprises ten items to assess the quality of the included references, which are related to six types of bias: selection bias, performance bias, detection bias, attrition bias, reporting bias, and others. We added the following item: it was assessed whether a power analysis or sample size calculation was reported. Based on signaling questions (refer to Table 2), each item was assessed with the outcome recorded as “YES” (indicates a low risk of bias), “NO” (indicates a high risk of bias), or “UNCLEAR” (indicates an unclear risk of bias). In one of the studies, only one experimental group was used; in this study, selected RoB items were rated “not applicable (NA).” For question Q1 “allocation sequence,” the method of randomization (e.g., randomizer.org, R) had to be defined to result in a low risk of bias evaluation, i.e., reporting “randomly” was insufficient. For question Q10 “other sources of bias,” we focused on the performance of the tests in terms of light and dark phases, the presence of industrial funding, and other suboptimal methods (e.g., picking the best photograph for pain assessment). One reviewer (K.A.) performed the quality assessment of all studies. A random subset of 10% of the extracted data (random selection as for data extraction) was quality checked by a second reviewer (K.Sc.).
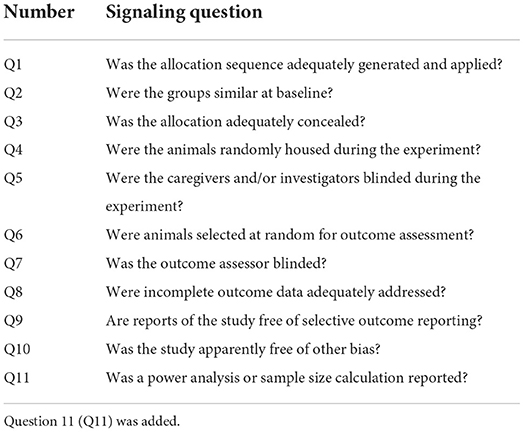
Table 2. SYRCLE's risk of bias tool signaling questions (48).
Data analysis
Extracted data were separately tabulated for each outcome measure (grimace scale, burrowing, and nest building) in Excel and Word. Thus, a paper describing multiple outcomes is listed in more than one table.
For the evaluation of analgesia and anesthesia, each paper was included once, except for one study that used both mice and rats, which were included as separate studies.
Excel's Pivot tables were used to analyze and plot the data.
Deviating from our protocol, we decided not to perform any meta-analyses in this review, since the heterogeneity in experimental design and outcome parameters between the included studies was considered to be too high.
Results
Identification of publications reporting an analysis of the parameters of interest in the post-surgical phase
Our searches of the databases retrieved a total of 3,355 papers. Exclusion of studies before 2005 (k = 759) and removing duplicates and triplicates (k = 712) yielded 1,884 papers for screening. In total, 1,532 papers were included after the title and abstract screening, and 64 papers were included after the full-text screening. The reference list screening of included papers revealed 10 additional papers. In total, 74 papers were thus included in the review. A summary of the study flow can be found in Figure 1.
We identified the following number of papers assessing the pain parameters of interest: 18 papers assessing the Mouse Grimace Scale (MGS), 29 papers assessing the Rat Grimace Scale (RGS), 20 papers assessing nest building in mice, one paper assessing nest building in rats, 10 papers assessing burrowing in mice, and seven papers assessing burrowing in rats.
Overall, we included 38 papers using mice as the animal model, 35 papers using rats, and one paper using both rats and mice.
The 38 papers about mice describe 106 study groups, and the 35 papers about rats describe 94 study groups.
Most papers (65/74) evaluated only one pain-associated outcome measure, whereas one paper assessed both grimace scale and nest building, two papers assessed both grimace scale and burrowing, five papers assessed both nest building and burrowing, and only one paper assessed all three pain parameters.
A list of all included papers and evaluated pain parameters is presented in Supplementary Table 1.
Post-surgical application of mouse and rat grimace scales
Screening identified 18 mouse (25, 33, 54–69) and 29 rat (24, 34, 55, 70–95) studies published between 2010 and 2020 that explored grimace scales in the post-surgical phase. The countries of origin of the first author comprise the United Kingdom and different European and North American countries for studies with an analysis of MGS. For rat studies, the respective list of countries includes Asia, Europe, North, and South America (Table 3). The first post-surgical grimace scale study in mice (MGS) was published in 2010; the first was in rats (RGS) in 2011 (Figures 2A,B).
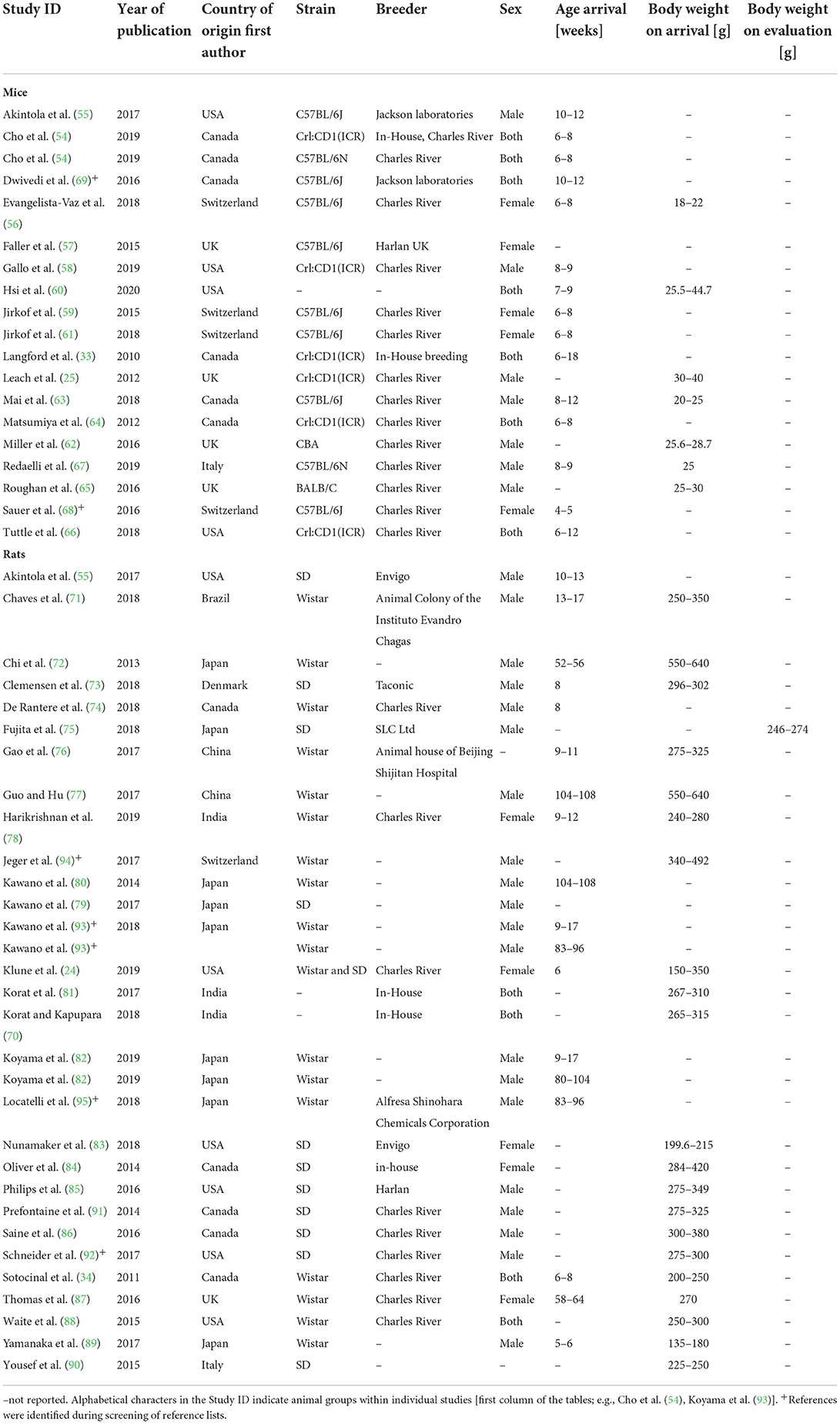
Table 3. Study characteristics and animal characteristics for Mouse Grimace Scale (k = 18 studies) and Rat Grimace Scale (k = 29 studies).
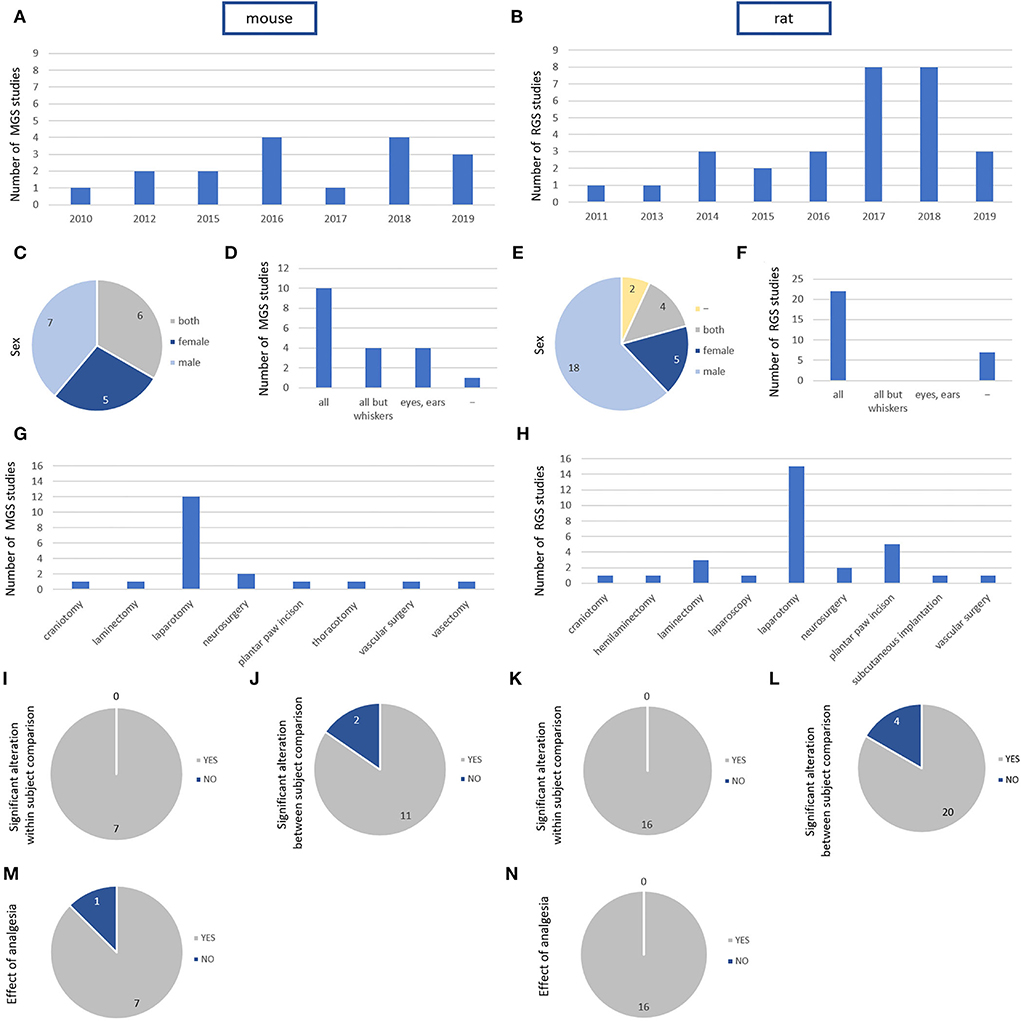
Figure 2. Study characteristics, animal characteristics, and intervention characteristics for Mouse Grimace Scale (k = 18 studies) and Rat Grimace Scale (k = 29 studies). (A,B) Number of published studies using grimace scale for the assessment of post-surgical pain in mice (A) and rats (B) during the last decade. X-axis represents years. (C,E) Number of grimace scale studies in mice (C) and rats (E) using females, males, and both sexes. (D,F) Scored action units (AUs) in mice (D) and rats (F). All but whisker: all AUs were scored except of whisker change. Eyes, ears: narrowing of the eyes and ears changes were evaluated. (G,H) Type of intervention in mice (G) and rats (H). (I,K) Studies describing within-subject comparison in mice (I) and rats (K). The within-subject comparison revealed an influence of the surgical intervention in all mouse and all rat studies. (J,L) Studies describing between-subject comparison in mice (J) and rats (L). (M,N) Studies describing an analysis of the impact of an analgetic drug on MGS (M) or RGS (N) in comparison with a control group. The analysis revealed an influence in all rat studies.
For both scores, an increase in the application was observed at the end of the decade (Figures 2A,B). Since the search in the databases was conducted in early 2020, the year 2020 was not included in the graphs.
While there was only a slight imbalance in the use of male and female animals in mouse studies, the majority of rat studies were conducted in male animals, totaling 62% (18/29 studies; Table 3, Figures 2C,E).
Information about the mouse and rat strains used could be extracted from the majority of papers (Table 3). The analysis revealed a predominance of studies in C57BL/6J mice (eight studies in total). The next most frequently used mouse strain was Crl:CD1(ICR). For further mouse strains reported in the identified publications, the number of studies per strain was one or two. All rat studies, except two where the strain was not reported, were conducted in Wistar rats (k = 16) and/or Sprague Dawley rats (k = 12).
Assuming a frequently applied habituation phase of 1–2 weeks following arrival, age on arrival indicates that the majority of studies (13/18) in mice were completed in young adult mice (Table 3). The exact body weight at the time of the surgical intervention or MGS assessment was not reported in all studies. In only one study, younger mice (<6 w; age 4–5 weeks on arrival) were ordered from the commercial breeder (68). In this study, animals were 7–8 weeks old during testing.
Another study reported an age of 6–18 weeks on arrival, indicating the use of adult mice in one of the subprojects (33). However, in this case, it was impossible to conclude the exact age at which the intervention was conducted.
For rats, a broader age range was indicated from the information about age on arrival ranging from 5 to 108 weeks. This indicated that studies were performed in young adult, adult, and aged rats (Table 3).
Whereas the majority of studies in rats considered all AUs, several studies in mice did not apply the whisker score (4/18 studies) or instead focused on orbital tightening and ear position (4/18 studies). The remaining studies in mice included all AUs in their analysis (10/18 studies). In Cho et al. (54), all AUs were examined in CD-1 mice, whereas in BL6 mice, all AUs were examined except whiskers due to poor visibility. A video- or image-based analysis was reported in 61% of the mouse and 72% of the rat studies (Table 4, Figures 2D,F).
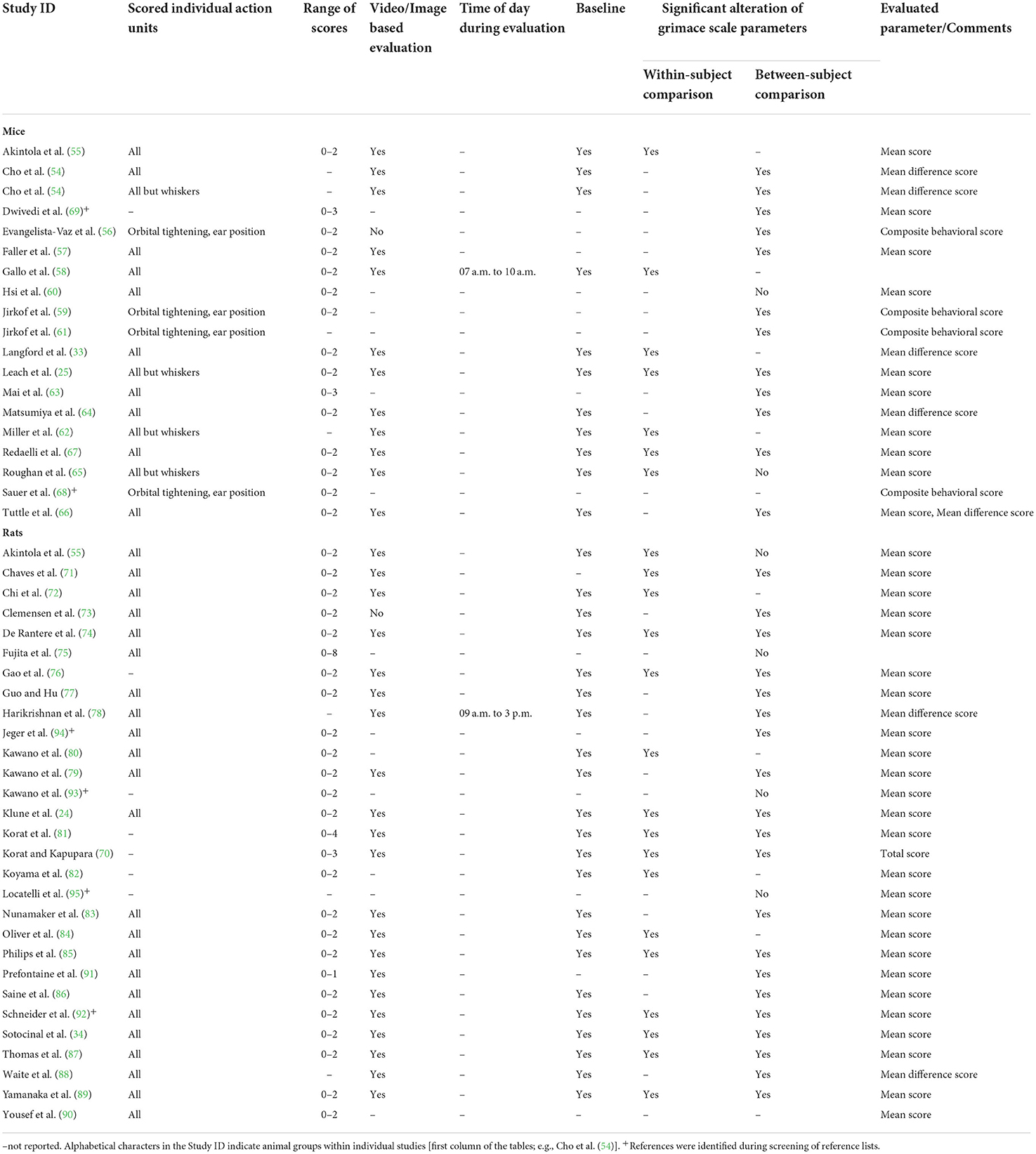
Table 4. Outcome characteristics for Mouse Grimace Scale (k = 18 studies) and Rat Grimace Scale (k = 29 studies).
Concerning the type of surgical intervention, laparotomy was the most frequently performed procedure, accounting for 67% of mouse and 52% of rat studies identified. Other interventions in mice included craniotomy, neurosurgery, laminectomy, thoracotomy, vascular surgery, plantar paw incision, and vasectomy. In rat studies, the list of further surgical techniques, in addition to the aforementioned, included hemilaminectomy, laparoscopy, and subcutaneous implantation (Figures 2G,H).
Baseline data were collected in 10 of the 18 mouse studies and 22 of the 29 rat studies. A comparison with baseline levels (within-subject design) was described in seven of the mouse studies and 16 of the rat studies. The within-subject comparison revealed an influence in seven of the mouse and 16 of the rat studies (Table 4, Figures 2I,K).
An impact of the surgical intervention on MGS and RGS based on a between-subject design was assessed in 13 mouse and 24 rat studies. The between-group comparison confirmed an effect in 11 and 20 of the mouse and rat studies (Table 4, Figures 2J,L).
An impact of a single analgetic or combination of analgetic drugs on MGS and RGS in comparison with the control group was analyzed in 8 of 18 mouse studies and 16 of 29 rat studies. The analysis revealed an impact in 7 mouse and 16 rat studies (Table 5, Figures 2M,N). The most frequently used drugs included NSAIDs and opioids, most often administered subcutaneously or intraperitoneally.

Table 5. Design and intervention characteristics for grimace scale, nest building, and burrowing in mice (k = 39 studies) and rats (k = 36 studies).
Post-surgical analysis of nest building activity and performance
Screening identified 20 studies published between 2007 and 2020 that explored nest-building activity and performance in the post-surgical phase of interventions in mice (27, 28, 58, 61, 96–111). For rats, we only identified one study from Germany published in 2018 that assessed the impact of surgery on nest building in female Sprague Dawley rats following craniotomy (112). Concerning mouse studies, the countries of origin of the first author comprise different European countries and the United States (Table 6). The number of mouse studies with analysis of nest building following surgery increased slightly toward the end of the studied decade (Figure 3A).
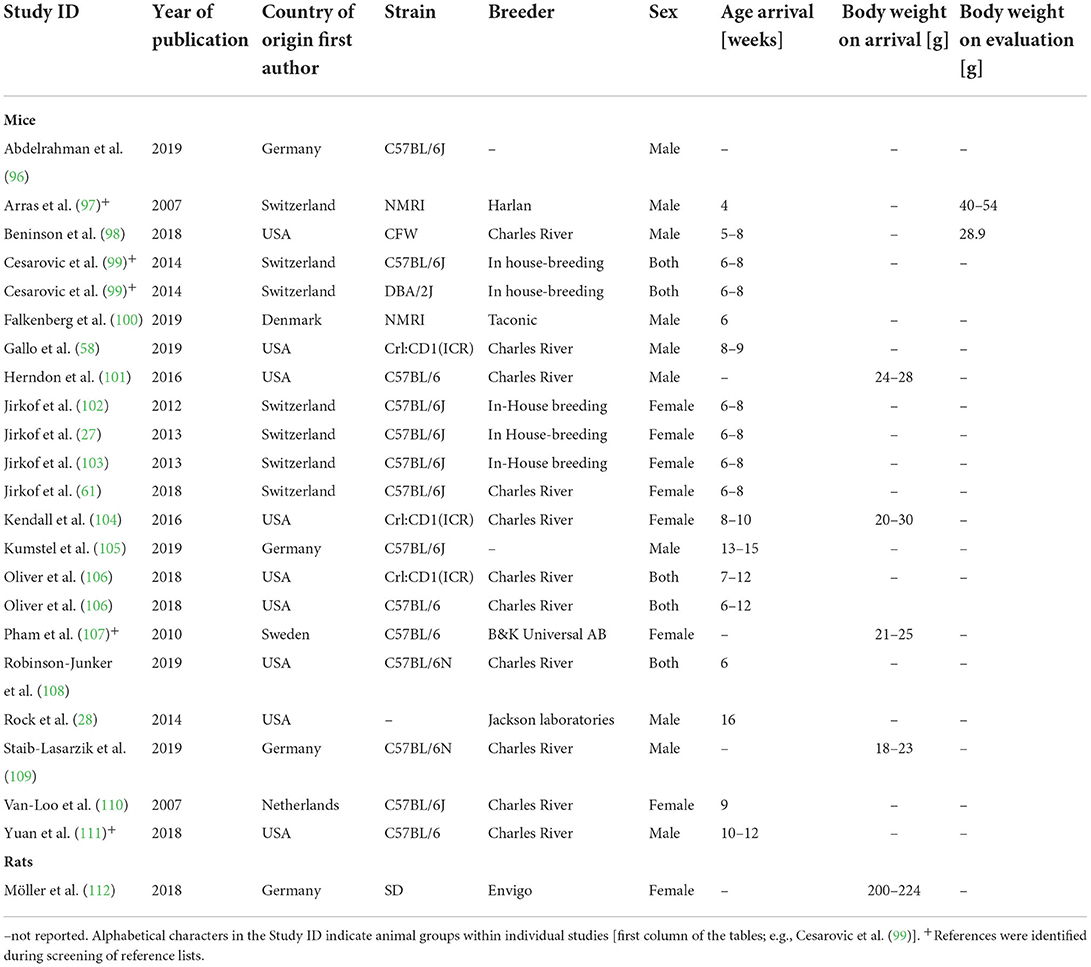
Table 6. Study and animal characteristics for nest building in mice (k = 20 studies) and rats (k = 1 study).
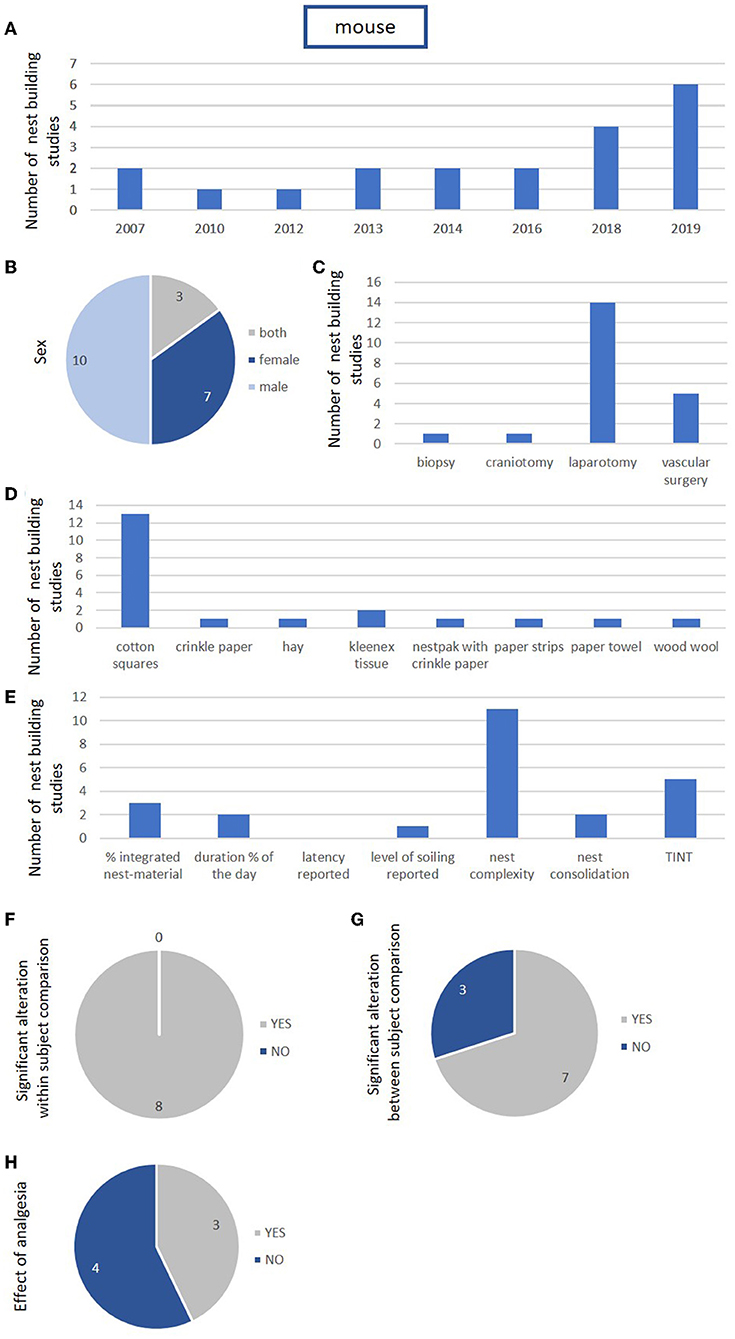
Figure 3. Study characteristics, animal characteristics, intervention characteristics, and outcome characteristics for nest building in mice (k = 20 studies). (A) Number of published studies using nest building for the assessment of post-surgical pain in mice during the last decade. X-axis represents years. (B) Number of included nest building studies using females, males, and both sexes. (C) Type of intervention in mice. (D) Used nest building material in mice. (E) Reported nest building parameters in mice. (F) Studies describing within-subject comparison in mice. The within-subject comparison revealed an influence of the surgical intervention in all studies. (G) Studies describing between-subject comparison in mice. (H) Studies describing an analysis of the impact of an analgetic drug in comparison with a control group.
The list of mouse studies included three studies that completed an analysis in both sexes, ten studies that focused on male mice, and seven studies that focused on female mice (Table 6, Figure 3B).
Information about the mouse strain used could be extracted from the majority of publications. The analysis revealed a predominance of studies in C57BL/6J mice with a total number of eight studies. In four publications, it was not specified which C57BL/6 substrain was used, i.e., J or N. For further mouse strains reported in the identified publications, the number of studies per strain amounted to one to two (Table 6).
Considering a frequently applied habituation phase of 1–2 weeks following arrival, age on arrival indicated that the vast majority of studies (15/20) in mice were completed in young adult mice. The body weight at the time of the surgical intervention or nest building assessment was only reported in two studies. Younger mice (<6 weeks) were ordered (age 4 or 5–8 weeks at arrival) from the commercial breeder in only two studies (97, 98). Arras et al. (97) reported that animals underwent laparotomy at 16 weeks of age to assess post-surgical pain 3 days after surgery. In the study by Beninson et al. (98), the age of the animals at the time of surgery and nest building assessment was not reported.
In all of the mouse studies, animals were no older than 15 weeks on arrival (Table 6).
Concerning the type of surgical intervention, laparotomy was the most frequently performed procedure in mice, conducted in 14 studies. Other interventions in mice comprised vascular surgery, biopsy, and craniotomy (Figure 3C).
Cotton squares represented the most frequently used nesting material in mouse studies (13/20 studies). Alternative materials comprised wood wool, crinkle paper, paper strips, kleenex tissues, paper towels, hay, and nestpak with crinkle paper (Table 7, Figure 3D). Only five mouse studies reported the amount of material offered. An image-based evaluation proved to be the exception, reported in just two publications (Table 7).
While seven publications failed to provide information about the time of the day for assessment, the majority of studies (12/20) focused on the light phase and only one study assessed the activity during the light and dark phase (Table 7). The type of assessment and parameters varied across the mouse studies with application of the TINT (= time-to-integrate to nest test) in five studies, assessment of nest consolidation in two studies, of nest complexity in eleven studies, of % integrated material in three studies, and duration of nest building activity (% of the day) in two studies. An additional analysis of the level of soiling was only described in one study. The analysis of more than one nest building parameter was rather an exception (Table 7, Figure 3E).
Baseline data were collected in 13 of the 20 mouse studies. A comparison with baseline levels (within-subject design) was described in eight of the mouse studies. An impact of the surgical intervention on nest building activity or performance based on a between-subject design was assessed in 10 mouse studies. The within-subject comparison revealed an effect in 8 and the between-subject comparison revealed an effect in seven mouse studies (Table 7, Figures 3F,G).
An impact of a single analgetic or combination of analgetic drugs on nest-building activity or performance in comparison with the control group was analyzed in seven of the 20 mouse studies. The analysis revealed an impact in three studies (Table 5, Figure 3H).
Post-surgical analysis of burrowing activity and performance
Screening identified 10 mouse (8, 56, 59, 61, 96, 102, 103, 105, 113, 114) and 7 rat studies (26, 31, 112, 115–118) published between 2010 and 2019 that explored burrowing activity in the post-surgical phase of interventions.
The countries of origin of the first author comprised Switzerland, Germany, the United States, and China for the mouse studies, and the United Kingdom, the United States, and four different European countries for the rat studies (Table 8).
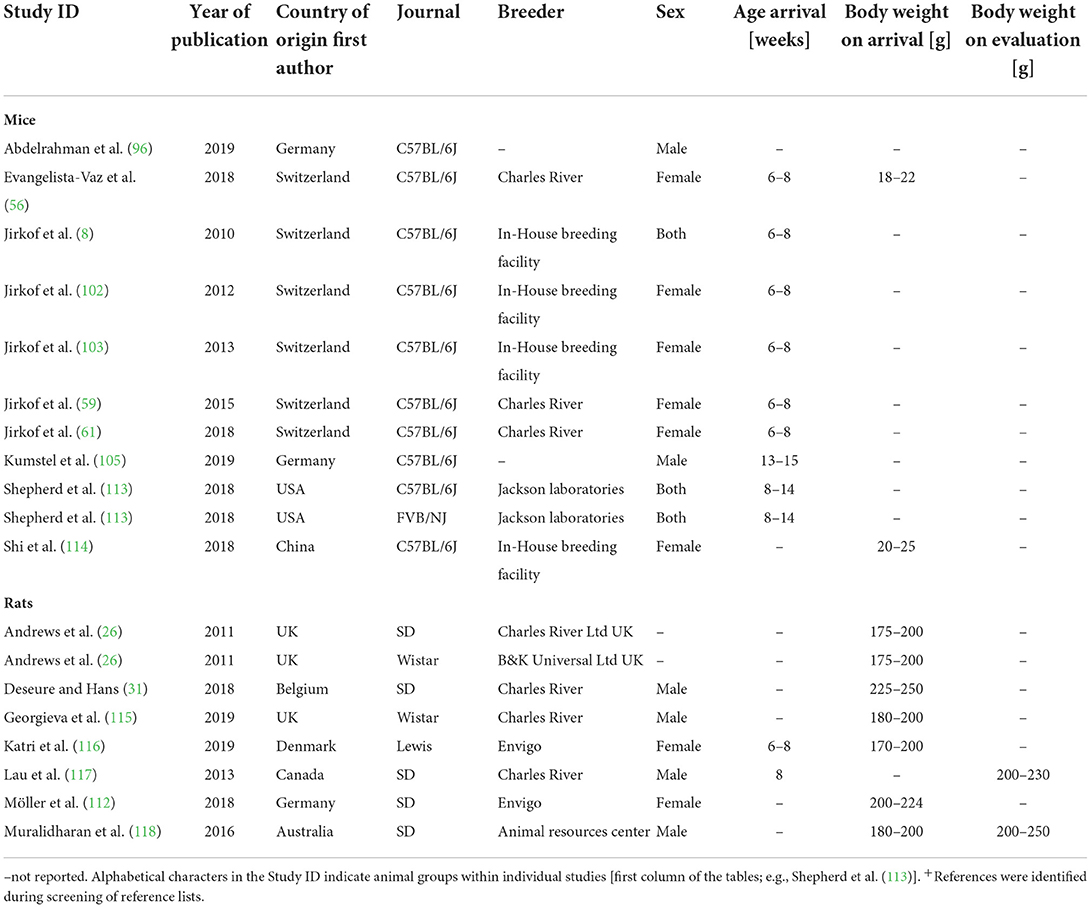
Table 8. Study and animal characteristics for burrowing mice (k = 10 studies) and rats (k = 7 studies).
The first mouse study assessing burrowing activity in the post-surgical phase was published in 2010 and the first rat study in 2011. In mice, a recent trend for an increase in the number of studies/year was identified (Table 8, Figures 4A,B).
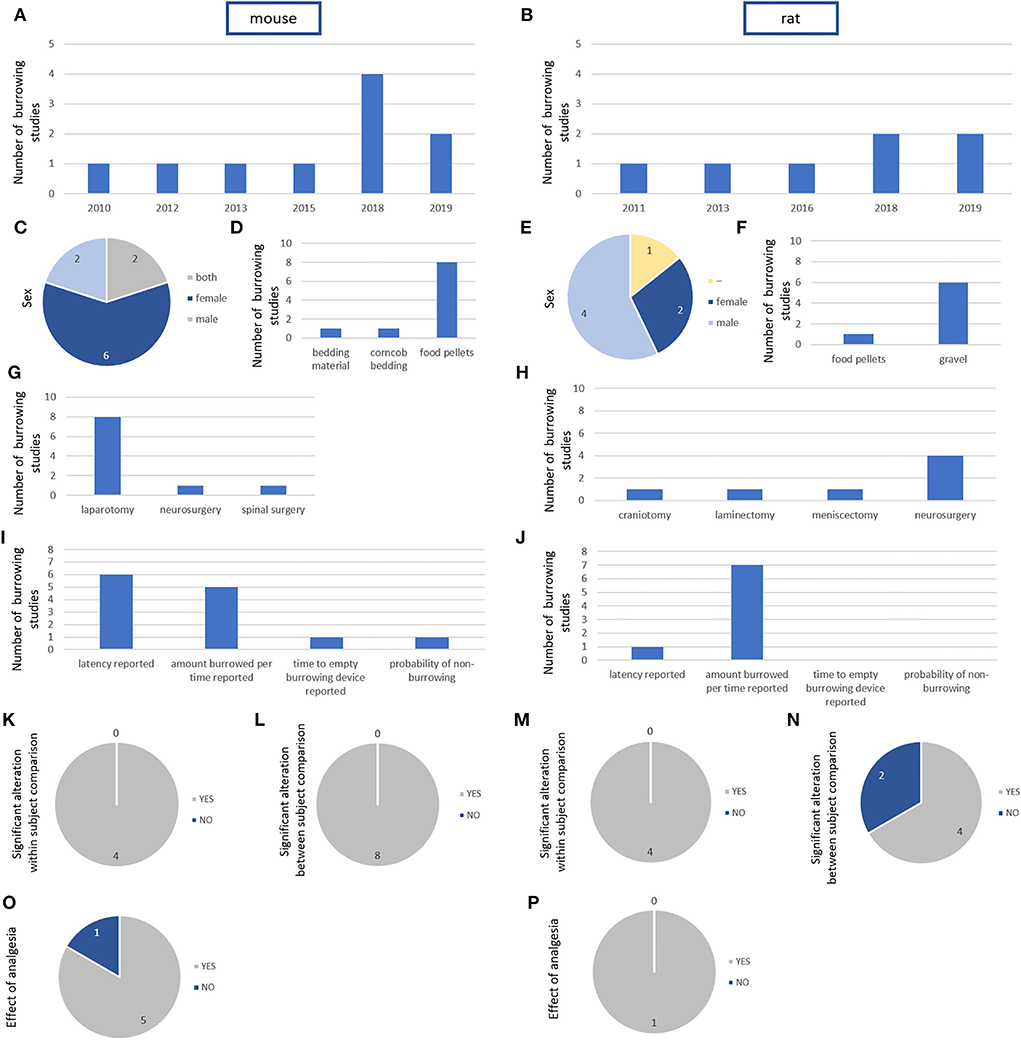
Figure 4. Study characteristics, animal characteristics, and intervention characteristics for burrowing in mice (k = 10 studies) and rats (k = 7 studies). (A,B) Number of published studies using burrowing for the assessment of post-surgical-pain in mice (A) and rats (B) during the last decade. X-axis represents years. (C,E) Number of burrowing studies in mice (C) and rats (E) using females, males, and both sexes. (D,F) Used burrowing material in mice (D) and rats (F). (G,H) Type of intervention in mice (G) and rats (H). (I,J) Reported burrowing parameter in mice (I) and rats (J). (K,M) Studies describing within-subject comparison in mice (K) and rats (M). The within-subject comparison revealed an influence of the surgical intervention in all mouse and all rat studies. (L,N) Studies describing between-subject comparison in mice (L) and rats (N). The between-subject comparison revealed an influence of the surgical intervention in all mouse studies. (O,P) Studies describing an analysis of the impact of an analgetic drug in comparison with a control group in mice (O) and rats (P). The analysis revealed an influence in all rat studies.
While two studies assessed burrowing in mice of both sexes, six studies focused on female mice and two on male mice. Among the publications reporting burrowing data from rats, one failed to report the sex of the animals, four focused on male rats and two on female rats (Table 8, Figures 4C,E).
Information about the mouse and rat strains used could be extracted from all the publications. In all mouse studies, the experiments were conducted in C57BL/6J mice. One study additionally used FVB/NJ mice (113). The strains used in the rat studies comprised Sprague Dawley rats (five studies), Wistar rats (two studies), and Lewis rats (one study; Table 8).
Considering a frequently applied habituation phase of 1–2 weeks following arrival, age or body weight on arrival indicated that the vast majority of studies (16/17) were completed in young adult or adult mice and rats. The body weight at the time of the surgical intervention or burrowing assessment was not reported in most studies (15/17; Table 8).
Food pellets represented the most frequently used burrowing material in mouse studies (8/10 studies). Only two studies used bedding material as an alternative for the burrowing test in mice. The amount of burrowing material offered varied greatly across the mouse studies, ranging from 50 to 201 g. Almost all studies in rats offered gravel (2,000 or 2,500 g) as the burrowing material except for one study using food pellets (1,000 g, Table 9, Figures 4D,F).
Concerning the type of surgical intervention, laparotomy proved to be the predominant procedure in mice (k = 8). Only two mouse studies focused on a neurosurgical procedure. In rats, the list of interventions comprised neurosurgery, craniotomy, laminectomy, and meniscectomy (Figures 4G,H).
Latency to start burrowing was the most frequently assessed parameter in the mouse studies with six studies reporting the respective data. The amount burrowed per time was analyzed in five mouse studies. In these studies, the duration of the test ranged from 10 to 120 min. Further parameters analyzed in just one study each were time to empty the burrowing device and probability of non-burrowing. All rat studies assessed the amount burrowed per time. The duration of the test varied ranging from 60 to 240 min. Only one rat study reported an additional analysis of the latency to start burrowing activity (Table 9, Figures 4I,J).
Concerning time-of-day for the analysis of burrowing in the mouse studies, three publications failed to provide the respective information, five studies conducted the assessment during the light phase, one study analyzed the activity during the dark phase, and one study during the light and dark phases. For rats, three publications failed to provide the respective information, three studies focused on an assessment during the light phase, and one study on an assessment during the dark phase (Table 9).
Baseline data were collected in 4 of the 10 mouse studies and all of the rat studies (7/7).
A comparison with baseline levels (within-subject design) was described in four of the mouse studies and three of the rat studies. The within-subject comparison revealed an influence in all four of these mouse studies and all three of these rat studies (Figures 4K,M). An impact of the surgical intervention on burrowing activity or performance compared to a separate control group (between-subject design) was assessed in eight of the mouse studies and six of the rat studies. The between-subject comparison revealed an influence in all eight of these mouse studies and four of these six rat studies (Table 9, Figures 4L,N).
An impact of a single analgetic or combination of analgetic drugs on burrowing activity or performance in comparison with the control group was analyzed in 6 of 10 mouse studies. The analysis revealed an impact in five of these studies (Figure 4O). In rats, the impact of analgetic or different analgetic drugs on burrowing activity or performance was assessed in only one study where an effect was present (Table 5, Figure 4P).
Anesthesia and analgesia
In both species, inhalational anesthesia was most frequently applied with the use of isoflurane most common, followed by the use of sevoflurane. Further types of anesthetic drugs and drug combinations comprised ketamine/xylazine in mice as well as ketamine, ketamine/xylazine, tiletamine/zolazepam, pentobarbital, and chloral hydrate in rats (Table 5).
Perioperative analgetic drugs were administered in the vast majority of studies (62/75). However, almost all studies used a monotherapeutic approach with the application of only one type of analgetic drug (Figure 5). An opioid was administered in 38% of the mouse studies (15/39) and 39% of the rat studies (14/36). The list of opioids comprised buprenorphine and tramadol in mice and buprenorphine, nalbuphine, morphine, fentanyl, and tramadol in rats. Use of a non-steroidal anti-inflammatory (NSAID) drug was reported in 44% of the mouse studies (17/39) and 36% of the rat studies (13/36). The list of NSAIDs included carprofen, ibuprofen, ketoprofen, meloxicam, flunixin, robenacoxib in mice and carprofen, (dex)ibuprofen, ketoprofen, and meloxicam in rats (Table 5).
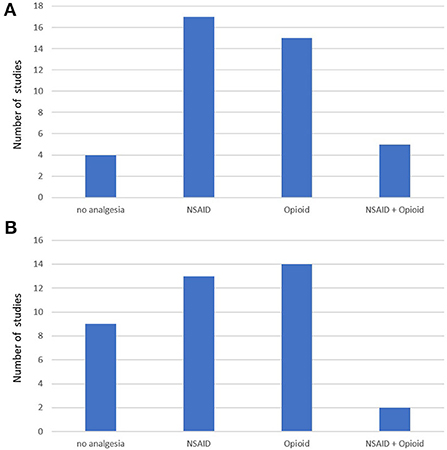
Figure 5. Type of analgesia in mice (k = 39 studies) and rats (k = 36 studies) combined for grimace, nest building and burrowing. (A) Type of analgesia used in mice. (B) Type of analgesia used in rats.
A multimodal approach was used in 13% of the mouse studies (5/39) and 6% of the rat studies (2/36). Local anesthetic drugs were only applied in 5% of the mouse (2/39) and 33% of the rat studies (12/36) with the use of the following drugs: lidocaine, bupivacaine in mice and lidocaine, ropivacaine, (levo)bupivacaine, xylocaine in rats (Table 5).
Moreover, the analgetic-antipyretic acetaminophen was administered in two mouse studies (61, 64) and one rat study (88). Further drugs used included metamizole and gabapentin in mice and thalidomide and gabapentin in rats (Table 5).
Application of measures to control the risk of bias and reporting quality
For each included study and each pain parameter, we have assessed the risk of bias (Figure 6).
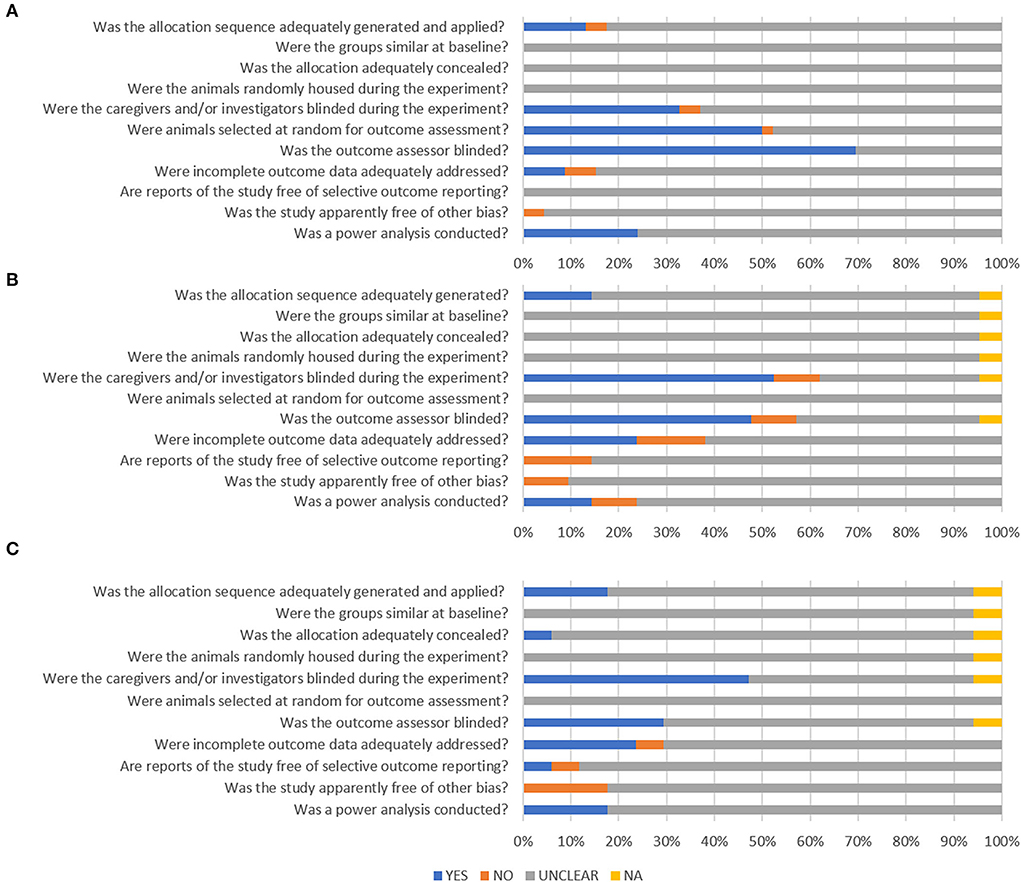
Figure 6. Risk of bias assessment for grimace scale (A), nest building (B), and burrowing (C). Mice and rats were pooled together per pain parameter. YES, scored a low risk of bias; NO, scored a high risk of bias; UNCLEAR, scored an unclear risk of bias; NA, not applicable; RoB item was not applicable as only one group was used in the study.
Regarding selection bias, information about baseline characteristics was missing in 99% and information about the procedures for allocation concealment was missing in 96% of all studies identified for the different pain parameters. Moreover, details about the randomization approach for sequence generation were only provided in 14% of the publications (Figure 6).
Assessment of performance bias focused on random housing and blinding. While information about the distribution of animals and cages in the animal facility was missing in 98% of the publications, blinding of caregivers and/or investigators from knowledge about the intervention was reported in 40% of the publications (Figure 6). While not formally analyzed, during data extraction, we noticed that details about the staff members that remained blinded were rarely provided.
Randomization of animals for testing or of videos and images for outcome assessment was reported in 50% of the studies assessing grimace scales, in none of the publications assessing nest building, and in none of the studies assessing burrowing.
Details about the blinding of assessors were provided in 70% of the grimace scale studies, 48% of the nest building studies, and 29% of the burrowing studies.
Incomplete reporting of attrition and exclusion was evident in the majority of the studies. The completeness of outcome data remained unclear in 76% of all studies identified for the different pain parameters. Moreover, in 94% of the publications, it remained unclear whether the reports were free of selective outcome reporting. Other types of bias were identified in 8% of the studies. It was not reported whether a power analysis was conducted in 77% of the studies (Figure 6).
Discussion
The reliable and robust monitoring and scoring of pain in laboratory rodents is an important basis for optimized perisurgical pain management, which always needs to be adjusted to the animal characteristics and the specific intervention. Moreover, for the selection of the analgetic regimen, a potential impact on scientific readout parameters needs to be considered (5). Considering the multitude of influencing factors, it is obvious that there are no universally valid analgetic concepts applicable to all experimental interventions. This, together with the general tendency of prey animals to hide signs of pain and suffering, implies the particular relevance of sensitive approaches to monitor pain and to check the success of pain management approaches.
Selected grimace scale AUs and behavioral parameters are considered as the key parameters for comprehensive composite evaluation schemes (56, 59, 61, 68). The relevance of these parameters is underscored by the fact that alterations in physiological and biochemical markers such as heart rate, respiratory rate, blood pressure, core temperature, and corticosterone are not specific for pain states.
This systematic review aimed to determine the use and evidence-base of three tests applied in the context of post-surgical pain assessment in mice and rats.
Traditional narrative reviews carry the risk of containing only a subset of the literature relevant to the topic that is known to the author conducting the review and thus may contain a bias based on the author's opinion and network (119, 120). In contrast, systematic reviews aim to analyze all relevant literature on a predefined research question, generate new data, and ideally summarize the results with a meta-analysis (120). We here present a systematic review based on a comprehensive search. The methodology was transparently described in a previously posted protocol. Furthermore, the selection of studies based on pre-defined eligibility criteria for inclusion was systematically performed in two screening phases. The quality of the studies was assessed using SYRCLE's risk of bias tool. The generated data from the included studies were systematically presented and analyzed in tabular form. A meta-analysis was not performed because the heterogeneity in experimental designs and outcomes between the included studies was considered to be too high. Although our search aimed to be fully comprehensive, reference list screening identified 10 additional papers. Part of this was due to some authors only using the relatively specific term “home cage behavior” instead of the specific test in their titles and abstracts or database indexing for the behavioral tests being suboptimal, but we also missed the relevant term “laminectomy” in the search string for surgery. We highly recommend adding this term to future searches for surgeries. The total number of studies identified amounted to 74. While the number of studies identified per parameter and year tended to increase slightly toward the second half of the last decade, the overall numbers are too low to conclude a trend for an increased application of the parameters. In rats, the RGS, which has initially been described by Sotocinal et al. (34), was the most frequently applied parameter. In mice, the highest numbers of studies were identified for MGS and nest building applied in the post-surgical phase.
Our findings with grimace scales being among the more frequently used are in line with recent reviews stating that facial expressions are widely used as a pain assessment parameter in laboratory rodents (41, 46). In this context, it needs to be emphasized that the analysis of the countries of origin of the first author indicated that we are far from a widespread global application of the parameters of interest.
As recently discussed by Turner et al. (10), the majority of commonly applied measures of pain are indirect and thus only provide an approximation of the actual pain state. Along this line, it has been emphasized that reliable pain detection in humans as well as animals requires multidimensional composite assessment schemes (10, 14, 121). Respective composite schemes can, for instance, combine a behavioral-based scale with fecal corticosterone metabolite levels (122). In this context, it seems unfortunate that only a small number of studies identified in the current review combined two or three of the parameters of interest. Thus, it is impossible to conclude about the relative or added informative value of the three parameters, which were in the focus of this review.
Grimace scales build on the fact that an interaction of neural pathways between peripheral receptors and efferent facial motor neurons results in changes in facial expression. These changes seem to be evolutionarily preserved as a “pain face” can, for instance, trigger social attention, protection, and care including maternal care (123–126). The initial development and characterization of the MGS comprised an assessment in various assays with an activation of the nociceptive system including the following surgical procedures: laparotomy, chronic constriction injury, and spared nerve injury conducted in female and male Crl:CD1(ICR) mice (33). The findings suggested that MGS can help to monitor visceral and somatic pain following laparotomy, but fail to detect neuropathic pain. Since this first report, a further seventeen studies have analyzed the MGS during the post-surgical phase. The majority of studies used young adult C57BL/6J or Crl:CD1(ICR) mice. Thus, it is evident that the application of the MGS following surgery has so far not been sufficiently assessed in other mouse strains as well as in younger and older mice. As strain and age may well impact the head shape, an impact on the different AUs of the MGS seems likely.
A comparable situation is evident for the RGS with a focus on young adult Wistar or Sprague Dawley rats in the majority of studies. While there was a lack of studies exploring the RGS in younger animals, some studies were identified that focused on aged rats (72, 77, 80, 82, 87, 93, 95). In the initial study, Sotocinal et al. (34) have already reported an RGS-based detection of post-laparotomy pain in young Wistar rats. Considering the since conducted studies, there is an obvious need to further evaluate the RGS in younger rats and in rat strains other than Wistar and Sprague Dawley rats. Concerning the type of surgery, the list of mouse and rat studies indicates that a higher level of experience has been reached for laparotomy since the first publications in 2010 and 2011. For the majority of other interventions, the number of studies identified per species did not exceed three studies.
Considering the technical aspects, the fact that the majority of studies report an image- or video-based analysis of MGS and RGS provides evidence that many scientists have tried to limit the risk of bias associated with a direct evaluation of grimace scores related to the observer's presence (10). In an earlier study, live scores proved to be lower than retrospective video-based scores (127). In this context, another important factor is that live or video-based scoring can better consider the changing facial expression so that transient blinking will not result in altered scores. Our data suggest that more research seems to be necessary to validate the different scoring approaches with live vs. video- vs. image-based scoring systems by direct comparison.
A recent systematic review focused on grimace scales in non-human mammals has already intensely studied the level of evidence for measurement properties of various grimace scales reporting a high level of evidence for MGS and RGS (46). Our analysis focusing on post-surgical pain revealed that the majority of studies analyzed all AUs of the MGS and RGS. However, some of the mouse studies did not consider whisker scores and a very small number of studies focused on orbital tightening and ear position only. This finding is in line with difficulties reported for a reliable assessment of whisker position (10, 25, 84), resulting in the decision to disregard this action unit. In this context, it is of interest that a recent study ranked the relative importance of MGS AUs based on two different mathematical approaches (128). While orbital tightening was identified as the best parameter, whisker change and nose bulge were the worst performing variables in this study focusing on pain responses to intraperitoneal CCl4 injection (128). The authors concluded that the findings suggest that the MGS can be simplified; however, they also emphasized that a model-specific assessment of the informative value of AUs might be necessary (128).
Several studies have assessed the ability to demonstrate the impact of the surgical intervention based on a within- and/or a between-subject comparison confirming the suitability and sensitivity of grimace scales to detect post-surgical pain following different procedures. An impact of the surgical intervention was observed in all within-subject comparisons for mice and rats, and in most studies including a between-subject comparison.
Further evidence comes from studies which explored an effect of the analgetic regimen based on a reduction in MGS and RGS scores, which was observed in the vast majority of studies.
While these data support the application of grimace scales for pain assessment, it needs to be considered that the evaluator's experience, knowledge, and training can have a tremendous impact on the assessment of subjective parameters such as facial expression and behavior (40, 129, 130).
The fact that we were only able to identify one rat study that explored nest-building activity and quality following a surgical intervention (112) seems to reflect difficulties to detect a reliable construction of complex nests in rats (131, 132). In apparent contrast, mice seem to be characterized by a higher intrinsic level of motivation for nest construction. This may be related to species differences in thermoregulation related to the ratio between body surface and body weight, resulting in a higher need for shelter and protection from weather and climate influences in wildlife mice as compared to wildlife rats.
Thus, it is not surprising that the validity of nest-building activity as an animal welfare and pain assessment parameter has been explored more intensely in mice (9, 10). Arras et al. (97) provided one of the first reports describing a post-surgical reduction in nest quality following laparotomy. Follow-up studies from the same group further tested the assessment of nest building for detection of post-surgical pain in mice of both sexes and different strains (27, 133).
Considering the publications identified by our systematic review, the majority of nest-building studies have focused on young adult mice with C57BL/6J as the most frequently used mouse strain and laparotomy as the most frequently applied surgical intervention. Thus, there are obvious gaps in knowledge concerning the post-surgical analysis of nest building in younger and aged mice, mouse strains other than C57BL/6J, and for surgical procedures other than laparotomy.
Concerning the methodological aspects, we identified a high variance in the type of parameters assessed ranging from time-to-integrate to nest (28, 58, 101, 104, 108), nest consolidation (98, 106), nest complexity (27, 58, 61, 96, 97, 100, 101, 105, 107, 109, 110), % integrated material (100, 104, 111), and % time spent nest building (102, 103). While it is of interest that efforts have been made to develop and explore different readout parameters, this of course limits the total level of evidence for the different parameters. Thus, it is recommended to directly compare the different nest building parameters in standardized approaches to provide information about potential differences in sensitivity, inter-rater and intra-rater reliabilities, and robustness.
An influence of the surgical intervention on nest building was reported by all studies with a within-subject design and the majority of studies with a between-subject comparison. While these data may support nest building as a parameter for post-surgical pain assessment, the low number of studies with a respective assessment and the heterogeneity in study design and parameters analyzed needs to be considered. Moreover, conflicting results were evident in studies assessing the impact of an analgetic. As already discussed by Jirkof (9), it still remains unclear whether the failure to detect an analgetic effect is due to residual pain resulting from insufficient pain control, high sensitivity of the parameters to low levels of residual pain control or a limited informative value of nest building as a pain parameter. In this context, it needs to be considered that nest-building activity is also compromised by impairments other than pain, including influences related to experimental infection or models of systemic inflammation, neurodegenerative, and psychiatric disorders (9, 134–138). Thus, based on the current state of knowledge, it is recommended that nest building such as other pain assessment parameters should only be applied as one parameter of a composite pain measurement scheme and under controlled environmental conditions.
Both mice and rats exhibit a high level of intrinsic motivation for burrowing behavior. A detrimental impact of post-laparotomy pain in mice has initially been described by Jirkof et al. (8). This study and follow-up studies from the same group (56, 59, 61) have not only sparked the interest of laboratory animal scientists but also of companies and academic groups engaged in development and assessment of novel analgetic drug candidates. This resulted in efforts to also assess burrowing as a potential pain assessment parameter in rats (139–141).
The total number of publications with the assessment of burrowing in the post-surgical phase in mice and rats was rather low, indicating that the parameter has not yet been well-characterized for post-surgical monitoring. Conclusions about general evidence are limited by the fact that the majority of studies focused on young adult or adult C57BL/6J mice and Sprague Dawley rats, and that the majority of studies in mice analyzed post-laparotomy pain. Thus, there is an apparent lack or paucity of knowledge concerning the application in younger and aged mice and rats, in other strains, and following different procedures.
Concerning the technical aspects, our systematic review confirms previous narrative reviews stating that food pellets and gravel are the predominant burrowing materials offered to mice and rats (9, 10). In this context, it is of interest that Wodarski et al. (139) have reported that the test was more sensitive when a material with a smaller particle size was offered to rats. This finding is not reflected by common approaches applied in rats with the continued use of gravel.
When comparing the readout parameters, species-specific differences in study design became evident with the majority of mouse studies focusing on the latency to start burrowing, and the majority of rat studies focusing on the amount burrowed per time. As further parameters including time to empty the burrowing device were only assessed in some studies, it is again recommended to conduct studies with direct standardized comparison allowing conclusions about the sensitivity and robustness of the different burrowing parameters.
It is of interest that in all mouse studies, both within-subject and between-subject comparisons confirmed the impact of surgical interventions. Furthermore, the effect of an analgetic regimen on burrowing was analyzed in six mouse studies with the majority (5 studies) confirming an impact. Thus, available data so far seem to support the application of burrowing as one parameter for pain assessment in the post-surgical phase.
However, in view of the low number of studies completing a respective analysis along with the failure to demonstrate an impact of the surgical intervention on burrowing performance in some studies, it is evident that more data are required to conclude the value of burrowing as a post-surgical pain parameter in rats.
While the anesthetic and analgetic management was not the focus of our analysis, we additionally extracted information about the drugs used. Interestingly, the vast majority of studies (62/75) identified by our systematic review protocol reported perioperative administration of analgetic drugs with frequent use of either opioids or NSAIDs. However, multimodal approaches were only applied in a small number of studies. In this context, it seems unfortunate that local anesthesia, which can efficaciously block the transduction of nociceptive signaling, is only rarely used in mice (133).
Concerning future directions, it is of particular interest that efforts are made to develop semi-automatic or automatic analysis based on the training and development of machine learning algorithms (142–144). Respective approaches might help to provide robust information and to standardize the scoring-based assessment of parameters such as the grimace scale. In addition, new approaches for imaging-based analysis might provide a basis for home-cage assessment of compromised well-being and pain. Infrared thermal imaging allows to collect information about changes in blood flow, heart rate, and respiratory rate (145–148), which in combination with automatic behavioral tracking provides an excellent basis for continuous monitoring of wellbeing.
Conclusions about the validity of pain parameters assessed in different studies need to take the study quality and risk of bias into consideration. The respective assessment using SYRCLE's risk of bias tool (48) demonstrated that the risk of bias seems to be unclear or high for most included studies.
In conclusion, the number of studies that analyzed grimace scales, nest building, and burrowing in the post-surgical phase in mice or rats is still relatively low. Gaps in knowledge are evident concerning the application of these parameters in different strains, age levels, and following different surgical procedures as well as their combined use. While findings concerning the impact of an intervention and the influence of an analgetic approach seem to be rather consistent for grimace scales, more data are needed for burrowing and nest building. In this context, further analyses are also necessary to directly compare the sensitivity and robustness of different performance parameters that can be applied for nest building and burrowing activities.
Data availability statement
The raw data supporting the conclusions of this article will be made available by the authors, without undue reservation.
Author contributions
HP and AB acquired the funding. HP supervised the review. KA wrote the review protocol and developed the search string under supervision from CL, HP, IK, and PJ. Screening was performed by KA, VB, HS, CH, MB, and HK. KS performed the quality check. KA and HP wrote the manuscript with contributions from HS and CL. All authors read and approved the manuscript.
Funding
This project was supported by the grants of Deutsche Forschungsgemeinschaft (FOR 2591, GZ: PO681/9-1 and 9-2, and BL 953/11-1 and 11-2). The funders had no role in the study design, data collection and analysis, decision to publish, or preparation of the manuscript.
Acknowledgments
The authors thank Sieglinde Fischlein and Agnes Geißelmann for their excellent assistance.
Conflict of interest
The authors declare that the research was conducted in the absence of any commercial or financial relationships that could be construed as a potential conflict of interest.
Publisher's note
All claims expressed in this article are solely those of the authors and do not necessarily represent those of their affiliated organizations, or those of the publisher, the editors and the reviewers. Any product that may be evaluated in this article, or claim that may be made by its manufacturer, is not guaranteed or endorsed by the publisher.
Supplementary material
The Supplementary Material for this article can be found online at: https://www.frontiersin.org/articles/10.3389/fvets.2022.930005/full#supplementary-material
References
1. European Commission. Working Document on a Severity Assessment Framework. Brussels: European Commission (2012).
2. Smith D, Anderson D, Degryse A-D, Bol C, Criado A, Ferrara A, et al. Classification and reporting of severity experienced by animals used in scientific procedures: felasa/eclam/eslav working group report. Lab Anim. (2018) 52:5–57. doi: 10.1177/0023677217744587
3. Lidster K, Jefferys JG, Blümcke I, Crunelli V, Flecknell P, Frenguelli BG, et al. Opportunities for improving animal welfare in rodent models of epilepsy and seizures. J Neurosci Methods. (2016) 260:2–25. doi: 10.1016/j.jneumeth.2015.09.007
4. Poole T. Happy animals make good science. Lab Anim. (1997) 31:116–24. doi: 10.1258/002367797780600198
5. Jirkof P, Potschka H. Effects of untreated pain, anesthesia, and analgesia in animal experimentation. In: Sánchez Morgado JM, Brønstad A, editors. Experimental Design and Reproducibility in Preclinical Animal Studies. Cham: Springer (2021). p. 105–26. doi: 10.1007/978-3-030-66147-2_5
6. Russell WMS, Burch RL. The Principles of Humane Experimental Technique. London: Methuen & Co., Ltd. (1959).
7. Molony V, Kent J. Assessment of acute pain in farm animals using behavioral and physiological measurements. J Anim Sci. (1997) 75:266–72. doi: 10.2527/1997.751266x
8. Jirkof P, Cesarovic N, Rettich A, Nicholls F, Seifert B, Arras M. Burrowing behavior as an indicator of post-laparotomy pain in mice. Front Behav Neurosci. (2010) 4:165. doi: 10.3389/fnbeh.2010.00165
9. Jirkof P. Burrowing and nest building behavior as indicators of well-being in mice. J Neurosci Methods. (2014) 234:139–46. doi: 10.1016/j.jneumeth.2014.02.001
10. Turner PV, Pang DS, Lofgren JL. A review of pain assessment methods in laboratory rodents. Comp Med. (2019) 69:451–67. doi: 10.30802/AALAS-CM-19-000042
11. Carbone L. Do “prey species” hide their pain? Implications for ethical care and use of laboratory animals. J Appl Anim Ethics Res. (2020) 2:216–36. doi: 10.1163/25889567-BJA10001
12. Apfelbach R, Blanchard CD, Blanchard RJ, Hayes RA, McGregor IS. The effects of predator odors in mammalian prey species: a review of field and laboratory studies. Neurosci Biobehav Rev. (2005) 29:1123–44. doi: 10.1016/j.neubiorev.2005.05.005
13. Deuis JR, Dvorakova LS, Vetter I. Methods used to evaluate pain behaviors in rodents. Front Mol Neurosci. (2017) 10:284. doi: 10.3389/fnmol.2017.00284
14. Flecknell P. Rodent analgesia: assessment and therapeutics. Vet J. (2018) 232:70–7. doi: 10.1016/j.tvjl.2017.12.017
15. Whittaker AL, Howarth GS. Use of spontaneous behaviour measures to assess pain in laboratory rats and mice: how are we progressing? Appl Anim Behav Sci. (2014) 151:1–12. doi: 10.1016/j.applanim.2013.11.001
16. Tappe-Theodor A, Kuner R. Studying ongoing and spontaneous pain in rodents–challenges and opportunities. Euro J Neurosci. (2014) 39:1881–90. doi: 10.1111/ejn.12643
17. Tappe-Theodor A, King T, Morgan MM. Pros and cons of clinically relevant methods to assess pain in rodents. Neurosci Biobehav Rev. (2019) 100:335–43. doi: 10.1016/j.neubiorev.2019.03.009
18. Larson CM, Wilcox GL, Fairbanks CA. The study of pain in rats and mice. Comp Med. (2019) 69:555–70. doi: 10.30802/AALAS-CM-19-000062
19. Oliver VL, Athavale S, Simon KE, Kendall LV, Nemzek JA, Lofgren JL. Evaluation of pain assessment techniques and analgesia efficacy in a female guinea pig (cavia porcellus) model of surgical pain. J Am Assoc Lab Anim Sci. (2017) 56:425–35.
20. Ellen Y, Flecknell P, Leach M. Evaluation of using behavioural changes to assess post-operative pain in the guinea pig (cavia porcellus). PLoS ONE. (2016) 11:e0161941. doi: 10.1371/journal.pone.0161941
21. Leach MC, Allweiler S, Richardson C, Roughan JV, Narbe R, Flecknell PA. Behavioural effects of ovariohysterectomy and oral administration of meloxicam in laboratory housed rabbits. Res Vet Sci. (2009) 87:336–47. doi: 10.1016/j.rvsc.2009.02.001
22. Roughan JV, Flecknell PA. Evaluation of a short duration behaviour-based post-operative pain scoring system in rats. Euro J Pain. (2003) 7:397–406. doi: 10.1016/S1090-3801(02)00140-4
23. Wright-Williams SL, Courade J-P, Richardson CA, Roughan JV, Flecknell PA. Effects of vasectomy surgery and meloxicam treatment on faecal corticosterone levels and behaviour in two strains of laboratory mouse. Pain. (2007) 130:108–18. doi: 10.1016/j.pain.2006.11.003
24. Klune CB, Larkin AE, Leung VS, Pang D. Comparing the rat grimace scale and a composite behaviour score in rats. PLoS ONE. (2019) 14:e0209467. doi: 10.1371/journal.pone.0209467
25. Leach MC, Klaus K, Miller AL, Scotto di Perrotolo M, Sotocinal SG, Flecknell PA. The assessment of post-vasectomy pain in mice using behaviour and the mouse grimace scale. PLoS ONE. (2012) 7:e35656. doi: 10.1371/journal.pone.0035656
26. Andrews N, Legg E, Lisak D, Issop Y, Richardson D, Harper S, et al. Spontaneous Burrowing behaviour in the rat is reduced by peripheral nerve injury or inflammation associated pain. Eur J Pain. (2012) 16:485–95. doi: 10.1016/j.ejpain.2011.07.012
27. Jirkof P, Fleischmann T, Cesarovic N, Rettich A, Vogel J, Arras M. Assessment of postsurgical distress and pain in laboratory mice by nest complexity scoring. Lab Anim. (2013) 47:153–61. doi: 10.1177/0023677213475603
28. Rock ML, Karas AZ, Rodriguez KB, Gallo MS, Pritchett-Corning K, Karas RH, et al. The time-to-integrate-to-nest test as an indicator of wellbeing in laboratory mice. J Am Assoc Lab Anim Sci. (2014) 53:24–8.
29. Jirkof P, Leucht K, Cesarovic N, Caj M, Nicholls F, Rogler G, et al. Burrowing is a sensitive behavioural assay for monitoring general wellbeing during dextran sulfate sodium colitis in laboratory mice. Lab Anim. (2013) 47:274–83. doi: 10.1177/0023677213493409
30. Das V, Kroin JS, Moric M, Buvanendran A. Biochemical and pharmacological characterization of a mice model of complex regional pain syndrome. Reg Anesth Pain Med. (2017) 42:507–16. doi: 10.1097/AAP.0000000000000622
31. Deseure K, Hans G. Orofacial neuropathic pain reduces spontaneous burrowing behavior in rats. Physiol Behav. (2018) 191:91–4. doi: 10.1016/j.physbeh.2018.04.020
32. Balcombe JP. Laboratory environments and rodents' behavioural needs: a review. Lab Anim. (2006) 40:217–35. doi: 10.1258/002367706777611488
33. Langford DJ, Bailey AL, Chanda ML, Clarke SE, Drummond TE, Echols S, et al. Coding of facial expressions of pain in the laboratory mouse. Nat Methods. (2010) 7:447–9. doi: 10.1038/nmeth.1455
34. Sotocinal SG, Sorge RE, Zaloum A, Tuttle AH, Martin LJ, Wieskopf JS, et al. The rat grimace scale: a partially automated method for quantifying pain in the laboratory rat via facial expressions. Mol Pain. (2011) 7:55. doi: 10.1186/1744-8069-7-55
35. Keating SC, Thomas AA, Flecknell PA, Leach MC. Evaluation of EMLA cream for preventing pain during tattooing of rabbits: changes in physiological, behavioural and facial expression responses. PLoS ONE. (2012) 7:e44437. doi: 10.1371/journal.pone.0044437
36. Dalla Costa E, Minero M, Lebelt D, Stucke D, Canali E, Leach MC. Development of the horse grimace scale (Hgs) as a pain assessment tool in horses undergoing routine castration. PLoS ONE. (2014) 9:e92281. doi: 10.1371/journal.pone.0092281
37. Häger C, Biernot S, Buettner M, Glage S, Keubler L, Held N, et al. The sheep grimace scale as an indicator of post-operative distress and pain in laboratory sheep. PLoS ONE. (2017) 12:e0175839. doi: 10.1371/journal.pone.0175839
38. Evangelista MC, Watanabe R, Leung VS, Monteiro BP, O'Toole E, Pang DS, et al. Facial expressions of pain in cats: the development and validation of a feline grimace scale. Sci Rep. (2019) 9:19128. doi: 10.1038/s41598-019-55693-8
39. Ekman P, Friesen WV. Facial Action Coding System Consulting. Palo Alto, CA: Psychologists Press (1978). doi: 10.1037/t27734-000
40. Hohlbaum K, Corte GM, Humpenöder M, Merle R, Thöne-Reineke C. Reliability of the mouse grimace scale in C57bl/6jrj mice. Animals. (2020) 10:1648. doi: 10.3390/ani10091648
41. Mota-Rojas D, Olmos-Hernández A, Verduzco-Mendoza A, Hernández E, Martínez-Burnes J, Whittaker AL. The utility of grimace scales for practical pain assessment in laboratory animals. Animals. (2020) 10:1838. doi: 10.3390/ani10101838
42. Kohn DF, Martin TE, Foley PL, Morris TH, Swindle MM, Vogler GA, et al. Guidelines for the assessment and management of pain in rodents and rabbits. J Am Assoc Lab Anim Sci. (2007) 46:97–108.
43. Mogil JS, Pang DS, Dutra GGS, Chambers CT. The development and use of facial grimace scales for pain measurement in animals. Neurosci Biobehav Rev. (2020) 116:480–93. doi: 10.1016/j.neubiorev.2020.07.013
44. Sorge RE, Martin LJ, Isbester KA, Sotocinal SG, Rosen S, Tuttle AH, et al. Olfactory exposure to males, including men, causes stress and related analgesia in rodents. Nat Methods. (2014) 11:629–32. doi: 10.1038/nmeth.2935
45. Whittaker AL, Liu Y, Barker TH. Methods used and application of the mouse grimace scale in biomedical research 10 years on: a scoping review. Animals. (2021) 11:673. doi: 10.3390/ani11030673
46. Evangelista MC, Monteiro BP, Steagall PV. Measurement properties of grimace scales for pain assessment in non-human mammals: a systematic review. Pain. (2022) 163:e697–714. doi: 10.1097/j.pain.0000000000002474
47. De Vries RB, Hooijmans CR, Langendam MW, van Luijk J, Leenaars M, Ritskes-Hoitinga M, et al. A protocol format for the preparation, registration and publication of systematic reviews of animal intervention studies. Evid Based Preclin Med. (2015) 2:1–9. doi: 10.1002/ebm2.7
48. Hooijmans CR, Rovers MM, de Vries RB, Leenaars M, Ritskes-Hoitinga M, Langendam MW. Syrcle's risk of bias tool for animal studies. BMC Med Res Methodol. (2014) 14:43. doi: 10.1186/1471-2288-14-43
49. Higgins JP, Altman DG, Gøtzsche PC, Jüni P, Moher D, Oxman AD, et al. The cochrane collaboration's tool for assessing risk of bias in randomised trials. Bmj. (2011) 343:d5928. doi: 10.1136/bmj.d5928
50. Page MJ, McKenzie JE, Bossuyt PM, Boutron I, Hoffmann TC, Mulrow CD, et al. The prisma 2020 statement: an updated guideline for reporting systematic reviews. BMJ. (2021) 372:n71. doi: 10.1136/bmj.n71
51. Van der Mierden S, Tsaioun K, Bleich A, Leenaars CH. Software tools for literature screening in systematic reviews in biomedical research. ALTEX. (2019) 36:508–17. doi: 10.14573/altex.1902131
52. Ouzzani M, Hammady H, Fedorowicz Z, Elmagarmid A. Rayyan—a web and mobile app for systematic reviews. Syst Rev. (2016) 5:1–10. doi: 10.1186/s13643-016-0384-4
53. R Core Team. R: A Language Environment for Statistical Computing. Vienna: R Foundation for Statistical Computing (2018). Available online at: https://www.r-project.org/
54. Cho C, Michailidis V, Lecker I, Collymore C, Hanwell D, Loka M, et al. Evaluating analgesic efficacy and administration route following craniotomy in mice using the grimace scale. Sci Rep. (2019) 9:359. doi: 10.1038/s41598-018-36897-w
55. Akintola T, Raver C, Studlack P, Uddin O, Masri R, Keller A. The grimace scale reliably assesses chronic pain in a rodent model of trigeminal neuropathic pain. Neurobiol Pain. (2017) 2:13–7. doi: 10.1016/j.ynpai.2017.10.001
56. Evangelista-Vaz R, Bergadano A, Arras M, Jirkof PD. Analgesic efficacy of subcutaneous-oral dosage of tramadol after surgery in C57bl/6j mice. J Am Assoc Lab Anim Sci. (2018) 57:368–75. doi: 10.30802/AALAS-JAALAS-17-000118
57. Faller KME, McAndrew DJ, Schneider JE, Lygate CA. Refinement of analgesia following thoracotomy and experimental myocardial infarction using the mouse grimace scale. Exp Physiol. (2015) 100:164–72. doi: 10.1113/expphysiol.2014.083139
58. Gallo MS, Karas AZ, Pritchett-Corning K, Garner Guy Mulder JP, Gaskill BN. Tell-Tale tint: does the time to incorporate into nest test evaluate postsurgical pain or welfare in mice? J Am Assoc Lab Anim Sci. (2020) 59:37–45. doi: 10.30802/AALAS-JAALAS-19-000044
59. Jirkof P, Tourvieille A, Cinelli P, Arras M. Buprenorphine for pain relief in mice: repeated injections vs sustained-release depot formulation. Lab Anim. (2015) 49:177–87. doi: 10.1177/0023677214562849
60. Hsi ZY, Stewart LA, Lloyd KCK, Grimsrud KN. Hypoglycemia after bariatric surgery in mice and optimal dosage and efficacy of glucose supplementation. Comp Med. (2020) 70:111–8. doi: 10.30802/AALAS-CM-19-000015
61. Jirkof P, Arras M, Cesarovic N. Tramadol:Paracetamol in drinking water for treatment of post-surgical pain in laboratory mice. Appl Anim Behav Sci. (2018) 198:95–100. doi: 10.1016/j.applanim.2017.09.021
62. Miller AL, Kitson GL, Skalkoyannis B, Flecknell PA, Leach MC. Using the mouse grimace scale and behaviour to assess pain in Cba mice following vasectomy. Appl Anim Behav Sci. (2016) 181:160–5. doi: 10.1016/j.applanim.2016.05.020
63. Mai SHC, Sharma N, Kwong AC, Dwivedi DJ, Khan M, Grin PM, et al. Body temperature and mouse scoring systems as surrogate markers of death in cecal ligation and puncture sepsis. Intensive Care Med Exp. (2018) 6:20. doi: 10.1186/s40635-018-0184-3
64. Matsumiya LC, Sorge RE, Sotocinal SG, Tabaka JM, Wieskopf JS, Zaloum A, et al. Using the mouse grimace scale to reevaluate the efficacy of postoperative analgesics in laboratory mice. J Am Assoc Lab Anim Sci. (2012) 51:42–9.
65. Roughan JV, Bertrand HGMJ, Isles HM. Meloxicam prevents cox-2-mediated post-surgical inflammation but not pain following laparotomy in mice. Euro J Pain. (2016) 20:231–40. doi: 10.1002/ejp.712
66. Tuttle AH, Molinaro MJ, Jethwa JF, Sotocinal SG, Prieto JC, Styner MA, et al. A deep neural network to assess spontaneous pain from mouse facial expressions. Mol Pain. (2018) 14:1744806918763658. doi: 10.1177/1744806918763658
67. Redaelli V, Papa S, Marsella G, Grignaschi G, Bosi A, Ludwig N, et al. A refinement approach in a mouse model of rehabilitation research. Analgesia strategy, reduction approach and infrared thermography in spinal cord injury. PLoS ONE. (2019) 14:e0224337. doi: 10.1371/journal.pone.0224337
68. Sauer M, Fleischmann T, Lipiski M, Arras M, Jirkof P. Buprenorphine via drinking water and combined oral-injection protocols for pain relief in mice. Appl Anim Behav Sci. (2016) 185:103–12. doi: 10.1016/j.applanim.2016.09.009
69. Dwivedi DJ, Grin PM, Khan M, Prat A, Zhou J, Fox-Robichaud AE, et al. Differential expression of Pcsk9 modulates infection, inflammation, and coagulation in a murine model of sepsis. Shock. (2016) 46:672–80. doi: 10.1097/SHK.0000000000000682
70. Korat PS, Kapupara P. Analgesic effect of local infiltration of the surgical wound containing levobupivacaine, ibuprofen, and epinephrine in rats undergoing laparotomy. Folia Med. (2018) 60:254–60. doi: 10.1515/folmed-2017-0096
71. Chaves RHF, Souza CC, Furlaneto IP, Teixeira RKC, Oliveira CP, Rodrigues EM, et al. Influence of tramadol on functional recovery of acute spinal cord injury in rats. Acta Cir Bras. (2018) 33:1087–94. doi: 10.1590/s0102-865020180120000006
72. Chi H, Kawano T, Tamura T, Iwata H, Takahashi Y, Eguchi S, et al. Postoperative pain impairs subsequent performance on a spatial memory task via effects on n-methyl-D-aspartate receptor in aged rats. Life Sci. (2013) 93:986–93. doi: 10.1016/j.lfs.2013.10.028
73. Clemensen J, Rasmussen LV, Abelson KSP. Transdermal fentanyl solution provides long-term analgesia in the hind-paw incisional model of postoperative pain in male rats. In Vivo. (2018) 32:713–9. doi: 10.21873/invivo.11299
74. De Rantere D, Schuster CJ, Reimer JN, Pang DS. The relationship between the rat grimace scale and mechanical hypersensitivity testing in three experimental pain models. Eur J Pain. (2016) 20:417–26. doi: 10.1002/ejp.742
75. Fujita M, Fukuda T, Sato Y, Takasusuki T, Tanaka M. Allopregnanolone suppresses mechanical allodynia and internalization of neurokinin-1 receptors at the spinal dorsal horn in a rat postoperative pain model. Korean J Pain. (2018) 31:10–5. doi: 10.3344/kjp.2018.31.1.10
76. Gao Z, Cui F, Cao X, Wang D, Li X, Li T. Local infiltration of the surgical wounds with levobupivacaine, dexibuprofen, and norepinephrine to reduce postoperative pain: a randomized, vehicle-controlled, and preclinical study. Biomed Pharmacother. (2017) 92:459–67. doi: 10.1016/j.biopha.2017.05.038
77. Guo P, Hu SP. Thalidomide alleviates postoperative pain and spatial memory deficit in aged rats. Biomed Pharmacother. (2017) 95:583–8. doi: 10.1016/j.biopha.2017.08.114
78. Harikrishnan VS, Krishnan LK, Abelson KSP. A novel technique to develop thoracic spinal laminectomy and a methodology to assess the functionality and welfare of the contusion spinal cord injury (sci) rat model. PLoS ONE. (2019) 14:e0219001. doi: 10.1371/journal.pone.0219001
79. Kawano T, Eguchi S, Iwata H, Yamanaka D, Tateiwa H, Locatelli FM, et al. Effects and underlying mechanisms of endotoxemia on post-incisional pain in rats. Life Sci. (2016) 148:145–53. doi: 10.1016/j.lfs.2016.01.046
80. Kawano T, Takahashi T, Iwata H, Morikawa A, Imori S, Waki S, et al. Effects of ketoprofen for prevention of postoperative cognitive dysfunction in aged rats. J Anesth. (2014) 28:932–6. doi: 10.1007/s00540-014-1821-y
81. Korat PS, Kapupara PP. Local infiltration of the surgical wound with levobupivacaine, ibuprofen, and epinephrine in postoperative pain: an experimental study. Biomed Pharmacother. (2017) 96:104–11. doi: 10.1016/j.biopha.2017.09.131
82. Koyama T, Kawano T, Iwata H, Aoyama B, Eguchi S, Nishigaki A, et al. Acute postoperative pain exacerbates neuroinflammation and related delirium-like cognitive dysfunction in rats. J Anesth. (2019) 33:482–6. doi: 10.1007/s00540-019-02635-3
83. Nunamaker EA, Goldman JL, Adams CR, Fortman JD. Evaluation of analgesic efficacy of meloxicam and 2 formulations of buprenorphine after laparotomy in female Sprague–Dawley rats. J Am Assoc Lab Anim Sci. (2018) 57:498–507. doi: 10.30802/AALAS-JAALAS-17-000129
84. Oliver V, De Rantere D, Ritchie R, Chisholm J, Hecker KG, Pang DS. Psychometric assessment of the rat grimace scale and development of an analgesic intervention score. PLoS ONE. (2014) 9:e97882. doi: 10.1371/journal.pone.0097882
85. Philips BH, Weisshaar CL, Winkelstein BA. Use of the rat grimace scale to evaluate neuropathic pain in a model of cervical radiculopathy. Comp Med. (2017) 67:34–42.
86. Saine L, Hélie P, Vachon P. Effects of fentanyl on pain and motor behaviors following a collagenase-induced intracerebral hemorrhage in rats. J Pain Res. (2016) 9:1039. doi: 10.2147/JPR.S121415
87. Thomas A, Miller A, Roughan J, Malik A, Haylor K, Sandersen C, et al. Efficacy of intrathecal morphine in a model of surgical pain in rats. PLoS ONE. (2016) 11:e0163909. doi: 10.1371/journal.pone.0163909
88. Waite ME, Tomkovich A, Quinn TL, Schumann AP, Dewberry L, Totsch SK, et al. Efficacy of common analgesics for postsurgical pain in rats. J Am Assoc Lab Anim Sci. (2015) 54:420–5.
89. Yamanaka D, Kawano T, Nishigaki A, Aoyama B, Tateiwa H, Shigematsu-Locatelli M, et al. The preventive effects of dexmedetomidine on endotoxin-induced exacerbated post-incisional pain in rats. J Anesth. (2017) 31:664–71. doi: 10.1007/s00540-017-2374-7
90. Yousef MAA, Dionigi P, Marconi S, Calligaro A, Cornaglia AI, Alfonsi E, et al. Successful reconstruction of nerve defects using distraction neurogenesis with a new experimental device. Basic Clin Neurosci. (2015) 6:253.
91. Préfontaine L, Hélie P, Vachon P. Postoperative pain in sprague dawley rats after liver biopsy by laparotomy versus laparoscopy. Lab Anim. (2015) 44:174–8. doi: 10.1038/laban.731
92. Schneider LE, Henley KY, Turner OA, Pat B, Niedzielko TL, Floyd CL. Application of the rat grimace scale as a marker of supraspinal pain sensation after cervical spinal cord injury. J Neurotrauma. (2017) 34:2982–93. doi: 10.1089/neu.2016.4665
93. Kawano T, Yamanaka D, Aoyama B, Tateiwa H, Shigematsu-Locatelli M, Nishigaki A, et al. Involvement of acute neuroinflammation in postoperative delirium-like cognitive deficits in rats. J Anesth. (2018) 32:506–17. doi: 10.1007/s00540-018-2504-x
94. Jeger V, Arrigo M, Hildenbrand FF, Müller D, Jirkof P, Hauffe T, et al. Improving animal welfare using continuous nalbuphine infusion in a long-term rat model of sepsis. Intensive Care Med Exp. (2017) 5:1–13. doi: 10.1186/s40635-017-0137-2
95. Locatelli FM, Kawano T, Iwata H, Aoyama B, Eguchi S, Nishigaki A, et al. Resveratrol-Loaded nanoemulsion prevents cognitive decline after abdominal surgery in aged rats. J Pharmacol Sci. (2018) 137:395–402. doi: 10.1016/j.jphs.2018.08.006
96. Abdelrahman A, Kumstel S, Zhang X, Liebig M, Wendt EHU, Eichberg J, et al. A novel multi-parametric analysis of non-invasive methods to assess animal distress during chronic pancreatitis. Sci Rep. (2019) 9:14084. doi: 10.1038/s41598-019-50682-3
97. Arras M, Rettich A, Cinelli P, Kasermann HP, Burki K. Assessment of post-laparotomy pain in laboratory mice by telemetric recording of heart rate and heart rate variability. BMC Vet Res. (2007) 3:16. doi: 10.1186/1746-6148-3-16
98. Beninson JA, Lofgren JL, Lester PA, Hileman MM, Berkowitz DJ, Myers DD Jr. Analgesic efficacy and hematologic effects of robenacoxib in mice. J Am Assoc Lab Anim Sci. (2018) 57:258–67.
99. Cesarovic N, Arras M, Jirkof P. Impact of inhalation anaesthesia, surgery and analgesic treatment on home cage behaviour in laboratory mice. Appl Anim Behav Sci. (2014) 157:137–45. doi: 10.1016/j.applanim.2014.04.010
100. Falkenberg MK, Teilmann AC, Henriksen T, Hau J, Poulsen HE, Abelson KS. Clinical, physiologic, and behavioral evaluation of permanently catheterized nmri mice. J Am Assoc Lab Anim Sci. (2019) 58:380–9. doi: 10.30802/AALAS-JAALAS-18-000060
101. Herndon NL, Bandyopadhyay S, Hod EA, Prestia KA. Sustained-Release buprenorphine improves postsurgical clinical condition but does not alter survival or cytokine levels in a murine model of polymicrobial sepsis. Comp Med. (2016) 66:455–62.
102. Jirkof P, Cesarovic N, Rettich A, Fleischmann T, Arras M. Individual housing of female mice: influence on postsurgical behaviour and recovery. Lab Anim. (2012) 46:325–34. doi: 10.1258/la.2012.012027
103. Jirkof P, Cesarovic N, Rettich A, Arras M. Housing of female mice in a new environment and its influence on post-surgical behaviour and recovery. Appl Anim Behav Sci. (2013) 148:209–17. doi: 10.1016/j.applanim.2013.08.006
104. Kendall LV, Wegenast DJ, Smith BJ, Dorsey KM, Kang S, Lee NY, et al. Efficacy of sustained-release buprenorphine in an experimental laparotomy model in female mice. J Am Assoc Lab Anim Sci. (2016) 55:66–73.
105. Kumstel S, Vasudevan P, Palme R, Zhang X, Wendt EHU, David R, et al. Benefits of non-invasive methods compared to telemetry for distress analysis in a murine model of pancreatic cancer. J Adv Res. (2020) 21:35–47. doi: 10.1016/j.jare.2019.09.002
106. Oliver VL, Thurston SE, Lofgren JL. Using cageside measures to evaluate analgesic efficacy in mice (Mus musculus) after surgery. J Am Assoc Lab Anim Sci. (2018) 57:186–201.
107. Pham T, Hagman B, Codita A, Van Loo P, Strömmer L, Baumans V. Housing environment influences the need for pain relief during post-operative recovery in mice. Physiol Behav. (2010) 99:663–8. doi: 10.1016/j.physbeh.2010.01.038
108. Robinson-Junker A, O'Hara B, Durkes A, Gaskill B. Sleeping through anything: the effects of unpredictable disruptions on mouse sleep, healing, and affect. PLoS ONE. (2019) 14:e0210620. doi: 10.1371/journal.pone.0210620
109. Staib-Lasarzik I, Nagel N, Sebastiani A, Griemert E-V, Thal SC. Analgesic treatment limits surrogate parameters for early stress and pain response after experimental subarachnoid hemorrhage. BMC Neurosci. (2019) 20:49. doi: 10.1186/s12868-019-0531-7
110. Van Loo PL, Kuin N, Sommer R, Avsaroglu H, Pham T, Baumans V. Impact of 'living apart together' on postoperative recovery of mice compared with social and individual housing. Lab Anim. (2007) 41:441–55. doi: 10.1258/002367707782314328
111. Yuan D, Liu C, Wu J, Hu B. Nest-Building activity as a reproducible and long-term stroke deficit test in a mouse model of stroke. Brain Behav. (2018) 8:e00993. doi: 10.1002/brb3.993
112. Möller C, Wolf F, van Dijk RM, Di Liberto V, Russmann V, Keck M, et al. Toward evidence-based severity assessment in rat models with repeated seizures: I. Electrical kindling. Epilepsia. (2018) 59:765–77. doi: 10.1111/epi.14028
113. Shepherd AJ, Cloud ME, Cao Y-Q, Mohapatra DP. Deficits in burrowing behaviors are associated with mouse models of neuropathic but not inflammatory pain or migraine. Front Behav Neurosci. (2018) 12:124. doi: 10.3389/fnbeh.2018.00124
114. Shi C, Das V, Li X, Kc R, Qiu S, O-Sullivan I, et al. Development of an in vivo mouse model of discogenic low back pain. J Cell Physiol. (2018) 233:6589–602. doi: 10.1002/jcp.26280
115. Georgieva M, Wei Y, Dumitrascuta M, Pertwee R, Finnerup NB, Huang W. Fatty acid suppression of glial activation prevents central neuropathic pain after spinal cord injury. Pain. (2019) 160:2724–42. doi: 10.1097/j.pain.0000000000001670
116. Katri A, Dabrowska A, Löfvall H, Karsdal MA, Andreassen KV, Thudium CS, et al. A dual amylin and calcitonin receptor agonist inhibits pain behavior and reduces cartilage pathology in an osteoarthritis rat model. Osteoarthritis Cartilage. (2019) 27:1339–46. doi: 10.1016/j.joca.2019.05.016
117. Lau W, Dykstra C, Thevarkunnel S, Silenieks LB, de Lannoy IA, Lee DK, et al. A back translation of pregabalin and carbamazepine against evoked and non-evoked endpoints in the rat spared nerve injury model of neuropathic pain. Neuropharmacology. (2013) 73:204–15. doi: 10.1016/j.neuropharm.2013.05.023
118. Muralidharan A, Kuo A, Jacob M, Lourdesamy JS, Carvalho LMSPD, Nicholson JR, et al. Comparison of burrowing and stimuli-evoked pain behaviors as end-points in rat models of inflammatory pain and peripheral neuropathic pain. Front Behav Neurosci. (2016) 10:88. doi: 10.3389/fnbeh.2016.00088
119. Leenaars C, Tsaioun K, Stafleu F, Rooney K, Meijboom F, Ritskes-Hoitinga M, et al. Reviewing the animal literature: how to describe and choose between different types of literature reviews. Lab Anim. (2021) 55:129–41. doi: 10.1177/0023677220968599
120. Rix A, Girbig R, Porte C, Lederle W, Leenaars C, Kiessling F. Development of a systematic review protocol and a scoping review of ultrasound-induced immune effects in peripheral tumors. Mol Imaging Biol. (2021) 24:288–97. doi: 10.1007/s11307-021-01686-x
121. Younger J, McCue R, Mackey S. Pain outcomes: a brief review of instruments and techniques. Curr Pain Headache Rep. (2009) 13:39–43. doi: 10.1007/s11916-009-0009-x
122. Wright-Williams S, Flecknell PA, Roughan JV. Comparative effects of vasectomy surgery and buprenorphine treatment on faecal corticosterone concentrations and behaviour assessed by manual and automated analysis methods in C57 and C3h mice. PLoS ONE. (2013) 8:e75948. doi: 10.1371/journal.pone.0075948
123. Williams ACdC. Facial expression of pain: an evolutionary account. Behav Brain Sci. (2002) 25:439–55. doi: 10.1017/S0140525X02000080
124. Kappesser J. The facial expression of pain in humans considered from a social perspective. Philos Trans R SocB. (2019) 374:20190284. doi: 10.1098/rstb.2019.0284
125. Grunau RV, Craig KD. Pain expression in neonates: facial action and cry. Pain. (1987) 28:395–410. doi: 10.1016/0304-3959(87)90073-X
126. Chambers CT, Mogil JS. Ontogeny and phylogeny of facial expression of pain. Pain. (2015) 156:798–9. doi: 10.1097/j.pain.0000000000000133
127. Miller AL, Leach MC. The mouse grimace scale: a clinically useful tool? PLoS ONE. (2015) 10:e0136000. doi: 10.1371/journal.pone.0136000
128. Ernst L, Bruch S, Kopaczka M, Merhof D, Bleich A, Tolba RH, et al. A model-specific simplification of the Mouse Grimace Scale based on the pain response of intraperitoneal CCl4 injections. Sci Rep. (2022) 12:1–10. doi: 10.1038/s41598-022-14852-0
129. Zhang EQ, Leung VS, Pang DS. Influence of rater training on inter-and intrarater reliability when using the rat grimace scale. J Am Assoc Lab Anim Sci. (2019) 58:178–83. doi: 10.30802/AALAS-JAALAS-18-000044
130. Roughan JV, Flecknell PA. Training in behaviour-based post-operative pain scoring in rats—an evaluation based on improved recognition of analgesic requirements. Appl Anim Behav Sci. (2006) 96:327–42. doi: 10.1016/j.applanim.2005.06.012
131. Van Loo P, Baumans V. The importance of learning young: the use of nesting material in laboratory rats. Lab Anim. (2004) 38:17–24. doi: 10.1258/00236770460734353
132. Schwabe K, Boldt L, Bleich A, van Dijk RM, Helgers SOA, Häger C, et al. Nest-Building performance in rats: impact of vendor, experience, and sex. Lab Anim. (2020) 54:17–25. doi: 10.1177/0023677219862004
133. Durst MS, Arras M, Palme R, Talbot SR, Jirkof P. Lidocaine and bupivacaine as part of multimodal pain management in a C57bl/6j laparotomy mouse model. Sci Rep. (2021) 11:10918. doi: 10.1038/s41598-021-90331-2
134. Cunningham C, Deacon R, Wells H, Boche D, Waters S, Diniz CP, et al. Synaptic changes characterize early behavioural signs in the Me7 model of murine prion disease. Euro J Neurosci. (2003) 17:2147–55. doi: 10.1046/j.1460-9568.2003.02662.x
135. Deacon RM, Reisel D, Perry VH, Nicholas J, Rawlins P. Hippocampal scrapie infection impairs operant Drl performance in mice. Behav Brain Res. (2005) 157:99–105. doi: 10.1016/j.bbr.2004.06.013
136. Aubert A, Goodall G, Dantzer R, Gheusi G. Differential effects of lipopolysaccharide on pup retrieving and nest building in lactating mice. Brain Behav Immun. (1997) 11:107–18. doi: 10.1006/brbi.1997.0485
137. Sager TN, Kirchhoff J, Mørk A, Van Beek J, Thirstrup K, Didriksen M, et al. Nest building performance following Mptp toxicity in mice. Behav Brain Res. (2010) 208:444–9. doi: 10.1016/j.bbr.2009.12.014
138. Belforte JE, Zsiros V, Sklar ER, Jiang Z, Yu G, Li Y, et al. Postnatal Nmda receptor ablation in corticolimbic interneurons confers schizophrenia-like phenotypes. Nat Neurosci. (2010) 13:76–83. doi: 10.1038/nn.2447
139. Wodarski R, Delaney A, Ultenius C, Morland R, Andrews N, Baastrup C, et al. Cross-Centre replication of suppressed burrowing behaviour as an ethologically relevant pain outcome measure in the rat: a prospective multicentre study. Pain. (2016) 157:2350–65. doi: 10.1097/j.pain.0000000000000657
140. Bryden LA, Nicholson JR, Doods H, Pekcec A. Deficits in spontaneous burrowing behavior in the rat bilateral monosodium iodoacetate model of osteoarthritis: an objective measure of pain-related behavior and analgesic efficacy. Osteoarthritis Cartilage. (2015) 23:1605–12. doi: 10.1016/j.joca.2015.05.001
141. Gould SA, Doods H, Lamla T, Pekcec A. Pharmacological characterization of intraplantar complete freund's adjuvant-induced burrowing deficits. Behav Brain Res. (2016) 301:142–51. doi: 10.1016/j.bbr.2015.12.019
142. Lencioni GC, de Sousa RV, de Souza Sardinha EJ, Corrêa RR, Zanella AJ. Pain assessment in horses using automatic facial expression recognition through deep learning-based modeling. PLoS ONE. (2021) 16:e0258672. doi: 10.1371/journal.pone.0258672
143. Ernst L, Kopaczka M, Schulz M, Talbot SR, Zieglowski L, Meyer M, et al. Improvement of the mouse grimace scale set-up for implementing a semi-automated mouse grimace scale scoring (part 1). Lab Anim. (2020) 54:83–91. doi: 10.1177/0023677219881655
144. Ernst L, Kopaczka M, Schulz M, Talbot SR, Struve B, Häger C, et al. Semi-Automated generation of pictures for the mouse grimace scale: a multi-laboratory analysis (part 2). Lab Anim. (2020) 54:92–8. doi: 10.1177/0023677219881664
145. Mota-Rojas D, Olmos-Hernández A, Verduzco-Mendoza A, Lecona-Butrón H, Martínez-Burnes J, Mora-Medina P, et al. Infrared thermal imaging associated with pain in laboratory animals. Exp Anim. (2021) 70:1–12. doi: 10.1538/expanim.20-0052
146. Verduzco-Mendoza A, Bueno-Nava A, Wang D, Martínez-Burnes J, Olmos-Hernández A, Casas A, et al. Experimental applications and factors involved in validating thermal windows using infrared thermography to assess the health and thermostability of laboratory animals. Animals. (2021) 11:3448. doi: 10.3390/ani11123448
147. Barbosa Pereira C, Czaplik M, Blazek V, Leonhardt S, Teichmann D. Monitoring of cardiorespiratory signals using thermal imaging: a pilot study on healthy human subjects. Sensors. (2018) 18:1541. doi: 10.3390/s18051541
Keywords: pain assessment, RGS, MGS, facial expression, home cage behavior, rodents
Citation: Aulehner K, Leenaars C, Buchecker V, Stirling H, Schönhoff K, King H, Häger C, Koska I, Jirkof P, Bleich A, Bankstahl M and Potschka H (2022) Grimace scale, burrowing, and nest building for the assessment of post-surgical pain in mice and rats—A systematic review. Front. Vet. Sci. 9:930005. doi: 10.3389/fvets.2022.930005
Received: 27 April 2022; Accepted: 22 August 2022;
Published: 06 October 2022.
Edited by:
Daniel Mota-Rojas, Metropolitan Autonomous University, MexicoReviewed by:
Alexandra Whittaker, University of Adelaide, AustraliaAntonio Bueno-Nava, National Institute of Rehabilitation Luis Guillermo Ibarra Ibarra, Mexico
Copyright © 2022 Aulehner, Leenaars, Buchecker, Stirling, Schönhoff, King, Häger, Koska, Jirkof, Bleich, Bankstahl and Potschka. This is an open-access article distributed under the terms of the Creative Commons Attribution License (CC BY). The use, distribution or reproduction in other forums is permitted, provided the original author(s) and the copyright owner(s) are credited and that the original publication in this journal is cited, in accordance with accepted academic practice. No use, distribution or reproduction is permitted which does not comply with these terms.
*Correspondence: Heidrun Potschka, potschka@pharmtox.vetmed.uni-muenchen.de
†Present address: Ines Koska, Department of Drug Design and Target Validation, Fraunhofer Institute for Cell Therapy and Immunology, Leipzig, Germany