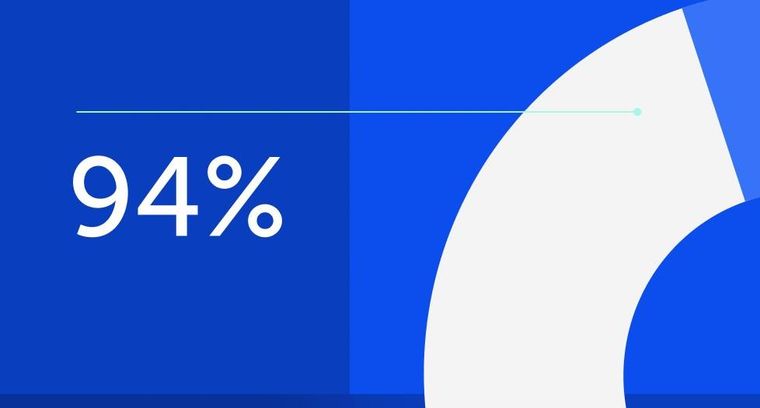
94% of researchers rate our articles as excellent or good
Learn more about the work of our research integrity team to safeguard the quality of each article we publish.
Find out more
REVIEW article
Front. Vet. Sci., 11 August 2022
Sec. Veterinary Infectious Diseases
Volume 9 - 2022 | https://doi.org/10.3389/fvets.2022.915475
Many pathogenic viruses infect camels, generally regarded as especially hardy livestock because of their ability to thrive in harsh and arid conditions. Transmission of these viruses has been facilitated by the commercialization of camel milk and meat and their byproducts, and vaccines are needed to prevent viruses from spreading. There is a paucity of information on the effectiveness of viral immunizations in camels, even though numerous studies have looked into the topic. More research is needed to create effective vaccines and treatments for camels. Because Camels are carriers of coronavirus, capable of producing a powerful immune response to recurrent coronavirus infections. As a result, camels may be a suitable model for viral vaccine trials since vaccines are simple to create and can prevent viral infection transfer from animals to humans. In this review, we present available data on the diagnostic, therapeutic, and preventative strategies for the following viral diseases in camels, most of which result in significant economic loss: camelpox, Rift Valley fever, peste des petits ruminants, bovine viral diarrhea, bluetongue, rotavirus, Middle East respiratory syndrome, and COVID-19. Although suitable vaccines have been developed for controlling viral infections and perhaps interrupting the transmission of the virus from the affected animals to blood-feeding vectors, there is a paucity of information on the effectiveness of viral immunizations in camels and more research is needed. Recent therapeutic trials that include specific antivirals or supportive care have helped manage viral infections.
Camels are known for their ability to survive and cope in the harshest environments (1), and millions of people living in the pastoral regions of central Asia, Africa, and several Western areas require camels to support their income and daily life (2–4). Because of the camel's importance, it is crucial to investigate the occurrence of viral infections in camel species, which constitute a major health and zoonotic hazard.
Historically, dromedary camels were thought to be especially resistant to infectious diseases to which most domestic animals were susceptible. This assumption has since been disproven, as camels have demonstrated susceptibility to many viral infections and can act in their transmission (5). Initially, camels were known to be the primary species to contract camelpox, which was host-specific and not zoonotic. The zoonotic potential of camel infections was first observed in 2011 in India when three people were diagnosed with disease transferred from camels (6). Today, camels are known to be carriers of several infectious diseases, including peste des petits ruminants (PPR), African horse sickness, Rift Valley fever (RVF), bluetongue (BT), and West Nile disease. In 2017, camels in Kenya exhibited the highest seroprevalence (99% after seroprevalence) of the influenza D virus (IDV), which causes respiratory infections primarily in cattle, sheep, goats, and other livestock species (7). Influenza C virus (ICV) has also been detected in dromedary camels in Kenya (8). Another cattle-specific viral infection is bovine viral diarrhea (BVD), caused by bovine viral diarrhea virus (BVDV), of which there are two primary variants, BVDV1 and BVDV2 (9). BVDV is known to infect a wide range of wild and domestic animals, including sheep, deer, swine, and camels. The susceptibility of old-world (dromedary and Bactrian) and New World camelids (llama, alpaca, guanacos, and vicunas) to BVDV varies, and further investigation is needed (10). Furthermore, the susceptibility of camels to epizootic hemorrhagic disease (EHD) requires further exploration.
The current review describes the importance of viral infectious diseases in camels. We provide available data on the treatment and prevention approaches for camel viral diseases, including camelpox, RVF, BVD, PPR, BT, Middle East respiratory syndrome (MERS) coronavirus infection, and severe acute respiratory syndrome (SARS) coronavirus (Figure 1).
Camelpox is an extremely infectious skin disorder and the most common infectious viral illness of camels, occurring in most regions where camel farming is common. The camelpox virus (CMLV; genus Orthopoxvirus, family Poxviridae), the cause of this infectious disease, is closely related to the variola virus. The genes associated with viral replication activities and most of those important in additional host-related processes are identical in the two viruses (11).
Symptoms of camelpox are fever, skin lesions, and lymph node inflammation. Pox lesions of different stages may form, most notably on the face, throat, and near the tail. The disease may be diagnosed based on clinical symptoms, although camel contagious ecthyma and camel papillomatosis induce similar symptoms (12). Multiple diagnostic methods are available, including transmission electron microscopy (TEM), the most rapid diagnostic tool for detecting the characteristic, brick-shaped orthopoxvirus in tissue samples or skin lesions (13). Immunohistochemistry can also be informative. PCR may be used to confirm the presence of viral nucleic acid, and DNA restriction enzyme testing can be used to identify specific strains of the CMLV (13, 14).
The infection, like smallpox in humans, may be controlled by separating diseased camels and injecting the remainder with the standard vaccinia virus vaccine or the recently developed CMLV vaccine, available live attenuated and inactivated (15). A booster vaccine dose is recommended for young camelids inoculated before 6–9 months. The inactivated vaccine can be injected yearly (16), and the live attenuated vaccine provides long-term protection.
A live attenuated candidate vaccine was produced in Sudan using a local strain of CMLV and assessed in a small-scale field study for safety and efficacy in experimental camels (17). Most tests revealed that the proposed vaccine is effective, safe, and can control the infection. Most vaccines are produced from the CMLV strains Ducapox 298/89, Jouf-78, VD47/25, and CMLV-T8. The attenuated Jouf-78 strain has been found to provide complete protection against CMLV (17). A new vaccine developed from serial multiplication of the KM-40 virus strain on the chorioallantoic membranes of 11-day-old embryonated chicken eggs has the potential to protect against camelpox in Old World camelids (Camelus dromedaries and Camelus bactrianus) (15).
General non-specific treatment for infected camels is the administration of 10 mg/kg oxytetracycline and 0.2 mg/kg meloxicam for 5 days (18). A spray containing gamma benzene hexachloride, proflavine hemisulphate, cetrimide, eucalyptus oil, turpentine oil, and neem oil can also be used for wound therapy and fly control (18). Other ethnopharmacological applications are also widely used to treat camelpox (19). In humans, cidofovir would likely be beneficial in the treatment and short-term prevention of smallpox and kindred poxvirus infections, as well as the treatment of vaccinia sequelae in immunocompromised individuals (20). Cidovir and its acyclic nucleoside phosphonate derivatives have shown promising therapeutic potency against camelpox (21).
Camel contagious ecthyma (CCE) is a highly contagious viral disease with a 38% fatality rate that mostly affects young camels, causing calf debility. Caused by a poxvirus (genus Parapoxvirus, family Poxviridae), CCE is distinguished by pustular lesions around the mouth, lips, and buccal cavity, and head swelling (22). The disease is distinguished by a rapid onset and lesion development. PCR targeted to the RPO30 gene is the most often used assay for samples from skin scrapings or pustules (23). Negative contrast electron microscopy, immunofluorescence, and immunoperoxidase assays are also employed.
Vaccination using CCE virus-containing material appears promising. However, immunization with vaccinia virus and a vaccine against infectious ecthyma in sheep and goats did not protect camels from infection (24).
Traditional therapies include cauterization of regional lymph nodes, the application of sesame oil and heated milk, and plant tar. Topical or systemic broad-spectrum antibiotics for 3–5 days prevent subsequent bacterial infection. Antipyretics, antihistamines, and multivitamins are also helpful in minimizing the consequences of the infection (22). Administering the preferred NSAID flunixin (1 mg/kg) intramuscularly once daily may be recommended (25).
The RVF is a severe mosquito-borne viral infection [genus Phlebovirus, family Bunyaviridae [26]] that affects animals (lambs, camels, and cattle) as well as humans in the Arabian Peninsula and Sub-Saharan Africa. Camelids have frequently been linked with RVF epidemics in East Africa and Egypt. RVF produces severe sickness in camels, with fever, weakness, abnormalities, infertility, and an increased death rate, especially among younger camels. Signs and symptoms of RVF are ocular discharge, tongue hemorrhages, and trough edema (26).
Reverse transcription-PCR (RT-PCR) can identify the virus in the blood (during infection) and tissues. The presence of RVFV is also confirmed by an enzyme-linked immunoassay (ELISA), which shows the presence of IgM autoantibodies that appear rapidly as an effective response to an acute infection and IgG antibodies which last for many years (27).
Many types of vaccines are available. Because of the necessity for several doses, inactivated or killed vaccines are unsustainable for regular field immunization. One of the oldest and most commonly used vaccines for managing RVF in Africa is the modified live Smithburn vaccine, which requires only a single dosage; however, it has been linked to birth abnormalities and miscarriages in pregnant calves, and it may only provide camels modest protection against RVF infection (28).
MP-12, a live-attenuated vaccine, has shown encouraging results in laboratory testing (29). Moreover, the live-attenuated Clone 13 vaccine has been approved for use in South Africa. Alternative immunizations based on recombinant molecular structures are now being investigated, with promising results. Camels showed a robust and long-lasting neutralizing immune response after receiving a single dose of the live CL13T RVF vaccine, demonstrating that the product is safe to use with no notable negative effects in the vaccinated camels (30).
Tilorone in vitro inhibited both the vaccine (MP-12) and the pathogenic (ZH501) strains of RVFV at low micromolar concentrations. In a mouse model, tilorone treatment significantly improved the survival outcomes of BALB/c mice exposed to RVFV ZH501. An 80% survival rate was achieved when 30 mg/kg/day was administered immediately after infection. One day after infection, 30% of animals administered tilorone at 45 mg/kg/day survived (31). Oral favipiravir (200 mg/kg/day) prevented death in ≥60% of hamsters challenged with RVFV when administered within 1 or 6 h of exposure and decreased initial RVFV titers in serum and tissues. Ribavirin (75 mg/kg/day) relieved some symptoms of peracute RVFV illness (32).
While pre-treatment with glucan was useful in identifying antiviral medicines to prevent RVFV, the most promising results were observed for the interferon inducer polyriboinosinic–polyribocytidylic acid complex with poly-l-lysine and carboxymethylcellulose [poly(ICLC)]. Ribavirin and poly(ICLC) have demonstrated the ability to prevent sickness in hamsters. Rhesus monkeys infected with RVFV had lower viremia after receiving ribavirin (50 mg/kg loading dose, then 10 mg/kg every 8 h for 9 days). Ribavirin also reduced viral production in infected cell cultures (33). Intramuscular administration of recombinant leukocyte A interferon and Sendai virus-induced human leukocyte interferon to RVFV-infected rhesus monkeys prevented viremia and hepatocellular damage (34). Curcumin was shown to be effective against highly virulent ZH501 and inhibited viral multiplication in infected animals' livers (35). Several other effective drugs against RVFV include suramin, sorafenib, sorafenib, rapamycin and 5,6-dimethoxyindan-1-one (36).
The PPR is an infectious disorder of sheep and goats that has unexpectedly reappeared and is now extensively dispersed throughout most of the Middle East, Africa, and Asia. Anorexia, diarrhea, oculonasal secretion, severe pyrexia, necrotic stomatitis, ulcerative, and respiratory failure are common symptoms of this infection. The virus that causes the infection is the peste des petits ruminants virus [PPRV; genus Morbillivirus, family Paramyxoviridae (37)]. Camels were not previously recognized as potential PPR hosts until a study in Egypt demonstrated positive serum reactivity in Sudanese camels (38). The first PPR epidemic in camelids was described in 1996 as a highly infectious respiratory illness with significant morbidity and low mortality rates. In 2004, an epidemic of a similar illness occurred in eastern Sudan, followed by outbreaks in Somalia and Kenya. Although the reports from Kenya and Somalia did not identify the causative agent, researchers in Sudan identified it as a PPR virus (39).
Pathological lesions and specific identification of viral antibodies or antigens in medical samples by different serologic tests and molecular assays may be used to diagnose the condition. Immunocapture ELISA and RT-PCR are common diagnostic methods.
Live-attenuated vaccines with long-lasting protection against PPRV are currently available. The first homologous PPR vaccine was developed using a live attenuated Nigerian strain (Nig 75/1) that exhibited solid protection for 3 years after 63 passes in Vero cells (40). Several immunization studies performed between 1989 and 1996 demonstrated the effectiveness of this vaccine. Under field conditions, the vaccine proved safe and induced protection in 98% of vaccinated camels. Following outbreaks with virulent PPRV strains, the vaccinated camelids did not develop an infection; therefore, this vaccine is currently used globally (Middle East, Africa, and southern Asia) for efficient PPR control. Sungri/96, PPRV Arasur/87, and CBE/97 stains are available as live attenuated PPR vaccines (41).
Out of 42 goats, 38 (93.23%) recovered after receiving an antibiotic and anti-inflammatory protocol of ampicillin trihydrate, colistin sulfate, and dexamethasone acetate IM once daily for 3 days, and metronidazole 500 mg/35 kg body weight orally three times daily for 3 days (42). Long-acting tetracyclines and topical gentian violet helped resolve the PPR lesions within 3 weeks (43). The antiparasitic endectocide ivermectin inhibited PPRV replication in vitro, suggesting that it might be used as a repurposed antiviral drug against PPRV (44).
The BVDV is one of the numerous pestiviruses that infect domesticated and wild ruminants, camelids, and pigs worldwide. For cattle farmers, infection results in commercial losses due to lower growth weight, reduced milk supply, reproductive losses, and mortality. Aerosol transmission is considered the most effective mode of BVDV transmission in camelids. The virus is primarily transmitted by inhalation or ingestion (45), is present in all bodily fluids and excretions, and can transfer from mother to fetus. Fever, mouth ulcers, anorexia, diarrhea, abortion, general poor health, and birth abnormalities are some possible symptoms (46).
One-time testing cannot provide a definitive diagnosis of chronic BVDV infection in camelids. Although the BVDV antigen ELISA test is used to diagnose chronic BVDV infections in bovines, it is unknown whether a comparable interpretation of the results in camelids would be valid. As a result, chronic infections in camelids should be determined by virus identification via PCR or viral isolation in samples collected over 3 to 4 consecutive weeks (47).
No BVDV vaccine is currently approved for use in camelids, although several vaccines are available for use in cattle. Vaccines cannot prevent infection, but they do lessen the clinical symptoms of illness (48). Vaccinating camelids is not currently recommended, pending further studies. Inappropriate vaccination may prevent accurate diagnostic testing and the capacity to identify infected camelids.
Maintaining a sealed herd, establishing strong biosecurity standards for all entering animals (recommended for many viral infections, such as coronavirus), and inspecting open herds regularly may all help to limit the infection frequency (49).
Non-pregnant female alpacas were challenged via nasal and ocular injection of BVDV type 1b strain 25 days after immunization with a modified BVDV vaccine (50). The animals experienced no adverse effects. The modified-live BVDV Singer strain type 1 and BVDV 125c type 2 vaccines (Vista 3SC) were administered intravenously at a dosage of 2 ml, as directed for calves. The type 1b BVDV test strain, isolated from a chronically infected alpaca, was not detected in the immunized alpacas but was identified in the two unvaccinated controls (51).
Inactivated vaccines, by contrast, are safer and may provide adequate protection. Vaccines based on ncp BVDV strains have been developed. Authors of a recent study examining new world camels detected seroconversion following three injections with an inactivated virus preparation (52).
In cell culture, IFN-α substantially inhibited viral development. In the presence of more than 103 units/ml of IFN-α, the replication of two cytopathogenic and two non-cytopathogenic strains of BVD was inhibited. The addition of TNF-α or TNF-β to IFN-α did not influence the suppressive effect (53). Huang qin Zhizi, a Chinese herbal medication, proved beneficial in treating BVD. According to network and pathway analysis, the medication suppressed inflammation and increased host immune responses to BVD infection (54).
BT is an infectious disease caused by the bluetongue virus (BTV; genus Orbivirus, family Reoviridae) transmitted by biting midges of Culicoides spp. It primarily affects ruminant and camelid animals. Symptoms of this infection are more common in sheep, whereas asymptomatic BT infections are more prevalent in cows, goats, and camelids (55). Twenty-seven variants of BTV have thus far been identified. BTV 1–24 strains are recognized as classical, while BTV 25–27 are atypical. BT is spread worldwide, including the Americas, Africa, southern Asia, and northern Australia (56). As BT outbreaks profoundly affect the international trade of animals and animal products, the World Organization for Animal Health (OIE) listed BT as a communicable disease. The animal death rate, illness, infertility, and decreased growth rate result in huge direct economic losses, while trade reduction and medical costs cause indirect losses (57).
Although alpacas and llamas are prone to BTV outbreaks, they are asymptomatic. However, they act as carriers because BTV infection lasts for several days in those species. Camels are considered low-risk animals for BTV; however, some studies have reported BTV infection in camels, such as BTV infection in an alpaca (Vicugna pacos), llamas, and BT epidemic in Europe (58–60). Competitive ELISA, real-time PCR, and agar gel immunodiffusion tests are reportedly reliable and efficient BTV diagnostic tests recommended by OIE for international trade purposes (61).
Subunit and viral vector-based vaccines have been developed for BTV infection and are used in ruminants (62–64). These vaccines are not approved for South American camelids, although many experts advocate their use (65).
BTV is resistant to ether, chloroform, and sodium deoxycholate but sensitive to trypsin treatment. The virus is stable within a pH range of 6–8. Below a pH of 6, BTV was rendered inactive at 37°C (66). Levamisole, an anthelmintic drug, stimulated a significant immune response to the BT vaccine when administered in repeated doses of 2.5 mg/kg before immunization (67). Tarantula cubensis extract (Theranekron) showed promising efficacy in treating oral BT lesions in cattle when combined with tetracycline and flunixin meglumine (68). Re-epithelization and recovery to normal body temperature occurred 24 h after Theranekron administration, with the treatment group recovering faster than the control group.
In 2013, MERS was first identified as a human disease caused by an emerging coronavirus (MERS-CoV) mainly found in the Middle East (69–71). Multiple subsequent studies suggested that severe MERS can also be transmitted zoonotically from various animals to humans.
In 2013, researchers conducted a case study of a 43-year-old retired military veteran experiencing initial symptoms of shortness of breath, fever, rhinorrhea, and malaise, which worsened over time. The patient's personal history indicated that he owned a herd of nine camels and had been visiting the camels in their barn every day for 3 days before the onset of symptoms. One of the patient's acquaintances, who also visited the camels with the patient, reported to the doctors that four of the eight camels were suffering from a serious illness with evident nasal discharge. After admission to the hospital, the patient's condition deteriorated, and he eventually died. MERS-CoV was detected in nasal swab samples from both the camel and the patients. Subsequent genetic sequencing revealed similar sequences from the camel and the patient, and serological testing revealed that the camel had been exposed to MERS-CoV prior to the patient's illness, implying that the infection was passed from the sick camel to the person (72).
Small chemical molecules, previously developed antivirals, and antiviral peptides have been used to treat MERS-CoV infection (73–79). Despite several human studies, no consensus has been reached on the best treatment for MERS-CoV (80). Several clinical trials and in vitro and in vivo studies have been attempted to identify an effective medication. The regimens investigated have included ribavirin for 5 days plus INF α-2b (81), lopinavir/ritonavir PO + ribavirin PO + PEG-INF (82), mycophenolate mofetil (83), small molecules, and fusion peptides (76, 78).
Coronavirus disease 2019, COVID-19, was declared a global pandemic by the World Health Organization in 2020. COVID-19 is caused by severe acute respiratory syndrome coronavirus 2 (SARS-CoV-2; genus Deltacoronavirus, family Coroniviridae), a single-stranded RNA virus. Over six million people worldwide have died from the disease (84). Camels are known to be reservoirs and carriers of various coronavirus strains, and as a result, they can produce a strong immune response against recurrent coronavirus infections. Given that they are the known carriers of MERS-CoV, their immunity can be used to develop immunogenic responses against recurrent coronavirus strains (85). Lactating female camel carriers of the dormant form of MERS-CoV can produce a strong immune response against the stated coronavirus strain by producing camel-specific IgG antibodies. These antibodies can be found in the serum and milk of lactating female camels (86). The preparations of these camel antibodies can act as a source for camel vaccines to prevent SARS-CoV-2 infections. In a recent study, camel serum demonstrated cross-neutralizing activity against SARS-CoV-2. These camels were seropositive for MERS-CoV, but antigens were not detected. This observation implies that camels could be a source of specific antibodies for controlling COVID-19 (87).
The camel antibodies and nanoantibodies have been shown to elicit a strong immunogenic response and efficient neutralizing of viral antigens. Even at extreme temperatures (80°C), they can neutralize the infectious antigen by presenting on the surface and within the infected cell (88–90). Before directly developing new human vaccines, it is essential to determine the intermediate hosts responsible for MERS-CoV infection in humans. SARS-CoV-2 has been isolated from various animals, including cats, raccoons, rats, and civets, indicating that all of these animals can act as intermediate hosts. Bats were originally also thought to be important intermediate hosts for various coronaviruses. Animal vaccines are easier to design and validate than human vaccines because fewer validating steps and trials are required. The primary reason for vaccinating intermediate hosts is that their vaccine is simple to develop and can prevent viral infection from spreading from animals to humans.
The relationship between camels and SARS-CoV-2 extends beyond the infection of camels to an era of including camels in COVID-19 control. A library of nanobodies (Nbs) produced from immunized camels has been created. It was discovered that seven Nbs had a high affinity for at least eight SARS-CoV-2 RBD mutants. Among these candidates, Nb11-59 demonstrated the most neutralizing activity while maintaining a high level of stability, indicating that Nb11-59 may be a potential therapeutic molecule for an inhalation COVID-19 therapy (91). Several Nbs that bind to the SARS-CoV-2 RBD has been identified from vaccinated camelids. NIH-CoVnb-112, the principal treatment candidate, demonstrated strong affinity in monomeric form and inhibited the interaction between SARS-CoV-2 and ACE2 (92).
Rotavirus (RV) has been found in New World and Old World camels (93). The first African camel rotavirus strain was reported in 2014 (94). Some portions of the strain were related to human–animal reassortant rotaviruses. Multiple reassortment events between mammalian strains resulted in strain MRC-DPRU447. In a camel calf diarrhea survey in Sudan using group A rotavirus antibody, 53% showed high antibody titers (95). ELISA and the observed characteristic wheel-like morphology of rotavirus particles by electron microscopy were among the diagnostic procedures (96, 97). When delivered orally to calves and pigs in the field, live attenuated rotavirus vaccines were ineffective due to colostral antibodies that hindered vaccine virus multiplication. The presence of rotavirus antibodies in colostrum prompted the development of maternal rotavirus vaccination techniques to improve lactogenic immunity and transfer passive antibodies to the neonate via colostrum and milk (98).
Rotavirus is the leading cause of death in children under the age of five. Camel milk is indicated in this case because it contains anti-rotavirus antibodies (99). The RV treatment strategy in humans depends mainly on rehydration, adjunctive therapy and probiotics (100). There is mounting evidence that certain probiotics can be used as an adjuvant to rehydration therapy. Lactobacillus rhamnosus GG (LGG), given at a daily dose of 10 billion colony-forming units per day, has been shown to lessen the length of diarrhea in individuals with rotavirus gastroenteritis. In treating rotavirus gastroenteritis in young children, a nitazoxanide antibiotic demonstrating activity against anaerobic bacteria, protozoa, and viruses enabled considerable decreases in time to symptom resolution (100). Racecadotril has been used as an antidiarrheal drug, decreasing the gastric transit time of RV.
Other viruses, such as rabies, have been detected in camels (101). Antibodies against several other viruses were detected in the serum of camels without specific fatalities, such as those causing African horse sickness, parainfluenza, akabane, foot and mouth disease (type O), and rinderpest (101).
Camels have been subjects of special interest in multiple seroprevalence surveys to detect viral diseases (Table 1). Antibodies to RVFV and CMLV have been detected in camels in various African and Asian nations, highlighting the potential influence of the zoonotic role of animals in disease transmission. In 2021, BVDV and PPRV were recorded for the first time in alpacas in northern China (113). In general, camels have been found to have a lower incidence of BDVV than other species. Notably, the BVD virus was found to be more prevalent in camels bred in the presence of other animals than in camels raised alone in camel barns (116). This observation implies that camels have a decreased overall susceptibility or self-limited BVDV infection. BT has been a less common viral disease among camels, although it is widespread in other species in the survey areas in the survey areas (119). The unexpectedly high frequency of MERS-CoV infection in camels is not linked with obvious clinical symptoms with the presence of high antibody titers. Camels may, as a result, defend themselves from the closely similar SARS-CoV-2. There has been no evidence of SARS-CoV-2 infection in camels. Vaccine trials against MERS-CoV based on DNA (122) or poxvirus vector vaccine (123) have shown promising results in camels. The recently developed antiviral vaccines and their efficacy are briefly summarized in Table 2.
Table 1. Summary of screening surveys conducted to evaluate the seroprevalence of various viruses in camels.
Camels are the known reservoirs and carriers of multiple viruses. Trading camels and their products, such as milk and meat, has enhanced the risk of virus transmission to other animals and humans. Several research studies have identified deadly viruses in camels through serological testing. Diagnostic testing depends on the clinical symptoms and signs of the viral infection. There is a need for effective vaccines to minimize the spread of viral infection. Although researchers have investigated using viral vaccines in many animal species, less information is available for camels. We recommend that future studies focus on designing effective vaccines for camels. Moreover, as camels are also carriers of coronavirus strains, they can produce a strong immune response against recurring coronavirus infections. Camels can act as hosts for vaccine trials against viral infections because the vaccine will likely be easy to design and can stop the transmission of viruses from animals to humans.
All authors have shared in all aspects and read and agreed to the published version of the manuscript.
This work was supported by the Deanship of Scientific Research, Vice Presidency for Graduate Studies and Scientific Research, King Faisal University, Saudi Arabia (Project No. GRANT222).
The authors acknowledge the Deanship of Scientific Research at King Faisal University for the financial support.
The authors declare that the research was conducted in the absence of any commercial or financial relationships that could be construed as a potential conflict of interest.
All claims expressed in this article are solely those of the authors and do not necessarily represent those of their affiliated organizations, or those of the publisher, the editors and the reviewers. Any product that may be evaluated in this article, or claim that may be made by its manufacturer, is not guaranteed or endorsed by the publisher.
1. Schwartz H. Productive performance and productivity of dromedaries (Camelus dromedarius). Anim Res Dev. (1992) 35:86–9.
2. Köhler-Rollefson I. The Raika dromedary breeders of Rajasthan: a pastoral system in crisis. Nomad People. (1992) 1992:74–83.
3. Lensch J. The Two-Humped Camel (Camelus bactrianus). World Animal Review (FAO) Revue Mondiale de Zootechnie (FAO) Revista Mundial de Zootecnia (FAO). Rome: FAO (1999).
4. Tefera M, Gebreah F. A study on the productivity and diseases of camels in eastern Ethiopia. Trop Anim Health Prod. (2001) 33:265–74. doi: 10.1023/a:1010580416485
5. MacFarlane B, Pheasants M. Minor Use Animal Drug Program/NRSP-7 Fall Meeting 2010. Janesville, WI: MacFarlane Pheasants, Inc. (2010).
6. Bera B, Shanmugasundaram K, Barua S, Venkatesan G, Virmani N, Riyesh T, et al. Zoonotic cases of camelpox infection in India. Vet Microbiol. (2011) 152:29–38. doi: 10.1016/j.vetmic.2011.04.010
7. Salem E, Cook EA, Lbacha HA, Oliva J, Awoume F, Aplogan GL, et al. Serologic evidence for influenza C and D virus among ruminants and camelids, Africa, 1991–2015. Emerg Infect Dis. (2017) 23:1556. doi: 10.3201/eid2309.170342
8. Murakami S, Odagiri T, Melaku SK, Bazartseren B, Ishida H, Takenaka-Uema A, et al. Influenza D virus infection in dromedary camels, Ethiopia. Emerg Infect Dis. (2019) 25:1224. doi: 10.3201/eid2506.181158
9. Simmonds P, Becher P, Collett M, Gould E, Heinz F, Meyers G, et al. Family flaviviridae. Virus Taxonomy. (2011) 9:1003–20.
10. Gao S, Luo J, Du J, Lang Y, Cong G, Shao J, et al. Serological and molecular evidence for natural infection of Bactrian camels with multiple subgenotypes of bovine viral diarrhea virus in Western China. Vet Microbiol. (2013) 163:172–6. doi: 10.1016/j.vetmic.2012.12.015
11. Balamurugan V, Venkatesan G, Bhanuprakash V, Singh RK. Camelpox, an emerging orthopox viral disease. Indian J Virol. (2013) 24:295–305. doi: 10.1007/s13337-013-0145-0
12. Bhanuprakash V, Balamurugan V, Hosamani M, Venkatesan G, Chauhan B, Srinivasan V, et al. Isolation and characterization of Indian isolates of camel pox virus. Trop Anim Health Prod. (2010) 42:1271–5. doi: 10.1007/s11250-010-9560-z
13. Duraffour S, Meyer H, Andrei G, Snoeck R. Camelpox virus. Antiviral Res. (2011) 92:167–86. doi: 10.1016/j.antiviral.2011.09.003
14. Balamurugan V, Bhanuprakash V, Hosamani M, Jayappa KD, Venkatesan G, Chauhan B, et al. A polymerase chain reaction strategy for the diagnosis of camelpox. J Vet Diagnost Investig. (2009) 21:231–7. doi: 10.1177/104063870902100209
15. Zhugunissov K, Kilibayev S, Mambetaliyev M, Zakarya K, Kassenov M, Abduraimov Y, et al. Development and evaluation of a live attenuated egg-based camelpox vaccine. Front Vet Sci. (2021) 8:721023. doi: 10.3389/fvets.2021.721023
16. Tadesse T, Mulatu E, Bekuma A. Review on camel pox: an economically overwhelming disease of pastorals. Int J Adv Res Biol Sci. (2018) 5:65–73. doi: 10.22192/ijarbs.2018.05.09.006
17. Abdellatif M, Ibrahim A, Khalafalla A. Development and evaluation of a live attenuated camelpox vaccine from a local field isolate of the virus. Rev Sci Tech. (2014) 33:831–8. doi: 10.20506/rst.33.3.2321
18. Kachhawaha S, Srivastava M, Kachhawa JP, Tanwar M, Sharma A, Singh NK, et al. Therapeutic management of camel pox–a case report. Adv Anim Vet Sci. (2014) 2:239–41. doi: 10.14737/journal.aavs/2014/2.4.239.241
19. Gupta L, Tiwari G, Garg R. Documentation of ethnoveterinary remedies of camel diseases in rajasthan, India. (2015). Indian J Tradit Knowl. (2015) 447–53.
20. De Clercq E. Cidofovir in the treatment of poxvirus infections. Antiviral Res. (2002) 55:1–13. doi: 10.1016/S0166-3542(02)00008-6
21. Duraffour S, Snoeck R, Krecmerová M, van Den Oord J, De Vos R, Holy A, et al. Activities of several classes of acyclic nucleoside phosphonates against camelpox virus replication in different cell culture models. Antimicrob Agents Chemother. (2007) 51:4410–9. doi: 10.1128/AAC.00838-07
22. Khalafalla AI. Camel Contagious Ecthyma. Infectious Diseases of Dromedary Camels. Berlin: Springer (2021). p. 33–41.
23. Adedeji AJ, Gamawa AA, Chima NC, Ahmed AI. First report of camel contagious ecthyma in Nigeria. Open Vet J. (2018) 8:208–11. doi: 10.4314/ovj.v8i2.16
24. Dashtseren T, Solovyev BV, Varejka F, Khokhoo A. Camel contagious ecthyma (pustular dermatitis). Acta Virol. (1984) 28:122–7.
25. Ranjan R, Kashinath SDN, Sawal R. Therapeutic management of early acute camel contagious ecthyma (cce) in a camel herd. Intas Polivet. (2019) 20:453–6.
26. Khalafalla AI. Emerging Infectious Diseases in Camelids. Emerging and Re-emerging Infectious Diseases of Livestock. Berlin: Springer (2017). p. 425-41.
27. Abdallah MM, Adam IA, Abdalla TM, Abdelaziz SA, Ahmed ME, Aradaib IE, et al. Survey of rift valley fever and associated risk factors among the one-humped camel (Camelus dromedaries) in Sudan. Ir Vet J. (2015) 69:1–6. doi: 10.1186/s13620-016-0065-6
28. Anyamba A, Linthicum KJ, Small J, Britch SC, Pak E, de La Rocque S, et al. Prediction, assessment of the Rift Valley fever activity in East and Southern Africa 2006–2008 and possible vector control strategies. Am J Trop Med Hyg. (2010) 83:43. doi: 10.4269/ajtmh.2010.09-0289
29. Ikegami T, Hill TE, Smith JK, Zhang L, Juelich TL, Gong B, et al. Rift Valley fever virus MP-12 vaccine is fully attenuated by a combination of partial attenuations in the S, M, and L segments. J Virol. (2015) 89:7262–76. doi: 10.1128/JVI.00135-15
30. Faburay B, LaBeaud AD, McVey DS, Wilson WC, Richt JA. Current status of Rift Valley fever vaccine development. Vaccines. (2017) 5:29. doi: 10.3390/vaccines5030029
31. Johnson KN, Kalveram B, Smith JK, Zhang L, Juelich T, Atkins C, et al. Tilorone-dihydrochloride protects against Rift Valley fever virus infection and disease in the mouse model. Microorganisms. (2021) 10:10092. doi: 10.3390/microorganisms10010092
32. Scharton D, Bailey KW, Vest Z, Westover JB, Kumaki Y, Van Wettere A, et al. Favipiravir (T-705) protects against peracute Rift Valley fever virus infection and reduces delayed-onset neurologic disease observed with ribavirin treatment. Antiviral Res. (2014) 104:84–92. doi: 10.1016/j.antiviral.2014.01.016
33. Peters CJ, Reynolds JA, Slone TW, Jones DE, Stephen EL. Prophylaxis of Rift Valley fever with antiviral drugs, immune serum, an interferon inducer, and a macrophage activator. Antiviral Res. (1986) 6:285–97. doi: 10.1016/0166-3542(86)90024-0
34. Morrill JC, Jennings GB, Cosgriff TM, Gibbs PH, Peters CJ. Prevention of Rift Valley fever in rhesus monkeys with interferon-alpha. Rev Infect Dis. (1989) 11(Suppl.4):S815–25. doi: 10.1093/clinids/11.Supplement_4.S815
35. Narayanan A, Kehn-Hall K, Senina S, Lundberg L, Van Duyne R, Guendel I, et al. Curcumin inhibits Rift Valley fever virus replication in human cells. J Biol Chem. (2012) 287:33198–214. doi: 10.1074/jbc.M112.356535
36. Atkins C, Freiberg AN. Recent advances in the development of antiviral therapeutics for Rift Valley fever virus infection. Future Virol. (2017) 12:651–65. doi: 10.2217/fvl-2017-0060
37. Khalafalla AI. Peste Des Petits Ruminants (PPR). Infectious Diseases of Dromedary Camels. Berlin: Springer (2021). p. 57-63.
38. Munir M. Role of wild small ruminants in the epidemiology of peste des petits ruminants. Transbound Emerg Dis. (2014) 61:411–24. doi: 10.1111/tbed.12052
39. Omani RN. Molecular Characterization Of Peste Des Petits Ruminants In Camels, Sheep And Goats In Northern Kenya. Nairobi: UON (2018).
40. Hodgson S. The Immune Response to Live, Attenuated Peste Des Petits Ruminants Virus Vaccines. Guildford: University of Surrey (2020).
41. Balamurugan V, Hemadri D, Gajendragad M, Singh R, Rahman H. Diagnosis and control of peste des petits ruminants: a comprehensive review. Virusdisease. (2014) 25:39–56. doi: 10.1007/s13337-013-0188-2
42. Yousuf MA, Giasuddin M, Islam SS, Islam MR. Management of an outbreak of peste des petits ruminants with antibiotic combined hyperimmune serum therapy. Asian J Medical Biol Res. (2015) 1:230–4. doi: 10.3329/ajmbr.v1i2.25616
43. Ugochukwu ICI, Ugochukwu EI, Chukwu CC. Therapeutic response of West African dwarf goats infected with peste des petits ruminants whose oral lesions were treated with oxytetracycline long acting and gentian violet topical spray. Asian Pacific J Trop Dis. (2017) 7:771–4. doi: 10.12980/apjtd.7.2017D7-234
44. Afzal S, Raza S, Rabbani M, Firyal S, Altaf I, Naeem Z. Antiviral potential of ivermectin against peste des petits ruminants virus (PPRV). Pak J Zool. (2020) 53:1201–601. doi: 10.17582/journal.pjz/20200704060712
45. Byers SR. Bovine Viral Diarrhea Virus: Evaluation of Persistent Infections, Acute Transmission, and Vaccination Protection in Alpacas. (Doctoral dissertation, Washington State University, USA) (2009).
46. Agnew D. Camelidae. Pathology of Wildlife and Zoo Animals. Amsterdam: Elsevier (2018). p. 185-205.
47. van Amstel S, Kennedy M. Bovine viral diarrhea infections in new world camelids—a review. Small Ruminant Res. (2010) 91:121–6. doi: 10.1016/j.smallrumres.2010.03.018
48. Dubovi EJ. Laboratory diagnosis of bovine viral diarrhea virus. Biologicals. (2013) 41:8–13. doi: 10.1016/j.biologicals.2012.06.004
49. Kapil S, Yeary T, Evermann JF. Viral diseases of new world camelids. Vet Clin. (2009) 25:323–37. doi: 10.1016/j.cvfa.2009.03.005
50. Byers SR, Evermann JF, Bradway DS, Parish SM, Barrington GM. Evaluation of a commercial bovine viral diarrhea virus vaccine in nonpregnant female alpacas (Vicugna pacos). Vaccine. (2010) 28:591–3. doi: 10.1016/j.vaccine.2009.10.026
51. Walz PH, Chamorro MF, Falkenberg MS, Passler T, van der Meer F, Woolums RA. Bovine viral diarrhea virus: An updated American College of Veterinary Internal Medicine consensus statement with focus on virus biology, hosts, immunosuppression, and vaccination. J Vet Internal Med. (2020) 34:1690–706. doi: 10.1111/jvim.15816
52. Wernery U. Bovine Viral diarrhea-an emerging disease in camelids a review. Am J Virol. (2012) 1:9–17. doi: 10.3844/ajvsp.2012.9-17
53. Sentsui H, Takami R, Nishimori T, Murakami K, Yokoyama T, Yokomizo Y. Anti-viral effect of interferon-α on bovine viral diarrhea virus. J Vet Medical Sci. (1998) 60:1329–33. doi: 10.1292/jvms.60.1329
54. Zheng C, Pei T, Huang C, Chen X, Bai Y, Xue J, et al. A novel systems pharmacology platform to dissect action mechanisms of traditional Chinese medicines for bovine viral diarrhea disease. Eur J Pharm Sci. (2016) 94:33–45. doi: 10.1016/j.ejps.2016.05.018
55. Elmahi MM, Hussien MO, Karrar ARE, Elhassan AM, El Hussein ARM. Sero-epidemiological survey of bluetongue disease in one-humped camel (Camelus dromedarius) in Kassala State, Eastern Sudan. Ir Vet J. (2021) 74:1–7. doi: 10.1186/s13620-021-00186-2
56. Mellor PS, Carpenter S, Harrup L, Baylis M, Mertens PP. Bluetongue in Europe and the Mediterranean Basin: history of occurrence prior to 2006. Prev Vet Med. (2008) 87:4–20. doi: 10.1016/j.prevetmed.2008.06.002
57. Velthuis A, Saatkamp H, Mourits M, De Koeijer A, Elbers A. Financial consequences of the Dutch bluetongue serotype 8 epidemics of 2006 and 2007. Prev Vet Med. (2010) 93:294–304. doi: 10.1016/j.prevetmed.2009.11.007
58. Henrich M, Reinacher M, Hamann H. Lethal bluetongue virus infection in an alpaca. Vet Rec. (2007) 161:764. doi: 10.1136/vr.161.22.764
59. Ortega J, Crossley B, Dechant JE, Drew CP, MacLachlan NJ. Fatal bluetongue virus infection in an alpaca (Vicugna pacos) in California. J Vet Diagnost Investig. (2010) 22:134–6. doi: 10.1177/104063871002200129
60. Meyer G, Lacroux C, Leger S, Top S, Goyeau K, Deplanche M, et al. Lethal bluetongue virus serotype 1 infection in llamas. Emerg Infect Dis. (2009) 15:608–10. doi: 10.3201/eid1504.081514
61. Rojas JM, Rodríguez-Martín D, Martín V, Sevilla N. Diagnosing bluetongue virus in domestic ruminants: current perspectives. Vet Med. (2019) 10:17. doi: 10.2147/VMRR.S163804
62. Calvo-Pinilla E, Rodríguez-Calvo T, Sevilla N, Ortego J. Heterologous prime boost vaccination with DNA and recombinant modified vaccinia virus Ankara protects IFNAR (–/–) mice against lethal bluetongue infection. Vaccine. (2009) 28:437–45. doi: 10.1016/j.vaccine.2009.10.027
63. Marín-López A, Calvo-Pinilla E, Barriales D, Lorenzo G, Benavente J, Brun A, et al. Microspheres-prime/rMVA-boost vaccination enhances humoral and cellular immune response in IFNAR (–/–) mice conferring protection against serotypes 1 and 4 of bluetongue virus. Antiviral Res. (2017) 142:55–62. doi: 10.1016/j.antiviral.2017.03.010
64. Utrilla-Trigo S, Jiménez-Cabello L, Alonso-Ravelo R, Calvo-Pinilla E, Marín-López A, Moreno S, et al. Heterologous combination of ChAdOx1 and MVA vectors expressing protein NS1 as vaccination strategy to induce durable and cross-protective CD8+ T cell immunity to bluetongue virus. Vaccines. (2020) 8:346. doi: 10.3390/vaccines8030346
65. Zanolari P, Bruckner L, Fricker R, Kaufmann C, Mudry M, Griot C, et al. Humoral response to 2 inactivated bluetongue virus serotype-8 vaccines in South American Camelids. J Vet Internal Medi. (2010) 24:956–9. doi: 10.1111/j.1939-1676.2010.0538.x
66. Svehag SE, Leendertsen L, Gorham JR. Sensitivity of bluetongue virus to lipid solvents, trypsin and pH changes and its serological relationship to arboviruses. J Hygiene. (2009) 64:339–46. doi: 10.1017/s0022172400040614
67. Stelletta C, Cuteri V, Bonizzi L, Frangipane di Regalbono A, Orsi F, Nisoli L, et al. Effect of levamisole administration on bluetongue vaccination in sheep. Veterinaria Italiana. (2004) 40:635–9.
68. Albay MK, Sahinduran S, Kale M, Karakurum MÇ, Sezer K. Influence of tarantula cubensis extract on the treatment of the oral Lesions in Cattle With Bluetongue Disease. Kafkas Univ Vet Fak Derg. (2010) 16:593–6.
69. Zaki AM, Van Boheemen S, Bestebroer TM, Osterhaus AD, Fouchier RA. Isolation of a novel coronavirus from a man with pneumonia in Saudi Arabia. N Engl J Med. (2012) 367:1814–20. doi: 10.1056/NEJMoa1211721
70. Corman V, Müller MA, Costabel U, Timm J, Binger T, Meyer B, et al. Assays for laboratory confirmation of novel human coronavirus (hCoV-EMC) infections. Eurosurveillance. (2012) 17:20334. doi: 10.2807/ese.17.49.20334-en
71. Bermingham A, Chand M, Brown C, Aarons E, Tong C, Langrish C, et al. Severe respiratory illness caused by a novel coronavirus, in a patient transferred to the United Kingdom from the Middle East, September 2012. Eurosurveillance. (2012) 17:20290. doi: 10.2807/ese.17.40.20290-en
72. Azhar EI, El-Kafrawy SA, Farraj SA, Hassan AM, Al-Saeed MS, Hashem AM, et al. Evidence for camel-to-human transmission of MERS coronavirus. N Engl J Med. (2014) 370:2499–505. doi: 10.1056/NEJMoa1401505
73. Falzarano D, De Wit E, Rasmussen AL, Feldmann F, Okumura A, Scott DP, et al. Treatment with interferon-α2b and ribavirin improves outcome in MERS-CoV–infected rhesus macaques. Nat Med. (2013) 19:1313–7. doi: 10.1038/nm.3362
74. de Wit E, Feldmann F, Cronin J, Jordan R, Okumura A, Thomas T, et al. Prophylactic and therapeutic remdesivir (GS-5734) treatment in the rhesus macaque model of MERS-CoV infection. Proc Nat Acad Sci USA. (2020) 117:6771–6. doi: 10.1073/pnas.1922083117
75. Chan JF-W, Yao Y, Yeung M-L, Deng W, Bao L, Jia L, et al. Treatment with lopinavir/ritonavir or interferon-β1b improves outcome of MERS-CoV infection in a nonhuman primate model of common marmoset. J Infect Dis. (2015) 212:1904–13. doi: 10.1093/infdis/jiv392
76. Kandeel M, Yamamoto M, Park BK, Al-Taher A, Watanabe A, Gohda J, et al. Discovery of new potent anti-MERS CoV fusion inhibitors. Front Pharmacol. (2021) 12:1241. doi: 10.3389/fphar.2021.685161
77. Mahmoud Kandeel Elsayed AYA-T, Kwon HJ, Al-Nazawi M. MERS-CoV Inhibitor Peptides. United States Patent Office, Patent no US10975126B1. (2021).
78. Kandeel M, Yamamoto M, Al-Taher A, Watanabe A, Oh-Hashi K, Park BK, et al. Small molecule inhibitors of Middle East respiratory syndrome coronavirus fusion by targeting cavities on heptad repeat trimers. Biomol Therapeut. (2020) 28:311–9. doi: 10.4062/biomolther.2019.202
79. Kandeel M, Park BK, Morsy MA, Venugopala KN, Oh-hashi K, Al-Nazawi M, et al. Virtual screening and inhibition of Middle East respiratory syndrome coronavirus replication by targeting papain-like protease. Sulaiman Al Habib Medical J. (2021) 3:179–87. doi: 10.2991/dsahmj.k.210921.001
80. Momattin H, Al-Ali AY, Al-Tawfiq JA, A. Systematic Review of therapeutic agents for the treatment of the Middle East Respiratory Syndrome Coronavirus (MERS-CoV). Travel Med Infect Dis. (2019) 30:9–18. doi: 10.1016/j.tmaid.2019.06.012
81. Al-Tawfiq JA, Momattin H, Dib J, Memish ZA. Ribavirin and interferon therapy in patients infected with the Middle East respiratory syndrome coronavirus: an observational study. Int J Infect Dis. (2014) 20:42–6. doi: 10.1016/j.ijid.2013.12.003
82. Kim UJ, Won E-J, Kee S-J, Jung S-I, Jang H-C. Combination therapy with lopinavir/ritonavir, ribavirin and interferon-α for Middle East respiratory syndrome. Antivir Ther. (2016) 21:455–9. doi: 10.3851/IMP3002
83. Al Ghamdi M, Alghamdi KM, Ghandoora Y, Alzahrani A, Salah F, Alsulami A, et al. Treatment outcomes for patients with Middle Eastern Respiratory Syndrome Coronavirus (MERS CoV) infection at a coronavirus referral center in the Kingdom of Saudi Arabia. BMC Infect Dis. (2016) 16:174. doi: 10.1186/s12879-016-1492-4
84. Wu F, Zhao S, Yu B, Chen Y-M, Wang W, Song Z-G, et al. A new coronavirus associated with human respiratory disease in China. Nature. (2020) 579:265–9. doi: 10.1038/s41586-020-2008-3
85. Memish ZA, Cotten M, Meyer B, Watson SJ, Alsahafi AJ, Al Rabeeah AA, et al. Human infection with MERS coronavirus after exposure to infected camels, Saudi Arabia, 2013. Emerg Infect Dis. (2014) 20:1012. doi: 10.3201/eid2006.140402
86. Hamers-Casterman C, Atarhouch T, Muyldermans S, Robinson G, Hammers C, Songa EB, et al. Naturally occurring antibodies devoid of light chains. Nature. (1993) 363:446–8. doi: 10.1038/363446a0
87. Chouchane L, Grivel J-C, Abd Farag EAB, Pavlovski I, Maacha S, Sathappan A, et al. Dromedary camels as a natural source of neutralizing nanobodies against SARS-CoV-2. JCI Insight. (2021) 6:145785. doi: 10.1172/jci.insight.145785
88. Cortez-Retamozo V, Lauwereys M, Hassanzadeh Gh G, Gobert M, Conrath K, Muyldermans S, et al. Efficient tumor targeting by single-domain antibody fragments of camels. Int J Cancer. (2002) 98:456–62. doi: 10.1002/ijc.10212
89. Hasson SS, Al-Jabri AA. Immunized camels and COVID-19. Asian Pac J Trop Med. (2020) 13:239. doi: 10.4103/1995-7645.282561
90. Jasion VS, Burnett BP. Survival and digestibility of orally-administered immunoglobulin preparations containing IgG through the gastrointestinal tract in humans. Nutr J. (2015) 14:1–8. doi: 10.1186/s12937-015-0010-7
91. Gai J, Ma L, Li G, Zhu M, Qiao P, Li X, et al. A potent neutralizing nanobody against SARS-CoV-2 with inhaled delivery potential. bioRxiv. (2020) 2020:2020.08.09.242867. doi: 10.1002/mco2.60
92. Esparza TJ, Martin NP, Anderson GP, Goldman ER, Brody DL. High affinity nanobodies block SARS-CoV-2 spike receptor binding domain interaction with human angiotensin converting enzyme. bioRxiv. (2020) 2020:2020.07.24.219857. doi: 10.1038/s41598-020-79036-0
93. Papp H, Malik YS, Farkas SL, Jakab F, Martella V, Bányai K. Rotavirus strains in neglected animal species including lambs, goats and camelids. VirusDisease. (2014) 25:215–22. doi: 10.1007/s13337-014-0203-2
94. Jere KC, Esona MD, Ali YH, Peenze I, Roy S, Bowen MD, et al. Novel NSP1 genotype characterised in an African camel G8P[11] rotavirus strain. Infect Genet Evol. (2014) 21:58–66. doi: 10.1016/j.meegid.2013.10.002
95. Ali Y, Khalafalla A, El Amin M. Epidemiology of camel calf diarrhoea in Sudan: seroprevalence of camel rotavirus infection. J Anim Vet Adv. (2005) 4:393–7.
96. El-Sabagh IM, Al-Ali AM, Al-Naeem AA. Group A rotavirus and Mycobacterium avium subspecies paratuberculosis associated with diarrhea in dromedary camels in Eastern province, Saudi Arabia. Thai J Vet Med. (2017) 47:383.
97. Ali YH, Khalafalla A, Gaffar M, Peenze I, Steele A. Detection and isolation of group A rotavirus from camel calves in Sudan. Vet Arhiv. (2008) 78:477–85.
98. Saif LJ, Fernandez FM. Group A rotavirus veterinary vaccines. J Infect Dis. (1996) 174(Suppl.1):S98–106.
100. Dennehy PH. Treatment and prevention of rotavirus infection in children. Curr Infect Dis Rep. (2013) 15:242–50. doi: 10.1007/s11908-013-0333-5
101. Abbas B, Omer O. Review of infectious diseases of the camel. Veterinary Bulletin. (2005) 75:1–16.
102. Adamu AM, Allam L, Sackey AK, Nma AB, Mshelbwala PP, Mambula-Machunga S, et al. Risk factors for Rift Valley fever virus seropositivity in one-humped camels (Camelus dromedarius) and pastoralist knowledge and practices in Northern Nigeria. One Health. (2021) 13:100340. doi: 10.1016/j.onehlt.2021.100340
103. Muturi M, Akoko J, Nthiwa D, Chege B, Nyamota R, Mutiiria M, et al. Serological evidence of single and mixed infections of Rift Valley fever virus, Brucella spp. and Coxiella burnetii in dromedary camels in Kenya. PLoS Negl Trop Dis. (2021) 15:e0009275. doi: 10.1371/journal.pntd.0009275
104. Cosseddu GM, Doumbia B, Scacchia M, Pinoni C, Di Provvido A, Polci A, et al. Sero-surveillance of emerging viral diseases in camels and cattle in Nouakchott, Mauritania: an abattoir study. Trop Anim Health Prod. (2021) 53:1–6. doi: 10.1007/s11250-021-02636-z
105. Kalthoum S, Arsevska E, Guesmi K, Mamlouk A, Cherni J, Gharbi R, et al. Risk based serological survey of Rift Valley fever in Tunisia (2017–2018). Heliyon. (2021) 7:e07932. doi: 10.1016/j.heliyon.2021.e07932
106. Musa HI, Kudi CA, Gashua MM, Muhammad AS, Tijjani AO, Saidu AS, et al. Survey of antibodies to Rift Valley fever virus and associated risk factors in one-humped camels (Camelus dromedarius) slaughtered in Maiduguri abattoir, Borno State, Nigeria. Trop Anim Health Prod. (2021) 53:1–8. doi: 10.1007/s11250-021-02956-0
107. Yousif A, Al-Naeem A. Recovery and molecular characterization of live Camelpox virus from skin 12 months after onset of clinical signs reveals possible mechanism of virus persistence in herds. Vet Microbiol. (2012) 159:320–6. doi: 10.1016/j.vetmic.2012.04.022
108. Khalafalla AI, Abdelazim F. Human and dromedary camel infection with camelpox virus in Eastern Sudan. Vector-Borne Zoonotic Dis. (2017) 17:281–4. doi: 10.1089/vbz.2016.2070
109. Dahiya SS, Kumar S, Mehta SC, Singh R, Nath K, Narnaware SD, et al. Molecular characterization of camelpox virus isolates from Bikaner, India: evidence of its endemicity. Acta Trop. (2017) 171:1–5. doi: 10.1016/j.actatropica.2017.03.011
110. Joseph S, Kinne J, Nagy P, Juhász J, Barua R, Patteril NAG, et al. Outbreak of a systemic form of camelpox in a dromedary herd (Camelus dromedarius) in the United Arab Emirates. Viruses. (2021) 13:1940. doi: 10.3390/v13101940
111. Nafea M, Elbakry M, Shahein M, Gamelat K, Farag G, Abdallah F, et al. Virological and molecular studies on peste des petits ruminants virus (PPRV) in small ruminants and camel in Egypt between 2017 and 2018. Adv Anim Vet Sci. (2019) 7:12. doi: 10.17582/journal.aavs/2019/7.s2.12.18
112. Fakri F-Z, Bamouh Z, Jazouli M, Tadlaoui KO, Elharrak M. Experimental infection of dromedary camels with virulent virus of Peste des Petits Ruminants. Vet Microbiol. (2019) 235:195–8. doi: 10.1016/j.vetmic.2019.07.004
113. Liu Q, Liu L, Meng Y-K, Wang C, Gao Y, Zheng F-G, et al. Serological evidence of bovine viral diarrhea virus and peste des petits ruminants virus infection in alpacas (Vicugna pacos) in Shanxi Province, northern China. Trop Anim Health Prod. (2021) 53:1–5. doi: 10.1007/s11250-021-02746-8
114. Ali MAB. Detection of Peste des Petits Ruminants Virus Antigen in Pneumonic Lungs from Camels at Al Sahafa Slaughterhouse, Khartoum State. Khartoum: Sudan University of Science and Technology (2021).
115. Tesfaye A, Omer A, Hussein A, Garoma A, Guyassa C, Paeshuyse J, et al. Seroprevalence of bovine viral diarrhea virus in local Borana cattle breed and camels (Camelus dromedarius) in Ethiopia. Vet Med. (2021) 12:141. doi: 10.2147/VMRR.S305198
116. Ataseven VS, Gürel K, Pestil Z, Ambarcioglu P, Dogan F, Kayhanlar M, et al. BHV-1 and BLV antibodies in dromedary camels of Turkey kept without and with ruminants. Trop Anim Health Prod. (2022) 54:1–6. doi: 10.1007/s11250-021-03030-5
117. El Bahgy HE, Abdelmegeed HK, Marawan MA. Epidemiological surveillance of bovine viral diarrhea and rift valley fever infections in camel. Vet World. (2018) 11:1331. doi: 10.14202/vetworld.2018.1331-1337
118. Abraheem HH, Elhassan AM, Hussien MO, Enan KA, Musa AB, El Hussein ARM. A survey of bluetongue infection and associated risk factors among the one-humped camel (Camelus dromedaries) in Gadarif State, Eastern Sudan. Vet Med Int. (2021) 2021:6613217. doi: 10.1155/2021/6613217
119. Yousef MR, Al-Eesa AA, Al-Blowi MH. High seroprevalence of bluetongue virus antibodies in Sheep, Goats, Cattle and Camel in different districts of Saudi Arabia. Vet World. (2012) 5:393. doi: 10.5455/vetworld.2012.389-393
120. Zohaib A, Saqib M, Athar MA, Chen J, Sial A-u-R, Khan S, et al. Countrywide survey for MERS-coronavirus antibodies in dromedaries and humans in Pakistan. Virologica Sinica. (2018) 33:410–7. doi: 10.1007/s12250-018-0051-0
121. Lau SK, Li KS, Luk HK, He Z, Teng JL, Yuen K-Y, et al. Middle east respiratory syndrome coronavirus antibodies in bactrian and hybrid camels from Dubai. Msphere. (2020) 5:e00898–19. doi: 10.1128/mSphere.00898-19
122. Muthumani K, Falzarano D, Reuschel EL, Tingey C, Flingai S, Villarreal DO, et al. A synthetic consensus anti–spike protein DNA vaccine induces protective immunity against Middle East respiratory syndrome coronavirus in nonhuman primates. Sci Transl Med. (2015) 7:301ra132. doi: 10.1126/scitranslmed.aac7462
123. Haagmans BL, Van Den Brand JM, Raj VS, Volz A, Wohlsein P, Smits SL, et al. An orthopoxvirus-based vaccine reduces virus excretion after MERS-CoV infection in dromedary camels. Science. (2016) 351:77–81. doi: 10.1126/science.aad1283
124. Warimwe GM, Gesharisha J, Carr BV, Otieno S, Otingah K, Wright D, et al. Chimpanzee adenovirus vaccine provides multispecies protection against Rift Valley fever. Sci Rep. (2016) 6:1–7. doi: 10.1038/srep20617
125. Daouam S, Ghzal F, Naouli Y, Tadlaoui K, Ennaji M, Oura C, et al. Safety and immunogenecity of a live attenuated Rift Valley fever vaccine (CL13T) in camels. BMC Vet Res. (2016) 12:1–5. doi: 10.1186/s12917-016-0775-8
126. Anthony T, van Schalkwyk A, Romito M, Odendaal L, Clift SJ, Davis AS. Vaccination with Rift Valley fever virus live attenuated vaccine strain Smithburn caused meningoencephalitis in alpacas. J Vet Diagnost Investig. (2021) 33:777–81. doi: 10.1177/10406387211015294
127. Berhe G, Minet C, Le Goff C, Barrett T, Ngangnou A, Grillet C, et al. Development of a dual recombinant vaccine to protect small ruminants against peste-des-petits-ruminants virus and capripoxvirus infections. J Virol. (2003) 77:1571–7. doi: 10.1128/JVI.77.2.1571-1577.2003
Keywords: viral diseases, camel, vaccine, drug, control
Citation: Kandeel M and Al-Mubarak AIA (2022) Camel viral diseases: Current diagnostic, therapeutic, and preventive strategies. Front. Vet. Sci. 9:915475. doi: 10.3389/fvets.2022.915475
Received: 08 April 2022; Accepted: 25 July 2022;
Published: 11 August 2022.
Edited by:
Rajeev Ranjan, International Centre for Foot and Mouth Disease (ICAR), IndiaReviewed by:
Muhammad Muddassir Ali, University of Veterinary and Animal Sciences, PakistanCopyright © 2022 Kandeel and Al-Mubarak. This is an open-access article distributed under the terms of the Creative Commons Attribution License (CC BY). The use, distribution or reproduction in other forums is permitted, provided the original author(s) and the copyright owner(s) are credited and that the original publication in this journal is cited, in accordance with accepted academic practice. No use, distribution or reproduction is permitted which does not comply with these terms.
*Correspondence: Mahmoud Kandeel, bWthbmRlZWxAa2Z1LmVkdS5zYQ==
Disclaimer: All claims expressed in this article are solely those of the authors and do not necessarily represent those of their affiliated organizations, or those of the publisher, the editors and the reviewers. Any product that may be evaluated in this article or claim that may be made by its manufacturer is not guaranteed or endorsed by the publisher.
Research integrity at Frontiers
Learn more about the work of our research integrity team to safeguard the quality of each article we publish.