- Department of Clinical Sciences, Faculty of Veterinary Medicine, Utrecht University, Utrecht, Netherlands
In many species, the insulin-like growth factors (IGF1 and IGF2), their receptors and IGF binding proteins play important roles in preparing the endometrium for implantation, and regulating conceptus growth and development. To determine whether the IGF system may contribute to conceptus-maternal interaction during equine pre-implantation development, we evaluated mRNA expression for IGF system components in conceptuses, and endometrium recovered from pregnant and cycling mares, on days 7, 14, 21 and 28 after ovulation. We also investigated expression of IGF1, IGF2 and their receptors 6 and 11 days after transfer of day 8 embryos to synchronous (day 8) or asynchronous (day 3) recipient mares. Expression of IGF1 and IGF2, IGF1R, IGF2R, INSR and IGFBPs 1, 2, 4 and 5 was evident in endometrium and conceptus membranes during days 7–28. Endometrial IGF2, INSR, IGFBP1 and IGFBP2 expression increased between days 7 and 28 of pregnancy. In conceptus membranes, expression of all IGF system components increased with developmental stage. Immunohistochemistry revealed strong expression of IGF1, IGF2 and IGF1R in both endometrium and conceptus membranes, whereas INSR was highly expressed in endometrium but barely detectable in the conceptus. Finally, a negatively asynchronous uterine environment retarded IGF1, IGF2 and INSR expression in the conceptus, whereas in the endometrium only INSR expression was altered by asynchrony. The presence of IGFs, their receptors and IGFBPs in the endometrium and conceptus during early equine pregnancy, and down-regulation in the conceptus following asynchronous embryo transfer, suggest a role in conceptus-maternal communication during the preparation for implantation.
Introduction
The insulin-like growth factor (IGF) system is thought to play important roles in endometrial preparation for successful implantation, and in embryo and placental growth and development. The IGF system comprises two principal growth factors, IGF1 and IGF2, which regulate growth, cell differentiation, migration and proliferation, and inhibit apoptosis via paracrine and autocrine pathways (1). The action of the IGFs is mediated by the IGF receptor type 1 (IGF1R) and the insulin receptor (INSR), whereas the IGF receptor type 2 (IGF2R) regulates IGF2 availability by internalization and degradation (1, 2). In murine pregnancy, deletion of any of IGF1, IGF2 or IGF1R leads to fetal growth retardation and reduced placental size (3–5), and IGF1 has been proposed to regulate placental nutrient exchange (5, 6). The IGFs also have high affinity for the IGF binding proteins 1–6 (IGFBP1–6) which regulate IGF1 and IGF2 availability by binding to them in a way that extends their circulatory half-life, either by sequestration or by regulating their release to the receptors in target tissues (1, 7). In the endometrium of women (4, 5, 8), rodents (3) and ruminants (9–13), all members of the IGF system are expressed, and the system is thought to stimulate endometrial cell differentiation and thereby aid preparation for implantation (8, 14).
Expression of some IGF family members has been studied during early equine pregnancy. IGF2 mRNA expression has been reported in equine placental membranes and the embryo/fetus between days 20 and 150 of gestation, having first appeared in the vascularized extra-embryonic mesoderm of the day 20 conceptus (15); the authors speculated that IGF2 might help promote invasiveness of the trophoblast cells in the chorionic girdle, and be involved with more general development of the placenta. Furthermore, IGF2 is an imprinted gene known to be paternally expressed in equine trophoblast, and therefore expected to promote conceptus growth (16). Reports of IGF2 expression in the endometrium are contradictory, in that an early study failed to detect IGF2 during the first half of pregnancy (15) whereas a subsequent article reported expression of IGF2 mRNA from day 11 of gestation (17). IGF1 has been reported to be secreted by both equine endometrium and the conceptus and is detectable in uterine luminal fluids (ULF), the endometrium and the blastocoel cavity (18). From as early as day 8 of pregnancy, IGF1 mRNA is expressed in the endometrium and the conceptus (17, 19), while the protein has been detected in the trophectoderm and endoderm of day 12 conceptuses (19) as well as in the placental microcotyledons during the second half of gestation (20). Although IGF1 expression by endometrium and/or conceptus was not affected by treatment with the progestogen, altrenogest, in vivo (17) or by estradiol 17β (E2) in vitro (18), the high concentrations of IGF1 detected during early equine pregnancy suggest that it may have a function during this period. Arai et al. (20) suggested that IGF1 may be involved in placentation because IGF1 expression in the microcotyledons increased at the time of chorioallantoic-endometrial interdigitation. Recently, transcriptomic studies have indicated that mRNA for other members of the IGF system are expressed in early equine conceptus membranes and pregnant endometrium. In the endometrium, IGFBPs 1-3 were up-regulated on day 12 compared to day 8 of pregnancy (21), and IGFBP1 expression was higher on day 13.5 of pregnancy than the equivalent day of the estrous cycle (22). In the conceptus, IGF1R and IGF2R were up-regulated between days 8 and 12 of pregnancy and IGFBP 2, 4 and 6 expression increased between days 8 and 14 (23). IGF2R, a maternally expressed imprinted gene, is expressed in equine trophoblast (16) and detected in blastocoel fluid and culture medium conditioned by day 9 and 10 equine conceptuses (24). Soluble IGF2R is an important carrier of IGF2 and mannose 6-P proteins during pregnancy, and serves as an antagonist to the cellular receptor by opposing the growth stimulating effects of IGF2 (25).
Equine pregnancy is characterized by a long pre-implantation period, with fixation of the conceptus occurring at around day 16–17 after ovulation, but true interdigitation between the chorion and the endometrial epithelium only starting at around day 40 (26). Another interesting characteristic of horse pregnancy is the ability of the equine embryo to tolerate considerable negative uterine asynchrony (i.e., being transferred to the uterus of a recipient that ovulated after the donor mare). Although increasing the degree of negative asynchrony up to 5 days does not seem to interfere with the establishment and maintenance of pregnancy, it causes a delay in subsequent conceptus development that is visible at macroscopic, transcriptomic, cellular and tissue levels (27–29). This makes asynchronous ET an interesting model for examining factors proposed to be involved in conceptus-maternal dialogue during the establishment of pregnancy. While P4 secreted by the corpus luteum is not the only factor responsible for stimulating the endometrium to prepare for its roles in implantation and support of conceptus development (30), it is one of the most important. Following negatively asynchronous embryo transfer, the duration of endometrial exposure to P4 is shorter than following synchronous transfer, for any given embryonic stage. While this abbreviated period of endometrial P4 priming undoubtedly contributes to subsequent retarded conceptus development, it is not yet clear how the conceptus senses the stage of the endometrium and consequently adapts its development, or which factors or signals regulate this interaction (29). However, we recently found that asynchronous transfer results in consistently higher expression of KNG1 and IGFBP3 in the endometrium of synchronous compared to asynchronous pregnancies on days 14 and 19 of embryo development, suggesting a role for both in matching conceptus development to uterine stage (31).
Given the importance of the IGF system for regulating conceptus development and preparing the endometrium for implantation in a range of mammalian species, we hypothesized that the IGFs, their receptors and binding proteins would be involved in pre-implantation equine conceptus-maternal interaction and play a role in the growth retardation evident after transferring embryos to an asynchronous uterus. To investigate these hypotheses, we first examined the expression of members of the IGF system in the embryo/conceptus and endometrium from pregnant and cycling mares. Next, we examined changes in expression of IGF system components after transfer of day 8 embryos into the uterus of synchronous (day 8) or negatively asynchronous (day 3) recipient mares.
Materials and methods
Animals
Animal procedures were approved by Utrecht University's Animal Experimentation Committee (permits 2007.III.02.036 and 2012.III.02.020). Material was recovered from two groups of 18 (study 1) and 22 (study 2) warmblood mares, aged 4–15 years and maintained on pasture. When the mares were in late estrus, follicle development was monitored daily transrectally using an ultrasound scanner equipped with a 7.5-MHz transducer (MyLab30Vet; Esaote, Maastricht, The Netherlands). When pregnancy was required, estrous mares with a dominant follicle ≥35 mm were inseminated with ≥500 x 106 sperm cells from a fertile stallion, and ovulation was confirmed within 48 h of insemination in all mares. Non-pregnant mares required as embryo recipients or for endometrial biopsy, were monitored identically, but were not inseminated. For ET, the desired degree of (a)synchrony between donor and recipient mares was ensured by using exogenous hormones to induce luteolysis (PGF2α analog: 37.5 mg D-cloprostenol; Genestranvet: Eurovet Animal Health B.V., Bladel, The Netherlands) and ovulation (hCG: 1500 IU Chorulon; Intervet, Boxmeer, The Netherlands). Day 7 or 8 pregnancy was confirmed by blastocyst recovery during uterine lavage, whereas later conceptus vesicle presence was detected, and development monitored, by transrectal ultrasonography.
Study design and tissue collection
Study 1 involved investigation of mRNA expression for IGF1, IGF2, IGF1R, IGF2R, INSR, IGFBPs 1, 2, 4 and 5, and of protein expression for IGF1, IGF2, IGF1R and INSR in conceptus membranes and endometrium collected during early pregnancy (days 7, 14, 21 and 28) and across the estrous cycle (days 7, 14 and 21 after ovulation). Despite numerous attempts, we were unable to validate primers to investigate IGFBP3 expression. On days 14, 21 and 28 of gestation, conceptuses (n = 4 per group) were collected using an endoscopically-guided net, after puncture of the membranes and aspiration of the conceptus fluid with a sharpened PTFE catheter, as described previously (32). Endometrial biopsies were recovered from the base of a uterine horn using uterine biopsy forceps on days 7, 14 and 21 after ovulation in cycling mares, and days 7 and 14 in pregnant mares; on days 21 and 28 of pregnancy, the biopsy was recovered from the site of conceptus apposition (visible as a hyperaemic area adjacent to the punctured membranes) under endoscopic guidance (n = 4 per group).
Study 2 investigated the effect of asynchronous ET on endometrial and conceptus expression of IGF1, IGF2, IGF1R, IGF2R and INSR. Twenty day 8 blastocysts were collected from donor mares by uterine lavage and transferred to synchronous (day 8; ovulated on the same day as the donor) or asynchronous (day 3; ovulated 5 days after the donor) recipient mares as described previously (29, 31). Resulting conceptuses (n = 5 per group) were recovered 6 or 11 days after ET (day 14 or 19 of conceptus development), and an endometrial biopsy (n = 5 per group) was recovered from the base of a uterine horn (day 6 after ET) or at the site of conceptus apposition (day 11 after ET).
After recovery and washing in 0.9 % NaCl, the embryonic disc (day 14) or embryo proper (day 19 and 21) were dissected from the membranes (yolk sac) using a stereomicroscope (Olympus SZ-ST; Olympus, Tokyo, Japan); for day 28 conceptuses, the embryo proper, yolk sac (YS) and allantochorion (AC) were separated. All tissues were then divided; one piece was snap-frozen in liquid nitrogen and stored at −80°C prior to RNA extraction, while the other piece was fixed overnight in 4% paraformaldehyde before embedding in paraffin in preparation for immunohistochemistry.
RNA extraction and cDNA synthesis
Total RNA was extracted using the AllPrep DNA/RNA/Protein Mini kit following the manufacturers' instructions (Qiagen, Venlo, The Netherlands). Samples (30 mg) were homogenized in 600 μl lysis buffer and RNA was eluted with 40 μl RNAse-free water. cDNA synthesis was performed on 1 μg of DNASE I treated RNA (37°C and 10 min at 65°C; 1 IU/μg; RNase-free DNase kit; Qiagen) using Superscript III (Invitrogen, Landsmeer, The Netherlands) in a final volume of 20 μl.
Quantitative PCR
Quantitative PCR was performed as described previously (29). Briefly, primer pairs were designed using PerlPrimer 1.1. (http://perlprimer.sourceforge.net/) the product specificity of the primer pairs (Eurogentec: Seraing, Belgium; Supp. Table 1) was assessed by DNA sequencing (ABI PRISM 310 Genetic analyzer; Applied Biosystem, Foster City, USA). For each gene, a 10-fold serial dilution of the target gene PCR product, of known quantity, was amplified simultaneously with the samples to produce a standard curve and thereby allow subsequent quantification. PCR was carried out in 15 μl reaction mixture containing 1 μl sample cDNA and iQ SYBR® Green Supermix, on an IQ5 real-time PCR system (Bio-Rad Laboratories; Veenendaal, The Netherlands). Cycle conditions were: 3 min denaturation at 95°C, followed by 40 cycles of amplification (15 s at 95°C, 30 s at the primer-specific annealing temperature and 30 s at 72°C). This was followed by 1 min at 95°C, 1 min at 55°C, and finished with a melting curve. Product specificity was evaluated by assessing the melting curve using iQ5 software, and starting quantities were quantified using the standard curve. Relative gene expression was calculated as the ratio of target gene mean expression to the geometric mean for the housekeeping genes selected for the specific tissue and experiment from 4 candidates (GAPDH, PGK1, HPRT1 and SRP14), after stability evaluation using GeNorm (33). Potential housekeeping genes had to have a stability value (M) below 0.5. The 4 genes were ranked in terms of the lowest M value and the 2 most stable genes were selected for normalization; additional genes were included in a stepwise fashion as long as overall stability was not affected. For endometrium in study 1 and conceptus membranes in study 2, the combination of GADPH, PGK1 and SRP14 was found to be optimal for normalization, whereas for conceptus membranes in study 1 and endometrium in study 2 GAPDH, PGK1, HRPT1 and SRP14 were selected.
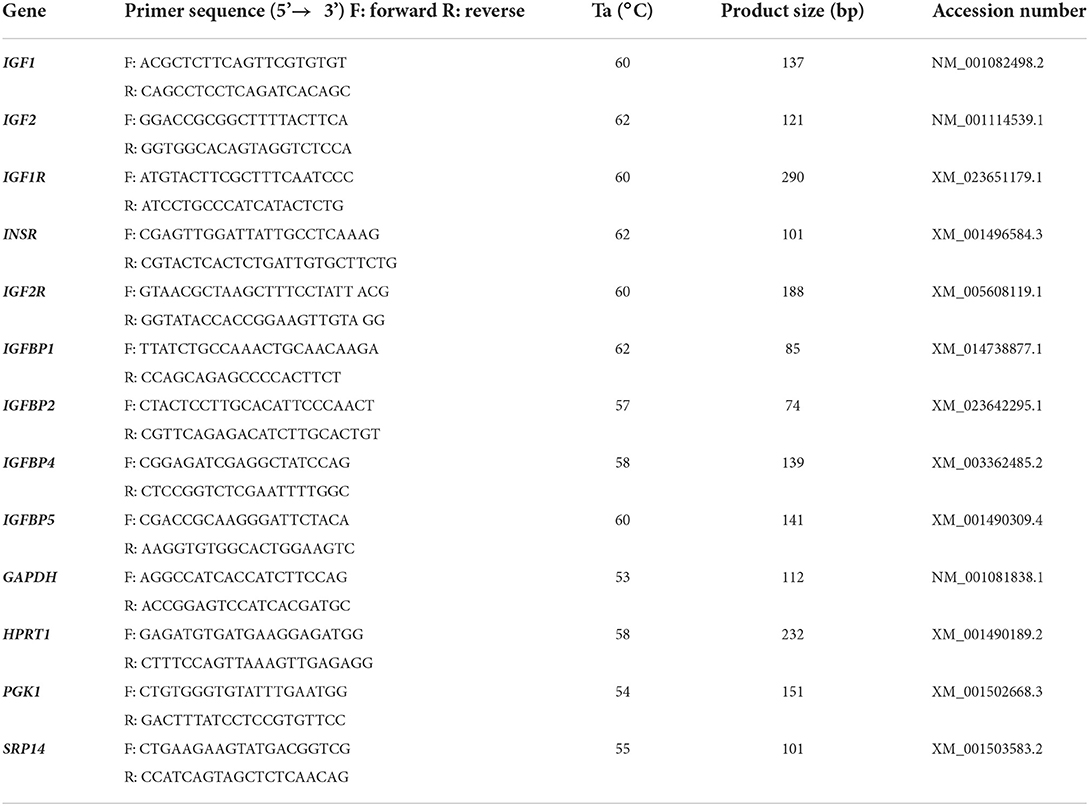
Table 1. Primer pairs for equine IGF system components; gene symbol, primer sequence, annealing temperature, product size and accession number of genes used for qRT-PCR analysis.
Immunohistochemistry
Immunohistochemistry was performed as described previously (34). Briefly, 5 μm sections cut from paraffin-embedded tissue were mounted on SuperFrost® Plus slides (VWR International, Leuven, Belgium). After the deparaffinization and rehydration steps, a 1% H2O2 solution in methanol was used to block endogenous peroxidase activity. Antigen retrieval was performed by microwaving (750 W) the sections for 15 min, in pre-heated Tris/EDTA-buffer for IGF1, IGF1R and IGF2 (0.01 M Tris; 0.001 M EDTA; 0.05% Tween-20; pH 9.0), or with citrate buffer for INSR (10 mM citric acid; pH 6.0). Slides were cooled to room temperature (RT) over 30 min and rinsed in PBS-Tween (PBST; 0.05% Tween-20 in PBS: 3 x 5 min). To block non-specific binding, the sections were incubated for 15 min with goat serum, for IGF1, IGF1R and INSR (1:10 in PBS), or rabbit serum for IGF2 (1:10 in PBS). Next, sections were incubated at 4°C overnight with the specific antibody (Table 2) and, after rinsing with PBST, sections were incubated with the second antibody diluted 1:250 for 30 min at RT (Table 2). Sections were then incubated with Avidin-Biotin-Complex (ABC)-peroxidase for 30 min (Vectastain® ABC Kit, PK-4000; Vector Laboratories), followed by a 10 min incubation in freshly prepared 3,3'-diaminobenzidine tetrahydrochloride (45 ml 0.05 M Tris/HCl, pH 7.6; 5 ml DAB and 5 μl H2O2), and counterstained with hematoxylin for 30 s. Finally, the sections were dehydrated and mounted under a coverslip with Eukitt™ Mounting Medium (Electron Microscopy Systems, Hatfield, USA). Imaging was performed using an Olympus BX60 microscope (Olympus Nederland, Leiderdorp, the Netherlands) coupled to a digital camera (Leica DFC425C: Leica Microsystems, Wetzlar, Germany) and Leica LAS-AF software. For negative controls, the primary antibody was substituted with purified immunoglobulin (IgG; Table 2).
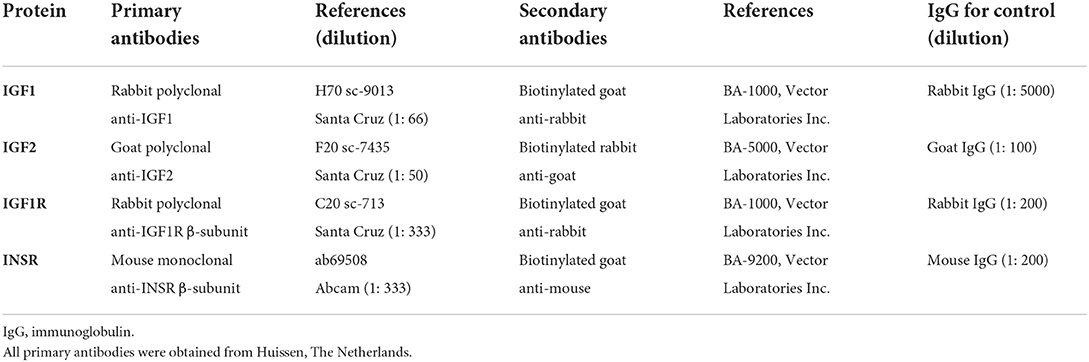
Table 2. List of antibodies and IgGs used for immunohistochemistry of equine endometrium and conceptus membranes.
Statistical analysis
Data were analyzed using SPSS 20 for Windows (SPSS Inc., Chicago, IL, USA). QRT-PCR data was log transformed to yield normally distributed data sets. Endometrial gene expression was then analyzed by two-way ANOVA, followed by post-hoc Tukey testing. If a significant interaction between pregnancy status and stage of pregnancy/cycle was apparent, data were sub-divided according to status or stage and reanalyzed by one-way ANOVA. Conceptus membrane gene expression was analyzed by one-way ANOVA with post-hoc Tukey testing. Data from the ET study was analyzed by two-way ANOVA to investigate effects of pregnancy stage and synchrony; significant effects were verified by independent T-tests. Statistical significance was assumed when P < 0.05.
Results
Gene expression for IGF system components in the endometrium of cycling and pregnant mares
IGF1 and IGF2R expression did not vary significantly across the estrous cycle and early pregnancy (Figure 1). Expression of IGF2 did not change markedly during the cycle, but in pregnant animals increased in two steps between days 7 and 28 of pregnancy (P < 0.001 and P < 0.05, respectively; Figure 1). IGF1R expression increased only between days 7 and 14 in both cycling and pregnant mares (P < 0.05; Figure 1). INSR expression increased after day 7 of pregnancy (P < 0.001), and was twice as high on day 21 of pregnancy as day 21 of the cycle (i.e., estrus; P < 0.01; Figure 1). IGFBP1 expression decreased between days 14 and 21 of the cycle (P < 0.05), and increased after day 7 of pregnancy (P < 0.01). IGFBP1 expression was higher in endometrium from pregnant mares than that of mares in estrus (day 21 of the cycle) (P < 0.001; Figure 1). Conversely, IGFBP2, IGFBP4 and IGFBP5 were more highly expressed in endometrium from day 21 cycling (estrus) than pregnant mares (P < 0.05; Figure 1). Nevertheless, IGFBP2 expression increased between days 14 and 28 of pregnancy (P < 0.01).
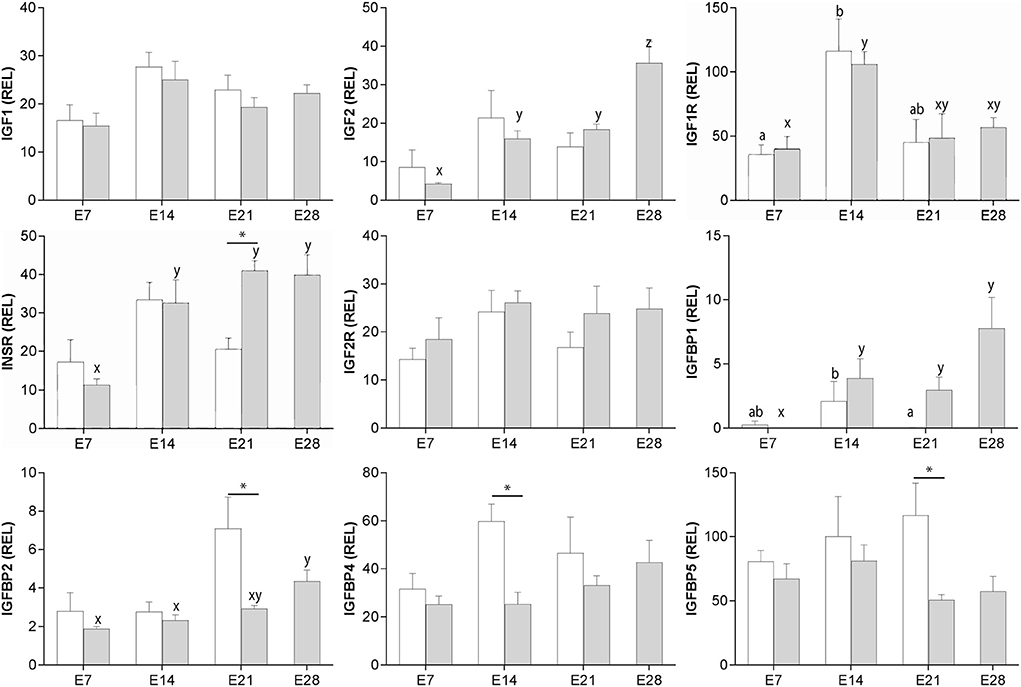
Figure 1. Relative gene expression (mean ± s.e.m) for IGF1, IGF2, IGF1R, INSR, IGF2R, IGFBP1, IGFBP2, IGFBP4 and IGFBP5 in equine endometrium from cyclic (white bars) or pregnant mares (gray bars) on days 7, 14, 21 and 28 (pregnancy only) after ovulation. Values are calculated as the ratio of the target gene mean value to the geometric mean for the reference genes GAPDH, PGK1 and SRP14. Significant differences between condition (cyclic vs. pregnant) within the same day are indicated by an asterisk (P < 0.05), while differences between days are denoted by different superscripts (cycle: a, b; pregnancy; x, y, z; P < 0.05).
Gene expression for IGF system components in early equine conceptus membranes
The expression of mRNA for all of the genes studied increased with the advance of early pregnancy in yolk sac (YS), in particular after day 14 (P < 0.05; Figure 2), and was relatively high in allantochorion (AC) once this membrane had developed.
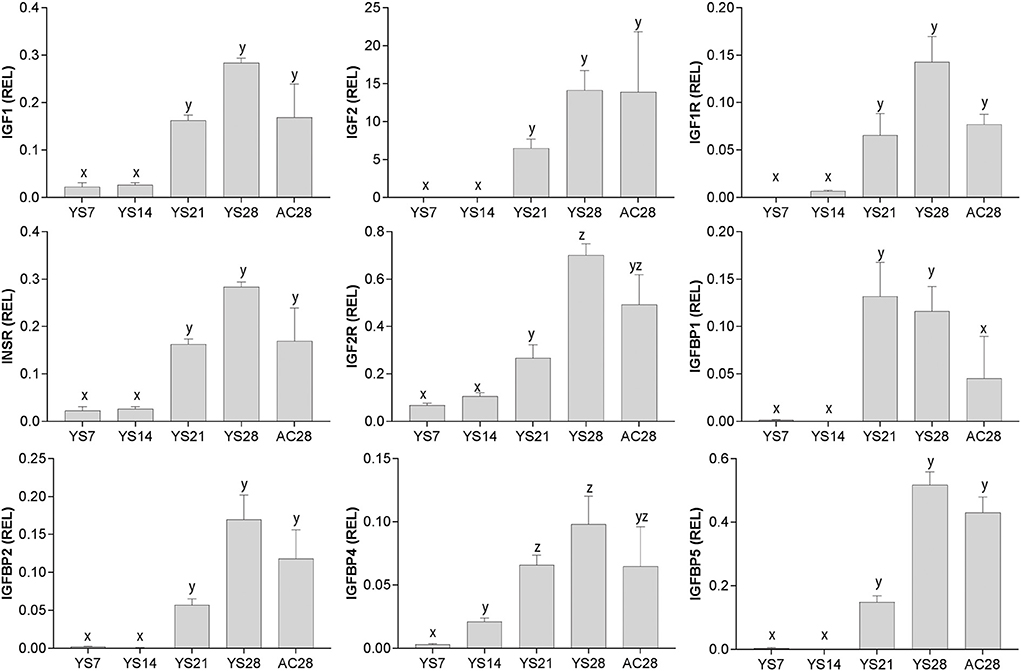
Figure 2. Relative gene expression (mean ± s.e.m) for IGF1, IGF2, IGF1R, INSR, IGF2R, IGFBP1, IGFBP2, IGFBP4 and IGFBP5 in equine conceptus membranes (yolk sac: YS; allantochorion: AC) on different days of pregnancy. Values are calculated as the ratio of the target gene mean value to the geometric mean for the reference genes GAPDH, HPRT1, PGK1 and SRP14. Significant differences between pregnancy stages are denoted by different superscripts (x, y, z; P < 0.05).
Localization of IGF system proteins in equine endometrium
Immunostaining for IGF1 was detectable in the cytoplasm of luminal epithelial cells (LE) and glandular epithelial cells (GE; Figure 3A) in endometrial samples from all cycling and pregnant mares. During the cycle, a strong signal was detected in the basal part of the LE on day 14, whereas on day 21 (estrus) the signal was mainly detected on the apical aspect of the LE (Figure 3A). During pregnancy, IGF1 was abundant in the GE and LE on day 21 of pregnancy, whereas on day 28 IGF1 staining was mainly localized to the apical side of the LE. IGF1 protein was not detected in the stroma (Figure 3A). IGF2 immunostaining was weak on day 7 of the cycle but subsequently increased in the LE, GE and stroma. On days 7 and 14 of pregnancy, weak IGF2 staining was evident in the LE, GE and the stroma (Figure 3B). From day 21 of pregnancy, moderate IGF2 staining was detected in the LE and stroma (Figure 3B). IGF1R protein was localized to the GE, stroma and the LE with strong staining apparent in the basal part of the LE on day 7 of the cycle and pregnancy (Figure 3C). By day 21, the staining was more diffuse within the LE, GE and stroma, with a stronger intensity in endometrium from pregnant mares. On day 28, IGF1R staining was stronger in the apical and basal aspects of the LE. INSR was abundant in the GE on day 7 of diestrus but staining intensity had reduced by days 14 and 21 when some staining appeared in the LE (Figure 3D). On day 28 of pregnancy, INSR expression was not detectable in the GE, but was moderately intense in the LE (Figure 3D).
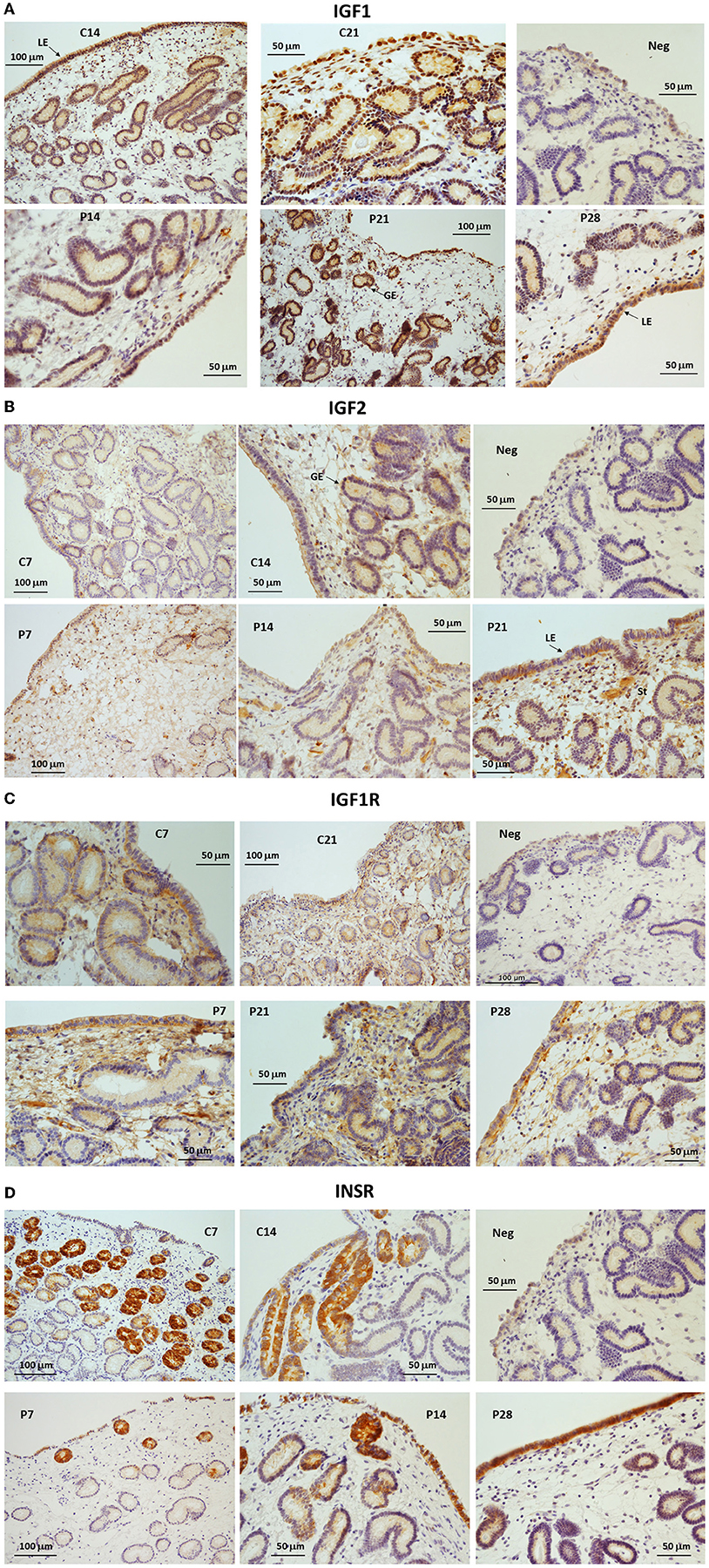
Figure 3. (A–D) Immunohistochemical localization of (A) IGF1, (B) IGF2, (C) IGF1R and (D) INSR in endometrium from cyclic (C: days 7, 14 or 21) and pregnant (P: days 7, 14, 21 or 28) horse mares. NC, negative control. LE, luminal epithelium; GE, glandular epithelium; St, stroma.
Localization of IGF system proteins in early equine conceptus membranes
IGF1 was detected from day 14 of pregnancy in trophectoderm (Te) and endoderm cells (En) and staining intensity increased in the apical part of the Te on days 21 and 28 (Figure 4A). Furthermore, IGF1-stained nucleated cells were visible within the blood vessels from day 21. IGF2 immunostaining was moderate in Te and En cells at all stages. IGF2 protein was more abundant on day 21 of pregnancy, from when it could also be detected in the mesoderm (Me). By day 28, the signal was strongest in the apical part of the Te (Figure 4A). IGF2 was not detected in endothelial cells. IGF1R protein staining was intense in the En and apical part of the Te, with weak staining appearing in the endothelium from day 21. On day 28, the staining of the En remained strong in the YS but was weaker in the AC (Figure 4B). Finally, staining for INSR was absent on days 14 and 21 and only weak staining was detected in the En and Me of day 28 YS and in the Te of AC (Figure 4B).
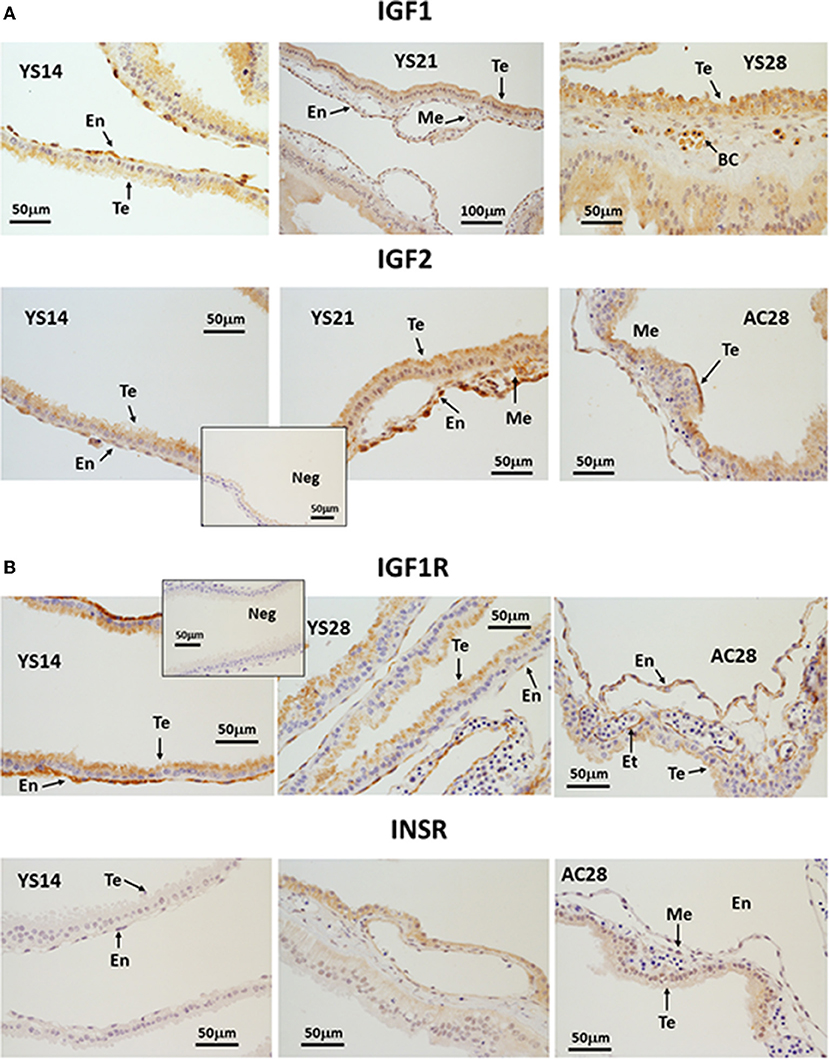
Figure 4. (A, B) Immunohistochemical localization of (A) IGF1 and IGF2, (B) IGF1R and INSR in equine conceptus membranes on days 14, 21 and 28 of pregnancy (YS, yolk sac; AC, allantochorion). Te, trophectoderm; En, endoderm; Me, mesoderm; BC, blood cells; Et, endothelium.
Impact of asynchronous ET on IGF system component mRNA expression
In the endometrium, only INSR expression was affected by asynchronous ET (Figure 5A), with expression higher 6 days after asynchronous than synchronous ET but lower 11 days after asynchronous ET (day 19 of embryo development). In addition, while INSR expression increased from day 14 to day 19 of pregnancy after synchronous ET, it decreased between days 14 and 19 in asynchronous pregnancies (Figure 5A).
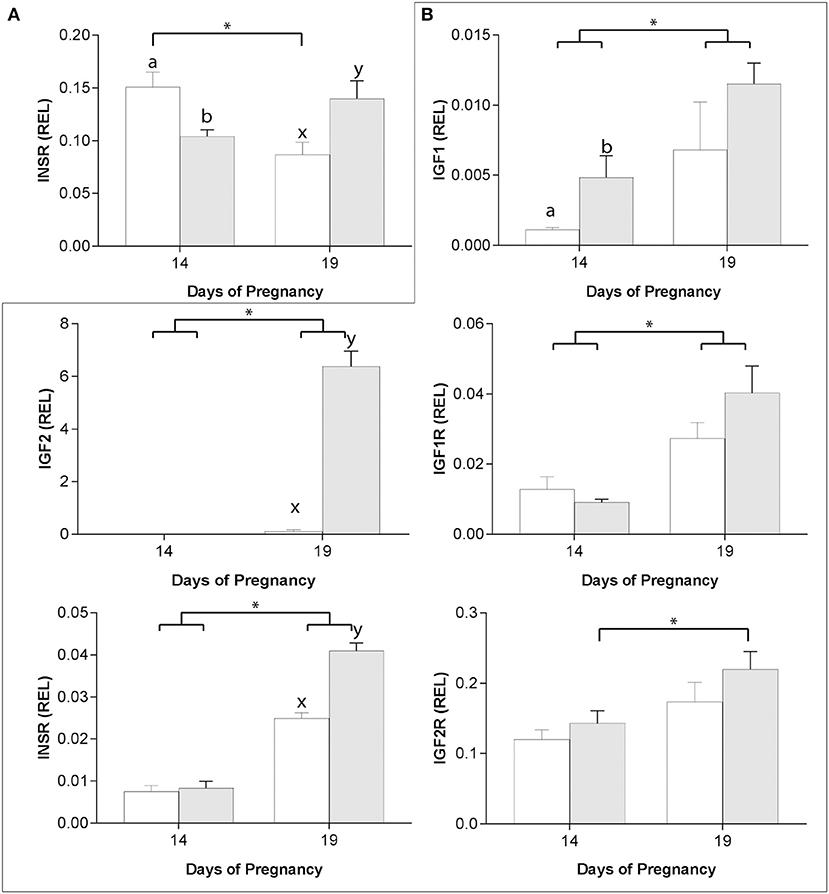
Figure 5. Relative gene expression (mean ± s.e.m) on days 14 and 19 of pregnancy after transfer of day 8 horse embryos to asynchronous (day 3: white bars) or synchronous (day 8: gray bars) recipient mares, for INSR in the endometrium (A) and for IGF1, IGF2, IGF1R, INSR, and IGF2R in the conceptus membranes (B). Values are calculated as the ratio of the target gene mean value to the geometric mean for the reference genes (GAPDH, PGK1 and SRP14 for the conceptus, and GAPDH, HPRT1, PGK1 and SRP14 for the endometrium). Significant differences (P < 0.05) between group (asynchronous vs. synchronous) within the same day are depicted by different superscripts (day 14: a, b; day 19: x, y) while pregnancy stage differences within a group are indicated by an asterisk (*).
In previous studies, we showed that the expression of the placental imprinted genes IGF2, INSR and IGF2R was affected by asynchronous ET (29). In the present study, we found that IGF1 and IGF1R expression was also affected by asynchronous ET. On day 14 of conceptus development, IGF1 was more highly expressed in YS after synchronous than asynchronous ET, with expression increasing between days 14 and 19 in both groups (P < 0.05; Figure 5B). IGF1R and INSR expression increased between days 14 and 19 of conceptus development in both groups (P < 0.05) and, on day 19, expression for INSR was higher in synchronous than asynchronous conceptuses (P < 0.001; Figure 5B). IGF2 was not detectable in day 14 YS in either group, and was higher in synchronous pregnancies on day 19 (P < 0.01; Figure 3B). Finally, IGF2R mRNA transcript increased between days 14 and 19 only in YS from synchronous conceptuses (P < 0.05; Figure 5B).
Discussion
This study demonstrated that most IGF system components are expressed in the endometrium of cycling and pregnant mares and in embryos and the conceptus membranes between days 7 and 28 of pregnancy, and are differentially regulated during the estrous cycle and pregnancy. IGF1 and IGF2, IGF1R, IGF2R and INSR and IGFBP 1, 2, 4 and 5 mRNA was detected in the endometrium and in conceptus membranes. In addition, IGF1, IGF2 and IGF1R protein was expressed strongly in the endometrium and the conceptus, whereas INSR was expressed primarily in the endometrium. Transferring an embryo to an asynchronous recipient retarded the upregulation of gene expression for IGF1, IGF2 and INSR in the yolk sac membrane, but altered the gene expression of only INSR in the endometrium.
Previous publications reported measurable quantities of IGF1 in uterine fluids, the endometrium and in blastocoel fluid (18) during early equine pregnancy, and found that both mRNA and protein were detectable in the endometrium and conceptus membranes (17, 19). We confirmed that IGF1 is produced by the endometrium and the conceptus between days 7 and 28 of pregnancy. However, equine endometrial expression of IGF1 does not seem to be regulated by P4 or conceptus factors, since mRNA levels were stable across both the cycle (including estrus) and early pregnancy and were not affected by uterine-conceptus asynchrony. Similarly, IGF1 expression in equine endometrium was not affected by treating mares with the synthetic progestogen, altrenogest (17), nor was it influenced in vitro by exposing endometrial explants to E2 (18). This contrasts to reports in mice and pigs, in which both E2 and P4 have been shown to stimulate uterine IGF1 expression (35, 36). Although we did not see any increase in IGF1 mRNA expression, we did find that IGF1 protein expression was relocated to the apical aspect of the luminal epithelium and the glandular epithelium during pregnancy. This suggests that IGF1 produced by the LE and the endometrial glands could be secreted more effectively into the uterine lumen, where it is better placed to influence conceptus development. Alternatively, IGF1 produced in the GE could act in a paracrine fashion on IGF1R expressed in surrounding endometrial cells to stimulate uterine function and thereby indirectly support conceptus development (13). In conceptus membranes, IGF1 mRNA and protein increased during pregnancy with expression evident in the TE, En, and nucleated blood cells. In rabbit blastocysts, IGF1 has a mainly mitogenic role inducing cell proliferation and growth, and promoting development of the embryonic disc (37, 38).
In contrast to IGF1, IGF2 expression in the endometrium did appear to be regulated by prolonged exposure to P4 and/or the conceptus, given that it increased during pregnancy but not diestrus. The intensity of IGF2 protein staining also increased during pregnancy, and it was highly expressed in the apical aspect of the LE on day 28, which suggests that it could be secreted into the uterine lumen to support conceptus growth. Previously, it was reported that IGF2 was expressed only from day 20 of pregnancy in the vascularized mesoderm, and that its expression increased in the allantochorion after implantation (15). However, we found IGF2 to be highly expressed in the trophectoderm, endoderm and mesoderm from day 14 of pregnancy. IGF2 could play a role in equine conceptus growth and placentation and, in particular, it has been proposed to play a role in cell migration and invasion because it is more highly expressed in the invasive cells of the chorionic girdle than in non-invasive trophoblast prior to implantation (15); in this respect, IGF2 is known to stimulate trophectoderm cell migration in sheep (39).
In endometrium, IGF1R expression increased on day 14 of the cycle and pregnancy, whereas protein expression was more intense on day 21 in both conditions, primarily in the stroma and the LE. It is possible that P4 is responsible for stimulating IGF1R expression, as described for human endometrial stromal cells (40), with protein expression maintained in day 21 cycling mares despite the return to estrus; alternatively, E2 produced by the follicle and/or the early conceptus may play a role in stimulating increased IGF1R expression following a period of P4 dominance. The increased abundance of IGF1R during early pregnancy would increase its availability for binding IGF1 and IGF2, and thereby presumably help promote mitogenic activity in the endometrium as part of the preparation for implantation (40). Previously, Klein et al. (23) found that IGF1R was up-regulated in the early equine conceptus and, here, we show that IGF1R is expressed in the apical part of the trophectoderm, the endoderm, and in endothelial cells. IGF1 has a higher affinity for IGF1R than IGF2, but both are apparently able to activate IGF1R in mouse embryos since deletion of the receptor results in a more severe growth retardation than deletion of either IGF1 or IGF2 (3, 41–43). It is likely that both endometrial IGF1 and IGF2 exert any actions on the equine conceptus via IGF1R (44). Furthermore, IGF1 was expressed by nucleated cells within the blood vessels while IGF1R was present in endothelial cells, therefore IGF1 could be involved in stimulating blood vessel formation and development in equine conceptus membranes via the IGF1R, as reported in other species (45, 46).
In this study, we found an increase in INSR expression during pregnancy with stage-specific changes in localization of the protein; initially, INSR was primarily detected in glandular epithelium but, between days 21 and 28, expression switched to the luminal epithelium. In addition, INSR was the only member of the IGF family to be differentially expressed in endometrium between synchronous and asynchronous pregnancies, with lower expression in asynchronous than synchronous pregnancies on day 19 of conceptus development. This either means that INSR requires a longer duration of P4 exposure for increased expression, or that factors expressed by a more advanced conceptus are involved in stimulating or maintaining expression. In the rat, INSR is expressed in the LE and stromal cells and is upregulated primarily in the LE at the site of implantation (47). In women, INSR is expressed in epithelial and stromal cells, and a treatment designed to mimic decidualization in human endometrial cells induced INSR up-regulation (40, 48). Since IGF2 has a high affinity for both the INSR homodimer and the IGF1R/INSR heterodimer, and the activation of these receptors by IGF2 triggers cellular proliferation and differentiation (16), these results suggest that endometrial INSR plays an important role in the preparation for implantation. While INSR mRNA was also detected in conceptus membranes, protein levels were very low and detectable only on day 28 of pregnancy, suggesting that any INSR does not play a direct role in conceptus development early in pregnancy.
Expression of the IGF2R and IGF2 genes in equine trophoblast has been shown to be imprinted, with IGF2R preferentially expressed from the maternal allele and IGF2 from the paternal allele (16). Both are involved in regulating conceptus growth and development, with deletion of IGF2R leading to placental and fetal overgrowth, whereas deletion of IGF2 leads to placental and fetal growth restriction, in mice (5, 41, 42, 48). In equine conceptuses, IGF2R mRNA was previously reported to be up-regulated between days 8 and 12 of pregnancy (23), and IGF2R protein was secreted by day 9 and 10 conceptuses (24). Our results support these previous studies, with conceptus membrane IGF2R expression also increasing as pregnancy progressed. IGF2R presumably plays a role in regulating IGF2 abundance to optimize fetal growth. On the other hand, it has been reported that IGF2 is able to stimulate cell migration mediated via IGF2R in bovine endothelial cells (49) and human extravillous trophoblast cells (43, 50).
IGFBP1 is one of the most studied IGFBPs during early pregnancy. In the horse, P4 and the presence of a conceptus appear to stimulate endometrial IGFBP1 expression, and E2 to supress, since IGFBP1 increased during pregnancy but decreased between days 14 and 21 of the cycle (late diestrus and estrus, respectively). Endometrial IGFBP1 has also been reported to be up-regulated between days 8 and 12 of pregnancy (21) and to be more highly expressed on day 13.5 of pregnancy than the cycle (22), with the respective authors suggesting that IGFBP1 could regulate IGF1 trafficking between the endometrium and the uterine luminal fluid. Similarly, in ruminants IGFBP1 is stimulated by interferon-tau, P4 and prostaglandins (9, 51, 52), and has been shown to stimulate ovine trophoblast cell migration and attachment (11, 51). In women, IGFBP1 is a major secretary product of decidual cells, a marker for decidualization and involved with modulation of trophoblast invasion (7, 53). In equine pregnancy, IGFBP1 has been proposed to play a role in conceptus-endometrium communication and in particular in modulating implantation by regulating trophoblast invasion (51, 54). IGFBP2 mRNA levels increased during estrus and later equine pregnancy (day 28), suggesting that its expression could be stimulated by E2 and/or the presence of the conceptus; IGFBP2 expression also increases during pregnancy in the pig and responds to E2 and P4 in human endometrial cells (54). While IGFBP4 and IGFBP5 were expressed in equine endometrium, they increased during estrus rather than pregnancy. In equine conceptus membranes, IGFBP1, 2, 4 and 5 mRNA expression increased during early pregnancy; however, protein expression and localization is not yet known. Previously, IGFBP4 was found to be up-regulated between days 8 and 12 of pregnancy (23), and the literature supports the idea that elevated IGFBP1 in the fetus is linked to growth restriction (4, 41, 55), suggesting that IGFBP1 could help regulate IGF1 availability in the conceptus or directly affect placental development. The functions of IGFBPs 2, 4 and 5 have been less studied, but as they are expressed in the endometrium and the conceptus, they presumably bind IGF1 and IGF2 and regulate their availability and/or have direct effects in preparing the endometrium or trophectoderm for implantation. The present study did not include IGFBP3 simply because, despite a number of attempts, we were unable to design successful PCR primers. However, in a related study in which next generation sequencing was used to examine the effects of asynchronous embryo transfer on the endometrial transcriptome, we found that IGFBP3 was consistently upregulated in the endometrium of synchronous compared to asynchronous pregnancy (31). Other transcriptomic studies have similarly indicated that IGFBP3 expression is upregulated in the endometrium of pregnant compared to non-pregnant mares (21, 22), while IGFBP3 protein has been detected in the uterine luminal fluids and associated to the blastocyst capsule (56). In studies designed to examine a direct effect of IGFBP3 on trophectoderm cells, we were unable to demonstrate a clear effect on cell proliferation or attachment to plastic in vitro (31), and are therefore currently left to speculate that it plays the proposed role of matching conceptus and endometrial stage indirectly, e.g., by regulating availability of the IGFs, as suggested for other IGFBPs.
In the conceptus, IGF1, IGF2, IGF1R and INSR increased between days 14 and 19 after both synchronous and asynchronous ET, whereas IGF2R only increased after synchronous ET. In addition, IGF2 and INSR were less highly expressed in day 19 yolk sac after asynchronous than synchronous ET. The reduced IGF2 and INSR expression in conceptus membranes after asynchronous ET is presumably a result of the associated delay in conceptus development. Interestingly, INSR expression was lower in the endometrium of asynchronous mares, and this decrease in INSR is presumably either a result of inadequate stimulation from the retarded conceptus or may contribute to the delay of conceptus development. In this respect, reduced availability of INSR could affect the ability of insulin and the IGFs to activate pathways via their receptors and IGFBP1 (57, 58).
In conclusion, members of the IGF system are highly expressed in both the endometrium and conceptus membranes during early equine pregnancy and are likely to play important roles in conceptus growth and development before implantation, and in preparing the endometrium to support implantation. IGF1 and IGF2 are expressed by the endometrium and the conceptus and presumably exert their effects via IGF1R and INSR in the endometrium and IGF1R in the conceptus membranes; these effects could include stimulating cell proliferation and differentiation. The expression of IGF1 and IGF1R in the conceptus membranes and the presence of IGF1 protein in blood cells suggests a role in vascularization and the development of endothelial cells. In the endometrium, although IGF1R is abundant, increased INSR expression in the luminal epithelium on day 28 of pregnancy implies a specific role in preparing for implantation.
Data availability statement
The raw data supporting the conclusions of this article will be made available by the authors, without undue reservation.
Ethics statement
The animal study was reviewed and approved by Utrecht University's Animal Experimentation Committee (permits 2007.III.02.036 and 2012.III.02.020).
Author contributions
CG performed the practical work, analysis and wrote the first draft of the manuscript. MDR-V contributed to project planning, performance, and supervision. TS involved in funding acquisition, supervision, and edited the manuscript. All authors have read and agreed to the final version of the manuscript.
Funding
This research was supported by the European Commission under the Marie Curie ITN project EpiHealthNet; FP7-PEOPLE-2012-ITN n° 317146.
Acknowledgments
The authors would like to thank Andras Dinnyes, the scientific co-ordinator of EpiHealthNet.
Conflict of interest
The authors declare that the research was conducted in the absence of any commercial or financial relationships that could be construed as a potential conflict of interest.
Publisher's note
All claims expressed in this article are solely those of the authors and do not necessarily represent those of their affiliated organizations, or those of the publisher, the editors and the reviewers. Any product that may be evaluated in this article, or claim that may be made by its manufacturer, is not guaranteed or endorsed by the publisher.
References
1. Annunziata M, Granata R, Ghigo E. The IGF system. Acta Diabetol. (2011) 48:1–9. doi: 10.1007/s00592-010-0227-z
2. Belfiore A, Frasca F, Pandini G, Sciacca L, Vigneri R. Insulin receptor isoforms and insulin receptor/insulin-like growth factor receptor hybrids in physiology and disease. Endocr Rev. (2009) 30:586–623. doi: 10.1210/er.2008-0047
3. Baker J, Liu J-P, Robertson EJA. Efstratiadis, Role of insulin-like growth factors in embryonic and postnatal growth. Cell. (1993) 75:73–82. doi: 10.1016/S0092-8674(05)80085-6
4. Randhawa R, Cohen P. The role of the insulin-like growth factor system in prenatal growth, Mol. Genet Metab. (2005) 86:84–90. doi: 10.1016/j.ymgme.2005.07.028
5. Angiolini E, Fowden A, Coan P, Sandovici I, Smith P, Dean W, et al. Constância, Regulation of placental efficiency for nutrient transport by imprinted genes. Placenta. (2006) 27 (Suppl. A):S98–102. doi: 10.1016/j.placenta.2005.12.008
6. Jones HN, Powell TL, Jansson T. Regulation of placental nutrient transport - a review, Placenta. (2007) 28:763–74. doi: 10.1016/j.placenta.2007.05.002
7. Nayak N, Giudice L. Comparative biology of the IGF system in endometrium, decidua, and placenta, and clinical implications for foetal growth and implantation disorders. Placenta. (2003) 24:281–96. doi: 10.1053/plac.2002.0906
8. Rutanen EM. Insulin-like growth factors and insulin-like growth factor binding proteins in the endometrium. Effect of intrauterine levonorgestrel delivery. Hum Reprod. (2000) 15 (Suppl. 3). 173–81. doi: 10.1093/humrep/15.suppl_3.173
9. R. Robinson. The expression of the IGF system in the bovine uterus throughout the oestrous cycle and early pregnancy. J Endocrinol. (2000) 165:231–43. doi: 10.1677/joe.0.1650231
10. Satterfield MC, Hayashi K, Song G, Black SG, Bazer FW, Spencer TE. Spencer, progesterone regulates FGF10, MET, IGFBP1, and IGFBP3 in the endometrium of the ovine uterus. Biol Reprod. (2008) 79:1226–36. doi: 10.1095/biolreprod.108.071787
11. Simmons RM, Erikson DW, Kim J, Burghardt RC, Bazer FW, Johnson GA, et al. Insulin-like growth factor binding protein-1 in the ruminant uterus: potential endometrial marker and regulator of conceptus elongation. Endocrinology. (2009) 150:4295–305. doi: 10.1210/en.2009-0060
12. Reynolds TS, Stevenson KR, Wathes DC. Pregnancy-Specific alterations in the expression of the insulin-like growth factor system during early placental development in the Ewe. Endocrinology. (1997) 138:886–97. doi: 10.1210/endo.138.3.4983
13. Ribeiro ES, Santos JE, Thatcher WW. Thatcher, role of lipids on elongation of the preimplantation conceptus in ruminants. Reproduction. (2016) 152:R115–26. doi: 10.1530/REP-16-0104
14. Matsumoto H, Sakai K, Iwashita M. Insulin-like growth factor binding protein-1 induces decidualization of human endometrial stromal cells via alpha5beta1 integrin. Mol Hum Reprod. (2008) 14:485–89. doi: 10.1093/molehr/gan038
15. Lennard SN, Stewart F, Allen WR. Insulin-like growth factor II gene expression in the fetus and placenta of the horse during the first half of gestation. J Reprod Fertil. (1995) 103:169–79. doi: 10.1530/jrf.0.1030169
16. Wang XX, Miller DC, Harman R, Antczak DF, Clark AG. Paternally expressed genes predominate in the placenta. Proc Natl Acad Sci U S A. (2013) 110:10705–10. doi: 10.1073/pnas.1308998110
17. Willmann C, Budik S, Walter I, Aurich C. Influences of treatment of early pregnant mares with the progestin altrenogest on embryonic development and gene expression in the endometrium and conceptus. Theriogenology. (2011) 76:61–73. doi: 10.1016/j.theriogenology.2011.01.018
18. Walters KW, Roser JF, Anderson GB. Maternal-conceptus signalling during early pregnancy in mares: Oestrogen and insulin-like growth factor I. Reproduction. (2001) 121:331–38. doi: 10.1530/rep.0.1210331
19. Beckelmann J, Budik S, Helmreich M, Palm F, Walter I, Aurich C. Aurich. Sex-dependent insulin like growth factor-1 expression in preattachment equine embryos. Theriogenology. (2013) 79 193–9. doi: 10.1016/j.theriogenology.2012.10.004
20. Arai KY, Tanaka Y, Taniyama H, Tsunoda N, Nambo Y, Nagamine N, et al. Expression of inhibins, activins, insulin-like growth factor-I and steroidogenic enzymes in the equine placenta. Domest Anim Endocrinol. (2006) 31:19–34. doi: 10.1016/j.domaniend.2005.09.005
21. Merkl M, Ulbrich SE, Otzdorff C, Herbach N, Wanke R, Wolf E, et al. Microarray analysis of equine endometrium at days 8 and 12 of pregnancy. Biol Reprod. (2010) 83:874–86. doi: 10.1095/biolreprod.110.085233
22. Klein C, Scoggin KE, Ealy AD, Troedsson MH. Transcriptional profiling of equine endometrium during the time of maternal recognition of pregnancy. Biol Reprod. (2010) 83:102–13. doi: 10.1095/biolreprod.109.081612
23. Klein MHT. Troedsson, transcriptional profiling of equine conceptuses reveals new aspects of embryo-maternal communication in the horse1. Biol Reprod. (2011) 84:872–85. doi: 10.1095/biolreprod.110.088732
24. Swegen A, Grupen CG, Gibb Z, Baker MA, de Ruijter-Villani M, Smith ND, et al. From peptide masses to pregnancy maintenance: a comprehensive proteomic analysis of the early equine embryo secretome, blastocoel fluid, and capsule. Proteomics. (2017). doi: 10.1002/pmic.201600433
25. Scott CD, Kiess W. Soluble M6P/IGFIIR in the circulation. Best Pract Res Clin Endocrinol Metab. (2015) 29:723–33. doi: 10.1016/j.beem.2015.08.001
26. Allen WR, Stewart F. Equine placentation. Reprod Fertil Dev. (2001) 13:1063. doi: 10.1071/RD01063
27. Wilsher S, Allen WR. Uterine influences on embryogenesis and early placentation in the horse revealed by transfer of day 10 embryos to day 3 recipient mares. Reproduction. (2009) 137:583–93. doi: 10.1530/REP-08-0328
28. Wilsher S, Clutton-Brock A, Allen WR. Successful transfer of day 10 horse embryos: influence of donor-recipient asynchrony on embryo development. Reproduction. (2010) 139:575–85. doi: 10.1530/REP-09-0306
29. Gibson C, de Ruijter-Villani M, Stout TAE. Negative uterine asynchrony retards early equine conceptus development and upregulation of placental imprinted genes. Placenta. (2017) 57:175–82. doi: 10.1016/j.placenta.2017.07.007
30. Spencer TE, Johnson GA, Burghardt RC, Bazer FW. Progesterone and placental hormone actions on the uterus: insights from domestic animals. Biol Reprod. (2004) 71:2–10. doi: 10.1095/biolreprod.103.024133
31. Gibson C, de Ruijter-Villani M, Bauersachs S, Stout TAE. Asynchronous embryo transfer followed by comparative transcriptomic analysis of conceptus membranes and endometrium identifies processes important to the establishment of equine pregnancy. Int J Mol Sci. (2020) 21:2562. doi: 10.3390/ijms21072562
32. de Ruijter-Villani M, Deelen C, Stout TA. Expression of leukaemia inhibitory factor at the conceptus-maternal interface during preimplantation development and in the endometrium during the oestrous cycle in the mare. Reprod Fertil Dev. (2016) 28:1642–51. doi: 10.1071/RD14334
33. Vandesompele J, Preter KD, Pattyn F, Poppe B, Van Roy N, Paepe AD, et al. Speleman, Accurate normalization of real-time quantitative RT-PCR data by geometric averaging of multiple internal control genes. Genome Biol. (2002) 3:34–1. doi: 10.1186/gb-2002-3-7-research0034
34. Gibson C, de Ruijter-Villani M, Rietveld J, Stout TAE. Amino acid transporter expression in the endometrium and conceptus membranes during early equine pregnancy. Reprod Fertil Dev. (2018). doi: 10.1071/RD17352
35. Bowman CJ, Streck RD, Chapin RE. Maternal-placental insulin-like growth factor (IGF) signaling and its importance to normal embryo-fetal development. Birth Defects Res Part B - Dev Reprod Toxicol. (2010) 89:339–49. doi: 10.1002/bdrb.20249
36. Simmen RC, Simmen FAA, Hofig SJ, Farmer FW, Bazer. Hormonal regulation of insulin-like growth factor gene expression in pig uterus. Endocrinology. (1990) 127 2166–74. doi: 10.1210/endo-127-5-2166
37. Navarrete Santos A, Ramin N, Tonack S, Fischer B. Cell lineage-specific signaling of insulin and insulin-like growth factor I in rabbit blastocysts. Endocrinology. (2008) 149:515–24. doi: 10.1210/en.2007-0821
38. Thieme R, Ramin N, Fischer S, Püschel B, Fischer B, Navarrete Santos A, et al. Gastrulation in rabbit blastocysts depends on insulin and insulin-like-growth-factor 1, Mol. Cell Endocrinol. (2012) 348:112–9. doi: 10.1016/j.mce.2011.07.044
39. Kim J, Song G, Gao H, Farmer JL, Satterfield MC, Burghardt RC, et al. Bazer, Insulin-like growth factor II activates phosphatidylinositol 3-kinase-protooncogenic protein kinase 1 and mitogen-activated protein kinase cell signaling pathways, and stimulates migration of ovine trophectoderm cells. Endocrinology. (2008) 149:3085–94. doi: 10.1210/en.2007-1367
40. Flannery CA, Saleh FL, Choe GH, Selen DJ, Kodaman PH, Kliman HJ, et al. Differential expression of IR-A, IR-B and IGF-1R in endometrial physiology and distinct signature in adenocarcinoma. J Clin Endocrinol Metab. (2016) 101:2874–82. doi: 10.1210/jc.2016-1795
42. Fowden AL. The insulin-like growth factors and feto-placental growth. Placenta. (2003) 24:803–12. doi: 10.1016/S0143-4004(03)00080-8
43. DeChiara TM, Efstratiadis A, Robertson EJ. A growth-deficiency phenotype in heterozygous mice carrying an insulin-like growth factor II gene disrupted by targeting. Nature. (1990) 345:78. doi: 10.1038/345078a0
44. Roberts CT, Owens JA, Sferruzzi-Perri AN. Distinct Actions of Insulin-Like Growth Factors (IGFs) on Placental Development and Fetal Growth: Lessons from Mice and Guinea Pigs. Placenta. (2008) 29:42–7. doi: 10.1016/j.placenta.2007.12.002
45. Tan KS, Inoue T, Kulkeaw K, Tanaka Y, Lai MI, Sugiyama D, et al. Localized SCF and IGF-1 secretion enhances erythropoiesis in the spleen of murine embryos, Biol. Open. (2015) 4:596–607. doi: 10.1242/bio.201410686
46. Piecewicz SM, Pandey A, Roy B, Hua Xiang S, Zetter BRS. Sengupta, insulin-like growth factors promote vasculogenesis in embryonic stem cells. PLoS One. (2012) 7:e32191. doi: 10.1371/journal.pone.0032191
47. Korgun ET, Dohr G, Desoye G, Demir R, Kayisli UA, Hahn T. Expression of insulin, insulin-like growth factor I and glucocorticoid receptor in rat uterus and embryo during decidualization, implantation and organogenesis. Reproduction. (2003) 125:75–84. doi: 10.1530/rep.0.1250075
48. Ganeff C, Chatel G, Munaut C, Frankenne F, Foidart JM, Winkler R. The IGF system in in-vitro human decidualization. Mol Hum Reprod. (2009) 15:27–38. doi: 10.1093/molehr/gan073
49. Constância M, Hemberger M, Hughes J, Dean W, Ferguson-Smith A, Fundele R, et al. Placental-specific IGF-II is a major modulator of placental and fetal growth. Nature. (2002) 417:945–8. doi: 10.1038/nature00819
50. Volpert O, Jackson D, Bouck N, Linzer DI. The insulin-like growth factor II/mannose 6-phosphate receptor is required for proliferin-induced angiogenesis. Endocrinology. (1996) 137:3871–6. doi: 10.1210/endo.137.9.8756559
51. McKinnon T, Chakraborty C, Gleeson LM, Chidiac P, Lala PK. Stimulation of human extravillous trophoblast migration by IGF-II is mediated by IGF type 2 receptor involving inhibitory G protein(s) and phosphorylation of MAPK. J Clin Endocrinol Metab. (2001) 86:3665–74. doi: 10.1210/jcem.86.8.7711
52. Satterfield MC, Song G, Kochan KJ, Riggs PK, Simmons RM, Elsik CG, et al. Discovery of candidate genes and pathways in the endometrium regulating ovine blastocyst growth and conceptus elongation. Physiol Genomics. (2009) 39:85–99. doi: 10.1152/physiolgenomics.00001.2009
53. Dorniak P, Bazer FW, Wu G, Spencer TE. Conceptus-derived prostaglandins regulate endometrial function in sheep. Biol Reprod. (2012) 87:1–7. doi: 10.1095/biolreprod.112.100487
54. Crossey PA, Pillai CC, Miell JP. Altered placental development and intrauterine growth restriction in IGF binding protein-1 transgenic mice. J Clin Invest. (2002) 110:411–8. doi: 10.1172/JCI0210077
55. Gleeson LM, Chakraborty C, McKinnon T, Lala PK. Insulin-Like growth factor-binding protein 1 stimulates human trophoblast migration by signaling through α5β1 integrin via mitogen-activated protein kinase pathway. J Clin Endocrinol Metab. (2001) 86:2484–93. doi: 10.1210/jc.86.6.2484
56. Østrup S, Bauersachs H, Blum E, Wolf P. Hyttel. Differential endometrial gene expression in pregnant and nonpregnant sows. Biol Reprod. (2010) 83:277–85. doi: 10.1095/biolreprod.109.082321
57. Herler A, Pell JM, Allen WR, Beier HM, Stewart F. Horse conceptuses secrete insulin-like growth factor-binding protein 3. Biol Reprod. (2000) 62:1804–11. doi: 10.1095/biolreprod62.6.1804
58. Lathi RB, Hess AP, Tulac S, Nayak NR, Conti M, Giudice LC, et al. Dose-Dependent insulin regulation of insulin-like growth factor binding protein-1 in human endometrial stromal cells is mediated by distinct signaling pathways. J Clin Endocrinol Metab. (2005) 90:1599–606. doi: 10.1210/jc.2004-1676
Keywords: equine, pregnancy, insulin-like growth factor, endometrium, conceptus, asynchronous embryo transfer
Citation: Gibson C, de Ruijter-Villani M and Stout TAE (2022) Insulin-like growth factor system components expressed at the conceptus-maternal interface during the establishment of equine pregnancy. Front. Vet. Sci. 9:912721. doi: 10.3389/fvets.2022.912721
Received: 04 April 2022; Accepted: 28 July 2022;
Published: 13 September 2022.
Edited by:
Pietro Medica, University of Messina, ItalyReviewed by:
Ali A. Fouladi-Nashta, Royal Veterinary College (RVC), United KingdomVictoria De Brun, Universidad de la República, Uruguay
Copyright © 2022 Gibson, de Ruijter-Villani and Stout. This is an open-access article distributed under the terms of the Creative Commons Attribution License (CC BY). The use, distribution or reproduction in other forums is permitted, provided the original author(s) and the copyright owner(s) are credited and that the original publication in this journal is cited, in accordance with accepted academic practice. No use, distribution or reproduction is permitted which does not comply with these terms.
*Correspondence: Tom A. E. Stout, dC5hLmUuc3RvdXRAdXUubmw=