- 1College of Veterinary Medicine, Shandong Agricultural University, Tai'an, China
- 2Shandong Provincial Key Laboratory of Animal Biotechnology and Disease Control and Prevention, Tai'an, China
- 3Shandong Provincial Engineering Technology Research Center of Animal Disease Control and Prevention, Tai'an, China
Chicken infectious anemia virus (CIAV) can be transmitted by contaminated live vaccines, and causes huge economic losses. This study evaluated the contamination status of CIAV in 24 batches of vaccines by recombinase-aided amplification assay (RAA), fluorescence quantitative PCR and dot blot assay, and then found a contaminated attenuated vaccine. The whole genome of the CIAV contaminant was then sequenced and named JS2020-PFV (Genbank accession number: MW234428, 2296bp). It showed 94.5 to 99.9% identities with reference strains and shared the closest evolution relationship with AB1K strain which was isolated from a chicken farm in Turkey. All of these suggested that the use of CIAV contaminated live vaccine may be one of the reason for its epidemic in poultry.
Introduction
Chicken infectious anemia virus (CIAV) induces severe anemia and immunosuppression in poultry (1). After its first report in 1979 (2), CIAV has been detected worldwide and has caused huge economic losses (3). Recently, CIAV infections have also frequently appeared in different chicken farms in China (4–8). The pathogenesis of CIAV has been well understood that it mainly causes the atrophy of bone marrow, hematopoietic tissue, and lymphatic tissues (e.g., thymus) in young chickens (9). CIAV can be transmitted both vertically and horizontally via respiratory and digestive tracts (1), but how it spreads across different regions and even countries remains unclear.
It is worth noting that the first strain of CIAV was isolated from the contaminated attenuated vaccine (2). And, after that, similar reports emerged in a lot of countries (10–12), revealing that the use of contaminated live vaccines is an important way of the transmission of CIAV. Through interactions with attenuated vaccine strains or co-infected viruses, CIAV triggers more serious clinical symptoms with a strong immunosuppressive ability (13–15). More importantly, the attenuated vaccines are usually inoculated at a low age, the CIAV infection caused by it will greatly interfere with the immune responses against vaccines, resulting in a secondary infection (16, 17). Therefore, monitoring the vaccine contaminations is of great significance for preventing CIAV infection in poultry.
This study aims to screen the potential CIAV contamination in live vaccines by recombinase-aided amplification (RAA), fluorescence quantitative PCR assay (qPCR) and dot blot. The whole genome of the contaminated CIAV strain was sequenced for further analysis.
Materials and Methods
Vaccine Collection
Twenty-four batches of live vaccines against Newcastle disease virus (n = 6), infectious bronchitis (n = 5), infectious laryngotracheitis encephalomyelitis (n = 4), fowl pox (n = 4), Marek disease virus (n = 3), mycoplasma gallisepticum (n = 1), viral arthritis (n = 1), were collected from a layer chicken farm in Heibei province from Jun 2020 to Jun 2021. All these vaccines were selected randomly from the same batch of vaccines purchased by this chicken farm before vaccination for detecting potential exogenous virus contaminations. All vaccines were not opened before testing. All these vaccines were diluted with normal saline according to the instructions, and 200 μl of the dilute was used for DNA extraction.
DNA Extraction
DNA was extracted using the DNA extraction kit (Omega, Bio-Tek, USA) according to the manufacturer's instructions as the template for RAA, qPCR and dot blot.
RAA
The primers and probe were designed according to the published CIAV genome (Tables 1, 2). The RAA fluorescence kit and RAA amplification instrument were provided by Hangzhou ZC Bio-Sci & Tech Co., Ltd. (Hangzhou, China). ddH2O was used as the negative control and standard plasmid containing full-length CIAV genomes was constructed by our lab and used as a positive control (7). RAA detection methods and primer probes are shown in Table 1.
Fluorescent Quantitative PCR
With the fluorescent quantitative PCR kit produced by TaKaRa Biotechnology (Takara, Dalian, Chian), the sample DNA was detected and verified by the fluorescent quantitative PCR method according to the references (7).
Dot Blot Hybridization
The samples were tested by PCR combined with dot blot hybridization (18), and the nitrocellulose membrane was purchased from Boehringer Millipore (Merck, Germany).
Amplification and Sequencing of the Whole Genome of Samples
According to the sequence published in the GenBank, three primers were designed to amplify the sample DNA using DNAStar 6.0 application. The primers are shown in Table 1. The amplification procedure was as follows: 94°C for 5 min; 94°C for 30 s, 55°C for 30 s, and 72°C for 30 s (34 cycles); 72°C for 10 min (35 cycles); and 4°C. The PCR products were identified using 1% agarose gel electrophoresis and stained with ethidium bromide. The bands were purified with an Omega gel recovery and purification kit (Omega Bio-Tek, Norcross, GA, USA), and the recovered target fragments were attached to the pMD18-T vector (Takara, Dalian, Chian). After overnight ligation at 16°C, the ligation products were transformed into competent cells of E. coli DH5α (Takara, Japan), and the positive clones were screened. Finally, the bacterial liquid identified as positive by PCR was sent to Shanghai Bioengineering Co., Ltd. for sequencing.
Sequence Analysis
The DNA sequences were assembled using DNAStar (version 6.0). Multiple sequence alignment was performed using the Clustal W (BioEdit version 7.0) program, and the comparison of sequence identity was performed using MegAlign software (DNAStar). Phylogenetic analysis was performed using the maximum likelihood (ML) method on RAxML.
Results
Results of CIAV Nucleic Acid Test in Vaccines
RAA, qPCR assay, and dot blot were employed to detect CIAV contamination in live vaccines. Results showed that a fowlpox attenuated vaccine sample was determined as CIAV positive in all three methods (Figure 1).
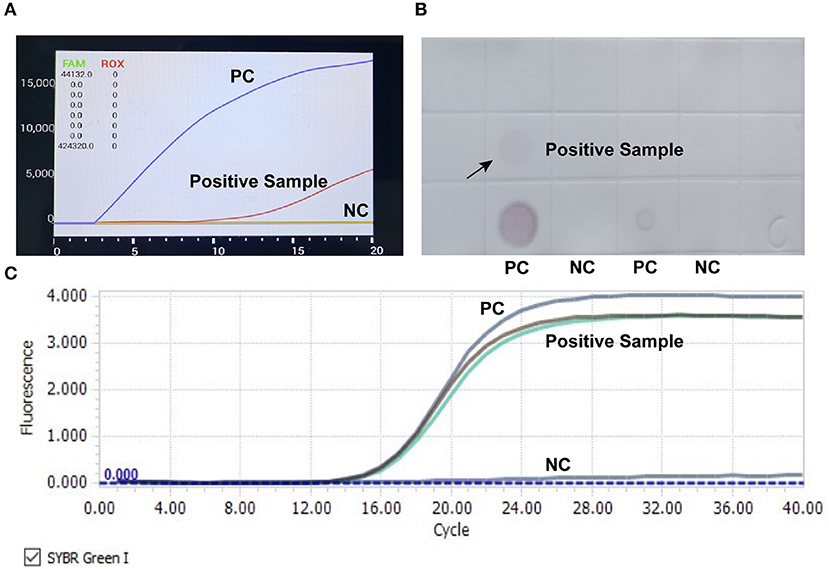
Figure 1. Detection of CIAV nucleic acid in attenuated chicken pox vaccine. RAA rapid assay (A), nucleic acid dot hybridization (B), and fluorescence quantitative PCR assay (C) were simultaneously employed to detect CIAV contamination in vaccine samples, and all the methods showed positive results. PC, positive control; NC, negative control.
Whole-Genome Sequencing
To further reveal the molecular characteristics of the CIAV contaminants, the whole genome of this strain was sequenced using three pairs of primers. The electrophoretic analysis confirmed the positive amplification by PCR, and the three segments were 842bp, 990bp and 802bp, respectively (Figure 2), which was consistent with the expected band size. Furthermore, the whole genome of this isolate was 2296 bp, covering three open-reading-frame (ORF), namely VP1, VP2, and VP3.
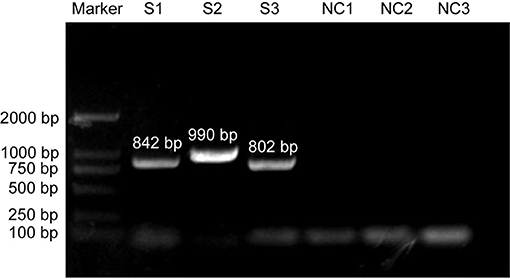
Figure 2. PCR amplication of CIAV in contaminated vaccine using three pairs of primers. S: segment of CIAV; NC: negative control.
The lengths of VP1, VP2 and VP3 were 1350 bp, 651 bp and 366 bp, respectively. The isolate was named JS2020-PFV and its sequence was uploaded to the GenBank gene library with the accession number of MW234428.
Genome Analysis of JS2020-PFV
The whole genome of the JS2020-PFV strain was compared with that of 56 other CIAV strains. Results showed that JS2020-PFV shared 94.5 to 99.9% of identities with other reference strains. Among them, JS2020-PFV had the highest identity (99%) with the AB1K strain (MT259319) isolated from a chicken farm in Turkey. A phylogenetic tree was constructed based on the whole genome sequence of JS2020-PFV and reference strains, and the results further confirmed the closest evolution relationship between JS2020-PFV and AB1K (Figure 3).
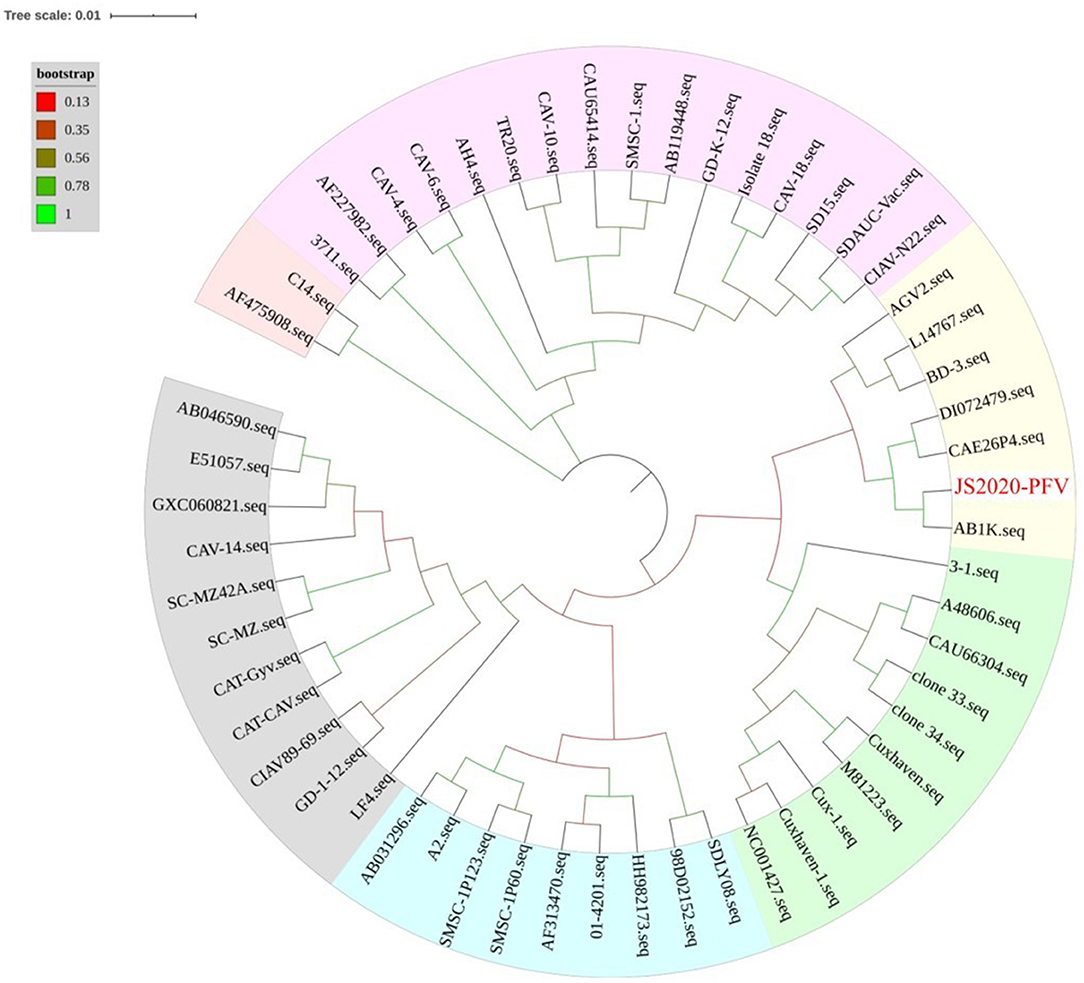
Figure 3. Phylogenetic tree of full-length viral genome of JS2020-PFV and reference strains. The isolated strain in this study has been highlighted in red. The tree was constructed by the maximum likelihood method with 1,000 bootstrap replicates using MEGA 5.0.
Analysis of VP1 Gene Variation in JS2020-PFV Strain
The VP1 gene of the JS2020-PFV strain shared 94.0 to 99.9% identities with the reference strains, with the highest identity (99.9%) with AB1K. The genetic evolution relationship between the VP1 gene of the JS2020-PFV strain and reference strains was consistent with the whole genome sequence (Figure 3). Further analysis of the VP1 gene of JS2020-PFV strain revealed that there are two amino acid mutations within which had never been found before, namely threonine at position 89 of VP1 protein changed to lysine and glutamine at position 448 changed to lysine (Table 3).
Difference Analysis of Transcriptional Regulatory Elements in Non-coding Regions
The Clustal W method was used for multiple alignment analysis of the non-coding regions of JS2020-PFV with 9 reference strains. Compared with the reference strains, the motif in non-coding regions of CIAV was highly conserved (Figure 4). Softberry's Nsite online service analysis showed that four CREB sites associated with viral apoptotic capacity were also conserved in the new isolate. The JS2020-PFV and most of the reference strains all had four DR regions in the non-coding region, except for one additional DR region in the CUX-1 strain.
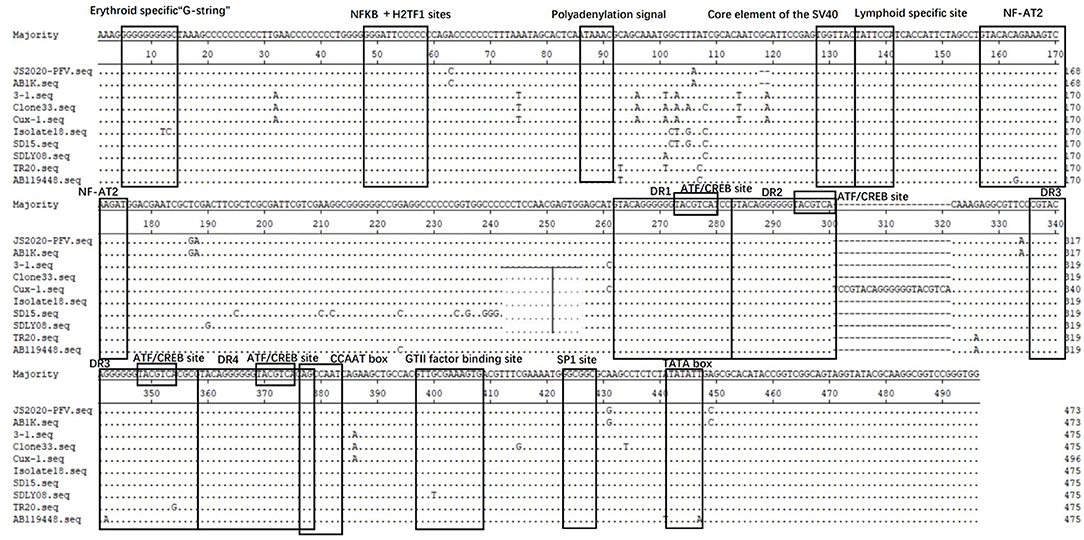
Figure 4. Structural analysis of the non-coding region of JS2020-PFV strain and reference strains. The sequences in black frames are the motifs of transcriptional regulatory elements in this study.
Discussion
Over the past two decades, many live vaccines have been proved to be contaminated with exogenous viruses, including avian leukosis virus (19), fowl adenovirus (20), reticuloendotheliosis virus (26), CIAV (6) and others. All these viruses share the common feature of being able to vertically propagate through the chicken embryo, suggesting such contamination is mainly caused by the use of SPF chicken embryos infected with exogenous viruses during vaccine production. Several studies have reported the infection of the above viruses in SPF chicken farms, which further confirmed the above hypothesis (21). The use of contaminated vaccines will not only cause the spread of the virus but also cause serious clinical symptoms, especially vaccines contaminated with CIAV that induce strong immunosuppression. Previous studies demonstrated that the co-infection with CIAV can significantly improve the pathogenicity of the LaSota strain in NDV vaccines, and decrease the antibody titer, which makes the vaccinated chickens vulnerable to wild NDV strains (7). Therefore, monitoring the exogenous virus contamination in live vaccination is of great significance for poultry farms.
In this study, RAA assay, the traditional qPCR and dot blot were used at the same time to screen the potential contamination of CIAV in live vaccines. Results found that one fowl pox vaccine was positive for CIAV in all these three methods, indicating that the detection results were true and reliable. It should be noted that RAA completed the whole detection process in only 15 min, while qPCR and dot blot took 1.5 and 14 h respectively. Now, there are also several handheld devices for RAA. Therefore, the RAA method is more suitable for monitoring vaccine contamination in farms because of its flexibility and convenience.
Furthermore, the whole genome sequence of CIAV was sequenced and named JS2020-PFV. It was found that JS2020-PFV had extremely high identities (99%) and the closest evolution relationship with the AB1K strain (MT259319) that was isolated from a chicken farm in Turkey. Besides, JS2020-PFV shared a close relationship with American isolate (DI072479) and Netherland isolate (CAE26P4), while it was relatively unrelated to Chinese isolates, including SDLY18 (FJ172347), GD-K-12 (KF224935) and SD15 (KX811526). These results are consistent with the fact that the vaccine was imported from a foreign company, suggesting that the transnational trade of vaccines may be an important way for some strains to spread across countries and regions.
The amino acid positions of VP1 protein are closely related to CIAV pathogenicity and cell proliferation (5). Previous studies have shown that amino acid positions 139 and 144 on VP1 were associated with the efficiency of virus proliferation and transmission in MSB1 cells (22). The amino acid positions 139 and 144 on the VP1 protein of JS2020-PFV are lysine (K) and glutamic acid (E), which are consistent with the strain with high replication ability (22). Besides, glutamine (Q) at position 394 was associated with higher pathogenicity, while histidine (H) at position 394 was related to lower pathogenicity (22). In this study, the amino acid at position 394 of VP1 protein of JS2020-PFV is glutamine (Q), suggesting that this strain may also be highly pathogenic. There are more than ten motifs related to transcriptional regulation in the non-coding region of CAV, which are closely related to viral replication and transcriptional regulation. After comparing and analyzing the non-coding regions of the selected strains in this paper, it was found that these motifs were very conservative. We speculate that these related motifs may be necessary for the replication of the virus itself.
Overall, CIAV contamination was detected from a fowlpox vaccine, and genome analysis suggested that it may be a highly pathogenic strain, which reminded us to pay close attention to the possible contamination of exogenous virus in live poultry vaccine. To prevent the recurrence of this phenomenon, SPF chicken farms, vaccine factories and farmers all should take reasonable countermeasures. First of all, SPF chicken farms should strengthen the daily monitoring of viruses, regularly detect the viruses with vertical transmission ability that have been reported in contaminated vaccines, and strictly eradicate their breeding chicken flocks. After purchasing chicken embryos, vaccine factories should also conduct viral detections of chicken embryos to eliminate the possibility of exogenous virus infection. In the last link, farmers should conduct a spot check on each batch of vaccines before using, to eliminate the possibility of exogenous virus contamination. The convenient and fast RAA method applies to the above three links, which is very conducive for on-site detection.
Data Availability Statement
The datasets presented in this study can be found in online repositories. The names of the repository/repositories and accession number(s) can be found below: https://www.ncbi.nlm.nih.gov/, MW234428.
Author Contributions
LC and QS conceived and performed the experiments, analyzed the data, and drafted the manuscript. PZ supervised the project and edited the manuscript. YL, JW, YZ, SC, and YW performed part of the experiments. All authors contributed to the article and approved the submitted version.
Funding
This work was supported by the National Key Research and Development Program of China (2018YFD0500106).
Conflict of Interest
The authors declare that the research was conducted in the absence of any commercial or financial relationships that could be construed as a potential conflict of interest.
Publisher's Note
All claims expressed in this article are solely those of the authors and do not necessarily represent those of their affiliated organizations, or those of the publisher, the editors and the reviewers. Any product that may be evaluated in this article, or claim that may be made by its manufacturer, is not guaranteed or endorsed by the publisher.
References
1. Rosenberger JK, Cloud SS. Chicken anemia virus. Poult Sci. (1998) 77:1190–2. doi: 10.1093/ps/77.8.1190
2. Yuasa N, Taniguchi T, Yoshida I. Isolation and some characteristics of an agent inducing anemia in chicks. Avian Dis. (1979) 366–85. doi: 10.2307/1589567
3. Fatoba AJ, Adeleke A. Chicken anemia virus: A deadly pathogen of poultry. Acta Virol. (2019) 63:19–25. doi: 10.4149/av_2019_110
4. Zhang X, Liu Y, Wu B, Sun B, Chen F, Ji J, et al. Phylogenetic and molecular characterization of chicken anemia virus in southern China from 2011 to 2012. Sci Rep. (2013) 3:3519. doi: 10.1038/srep03519
5. Fang L, Li Y, Wang Y, Fu J, Cui S, Li X, et al. Genetic Analysis of Two Chicken Infectious Anemia Virus Variants-Related Gyrovirus in Stray Mice and Dogs: The First Report in China, 2015. Biomed Res Int. (2017) 2017:6707868. doi: 10.1155/2017/6707868
6. Li Y, Fang L, Cui S, Fu J, Li X, Zhang H, et al. Genomic characterization of recent chicken anemia virus isolates in China. Front Microbiol. (2017) 8:401. doi: 10.3389/fmicb.2017.00401
7. Su Q, Meng F, Li Y, Zhang Y, Zhang Z, Cui Z, et al. Chicken infectious anemia virus helps fowl adenovirus break the protection of maternal antibody and cause inclusion body hepatitis-hydropericardium syndrome in layers after using co-contaminated Newcastle disease virus-attenuated vaccine. Poult Sci. (2019) 98:621–8. doi: 10.3382/ps/pey153
8. Tan C, Wang Z, Lei X, Lu J, Yan Z, Qin J, et al. Epidemiology, molecular characterization, and recombination analysis of chicken anemia virus in Guangdong province, China. Arch Virol. (2020) 165:1409–17. doi: 10.1007/s00705-020-04604-8
9. Adair BM. Immunopathogenesis of chicken anemia virus infection. Dev Comp Immunol. (2000) 24:247–55. doi: 10.1016/S0145-305X(99)00076-2
10. Marin SY, Barrios PR, Rios RL, Resende M, Resende JS, Santos BM, et al. Molecular characterization of contaminating infectious anemia virus of chickens in live commercial vaccines produced in the 1990s. Avian Dis. (2013) 57:15–21. doi: 10.1637/10056-011212-Reg.1
11. Varela APM, Dos Santos HF, Cibulski SP, Scheffer CM, Schmidt C, Lima FES, et al. Chicken anemia virus and avian gyrovirus 2 as contaminants in poultry vaccines. Biologicals. (2014) 42:346–50. doi: 10.1016/j.biologicals.2014.08.002
12. Li Y, Hu Y, Cui S, Fu J, Wang Y, Cui Z, et al. Molecular characterization of chicken infectious anemia virus from contaminated live-virus vaccines. Poult Sci. (2017) 96:1045–51. doi: 10.3382/ps/pew406
13. Su Q, Li Y, Meng F, Cui Z, Chang S, Zhao P. Newcastle disease virus-attenuated vaccine co-contaminated with fowl adenovirus and chicken infectious anemia virus results in inclusion body hepatitis-hydropericardium syndrome in poultry. Vet Microbiol. (2018) 218:52–9. doi: 10.1016/j.vetmic.2018.03.019
14. Su Q, Li Y, Zhang Y, Zhang Z, Meng F, Cui Z, et al. Newcastle disease virus-attenuated vaccine LaSota played a key role in the pathogenicity of contaminated exogenous virus. Vet Res. (2018) 49:80. doi: 10.1186/s13567-018-0577-z
15. Su Q, Wang T, Meng F, Cui Z, Chang S, Zhao P. Synergetic pathogenicity of Newcastle disease vaccines LaSota strain and contaminated chicken infectious anemia virus. Poult Sci. (2019) 98:1985–92. doi: 10.3382/ps/pey555
16. Zhang Y, Cui N, Han N, Wu J, Cui Z, Su S. Depression of Vaccinal Immunity to Marek's Disease by Infection with Chicken Infectious Anemia Virus. Front Microbiol. (2017) 8:1863. doi: 10.3389/fmicb.2017.01863
17. Su Q, Zhang Y, Li Y, Cui Z, Chang S, Zhao P. Epidemiological investigation of the novel genotype avian hepatitis E virus and co-infected immunosuppressive viruses in farms with hepatic rupture haemorrhage syndrome, recently emerged in China. Transbound Emerg Dis. (2019) 66:776–84. doi: 10.1111/tbed.13082
18. Meng F, Dong G, Zhang Y, Tian S, Cui Z, Chang S, et al. Co-infection of fowl adenovirus with different immunosuppressive viruses in a chicken flock. Poult Sci. (2018) 97:1699–705. doi: 10.3382/ps/pex414
19. Mao Y, Su Q, Li J, Jiang T, Wang Y. Avian leukosis virus contamination in live vaccines: a retrospective investigation in China. Vet Microbiol. (2020) 246:108712. doi: 10.1016/j.vetmic.2020.108712
20. Su Q, Hou L, Gao Y, Liu X, Cui Z, Chang S, et al. Research Note: Molecular relationship of the fowl adenovirus serotype 4 isolated from the contaminated live vaccine and wild strains isolated in China, 2013-2018. Poult Sci. (2020) 99:6643–6. doi: 10.1016/j.psj.2020.08.063
21. Li Y, Wang Y, Fang L, Fu J, Cui S, Zhao Y, et al. Genomic Analysis of the Chicken Infectious Anemia Virus in a Specific Pathogen-Free Chicken Population in China. Biomed Res Int. (2016) 2016:4275718. doi: 10.1155/2016/4275718
Keywords: chicken infectious anemia virus, recombinase-aided amplification assay, contamination, genome analysis, live vaccines
Citation: Chen L, Su Q, Li Y, Wang J, Zhang Y, Chang S, Wang Y and Zhao P (2022) Genomic Characteristics of a Chicken Infectious Anemia Virus in Contaminated Attenuated Vaccine. Front. Vet. Sci. 9:925935. doi: 10.3389/fvets.2022.925935
Received: 22 April 2022; Accepted: 25 May 2022;
Published: 16 June 2022.
Edited by:
Yulong Gao, Chinese Academy of Agricultural Sciences, ChinaReviewed by:
Hualei Liu, China Animal Health and Epidemiology Center, ChinaBasavaraj S. Mathapati, Indian Council of Medical Research (ICMR), India
Copyright © 2022 Chen, Su, Li, Wang, Zhang, Chang, Wang and Zhao. This is an open-access article distributed under the terms of the Creative Commons Attribution License (CC BY). The use, distribution or reproduction in other forums is permitted, provided the original author(s) and the copyright owner(s) are credited and that the original publication in this journal is cited, in accordance with accepted academic practice. No use, distribution or reproduction is permitted which does not comply with these terms.
*Correspondence: Peng Zhao, emhhb3BlbmdAc2RhdS5lZHUuY24=
†These authors have contributed equally to this work