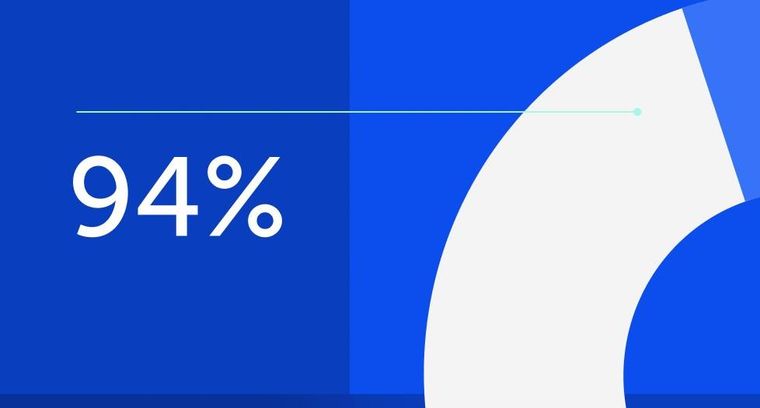
94% of researchers rate our articles as excellent or good
Learn more about the work of our research integrity team to safeguard the quality of each article we publish.
Find out more
ORIGINAL RESEARCH article
Front. Vet. Sci., 17 June 2022
Sec. Comparative and Clinical Medicine
Volume 9 - 2022 | https://doi.org/10.3389/fvets.2022.900453
Neutrophils play a major role in many equine conditions, including equine asthma, laminitis, and intestinal ischemia and reperfusion injury, and therefore represent an attractive target for innovative therapeutic approaches. Novel strategies for reducing neutrophilic inflammation include modulation of neutrophil functions and lifespan. Withaferin A (WFA) is a phytochemical with well-established in vitro and in vivo anti-inflammatory properties, but its direct effects on neutrophils are largely unknown. We hypothesized that WFA would inhibit adhesion, migration, and respiratory burst by equine neutrophils and promote timely apoptosis of primed equine neutrophils. Consistent with this hypothesis, our data show that WFA causes a significant, concentration-dependent inhibition of equine neutrophil adhesion, migration, and respiratory burst in response to diverse stimuli. Further, WFA treatment increased apoptosis of equine neutrophils exposed to GM-CSF for 24 h. This pro-apoptotic effect of WFA was not observed in unprimed neutrophils, nor at the 2-h time point relevant to our functional neutrophil experiments. Our data demonstrate that WFA may reduce neutrophil-mediated inflammation through multiple mechanisms, including suppression of inflammatory responses and promotion of apoptosis. Additional research is needed to elucidate the molecular mechanisms for these effects and evaluate the potential clinical use of WFA in veterinary and human patients.
While neutrophils are an integral part of the innate immune system, dysregulation of neutrophil functions can cause substantial damage to host tissue. In horses, neutrophilic inflammation has been implicated in the pathophysiology of diseases that can be acutely life-threatening or compromise quality of life and/or athletic performance (1–6). The common role of neutrophils in these conditions makes these cells a potential target for treating inflammatory conditions. There is also ample evidence that neutrophils contribute to disease in humans and other veterinary species, expanding the potential impact of neutrophil-targeted therapeutic strategies (7–11).
Neutrophil participation in immune responses can be characterized by a series of interrelated and overlapping events; these processes, from initial neutrophil recruitment to microbicidal activity at the site of inflammation, represent potential opportunities for collateral tissue injury. Adhesion of circulating neutrophils to endothelium is an early requisite step for neutrophil trafficking to sites of inflammation and for preparing neutrophils to respond to subsequent inflammatory mediators (12, 13). After adhesion, neutrophils extravasate across the endothelium, follow chemotactic gradients through interstitium, and, in some cases, undergo transepithelial migration (14). The movement of neutrophils across epithelium can compromise intestinal barrier function (15–17) and increase pulmonary epithelial permeability (18). Upon reaching target tissues, neutrophils perform effector functions, such as respiratory burst, degranulation, phagocytosis, and extrusion of neutrophil extracellular traps (NETs) (19). Though these effector functions are designed to benefit host defense against pathogens or healing from injury, inappropriate or prolonged release of reactive oxygen species, granule cytotoxic proteins, and NETs also cause damage of host tissue, exacerbating disease pathology (19). Because adhesion, migration, and respiratory burst are key steps in neutrophilic inflammation, they also represent promising therapeutic targets to reduce neutrophil-mediated tissue injury (19).
Under healthy conditions, the detrimental potential of neutrophils is controlled by constitutive apoptosis (20), limiting their average lifespan in circulation to 25 h (21). However, once exposed to inflammatory molecules, such as granulocyte-macrophage colony-stimulating factor (GM-CSF) and lipopolysaccharide (LPS), neutrophils become primed, the functional lifespan of neutrophils is extended (22, 23), and neutrophil apoptosis becomes a critical step in the resolution of inflammation. Apoptotic neutrophils have decreased expression of proinflammatory genes (24) and reduced capacity to perform deleterious effect or functions (25). Additionally, phagocytosis of apoptotic neutrophils by macrophages induces a shift toward an anti-inflammatory macrophage phenotype which promotes resolution of inflammation (26). Importantly, delayed neutrophil apoptosis has been documented in a variety of inflammatory conditions affecting horses and humans, and may contribute to exacerbation of disease pathophysiology (27–34). Accordingly, therapies that promote timely neutrophil apoptosis could hasten resolution of inflammation and recovery/remission from clinical disease.
Regulation of neutrophil effector functions and apoptosis are potential strategies for novel anti-inflammatory therapies (35–40). However, there are currently no therapies approved by the US Food and Drug Administration for these indications, underscoring the importance of further research to identify and characterize potential novel neutrophil-directed anti-inflammatory treatments. A growing number of these experimental anti-inflammatory agents are natural products, often isolated from plants used in traditional medicine (37–40).
Withaferin A (WFA) is a steroidal lactone derived from the Withania somnifera plant, which has been used for millennia in Ayurvedic medicine to treat many different conditions (41). More recently, WFA has been recognized as a primary bioactive constituent of this plant, and in vitro and in vivo studies have documented beneficial effects of WFA for inflammatory diseases and multiple types of cancer (42–50). Neutrophils are central to the pathophysiology of several of these diseases, including acute lung injury, acute pancreatitis, and gout (51–56). In animal models of disease, treatment with WFA was associated with both clinical improvement and reduced neutrophil infiltration into relevant tissues (42–44, 57). These in vivo findings are further supported by an in vitro study showing that WFA reduced neutrophil transmigration across an endothelial monolayer toward Escherichia coli (58). However, a thorough investigation of specific effects of WFA on neutrophil functions, including adhesion, migration, and respiratory burst in response to diverse stimuli, has not been published.
In addition to being anti-inflammatory, WFA is also reported to induce apoptosis in a variety of cancer cell lines (59–66). Interestingly, WFA has no effect on apoptosis of normal lymphocytes and monocytes (67, 68) and is actually anti-apoptotic in different types of non-neoplastic cells, including cardiomyocytes (69, 70), pancreatic islet cells (71), renal epithelium (57, 72), and cells within the spinal cord (73). Although the effect of WFA on neutrophil apoptosis has not been described, promotion of neutrophil apoptosis by WFA could help to explain previous reports of the benefits of WFA for treating inflammatory conditions.
Based on previous evidence of WFA's anti-inflammatory and pro-apoptotic properties, we hypothesized that WFA would inhibit neutrophil adhesion, migration, and respiratory burst and would promote apoptosis of primed neutrophils. Here we report that WFA significantly attenuated neutrophil functions without affecting short-term neutrophil viability. Additionally, WFA treatment promoted timely apoptosis in GM-CSF-primed neutrophils. These findings provide strong in vitro support for WFA as a therapeutic for neutrophil-mediated diseases, which could have important implications for both veterinary and human patients.
Withaferin A (WFA), leukotriene B4 (LTB4), and platelet activating factor (PAF; C-16) were from Cayman Chemical (Ann Arbor, MI, USA); dimethyl sulfoxide (DMSO), ethanol (EtOH), recombinant human interleukin-8 (IL-8), phorbol 12-myristate 13-acetate (PMA), bovine serum albumin (BSA), rabbit anti-bovine albumin antiserum (anti-BSA), lipopolysaccharide (LPS) from Escherichia coli (O111:B4), luminol sodium salt, and penicillin-streptomycin were from MilliporeSigma (Burlington, MA, USA); recombinant equine granulocyte-macrophage colony-stimulating factor (GM-CSF) was from Kingfisher Biotech, Inc. (Saint Paul, MN, USA); heat-inactivated fetal bovine serum (FBS) and Roswell Park Memorial Institute (RPMI) 1640 Medium were from Thermo Fisher Scientific (Waltham, MA, USA); Hank's Balanced Salt Solution (HBSS) was from Fisher Scientific (Hampton, NH, USA).
Crystalline WFA was dissolved in anhydrous DMSO, producing a 35.4 mM WFA stock solution that was aliquoted and stored at −20°C. WFA treatment solutions were prepared fresh each experiment by diluting WFA stock in the assay media. The treatment vehicle control (VC) was equivalent to the concentration of DMSO in the highest concentration of WFA for each assay.
University-owned horses at North Carolina State University were blood donors for this study. All horses were considered healthy based on physical examination and history. The blood collection protocol was approved by the North Carolina State University Institutional Animal Care and Use Committee (IACUC protocol #19-779).
Neutrophils were isolated from equine whole blood as previously described (74, 75). Briefly, heparinized whole blood was collected via jugular venipuncture. Whole blood was placed into sterile conical tubes for 1 h at room temperature to allow erythrocyte sedimentation. Leukocyte-rich plasma was layered on top of Ficoll-Paque Plus (GE Healthcare, Sweden) in a 2:1 ratio and centrifuged at 610 × g for 20 min. Residual erythrocytes were removed from the neutrophil pellet via hypotonic lysis, and the neutrophil concentration, viability (≥98%), and purity (≥95%) were established using a hemocytometer and trypan blue stain. Neutrophils were resuspended in assay media as described.
Isolated neutrophils were resuspended in HBSS and loaded with calcein-AM (2 μg/mL; Fisher Scientific) for 30 min at room temperature prior to resuspension in HBSS containing 1 mM CaCl2, 1 mM MgCl2, and 5% FBS (HBSS++ + 5% FBS). Neutrophils (1 x 105) and treatment solutions (WFA, VC, or media) were combined in wells of high binding plates coated with FBS and allowed to settle for 10 min at 37°C. Neutrophils were stimulated with interleukin-8 (IL-8; 100 ng/mL), leukotriene B4 (LTB4; 100 nM), platelet activating factor (PAF; 100 nM), phorbol 12-myristate 13-acetate (PMA; 10 ng/mL), or respective stimulus controls (IL-8 & PMA: HBSS; LTB4 & PAF: 0.03% EtOH) and incubated for 3 min (IL-8, LTB4, PAF) or 30 min (PMA) at 37°C. For insoluble immune complex (IIC) stimulation, BSA-coated plates were incubated with rabbit anti-BSA antibody for 2 h at 37°C to reach IIC densities of 5 μg and 20 μg per well. Unstimulated wells were coated only with 100 μg/mL BSA. The plates were washed to remove non-immobilized IIC, and neutrophils (1 x 105) and treatment solutions were combined in wells and incubated for 30 min at 37°C. All treatment/stimulation conditions were performed in triplicate. Fluorescence was measured at the end of the incubation period using a plate reader (FilterMax F5, Molecular Devices) and in between serial washing of the wells with HBSS. Percent adhesion was calculated by dividing fluorescence after washing by the initial fluorescence, and the wash at which adhesion of media control, unstimulated neutrophils was approximately 10% was used for data analysis.
Neutrophils were loaded with calcein-AM and resuspended in HBSS++ + 2% FBS as described for adhesion assays and pre-treated with WFA, VC, or media for 30 min at 37°C. Chemoattractants (IL-8 [100 ng/mL], LTB4 [10 nM], or PAF [100 nM]), chemoattractant controls (HBSS, 0.003% EtOH, or 0.03% EtOH, respectively), or media were added to the lower wells of a ChemoTx system (Neuro Probe) and overlaid by a membrane with 5 μm pores. Pre-treated neutrophils (1 x 104 for IL-8, 4 x 104 for LTB4 or PAF) were loaded on top of the membrane. All treatment/stimulation conditions were performed in triplicate. Control (100% migration) wells consisted of the same number of neutrophils placed directly into the lower wells, representative of the fluorescence if all the neutrophils had migrated. After incubation for 1 h at 37°C, non-migrated cells remaining above the membrane were removed using a scraper. EDTA (0.5 M; Thermo Fisher Scientific) was added to membranes above each well and incubated for 10 min at room temperature before centrifugation of the plate for 5 min at 100 × g. The membrane was removed, and fluorescence of each microplate well was determined using a plate reader. Percent migration was calculated by dividing fluorescence of experiment wells by the mean fluorescence of 100% migration control wells.
Neutrophils were resuspended in HBSS++ + 5% FBS and pre-treated with WFA, VC, or media for 30 min at 37°C. Where indicated, priming with GM-CSF (1 ng/mL) was performed during the pre-treatment period. Pre-treated neutrophils (1 x 105 for PMA, 3 x 105 for GM-CSF/LPS) were plated in FBS-coated wells, and luminol sodium salt (1 mM) was added to each well. Once stimuli (100 ng/mL LPS or 100 ng/mL PMA) or the appropriate stimulus control (HBSS or 0.0006% DMSO, respectively) had been added to each well, the plate was incubated at 37°C in a plate reader, and luminescence values were recorded every 5 min (t = 0–90 min). In separate experiments, pre-treated neutrophils (3 x 105) were added to wells coated with 5 μg insoluble immune complexes (IIC composed of BSA/anti-BSA antibodies; wells coated with 100 μg/mL BSA alone served as unstimulated conditions). These plates were also incubated at 37°C with luminescence values recorded every 5 min for 90 min. The timepoint at which maximum luminescence of media control, stimulated neutrophils was determined and used to establish the timepoint for data analysis for each stimuli: GM-CSF/LPS: 40 min, PMA: 60 min, IIC: 30 min (Supplementary Figure S1) (74). Luminescence values for WFA-treated neutrophils were expressed relative to the luminescence value of neutrophils treated with VC, yielding percent inhibition of respiratory burst relative to treatment VC. The effect of WFA on the overall respiratory burst was also evaluated by calculating the area under the curve (AUC) during the 90-min luminescence monitoring period.
Isolated neutrophils resuspended (1 x 106/mL) in RPMI with 10% FBS, 100 U/mL penicillin, and 100 μg/mL streptomycin were simultaneously treated with WFA, VC, or media and primed with GM-CSF (50 ng/mL) or left unprimed. Primed and unprimed neutrophils were also treated with staurosporine (1 μM) as a positive control for induction of neutrophil apoptosis (76). After 2- or 24-hours incubation at 37°C/5% CO2, neutrophils were washed with cold HBSS and stained with Alexa Fluor® 488 annexin V and propidium iodide (PI) according to manufacturer instructions (Invitrogen, Thermo Fisher Scientific). Cells were analyzed via flow cytometry (CytoFLEX, Beckman Coulter), and unstained samples were used to gate neutrophils based on size (forward scatter) and granularity (side scatter). Quadrants were defined using cells singly stained with annexin V or PI. Populations of live (annexin V−/PI−), apoptotic (annexin V+/PI−), and dead (annexin V+/PI+) neutrophil singlets were quantified based upon at least 10,000 events. Post-acquisition data analysis was performed using commercial software (FlowJo v. 10.7.1). Raw percentages of neutrophil viability, apoptosis, and death are available in Supplementary Figures S2, S3. For data analysis, the percentages of live, apoptotic, or dead neutrophils were expressed relative to media control, unprimed neutrophils.
For functional assays, one-tailed paired t-tests were performed to compare VC-treated neutrophils under stimulated vs. unstimulated conditions. Withaferin A treatment groups and the media control group were compared to the VC group within each stimulation condition using one-way repeated measures ANOVA with post hoc Holm–Šidák multiple comparisons. The WFA concentration at which 50% inhibition of each function was achieved relative to the VC (IC50) was determined using commercial software based upon four parameter nonlinear fit.
For apoptosis/viability data, apoptosis or viability of media control neutrophils under GM-CSF-primed conditions was compared to that of media control, unprimed neutrophils using one-tailed paired t-tests. Results from WFA-, VC-, or staurosporine positive control-treated neutrophils were compared to findings from media control neutrophils within each priming condition using one-way repeated measures ANOVA with post hoc Holm–Šidák multiple comparisons. All analyses described in this section were performed using GraphPad Prism v. 9.3.0. Significance was set at p < 0.05 for all analyses.
Adhesion of circulating neutrophils to endothelial ligands is a critical initial event in neutrophilic inflammation. Using a modified protocol previously established by our laboratory, we evaluated the effect of WFA on neutrophil static adhesion induced by IL-8, LTB4, PAF, PMA, or IIC (74, 75). Each stimulus induced significant adhesion of media control neutrophils compared to unstimulated conditions (Figure 1). Treatment of neutrophils with WFA 10 min prior to stimulation caused a concentration-dependent inhibition of adhesion across all five stimuli relative to the neutrophils treated with VC (Figure 1). At the highest concentrations of WFA tested for each stimulus, percent adhesion of stimulated neutrophils approximated the percent adhesion of unstimulated, media control neutrophils. The adhesion IC50 values ranged from 6.8 to 21.3 μM (IL-8: 6.8 μM, LTB4: 7.5 μM, PAF: 9.1 μM, PMA: 12.4 μM, high density IIC: 18.5, low density IIC 21.3 μM). There was no significant effect of WFA on adhesion of unstimulated neutrophils. There was a small but statistically significant difference between percent adhesion of VC-treated neutrophils (78 ± 2.1%) compared to media control (71 ± 4.8%) with PMA stimulation. This was not observed with the other stimuli.
Figure 1. Withaferin A inhibits neutrophil adhesion to substrate in response to PMA, insoluble immune complexes (IIC), IL-8, LTB4, and PAF. Mean (SEM) percent adhesion of treated equine neutrophils to 5% FBS-coated plastic with IL-8 [(A) n = 4 horses], LTB4 [(B) n = 5 horses], PAF [(B) n = 5 horses], or PMA [(C) n = 5 horses] stimulation or to IIC immobilized to plastic [(D) n = 5 horses]. Fluorescent detection of calcein AM-loaded neutrophils after serial washes. One-tailed paired t-test of VC neutrophils; ##p < 0.01, ###p < 0.001, ####p < 0.0001 compared to unstimulated VC. One-way repeated measures ANOVA (Holm-Sidak multiple comparison testing); *p < 0.05, **p < 0.01, ***p < 0.001, as compared to VC within same stimulation condition.
Next, we investigated the effect of WFA on neutrophil migration. The capacity of neutrophils to migrate toward chemoattractants was assessed using a commercial chemotaxis system and fluorescently-labeled neutrophils (74, 75). Withaferin A pretreatment of equine neutrophils significantly suppressed migration toward IL-8, LTB4, and PAF compared to pretreatment with VC (Figure 2). The magnitude of migration inhibition was marked, with concentrations of WFA as low as 5 μM suppressing percent migration of stimulated neutrophils below that of unstimulated, VC- or media-treated neutrophils. Migration IC50 values for IL-8, LTB4, and PAF stimulation were 2.5 μM, 2.9 μM, and 1.0 μM, respectively. Withaferin A also decreased neutrophil migration in the absence of stimulation relative to the VC treatment (Figures 2B,C). There were no differences between migration of VC or media control neutrophils under any stimulation condition.
Figure 2. Withaferin A inhibits neutrophil migration toward IL-8, LTB4, and PAF. Mean (SEM) percent migration of treated equine neutrophils toward IL-8 [(A) n = 5 horses], LTB4 [(B) n = 4 horses], or PAF [(C) n = 4 horses]. Fluorescent detection of calcein AM-loaded neutrophils relative to 100% migration control wells. One-tailed paired t-test of VC neutrophils; ##p < 0.01 compared to unstimulated VC. One-way repeated measures ANOVA (Holm-Sidak multiple comparison testing); *p < 0.05, **p < 0.01, as compared to VC within same stimulation condition.
After demonstrating that WFA interferes with key steps corresponding to neutrophil extravasation, we next sought to determine the effect of WFA on production of reactive oxygen species, a major contributor to neutrophil-mediated host cytotoxicity. We quantified the production of reactive oxygen species via luminol-enhanced chemiluminescence (74). Results were expressed as percent inhibition relative to VC-treated neutrophils under each stimulation condition. Figure 3 demonstrates a concentration-dependent inhibition of respiratory burst by WFA compared to the treatment VC for all stimulation conditions. Respiratory burst IC50 values for GM-CSF/LPS, PMA, and IIC stimulation were 0.9 μM, 6.5 μM, and 6.5 μM, respectively. Withaferin A also significantly reduced the luminescence AUC in PMA- and IIC-stimulated neutrophils compared to vehicle control (Supplementary Figures S4A,B). The decrease in luminescence AUC in WFA-treated, GM-CSF/LPS-stimulated neutrophils did not reach significance due to variability in vehicle control luminescence magnitude between independent experiments (Supplementary Figure S4C). Production of reactive oxygen species was low in unstimulated neutrophils and was not significantly affected by WFA (Supplementary Figures S1, S4). There was no significant difference between respiratory burst of VC and media control neutrophils under stimulated conditions (Supplementary Figure S4).
Figure 3. Withaferin A inhibits neutrophil respiratory burst stimulated by GM-CSF/LPS, PMA, and insoluble immune complexes (IIC). Mean (SEM) percent difference in mean luminescence relative to VC in WFA-treated equine neutrophils post-stimulation with GM-CSF/LPS (circles, 40 min, n = 6 horses), PMA (squares, 60 min, n = 5 horses), or IIC (diamonds, 30 min, n = 5 horses). Luminol-enhanced chemiluminescence detection of reactive oxygen species. One-way repeated measures ANOVA (Holm-Sidak multiple comparison testing); *p < 0.05, **p < 0.01, ***p < 0.001, as compared to 0% difference from VC.
Next, we investigated the possibility of cell death or apoptosis as an explanation for WFA inhibition of neutrophil functions. We incubated neutrophils with trypan blue at the end of respiratory burst assays: following 30 min of 50 μM WFA pretreatment and an additional 90 min of stimulation. Viability of treated neutrophils, as assessed by ability to exclude trypan blue dye, was consistently ≥98% (data not shown). This finding suggests that WFA treatment does not suppress neutrophil functions by causing neutrophil cell death. However, we recognize that compromise of plasma membrane is a relatively late event in the process of cell death, so inability to exclude trypan blue has limited sensitivity for evaluating cell viability. Trypan blue staining also does not identify cells in early apoptosis. Therefore, we used flow cytometry to assess neutrophil viability using a more stringent definition of live neutrophils (annexin V−/propidium iodide−) and to quantify apoptotic neutrophils (annexin V+/propidium iodide−). After 2 h incubation in the presence or absence of GM-CSF priming, the percentage of live neutrophils was not different in WFA-treated cells (0.1–25 μM WFA) compared to media control-treated cells (Figure 4A). Similarly, 2-h treatment with WFA did not significantly increase apoptosis nor cell death relative to media control in GM-CSF-primed or unprimed neutrophils (Figure 4B, Supplementary Figure S5).
Figure 4. Withaferin A does not impair short-term neutrophil viability or cause rapid neutrophil apoptosis. Mean (SEM) percentage of live (A) and apoptotic (B) neutrophils relative to media control, unprimed neutrophils following 2-h treatment of equine neutrophils under GM-CSF-primed (n = 3 horses) or unprimed (n = 4 horses) conditions. Flow cytometry quantification of annexin V binding and propidium iodide staining. One-way repeated measures ANOVA (Holm-Sidak multiple comparison testing); *p < 0.05 compared to media control within same priming condition.
We next evaluated the effect of WFA on 24-h neutrophil apoptosis under primed or unprimed conditions. Exposure of media control neutrophils to GM-CSF for 24 h decreased neutrophil apoptosis by 25% relative to unprimed conditions, confirming the pro-survival effects of GM-CSF in our model (Figure 5, Supplementary Figure S6A). As expected, staurosporine (positive control) significantly increased the percentage of apoptotic cells compared to media treatment. Similarly, and consistent with our hypothesis, neutrophils exposed to GM-CSF and treated with 5 μM or 0.5 μM WFA had significantly higher rates of apoptosis compared to media control, suggesting a pro-apoptotic effect of WFA under these conditions (Figure 5). Interestingly, the effect of WFA or staurosporine on increasing neutrophil apoptosis did not reach significance in neutrophils that were not exposed to GM-CSF. Regardless of GM-CSF exposure, 24-h treatment with 25 μM WFA was associated with a significant reduction in apoptosis, reflective of an increased percentage of dead cells that were annexin V+/propidium iodide+ (Supplementary Figure S6B). The VC had no effect on neutrophil apoptosis under primed or unprimed conditions.
Figure 5. Withaferin A promotes apoptosis of primed neutrophils by 24 h. Mean (SEM) percentage of apoptotic neutrophils relative to media control, unprimed neutrophils following 24-h treatment of equine neutrophils under GM-CSF-primed or unprimed conditions (n = 6 horses). Paired t-test of media control neutrophils; #p < 0.05 compared to unprimed media control. One-way repeated measures ANOVA (Holm-Sidak multiple comparison testing); *p < 0.05, ****p < 0.0001 compared to media control within same priming condition.
Neutrophils are central to the pathophysiology of several common equine diseases, though current therapeutics that target neutrophilic inflammation are limited. Withaferin A demonstrates promise as a therapeutic agent for inflammatory diseases and mitigates pathology in animal models of neutrophil-mediated conditions. However, the effects of WFA on neutrophils have not been well described. For this study, our objectives were to determine the effects of WFA on equine neutrophil functions and apoptosis. Based on previously reported anti-inflammatory effects of WFA in neutrophil-mediated diseases, we hypothesized that WFA would inhibit adhesion, migration, and respiratory burst of equine neutrophils and would promote apoptosis of primed equine neutrophils.
For this project, we used multiple stimuli that utilize different signaling pathways to show that WFA significantly attenuates neutrophil adhesion, migration, and respiratory burst. Each of these neutrophil functions contributes to tissue damage, underscoring their relevance as potential therapeutic anti-inflammatory targets. These novel findings expand upon previous publications containing limited descriptions of WFA effects on leukocyte functions. Specifically, Che et al. reported that WFA inhibited Escherichia coli-induced neutrophil migration (58), while Sabina et al. showed decreased in vitro degranulation of WFA-treated human neutrophils (45). Lee et al. showed that WFA treatment of cultured primary human endothelium suppressed adhesion and migration of monocytes (THP-1), which the authors attributed to reduced expression of endothelial cell adhesion molecules (77). Finally, while our report is the first to examine the effect of WFA on neutrophil adhesion and respiratory burst, a withanolide compound with similar structure to WFA was shown to inhibit neutrophil respiratory burst stimulated by combined N-formyl-methionyl-leucyl-phenyl-alanine/cytochalasin B (78).
Once isolated, the majority of neutrophils undergo constitutive apoptosis in vitro within 24 h (23, 79), mirroring the short in vivo lifespan of blood neutrophils in healthy subjects (21). However, exposure to GM-CSF, which occurs during many inflammatory conditions in vivo, reduces the rate of apoptosis and prolongs neutrophil survival (23). Therefore, 24-hour incubation of neutrophils with GM-CSF represents a physiologically relevant in vitro model for delayed neutrophil apoptosis under inflammatory conditions. The additional 2-hour time point for apoptosis experiments served two purposes. First, it allowed us to better understand the timeline of WFA's effects on neutrophil apoptosis. Second, it helped to validate the trypan blue viability data from our functional assays.
Consistent with previous reports, in this study, exposure to GM-CSF for 24 h reduced neutrophil apoptosis and enhanced neutrophil survival (23, 80, 81). Withaferin A treatment significantly increased apoptosis in primed neutrophils, mitigating the pro-survival effects of GM-CSF. The promotion of neutrophil apoptosis by WFA stands in contrasts to the anti-apoptotic effect of glucocorticoids on neutrophils (82, 83), which is often cited as a downside to glucocorticoid therapy (84, 85). Interestingly, in unprimed neutrophils, WFA did not have a significant pro-apoptotic effect. This finding suggests that WFA promotion of neutrophil apoptosis was mediated through inhibitory effects on anti-apoptotic/pro-survival signaling that occurs in primed neutrophils, rather than direct action on apoptotic machinery. Based on interpretation of our data, we further hypothesize that WFA interferes with pro-inflammatory signaling pathways that antagonize constitutive neutrophil apoptosis, facilitating a pro-apoptotic balance. This potential mechanism offers a plausible explanation of the differential effects of WFA on apoptosis of neutrophils and tumor cells (pro-apoptotic) (60, 61, 65, 66) vs. most non-neoplastic cells (anti-apoptotic) (70–73).
Withaferin A had no significant effect on neutrophil apoptosis at 2 h, regardless of GM-CSF exposure. This finding suggests that the overall pro-apoptotic effects of WFA in neutrophils are not rapid in onset. This would fit with a proposed mechanism of interference with anti-apoptotic signaling as opposed to direct activation of caspases. These data also exclude apoptosis as an explanation for our demonstrated suppression of neutrophil function. In accordance with the lack of effect of WFA on neutrophil apoptosis after 2 h, we also found that viability of WFA-treated neutrophils was not significantly different than media control at this timepoint. Taken together with neutrophil function data, we have established that WFA inhibition of neutrophil adhesion, migration, and respiratory burst is not mediated through a decrease in neutrophil viability.
The cellular mechanisms underlying WFA's effects on neutrophil inflammatory functions and apoptosis are currently unknown. Our 2-h flow cytometry viability data indicate that WFA suppression of multiple neutrophil functions is not attributable to cell death or apoptosis. The marked suppression of all neutrophil functions evaluated in this study provides further insight into potential mechanisms of action of WFA in neutrophils. Universal inhibition of neutrophil adhesion, migration, and respiratory burst suggests that WFA is preventing one or more events that are central to overall neutrophil activation, such as activation of β2 integrins on the neutrophil surface, reorganization of actin cytoskeletal structure, and/or regulation of intracellular calcium (12). Additionally, WFA's ability to suppress neutrophil responses to multiple stimuli also informs possible mechanisms of action. The diverse stimuli utilized in our functional assays signal through multiple cell signaling pathways. Therefore, the ability of WFA to inhibit responses to a variety of stimuli suggests that WFA is interfering with cell signaling that is essential for all of these methods of neutrophil stimulation.
There are several reported mechanisms of action for WFA effects in other cell types. Described mechanisms that are potentially relevant to our findings include inhibition of phosphorylation of major kinases and interference with nuclear factor kappa B (NF-κB) signaling (86–91). One or more mitogen-activated protein kinases (MAPKs), including extracellular signal-regulated kinase (ERK1/2), p38 MAPK, and/or c-JUN N-terminal kinase (JNK), are phosphorylated following priming of neutrophils with GM-CSF (92) or stimulation with IL-8 (93), LTB4 (94), PAF (95), PMA (96), LPS (97), and IIC (98). Many of these stimuli also trigger phosphorylation of Akt in neutrophils (98–102). Although the relative involvement of the different MAPKs and Akt in neutrophil functions are stimulus-dependent, all four are known to play a role in neutrophil adhesion, migration, and/or respiratory burst, as evidenced by decreases in neutrophil functionality after treatment with specific inhibitors of ERK1/2, p38 MAPK, JNK, and/or Akt (94, 103–105). Our laboratory and other groups have confirmed roles of these signaling pathways in equine neutrophil functions (94, 106, 107). Delayed neutrophil apoptosis during inflammation is mediated through ERK1/2 (81, 108), Akt (81, 108–110), and NF-κB (110) signaling pathways. Withaferin A interference with these signaling molecules could explain the increased apoptosis we observed in GM-CSF-primed neutrophils. While there is clear evidence from other cell types that WFA interferes with signaling molecules that regulate neutrophil function and lifespan, it is important to note that conflicting data (i.e., WFA enhancement of kinase activation) has also been reported in both neoplastic and nonneoplastic cells (49, 70, 111). These discrepancies underscore the need for additional work to determine the effects of WFA on these molecular targets in neutrophils and their relation to our findings presented here.
We recognize several limitations of this study. First, neutrophils used in the functional assays were pretreated with WFA for 10 min (adhesion experiments) or 30 min (migration and respiratory burst experiments) prior to stimulation. This timeline does not replicate clinical conditions in which WFA may be a valuable therapeutic, since treatment administration prior to the onset of inflammation is generally not feasible for acute diseases. However, it is important to note that inflammatory events, particularly those involving dysregulated neutrophils responses, are cyclical, with initial neutrophilic inflammation contributing to tissue damage that often recruits additional neutrophils. In these situations, anti-inflammatories such as WFA might be useful for “interrupting” a dysregulated cycle of inflammation. Additionally, our 24-h apoptosis findings suggest that WFA may actively promote resolution of existing inflammation. In other scenarios, particularly for diseases that are chronic with episodes of exacerbation, WFA could be used in a chemopreventive capacity to reduce inflammatory flares. Second, our experiments were conducted with isolated neutrophils containing a low percentage of other leukocyte populations. While this reductionist approach is essential for examining direct effects of WFA on neutrophils to better understand mechanisms, it ignores the effects of WFA on other cell types (e.g., leukocytes, epithelium, endothelium, etc.) and the resulting complex interactions that may influence neutrophils indirectly. We plan to incorporate other relevant cell types into future experiments in preparation for subsequent in vivo WFA studies. Third, our population of dead neutrophils was defined by cells that were double positive for annexin V and propidium iodide staining and includes both late apoptotic and necrotic cells. We attempted to differentiate the two processes using a pan-caspase inhibitor, z-VAD-FMK, but none of the three concentrations of z-VAD-FMK previously reported to inhibit neutrophil apoptosis (20 μM, 50 μM, 100 μM z-VAD-FMK) (80, 102, 112) had an effect on the percentage of annexin V+/propidium iodide− neutrophils in our experiments (data not shown). Therefore, we were unable to determine whether the higher percentage of dead neutrophils following 25 μM WFA treatment for 24 h was reflective of an earlier onset and/or more rapid progression of apoptosis leading to secondary loss of membrane integrity or whether the 25 μM WFA caused necrosis rather than apoptosis. Since necrosis is an inherently pro-inflammatory process, understanding the potential of this higher concentration of WFA to cause necrosis of neutrophils is relevant to its future clinical use. Finally, we base our assertion that WFA does not compromise short-term neutrophil viability on flow cytometry data from neutrophils simultaneously treated with WFA and primed with GM-CSF or left unprimed for 2 h. The 2-h treatment time exceeds the length of exposure to WFA during our functional assays (total WFA exposure ranged from 40 to 90 min). However, we acknowledge the possibility that stimuli used in the functional assays may affect impact of WFA on neutrophil viability. We repeated apoptosis/cell death flow cytometry experiments using similar conditions as adhesion and respiratory burst assays, including a 30-min WFA pretreatment period followed by stimulation with PMA or GM-CSF/LPS or no stimulation (30–60 min). There was no evidence that WFA reduced neutrophil viability under conditions used in functional assays (data not shown).
Here we report exciting in vitro results that provide proof of principle evidence for WFA as a novel anti-inflammatory strategy for neutrophil-mediated diseases. We have shown that WFA inhibits neutrophil adhesion, migration, and respiratory burst across a wide range of stimuli, including those that are relevant to inflammatory diseases, through a mechanism that does not impair short-term neutrophil viability. We also demonstrate that WFA promotes timely apoptosis of GM-CSF-primed neutrophils, a rare example of pro-apoptotic effects of WFA in non-neoplastic cells. The combination of these effects may have clinical benefit in reducing inflammation and hastening resolution of inflammation. Further studies on WFA are needed to better understand the molecular mechanisms underlying these effects, confirm the favorable safety profile suggested by laboratory animal and human data (113, 114), and evaluate feasibility and optimal delivery in veterinary species and humans.
The raw data supporting the conclusions of this article will be made available by the authors, without undue reservation.
The animal study was reviewed and approved by North Carolina State University Institutional Animal Care and Use Committee (IACUC protocol #19-779).
RB: conceptualization, study design, funding acquisition, study execution, data analysis and interpretation, original draft preparation, manuscript review and editing, and final approval. MS: supervision, data interpretation, manuscript review and editing, and final approval. SJ: conceptualization, study design, funding acquisition, supervision, data interpretation, manuscript review and editing, and final approval. All authors contributed to the article and approved the submitted version.
Stipend support for RB and partial funding for study supplies were provided by the NIH T32 Ruth Kirschstein Institutional National Research Service Award (NRSA) Training Grant (NIH T32 OD011130). Other sources of funding for experiments included the North Carolina State University College of Veterinary Medicine Competitive Research Grants Program and the North Carolina State University Comparative Medicine Institute Associate Member Flash Grant Program.
The authors declare that the research was conducted in the absence of any commercial or financial relationships that could be construed as a potential conflict of interest.
All claims expressed in this article are solely those of the authors and do not necessarily represent those of their affiliated organizations, or those of the publisher, the editors and the reviewers. Any product that may be evaluated in this article, or claim that may be made by its manufacturer, is not guaranteed or endorsed by the publisher.
We would like to thank Jenna Schirmer and staff at the North Carolina State University Teaching Animal Unit and Reedy Creek Equine Farm for assistance with equine blood collection. Flow Cytometry experiments were performed in the Flow Cytometry and Cell Sorting facility at North Carolina State University-College of Veterinary Medicine and we appreciate flow cytometry assistance and training from Javid Mohammed.
The Supplementary Material for this article can be found online at: https://www.frontiersin.org/articles/10.3389/fvets.2022.900453/full#supplementary-material
Supplementary Figure S1. Kinetics of neutrophil respiratory burst are stimulus dependent. Mean (SEM) percent luminescence of media control neutrophils stimulated with GM-CSF/LPS [(A) circles, n = 6 horses], PMA [(B) squares, n = 5 horses], or IIC [(C) diamonds, n = 5 horses] or appropriate stimulus controls (A–C) black diamonds), relative to maximum luminescence of stimulated neutrophils during assay time course. Luminol-enhanced chemiluminescence detection of reactive oxygen species. Vertical dashed line indicates time point selected to compare effect of treatments under each stimulation condition. One-tailed paired t-test at selected time point; ####p < 0.0001 compared to unstimulated control.
Supplementary Figure S2. Raw percentages of neutrophil viability, apoptosis, death after 2-h incubation. Mean (SEM) percentages of live (A), apoptotic (B) and dead (C) neutrophils following 2-h treatment of equine neutrophils under GM-CSF-primed (n = 3 horses) or unprimed (n = 4 horses) conditions. Flow cytometry quantification of annexin V binding and propidium iodide staining. No statistical analyses performed on raw data.
Supplementary Figure S3. Raw percentages of neutrophil viability, apoptosis, death after 24-h incubation. Mean (SEM) percentages of live (A), apoptotic (B) and dead (C) neutrophils following 24-h treatment of equine neutrophils under GM-CSF-primed or unprimed (n = 6 horses) conditions. Flow cytometry quantification of annexin V binding and propidium iodide staining. No statistical analyses performed on raw data.
Supplementary Figure S4. Withaferin A decreases overall respiratory burst in neutrophils stimulated with PMA or insoluble immune complexes (IIC). Mean (SEM) luminescence area under the curve (AUC) in neutrophils pretreated with WFA, VC, or media control under PMA [(A) n = 5 horses], IIC [(B) n = 5 horses], or GM-CSF/LPS [(C) n = 6 horses] stimulation. Luminol-enhanced chemiluminescence detection of reactive oxygen species for 90 minutes post-stimulation. One-tailed paired t-test of VC neutrophils; ###p < 0.001 compared to unstimulated VC. One-way repeated measures ANOVA (Holm-Sidak multiple comparison testing); *p < 0.05, **p < 0.01, as compared to VC within same stimulation condition.
Supplementary Figure S5. Withaferin A does not cause rapid neutrophil cell death. Mean (SEM) percentage of dead neutrophils relative to media control, unprimed neutrophils following 2-h treatment of equine neutrophils under GM-CSF-primed (n = 3 horses) or unprimed (n = 4 horses) conditions. Flow cytometry quantification of annexin V binding and propidium iodide staining. Paired t-test of media control neutrophils; no significant difference between GM-CSF-primed and unprimed media control neutrophils. One-way repeated measures ANOVA (Holm-Sidak multiple comparison testing); no significant difference between treatments and media control within same priming condition.
Supplementary Figure S6. Higher concentrations of Withaferin A decrease neutrophil viability and increase neutrophil death by 24 h. Mean (SEM) percentage of live (A) and dead (B) neutrophils relative to media control, unprimed neutrophils following 24-h treatment of equine neutrophils under GM-CSF-primed or unprimed (n = 6 horses) conditions. Flow cytometry quantification of annexin V binding and propidium iodide staining. Paired t-test of media control neutrophils; #p < 0.05, ####p < 0.0001 compared to unprimed media control. One-way repeated measures ANOVA (Holm-Sidak multiple comparison testing); *p < 0.05, **p < 0.01, ***p < 0.001 compared to media control within same priming condition.
1. Uberti B, Morán G. Role of neutrophils in equine asthma. Anim Heal Res Rev. (2018) 19:65–73. doi: 10.1017/S146625231800004X
2. Davis KU, Sheats MK. The role of neutrophils in the pathophysiology of asthma in humans and horses. Inflammation. (2021) 44:450–65. doi: 10.1007/s10753-020-01362-2
3. de la Rebière de Pouyade G, Serteyn D. The role of activated neutrophils in the early stage of equine laminitis. Vet J. (2011) 189:27–33. doi: 10.1016/j.tvjl.2010.06.008
4. Leise B. The role of neutrophils in equine laminitis. Cell Tissue Res. (2018) 371:541–50. doi: 10.1007/s00441-018-2788-z
5. Gayle JM, Blikslager AT, Jones SL. Role of neutrophils in intestinal mucosal injury. J Am Vet Med Assoc. (2000) 217:498–500. doi: 10.2460/javma.2000.217.498
6. Moore RM, Bertone AL, Bailey MQ, Muir WW, Beard WL. Neutrophil accumulation in the large colon of horses during low-flow ischemia and reperfusion. Am J Vet Res. (1994) 55:1454–63.
7. Brown K, Brain S, Pearson J, Edgeworth J, Lewis S, Treacher D. Neutrophils in development of multiple organ failure in sepsis. Lancet. (2006) 368:157–69. doi: 10.1016/S0140-6736(06)69005-3
8. Gifford AM, Chalmers JD. The role of neutrophils in cystic fibrosis. Curr Opin Hematol. (2014) 21:16–22. doi: 10.1097/MOH.0000000000000009
9. Bhatia M, Saluja AK, Hofbauer B, Lee HS, Frossard JL, Steer ML. The effects of neutrophil depletion on a completely noninvasive model of acute pancreatitis-associated lung injury. Int J Pancreatol. (1998) 24:77–83. doi: 10.1007/BF02788564
10. Johnson KC, Mackin A. Canine immune-mediated polyarthritis. J Am Anim Hosp Assoc. (2012) 48:12–7. doi: 10.5326/JAAHA-MS-5756
11. Slocombe R, Malark J, Ingersoll R, Derksen F, NE R. Importance of neutrophils in the pathogenesis of acute pneumonic pasteurellosis in calves. Am J Vet Res. (1985) 46:2253–8.
12. Bouti P, Webbers SDS, Fagerholm SC, Alon R, Moser M, Matlung HL, et al. β2 integrin signaling cascade in neutrophils: more than a single function. Front Immunol. (2021) 11:619925. doi: 10.3389/fimmu.2020.619925
13. Berton G, Yan SR, Fumagalli L, Lowell CA. Neutrophil activation by adhesion: mechanisms and pathophysiological implications. Int J Clin Lab Res. (1996) 26:160–77. doi: 10.1007/BF02592978
14. Brazil JC, Parkos CA. Pathobiology of neutrophil–epithelial interactions. Immunol Rev. (2016) 273:94–111. doi: 10.1111/imr.12446
15. Gayle J, Jones SL, Argenzio RA, Blikslager AT. Neutrophils increase paracellular permeability of restituted ischemic-injured porcine ileum. Surgery. (2002) 132:461–70. doi: 10.1067/msy.2002.125320
16. Nash S, Stafford J, Madara JL. Effects of polymorphonuclear leukocyte transmigration on the barrier function of cultured intestinal epithelial monolayers. J Clin Invest. (1987) 80:1104–13. doi: 10.1172/JCI113167
17. Nusrat A, Parkos CA, Liang TW, Carnes DK, Madara JL. Neutrophil migration across model intestinal epithelia: monolayer disruption and subsequent events in epithelial repair. Gastroenterology. (1997) 113:1489–500. doi: 10.1053/gast.1997.v113.pm9352851
18. Kantrow SP, Shen Z, Jagneaux T, Zhang P, Nelson S. Neutrophil-mediated lung permeability and host defense proteins. Am J Physiol - Lung Cell Mol Physiol. (2009) 297:738–45. doi: 10.1152/ajplung.00045.2009
19. van der Linden M, Meyaard L. Fine-tuning neutrophil activation: strategies and consequences. Immunol Lett. (2016) 178:3–9. doi: 10.1016/j.imlet.2016.05.015
20. McCracken JM, Allen L-AH. Regulation of human neutrophil apoptosis and lifespan in health and disease. J Cell Death. (2014) 7:15–23. doi: 10.4137/JCD.S11038
21. Lahoz-Beneytez J, Elemans M, Zhang Y, Ahmed R, Salam A, Block M, et al. Human neutrophil kinetics: modeling of stable isotope labeling data supports short blood neutrophil half-lives. Blood. (2016) 127:3431–8. doi: 10.1182/blood-2016-03-700336
22. Lee A, Whyte MKB, Haslett C. Inhibition of apoptosis and prolongation of neutrophil functional longevity by inflammatory mediators. J Leukoc Biol. (1993) 54:283–8. doi: 10.1002/jlb.54.4.283
23. Kobayashi SD, Voyich JM, Whitney AR, DeLeo FR. Spontaneous neutrophil apoptosis and regulation of cell survival by granulocyte macrophage-colony stimulating factor. J Leukoc Biol. (2005) 78:1408–18. doi: 10.1189/jlb.0605289
24. Kobayashi SD, Voyich JM, Braughton KR, DeLeo FR. Down-regulation of proinflammatory capacity during apoptosis in human polymorphonuclear leukocytes. J Immunol. (2003) 170:3357–68. doi: 10.4049/jimmunol.170.6.3357
25. Whyte MK, Meagher LC, MacDermot J, Haslett C. Impairment of function in aging neutrophils is associated with apoptosis. J Immunol. (1993) 150:5124–34.
26. Fadok VA, Bratton DL, Konowal A, Freed PW, Westcott JY, Henson PM. Macrophages that have ingested apoptotic cells in vitro inhibit proinflammatory cytokine production through autocrine/paracrine mechanisms involving TGF-β, PGE2, and PAF. J Clin Invest. (1998) 101:890–8. doi: 10.1172/JCI1112
27. Krista KM, White NA, Barrett JG, Furr MO, Buechner-Maxwell VA. Evaluation of neutrophil apoptosis in horses with acute abdominal disease. Am J Vet Res. (2013) 74:999–1004. doi: 10.2460/ajvr.74.7.999
28. Niedzwiedz A, Jaworski Z, Tykalowski B, Smialek M. Neutrophil and macrophage apoptosis in bronchoalveolar lavage fluid from healthy horses and horses with recurrent airway obstruction (RAO). BMC Vet Res. (2014) 10:29. doi: 10.1186/1746-6148-10-29
29. Anderson SL, Singh B. Neutrophil apoptosis is delayed in an equine model of colitis: implications for the development of systemic inflammatory response syndrome. Equine Vet J. (2017) 49:383–8. doi: 10.1111/evj.12576
30. Taneja R, Parodo J, Song HJ, Kapus A, Rotstein OD, Marshall JC. Delayed neutrophil apoptosis in sepsis is associated with maintenance of mitochondrial transmembrane potential and reduced caspase-9 activity. Crit Care Med. (2004) 32:1460–9. doi: 10.1097/01.CCM.0000129975.26905.77
31. Jimenez MF, Watson RWG, Parodo J, Evans D, Foster D, Steinberg M, et al. Dysregulated expression of neutrophil apoptosis in the Systemic Inflammatory Response Syndrome. Arch Surg. (1997) 132:1263–9. doi: 10.1001/archsurg.1997.01430360009002
32. McKeon D, Condliffe A, Cowburn A, Cadwallader K, Farahi N, Bilton D, et al. Prolonged survival of neutrophils from patients with DF508 CFTR mutations. Thorax. (2008) 63:660–1. doi: 10.1136/thx.2008.096834
33. Moriceau S, Lenoir G, Witko-Sarsat V. In cystic fibrosis homozygotes and heterozygotes, neutrophil apoptosis is delayed and modulated by diamide or roscovitine: evidence for an innate neutrophil disturbance. J Innate Immun. (2010) 2:260–6. doi: 10.1159/000295791
34. Uddin M, Nong G, Ward J, Seumois G, Prince LR, Wilson SJ, et al. Prosurvival activity for airway neutrophils in severe asthma. Thorax. (2010) 65:684–9. doi: 10.1136/thx.2009.120741
35. Jie H, He Y, Huang X, Zhou Q, Han Y, Li X, et al. Necrostatin-1 enhances the resolution of inflammation by specifically inducing neutrophil apoptosis. Oncotarget. (2016) 7:19367–81. doi: 10.18632/oncotarget.8346
36. Rossi AG, Sawatzky DA, Walker A, Ward C, Sheldrake TA, Riley NA, et al. Cyclin-dependent kinase inhibitors enhance the resolution of inflammation by promoting inflammatory cell apoptosis. Nat Med. (2006) 12:1056–64. doi: 10.1038/nm1468
37. Tsai YF, Chu TC, Chang WY, Wu YC, Chang FR, Yang SC, et al. 6-Hydroxy-5,7-dimethoxy-flavone suppresses the neutrophil respiratory burst via selective PDE4 inhibition to ameliorate acute lung injury. Free Radic Biol Med. (2017) 106:379–92. doi: 10.1016/j.freeradbiomed.2017.03.002
38. Liou KT, Shen YC, Chen CF, Tsao CM, Tsai SK. The anti-inflammatory effect of honokiol on neutrophils: mechanisms in the inhibition of reactive oxygen species production. Eur J Pharmacol. (2003) 475:19–27. doi: 10.1016/S0014-2999(03)02121-6
39. Tsai YF, Chen CY, Lin IW, Leu YL, Yang SC Syu YT, Chen PJ, et al. Imperatorin alleviates psoriasiform dermatitis by blocking neutrophil respiratory burst, adhesion, and chemotaxis through selective phosphodiesterase 4 inhibition. Antioxidants Redox Signal. (2021) 35:885–903. doi: 10.1089/ars.2019.7835
40. Nosál R, Drábiková K, Jančinová V, Mačičková T, Pečivová J, Perečko T, et al. Pharmacological intervention with oxidative burst in human neutrophils. Interdiscip Toxicol. (2017) 10:56–60. doi: 10.1515/intox-2017-0009
41. Behl T, Sharma A, Sharma L, Sehgal A, Zengin G, Brata R, et al. Exploring the multifaceted therapeutic potential of withaferin A and its derivatives. Biomedicines. (2020) 8:571. doi: 10.3390/biomedicines8120571
42. Gao S, Li H, Zhou X-Q, You J-B, Tu D-N, Xia G, et al. Withaferin A attenuates lipopolysaccharide-induced acute lung injury in neonatal rats. Cell Mol Biol. (2015) 61:102–6.
43. Kanak MA, Shahbazov R, Yoshimatsu G, Levy MF, Lawrence MC, Naziruddin B, et al. small molecule inhibitor of NFκB blocks ER stress and the NLRP3 inflammasome and prevents progression of pancreatitis. J Gastroenterol. (2017) 52:352–65. doi: 10.1007/s00535-016-1238-5
44. Tiruveedi VL, Bale S, Khurana A, Godugu C. Withaferin A, a novel compound of Indian ginseng (Withania somnifera), ameliorates cerulein-induced acute pancreatitis: possible role of oxidative stress and inflammation. Phyther Res. (2018) 32:2586–96. doi: 10.1002/ptr.6200
45. Sabina EP, Chandal S, Rasool MK. Inhibition of monosodium urate crystal-induced inflammation by withaferin A. J Pharm Pharm Sci. (2008) 11:46–55. doi: 10.18433/J35K58
46. Zhao H, Gao Z, Xie S, Han X, Sun Q. Withaferin A attenuates ovalbumin induced airway inflammation. Front Biosci. (2019) 24:576–96. doi: 10.2741/4737
47. Szarc Vel Szic K, Op De Beeck K, Ratman D, Wouters A, Beck IM, Declerck K, et al. Pharmacological levels of Withaferin A (Withania somnifera) trigger clinically relevant anticancer effects specific to triple negative breast cancer cells. PLoS ONE. (2014) 9:e87850. doi: 10.1371/journal.pone.0087850
48. Chung SS, Wu Y, Okobi Q, Adekoya D, Atefi M, Clarke O, et al. Proinflammatory cytokines IL-6 and TNF- α increased telomerase activity through NF- κ B/STAT1/STAT3 Activation, and withaferin A inhibited the signaling in colorectal cancer cells. Mediators Inflamm. (2017) 5958429. doi: 10.1155/2017/5958429
49. Kuppusamy P, Nagalingam A, Muniraj N, Saxena NK, Sharma D. Concomitant activation of ETS-like transcription factor-1 and Death Receptor-5 via extracellular signal-regulated kinase in withaferin A-mediated inhibition of hepatocarcinogenesis in mice. Sci Rep. (2017) 7:17943. doi: 10.1038/s41598-017-18190-4
50. Samadi AK, Mukerji R, Shah A, Timmermann BN, Cohen MS. A novel RET inhibitor with potent efficacy against medullary thyroid cancer in vivo. Surgery. (2010) 148:1228–36. doi: 10.1016/j.surg.2010.09.026
51. Grommes J, Soehnlein O. Contribution of neutrophils to acute lung injury. Mol Med. (2011) 17:293–307. doi: 10.2119/molmed.2010.00138
52. Abraham E, Carmody A, Shenkar R, Arcaroli J. Neutrophils as early immunologic effectors in hemorrhage- or endotoxemia-induced acute lung injury. Am J Physiol - Lung Cell Mol Physiol. (2000) 279:L1137–45. doi: 10.1152/ajplung.2000.279.6.L1137
53. Fujimoto K, Hosotani R, Doi R, Wada M, Lee JU, Koshiba T, et al. Role of neutrophils in cerulein-induced pancreatitis in rats: possible involvement of apoptosis. Digestion. (1997) 58:421–30. doi: 10.1159/000201478
54. Pastor CM, Vonlaufen A, Georgi F, Hadengue A, Morel P, Frossard JL. Neutrophil depletion-but not prevention of Kupffer cell activation-decreases the severity of cerulein-induced acute pancreatitis. World J Gastroenterol. (2006) 12:1219–24. doi: 10.3748/wjg.v12.i8.1219
55. So AK, Martinon F. Inflammation in gout: mechanisms and therapeutic targets. Nat Rev Rheumatol. (2017) 13:639–47. doi: 10.1038/nrrheum.2017.155
56. Reutershan J, Basit A, Galkina EV, Ley K. Sequential recruitment of neutrophils into lung and bronchoalveolar lavage fluid in LPS-induced acute lung injury. Am J Physiol - Lung Cell Mol Physiol. (2005) 289:L807–15. doi: 10.1152/ajplung.00477.2004
57. Chen CM, Chung YP, Liu CH, Huang KT, Guan SS, Chiang CK, et al. Withaferin A protects against endoplasmic reticulum stress-associated apoptosis, inflammation, and fibrosis in the kidney of a mouse model of unilateral ureteral obstruction. Phytomedicine. (2020) 79:153352. doi: 10.1016/j.phymed.2020.153352
58. Che X, Chi F, Wang L, Jong TD, Wu C-H, Wang X, et al. Involvement of IbeA in meningitic Escherichia coli K1-induced polymorphonuclear leukocyte transmigration across brain endothelial cells. Brain Pathol. (2011) 21:389–404. doi: 10.1111/j.1750-3639.2010.00463.x
59. Chang H, Li R, Wang H, Liu J, Tang J. Withaferin A induces oxidative stress-mediated apoptosis and DNA damage in oral cancer cells. Front Physiol. (2017) 8:634. doi: 10.3389/fphys.2017.00634
60. Hahm ER, Singh SV. Withaferin A-induced apoptosis in human breast cancer cells is associated with suppression of inhibitor of apoptosis family protein expression. Cancer Lett. (2013) 334:101–8. doi: 10.1016/j.canlet.2012.08.026
61. Xia S, Miao Y, Liu S. Withaferin A induces apoptosis by ROS-dependent mitochondrial dysfunction in human colorectal cancer cells. Biochem Biophys Res Commun. (2018) 503:2363–9. doi: 10.1016/j.bbrc.2018.06.162
62. Aliebrahimi S, Montasser S, Shahriar S, Shadboorestan A. Phytochemicals, withaferin A and carnosol, overcome pancreatic cancer stem cells as c-Met inhibitors. Biomed Pharmacother. (2018) 106:1527–36. doi: 10.1016/j.biopha.2018.07.055
63. Hahm ER, Moura MB, Kelley EE, van Houten B, Shiva S, Singh SV. Withaferin A-induced apoptosis in human breast cancer cells is mediated by reactive oxygen species. PLoS One. (2011) 6:e23354. doi: 10.1371/journal.pone.0023354
64. Malik F, Kumar A, Bhushan S, Khan S, Bhatia A, Suri KA, et al. Reactive oxygen species generation and mitochondrial dysfunction in the apoptotic cell death of human myeloid leukemia HL-60 cells by a dietary compound withaferin A with concomitant protection by N-acetyl cysteine. Apoptosis. (2007) 12:2115–33. doi: 10.1007/s10495-007-0129-x
65. Mayola E, Gallerne C, Esposti DD, Martel C, Pervaiz S, Larue L, et al. Withaferin A induces apoptosis in human melanoma cells through generation of reactive oxygen species and down-regulation of Bcl-2. Apoptosis. (2011) 16:1014–27. doi: 10.1007/s10495-011-0625-x
66. Liu X, Chen L, Liang T, Tian XD, Liu Y, Zhang T. Withaferin A induces mitochondrial-dependent apoptosis in non-small cell lung cancer cells via generation of reactive oxygen species. J BUON. (2017) 22:244–50.
67. Mandal C, Dutta A, Mallick A, Chandra S, Misra L, Sangwan RS, et al. Withaferin A induces apoptosis by activating p38 mitogen-activated protein kinase signaling cascade in leukemic cells of lymphoid and myeloid origin through mitochondrial death cascade. Apoptosis. (2008) 13:1450–64. doi: 10.1007/s10495-008-0271-0
68. Mehrotra A, Kaul D, Joshi K. LXR-α selectively reprogrammes cancer cells to enter into apoptosis. Mol Cell Biochem. (2011) 349:41–55. doi: 10.1007/s11010-010-0659-3
69. Guo R, Gan L, Lau WB, Yan Z, Lopez BL, Ma X, et al. Withaferin A prevents myocardial ischemia/reperfusion injury by upregulating AMP-activated protein kinase- dependent B-cell lymphoma2 signaling. Circ J. (2019) 83:1726–36. doi: 10.1253/circj.CJ-18-1391
70. Yan Z, Guo R, Gan L, Bond W, Cao X, Zhao J, et al. Withaferin A inhibits apoptosis via activated Akt-mediated inhibition of oxidative stress. Life Sci. (2018) 211:91–101. doi: 10.1016/j.lfs.2018.09.020
71. Tekula S, Khurana A, Anchi P, Godugu C. Withaferin-A attenuates multiple low doses of Streptozotocin (MLD-STZ) induced type 1 diabetes. Biomed Pharmacother. (2018) 106:1428–40. doi: 10.1016/j.biopha.2018.07.090
72. Zhao X, Wang J, Tang L, Li P, Ru J, Bai Y. Withaferin A protects against hyperuricemia induced kidney injury and its possible mechanisms. Bioengineered. (2021) 12:589–600. doi: 10.1080/21655979.2021.1882761
73. Yan X, Huang G, Liu Q, Zheng J, Chen H, Huang Q, et al. Withaferin A protects against spinal cord injury by inhibiting apoptosis and inflammation in mice. Pharm Biol. (2017) 55:1171–6. doi: 10.1080/13880209.2017.1288262
74. Martin EM, Till RL, Sheats MK, Jones SL. Misoprostol inhibits equine neutrophil adhesion, migration, and respiratory burst in an in vitro model of inflammation. Front Vet Sci. (2017) 4:159. doi: 10.3389/fvets.2017.00159
75. Sheats MK, Pescosolido KC, Hefner EM, Jae SE, Adler KB, Jones SL. Myristoylated Alanine Rich C Kinase Substrate (MARCKS) is essential to β2-integrin dependent responses of equine neutrophils. Vet Immunol Immunopathol. (2014) 160:167–76. doi: 10.1016/j.vetimm.2014.04.009
76. Gupta S, Chan DW, Zaal KJ, Kaplan MJ. A high-throughput real-time imaging technique to quantify NETosis and distinguish mechanisms of cell death in human neutrophils. J Immunol. (2018) 200:869–79. doi: 10.4049/jimmunol.1700905
77. Lee W, Kim TH, Ku S-K, Min K, Lee H-S, Kwon TK, et al. Barrier protective effects of withaferin A in HMGB1-induced inflammatory responses in both cellular and animal models. Toxicol Appl Pharmacol. (2012) 262:91–8. doi: 10.1016/j.taap.2012.04.025
78. Chen BW, Chen YY, Lin YC, Huang CY, Uvarani C, Hwang TL, et al. Capsisteroids A-F, withanolides from the leaves of Solanum capsicoides. RSC Adv. (2015) 5:88841–7. doi: 10.1039/C5RA12014D
79. Anderson SL, Townsend HGG, Singh B. Role of toll-like receptor 4 and caspase-3,-8, and-9 in lipopolysaccharide-induced delay of apoptosis in equine neutrophils. Am J Vet Res. (2018) 79:424–32. doi: 10.2460/ajvr.79.4.424
80. Savoie A, Lavastre V, Pelletier M, Hajto T, Hostanska K, Girard D. Activation of human neutrophils by the plant lectin Viscum album agglutinin-I: Modulation of de novo protein synthesis and evidence that caspases are involved in induction of apoptosis. J Leukoc Biol. (2000) 68:845–53. doi: 10.1189/jlb.68.6.845
81. Derouet M, Thomas L, Cross A, Moots RJ, Edwards SW. Granulocyte macrophage colony-stimulating factor signaling and proteasome inhibition delay neutrophil apoptosis by increasing the stability of Mcl-1. J Biol Chem. (2004) 279:26915–21. doi: 10.1074/jbc.M313875200
82. Liles W, Dale D, Klebanoff S. Glucocorticoids inhibit apoptosis of human neutrophils. Blood. (1995) 86:3181–8. doi: 10.1182/blood.V86.8.3181.3181
83. Saffar AS, Dragon S, Ezzati P, Shan L, Gounni AS. Phosphatidylinositol 3-kinase and p38 mitogen-activated protein kinase regulate induction of Mcl-1 and survival in glucocorticoid-treated human neutrophils. J Allergy Clin Immunol. (2008) 121:492–8. doi: 10.1016/j.jaci.2007.10.003
84. Saffar AS, Ashdown H, Gounni AS. The molecular mechanisms of glucocorticoids-mediated neutrophil survival. Curr Drug Targets. (2011) 12:556–62. doi: 10.2174/138945011794751555
85. Cox G. Glucocorticoid treatment inhibits apoptosis in human neutrophils. Separation of survival and activation outcomes. J Immunol. (1995) 154:4719–25.
86. Noh E-J, Kang M-J, Jeong Y-J, Lee J-Y, Choi H-J, Oh S-M, et al. Withaferin A inhibits inflammatory responses induced by Fusobacterium nucleatum and Aggregatibacter actinomycetemcomitans in macrophages. Mol Med Rep. (2016) 14:983–8. doi: 10.3892/mmr.2016.5326
87. Kaileh M, Vanden Berghe W, Heyerick A, Horion J, Piette J, Libert C, et al. Withaferin A strongly elicits IκB kinase β hyperphosphorylation concomitant with potent inhibition of its kinase activity. J Biol Chem. (2007) 282:4253–64. doi: 10.1074/jbc.M606728200
88. Heyninck K, Lahtela-Kakkonen M, Van Der Veken P, Haegeman G, Berghe W. Vanden. Withaferin A inhibits NF-kappaB activation by targeting cysteine 179 in IKKβ. Biochem Pharmacol. (2014) 91:501–9. doi: 10.1016/j.bcp.2014.08.004
89. Mohan R, Hammers H, Bargagna-Mohan P, Zhan X, Herbstritt C, Ruiz A, et al. Withaferin A is a potent inhibitor of angiogenesis. Angiogenesis. (2004) 7:115–22. doi: 10.1007/s10456-004-1026-3
90. Oh JH, Lee T-J, Park J-W. Kwon TK. Withaferin A inhibits iNOS expression and nitric oxide production by Akt inactivation and down-regulating LPS-induced activity of NF-κB in RAW 2647 cells. Eur J Pharmacol. (2008) 599:11–7. doi: 10.1016/j.ejphar.2008.09.017
91. Oh JH, Kwon TK. Withaferin A inhibits tumor necrosis factor-α-induced expression of cell adhesion molecules by inactivation of Akt and NF-κB in human pulmonary epithelial cells. Int Immunopharmacol. (2009) 9:614–9. doi: 10.1016/j.intimp.2009.02.002
92. McLeish KR, Knall C, Ward RA, Gerwins P, Coxon PY, Klein JB, et al. Activation of mitogen-activated protein kinase cascades during priming of human neutrophils by TNF-α and GM-CSF. J Leukoc Biol. (1998) 64:537–45. doi: 10.1002/jlb.64.4.537
93. Takami M, Terry V, Petruzzelli L. Signaling pathways involved in IL-8-dependent activation of adhesion through Mac-1. J Immunol. (2002) 168:4559–66. doi: 10.4049/jimmunol.168.9.4559
94. Eckert RE, Sharief Y, Jones SL. p38 mitogen-activated kinase (MAPK) is essential for equine neutrophil migration. Vet Immunol Immunopathol. (2009) 129:181–91. doi: 10.1016/j.vetimm.2008.11.007
95. Nick JA, Avdi NJ, Young SK, Knall C, Gerwins P, Johnson GL, et al. Common and distinct intracellular signaling pathways in human neutrophils utilized by platelet activating factor and FMLP. J Clin Invest. (1997) 99:975–86. doi: 10.1172/JCI119263
96. Zu YL, Qi J, Gilchrist A, Fernandez GA, Vazquez-Abad D, Kreutzer DL, Huang CK, Sha'afi RI. p38 mitogen-activated protein kinase activation is required for human neutrophil function triggered by TNF-α or FMLP stimulation. J Immunol. (1998) 160:1982–9.
97. Nick JA, Avdi NJ, Gerwins P, Johnson GL, Worthen GS. Activation of a p38 mitogen-activated protein kinase in human neutrophils by lipopolysaccharide. J Immunol. (1996) 156:4867–75.
98. Behnen M, Leschczyk C, Möller S, Batel T, Klinger M, Solbach W, et al. Immobilized immune complexes induce neutrophil extracellular trap release by human neutrophil granulocytes via FcγRIIIB and Mac-1. J Immunol. (2014) 193:1954–65. doi: 10.4049/jimmunol.1400478
99. Kamata N, Kutsuna H, Hato F, Kato T, Oshitani N, Arakawa T, et al. Activation of human neutrophils by granulocyte colony-stimulating factor, granulocyte-macrophage colony-stimulating factor, and tumor necrosis factor α: Role of phosphatidylinositol 3-kinase. Int J Hematol. (2004) 80:421–7. doi: 10.1532/IJH97.04122
100. Lane HC, Anand AR, Ganju RK. Cbl and Akt regulate CXCL8-induced and CXCR1- and CXCR2-mediated chemotaxis. Int Immunol. (2006) 18:1315–25. doi: 10.1093/intimm/dxl064
101. Sandoval AJ, Riquelme JP, Carretta MD, Hancke JL, Hidalgo MA, Burgos RA. Store-operated calcium entry mediates intracellular alkalinization, ERK1/2, and Akt/PKB phosphorylation in bovine neutrophils. J Leukoc Biol. (2007) 82:1266–77. doi: 10.1189/jlb.0307196
102. Gong J, Liu H, Wu J, Qi H, Wu ZY, Shu HQ, Bin, Chen L, Wang YX, Li B, et al. Maresin 1 prevents lipopolysaccharide-induced neutrophil survival and accelerates resolution of acute lung injury. Shock. (2015) 44:371–80. doi: 10.1097/SHK.0000000000000434
103. Chen Q, Powell DW, Rane MJ, Singh S, Butt W, Klein JB, et al. Akt phosphorylates p47phox and mediates respiratory burst activity in human neutrophils. J Immunol. (2003) 170:5302–8. doi: 10.4049/jimmunol.170.10.5302
104. Zhang P, Fu Y, Ju J, Wan D, Su H, Wang Z, et al. Estradiol inhibits fMLP-induced neutrophil migration and superoxide production by upregulating MKP-2 and dephosphorylating ERK. Int Immunopharmacol. (2019) 75:105787. doi: 10.1016/j.intimp.2019.105787
105. Lai K-H, Chen P-J, Chen C-C, Yang S-H, El-Shazly M, Chang YC, et al. Lophatherum gracile Brongn. attenuates neutrophilic inflammation through inhibition of JNK and calcium. J Ethnopharmacol. (2021) 264:113224. doi: 10.1016/j.jep.2020.113224
106. Bullone M, Moran K, Lavoie-Lamoureux A, Lavoie JP. PI3K and MAPKs regulate neutrophil migration toward the airways in heaves. J Vet Intern Med. (2013) 27:164–70. doi: 10.1111/jvim.12008
107. Pickles KJ, Brooks AC, Rickards KJ, Cunningham FM. Expression of annexin-1 in equine leucocytes and the effects of the N-terminal annexin-1 peptide, Ac2-26, on equine neutrophil superoxide production. Vet Immunol Immunopathol. (2010) 135:226–33. doi: 10.1016/j.vetimm.2009.12.002
108. Klein JB, Rane MJ, Scherzer JA, Coxon PY, Kettritz R, Mathiesen JM, et al. Granulocyte-macrophage colony-stimulating factor delays neutrophil constitutive apoptosis through phosphoinositide 3-kinase and extracellular signal-regulated kinase pathways. J Immunol. (2000) 164:4286–91. doi: 10.4049/jimmunol.164.8.4286
109. Rane MJ, Klein JB. Regulation of neutrophil apoptosis by modulation of PKB/Akt activation. Front Biosci. (2009) 14:2400–12. doi: 10.2741/3386
110. François S, El Benna J, Dang PMC, Pedruzzi E, Gougerot-Pocidalo M-A, Elbim C. Inhibition of neutrophil apoptosis by TLR agonists in whole blood: involvement of the phosphoinositide 3-kinase/Akt and NF-κB signaling pathways, leading to increased levels of Mcl-1, A1, and phosphorylated Bad. J Immunol. (2005) 174:3633–42. doi: 10.4049/jimmunol.174.6.3633
111. Hahm E-R, Lee J, Singh SV. Role of mitogen-activated protein kinases and Mcl-1 in apoptosis induction by withaferin A in human breast cancer cells. Mol Carcinog. (2014) 53:907–16. doi: 10.1002/mc.22050
112. Aoyama M, Kotani J, Usami M. Butyrate and propionate induced activated or non-activated neutrophil apoptosis via HDAC inhibitor activity but without activating GPR-41/GPR-43 pathways. Nutrition. (2010) 26:653–61. doi: 10.1016/j.nut.2009.07.006
113. Patel SB, Rao NJ, Hingorani LL. Safety assessment of Withania somnifera extract standardized for Withaferin A: Acute and sub-acute toxicity study. J Ayurveda Integr Med. (2016) 7:30–7. doi: 10.1016/j.jaim.2015.08.001
Keywords: neutrophil (PMN), Withaferin A (PubChem CID: 265237), equine, inflammation, phytochemical, veterinary medicine, novel therapeutic
Citation: Bayless RL, Sheats MK and Jones SL (2022) Withaferin A Inhibits Neutrophil Adhesion, Migration, and Respiratory Burst and Promotes Timely Neutrophil Apoptosis. Front. Vet. Sci. 9:900453. doi: 10.3389/fvets.2022.900453
Received: 20 March 2022; Accepted: 24 May 2022;
Published: 17 June 2022.
Edited by:
Isaac Karimi, Razi University, IranReviewed by:
Kenneth James Genovese, Agricultural Research Service, United StatesCopyright © 2022 Bayless, Sheats and Jones. This is an open-access article distributed under the terms of the Creative Commons Attribution License (CC BY). The use, distribution or reproduction in other forums is permitted, provided the original author(s) and the copyright owner(s) are credited and that the original publication in this journal is cited, in accordance with accepted academic practice. No use, distribution or reproduction is permitted which does not comply with these terms.
*Correspondence: Rosemary L. Bayless, UkxCYXlsZXNAbmNzdS5lZHU=
Disclaimer: All claims expressed in this article are solely those of the authors and do not necessarily represent those of their affiliated organizations, or those of the publisher, the editors and the reviewers. Any product that may be evaluated in this article or claim that may be made by its manufacturer is not guaranteed or endorsed by the publisher.
Research integrity at Frontiers
Learn more about the work of our research integrity team to safeguard the quality of each article we publish.