- 1College of Animal Science and Technology, Hebei Agricultural University, Baoding, China
- 2College of Food Science and Technology, Hebei Agricultural University, Baoding, China
Inorganic zinc (Zn) supplements are commonly used in poultry feeds, but their low utilization results in the increase of Zn excretion. Thus, to provide a new perspective for the substitution of inorganic Zn, a novel Zn methionine hydroxy analog chelate (Zn-MHA) was studied in the present study to evaluate its effects on laying performance, serum hormone indexes and reproductive axis-related genes in broilers breeders. A total of 480 Hubbard breeders (56-week-old) were fed a basal diet (containing 27.81 mg Zn/kg) without Zn addition for 2 weeks, and then allocated to 4 groups with 6 replicates (each replicate consisting of 10 cages and 2 breeders per cage) for 10 weeks. Four treatment diets given to broiler breeders included the basal diet added with 25, 50, and 75 mg/kg of Zn-MHA and 100 mg/kg of Zn sulfate (ZnSO4). The laying rate, egg weight and feed conversation ratio increased in the 75 mg/kg Zn-MHA group compared to the ZnSO4 group. The eggshell thickness was not decreased with the addition of 50 mg/kg and 75 mg/kg Zn-MHA in the diet compared to the 100 mg/kg ZnSO4 group. There was a significant improvement in the reproductive performance of breeders in the 75 mg/kg Zn-MHA group, including the fertility and 1-day-old offspring weight. Besides, serum sex hormone levels including FSH and P4 increased significantly in 75 mg/kg Zn-MHA group. No significant effect on the ovarian weight or the number of follicles in broiler breeders was observed by supplementing Zn-MHA. Compared to the 100 mg/kg ZnSO4 group, dietary supplementation with 75 mg/kg of Zn-MHA showed an up-regulation of the FSHR mRNA in the granular layer of follicles. However, dietary supplementation of Zn-MHA had no effects on mRNA expressions of the ovarian LHR and PRLR genes. These findings reinforce the suggestion that Zn-MHA (75 mg/kg) could replace ZnSO4 (100 mg/kg) as a Zn supplement in diet of broiler breeders, which resulted in better laying and reproduction performances by regulating the expression levels of reproductive axis related genes and serum hormone levels.
Introduction
Zinc (Zn) is an essential trace element that participates in the composition of numerous enzymatic systems and has various functions, involving energy, carbohydrate, nucleic acid and protein metabolism (1). In the poultry industry, Zn plays a critical role in laying performance, egg quality (2), reproductive performance (3), and antioxidant activity (4). In the plant-feeding ingredients, Zn is often complexed with phytic acid, thereby hindering its absorption (5). Therefore, additional Zn supplements are usually required to meet the Zn need in the poultry industry. Inorganic Zn supplements, such as ZnO and ZnSO4, are the most commonly used zinc supplements in poultry feed due to their low price and easy preparation. However, adversary effects of inorganic Zn, such as low bioavailability, large excretion, high degree of oxidation, and destruction of nutrition, have been criticized by poultry farming practitioners (6). Zn often competes with other trace elements or is inhibited by antagonists, which affects its bioavailability (7). Therefore, finding a pathway that is not affected by other trace elements or inhibitors will be the key to improving Zn utilization.
Zinc methionine hydroxy analog chelate (Zn-MHA), a newly designed Zn fortifier, has a ring structure like other Zn amino acid chelates. The two deprotonated methionine hydroxy analog (MHA) molecules coordinate with the metal cations through the two oxygen atoms of the carboxyl and hydroxyl groups, respectively, to form two penta-atomic chelate rings (8). The special ring structure that surrounds Zn ions protects them from the inhibitors (9). The MHA, also known as 2-hydroxy-4-(methylthio) butanoic acid, is a precursor used to supplement methionine (10). MHA has higher bioavailability than DL-methionine in poultry (11). Importantly, MHA can form stable chelates with divalent metals. The preparation of Zn-MHA is to put MHA and metal inorganic salt aqueous solution in the presence of alkaline solution to maintain a certain reaction temperature (8). Therefore, compared with other amino acid chelates, Zn-MHA has the advantages of simple preparation and lower price. The biosafety of MHA metal chelate was verified by human intestinal CACO-2 cells in vitro (8). Previous study in rats showed that Zn-MHA had higher bioavailability than ZnSO4, which was manifested as decreased Zn excretion and increased hepatic Zn deposition (12). Similarly, Zn methionine (Zn-Met) has been proved to be a more available source of Zn than inorganic forms like ZnO or ZnSO4 (13). Zn-Met declines the antagonistic interactions between Zn and nutrients or non-nutritive components in the gastrointestinal tract, which enhances the bioavailability of Zn (14, 15).
Zn is of great significance to ensure the laying performance and egg quality of breeders (16). In the late phase of egg-laying, laying performance and thickness of eggshells are poor, especially the expensive breeding eggs will bring greater economic losses (17). Therefore, finding a way to improve the utilization rate of Zn in the diet will be an effective way to ensure the performance of broiler breeders in the late phase. The effects of Zn-MHA on broiler breeders during the late phase are poorly known. Hence, the effects of total replacement of inorganic Zn by Zn-MHA on performance, serum hormone levels, and expression of reproductive axis related genes of aged broiler breeders were evaluated in the present study.
Materials and Methods
Animals, Diets, and Design
The experimental Zn-MHA (16% of Zn and 80% of methionine) was provided by Novus Inc. (St. Charles, MO, USA). A total of 480 (56-week-old) Hubbard breeding hens with similar body weight and initial egg production were housed in cages and randomly assigned to 4 treatment groups with 6 replicates. Each replicate contained 10 cages and 2 breeders per cage. Four treatment diets given to broiler breeders were as follows: basal diet supplemented with 100 mg/kg ZnSO4 as control group; basal diet with 25, 50, and 75 mg/kg Zn-MHA as three trail groups, respectively. The available Zn contents in the four experimental diets were: 129.80, 53.20, 80.71, and 108.60 mg/kg, respectively. Each breeder was fed a fixed diet of 158 g/d. During the experiment, water was free to access for the breeders. The feeding trial lasted for 10 weeks, after being fed a basal Zn diet (27.81 mg/kg) without extra Zn for 2 weeks to deplete the Zn in breeders. The basal diet was formulated with reference to NRC (1994). Methionine was supplemented in the premix for each treatment group to eliminate nutrient imbalances. The basal diet formula and nutrients are listed in Table 1. During the experiment, breeders were housed under a controlled temperature (25 ± 2°C) and relative humidity (74 ± 10%). Regular lighting (16L:8D) and ventilation were also performed throughout the experiments.
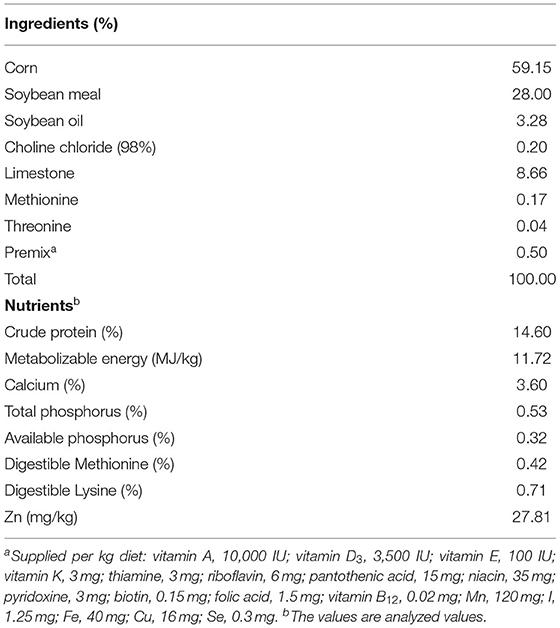
Table 1. Dietary composition and nutrient levels of the basal diet for broiler breeders (air-dried basis).
Sample Preparation
Egg numbers and egg weight were measured every day at a fixed time during the feeding trial. On the last day of the feeding trial, 5 eggs per replicate were chosen for quality determination. One hen was randomly chosen from each replicate for blood collection. Blood was taken from the vein under the wings into a coagulation-promoting tube. After centrifugation at 1,500 × g at 4°C for 15 min, serum was collected. Three hens from each treatment were then killed by cervical dislocation. Ovaries were removed and weighed. Follicles were classified as small yellow follicles (SYFs, 4 to 10 mm) and large white follicles (LWFs, 2 to 4 mm) according to their size. All types of follicles in the ovary were counted after sorting by size. The first three largest preovulatory follicles (POFs) were removed from the ovary and were designated as F1, F2, and F3 (18). The granulosa layers of SYFs, LWFs, and POFs were collected and frozen by liquid nitrogen and stored at −80°C.
Laying Performance and Egg Quality
The laying rate was calculated based on the daily records, whereas the feed conversion rate (FCR) was calculated weekly and was expressed as feed to egg weight.
The egg quality indexes included shape index, eggshell strength, eggshell thickness, yolk color, yolk weight, albumen height, and Haugh unit. The shape index was calculated by dividing egg width by egg length. Eggshell strength was evaluated performed by Egg Force Reader. The albumen height, yolk color, and Haugh unit were measured using an egg analyzer. The above two instruments used were procured from Orka Ltd. (Ramat HaSharon, Israel). Eggshell thickness was measured by a vernier caliper.
Hatching Performance
To evaluate the hatching traits, eggs were incubated in week 8 to 10. A total of 15 clean eggs without visible abnormalities were selected for incubation of each replicate per week. The eggs were stored in a controlled environment room for 7 d and the temperature was maintained at 18 to 20°C with a relative humidity of 75% to 80%. The incubation was then conducted in a commercial multi-stage incubator (YALN-19200, Jinan, China) at 37.5°C with 60% relative humidity. Unfertilized eggs were removed following candling on day 7 and 18 of the incubation were also examined to pick out non-viable eggs during incubation. The reproductive performance included fertility, hatchling capacity, hatchling capacity of fertile eggs, total embryonic mortality, healthy offspring percentage, and 1-day-old offspring weight.
Serum Hormone Analysis
The concentrations of follicle-stimulating hormone (FSH), luteinizing hormone (LH), prolactin (PRL), estradiol (E2), progesterone (P4), triiodothyronine (T3) and thyroxine (T4) were determined by assay kits obtained from J&L Biotechnology Technology Co., Ltd (Shanghai, China).
mRNA Relative Expression Levels of Reproductive Axis Genes by Real-Time Quantitative PCR
The determination of mRNA relative expression levels including follicle-stimulating hormone receptor (FSHR), luteinizing hormone receptor (LHR), and prolactin receptor (PRLR) were conducted by the SYBR Green I Real-time qPCR analysis. Total RNA was extracted from granulosa layer samples, using Total RNA extraction kit (GenePool Biotech Co., Ltd., Beijing, China). The output of RNA was measured by a spectrophotometer (Thermo Scientific, Wilmington, DE, USA), and electrophoresis was used to evaluate its quality.
Quantification was performed by reverse transcription (RT) and PCR. Following the technical manual of the mRNA-cDNA synthesis kit (GenePool Biotech Co., Ltd., Beijing, China), each RT reaction consisted of 1 μg RNA, 4 μL of 5 × RT Buffer, 4 μL of dNTP Mix (2.5 mM each), 1 μL of total RNA, 2 μL of DTT (0.1 M), 1 μL of HiFiScript (200 U/μL), and 2 μL of Primer Mix, and adding RNase-free water to 20 μL. Reactions were performed for 50 min at 42°C, followed by heat inactivation of reaction for 5 min at 85°C. The 20 μL RT reaction mix was kept at −20°C.
Analysis of each sample was performed in triplicate. The primers were synthesized by GenePool Biotech Co., Ltd. (Beijing, China) according to previous study (19), as shown in Table 2. Real-time qPCR was conducted using a LineGene 9600 Plus (Bioer Technology Co. Ltd., Hangzhou, China) PCR system with a total volume of 20 μL containing 2 μL of cDNA, 10 μL of 2 × fast SYBR mixture, 0.4 μL of forward primer, 0.4 μL of reverse primers, and 7.2 μL of nuclease-free water. Reaction mixtures were incubated at 95°C for 10 min, followed by 40 cycles at 95°C for 10 s, and 60°C for 30 s. After the PCR cycles, melting curve analysis was carried out to confirm the specific generation of the PCR products.
The relative expression of the mRNA was normalized to the expression of β-actin, and the 2−ΔΔCt method was used to calculate the levels of relative expressions (20).
Statistical Analysis
The difference between the control (ZnSO4) and each experimental group was carried out using the independent-samples t test by statistical analysis system (SAS Institute Inc., Cary, NC, USA). The orthogonal contrast was performed to test the linear and quadratic P-values of increasing dietary Zn-MHA levels. P < 0.05 was considered to be significant. The replicate was the experimental unit for the laying performance. The results are expressed as mean and standard deviation.
Results
Effect of Zn-MHA on Laying Performance of Broiler Breeders
It can be seen from Table 3 that the laying rate and average egg weight increased linearly and quadratic (P < 0.001) by increasing the concentration of Zn-MHA, and reached the highest in the group fed with a 75 mg/kg Zn-MHA diet. Compared to the ZnSO4 group, dietary supplementation of 75 mg/kg Zn-MHA increased the laying rate (P < 0.05). However, 25 mg/kg Zn-MHA group showed a decrease in laying rate compared to the ZnSO4 group. We found that dietary supplementation of 50 and 75 mg/kg Zn-MHA showed an improvement both in egg weight and FCR value (P < 0.05). The broken egg rate was not affected by whatever source of Zn (P > 0.05).
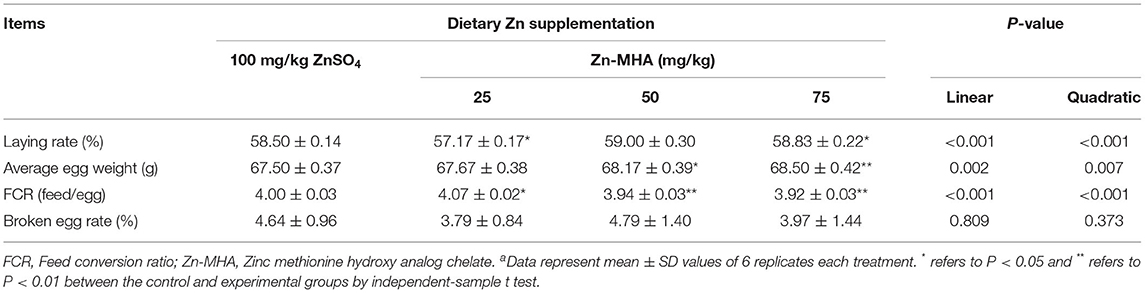
Table 3. Effects of dietary Zn-MHA supplementation on laying performance of broiler breedersa.
Effect of Zn-MHA on Egg Quality of Broiler Breeders
Dietary Zn-MHA supplementation had no effects on egg quality of breeders at the end of the trial based on the data of shape index, yolk color, yolk weight, albumen height, Haugh unit or eggshell strength compared with the ZnSO4 group (P > 0.05; Table 4). The eggshell thickness decreased in the 25 mg/kg Zn-MHA group in the diet (P < 0.05). However, the eggshell thickness of 50 mg/kg and 75 mg/kg Zn-MHA group did not differ with the control group.
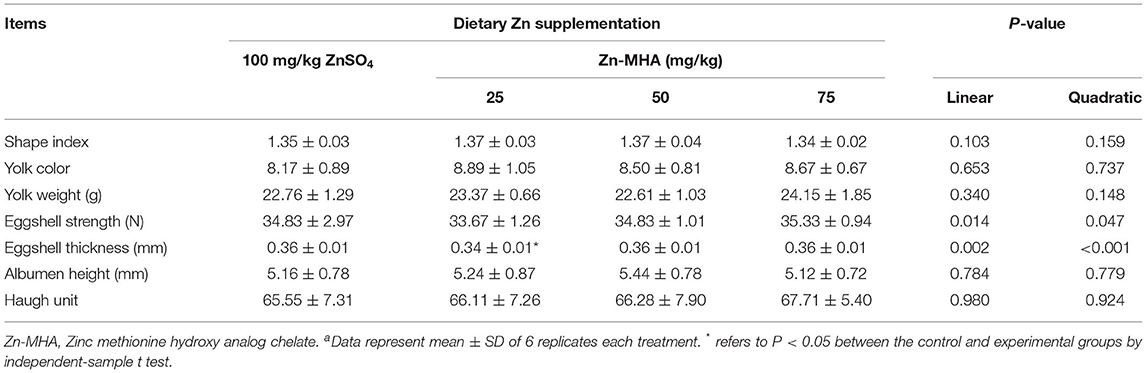
Table 4. Effects of dietary Zn-MHA supplementation on egg quality of broiler breedersa.
Effect of Zn-MHA on Hatching Performance of Broiler Breeders
As shown in Table 5, there was a significant improvement in the fertility rate of the broilers belonging to the 75 mg/kg Zn-MHA diet-fed group compared to the ZnSO4 group (P < 0.05). No effects of dietary Zn-MHA supplementation on hatchling capacity, hatching capacity of fertile eggs, percentage of healthy offspring, and total embryonic mortality were observed. Compared to the ZnSO4 group, the offspring weight of the 50 and 75 mg/kg Zn-MHA diet fed groups was significantly increased (P < 0.05).
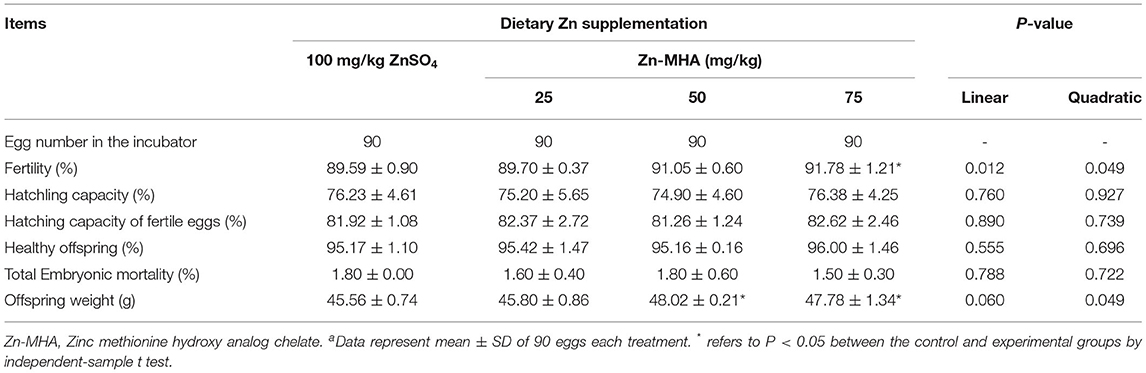
Table 5. Effects of dietary Zn-MHA supplementation on hatching performance in broiler breedersa.
Effect of Zn-MHA on Serum Hormone of Broiler Breeders
Dietary supplementation of Zn-MHA had a significant impact on the serum hormone indexes (Table 6). In general, the impact was associated with increasing supplement levels of Zn-MHA. Most obviously, serum FSH level in 25 mg/kg Zn-MHA group decreased (P < 0.05), while that in 75 mg/kg group increased (P < 0.01). The change of FSH level in serum was linear and quadratic (P < 0.001) with the supplement level of Zn-MHA in diet. Another thing worth noting is that P4 level in serum of breeders was also increased in the 75 mg/kg Zn-MHA group (P < 0.05). Serum T3 and E2 level in 25 mg/kg Zn-MHA group was lower than that in the ZnSO4 group (P < 0.05). No significant changes were observed in serum LH, PRL and T4 levels in all treatment groups.
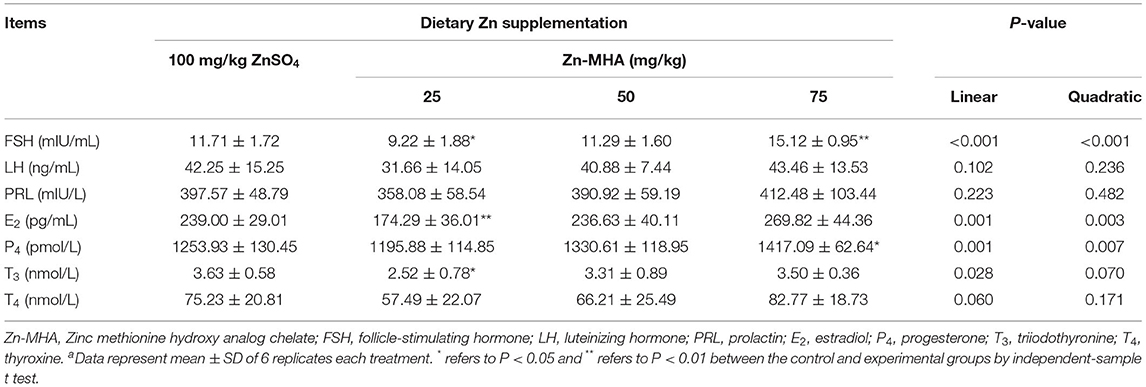
Table 6. Effects of dietary Zn-MHA supplementation on serum hormone analysis of broiler breedersa.
Effect of Zn-MHA on Reproductive Organ Development of Broiler Breeders
Compared with the ZnSO4 group, dietary supplementation of Zn-MHA did not affect the ovarian weight or the number of SYFs and LWFs in broiler breeders (Table 7).
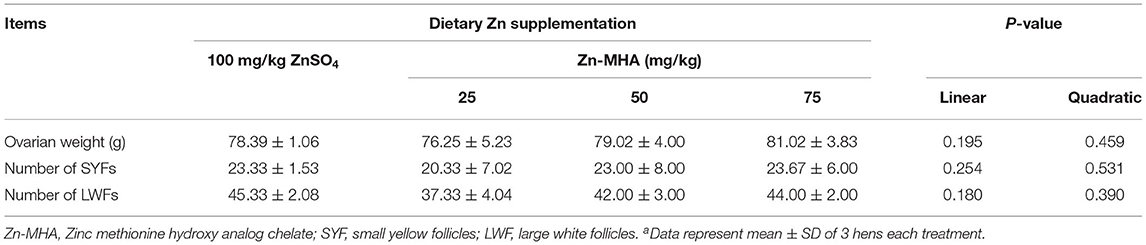
Table 7. Effects of dietary Zn-MHA supplementation on ovarian development in broiler breedersa.
Effect of Zn-MHA on Reproductive Axis Related MRNA Expressions of Broiler Breeders
Dietary supplementation of Zn-MHA affected mRNA expression of FSHR in LWFs, F2 and F3 (Figure 1A). The FSHR expression was down-regulated in 25 mg/kg Zn-MHA groups in LWFs compared to ZnSO4 group. However, no differences were observed between the 50 mg/kg and 75 mg/kg Zn-MHA diet-fed group and the ZnSO4 group. The FSHR mRNA expression was increased in the dietary supplementation of 75 mg/kg Zn-MHA in F2 (P < 0.05). However, there was a down-regulation in F3 between the ZnSO4 group and the 25 mg/kg, 50 mg/kg and 75 mg/kg Zn-MHA groups. No differences were detected for all the treatments of Zn-MHA in SYFs and F1.
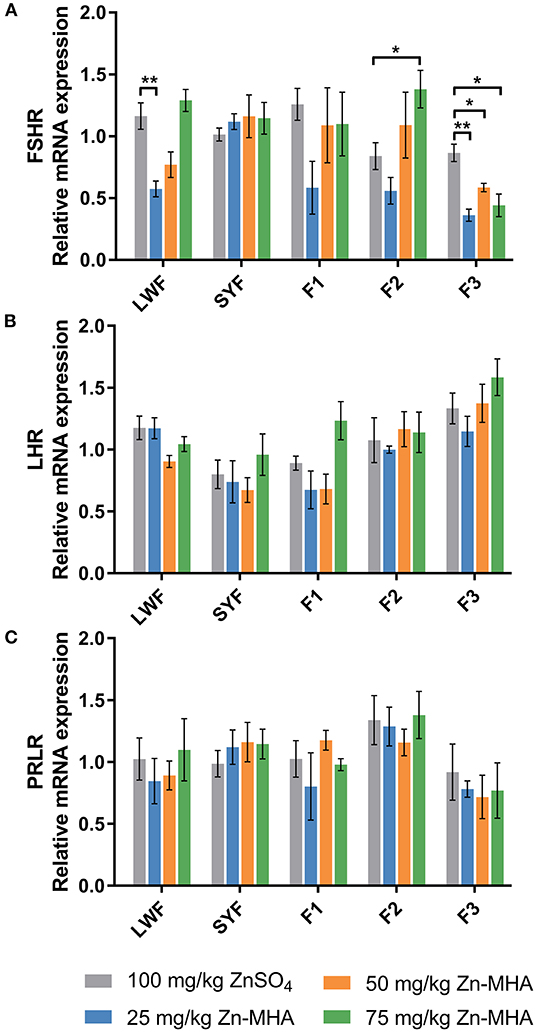
Figure 1. Effects of dietary Zn-MHA supplementation on the mRNA relative expressions of reproductive axis genes in broiler breeders. SYF, small yellow follicles (4 to 10 mm in diameter). LWF, large white follicles (2 to 4 mm in diameter). F1, the first largest one of preovulatory follicles. F2, the second largest one of preovulatory follicles. F3, the third largest one of preovulatory follicles. FSHR, follicle-stimulating hormone receptor; LHR, luteinizing hormone receptor; PRLR, prolactin receptor. (A–C) Data represent mean values of 3 hens each treatment as mean ± SEM. * refers to P < 0.05 and ** refers to P < 0.01 between the control and experimental groups by independent-sample t test.
The mRNA expression of LHR and PRLR measured in LWFs, SYFs and POFs was not affected by different Zn source (Figures 1B,C).
Discussion
The role of organic Zn, especially amino acid chelated Zn, for human and animal health has been of constant interest (21). The concept of environmental protection and emission reduction is deeply rooted among the people, therefore, the studies on replacing inorganic Zn with organic Zn in broilers are of significant importance. In our study, supplementation of Zn-MHA to the broilers significantly affected FCR and egg weight. The results are consistent with the previous findings that the organic Zn has more benefits than inorganic Zn in promoting the laying performance of hens (22–24). The increase in FCR may be attributed to the effect of Zn on the increase in the related hormones such as P4 and FSH (16). The egg weight increased linearly with the increasing levels of Zn-MHA in diet. It is reported that an increase in hen's egg weight was observed of hens supplemented with organic Zn, which was similar to the present study (25). These improvements may be owing to the higher bioavailability of Zn-MHA, which can be better absorbed by animals and exert its important functions (26, 27). Different forms of dietary Zn have been reported to be efficiently deposited in eggs and transported to different tissues of offspring as embryos develop (28, 29). In addition, Stefanello et al. (30) found that using organic Zn instead of inorganic Zn did not exhibit a negative effect on FCR. In our study, we found that diets supplemented with 50 and 75 mg/kg Zn-MHA significantly improved FCR compared to the ZnSO4 group. Abd El-Hack et al. (31) reported that Zn-Met improved the feed conversion ratio in laying hens. However, Roshanzamir et al. (32) reported that dietary supplementation of organic Zn had no significant effect on the feed conversion ratio. The above inconsistencies might be due to the differences in Zn levels or experimental phases. The higher bioavailability of Zn-MHA could be responsible for the improvement of laying performance, which made hens make better use of Zn to secrete sex hormones. Moreover, the lower dose of Zn-MHA (75 mg/kg) appeared to have the same or even better effect than the 100 mg/kg inorganic zinc, which was attributed to the structural specificity advantage of the amino acid chelate.
The improvement of egg quality of hens by the dietary supplementation of Zn in the aged breeders is also a topic of concern. Age and nutrition are constant factors that affect egg quality. The decline of eggshell quality, such as eggshell thickness and eggshell strength, is a common phenomenon in aged laying hens, especially the expensive breeding eggs could bring greater economic losses (33). Results from previous studies have shown that supplementation of Zn in diets with either inorganic or organic forms had an impact on the egg quality (31, 34, 35). It is reported that adding Zn-MHA to the layer diet increased eggshell weight, eggshell thickness, eggshell strength and eggshell density (36). Also, partial or complete replacement of inorganic trace element compounds by organic trace element compounds has been proved to improve the eggshell quality and increase mineral deposition in eggs (37). These shreds of evidence also support our findings. A number of studies have shown that adding organic Zn to diets can significantly increase the Haugh unit score of eggs compared with inorganic Zn (15). Similar to these reports, we also detected some differences in egg quality between the Zn-MHA groups and ZnSO4 group, confirming that supplementation of Zn-MHA at the concentration of 75 mg/kg could not affect the egg quality. Also, we observed that lower doses of Zn-MHA (25 mg/kg) were not sufficient to meet the needs of breeders, resulting in reduced eggshell thickness.
Zn plays an indispensable role in the early meiosis of cells (38). Also, Zn functions in follicular rupture and cumulus expansion (39). These important effects may be directly related to the activity of Zn-dependent enzymes matrix metalloproteinases (40). In mammals, Zn enters the fetus through metallothionein, which is necessary for fetal growth and development (41). Zn also plays an important role in embryo development and egg hatchability, because the content of Zn in eggs is positively correlated with egg hatchability (3). It has been reported that “Zn sparks,” consisting of thousands of zinc vesicles, are triggered after the fertilization of a mammalian egg and are required to induce the development of the fertilized egg toward the embryo (42). Zhu et al. (43) reported that maternal dietary supplementation with the organic Zn improved hatchability. In general, Zn was reported to improve male sperm quality and is essential for the female reproductive system including embryogenesis and development (44). It is reported that Zn deficiency affected the reproductive function of rats (45, 46). In agreement with the results obtained by Favero et al. (47), we can detect some difference in egg fertility and hatchability between the supplementation levels of Zn-MHA and between Zn-MHA and ZnSO4. The hatching capacity, healthy offspring rates, and embryo mortality were not affected by treatment in the present study. Supplementation of 75 mg/kg Zn-MHA to diets of hens affects the quality of fertilized eggs. Also, no benefit was observed in reproductive performance in layers when dietary Zn level was over 150 mg/kg feed (48). One possible reason for this could be that the level of Zn in the basic diet was different and Zn-MHA had a higher bioavailability resulting in the supplementation of Zn in the diet thereby meeting the layer poultry requirement. More Zn enters into the body of hens to promote the secretion of reproductive hormones, which leads to the improvement of reproductive performance. And Zn also entered the eggs to promote the development of offspring.
Gonadotropins, such as FSH and LH, play a particularly important role in the course of follicular development and ovulation (49). FSH enhances the secretion of P4 by follicular granulosa cells through P450 side-chain cleavage in the preovulatory stage, so as to promote follicular development and maturation (50). LH, which has the same α-subunit as FSH, also has the function of promoting P4 secretion (51). Increasing levels of FSH and LH can also stimulate the secretion of E2 in follicles (52, 53). The raise of E2 could improve the sensitivity of the hypothalamic-pituitary axis to secrete more P4 (54). PRL secreted by the anterior pituitary is also important for the maintenance and secretory activity of the corpus luteum (55). The thyroid hormones constitute the endocrine system which has important functions in growth and energy utilization in birds (56). Therefore, these serum hormones have been considered as instructive indicators of laying performance (57). Root et al. (58) evidenced that Zn influenced FSH and LH activities in rats. It is reported that dietary zinc-methionine supplementation, at a level of 50 mg/kg DM, improved milk production and hormones in dairy camels (59). In the present study, we found that serum FSH and P4 levels were elevated with the increasing level of Zn-MHA supplementation. At the same time, we also detected such a trend in FCR, suggesting that dietary Zn-MHA could have a potential impact on the laying performance.
The increase of reproductive hormones in serum helps to increase the expression of related receptor genes in the ovary. FSH has been proved to stimulate ovarian FSHR expression (60, 61). Also, FSH mediates the expression of LHR by silencing Insig siRNA (62). The up-regulation of the above two receptors contributes to the binding of follicles to FSH and LH to promote follicular maturation (63). In the present study, the lower FSHR gene expression observed in broilers fed with 25 mg/kg Zn-MHA was consistent with the lower serum concentrations of gonadal hormones FSH and E2. While the increase in serum FSH level can be responsible for the up-regulation of FSHR mRNA in the 75 mg/kg Zn-MHA group. These hormones participate in the regulation of follicle development and pregnancy maintenance (64). Furthermore, we found that the changes of LHR and PRLR mRNA expression were consistent with the changes of corresponding hormones in serum, and there was no significant difference. The biochemical mechanisms for these changes need to be further studied.
Conclusions
Overall, the present study drew the conclusion that replacing ZnSO4 (100 mg/kg) with a lower dose of Zn-MHA (75 mg/kg) in the diet of broiler breeders improved the laying and reproductive performance. Moreover, this increase was achieved by increasing levels of FSH and P4 in serum and up-regulated the expression levels of FSHR in the broiler ovary. In conclusion, it is suggested that Zn-MHA may be a potential substitute for inorganic zinc supplements in broiler breeders with higher bioavailability.
Data Availability Statement
The raw data supporting the conclusions of this article will be made available by the authors, without undue reservation.
Ethics Statement
The animal study was reviewed and approved by Animal Care and Use Committee of Hebei Agricultural University.
Author Contributions
BY performed the experiments and drafted the manuscript. BY, JG, JJ, SL, and YH carried out the statistical analysis. ZF, GL, GZ, and YH helped the revision of this manuscript. ZF and GZ contributed to the supervision and guidance of the present study. All authors read and approved the final manuscript.
Funding
This study was supported by the Hebei Province Technology R&D Program (20326617D and 19227305D).
Conflict of Interest
The authors declare that the research was conducted in the absence of any commercial or financial relationships that could be construed as a potential conflict of interest.
Publisher's Note
All claims expressed in this article are solely those of the authors and do not necessarily represent those of their affiliated organizations, or those of the publisher, the editors and the reviewers. Any product that may be evaluated in this article, or claim that may be made by its manufacturer, is not guaranteed or endorsed by the publisher.
Acknowledgments
The authors would like to express their gratitude to EditSprings (https://www.editsprings.cn/) for the expert linguistic services provided.
References
1. Sanna A, Firinu D, Zavattari P, Valera P. Zinc status and autoimmunity: a systematic review and meta-analysis. Nutrients. (2018) 10:68. doi: 10.3390/nu10010068
2. Olgun O, Yildiz A. Effects of dietary supplementation of inorganic, organic or nano zinc forms on performance, eggshell quality, and bone characteristics in laying hens. Ann Anim Sci. (2017) 17:463–76. doi: 10.1515/aoas-2016-0055
3. Prabakar G, Gopi M, Kolluri G, Rokade JJ, Khillare G, Pearlin BV, et al. Effect of supplementation of zinc-methionine on egg production, semen quality, reproductive hormones, and hatchability in broiler breeders. Biol Trace Elem Res. (2021) 199:4721–30. doi: 10.1007/s12011-021-02590-9
4. Naz S, Idris M, Khalique M, Alhidary I, Abdelrahman M, Khan R, et al. The activity and use of zinc in poultry diets. Worlds Poult Sci J. (2016) 72:159–67. doi: 10.1017/S0043933915002755
5. Brouns F. Phytic acid and whole grains for health controversy. Nutrients. (2021) 14:25. doi: 10.3390/nu14010025
6. Cao J, Henry PR, Guo R, Holwerda RA, Toth JP, Littell RC, et al. Chemical characteristics and relative bioavailability of supplemental organic zinc sources for poultry and ruminants. J Anim Sci. (2000) 78:2039–54. doi: 10.2527/2000.7882039x
7. Lonnerdal B. Dietary factors influencing zinc absorption. J Nutr. (2000) 130:1378S−83. doi: 10.1093/jn/130.5.1378S
8. Predieri G, Tegoni M, Cinti E, Leonardi G, Ferruzza S. Metal chelates of 2-Hydroxy-4-methylthiobutanoic acid in animal feeding: preliminary investigations on stability and bioavailability. J Inorg Biochem. (2003) 95:221–4. doi: 10.1016/S0162-0134(03)00067-9
9. Udechukwu M, Collins S, Udenigwe C. Prospects of enhancing dietary zinc bioavailability with food-derived zinc-chelating peptides. Food Funct. (2016) 7:4137–44. doi: 10.1039/C6FO00706F
10. Dibner J, Knight C. Conversion of 2-Hydroxy-4-(Methylthio)butanoic acid to L-methionine in the chick: a stereospecific pathway. J Nutr. (1984) 114:1716–23. doi: 10.1093/jn/114.9.1716
11. Agostini PS, Dalibard P, Mercier Y, Van der Aar P, Van der Klis JD. Comparison of methionine sources around requirement levels using a methionine efficacy method in 0 to 28 day old broilers. Poult Sci. (2016) 95:560–9. doi: 10.3382/ps/pev340
12. Predieri G, Elviri L, Tegoni M, Zagnoni I, Cinti E, Biagi G, et al. Metal chelates of 2-hydroxy-4-methylthiobutanoic acid in animal feeding: part 2: further characterizations, in vitro and in vivo investigations. J Inorg Biochem. (2005) 99:627–36. doi: 10.1016/j.jinorgbio.2004.11.011
13. Rahman MM, Wahed MA, Fuchs GJ, Baqui AH, Alvarez JO. Synergistic effect of zinc and vitamin a on the biochemical indexes of vitamin a nutrition in children. Am J Clin Nutr. (2002) 75:92–8. doi: 10.1093/ajcn/75.1.92
14. Behjatian Esfahani M, Moravej H, Ghaffarzadeh M, Nehzati Paghaleh GA. Comparison the Zn-Threonine, Zn-Methionine, and Zn oxide on performance, egg quality, zn bioavailability, and zn content in egg and excreta of laying hens. Biol Trace Elem Res. (2021) 199:292–304. doi: 10.1007/s12011-020-02141-8
15. Li L, Gong Y, Zhan H, Zheng Y, Zou X. Effects of dietary Zn-Methionine supplementation on the laying performance, egg quality, antioxidant capacity, and serum parameters of laying hens. Poult Sci. (2018) 98:923–31. doi: 10.3382/ps/pey440
16. Park S-Y, Birkhold S, Kubena L, Nisbet D, Ricke S. Review on the role of dietary zinc in poultry nutrition, immunity, and reproduction. Biol Trace Elem Res. (2004) 101:147–63. doi: 10.1385/BTER:101:2:147
17. Sirri F, Zampiga M, Berardinelli A, Meluzzi A. Variability and interaction of some egg physical and eggshell quality attributes during the entire laying hen cycle. Poult Sci. (2018) 97:1818–23. doi: 10.3382/ps/pex456
18. Gilbert AB, Evans AJ, Perry MM, Davidson MH. A method for separating the granulosa cells, the basal lamina and the theca of the preovulatory ovarian follicle of the domestic fowl (Gallus Domesticus). J Reprod Fertil. (1977) 50:179–81. doi: 10.1530/jrf.0.0500179
19. Long L, Wu S, Yuan F, Zhang H, Wang J, Qi G. Effects of dietary octacosanol supplementation on laying performance, egg quality, serum hormone levels, and expression of genes related to the reproductive axis in laying hens. Poult Sci. (2016) 96:894–903. doi: 10.3382/ps/pew316
20. Livak KJ, Schmittgen T. Analysis of relative gene expression data using real-time quantitative Pcr and the 2−ΔΔct method. Methods. (2001) 25:402–8. doi: 10.1006/meth.2001.1262
21. Chien XX, Zafra-Stone S, Bagchi M, Bagchi D. Bioavailability, antioxidant and immune-enhancing properties of zinc methionine. Biofactors. (2006) 27:231–44. doi: 10.1002/biof.5520270120
22. Skrivan M, Skrivanová V, Marounek M. Effect of various copper supplements to feed of laying hens on Cu content in eggs, liver, excreta, soil, and herbage. Arch Environ Contam Toxicol. (2006) 50:280–3. doi: 10.1007/s00244-005-1028-1
23. Jahanian R, Moghaddam HN, Rezaei A. Improved broiler chick performance by dietary supplementation of organic zinc sources. Asian-Australas J Anim Sci. (2008) 21:1348–54. doi: 10.5713/ajas.2008.70699
24. Sunder GS, Panda AK, Gopinath NCS, Rao SVR, Raju MVLN, Reddy MR, et al. Effects of higher levels of zinc supplementation on performance, mineral availability, and immune competence in broiler chickens. J Appl Poult Res. (2008) 17:79–86. doi: 10.3382/japr.2007-00029
25. Paik I-K. Application of chelated minerals in animal production. Asian-Australas J Anim Sci. (2001) 14:191–8. doi: 10.5713/ajas.2001.384
26. Chan S, Gerson B, Subramaniam S. The role of copper, molybdenum, selenium, and zinc in nutrition and health. Clin Lab Med. (1999) 18:673–85. doi: 10.1016/S0272-2712(18)30143-4
27. Ibs K-H, Rink L. Zinc-altered immune function. J Nutr. (2003) 133:1452S−6. doi: 10.1093/jn/133.5.1452S
28. Iñiguez C, Casas J, Carreres J. Effects of zinc deficiency on the chick embryo blastoderm. Acta Anat. (1978) 101:120–9. doi: 10.1159/000144957
29. Mabe I, Rapp C, Bain M, Nys Y. Supplementation of a corn-soybean meal diet with manganese, copper, and zinc from organic or inorganic sources improves eggshell quality in aged laying hens. Poult Sci. (2004) 82:1903–13. doi: 10.1093/ps/82.12.1903
30. Stefanello C, Santos TC, Murakami AE, Martins EN, Carneiro TC. Productive performance, eggshell quality, and eggshell ultrastructure of laying hens fed diets supplemented with organic trace minerals. Poult Sci. (2014) 93:104–13. doi: 10.3382/ps.2013-03190
31. Abd El-Hack ME, Alagawany M, Amer S, Arif M, Wahdan KM, El-Kholy M. Effect of dietary supplementation of organic zinc on laying performance, egg quality and some biochemical parameters of laying hens. J Anim Physiol Anim Nutr. (2017) 102:e542–e9. doi: 10.1111/jpn.12793
32. Roshanzamir H, Rezaei J, Fazaeli H. Colostrum and milk performance, and blood immunity indices and minerals of holstein cows receiving organic Mn, Zn and Cu sources. Anim Nutr. (2020) 6:61–8. doi: 10.1016/j.aninu.2019.08.003
33. Feng J, Zhang H-J, Wu S-G, Qi G-H, Wang J. Uterine transcriptome analysis reveals mrna expression changes associated with the ultrastructure differences of eggshell in young and aged laying hens. BMC Genom. (2020) 21:770. doi: 10.1186/s12864-020-07177-7
34. Zhang YN, Zhang HJ, Wang J, Yue HY, Qi XL, Wu SG, et al. Effect of dietary supplementation of organic or inorganic zinc on carbonic anhydrase activity in eggshell formation and quality of aged laying hens. Poult Sci. (2017) 96:2176–83. doi: 10.3382/ps/pew490
35. Qiu JL, Zhou Q, Zhu JM, Lu XT, Liu B, Yu DY, et al. Organic trace minerals improve eggshell quality by improving the eggshell ultrastructure of laying hens during the late laying period. Poult Sci. (2020) 99:1483–90. doi: 10.1016/j.psj.2019.11.006
36. Min YN, Liu FX, Qi X, Ji S, Ma SX, Liu X, et al. Effects of methionine hydroxyl analog chelated zinc on laying performance, eggshell quality, eggshell mineral deposition, and activities of Zn-Containing enzymes in aged laying hens. Poult Sci. (2018) 97:3587–93. doi: 10.3382/ps/pey203
37. Zhang KK, Han MM, Dong YY, Miao ZQ, Zhang JZ, Song XY, et al. Low levels of organic compound trace elements improve the eggshell quality, antioxidant capacity, immune function, and mineral deposition of aged laying hens. Animal. (2021) 15:100401. doi: 10.1016/j.animal.2021.100401
38. Hester J, Hanna-Rose W, Diaz F. Zinc deficiency reduces fertility in C. elegans hermaphrodites and disrupts oogenesis and meiotic progression. Comp Biochem Physiol C Toxicol Pharmacol. (2017) 191:203–9. doi: 10.1016/j.cbpc.2016.09.006
39. Tian X, Diaz FJ. Zinc depletion causes multiple defects in ovarian function during the periovulatory period in mice. Endocrinology. (2012) 153:873–86. doi: 10.1210/en.2011-1599
40. Peluffo MC, Murphy MJ, Baughman ST, Stouffer RL, Hennebold JD. Systematic analysis of protease gene expression in the rhesus macaque ovulatory follicle: metalloproteinase involvement in follicle rupture. Endocrinology. (2011) 152:3963–74. doi: 10.1210/en.2011-1172
41. Coyle P, Philcox JC, Carey LC, Rofe AM. Metallothionein: the multipurpose protein. Cell Mol Life Sci. (2002) 59:627–47. doi: 10.1007/s00018-002-8454-2
42. Que EL, Bleher R, Duncan FE, Kong BY, Gleber SC, Vogt S, et al. Quantitative mapping of zinc fluxes in the mammalian egg reveals the origin of fertilization-induced zinc sparks. Nat Chem. (2015) 7:130–9. doi: 10.1038/nchem.2133
43. Zhu YW, Li WX, Lu L, Zhang LY, Ji C, Lin X, et al. Impact of maternal heat stress in conjunction with dietary zinc supplementation on hatchability, embryonic development, and growth performance in offspring broilers. Poult Sci. (2017) 96:2351–9. doi: 10.3382/ps/pew481
44. Fallah A, Mohammad-Hasani A, Colagar A. Zinc is an essential element for male fertility: a review of zn roles in men's health, germination, sperm quality, and fertilization. J Reprod Infertil. (2018) 19:69–81.
45. Om AS, Chung KW. Dietary zinc deficiency alters 5α-reduction and aromatization of testosterone and androgen and estrogen receptors in rat liver. J Nutr. (1996) 126:842–8. doi: 10.1093/jn/126.4.842
46. Gilabert ER, Ruiz E, Osorio C, Ortega E. Effect of dietary zinc deficiency on reproductive function in male rats: biochemical and morphometric parameters. J Nutr Biochem. (1996) 7:403–7. doi: 10.1016/S0955-2863(96)00063-0
47. Favero A, Vieira S, Angel C, Bess F, Scher Cemin H, Ward T. Reproductive performance of Cobb 500 breeder hens fed diets supplemented with zinc, manganese, and copper from inorganic and amino acid-complexed sources. J Appl Poult Res. (2013) 22:80–91. doi: 10.3382/japr.2012-00607
48. Lim HS, Paik I-K. Effects of supplementary mineral methionine chelates (Zn, Cu, Mn) on the performance and eggshell quality of laying hens. Asian Australas J Anim Sci. (2003) 16:1804–8. doi: 10.5713/ajas.2003.1804
49. Anderson RC, Newton CL, Anderson RA, Millar RPJER. Gonadotropins and their analogs: current and potential clinical applications. Endocr Rev. (2018) 39:911–37. doi: 10.1210/er.2018-00052
50. Hernandez A, Bahr J. Role of Fsh and epidermal growth factor (Egf) in the initiation of steroidogenesis in granulosa cells associated with follicular selection in chicken ovaries. Reproduction. (2003) 125:683–91. doi: 10.1530/rep.0.1250683
51. Thompson IR, Kaiser UB. Gnrh pulse frequency-dependent differential regulation of Lh and Fsh gene expression. Mol Cell Endocrinol. (2014) 385:28–35. doi: 10.1016/j.mce.2013.09.012
52. Das N, Kumar TR. Molecular regulation of follicle-stimulating hormone synthesis, secretion and action. J Mol Endocrinol. (2018) 60:R131–55. doi: 10.1530/JME-17-0308
53. Shoham Z, Jacobs HS, Insler V. Luteinizing hormone: its role, mechanism of action, and detrimental effects when hypersecreted during the follicular phase. Fertil Steril. (1993) 59:1153–61. doi: 10.1016/S0015-0282(16)55968-8
54. Tarumi W, Itoh MT, Suzuki N. Effects of 5α-dihydrotestosterone and 17β-Estradiol on the mouse ovarian follicle development and oocyte maturation. PLoS ONE. (2014) 9:e99423. doi: 10.1371/journal.pone.0099423
55. Freeman M, Kanyicska B, Lerant A, Nagy G. Prolactin: structure, function, and regulation of secretion. Physiol Rev. (2000) 80:1523–631. doi: 10.1152/physrev.2000.80.4.1523
56. Carew L, Evarts K, Alster FA. Growth, feed intake, and plasma thyroid hormone levels in chicks fed dietary excesses of essential amino acids. Poult Sci. (1998) 77:295–8. doi: 10.1093/ps/77.2.295
57. Mohammadi H, Ansari-Pirsaraei Z. Changes in some blood parameters and production performance of old laying hens due to growth hormone and testosterone injection. J Anim Physiol Anim Nutr. (2013) 98:483–90. doi: 10.1111/jpn.12095
58. Root AW, Duckett G, Sweetland M, Reiter EO. Effects of zinc deficiency upon pituitary function in sexually mature and immature male rats. J Nutr. (1979) 109:958–64. doi: 10.1093/jn/109.6.958
59. Mostafa TH, Bakr AA, Ayyat MS. Reproductive and productive efficiency of maghrebi dairy she-camels fed diets supplemented with zinc-methionine. Biol Trace Elem Res. (2020) 194:135–44. doi: 10.1007/s12011-019-01744-0
60. LaPolt P, Tilly J, Aihara T, Nishimori K, Hsueh AJW. Gonadotropin-induced up- and down-regulation of ovarian follicle-stimulating hormone (Fsh) receptor gene expression in immature rats: effects of pregnant mare's serum gonadotropin, human chorionic gonadotropin, and recombinant Fsh. Endocrinology. (1992) 130:1289–95. doi: 10.1210/endo.130.3.1537292
61. Simoni M. The follicle-stimulating hormone receptor: biochemistry, molecular biology, physiology, and pathophysiology. Endocr Rev. (1997) 18:739–73. doi: 10.1210/er.18.6.739
62. Menon B, Guo X, Garcia N, Gulappa T, Menon K. Mir-122 regulates Lhr expression in rat granulosa cells by targeting Insig1 Mrna. Endocrinology. (2018) 159:2075–82. doi: 10.1210/en.2017-03270
63. Xu Z. Expression of follicle-stimulating hormone and luteinizing hormone receptor messenger ribonucleic acids in bovine follicles during the first follicular wave1. Biol Reprod. (1995) 53:951–7. doi: 10.1095/biolreprod53.4.951
Keywords: Zinc methionine hydroxy analog chelate, laying performance, hormone levels, reproduction, gene expression, broiler breeder
Citation: Yang B, Gong J, Jing J, Hao Y, Li S, Liu G, Feng Z and Zhao G (2022) Effects of Zinc Methionine Hydroxy Analog Chelate on Laying Performance, Serum Hormone Levels, and Expression of Reproductive Axis Related Genes in Aged Broiler Breeders. Front. Vet. Sci. 9:918283. doi: 10.3389/fvets.2022.918283
Received: 12 April 2022; Accepted: 13 June 2022;
Published: 04 July 2022.
Edited by:
Kai Wang, Chinese Academy of Agricultural Sciences (CAAS), ChinaReviewed by:
Shiping Bai, Sichuan Agricultural University, ChinaBasheer Nusairat, Jordan University of Science and Technology, Jordan
Lihong Zhao, China Agricultural University, China
Copyright © 2022 Yang, Gong, Jing, Hao, Li, Liu, Feng and Zhao. This is an open-access article distributed under the terms of the Creative Commons Attribution License (CC BY). The use, distribution or reproduction in other forums is permitted, provided the original author(s) and the copyright owner(s) are credited and that the original publication in this journal is cited, in accordance with accepted academic practice. No use, distribution or reproduction is permitted which does not comply with these terms.
*Correspondence: Zhihua Feng, fzhauh@163.com; Guoxian Zhao, zgx959@163.com