- 1Department of Clinical Sciences, College of Veterinary Medicine and Comparative Medicine Institute, North Carolina State University, Raleigh, NC, United States
- 2Department of Environmental Services, Wake County Animal Center, Raleigh, NC, United States
- 3Orange County Animal Services, Chapel Hill, NC, United States
- 4Department of Plant and Microbial Biology, North Carolina State University, Raleigh, NC, United States
- 5Veterinary Hospital Pharmacy, College of Veterinary Medicine and Comparative Medicine Institute, North Carolina State University, Raleigh, NC, United States
- 6University of Wisconsin Veterinary Care, Madison, WI, United States
- 7Department of Clinical Sciences, College of Veterinary Medicine, Mississippi State University, Starkville, MS, United States
- 8Division of Gastroenterology and Hepatology, and UNC Microbiome Core, Department of Medicine, Center for Gastrointestinal Biology and Disease, School of Medicine, University of North Carolina, Chapel Hill, NC, United States
- 9IDEXX Laboratories, Inc., West Sacramento, CA, United States
- 10Vet Med Labor GmbH Division, IDEXX Laboratories, Inc., Kornwestheim, Germany
Introduction: Diarrhea is the second most common cause of mortality in shelter kittens. Studies examining prevention strategies in this population are lacking. Probiotics are of particular interest but studies in cats are largely limited to healthy adults or those with induced disease. Only one study in domestic cats describes the use of host-derived bacteria as a probiotic. We previously identified Enterococcus hirae as a dominant species colonizing the small intestinal mucosa in healthy shelter kittens. Oral administration of a probiotic formulation of kitten-origin E. hirae (strain 1002-2) mitigated the increase in intestinal permeability and fecal water loss resulting from experimental enteropathogenic E. coli infection in purpose-bred kittens. Based on these findings, we hypothesized that administration of kitten-origin E. hirae to weaned fostered shelter kittens could provide a measurable preventative health benefit.
Methods: We conducted a randomized, placebo-controlled, blinded clinical trial to determine the impact of a freeze-dried E. hirae probiotic on body weight gain, incidence of diarrhea, carriage of potential diarrheal pathogens, and composition of the intestinal microbiota in weaned fostered shelter kittens.
Results: One-hundred thirty kittens completed the study. Fifty-eight kittens received the probiotic and 72 received the placebo. There were no significant differences in age, weight upon initiation of the study, number of days in the study, average daily gain in body weight, or weight at completion of the study. Kittens treated with E. hirae were 3.4 times less likely to develop diarrhea compared to kittens treated with placebo (odds ratio = 0.294, 95% CI 0.109–0.792, p = 0.022). A significant impact of E. hirae was not observed on the presence or abundance of 30 different bacterial, viral, protozoal, fungal, algal, and parasitic agents in feces examined by qPCR. With exception to a decrease in Megamonas, administration of the E. hirae probiotic did not alter the predominant bacterial phyla present in feces based on 16S rRNA gene amplicon sequencing.
Discussion: Decreased incidence of diarrhea associated with preventative administration of E. hirae to foster kittens supports a rationale for use of E. hirae for disease prevention in this young population at high risk for intestinal disease though additional studies are warranted.
Introduction
It is estimated that 180 million kittens are born each year in the United States and hundreds of thousands of orphaned and abandoned kittens are fostered by U.S. animal shelters (1–3). Diarrheal disease is the second most common illness to afflict shelter kittens and many will die from diarrhea before they reach an age of 8 weeks (4, 5). The causes of diarrhea are likely multifactorial including an immature immune system, weaning stress, independent eating, change in food type, waning maternal antibodies, and increased exposure to diarrheal pathogens (1, 4, 6, 7). Efforts aimed at diagnosis and treatment of infectious causes of diarrhea in this population is challenging due to the large number and diversity of potential pathogens (viral, bacterial, parasitic and protozoal), frequent carriage of these pathogens by kittens without diarrhea, many agents for which there is no specific treatment, and limited resources. Consequently, orphan kittens that develop diarrhea are often treated empirically for intestinal bacterial, protozoal, or parasitic infections and are commonly provided supportive care in the form of antibiotics, probiotics, vitamin supplements, subcutaneous fluids, or assisted feeding (8).
Among the many knowledge gaps including causes and treatment outcomes of foster kitten diarrhea is a lack of studies examining prevention strategies for diarrhea in this population. In particular, probiotics (9) are of particular interest due to the multiple proposed beneficial effects such as promoting resistance to colonization by pathogens, stabilization of the intestinal microbiota, immunomodulatory effects on gut function, and metabolic impact on nutrient digestion. To date however, research examining the impact of probiotics in cats are mostly limited to investigations of healthy, adult-aged, purpose-bred cats, or cats with experimentally-induced disease (e.g., FIV and FHV-1) or administered antibiotics (10–20). Only 2 studies have examined the effect of probiotics on cats with diarrhea. In a study of older stray and feral cats housed short-term in a shelter setting, administration of a probiotic containing Enterococcus faecium SF68 did not protect against diarrhea but decreased the number of cats having diarrhea of ≥2 days duration (21). In a second study of adult cats with naturally-occurring chronic diarrhea, treatment with a synbiotic containing Bifidobacterium, Enterococcus, and Lactobacillus was reported to significantly improve fecal score (22).
In particular, the enterococci are components of the normal microbiota of the gastrointestinal tract and are ubiquitous in ecosystems ranging from soil, water, plants and food products (23). We have previously identified that a specific species of enterococcus, Enterococcus hirae, is the principal species inhabiting the mucosa-associated microbiota of the small intestine in healthy fostered shelter kittens (4) and is frequently observed to extensively colonize the surface of the intestinal epithelium (24). In kittens succumbing to severe illness, E. hirae are displaced by opportunistic and virulent members of enterococci such as Enterococcus faecalis and such kittens are more likely to be diagnosed with enteroadherent enteropathogenic Escherichia coli infection (EPEC) (4). Using a strain of E. hirae, cultured from the mucosa-associated microbiota of a healthy kitten and manufactured into a freeze-dried probiotic, we demonstrated that oral administration of E. hirae could mitigate the increase in intestinal permeability and fecal water loss resulting from experimental EPEC infection in purpose-bred kittens (25).
Based on these findings, we hypothesized that administration of feline-origin E. hirae to weaned fostered shelter kittens could provide a measurable preventative health benefit. To test this hypothesis we conducted a randomized, placebo-controlled, blinded clinical trial to determine the impact of a freeze-dried E. hirae probiotic on parameters of body weight gain, incidence of diarrhea requiring examination by a veterinarian, carriage of potential diarrheal pathogens, and composition of the intestinal microbiota in weaned fostered shelter kittens.
Materials and methods
Enterococcus hirae probiotic
Formulation
The administered freeze-dried probiotic was manufactured from a pure, well-characterized isolate of Enterococcus hirae. The strain (1002-2) was isolated by swab of the ileum mucosa performed during autopsy of a healthy kitten in which histological evidence of E. hirae attachment to the intestinal epithelium was concurrently documented (4). The isolate lacked all virulence characteristics, the results of which were previously reported (4). The selected strain was cultured for use as a probiotic in Biostat B-plus reactors continuously fed with MB medium (26) containing 20 g/L glucose. Chemostat maintained a dilution rate (D = 0.17 h−1), temperature (37°C), and pH (5.5). The viable biomass was collected from the reactor overflow, concentrated, and suspended in bacteriological peptone prior to lyophilization. Glucose consumption, accumulation of lactate and absence of alternative fermentation products were monitored with high-performance liquid chromatography (Shimadzu Corporation, Kyoto, Japan) performed under isocratic conditions at 65°C, a mobile phase of 5 mM sulfuric acid (H2SO4) at a 0.4 ml/min flow rate using an Alltech IOA-1000 organic acids column (300 mm x 7.8 mm, Alltech, IL, USA), and coupled to a refractive-index detector. Numbers of viable cells were confirmed by plating on M17 agar (27) and incubating at 37°C for 72 h. For each sample, three plates were prepared from each dilution and recorded as the average of three independent counts. Finalized freeze-dried peptone containing probiotic and vehicle (sterile ultrapure bacteriological peptone, Thermo Scientific) were stored at −80°C.
Compounding
Capsules containing either the probiotic or placebo (bacteriological peptone) were compounded by the NC State Veterinary Hospital Pharmacy into blue or yellow opaque capsules whose contents remained blinded to study investigators.
Pre-compounding E. hirae stock containing 2.24 × 107 colony forming units (CFU) per milligram was stored at −80°C and transferred to dry ice when in use. A desired dose of 1 × 108 CFU was achieved by compounding 4.5 mg of E. hirae stock with 110.5 mg of bacteriological peptone (ThermoFisher Scientific, Waltham, MA) to achieve an average pack weight of 115 mg ± 5 mg. Placebo consisted of 115 mg of bacteriological peptone only. Compounding was performed in a 4°C walk-in environmental chamber. Batch sizes of 300 capsules were compounded using a pre-chilled mortar and pestle, ProFiller 3600 capsule machine, and Letco #4 size gelatin capsules. Capsules were transferred to 30–count vials labeled with capsule color and date of manufacture and stored at −20°C.
Viability
Sustained numbers of E. hirae in the compounded probiotic was confirmed by culture of the contents of a subset of capsules immediately after formulation, after 5 months of storage at −20°C on location at the shelter, and following return of leftover (unused) capsules to the shelter after storage in the home freezer of a foster care provider. Briefly, probiotic and placebo capsule contents were dissolved separately in 1 ml of sterile phosphate-buffered saline (PBS) to generate a working stock. Serial dilutions were performed to generate concentrations ranging from 1 × 107 to 1 × 102 CFUs per 1 ml and the equivalent for the placebo. For each of the 104, 103, and 102 dilutions and sterile PBS, 100 μl was spread onto three separate plates of Difco m-Enterococcus Agar (Becton Dickinson, Franklin Lakes, NJ). Plates were incubated at 37°C for 24 h. Following incubation, colonies were counted on the plates of the dilution(s) that resulted in 30 and 300 CFUs. The average colony count was calculated, allowing for the current CFU concentration of the working stock to be extrapolated. Viability of the probiotic capsules were then determined by dividing the extrapolated concentration by the initial presumed concentration of 1 × 108 CFUs/ml.
Clinical trial design
A prospective, randomized, placebo-controlled, blinded clinical study was conducted at a large municipal animal shelter [Wake County Animal Center (WCAC)] over the time interval from May 1 to October 1 in the year 2018 and 2019.
Eligibility criteria included any weaned and apparently healthy kitten aged <12 weeks and admitted to the shelter with a body weight <0.9 kg (2 lbs) and therefore requiring temporary care prior to fitness for surgical sterilization and adoption. Kittens destined for fostering by a WCAC-trained care provider were eligible for participation in the study. Kittens that were alternatively transferred to the care of rescue partner organizations were not eligible to participate.
Preventative care
All kittens were housed for an average of 2–3 days in a dedicated room within the shelter prior to transfer to the home of a volunteer foster care provider. During this time, each kitten was assigned an individual identification/medical record number for tracking using a web-based shelter software program (ShelterBuddy, Wacol Brisbane QLD AUSTRALIA). Kittens were administered a single oral dose of ponazuril paste (Marquis®, Boehringer Ingelheim; 30mg/kg) and pyrantel pamoate (Columbia Laboratories; 11 mg/kg) and topical administration of selamectin (Revolution®, Zoetis) for treatment of endo- and ectoparasites. Kittens that were ≥4 weeks of age and ≥0.45 kg were vaccinated by subcutaneous injection with feline viral rhinotracheitis, calicivirus, and panleukopenia antigen (NobivacR:Feline 1-HCP, Intervet INC Merck Animal Health division US Omaha NE 68103) and revaccinated every 2–3 weeks until 16–20 weeks (28).
Randomization
In advance of the study, each day of each month from May 1 to October 1 was randomly designated as blue or yellow using an online program (Random.org). The designation of blue or yellow indicated the treatment group to which any kittens entering foster care on that day would be assigned and was unconcealed. Participation in the study was voluntary and each foster volunteer signed an informed consent. To prevent cross-contamination of treatments, participating foster-care providers were only allowed to simultaneously foster kittens designated to the same treatment group at any given time.
Blinding
The placebo and probiotic were formulated into opaque size number 4 gelatin capsules colored blue and yellow to correspond with the treatment group allocation color. Study investigators, shelter staff, and foster care providers were blinded to identity of the capsule contents. Only the pharmacists (SW, EW, and GD) responsible for compounding the capsules and technician (SHS) responsible for documenting bacterial viability of capsule contents were aware of the capsule contents. All other investigators remained blinded to group allocation until all data were analyzed and results of the study were written in manuscript form.
Treatment and monitoring
Participating foster care providers were provided with capsules containing probiotic or placebo based on prior random allocation, two zip-lock bags for collection of a pre- and end-of-study fecal sample with labels for providing the collection date and kitten shelter identification number, observation worksheet for recording pre- and end-of-study body weight, and instructions for treatment and sample collections. Foster care providers were instructed to store the dispensed capsules in their home freezer throughout the study.
During the study, the type of diet provided to kittens was chosen at the discretion of the foster care provider. Supplements such as vitamins were permitted but not administration of non-study probiotics. Prior to beginning administration of the probiotic or placebo, foster care providers were instructed to obtain an initial body weight for which a scale (AccuWeight AW-KS001BB) was provided, and to collect a voided fecal sample with instructions to store the sample in their home freezer. After recording of an initial body weight and collection of a fecal sample, foster care providers were instructed to administer a single capsule of probiotic/placebo daily by opening the contents of the capsule onto a small portion of canned kitten food and monitoring consumption by the kitten until complete. Each foster-care provider was provided with an observation sheet for documenting daily probiotic administration and encouraged, but not required, to take periodic body weight measurements over the course of the study. Upon reaching a weight of ≥2 pounds, foster-care providers were instructed to obtain a final body weight and fecal sample while the kitten was still receiving the probiotic and store as per the initial sample. Upon completion of the study, fecal samples were transferred by the foster care provider back to Wake County Animal Center and stored at −20°C prior to transport to the laboratory on dry ice where they were subsequently stored at −80°C until analysis.
Incidence of diarrhea
Kittens were diagnosed as having significant diarrhea if they met criteria for unscheduled examination by the WCAC veterinary team. These criteria included diarrhea lasting more than 24 h and accompanied by straining to defecate, discomfort during defecation, or decreased appetite. Foster care providers were not trained to provide fecal scoring and fecal scores were not requested or recorded as part of the study.
Criteria for inclusion of gathered data
Minimum criteria for inclusion in data analysis were (1) uninterrupted daily administration of the placebo/probiotic and (2) recording of a pre- and end-of-study body weight. An end-of-study body weight was not required for kittens that died or were euthanized while on the study.
Molecular assays
Fecal PCR testing
Paired fecal samples, collected from kittens prior to beginning and immediately prior to completion of the study, were batched and shipped on dry ice to a collaborating laboratory (IDEXX Diagnostics Laboratory, Sacramento CA) where they were stored at −80°C prior to assay.
Fecal samples were suspended in 3 ml guanidinium thiocyanate based lysis solution (29), vortexed to facilitate organism detachment and rapid protein denaturation and incubated at room temperature for 15 min. The lysate was then used to extract total nucleic acid on a MagMax 96 Flex (Life Technologies, Valencia, CA) with magnetic beads (Roche, Indianapolis, IN) using manufacturer's guidelines. Total nucleic acid was eluted in 200 μl of PCR-grade nuclease-free water and 5 μl amplified in subsequent single plex real-time PCR reactions.
Real-time PCR was performed with proprietary forward and reverse primers and hydrolysis probes. Target genes for enteropathogen detection were as follows: Feline enteric coronavirus 7b gene (DQ010921.1), Feline panleukopenia virus VP2 gene (EU252145), Rotavirus VP7 gene (EU708950), Salmonella enterica invasion A gene (EU348366), Campylobacter (coli and jejuni) IpxA gene [AY531496 (C. coli) and AL111168 (C. jejuni)], Clostridium perfringens alpha toxin gene (L43545), Clostridium perfringens enterotoxin gene (X81849), Shigella spp. RFC gene (AE005674), E. coli virulence factor genes (F4 (K88), F5 (K99), F6 (987P) and F18ab fimbrial adhesin genes; STX2e gene; Intimin adhesion gene; LT1 gene, ST1a and ST1b genes) (EU570252; M35282; M35257; GQ325633; GU945540; ECU38618; S60731.1; M34916), Yersinia spp. invasion locus protein (ail) gene (AJ605740), Cryptosporidium spp. ssrRNA gene (A093489), Giardia small-subunit rRNA gene (DQ836339), Toxoplasma gondii internal transcribed spacer-1 gene (L49390, Tritrichomonas foetus 5.8S rRNA gene (AF339736), Blastocystis spp. SSU rRNA gene (AB023499; KP890050; AB070992; AB071000; AB070999; AB070990; AB070991; AB107971; AF408426), Toxocaris leoni ITS-2 gene (Y09490), Toxocara (malayasiensis and cati) ITS-1 gene, Internal transcribed spacer-2 (ITS-2) gene (AB110033; AM231609), Ancylostoma spp. ITS-1 gene (DQ438074; DQ438063; DQ780009), Trichuris spp. ITS-2 gene (AM234616), Uncinaria spp. ITS-1 gene (AF217890), Parascaris spp. ITS-1 gene (AJ007459), Echinococcus spp. rRNA between the Cox 1 and Cox 2 genes (NC_000928), Pythium spp. ITS-1 gene (EF016907.1), and Prototheca spp. ITS-1 gene (AJ245645).
Real-time PCR was run with six quality controls as previously described (30) including (1) PCR positive controls [synthetic DNA (Integrated DNA Technologies IDT, Coralville, IA), run quantitatively], (2) PCR negative controls (RNase-free PCR-grade water, Fisher Scientific, Waltham MA), (3) negative extraction controls (lysis solution only), (4) DNA pre-analytical quality control targeting mammalian ssr rRNA (18S rRNA) gene complex, (5) environmental contamination monitoring control (swab-based laboratory monitoring), and (6) spike-in internal positive control. These controls assessed the functionality of the PCR test protocols for the (1), functional assessment of the real-time PCR test performance (2) absence of contamination (both PCR product carry-over and sample cross-contamination), (3) absence of detectable cross-contamination during the extraction process, (4) quality and integrity of the DNA as a measure of sample validity (by quantitatively assessing 18S gene load), reverse transcription protocol (5) absence of aerosol-based contamination within the PCR laboratory space, and (6) absence of PCR inhibitory substances as a carryover from the sample matrix (internal sample control with spike-in DNA).
Analysis was performed on a Roche LightCycler 480 (Roche Applied Science, Indianapolis (IN) on which amplification data were analyzed using the 2nd derivative maximum method to generate crossing points (Cp values). Each Cp value was quantitatively validated by prior calibration to a standard curve of DNA for each target assay.
16S rRNA gene amplicon sequencing
Fecal samples that were collected immediately prior to completion of the study were selected for microbiota analysis. To isolate the impact of the probiotic intervention and minimize bias in the data, sibling kittens had only a single/representative fecal sample chosen for analysis.
DNA isolation from fecal samples was performed as previously described (31). Briefly, 0.3 ml of Qiagen ATL buffer (Valencia, CA) supplemented with 60 mg/ml lysozyme (Thermo Fisher Scientific, Grand Island, NY) was added to the samples. The suspensions were transferred to 2 ml tubes containing 106/500 μm glass beads (Sigma, St. Louis, MO) and incubated for 1 h at 37°C with occasional agitation. The suspensions were agitated for 40 min on a Digital Vortex Mixer. Subsequently, the suspensions were supplemented with 20 μL of Qiagen proteinase K and 0.3 ml of Qiagen AL buffer and incubated at 55°C overnight. After brief centrifugation, supernatants were aspirated and transferred to a new tube containing 0.3 ml of ethanol. DNA was purified using a standard on-column purification method with Qiagen buffers AW1 and AW2 as washing agents and eluted in 27.5 μl of DNase free water and quantified using QuantIT® PicoGreen®.
Extracted DNA was quantified via PicoGreen analysis and used for bacterial 16S rRNA gene amplicon sequencing. Total DNA (12.5 ng) was amplified using universal primers targeting the V4 region of the bacterial 16S rRNA gene (32). The sequences of the primers were: 515F−5′ TCGTCGGCAGCGTCAGATGTGTATAAGAGACAGGTGCCAGCMGCCGCGGTAA 3′ and 806R−5′GTCTCGTGGGCTCGGAGATGTGTATAAGAGACAGG GACTACHVGGGTWTCTAAT 3′. Overhang adapters were appended to the 5′ end of each primer sequence for compatibility with the Illumina sequencing platform. Master mixes contained 12.5 ng of total DNA, 0.2 μM of each primer and 2× KAPA HiFi HotStart ReadyMix (KAPA Biosystems, Wilmington, MA). The thermal profile for the amplification of each sample had an initial denaturing step at 95°C for 3 min, followed by 25 cycles of denaturing of 95°C for 30 s, annealing at 55°C for 30 s for 16S rRNA and a 30 s extension at 72°C, a 5 min extension at 72°C and a final hold at 4°C. Each 16S amplicon was purified using the AMPure XP reagent (Beckman Coulter, Indianapolis, IN). In the next step each sample was amplified using a limited cycle PCR program, adding Illumina sequencing adapters and dual index barcodes [index 1(i7) and index 2(i5)] (Illumina, San Diego, CA) to the amplicon target. The thermal profile for the amplification of each sample had an initial denaturing step at 95°C for 3 min, followed by a denaturing cycle of 95°C for 30 s, annealing at 55°C for 30 s and a 30 second extension at 72°C (8 cycles), a 5 min extension at 72°C and a final hold at 4°C. The final libraries were again purified using the AMPure XP reagent (Beckman Coulter), quantified with Quant-iT™ PicoGreen® dsDNA Reagent (Molecular Probes, Thermo Fisher Scientific, Waltham, MA), and normalized prior to equimolar pooling. The DNA library pool was then denatured with NaOH, diluted with hybridization buffer and heat denatured before loading on the MiSeq reagent cartridge MiSeq instrument (Illumina). Automated cluster generation and paired–end sequencing with dual reads were performed according to the manufacturer's instructions.
Data analysis
Kitten populations were described in terms of age, sex, weight on intake to the shelter, number of kittens in litter, time spent on study, average daily gain in body weight, weight on exit from study, episodes of diarrhea requiring examination by shelter veterinary staff, and outcome (alive–vs.–died or euthanized). Categorical data (e.g., proportions) were tested for significant differences between treatment groups using a Chi-square with calculation of odds ratios and 95% confidence intervals. Continuous data were tested for normal distribution and equal variance followed by parametric (one-way ANOVA) or non-parametric (Kruskal-Wallis one-way ANOVA on ranks) analysis as appropriate.
The proportion of kittens testing PCR positive for detection of selected infectious agents in feces were compared for significant differences between pre- and post-study samples within each treatment group using a Chi Square test or Fisher Exact test. Infectious agents for which ≤5 kittens tested positive at both time points were not subjected to statistical analysis. The median burden of selected infectious agent RNA/DNA detected within feces of test-positive kittens, as represented by the PCR Cp values, was compared for significant differences between pre- and post-study samples within each treatment group using a Kruskal-Wallis one-way ANOVA on ranks. Testing was performed using Systat software (SigmaPlot 12).
Sequencing output from the Illumina MiSeq platform were converted to fastq format and demultiplexed using Illumina Bcl2Fastq 2.18.0.12. The resulting paired-end reads were processed using QIIME 2 (33) 2018.11. Index and linker primer sequences were trimmed using the QIIME 2 invocation of cutadapt. The resulting paired-end reads were processed with DADA2 through QIIME 2 including merging paired ends, quality filtering, error correction, and chimera detection. Amplicon sequencing units from DADA2 were assigned taxonomic identifiers with respect to Green Genes release 13_08.
Alpha diversity estimates were calculated within QIIME 2 using Evenness (Shannon) index and observed species number metrics at a rarefaction depth of 5,000 reads. Pairwise significance was tested using a Kruskal-Wallis ANOVA with Benjamini-Hochberg corrected q-values calculated as implemented in QIIME 2. Beta diversity estimates were calculated within QIIME 2 using weighted and unweighted Unifrac distances as well as Bray-Curtis dissimilarity between samples at a subsampling depth of 5,000 reads. Results were summarized, visualized through principal coordinate analysis in Emperor, and significance was estimated by PERMANOVA with Benjamini-Hochberg corrected q-values calculated as implemented in QIIME 2. Relative abundance data were normalized by sample library size and taxa were removed if they were present in <10% of all samples or if lower than 0.01% average abundance (34). Differences in abundance were tested for significance using a Kruskal-Wallis One-Way ANOVA on Ranks. When significant, pairwise comparisons between groups were performed using a Dunn's test. Generated p-values were corrected for multiple testing using the Benjamini-Hochberg procedure (35) at a false discovery rate of 0.25. For selected taxa, odds ratio and 95% confidence intervals for association with treatment group were calculated using a Chi-square Test with Yates Continuity Correction. Statistical examination of abundance data was conducted using Systat Software (SigmaPlot 12.0, San Jose, CA). Results were represented graphically using GraphPad Software (Prism version 7.03, San Diego, CA).
Results
Population description
Over the time interval from May 1 to October 1 in the years 2018 and 2019, a total of 1,471 kittens were assessed for eligibility to participate in the study. Among these kittens, 220 (15%) were randomly allocated to treatment with probiotic or placebo. One-hundred thirty kittens completed the study. Fifty-eight kittens from 40 unrelated litters received the probiotic (blue group) and 72 kittens from 37 unrelated litters received the placebo (yellow group) (Figure 1). A total of 28 different foster care providers participated over the 2-year course of the study, each fostering a median of 3 kittens (range, 1–18 kittens).
Clinical outcome
Viability of the probiotic (target dose, 1 × 108 CFU) was not diminished when quantified after storage at −20°C at the shelter (2.2–8.9 × 108 CFU), nor followed by storage in the home freezer of a foster care provider (0.94 × 108 CFU). All kittens readily consumed the probiotic or placebo when offered. When comparing the probiotic and placebo groups, there were no significant differences in starting age, weight upon initiation of the study, number of days in the study, average daily gain in body weight, or weight at completion of the study (Table 1 and Figure 2).
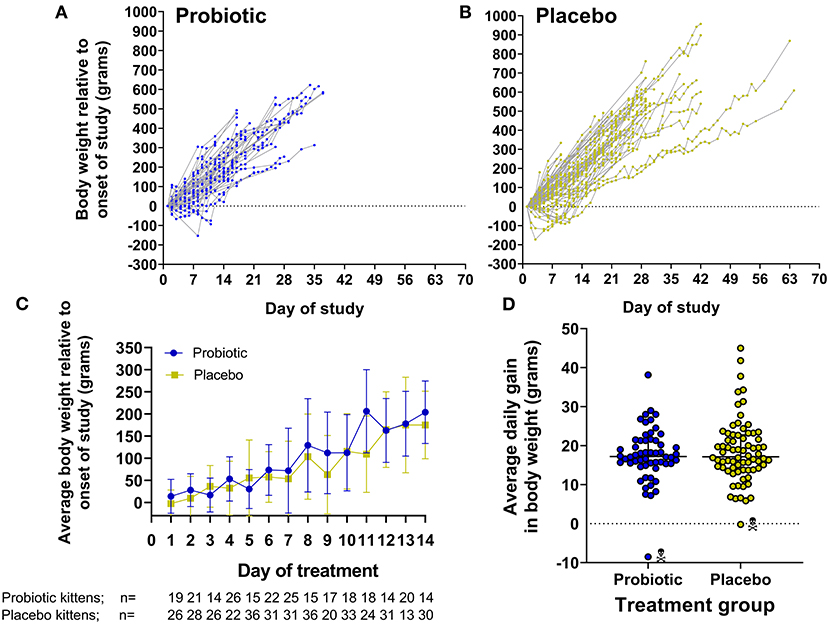
Figure 2. Gain in body weight by kittens receiving probiotic or placebo. (A,B) Show daily gain in body weight normalized by initial body weight for individual kittens in each group over the course of the study. (C) Represents the average ± SD body weight of kittens comparing both groups on each day during the first 14 days of the study. Note that each time point represents only those kittens having their body weight measured on the given day which is indicated below the x-axis legend. (D) Demonstrates the average daily gain in body weight over the course of the study [(end weight – start weight)/number of days between weight measurements] in individual kittens comparing both groups. Kittens that died/where euthanized are designated by skull symbol.
Kittens treated with the probiotic were 3.4 times less likely to develop diarrhea compared to kittens treated with the placebo (odds ratio = 0.294, 95% CI 0.109–0.792, p = 0.022). No kittens were euthanized due to diarrheal illness and overall survival was not significantly different between the 2 groups. Reasons for euthanasia of kittens in the study included failure to thrive (2 probiotic kittens), panleukopenia (1 probiotic kitten), and major medical (1 placebo kitten) (Table 1).
Prevalence and abundance of selected infectious agents
Quantitative PCR was performed on paired fecal samples, collected prior to beginning and immediately prior to completion of the study, from 37 kittens receiving the probiotic and 55 kittens receiving placebo. Reasons for exclusion of kittens were lack of either a pre or post-fecal sample (13 probiotic kittens, 15 placebo kittens), insufficient feces for both microbiome and PCR analysis (5 probiotic kittens, 1 placebo kitten), or euthanasia prior to final fecal sample collection (3 probiotic kittens, 1 placebo kitten).
Fecal samples were tested for the presence of 30 different bacterial, viral, protozoal, fungal, algal, and parasitic agents, among which 17 were detected in ≥1 kitten (Tables 2 and 3). Prior to initiation of the study, 92% (34/37) of kittens receiving probiotic and 87% (48/55) of kittens receiving placebo had a median of 2 infectious agents (range, 0–4) identified. Upon completion of the study, 86% (32/37) of kittens receiving probiotic and 78% (43/55) of kittens receiving placebo had a median of 2 (range, 0–4) and 1 (range, 0–4) respectively, infectious agents identified. The number of identified agents did not differ significantly from pre-study values in either group. Examination of individual kittens demonstrated that 22% (8/37) of kittens receiving the probiotic had a median gain or loss in number of infectious agents of 0 (range, −3 to +3) and 20% (11/55) of kittens receiving the placebo had a median gain or loss in number of infectious agents of 0 (range, −4 to +3) which did not differ significantly between the groups.
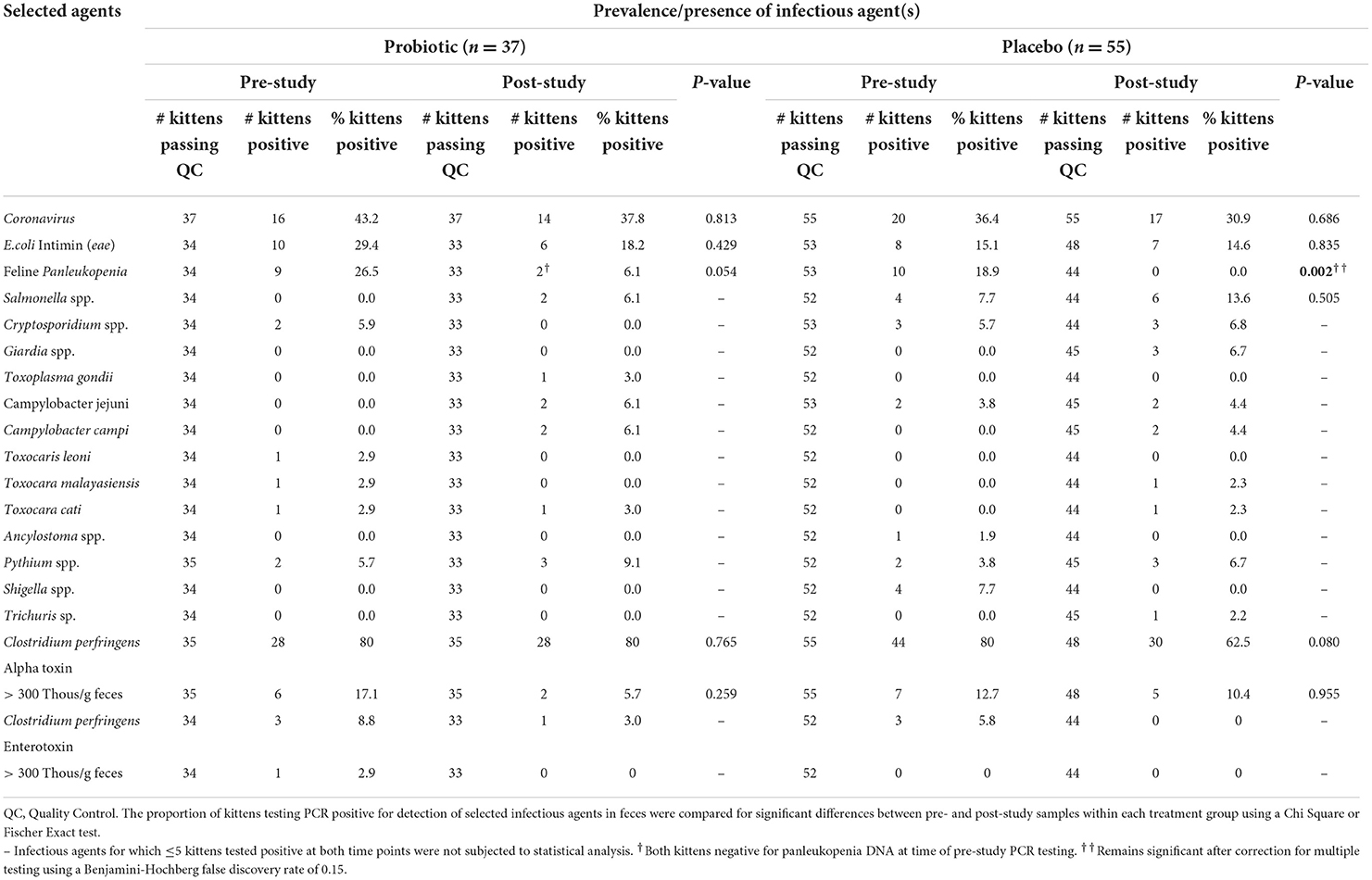
Table 2. Prevalence of infectious agents identified by RT-PCR or PCR in RNA/DNA extracted from voided feces collected from fostered kittens prior to (pre-study) and upon completion (post-study) of ongoing daily treatment with a probiotic or placebo.
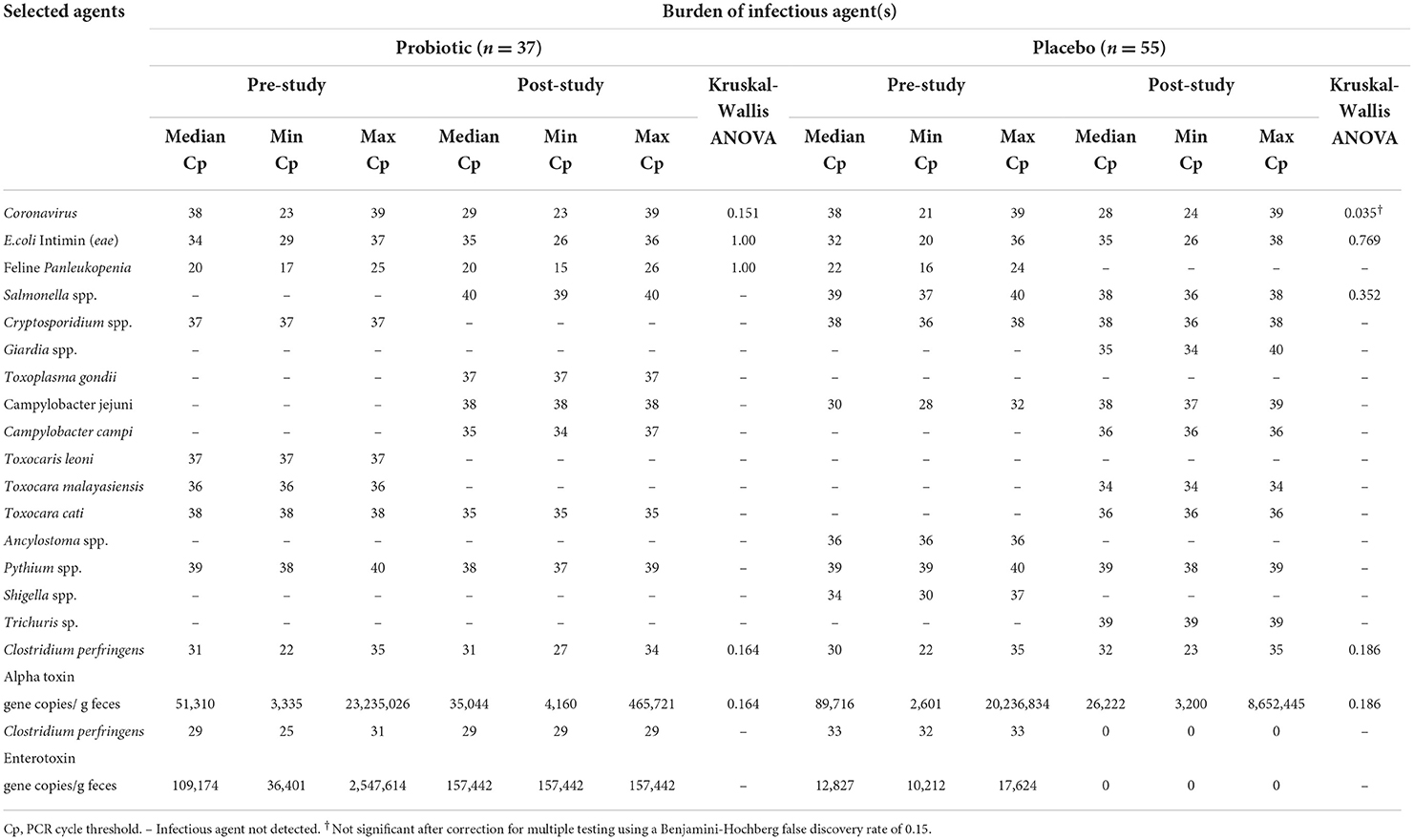
Table 3. Abundance of infectious agents identified by RT-PCR or PCR in RNA/DNA extracted from voided feces collected from fostered kittens prior to (pre-study) and upon completion (post-study) of ongoing daily treatment with a probiotic or placebo.
The most common infectious agents having RNA or DNA demonstrated in feces were feline enteric coronavirus, attaching and effacing E. coli (presence of eae), panleukopenia, and Clostridium perfringens (alpha-toxin). There were no significant differences in prevalence (Table 2) or abundance (Table 3) of infectious agents between the 2 groups of kittens for fecal samples collected prior to initiation of the study. Upon completion of the study there was a significant decrease in prevalence of detection of panleukopenia DNA in kittens treated with the placebo (Table 2). Decrease in abundance of coronavirus RNA in kittens treated with placebo was not significant after correction for multiple comparisons (Table 3).
Infectious agent PCR testing was performed on feces collected from 16/26 kittens that developed diarrhea over the course of the study. Identified infectious agents having a potential to contribute to diarrhea included panleukopenia (4 kittens from 2 litters), feline enteric coronavirus (5 kittens from 3 litters), Campylobacter spp. (3 kittens from 2 litters), attaching and effacing E. coli (2 kittens), and alpha-toxin positive Clostridium perfringens (alpha toxin gene copies >30,000/gram feces) (2 kittens).
Microbial community composition
Analysis of the fecal microbiota by 16S rRNA gene amplicon sequencing was performed for 32/58 (55%) unrelated kittens that received the probiotic (32/40 (80%) of litters represented) and 35/72 (49%) unrelated kittens that received the placebo (35/37 (94%) of litters represented). Included in the analysis were 6 kittens from 6 individual litters that received probiotic and were examined for diarrheal illness during the course of the study. Eight litters in the probiotic group did not have a kitten represented in the analysis due to lack of an end-of-study fecal sample (5 litters) or euthanasia (3 litters). Also included in the analysis were 7 kittens from 7 individual litters that received placebo and were examined for diarrheal illness during the course of the study. Two litters in the placebo group did not have a kitten represented in the analysis due to lack of an end-of-study fecal sample (one of which included a kitten that developed diarrhea during the course of the study).
The sequence analysis of all 67 kittens yielded 5,675,488 quality sequences (mean ± SD = 84,709 ± 34,177). The major phyla represented in the fecal microbiota of kittens were Firmicutes, Actinobacteria, Bacteroidetes, Proteobacteria, and Fusobacteria (Figure 3). There were no significant differences in alpha diversity measures of evenness (Shannon), diversity (Faith), or observed number of taxa between kittens receiving the probiotic compared to placebo. Both weighted and unweighted measures of beta diversity did not identify significant differences in phylogenetic composition of the microbiota between kittens receiving the probiotic vs. placebo (Figure 4).
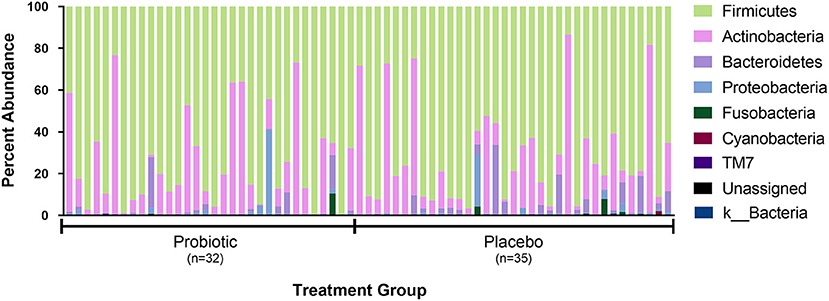
Figure 3. Percent abundance of the major phyla observed in feces from 67 individual kittens. Each sample is designated as having been obtained from a kitten receiving either the probiotic or placebo. Not shown in legend are phyla observed in <10 kittens and at an abundance <0.15% in all samples (i.e., Acidobacteria, Chlamydiae, Chlorobi, Chlorflexi, Gemmatimonadetes, Tenericutes, and Verrucomicrobia).
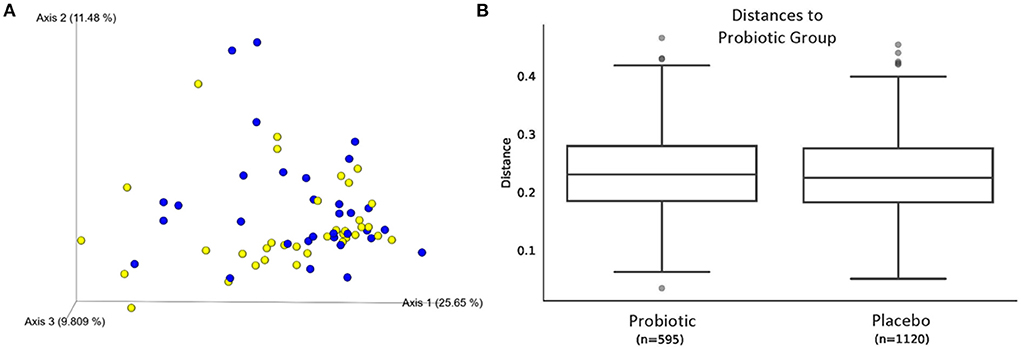
Figure 4. Beta diversity of microbiota from 67 kittens receiving probiotic or placebo. (A) Weighted UniFrac principal coordinates analysis plot showing similar phylogenic diversity of microbial communities from feces of kittens receiving the probiotic (blue circles) or placebo (yellow circles). Axis 1, 25.65%; Axis 2, 11.48%; Axis 3, 9.809%. (B) Weighted Unifrac distances between microbial communities shown in (A). PERMANOVA q = 0.755.
Kittens receiving the probiotic had a significantly higher median relative abundance of the genus Enterococcus and lower abundance and prevalence of Megamonas (Figure 5). Abundance of specific taxa observed in the feces of kittens receiving probiotic vs. placebo are shown in Table 4. Kittens receiving the probiotic were 10.9 times less likely to have detectable Megamonas (odds ratio = 0.157; 95%CI = 0.054–0.453, p < 0.001, Chi-square test) present in the microbial community compared to kittens receiving the placebo.
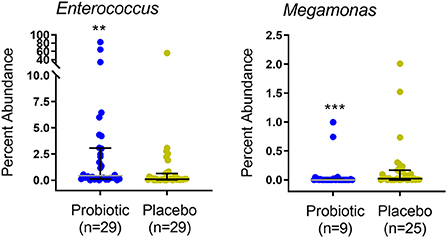
Figure 5. Percent abundance of Enterococcus and Megamonas in feces of 67 kittens receiving probiotic (32 kittens, blue circles) or placebo (35 kittens, yellow circles). Data points represent individual kittens. Bars represent median and interquartile range. n = number of kittens having the OTU at an abundance >0. Kruskal-Wallis ANOVA **p = 0.005 (BH-corrected p = 0.24). ***p < 0.001 (BH-corrected p < 0.09). BH, Benjamini-Hochberg.
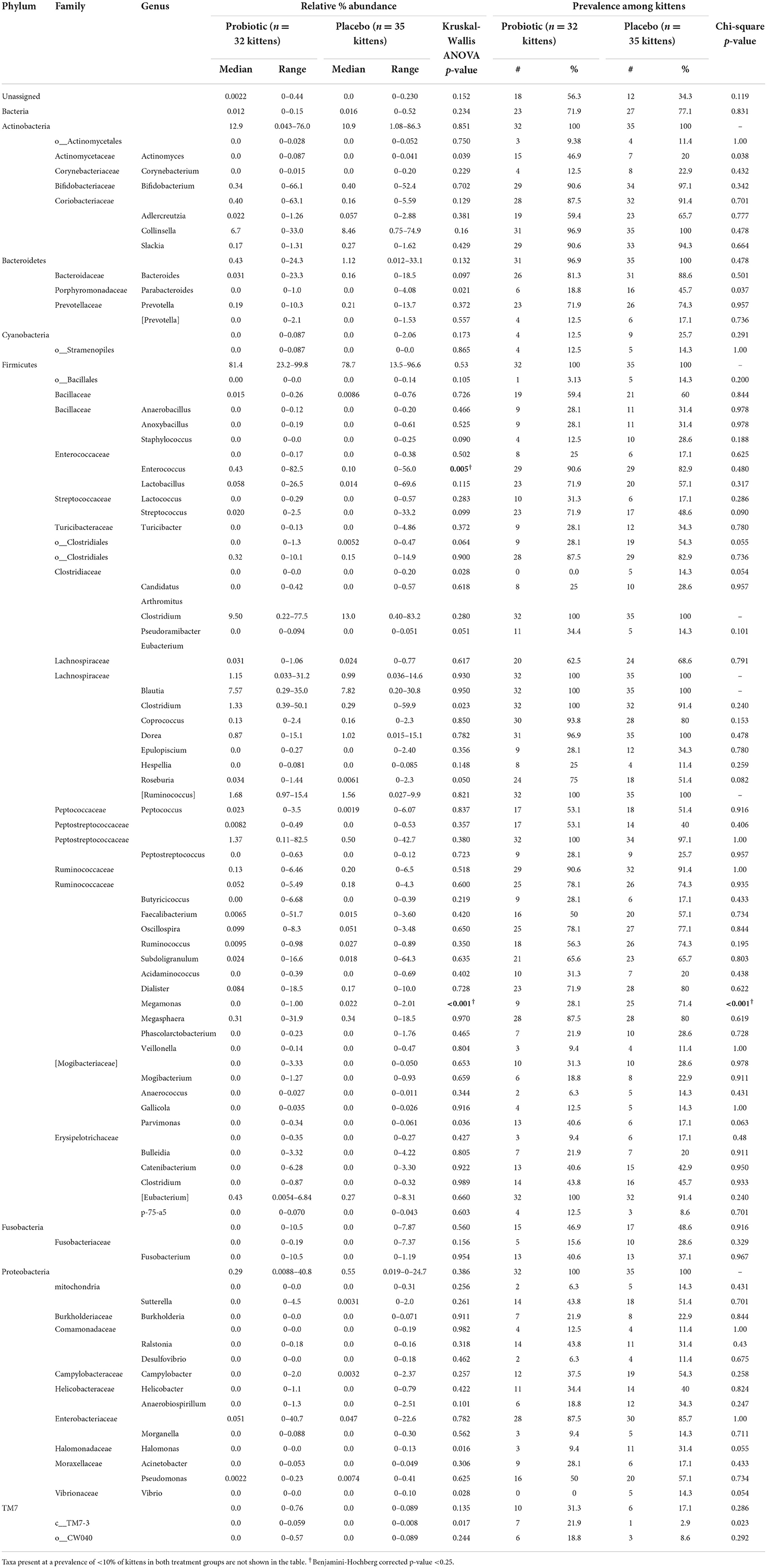
Table 4. Relative abundance and prevalence of taxa (percent of total sequences) observed by amplification and sequencing of 16S rDNA from feces of 67 kittens randomized to treatment with daily E. hirae probiotic (32 kittens) or placebo (35 kittens).
Discussion
Some unique features of this study are the high-risk target population (shelter kittens), realistic environmental conditions, preventative strategy, naturally-occurring disease outcome measurement, randomized controlled trial design, and use of a host-origin probiotic. Only 2 prior studies describe use of a host-origin probiotic in cats. The first involved administration of Bifidobacterium pseudocatenulatum previously isolated from the feces of adult healthy cats to other healthy adult client-owned cats (36). In the second study, E. faecium and Lactobacillus isolated via endoscopic duodenal aspirate from a healthy cheetah, increased body weight and improved fecal quality when given to 8–13 month old cheetah cubs (37).
The present study demonstrates that feline-origin E. hirae, administered as a probiotic, is voluntarily consumed by kittens when added to canned cat food, maintained a stable number of colony forming units of bacteria as kept frozen over the course of the study, and had no adverse effects on body weight gain during administration. Based on results of 16S rRNA gene amplicon sequencing, kittens receiving E. hirae had a significantly higher relative abundance of Enterococcus spp. identified in feces compared to those receiving the placebo. We have previously shown that administration of the same probiotic formulation of E. hirae to purpose-bred kittens results in significant increases in the specific identity of E. hirae in feces using both qPCR and quantitative culture (25). Inadequate numbers of samples had sufficient DNA remaining after sequencing to perform a specific qPCR assay for E. hirae in this study.
The incidence of diarrhea among foster kittens over the course of the study was relatively low. Despite this, there was a significantly lower incidence of diarrhea among kittens receiving the probiotic compared to the placebo which supports a beneficial effect. It is interesting that diarrhea in the placebo group involved a number of litters with multiple affected kittens whereas diarrhea occurred only among singletons in the probiotic group. Individual kittens with diarrhea did not undergo additional diagnostic testing as this was outside the scope of the study. Another limitation of our study is that data on daily fecal consistency of kittens was not obtained. Instead, we chose to conservatively define diarrhea based on a severity prompting examination of the kitten by the shelter veterinary team. This is the precedent means by which the shelter recognizes and treats diarrhea in foster kittens and best represents the subset of kittens at risk for diarrheal morbidity and mortality in the population. An experimental design requiring foster care providers to first witness and then assign a daily fecal score to individual kittens was considered unfeasible and a probable deterrent to participation in the study. Given the lower incidence of diarrhea in kittens receiving the probiotic in this investigation, a targeted study to examine fecal quality of kittens receiving E. hirae is of interest.
Our interest in E. hirae as a probiotic was based on identification of this species as the predominant mucosa-associated flora in the small intestine of healthy kittens (4). In healthy kittens, E. hirae are frequently observed to colonize the epithelial surface (4, 24). Unknown to us at the time of our observation was a fairly robust preexisting attraction to use of E. hirae as a probiotic. Numerous isolates of E. hirae, ranging in origin from the rumen (38), intestinal tract of ocean (39) and freshwater fish (40) to goats milk (37) have been studied for probiotic effects both in-vivo and in-vitro. E. hirae is bile salt and acid tolerant (41) which promotes its survival through the upper gastrointestinal tract (25) and demonstrate cell wall hydrophobicity which enables it to colonize by interaction with host intestinal epithelial cells (22, 38, 40). A lipoteichoic acid of E. hirae has been demonstrated to ameliorate the loss of barrier function caused by the pro-inflammatory cytokine TNF-α on Caco-2 cells by modulating the expression of tight junction regulatory proteins (42). We have previously shown that administration of E. hirae to purpose-bred kittens mitigates the increase in intestinal permeability resulting from experimental infection with feline-origin EPEC (25). Other beneficial mechanisms attributed to E. hirae include free radical scavenging and lipase activity (17, 40).
We previously observed a decrease in prevalence of mucosa-associated E. hirae in the ileum of terminally-ill foster kittens. Loss of E. hirae was associated with colonization by virulent genotypes of E. faecalis and ability of EPEC to attach to the intestinal epithelium (4). The enterococci, including E. hirae (38, 40, 43), produce peptides (i.e., bacteriocins) with a wide range of antibacterial and in some cases antiviral activity (44). Because bacteriocins can promote niche competition with intestinal pathogens, their production is considered to be a favorable attribute of a probiotic (44). For example, when administered to hybrid catfish as a probiotic, E. hirae protected against infection by Aeromonas hydrophila, enhanced disease protection, and stimulated immunity-related gene expression (45). Similarly, we sought to explore whether administration of feline-origin E. hirae probiotic to foster kittens might augment host defense by promoting clearance of existing infectious agents or preventing acquisition of new infectious agents during the course of foster care. To examine this possibility we used a commercial multiplex qPCR-based assay to determine the presence and quantity of intestinal microbes in fecal samples collected prior to onset and upon conclusion of the study. A high prevalence for carriage of several infectious agents was demonstrated prior to onset of the study, which included feline enteric coronavirus, attaching and effacing E. coli (presence of eae), and panleukopenia virus. A similar prevalence for carriage of these agents in asymptomatic communally housed cats and kittens has been reported by others (46–50). Overall, our study did not provide strong evidence for an impact of E. hirae on promoting clearance or preventing acquisition of these selected agents during the course of foster care. The only difference observed between the placebo and E. hirae treatment groups was a significant decrease in prevalence of panleukopenia virus DNA among kittens that received the placebo (p = 0.002) and a non-significant decrease among kittens that received E. hirae (P = 0.054). Interpretation of this finding is confounded by concurrent vaccination of kittens with modified-live panleukopenia virus vaccine every 2–3 weeks over the course of the study and the fact that no kittens were clinically unwell at the time of fecal PCR testing. In effort to distinguish between positive PCR results due to modified live virus replication vs. those arising from clinical infection, the reference laboratory applies an a-priori cut-off for positive test results at a Cp value of ≤26. All panleukopenia PCR-positive samples in the study met these criteria. However, recent studies have shown that 21.6% of healthy cats and kittens will shed panleukopenia virus in the positive test result range within 7 days of vaccination (51). Remarkably, the percentage of asymptomatic kittens testing positive for panleukopenia virus DNA in this study is nearly identical at 21.8%. Accordingly it is not unreasonable to conclude that vaccine-origin panleukopenia was largely responsible for PCR-positive results in these healthy kittens. In interpreting the results of the infectious disease testing in this study, it is important to recognize that the presence of microbial DNA does not prove agent viability or measure a potential impact of E. hirae on microbial behavior. For example we have previously documented that E. hirae can mitigate intestinal injury caused by EPEC infection in kittens while having no measureable impact on EPEC shedding (25).
Based on 16S rRNA gene amplicon sequencing, administration of the E. hirae probiotic did not alter the predominant bacterial phyla present in feces which were similar to prior descriptions of the fecal microbiota in cats. These phyla were represented mainly by Firmicutes, Bacteroidetes, Proteobacteria, Actinobacteria, and Fusobacteria (20, 52–56). The presence of E. hirae also did not affect the overall number or diversity of different taxa present in the microbial community which is a desirable attribute of a probiotic. Kittens receiving E. hirae had a significant decrease in presence and relative abundance of the genus Megamonas (phylum Firmicutes, family Veillonellaceae). Because Megamonas was present at low abundance among the fecal microbiota from kittens, the clinical significance of decreased representation in kittens receiving E. hirae is unclear. Arguably the most pertinent location to determine an impact of the E. hirae probiotic would be on composition of the small intestinal microbiota where mucosa-associated E. hirae dominate the enterococci and from which the probiotic strain was isolated. Obtaining samples from the small intestine of kittens in this study was not feasible using the current study design. In prior reports, dietary supplementation with fermentable prebiotics increased the abundance of Megamonas (57–59) while decreased in abundance of Megamonas have been described in cats with diarrhea (60). Interestingly, Megamonas was increased in feces of cats that were naturally infected with the diarrheal pathogen Tritrichomonas foetus, but decreased in feces of cats experimentally infected with the same pathogen (56). These collective observations suggest a vulnerability of Megamonas to specific, albeit unidentified changes in the feline intestinal tract. Because the enterococci are lactic acid producers and Megamonas utilizes lactate to form propionate (61) we would not have predicted a decrease in Megamonas in kittens receiving the E. hirae probiotic. In fact, in dogs fed a raw diet, increases in lactate production were positively correlated with abundance of Megamonas (62). Ultimately a deeper understanding of any interaction between E. hirae and Megamonas and the clinical relevance of our observation will require additional study.
In conclusion, a decreased incidence of diarrhea associated with preventative administration of feline-origin E. hirae to foster kittens in this study supports a rationale for use of E. hirae for disease prevention in this large, young, and vulnerable population.
Data availability statement
The datasets presented in this study can be found in online repositories. The names of the repository/repositories and accession number(s) can be found below: https://www.ncbi.nlm.nih.gov/, PRJNA776011.
Ethics statement
The animal study was reviewed and approved by Institutional Animal Care and Use Committee of North Carolina State University (Protocol Number: 18-067-O).
Author contributions
JG, SJS, JB-B, MA-P, and GD designed the project and secured funding. JG, SHS, SW, EW, and ME performed the experiments. JG, SJS, MA-P, AS, and GD supervised the experiments. JG, SJS, SHS, MA-P, ME, and AS performed bioinformatics analysis. JG, SJS, JB-B, MA-P, ME, AS, and JB interpreted the data. JG, SJS, JB-B, SW, MA-P, ME, AS, and JB wrote sections of the manuscript. All authors have read and approved the final manuscript.
Funding
This work was supported by grants from the Winn Feline Foundation and PetSmart Charities® (Grant W18-002) and a Research Innovation and Seed Grant from North Carolina State University. The UNC Microbiome Core is supported in part by P30 DK034987 Center for Gastrointestinal Biology and Disease (CGIBD) and the UNC Nutrition Obesity Research Center (NORC P30 DK056350).
Acknowledgments
The authors thank Jennifer Federico, Sarah McCain, Joseph Threadcraft, Joanne Duda, Diadra Cooley, Cindy Lynch, Greg Ceseretti, Elliot Ser, Maddie Mesaros, Rebecca Williams, Melissa Reel, Abigail Hiley, Gabby Vasek, Lindsay Stone, and Taylor Raines for their help with this study.
Conflict of interest
Authors ME and AS were employed by IDEXX Laboratories, Inc. Author JB was employed by Vet Med Labor GmbH Division, IDEXX Laboratories, Inc.
The remaining authors declare that the research was conducted in the absence of any commercial or financial relationships that could be construed as a potential conflict of interest.
Publisher's note
All claims expressed in this article are solely those of the authors and do not necessarily represent those of their affiliated organizations, or those of the publisher, the editors and the reviewers. Any product that may be evaluated in this article, or claim that may be made by its manufacturer, is not guaranteed or endorsed by the publisher.
References
1. Cave TA, Thompson H, Reid SW, Hodgson DR, Addie DD. Kitten mortality in the United Kingdom: a retrospective analysis of 274 histopathological examinations (1986 to 2000). Vet Rec. (2002) 151:497–501. doi: 10.1136/vr.151.17.497
2. New JC, Kelch WJ, Hutchison JM, Salman MD, King M, Scarlett JM, et al. Birth and death rate estimates of cats and dogs in U.S households and related factors. J Appl Animal welfare science : JAAWS. (2004) 7:229–41. doi: 10.1207/s15327604jaws0704_1
3. Kitts-Morgan SE, COMPANION ANIMALS SYMPOSIUM. Sustainable ecosystems: domestic cats and their effect on wildlife populations. J Anim Sci. (2015) 93:848–59. doi: 10.2527/jas.2014-8557
4. Ghosh A, Borst L, Stauffer SH, Suyemoto M, Moisan P, Zurek L, et al. Mortality in kittens is associated with a shift in ileum mucosa-associated enterococci from Enterococcus hirae to biofilm-forming Enterococcus faecalis and adherent Escherichia coli. J Clin Microbiol. (2013) 51:3567–78. doi: 10.1128/JCM.00481-13
5. Dolan ED, Doyle E, Tran HR, Slater MR. Pre-mortem risk factors for mortality in kittens less than 8 weeks old at a dedicated kitten nursery. J Feline Med Surg. (2021) 23:730–7. doi: 10.1177/1098612X20974960
6. Nutter FB, Levine JF, Stoskopf MK. Reproductive capacity of free-roaming domestic cats and kitten survival rate. J Am Vet Med Assoc. (2004) 225:1399–402. doi: 10.2460/javma.2004.225.1399
7. Sparkes AH, Rogers K, Henley WE, Gunn-Moore DA, May JM, Gruffydd-Jones TJ, et al. A questionnaire-based study of gestation, parturition and neonatal mortality in pedigree breeding cats in the UK. J Feline Med Surg. (2006) 8:145–57. doi: 10.1016/j.jfms.2005.10.003
8. Strong SJ, Gookin JL, Correa MT, Banks RE. Interventions and observations associated with survival of orphaned shelter kittens undergoing treatment for diarrhea. J Feline Med Surg. (2020) 22:292–8. doi: 10.1177/1098612X19840459
9. Hill C, Guarner F, Reid G, Gibson GR, Merenstein DJ, Pot B, et al. Expert consensus document. The International Scientific Association for Probiotics and Prebiotics consensus statement on the scope and appropriate use of the term probiotic. Nat Rev Gastroenterol Hepatol. (2014) 11:506–14. doi: 10.1038/nrgastro.2014.66
10. Vientos-Plotts AI, Ericsson AC, Rindt H, Reinero CR. Oral probiotics alter healthy feline respiratory microbiota. Front Microbiol. (2017) 8:1287. doi: 10.3389/fmicb.2017.01287
11. Marshall-Jones ZV, Baillon ML, Croft JM, Butterwick RF. Effects of Lactobacillus acidophilus DSM13241 as a probiotic in healthy adult cats. Am J Vet Res. (2006) 67:1005–12. doi: 10.2460/ajvr.67.6.1005
12. Fusi E, Rizzi R, Polli M, Cannas S, Giardini A, Bruni N, et al. Effects of Lactobacillus acidophilus D2/CSL (CECT 4529) supplementation on healthy cat performance. Veterinary Record Open. (2019) 6:e000368. doi: 10.1136/vetreco-2019-000368
13. Torres-Henderson C, Summers S, Suchodolski J, Lappin MR. Effect of enterococcus faecium strain SF68 on gastrointestinal signs and fecal microbiome in cats administered amoxicillin-clavulanate. Top Companion Anim Med. (2017) 32:104–8. doi: 10.1053/j.tcam.2017.11.002
14. Stokes JE, Price JM, Whittemore JC. Randomized, controlled, crossover trial of prevention of clindamycin-induced gastrointestinal signs using a synbiotic in healthy research cats. J Vet Internal Med. (2017) 31:1406–13. doi: 10.1111/jvim.14795
15. Whittemore JC, Stokes JE, Laia NL, Price JM, Suchodolski JS. Short and long-term effects of a synbiotic on clinical signs, the fecal microbiome, and metabolomic profiles in healthy research cats receiving clindamycin: a randomized, controlled trial. PeerJ. (2018) 6:e5130. doi: 10.7717/peerj.5130
16. Whittemore JC, Stokes JE, Price JM, Suchodolski JS. Effects of a synbiotic on the fecal microbiome and metabolomic profiles of healthy research cats administered clindamycin: a randomized, controlled trial. Gut Microbes. (2019) 10:521–39. doi: 10.1080/19490976.2018.1560754
17. Stoeker LL, Overman EL, Nordone SK, Moeser AJ, Simoes RD, Dean GA. Infection with feline immunodeficiency virus alters intestinal epithelial transport and mucosal immune responses to probiotics. Vet Immunol Immunopathol. (2013) 153:146–52. doi: 10.1016/j.vetimm.2013.01.017
18. Kathrani A, Larsen JA, Kass PH, Fascetti AJ. Effect of short-term probiotic Enterococcus faecium SF68 dietary supplementation in overweight and obese cats without comorbidities. Vet Record Open. (2016) 3:e000164. doi: 10.1136/vetreco-2015-000164
19. Lappin MR, Veir JK, Satyaraj E, Czarnecki-Maulden G. Pilot study to evaluate the effect of oral supplementation of Enterococcus faecium SF68 on cats with latent feline herpesvirus 1. J Feline Med Surg. (2009) 11:650–4. doi: 10.1016/j.jfms.2008.12.006
20. Garcia-Mazcorro JF, Lanerie DJ, Dowd SE, Paddock CG, Grutzner N, Steiner JM, et al. Effect of a multi-species synbiotic formulation on fecal bacterial microbiota of healthy cats and dogs as evaluated by pyrosequencing. FEMS Microbiol Ecol. (2011) 78:542–54. doi: 10.1111/j.1574-6941.2011.01185.x
21. Bybee SN, Scorza AV, Lappin MR. Effect of the probiotic Enterococcus faecium SF68 on presence of diarrhea in cats and dogs housed in an animal shelter. J Vet Internal Med. (2011) 25:856–60. doi: 10.1111/j.1939-1676.2011.0738.x
22. Hart ML, Suchodolski JS, Steiner JM, Webb CB. Open-label trial of a multi-strain synbiotic in cats with chronic diarrhea. J Feline Med Surg. (2012) 14:240–5. doi: 10.1177/1098612X11434386
23. Holzapfel W, Arini A, Aeschbacher M, Coppolecchia R, Pot B. Enterococcus faecium SF68 as a model for efficacy and safety evaluation of pharmaceutical probiotics. Benef Microbes. (2018) 9:375–88. doi: 10.3920/BM2017.0148
24. Nicklas JL, Moisan P, Stone MR, Gookin JL. In situ molecular diagnosis and histopathological characterization of enteroadherent Enterococcus hirae infection in pre-weaning-age kittens. J Clin Microbiol. (2010) 48:2814–20. doi: 10.1128/JCM.00916-09
25. Watson VE, Jacob ME, Bruno-Barcena JM, Amirsultan S, Stauffer SH, Piqueras VO, et al. Influence of the intestinal microbiota on disease susceptibility in kittens with experimentally-induced carriage of atypical enteropathogenic Escherichia coli. Vet Microbiol. (2019) 231:197–206. doi: 10.1016/j.vetmic.2019.03.020
26. Bruno-Barcena JM, Ragout AL, Sineriz F. Microbial physiology applied to process optimisation: lactic acid bacteria. Adv Bioproc Eng. Ii:1998:97-110. doi: 10.1007/978-94-017-0643-8_6
27. Terzaghi BE, Sandine WE. Improved medium for lactic streptococci and their bacteriophages. Appl Microbiol. (1975) 29:807–13. doi: 10.1128/am.29.6.807-813.1975
28. Stone AES, Brummet GO, Carozza EM, Kass PH, Petersen EP, Sykes J, et al. 2020 AAHA/AAFP feline vaccination guidelines. J Am Anim Hosp Assoc. (2020) 56:249–65. doi: 10.5326/JAAHA-MS-7123
29. Boom R, Sol CJ, Salimans MM, Jansen CL. Wertheim-van Dillen PM, van der Noordaa J. Rapid and simple method for purification of nucleic acids. J Clin Microbiol. (1990) 28:495–503. doi: 10.1128/jcm.28.3.495-503.1990
30. Moriello KA, Leutenegger CM. Use of a commercial qPCR assay in 52 high risk shelter cats for disease identification of dermatophytosis and mycological cure. Vet Dermatol. (2018) 29:66–e26. doi: 10.1111/vde.12485
31. Arnold JW, Roach J, Fabela S, Moorfield E, Ding S, Blue E, et al. The pleiotropic effects of prebiotic galacto-oligosaccharides on the aging gut. Microbiome. (2021) 9:31. doi: 10.1186/s40168-020–00980–0
32. Caporaso JG, Lauber CL, Walters WA, Berg-Lyons D, Lozupone CA, Turnbaugh PJ, et al. Global patterns of 16S rRNA diversity at a depth of millions of sequences per sample. Proc Natl Acad Sci U S A. (2011) 108:4516–22. doi: 10.1073/pnas.1000080107
33. Bolyen E, Rideout JR, Dillon MR, Bokulich NA, Abnet CC, Al-Ghalith GA, et al. Reproducible, interactive, scalable and extensible microbiome data science using QIIME 2. Nat Biotechnol. (2019) 37:852–7.
34. Bourgon R, Gentleman R, Huber W. Independent filtering increases detection power for high-throughput experiments. Proc Natl Acad Sci U S A. (2010) 107:9546–51. doi: 10.1073/pnas.0914005107
35. Benjamini Y, Hochberg Y. Controlling the false discovery rate: a practical and powerful approach to multiple testing. J Royal Stat Soc Ser B (Methodological). (1995) 57:289–300. doi: 10.1111/j.2517-6161.1995.tb02031.x
36. Biagi G, Cipollini I, Bonaldo A, Grandi M, Pompei A, Stefanelli C, et al. Effect of feeding a selected combination of galacto-oligosaccharides and a strain of Bifidobacterium pseudocatenulatum on the intestinal microbiota of cats. Am J Vet Res. (2013) 74:90–5. doi: 10.2460/ajvr.74.1.90
37. Koeppel KN, Bertschinger H, van Vuuren M, Picard J, Steiner J, Williams D, et al. The use of a probiotic in captive cheetahs (Acinonyx jubatus). J S Afr Vet Assoc. (2006) 77:127–30. doi: 10.4102/jsava.v77i3.359
38. Arokiyaraj S, Hairul Islam VI, Bharanidharan R, Raveendar S, Lee J, Kim DH, et al. Antibacterial, anti-inflammatory and probiotic potential of Enterococcus hirae isolated from the rumen of Bos primigenius. World J Microbiol Biotechnol. (2014) 30:2111–8. doi: 10.1007/s11274-014-1625-0
39. Masduki F. Y JM, Min CC, Karim M. Characterization of Enterococcus hirae Isolated from the Intestine of Seabass (Lates Calcarifer) as a New Potential Probiotic against Pathogenic Vibrios. Curr Microbiol. (2020) 77:3962–8. doi: 10.1007/s00284-020–02228-4
40. Adnan M, Patel M, Hadi S. Functional and health promoting inherent attributes of Enterococcus hirae F2 as a novel probiotic isolated from the digestive tract of the freshwater fish Catla catla. PeerJ. (2017) 5:e3085. doi: 10.7717/peerj.3085
41. de Castro Santos Melo C, da Silva Freire A, Galdeano MA, da Costa CF, de Oliveira Goncalves APD, Dias FS, et al. Probiotic potential of Enterococcus hirae in goat milk and its survival in canine gastrointestinal conditions simulated in vitro. Res Vet Sci. (2021) 138:188–95. doi: 10.1016/j.rvsc.2021.06.010
42. Miyauchi E, Morita H, Okuda J, Sashihara T, Shimizu M, Tanabe S. Cell wall fraction of Enterococcus hirae ameliorates TNF-alpha-induced barrier impairment in the human epithelial tight junction. Lett Appl Microbiol. (2008) 46:469–76. doi: 10.1111/j.1472-765X.2008.02332.x
43. Sheoran P, Tiwari SK. Enterocin LD3 from Enterococcus hirae LD3 causing efflux of intracellular ions and UV-absorbing materials in Gram-negative bacteria. J Appl Microbiol. (2019) 126:1059–69. doi: 10.1111/jam.14203
44. Hanchi H, Mottawea W, Sebei K, Hammami R. The genus enterococcus: between probiotic potential and safety concerns-an update. Front Microbiol. (2018) 9:1791. doi: 10.3389/fmicb.2018.01791
45. Hamid NH, Daud HM, Kayansamruaj P, Hassim HA, Mohd Yusoff MS, Abu Bakar SN, et al. Short- and long-term probiotic effects of Enterococcus hirae isolated from fermented vegetable wastes on the growth, immune responses, and disease resistance of hybrid catfish (Clarias gariepinus x Clarias macrocephalus). Fish Shellfish Immunol. (2021) 114:1–19. doi: 10.1016/j.fsi.2021.04.012
46. Arnold JW, Whittington HD, Dagher SF, Roach J, Azcarate-Peril MA, Bruno-Barcena JM. Safety and modulatory effects of humanized galacto-oligosaccharides on the gut microbiome. Front Nutr. (2021) 8:640100. doi: 10.3389/fnut.2021.640100
47. Arnold JW, Roach J, Azcarate-Peril MA. Emerging technologies for gut microbiome research. Trends Microbiol. (2016) 24:887–901. doi: 10.1016/j.tim.2016.06.008
48. Watson VE, Jacob ME, Flowers JR, Strong SJ, DebRoy C, Gookin JL. Association of atypical enteropathogenic escherichia coli with diarrhea and related mortality in kittens. J Clin Microbiol. (2017) 55:2719–35. doi: 10.1128/JCM.00403-17
49. Polak KC, Levy JK, Crawford PC, Leutenegger CM, Moriello KA. Infectious diseases in large-scale cat hoarding investigations. Vet J. (2014) 201:189–95. doi: 10.1016/j.tvjl.2014.05.020
50. Sabshin SJ, Levy JK, Tupler T, Tucker SJ, Greiner EC, Leutenegger CM. Enteropathogens identified in cats entering a Florida animal shelter with normal feces or diarrhea. J Am Vet Med Assoc. (2012) 241:331–7. doi: 10.2460/javma.241.3.331
51. Jacobson LS, Janke KJ, Ha K, Giacinti JA, Weese JS. Feline panleukopenia virus DNA shedding following modified live virus vaccination in a shelter setting. Vet J. (2022) 279:105783. doi: 10.1016/j.tvjl.2021.105783
52. Hooda S, Vester Boler BM, Kerr KR, Dowd SE, Swanson KS. The gut microbiome of kittens is affected by dietary protein:carbohydrate ratio and associated with blood metabolite and hormone concentrations. Br J Nutr. (2013) 109:1637–46. doi: 10.1017/S0007114512003479
53. Jia J, Frantz N, Khoo C, Gibson GR, Rastall RA, McCartney AL. Investigation of the faecal microbiota of kittens: monitoring bacterial succession and effect of diet. FEMS Microbiol Ecol. (2011) 78:395–404. doi: 10.1111/j.1574-6941.2011.01172.x
54. Deusch O, O'Flynn C, Colyer A, Swanson KS, Allaway D, Morris P, et al. Longitudinal study of the feline faecal microbiome identifies changes into early adulthood irrespective of sexual development. PLoS ONE. (2015) 10:e0144881. doi: 10.1371/journal.pone.0144881
55. Bermingham EN, Young W, Kittelmann S, Kerr KR, Swanson KS, Roy NC, et al. Dietary format alters fecal bacterial populations in the domestic cat (Felis catus). Microbiologyopen. (2013) 2:173–81. doi: 10.1002/mbo3.60
56. Bierlein M, Hedgespeth BA, Azcarate-Peril MA, Stauffer SH, Gookin JL. Dysbiosis of fecal microbiota in cats with naturally occurring and experimentally induced Tritrichomonas foetus infection. PLoS ONE. (2021) 16:e0246957. doi: 10.1371/journal.pone.0246957
57. Kerr KR, Dowd SE, Swanson KS. Faecal microbiota of domestic cats fed raw whole chicks v. an extruded chicken-based diet. J Nutr Sci. (2014) 3:e22. doi: 10.1017/jns.2014.21
58. Garcia-Mazcorro JF, Barcenas-Walls JR, Suchodolski JS, Steiner JM. Molecular assessment of the fecal microbiota in healthy cats and dogs before and during supplementation with fructo-oligosaccharides (FOS) and inulin using high-throughput 454-pyrosequencing. PeerJ. (2017) 5:e3184. doi: 10.7717/peerj.3184
59. Beloshapka AN, Dowd SE, Suchodolski JS, Steiner JM, Duclos L, Swanson KS. Fecal microbial communities of healthy adult dogs fed raw meat-based diets with or without inulin or yeast cell wall extracts as assessed by 454 pyrosequencing. FEMS Microbiol Ecol. (2013) 84:532–41. doi: 10.1111/1574-6941.12081
60. Suchodolski JS, Foster ML, Sohail MU, Leutenegger C, Queen EV, Steiner JM, et al. The fecal microbiome in cats with diarrhea. PLoS ONE. (2015) 10:e0127378. doi: 10.1371/journal.pone.0127378
61. Polansky O, Sekelova Z, Faldynova M, Sebkova A, Sisak F, Rychlik I. Important metabolic pathways and biological processes expressed by chicken cecal microbiota. Appl Environ Microbiol. (2015) 82:1569–76. doi: 10.1128/AEM.03473-15
Keywords: 16S rRNA gene amplicon sequencing, diarrheal pathogens, growth and survival, infection, polymerase chain reaction, shelter medicine
Citation: Gookin JL, Strong SJ, Bruno-Bárcena JM, Stauffer SH, Williams S, Wassack E, Azcarate-Peril MA, Estrada M, Seguin A, Balzer J and Davidson G (2022) Randomized placebo-controlled trial of feline-origin Enterococcus hirae probiotic effects on preventative health and fecal microbiota composition of fostered shelter kittens. Front. Vet. Sci. 9:923792. doi: 10.3389/fvets.2022.923792
Received: 19 April 2022; Accepted: 02 November 2022;
Published: 17 November 2022.
Edited by:
Andrea Lauková, Slovak Academy of Sciences, SlovakiaReviewed by:
Jan S. Suchodolski, Texas A&M University, United StatesMartin Fraga, National Institute for Agricultural Research (INIA), Uruguay
Copyright © 2022 Gookin, Strong, Bruno-Bárcena, Stauffer, Williams, Wassack, Azcarate-Peril, Estrada, Seguin, Balzer and Davidson. This is an open-access article distributed under the terms of the Creative Commons Attribution License (CC BY). The use, distribution or reproduction in other forums is permitted, provided the original author(s) and the copyright owner(s) are credited and that the original publication in this journal is cited, in accordance with accepted academic practice. No use, distribution or reproduction is permitted which does not comply with these terms.
*Correspondence: Jody L. Gookin, Sm9keV9Hb29raW5AbmNzdS5lZHU=