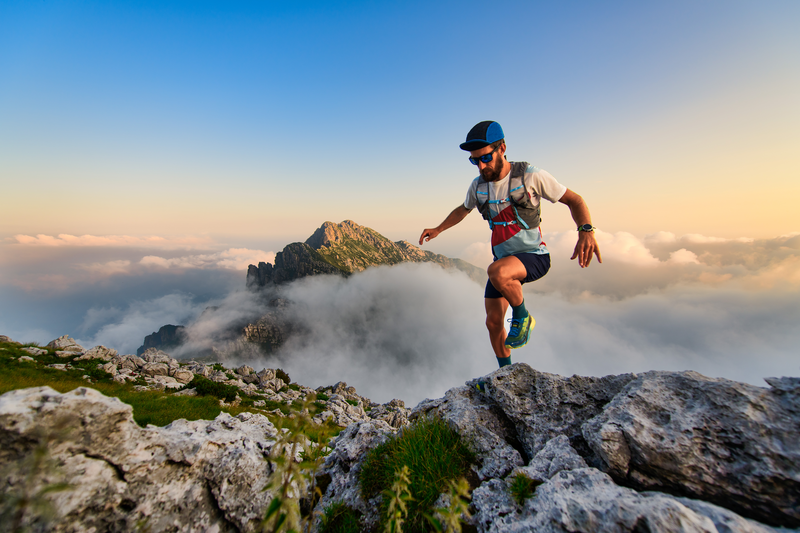
94% of researchers rate our articles as excellent or good
Learn more about the work of our research integrity team to safeguard the quality of each article we publish.
Find out more
REVIEW article
Front. Vet. Sci. , 17 May 2022
Sec. Animal Nutrition and Metabolism
Volume 9 - 2022 | https://doi.org/10.3389/fvets.2022.898295
Waste milk (WM) is a part of the milk produced on dairy farms, which is usually unsuitable for human consumption. The WM contains transition milk, mastitis milk, colostrum, milk with somatic cells, blood (Hemolactia), harmful pathogens, pathogenic and antibiotic residues. Due to the high cost of milk replacer (MR), dairy farmers prefer raw WM to feed their calves. It has been well established that WM has a greater nutritive value than MR. Hence WM can contribute to improved growth, rumen development, and immune-associated parameters when fed to dairy calves. However, feeding raw WM before weaning has continuously raised some critical concerns. The pathogenic load and antibiotic residues in raw WM may increase the risk of diseases and antibacterial resistance in calves. Thus, pasteurization has been recommended as an effective method to decrease the risk of diseases in calves by killing/inhibiting the pathogenic microorganisms in the raw WM. Altogether, the current review provides a brief overview of the interplay between the positive role of raw WM in the overall performance of dairy calves, limitations of raw WM as a feed source and how to overcome these issues arising from feeding raw WM.
The productive efficiency of dairy farms is determined by many factors, including good management of dairy calves. There are no returns for the dairy farm in the first 2 years of rearing heifers, with average costs for raising a Holstein heifer estimated at $1,225 in the US (1, 2). It has been suggested that these costs are much higher in China, with estimates projected to be almost 1.34 times those of the US (3).
On most Chinese dairy farms, calves are weaned at least 2–3 months after birth (4). A calf is most likely to have consumed ~350–500 kg of standard milk (SM) or milk replacer (MR) during that period. Therefore, in production systems that rely solely on feeding raw SM, the rearing costs might be greater because of the opportunity cost involved; SM is the sole income source. Hence, several feeding techniques have been explored in the past few decades to minimize calf rearing costs and ultimately reduce farm costs during the non-productive period.
Waste milk (WM) has gotten considerable attention among dairy farmers because of its perceived low cost and desirable nutritive value. All non-saleable milk, including colostrum, transition milk, mastitis milk, colostrum, milk with somatic cells, blood (Hemolactia), harmful pathogens and pathogenic and antibiotic residues, is usually graded as raw WM (5). Mei et al. (6) reported that the raw WM accounts for about 2–4 percent of China's total milk production, equating to 0.8–1.6 million tons of milk produced annually in China. These significant amounts of milk represent a loss of income for the dairy farm and can also be an environmental hazard. However, since some dairy farms can have a significant amount of raw WM with a nutrient profile as good as that of salable raw SM, farms dispose of the milk by feeding it to dairy calves (7). For example, a study from the United States reported that raw WM was the main dietary liquid feed fed to 40.1%, while MR was fed to slightly <35.0% of calves surveyed (8). Although feeding WM to calves is considered cost-effective, its use on dairy farms remains controversial (9).
Raw WM contains a high concentration of viable bacteria and antibiotic residues (10), which are linked to an increased incidence of diarrhea in calves (11). It has been reported that Salmonella spp. and Mycoplasma spp. in raw WM are among the major pathogenic bacteria that pose a threat to calf health (12). Consequently, some studies have documented that feeding raw WM might contribute to the development of antimicrobial-resistant bacteria in the gut of calves (13, 14). Indeed, a shift in the prevalence of antimicrobial resistance has been observed in calves fed raw WM (15, 16). Furthermore, the bovine viral diarrhea virus (BVDV) is an important global pathogen in the livestock industry (17). It was recently reported that feeding calves with milk from lactating cows carrying BVDV resulted in serious health issues (18). Proper treatment of raw WM is highly recommended to overcome these health challenges. Pasteurization has been promoted as a viable option for treating WM and reducing levels of harmful bacteria in milk to acceptable limits (19). It is plausible that feeding calves with pasteurized raw WM could cost ($0.69/day) less to raise than those fed commercial MR, even when the cost of purchasing a pasteurizer is included (9). Moreover, calves fed pasteurized waste milk (PWM) showed increased growth and had lower morbidity and mortality during the pre-weaning period (9). Alternatively, organic acids could be used to acidify WM (AWM) and reduce the health risk of feeding raw WM to the calves (20). The effect of raw WM on overall calf performance is summarized in Figure 1. The current review discusses the drawbacks of utilizing raw WM to feed dairy calves. In addition, the impact of pasteurization in reducing bacterial load in milk has also been explored in depth.
Figure 1. Raw waste milk (WM) refers to milk that cannot be sold to the market for human consumption. The milk is obtained mainly from fresh cows and diseased cows under treatment with antibiotics or whose antibiotic withdrawal period has not elapsed, resulting in very low concentrations of these antibiotics being passed through milk. Pasteurization is often performed before feeding WM to reduce health risks in calves. Pasteurized WM reduces the number of harmful bacteria, IL-8 and increases the concentration of beneficial metabolites and volatile fatty acids (VFA). Finally, the growth performance, rumen fermentation and immune system of the calves are improved.
From birth to weaning, dairy calves endure numerous challenges and stressors, including greater morbidity rates (21), which might affect their growth performance. The nutritional and health characteristics of these calves, if not effectively controlled, will lead to increased production costs and environmental hazards. A range of factors such as the quantity and quality of liquid feed, environmental changes, feeding methods, and solid feed intake are critical to calf growth and health (22).
Calves fed raw WM had greater average daily gain and serum total protein, albumin, total cholesterol, high-density lipoprotein, triglycerides, growth hormone, immunoglobulin (Ig) A and IgM concentrations compared to raw SM (23), which could be attributed to the high amount of milk fat and solid non-fat percentages, or presence of antibiotic residues in raw WM (5). Similar studies showed that calves fed raw WM gained more body weight than calves fed MR or SM (5, 23–25). One probable mechanism is the growth-promoting effects of raw WM, whereby the antibiotic residues present in the raw WM alters the intestinal flora resulting in a healthy intestinal environment (26). Subsequently, the calves direct the extra energy available toward growth rather than fueling the immune function while trying to counteract the pathogen and antigens in the gut lumen (27).
The range of penicillin concentrations utilized in a dose-regulated experiment was determined using the concentration of β-lactam antibiotic residues discovered in milk from treated cows (13). In addition, they also reported that the resistance of gut bacteria to antibiotics increased with the supplementation of raw WM in calves having higher concentrations of penicillin in the milk (13).
The effect of substituting PWM with raw SM for 3 weeks on dairy calves' growth and health performance has been well documented (28). It has consistently been reported that calves fed PWM gained more BW than those fed raw SM during the post-weaning and overall periods. In the pre-weaning, post-weaning, and overall periods, calves fed PWM had higher average daily gains than those fed raw SM. Moreover, feed efficiency and health were improved with feeding the PWM during all the studied periods. A study reported that calves fed PSM improved daily gain (29).
Unlike raw SM, the effect of MR on calf growth performance depends on its composition. Hence, studies that have compared raw SM or PWM and MR have shown inconsistent results. It is well established that source of protein in milk replacer can interfere with growth, with some early studies reporting that calves fed milk-derived protein MR performed better than those fed soybean protein concentrate as the main protein source (30). On the contrary, calves fed raw SM and MR had similar growth performance, though the MR contained plant protein (31). Additionally, an optimal protein level in MR is critical to support growth and reduce environmental pollution. Calves fed an MR containing 22% crude protein had better growth performance and nutrient utilization than animals fed either 18 or 26% of crude protein on a DM basis (32).
Alternatively, AWM could be fed to promote calf growth (33). Adding an organic acid to milk decreases the WM pH and diarrhea and increases the daily gain in dairy calves (23). The AWM might have an improved flavor, which promotes appetite in calves (34). Presently economic considerations generally hinder the use of raw SM on dairy farms. Partial replacement of raw SM with raw WM is recommended in dairy calves at an early stage of life to maintain a maximum growth rate and health benefits (9). A summary of the effects of raw WM and PWM on calf growth performance is shown in Table 1.
Rumen development relies on the pre-weaning colonization of the gastrointestinal tract with bacteria, which results in the physiological changes and transition from a non-ruminant to a ruminant (40). On the other hand, dietary changes may result in long-term changes in the composition of rumen bacteria (41–43).
Pasteurized waste milk substantially altered the gut microbial composition in pre-weaning calves, especially Bacteroidetes spp. and Firmicutes spp. (44) which significantly increased in calves fed PWM compared to raw SM. In a different study, calves fed raw WM exhibited a richer rumen microbiota than those fed raw SM (35). The raw WM contains greater milk protein content entering the rumen than raw SM (45) due to its substantial amount of transition milk. The rumen of raw WM-fed calves tends to have a higher concentration of isovalerate, a true reflection of the high protein content in the raw WM. The higher concentration of isovalerate has been associated with increased numbers of Butyrivibrio fibrisolvens in calves (46).
In the rumen, microorganisms produce branched-chain volatile fatty acids (VFAs) through decarboxylation of branched-chain amino acids and peroxide deamination (45). The VFAs, especially butyrate, stimulate the growth of the rumen papilla and epithelium and are considered the most critical elements influencing rumen development (47). Calves fed MR plus four antibiotics (0.024 mg/L penicillin, 0.025 mg/L streptomycins, 0.1 mg/L tetracyclines, and 0.33 mg/L ceftiofur) had a reduced and higher richness of Prevotella spp. and Acetitomaculum spp., respectively, at the genus level. As a result, residual antibiotics administered to raw WM calves enhanced rumen fermentation, ruminal papillae growth, and increased rumen acetic acid production (2). The relative abundance of Prevotella spp., one of the most diverse and core flora at the genus level (48), was reduced by antibiotic residues, possibly due to their sensitivity to a penicillin (49) and tetracycline (50) antibiotics. Antibiotics such as monensin, gentamicin, virginithromycin, ampicillin, ceftriazine, penicillin, and oxytetracycline are routinely used to treat mastitis in dairy cows. Chlortetracycline, oxytetracycline, tylosin, and monensin have been demonstrated to prevent rumen bacteria from breaking down cellulose and producing VFA in vivo (51). In addition, ampicillin, ceftriazine, penicillin and oxytetracycline inclusion in calf milk can affect the fecal microbial population composition (35, 52). A recent study supported the idea that gentamicin inhibits Prevotella intermedia (53). The abundance of Prevotella spp. was likewise reduced in calves given raw WM with concentrations of 0.024 mg/L penicillin, 0.025 mg/L streptomycins, 0.10 mg/L tetracycline, and 0.33 mg/L ceftiofur (2). Feeding raw SM has an important effect on calf rumen development. A study reported lower acetate and propionate levels in the rumen of calves fed raw SM compared to PWM (37). A study found that feeding acidified SM to calves within 14 weeks of age was helpful for rumen development (54). Calf rumen pH is an important indicator for assessing rumen development. The optimal pH of calf rumen should be between 5.8 and 6.4 pH units under good feeding practice (55). A study reported that the addition of inulin to raw SM can influence the pH of the rumen by making it more alkaline, and can accelerate postnatal rumen development and improve its functionality (56), and adding 12 g of inulin to raw SM when feeding calves could improve rumen papillae development, as seen from increased length and width of the papilla, especially in the Saccus ventralis region. A study in our research group tried to improve rumen development by adding butyrate to raw SM (57). However, raw SM with butyrate had no significant effect on rumen development (57). A study reported that PSM reduced the calf diarrhea rates and improved health compared to SM (58). Likewise, MR can positively affect calf rumen development (59). Interestingly, most studies have focused on the effects of combining milk replacer with other beneficial supplements, such as probiotics and milk replacer, on the calf rumen (60, 61). Indeed, a study found that adding probiotics to MR improved rumen development (61).
Injury to the intestinal mucosa disrupts the intestinal epithelial barrier function resulting in enteritis and diarrhea in dairy calves (62). Calves fed both raw WM and PWM had uniform villi in the ileum, while those fed raw SM were irregular (23). However, pasteurization seems to further improve the villi distribution and conformity in calves fed PWM compared to raw WM. The high concentration of growth factors and bioactive peptides in PWM might partially explain the superior developed intestinal villi compared to raw WM (63, 64).
Shortly after birth, different bacteria colonize the calf's gastrointestinal system (27, 65). The composition and activity of these microbiotas can have an impact on dairy calves' growth and development (65, 66). The gut microbiota exists symbiotically with the host by stimulating the immune system and intestinal tissue development while obtaining energy from the host's diet (67). Calves' health depends on a healthy intestinal microbiota, which contributes to a stable intestinal environment (17) that promotes epithelial cell growth and development (68).
Pre-weaning calves get most of their energy and protein from partially digested and absorbed food elements in the small intestines (69). Diet has a significant impact on the development of intestinal microbiota (65, 70) and is regarded as one of the most essential elements in gut microbial structure regulation (71–73). This is especially important later in life, as the early intestinal microbiota improves health and productivity (43). As a result, low incidences of illnesses in calves fed PWM compared to raw SM (1, 74) could be attributed to the intestinal microbiota being regulated prior to weaning (52). However, the long-term effects of feeding raw WM on calf health and performance are yet to be elucidated. Interestingly, there were no differences in the alpha diversity of colon and caecum digesta in calves fed raw WM, PWM and raw SM as determined by Chao 1 and Shannon indices (75). However, there were differences in the dominant microbiota in the colon and caecum digesta. In caecum digesta samples, the dominant bacterial phyla in raw WM and PWM-fed calf were Bacteroidetes spp. and Firmicutes spp., while the dominant bacterial phylum in raw SM calf was Fusobacteria spp. In colon digesta samples, Bacteroidetes spp. and Firmicutes spp. were the dominant phyla in the PWM calves, whereas Proteobacteria spp was the most abundant phyla in the raw WM and SM calves. Meanwhile, the study also indicated that feeding calves PWM elevated the relative abundance of Ruminococcus spp., Megamonas spp., and Oxalobacter spp. in the caecum. Notably, Ruminococcus spp. produce short-chain fatty acids, which are important sources of energy for ruminants (76). These findings implied that feeding calves PWM may exert beneficial effects on calf health by elevating the abundance of beneficial bacteria in the caecum. Calves fed SM increased the relative abundance of the genus Comamonas spp. in the colon (76), which is associated with the degradation of steroids and various aromatic acids (77). This means that the hydrolytic and fermentative functions of raw SM in the colon may have been enhanced. The combination of MR and butyric acid can increase the abundance of Prevotella spp. in the colon and cecum (78). Prevotella spp. has been established as an important member of the mammalian gut ecosystem, comprising species capable of fermenting a wide range of non-cellulosic plant polysaccharides and protein (79). Studies have shown a decrease in the relative abundance of Clostridium spp. and Peptostreptococcus spp. in the gut of ASM and PSM calves (80). A study reported that adding sodium humate and glutamine to MR significantly increased the abundance of calves' intestinal beneficial microbiota (81). The nutritional composition of MR affects the calf gut microbiome. A study found that feeding calves two different commercial MRs had different effects on the calves' gut microbiome (82). In addition to the different crude protein and crude fat content of the two MRs, there were also differences in their production methods. Among them, MR with more conjugated milk oligosaccharides significantly increased the abundance of Bifidobacterium spp. and Faecalibacterium prausnitzii spp. in the gut of calves (82). Bifidobacterium spp. is well known to utilize milk oligosaccharides (83).
Due to the increased attention toward antimicrobial resistance in recent years, the antimicrobial residues in raw WM have also attracted interest among animal scientists. Studies have shown that if raw WM is fed, the antimicrobial residues in the milk might exert selective pressure on the intestinal microbiota in calves, which may increase the prevalence of drug-resistant bacteria in the rumen and intestines (84). Wray et al. (24) evaluated the effect of antibiotic-containing WM feeding calves in two trials. Fecal E. coli were monitored for antibiotic resistance. They used fermented and unfermented WM in the first trial. According to these researchers, the geometric mean minimum inhibitory concentration (MIC) for streptomycin was considerably greater for isolates from calves given unfermented WM. In the second study, only unfermented milk was utilized, and no changes in the percentage of resistant E. coli and Enterococci were found between isolates from calves fed antibiotic-containing milk and the controls when it came to identifying the percentage of resistant E. coli and Enterococci (24). Consistently, it has been reported that fermentation can reduce the antibiotic content in raw WM, and the high numbers of bacteria in the raw WM might have presented a disease risk in these two experiments. Studies that investigated the impact of feeding raw SM or WM—PWM or not—on calf antibiotic resistance of specific fecal bacteria, found that the proportion of resistant E. coli isolates was significantly higher in calves fed raw WM (inclusive of PWM; most pronounced for cephalosporins) than in calves receiving raw SM (15). This implies that PWM represents an acceptable feed for young calves (5). Maynou et al. (15, 38) studied the antimicrobial resistance patterns in fecal E. coli, and nasal Pasteurella multocida (P. multocida) isolates from calves fed either MR or raw WM in 8 commercial dairy farms (4 farms per feeding program). These authors reported a greater number of fecal E. coli resistant to enrofloxacin, florfenicol, and streptomycin. Moreover, multidrug-resistant E. coli phenotypes were isolated in the feces of calves fed raw WM than in those fed MR (15). Hence, feeding calves raw WM fosters resistant bacteria in the lower gut and respiratory tract of dairy calves. Moreover, feeding raw WM increases the prevalence of pathogenic microbiota resistant to antimicrobials and other non-antimicrobial treatments used on farms (15, 38). Furthermore, feeding raw WM to dairy calves increased the prevalence of antimicrobial-resistant bacteria on the farms as well as other antibiotics that had never been used before (5, 14). Consistently, a study reported that bacterial isolates from human, animal and environmental sources shared genetically identical plasmids that mediate resistance to various antimicrobial classes (85). Although fecal shedding is influenced by a variety of factors, such as the environment and calf age, feeding milk with antimicrobial residues contributes significantly to an increased prevalence of antimicrobial-resistant (AMR) bacteria, such as extended-spectrum beta-lactamase (ESBL)-producing E. coli. A study assessed the impact of feeding raw WM on the prevalence of these bacteria in the feces of calves and found an increase in the prevalence of resistant bacteria shedding in the feces (25). Several other studies have reported that feeding raw WM to calves could change their microbiome (86). Consequently, feeding raw WM might lead to poor health by reducing intestinal microbiota diversity (87–89).
Raw WM may also contain non-antimicrobial drugs, such as non-steroidal anti-inflammatory drugs (NSAIDs) used to treat cows with pain and inflammation (90). In addition to variations in the level of antimicrobial residues, it should be noted that raw WM may vary in its composition from one cow to another and farm to farm. A longitudinal study investigated the presence of E. coli that produces broad-spectrum-lactamase (ESBL) on a single farm with 250 Holstein-Friesian cows and 40 un-weaned calves (25). All calves were fed raw WM from sick dairy cows or freshly calved cows that had previously received antibiotic dry cow treatment (DCT). In this study, three raw WM samples contained cefquinome and cephalexin residues. Two of the three raw WM samples also contained CTX-M-producing E.coli. Moreover, ten calves were individually assessed for the excretion of CTX-M ESBL and found that the excretion time of these AMR pathogens took as long as 64 days (median of 36 days) after the calf had been weaned. Meanwhile, the frequency of excretion of resistant bacteria decreases with time (91). In the first weeks of dairy calves' life, the excretion of resistant bacteria increases, followed by a subsequent reduction (92). Furthermore, the development of E. coli resistance in feces and P. multocida in nasal samples of calves was documented when fed MR compared to raw WM (15). In the feces, calves that received raw WM reported a higher occurrence of resistant and multi-resistant E. coli bacteria. The group fed raw WM had a greater proportion of resistant P. multocida to colistin in nasal swab samples. Overall, it was shown that giving raw WM to animals increased the prevalence of antimicrobial-resistant bacteria (15). Much of the antibiotics used on dairy farms are for disease management in mature cows, and AMR in their fecal microorganisms is relatively rare. However, the feces from young dairy calves tend to contain high levels of antimicrobial resistance genes of E. coli and Salmonella enterica, which may provide dairy farms with potential hosts for antimicrobial resistance genes (93). The antimicrobial resistance in calf feces may be mainly from raw WM feeding. The presence of multidrug-resistant E. coli in raw WM urges the need for on-farm practices, such as pasteurization, that reduce the risk of exposure to calves and dissemination of resistant bacteria into the environment (94). A study documented that calves were fed raw SM from birth to weaning, with half of the calves receiving milk spiked with four antibiotics, and the other half fed the same raw SM but without the addition of any antimicrobials. The results showed a significant increase in the proportion of fecal shedding of E. coli resistant to ceftiofur as well as several multidrug-resistant bacteria (14). Limited information on antimicrobial resistance to ASM and PSM is available. The effects of raw WM and PWM on calf intestinal microbiota and antimicrobial resistance were summarized in Table 2.
Limiting intestinal microbiota diversity leads to poor gut health (88, 89), which has far-reaching consequences on the development of the host immune system (87). It has been documented that an inflammatory reaction might occur in the jejunum and ileum in calves fed either untreated or acidified raw WM (75). However, calves fed raw WM had much higher risks of getting diarrhea than calves fed treated (i.e., pasteurized or acidified) WM in a recent study (e.g., body condition score, nasal discharge, the occurrence of diarrhea) (20). However, some studies have shown that feeding raw WM to calves can reduce diarrhea (107).
The effects of feeding MR both with and without antimicrobials have been well documented in previous studies (108, 109). A study found that feeding a low cocktail of antimicrobials in MR led to a shift in the bacterial taxa of the intestinal microbiome, including a reduction in E. coli, which might positively affect calf health by reducing the occurrence of diarrhea in young calves (110). Pereira and colleagues studied the functional profile of fecal bacteria till 6 weeks of age in calves fed milk with or without antimicrobial residues (98). After 1 week, the two feeding groups showed a significant difference in the abundance of genes in fecal bacteria for stress response, nitrogen metabolism, regulation, and cell signaling.
It has been reported that adding antibiotics to feed can alter the composition of certain microbiota, such as E. coli in calves (111, 112). E. coli is the most common facultative anaerobe in human and animal gastrointestinal tracts, causing a number of diseases such as diarrhea (113). Calves fed raw WM had reduced relative abundance of Clostridium spp. and Streptococcus spp., resulting in changes at the genus level (75). This implies that feeding raw WM may reduce calf diarrhea caused by Clostridium spp. and Streptococcus spp. The potential effect of feeding raw WM to calves on drug resistance has not been studied deeply because the selection of resistant bacteria has traditionally been assumed to occur at concentrations between the susceptible wild-type population's minimal inhibitory (MIC) and the resistant bacteria's MIC. Antimicrobial medications at concentrations below the MIC (sub-MIC) have been shown in other investigations to increase mutagenesis and recombination, resulting in bacterial adaptability to diverse stresses, including antimicrobial pressure (114, 115). An increase in mutagenesis can also result in a heterogeneous increase in the MIC of the bacteria across a range of antimicrobials.
In a controlled study, antibiotics in medicated MR have reported lower morbidity compared to those fed with non-medicated MR (11). The pre-weaning modulation of the intestinal and fecal microbiota may be responsible for this reduction (14). The utilization of discarded milk as calf feed has always been a source of contention. Increased environmental hygiene or WM's role as a vector for several infections has been the main concern (43). Since the establishment of antimicrobial resistance has accelerated in recent years (116), greater attention has been focused on the antibiotic residue concentration in raw WM. According to one theory, the intestinal microbiota of calves given raw WM may be subjected to selection pressure as a result of such residues. This could lead to an increase in the predominance of resistant bacteria in the calf's intestines. Although a few studies have looked into this topic, more results are required for validation. The main controversy surrounding raw WM feeding has been around the high load of pathogenic microbiota (10), especially coliform bacteria that can result in infection in calves (117). There remain controversies over whether raw WM should be used in calf feeding programs, especially due to the presence of pathogenic bacteria (11, 118). High coliform counts in raw WM could lead to high endotoxin levels, which might cause harm to neonatal calves (117). Several species such as Streptococcus spp., Enterobacteriaceae spp., Staphylococcus spp., and E. coli have been isolated from raw WM (5). E.coli is a major risk for diarrhea during calves' first week of life. Thus PWM would be the best choice to decrease the risk of these harmful bacteria in calves. It has been reported that ASM can inhibit the activity of Salmonella spp. by reducing the pH value (119).
Although raw WM may contain many harmful pathogens, however, PWM can kill the harmful bacteria, hence reducing the risk of spreading infectious diseases through feeding raw WM. Despite several studies exploring this question, their results are inconclusive (100). Pasteurization of WM inactivates bacteria, such as the destruction of Mycobacterium paratuberculosis, spp. Salmonella spp. and Mycoplasma spp. (12, 120, 121), most viruses, such as the bovine leukemia virus (122) and protozoa, such as Cryptosporidium parvum oocysts (123).
The profile of serum metabolites is a good indicator of the health and nutritional performance of the calf. Among the most important blood parameters, total protein (TP) is a good indicator of health in the first few weeks of life. Twenty-four hours after birth, calves are considered to have a failed passive immunity transfer when their serum TP is less than 5.2 g/dL (64). Furthermore, the Brix value is another important indicator for evaluating the passive immunity of calves. It is generally believed that when the Brix value is lower than 7.8% or 8.4%, calves have failed the passive transfer immunity (124, 125). Besides TP, albumin (ALB) and blood urea nitrogen (BUN) reflect protein utilization (126), while triglycerides (TG) and total cholesterol (TC) reflect the lipid metabolism (127) in young calves. The serum TP decreases when calf feed intake is reduced, there are nutritional imbalances in the diet or failure of passive immunity (124, 128). A previous study reported that the serum TP, ALB, high-density lipoprotein cholesterol (HDL-C), TG and TC concentrations of raw WM fed calves were higher than those in calves fed raw SM (23). This implies that raw WM can improve protein and lipid synthesis in dairy calves (129). In the same study, calves fed raw WM had a greater concentration of growth hormone and glucocorticoids (GC) compared to calves fed raw SM (22). This may signify that calves fed with raw WM had better growth performance (Figure 2). Some studies reported that the retinol metabolic pathway of calves in the MR group was significantly up-regulated compared with the raw SM group (31). Studies have shown that retinol metabolism is related to feeding efficiency (130). This suggests that MR may have a positive effect on calf feeding efficiency. However, it has been reported that MR down-regulates the fatty acid synthesis pathway (104). Notably, the nutrient composition of different MRs varies widely, and more studies are needed to assess the effects of different nutrient compositions of MRs on calf metabolism. The research on the effects of raw SM, WM, PWM and MR on the metabolism of calves is limited, and hence, more in-depth studies are warranted to determine the impact of raw WM and PWM on the metabolic performance of calves in order to provide more practical feeding guidelines. The effects of raw WM and PWM on calf metabolism were shown in Table 3.
Figure 2. Raw waste milk improves growth performance by increasing serum metabolites total protein (TP), albumin (ALB), total cholesterol (TC), glucagon (GC), growth hormone (GH), human epidermal growth factor (h-EGF) and insulin-like growth factor-1 (IGF-1).
The antioxidant system in mammals can protect against the harmful effects of free oxygen species and their metabolites. The system is normally in a dynamic state of balance between free radical generation and clearance, ensuring the animal's optimal health. Calves continue to build their antioxidative defense system after birth (133). Copper and zinc superoxide dismutase are found in milk (134, 135). One of the most common enzymes used to assess oxidative state in animals is superoxide dismutase (SOD) (136, 137). A study reported no difference in the concentration of serum SOD between calves fed raw SM, raw WM and PWM (23). Glutathione peroxidase GSH-px converts superoxide to water (138). A study reported that the serum GSH-px concentration of calves fed with raw SM was significantly higher than calves fed with PWM (38). Furthermore, the level of serum malondialdehyde (MDA) is used to monitor lipid peroxidation via reactive oxygen species (139), representing the degree of lipid peroxidation as well as the degree of free radical damage. Studies have demonstrated higher MDA in serum from calves fed raw SM compared to raw WM and PWM (23). These studies implied that raw SM could activate the antioxidant mechanisms of SOD and GSH-px, while lowering the oxidation mechanisms by MDA production. The results further suggested that high-quality nutrients and microbial activity in raw SM contributed to establishing antioxidant defense mechanisms in calves. However, few dairy farms feed calves with SM due to the associated production costs.
Intrinsic components of the complement system include C3 and C4. Thus the immune system can only function properly if the levels of C3 and C4 are maintained within given ranges. However, compared to raw WM and raw SM, no effect was noted on the content of C3 and C4 when PWM was fed to preweaning calves (23). This means that feeding PWM had no adverse effects on the immune system of the calves. On the other hand, serum immunoglobulin (Igs) levels can directly reflect the regulatory mechanism of humoral immunity. For example, a study showed that the serum IgG content in calves with diarrhea was significantly lower than that of healthy calves and the serum IgG concentration was positively correlated with the incidences of diarrhea (140). A study reported that the IgG content in the serum of calves fed with WM was lower than that of calves fed SM (23). More harmful microorganisms might have caused contributed to the observed results, further buttressing the assertion that direct feeding of raw WM poses a threat to the health of the calf. The effects of raw WM and PWM on calf antioxidant were shown in Table 4.
When the body experiences inflammation, interleukins, types of immunological factors, are activated and regulate the immune system. Interleukins including IL-6, IL-8, and IL-10 are inflammatory markers (141, 142). Tumor necrosis factor (TNF) is engaged in cell-mediated immune responses and plays a key role in intracellular viral and mycoplasmal resistance and defense (143, 144). It has been reported that calves fed with raw WM showed up-regulation of some of the immune factors (e.g., serum IL) in serum, jejunal mucosa and mesenteric lymph nodes (145). This might be linked to the microbial activity of different microbial species and quantities. A study reported that compared with ASM, PSM significantly increased calf serum TNF-α, IL-6 and IL-1β (146). The effects of raw WM and PWM on calf immunity were shown in Table 4.
As bacteria can be transmitted directly from cows (cows with mastitis) to milk, poor hygiene during milking, transportation, and milk storage often exacerbates contamination. Raw WM that has not been properly treated should be used cautiously to feed calves, as it has a greater microbial load that can be harmful to calves. The geometric mean of the cumulative number of bacteria for raw WM samples was shown to be significantly higher than in raw SM; Streptococcus spp. and Enterobacteriaceae spp. were the predominant bacteria identified, followed by Staphylococcus spp. (10). Milk pasteurization is currently used on many dairy farms to minimize the potential risk of infectious diseases in calves (147). It has been documented that PWM could reduce the morbidity and mortality of calves and increase their growth rate compared to those fed conventional MR (9). Dairy producers and ranchers use the pasteurization process to kill off a large number of the pathogenic microorganisms in milk, such as Mycobacterium avium subsp. paratuberculosis (148–150) and Salmonella spp. or Mycoplasma spp. (10, 121). Milk pasteurization can be accomplished by heating the raw WM to 63°C for 30 min (low temperature, long time) or 72°C for 15 s (high temperature, short time) (5). A study reported that pasteurization of raw WM at 63°C for 35 min could increase phenotypic resistance to ampicillin, cephalotin, ceftiofur, and florfenicol in fecal E. coli (38). Although, Grant et al. (151) suggested that pasteurization of raw WM with a longer holding period was more successful in inactivating harmful bacteria, more research has shown that optimal raw WM pasteurization conditions were attained at 63°C for 30 min (19, 152). Compared with raw WM or MR, feeding PWM can ensure better growth performance and health status and obtain higher economic profits (9, 153).
Acidifying WM has also been recognized as a labor-saving, simple and cost-effective method in calf feeding operations (154). Consequently, the method can reduce the rapid growth of pathogens in the digestive tract and decrease the incidences of infectious bacteria (155).
Although raw WM can be pasteurized to inactivate bacteria (12, 120, 156), the method might not be effective on spore-forming bacteria (157), some viruses and protozoan (158). For example, M. avium spp. cannot completely be inactivated by pasteurization (159–161). Aust et al. (5) reported the importance of pasteurization and its link to a decrease in the threat of transmission of Streptococcus agalactiae, Mycobacterium paratuberculosis, E. coli and Mycoplasma spp. Notably, certain bacterial toxins (117) and residual antibiotic concentrations may not respond to pasteurization (162).
The health status of the dairy calves should be prioritized when deciding whether to feed raw WM. Firstly, cows with BVDV or Johne's disease can easily spread the diseases to other animals and calves. Thus, it would be better to avoid feeding milk from such cows to calves. Secondly, the WM should not be kept at room temperature for an extended period since this may cause major changes in microbial load. Thirdly, the milk obtained from the first milking soon after antibiotic use should be avoided because this milk is a major source of antibiotic residues (163). Moreover, Feeding calves raw WM from cows infected with E. coli or Pasteurella spp. should also be avoided because these bacteria stay in the milk for a long time and can likely invade the intestinal barrier, contributing to the calf sickness. Collectively, it is recommended that the colostrum obtained from cows must be free from diseases like enzootic bovine leukosis, tuberculosis and brucellosis. Similarly, colostrum from cows showing any sign of infectious disease at the time of milking should not be used. Pasteurization conditions and effects on calf health are shown in Table 5.
Altogether, we concluded that raw WM is economical and contributes positively to the growth and performance of dairy calves. However, the antibiotic residues and the presence of some pathogenic bacteria, such as Mycobacterium avium subsp. Paratuberculosis, Salmonella spp. and Mycoplasma spp., may limit the use of raw WM as a feed resource in calves. Consuming WM results in the shedding of antibiotic-resistant bacteria in the feces of calves. Feeding properly pasteurized WM can decrease bacterial load in the milk and reduce the risk of diseases in calves. However, it is advisable to avoid feeding raw WM obtained from cows that have been treated with antibiotics over a long time during lactation.
YM, MK, and ZC: conceptualization and writing—original draft preparation. YM, MK, JX, GA, XC, SL, YW, and ZC: editing and technical review. YM, MK, JX, GA, and XC: Searching for literatures. ZC: visualization and supervision. All authors have read and agreed to the published version of the manuscript.
The review was supported by the China Animal Husbandry Group (DR201905) and the 2115 Talent Development Program of China Agricultural University. The funders had no role in the study design, data collection, analysis, decision to publish, and manuscript preparation.
The authors declare that the research was conducted in the absence of any commercial or financial relationships that could be construed as a potential conflict of interest.
All claims expressed in this article are solely those of the authors and do not necessarily represent those of their affiliated organizations, or those of the publisher, the editors and the reviewers. Any product that may be evaluated in this article, or claim that may be made by its manufacturer, is not guaranteed or endorsed by the publisher.
We acknowledge the National Natural Science Foundation of China (U20A2062) for its financial support.
1. Tozer PR, Heinrichs AJ. What affects the costs of raising replacement dairy heifers: a multiple-component analysis. J dairy sci. (2001) 84:1836–44. doi: 10.3168/jds.S0022-0302(01)74623-1
2. Li JH, Yousif MH, Li ZQ, Wu ZH, Li SL, Yang HJ, et al. Effects of antibiotic residues in milk on growth, ruminal fermentation, and microbial community of preweaning dairy calves. J Dairy Sci. (2019) 102:2298–307. doi: 10.3168/jds.2018-15506
4. Diao QY, Zhang R, Tu Y. Current research progresses on calf rearing and nutrition in China. J integr agr. (2017) 016:2805–14. doi: 10.1016/S2095-3119(17)61767-2
5. Aust V, Knappstein K, Kunz HJ, Kaspar H, Wallmann J, Kaske M. Feeding untreated and pasteurized waste milk and bulk milk to calves: effects on calf performance, health status and antibiotic resistance of faecal bacteria. J Anim Physiol Anim Nutr (Berl). (2013) 97:1091–103. doi: 10.1111/jpn.12019
6. Mei GU, Zheng N, Liu H, et al. A comparison of china's previous and current national standards for raw milk with respect to major quality indexes. Food Sci. (2019) 28:478–563. doi: 10.7506/spkx1002-6630-20171203-022
7. Brunton LA, Duncan D, Coldham NG, Snow LC, Jones JR. A survey of antimicrobial usage on dairy farms and waste milk feeding practices in England and Wales. Veterinary Record. (2012) 171:296. doi: 10.1136/vr.100924
8. Urie NJ, Lombard JE, Shivley CB, Kopral CA, Adams AE, Earleywine TJ, et al. Preweaned heifer management on US dairy operations: Part I. Descriptive characteristics of preweaned heifer raising practices. J Dairy Sci. (2018) 101:9168–84. doi: 10.3168/jds.2017-14010
9. Godden SM, Fetrow JP, Feirtag JM, Green LR, Wells SJ. Economic analysis of feeding pasteurized nonsaleable milk versus conventional milk replacer to dairy calves. J amer veter med ass. (2005) 226:1547–54. doi: 10.2460/javma.2005.226.1547
10. Selim SA, Cullor JS. Number of viable bacteria and presumptive antibiotic residues in milk fed to calves on commercial dairies. J Amer Veter Med Ass. (1997) 211:1029.
11. Walz PH, Mullaney TP, Render JA, Walker RD, Mosser T, Baker JC. Otitis media in preweaned Holstein dairy calves in Michigan due to Mycoplasma bovis. J Veter Dia Invest. (1997) 9:250–4. doi: 10.1177/104063879700900305
12. Stabel JR, Hurd S, Calvente L, Rosenbusch RF. Destruction of Mycobacterium paratuberculosis, Salmonella spp, and Mycoplasma spp. in raw milk by a commercial on-farm high-temperature, short-time pasteurizer. J dairy sci. (2004) 87:2177–183. doi: 10.3168/jds.S0022-0302(04)70038-7
13. Langford FM, Weary DM, Fisher L. Antibiotic resistance in gut bacteria from dairy calves: a dose response to the level of antibiotics fed in milk. J dairy sci. (2003) 86:3963–6. doi: 10.3168/jds.S0022-0302(03)74006-5
14. Pereira RV, Siler JD, Bicalho RC, Warnick LD. In vivo selection of resistant E. coli after ingestion of milk with added drug residues. PLoS ONE. (2014) 9:e115223. doi: 10.1371/journal.pone.0115223
15. Maynou G, Bach A, Terré M. Feeding of waste milk to Holstein calves affects antimicrobial resistance of Escherichia coli and Pasteurella multocida isolated from fecal and nasal swabs. J dairy sci. (2017) 100:2682–94. doi: 10.3168/jds.2016-11891
16. Edrington TS, Garcia Buitrago JA, Hagevoort GR, Loneragan GH, Bricta-Harhay DM, Callaway TR, et al. Effect of waste milk pasteurization on fecal shedding of Salmonella in preweaned calves. J Dairy Sci. (2018) 101:9266–74. doi: 10.3168/jds.2018-14668
17. Yu D, Sun CQ, Cao SJ, Lin T, Yuan SS, Zhang HB, et al. High prevalence of bovine viral diarrhea virus 1 in Chinese swine herds. Vet Microbiol. (2012) 159:490–3. doi: 10.1016/j.vetmic.2012.04.023
18. Buo Nav Oglia C. Natural bovine coronavirus infection in a calf persistently infected with bovine viral diarrhea virus: viral shedding, immunological features and S gene variations. Animals. (2021) 11:3350. doi: 10.3390/ani11123350
19. Elizondo-Salazar JA, Jones CM, Heinrichs AJ. Evaluation of calf milk pasteurization systems on 6 Pennsylvania dairy farms. J Dairy Sci. (2010) 93:5509–13. doi: 10.3168/jds.2010-3460
20. Calderón-Amor J, Gallo C. Dairy calf welfare and factors associated with diarrhea and respiratory disease among chilean dairy farms. Animals (Basel). (2020) 10:1115. doi: 10.3390/ani10071115
21. Ballou MA, Hanson DL, Cobb CJ, Obeidat BS, Sellers MD, Pepper-Yowell AR, et al. Plane of nutrition influences the performance, innate leukocyte responses, and resistance to an oral Salmonella enterica serotype Typhimurium challenge in Jersey calves. J Dairy Sci. (2015) 98:1972–82. doi: 10.3168/jds.2014-8783
22. Omidi-Mirzaei H, Khorvash M, Ghorbani GR, Moshiri B, Mirzaei M, Pezeshki A, et al. Effects of the step-up/step-down and step-down milk feeding procedures on the performance, structural growth, and blood metabolites of Holstein dairy calves. J Dairy Sci. (2015) 98:7975–81. doi: 10.3168/jds.2014-9260
23. Zou Y, Wang Y, Deng Y, Cao Z, Li S, Wang J. Effects of feeding untreated, pasteurized and acidified waste milk and bunk tank milk on the performance, serum metabolic profiles, immunity, and intestinal development in Holstein calves. J Anim Sci Biotechnol. (2017) 8:53. doi: 10.1186/s40104-017-0182-4
24. Wray C, Furniss S, Benham CL. Feeding antibiotic-contaminated waste milk to calves—Effects on physical performance and antibiotic sensitivity of gut flora. Br Vet J. (1990) 146:80–7. doi: 10.1016/0007-1935(90)90080-M
25. Brunton LA, Reeves HE, Snow LC, Jones JR. A longitudinal field trial assesing the impact of feeding waste milk containing antibiotic residues on the prevalence of ESBL-producing Escherichia coli in calves. Prev Vet Med. (2014) 117:403–12. doi: 10.1016/j.prevetmed.2014.08.005
26. Gaskins HR, Collier CT, Anderson DB. Antibiotics as growth promotants: mode of action. Anim Biotechnol. (2002) 13:29–42. doi: 10.1081/ABIO-120005768
27. Hulbert LE, Moisa SJ. Stress, immunity, and the management of calves1. J Dairy Sci. (2016) 99:3199–216. doi: 10.3168/jds.2015-10198
28. Kargar S, Bahadori-Moghaddam M, Ghoreishi SM, Akhlaghi A, Kanani M, Pazoki A, et al. Extended transition milk feeding for 3 weeks improves growth performance and reduces the susceptibility to diarrhea in newborn female Holstein calves. Animal. (2021) 15:100151. doi: 10.1016/j.animal.2020.100151
29. Kargar S, Roshan M, Ghoreishi SM, Akhlaghi A, Kanani M, Abedi Shams-Abadi AR, et al. Extended colostrum feeding for 2 weeks improves growth performance and reduces the susceptibility to diarrhea and pneumonia in neonatal Holstein dairy calves. J Dairy Sci. (2020) 103:8130–42. doi: 10.3168/jds.2020-18355
30. Dawson DP, Morrill JL, Reddy PG, Minocha HC, Ramsey HA. Soy protein concentrate and heated soy flours as protein sources in milk replacer for preruminant calves. J Dairy Sci. (1988) 71:1301–9. doi: 10.3168/jds.S0022-0302(88)79687-3
31. Wang S Hu F Diao Q Li S Tu Y and Bi Y. (2022). Comparison of growth performance, immunity, antioxidant capacity, and liver transcriptome of calves between whole milk and plant protein-based milk replacer under the same energy and protein levels. Antioxidants (Basel). 11:270. doi: 10.3390/antiox11020270
32. Li H, Diao QY, Zhang NF, Fan ZY. Growth, nutrient utilization and amino acid digestibility of dairy calves fed milk replacers containing different amounts of protein in the preruminant period. Asian Australasian J Anim Sci. (2008) 21:1151–8. doi: 10.5713/ajas.2008.70562
33. Johnson KF, Chancellor N, Burn CC, Wathes DC. Analysis of pre-weaning feeding policies and other risk factors influencing growth rates in calves on 11 commercial dairy farms. Animal. (2018) 12:1413–23. doi: 10.1017/S1751731117003160
34. Alugongo GM, Xiao J, Azarfar A, Liu S, Yousif MH, Ma Y, et al. Effects of milk feeding strategy and acidification on growth performance, metabolic traits, oxidative stress, and health of holstein calves. Front Anim Sci. (2022) 3. doi: 10.3389/fanim.2022.822707
35. Zhang R, Zhang WB, Bi YL, Tu Y, Beckers Y, Du HC, et al. Early feeding regime of waste milk, milk, and milk replacer for calves has different effects on rumen fermentation and the bacterial community. Animals (Basel). (2019) 9:443. doi: 10.3390/ani9070443
36. Leão JM, Lima JA, Lana ÂM, Saturnino HM, Reis RB, Barbosa FA, et al. Performance of crossbred heifers in different step-down waste milk-feeding strategies. Trop Anim Health Prod. (2016) 48:1607–12. doi: 10.1007/s11250-016-1134-2
37. Vieira SF, Coelho SG, Diniz Neto HDC, Sá HCM, Pereira BP, Albuquerque BSF, et al. Effects of Bulk Tank milk, waste milk, and pasteurized waste milk on the intake, ruminal parameters, blood parameters, health, and performance of dairy calves. Animals (Basel). 11:270. doi: 10.3390/ani11123552
38. Maynou G, Migura-Garcia L, Chester-Jones H, Ziegler D, Bach A, Terre M. Effects of feeding pasteurized waste milk to dairy calves on phenotypes and genotypes of antimicrobial resistance in fecal Escherichia coli isolates before and after weaning. J dairy sci. (2017) 100:7967–79. doi: 10.3168/jds.2017-13040
39. Vazquez-Mendoza O, Elghandour MMY, Salem AZM, Cheng L, Sun X, Lisete Garcia-Flor V, et al. Effects of sodium butyrate and active Bacillus amyloliquefaciens supplemented to pasteurized waste milk on growth performance and health condition of Holstein dairy calves. Anim Biotechnol. (2020) 31:209–16. doi: 10.1080/10495398.2019.1578785
40. Yeoman CJ, Ishaq SL, Bichi E, Olivo SK, Lowe J, Aldridge BM. Biogeographical differences in the influence of maternal microbial sources on the early successional development of the bovine neonatal gastrointestinal tract. Sci Rep. (2018) 8:3197. doi: 10.1038/s41598-018-21440-8
41. Abecia L, Martín-García AI, Martínez G, Newbold CJ, Yáñez-Ruiz DR. Nutritional intervention in early life to manipulate rumen microbial colonization and methane output by kid goats postweaning. J Anim Sci. (2013) 91:4832–40. doi: 10.2527/jas.2012-6142
42. Leticia A, Waddams KE, Gonzalo M-F, Ignacio M-GA, Eva RM, Jamie NC, et al. An antimethanogenic nutritional intervention in early life of ruminants modifies ruminal colonization by archaea. Archaea. (2014) 2014:1–12. doi: 10.1155/2014/841463
43. Yáñez-Ruiz DR, Abecia L, Newbold CJ. Manipulating rumen microbiome and fermentation through interventions during early life: a review. Front Microbiol. (2015) 6:1133–7. doi: 10.3389/fmicb.2015.01133
44. Edrington TS, Dowd SE, Farrow RF, Hagevoort GR, Callaway TR, Anderson RC, et al. Development of colonic microflora as assessed by pyrosequencing in dairy calves fed waste milk. J Dairy Sci. (2012) 95:4519–25. doi: 10.3168/jds.2011-5119
46. Liu Q, Wang C, Pei CX, Li HY, Wang YX, Zhang SL, et al. Effects of isovalerate supplementation on microbial status and rumen enzyme profile in steers fed on corn stover based diet. Livest Sci. (2014) 161:60–8. doi: 10.1016/j.livsci.2013.12.034
47. Tamate H, Mcgilliard AD, Jacobson NL, Getty R. Effect of various dietaries on the anatomical development of the stomach in the calf1. J Dairy Sci. (1962) 45:408–20. doi: 10.3168/jds.S0022-0302(62)89406-5
48. Henderson G, Cox F, Ganesh S, Jonker A, Young W, Janssen PH. Erratum: rumen microbial community composition varies with diet and host, but a core microbiome is found across a wide geographical range. Sci Rep. (2016) 6:19175. doi: 10.1038/srep19175
49. Falagas ME, Siakavellas E. Bacteroides, Prevotella, and Porphyromonas species: a review of antibiotic resistance and therapeutic options. Int J Antimicrob Agents. (2000) 15:1–9. doi: 10.1016/S0924-8579(99)00164-8
50. Niederau W, Höffler U, Pulverer G. Susceptibility of Bacteroides melaninogenicus to 45 antibiotics. Chemotherapy. (1980) 26:121–7. doi: 10.1159/000237893
51. Baldwin KA, Bitman J, Thompson MJ. Comparison of N,N-dimethyldodecanamine with antibiotics on in vitro cellulose digestion and volatile fatty acid production by ruminal microorganisms. J Anim Sci. (1982) 55:673–9. doi: 10.2527/jas1982.553673x
52. Pereira RVV, Lima S, Siler JD, Foditsch C, Bicalho RC. Ingestion of milk containing very low concentration of antimicrobials: longitudinal effect on fecal microbiota composition in preweaned calves. PLoS ONE. (2016) 11:797–806. doi: 10.1371/journal.pone.0147525
53. Anumala D, Pasupuleti MK, Nagireddy RR. Detection and characterization of prevotella intermedia and its in vitro susceptibility to selected antimicrobial agents in chronic periodontitis and acute myocardial infarction. Perio J. (2019) 3:1–6. doi: 10.26810/perioj.2019.a1
54. Poier G, Terler G, Klevenhusen F, Sharma S, Zebeli Q. Replacing concentrates with a high-quality hay in the starter feed of dairy calves: II. Effects on the development of chewing and gut fermentation, and selected systemic health variables. J Dairy Sci. (2022) 105:3113–28. doi: 10.3168/jds.2021-21346
55. Arroyo-Aguilu JA, Evans JL. Nutrient digestibility of lower-fiber rations in the ruminant animal. J Dairy Sci. (1972) 55:1266–74. doi: 10.3168/jds.S0022-0302(72)85660-1
56. Arne A, Ilgaza A. Prebiotic and synbiotic effect on rumen papilla length development and rumen pH in 12-week-old calves. Vet World. (2021) 14:2883–88. doi: 10.14202/vetworld.2021.2883-2888
57. Liu W, La ATZ, Evans A, Gao S, Yu Z, Bu D, et al. Supplementation with sodium butyrate improves growth and antioxidant function in dairy calves before weaning. J Anim Sci Biotechnol. (2021) 12:2. doi: 10.1186/s40104-020-00521-7
58. Reiten M, Rousing T, Thomsen PT, Sørensen JT. Short communication: are group size and pasteurization of whole milk associated with diarrhea and growth of pre-weaned organic dairy calves? Res Vet Sci. (2019) 123:32–4. doi: 10.1016/j.rvsc.2018.11.021
59. Guo W, van Niekerk JK, Zhou M, Steele MA, Guan LL. Longitudinal assessment revealed the shifts in rumen and colon mucosal-attached microbiota of dairy calves during weaning transition. J Dairy Sci. (2021) 104:5948–63. doi: 10.3168/jds.2020-19252
60. Varada VV, Tyagi AK, Banakar PS, Das A, Tyagi N, Mallapa RH, et al. Autochthonous Limosilactobacillus reuteri BFE7 and Ligilactobacillus salivarius BF17 probiotics consortium supplementation improves performance, immunity, and selected gut health indices in Murrah buffalo calves. Vet Res Commun. (2022). 2:1–11. doi: 10.1007/s11259-022-09896-6
61. Wang H, Yu Z, Gao Z, Li Q, Qiu X, Wu F, et al. Effects of compound probiotics on growth performance, rumen fermentation, blood parameters, and health status of neonatal Holstein calves. J Dairy Sci. (2022) 105:2190–200. doi: 10.3168/jds.2021-20721
62. Broz P, Ohlson MB, Monack DM. Innate immune response to Salmonella typhimurium, a model enteric pathogen. Gut Microbes. (2012) 3:62–70. doi: 10.4161/gmic.19141
63. Guilloteau P, Huërou-Luron IL, Chayvialle JA, Toullec R, Zabielski R, Blum JW. Gut regulatory peptides in young cattle and sheep. Zentralbl Veterinarmed A. (1997) 44:1–23. doi: 10.1111/j.1439-0442.1997.tb01082.x
64. Blum JW, Hammon H. Colostrum effects on the gastrointestinal tract, and on nutritional, endocrine and metabolic parameters in neonatal calves. Livestock Production ence. (2000) 66:151–9. doi: 10.1016/S0301-6226(00)00222-0
65. Malmuthuge N, Griebel PJ, Guan le L. The Gut Microbiome and Its Potential Role in the Development and Function of Newborn Calf Gastrointestinal Tract. Front Vet Sci. (2015) 2:36. doi: 10.3389/fvets.2015.00036
66. Malmuthuge N, Guan LL. Understanding the gut microbiome of dairy calves: opportunities to improve early-life gut health. J dairy sci. (2017) 100:5996–6005. doi: 10.3168/jds.2016-12239
67. Guzman CE, Bereza-Malcolm LT, De Groef B, Franks AE. Presence of selected methanogens, fibrolytic bacteria, and proteobacteria in the gastrointestinal tract of neonatal dairy calves from birth to 72 hours. PLoS ONE. (2015) 10:e0133048. doi: 10.1371/journal.pone.0133048
68. Sommer F, Bckhed F. The gut microbiota-Masters of host development and physiology. Nat Rev Microbiol. (2013) 11:227–38. doi: 10.1038/nrmicro2974
69. Górka P, Kowalski ZM, Pietrzak P, Kotunia A, Jagusiak W, Zabielski R. Is rumen development in newborn calves affected by different liquid feeds and small intestine development?. J Dairy Sci. (2011) 94:3002–13. doi: 10.3168/jds.2010-3499
70. Fomenky BE, Talbot G, Chiquette J, Bissonnette N, Chouinard YP, Lessard M, et al. Direct-fed microbial supplementation influences the bacteria community composition of the gastrointestinal tract of pre- and post-weaned calves. Sci Rep. (2018) 8:14147. doi: 10.1038/s41598-018-32375-5
71. Maslowski KM, Mackay CR. Diet, gut microbiota and immune responses. Nat Immunol. (2011) 12:5–9. doi: 10.1038/ni0111-5
72. Li RW, Connor EE, Li C, Baldwin RL VI, Sparks ME. Characterization of the rumen microbiota of pre-ruminant calves using metagenomic tools. Environ. Microbiol. (2012) 14:129–39. doi: 10.1111/j.1462-2920.2011.02543.x
73. Kasparovska J, Pecinkova M, Dadakova K, Krizova L, Hadrova S, Lexa M, et al. Effects of isoflavone-enriched feed on the rumen microbiota in dairy cows. PLoS ONE. (2016) 11:e0154642. doi: 10.1371/journal.pone.0154642
74. Wileman BW, Thomson DU, Reinhardt CD, Renter DG. Analysis of modern technologies commonly used in beef cattle production: conventional beef production versus nonconventional production using meta-analysis. J Anim Sci. (2009) 87:3418–26. doi: 10.2527/jas.2009-1778
75. Deng YF, Wang YJ, Zou Y, Azarfar A, Wei XL, Ji SK, et al. Influence of dairy by-product waste milk on the microbiomes of different gastrointestinal tract components in pre-weaned dairy calves. Sci Rep. (2017) 7:42689. doi: 10.1038/srep42689
76. Minamida K, Ohashi M, Hara H, Asano K, Tomita F. Effects of ingestion of difructose anhydride III (DFA III) and the DFA III-assimilating bacterium Ruminococcus productus on rat intestine. Biosci Biotechnol Biochem. (2006) 70:332–9. doi: 10.1271/bbb.70.332
77. Horinouchi M, Koshino H, Malon M, Hirota H, Hayashi T. Steroid degradation in comamonas testosteroni TA441: identification of metabolites and the genes involved in the reactions necessary before D-ring cleavage. Appl Environ Microbiol. (2018) 84:e01324–18. doi: 10.1128/AEM.01324-18
78. O'Hara E, Kelly A, Mccabe M, Kenny DA, Guan LL, Waters SM. Effect of a butyrate-fortified milk replacer on gastrointestinal microbiota and products of fermentation in artificially reared dairy calves at weaning. Sci Rep. (2018) 8:14901. doi: 10.1038/s41598-018-33122-6
79. Purushe J, Fouts DE, Morrison M, White BA, Mackie RI, Coutinho PM, et al. Comparative genome analysis of Prevotella ruminicola and Prevotella bryantii: insights into their environmental niche. Microb Ecol. (2010) 60:721–9. doi: 10.1007/s00248-010-9692-8
80. Hennessy M, Indugu N, Vecchiarelli B, Redding L, Bender J, Pappalardo C, et al. Short communication: Comparison of the fecal bacterial communities in diarrheic and nondiarrheic dairy calves from multiple farms in southeastern Pennsylvania. J Dairy Sci. (2021) 104:7225–32. doi: 10.3168/jds.2020-19108
81. Wang D, Du Y, Huang S, You Z, Zheng D, Liu Y. Combined supplementation of sodium humate and glutamine reduced diarrhea incidence of weaned calves by intestinal microbiota and metabolites changes. J Anim Sci. (2021) 99:skab305. doi: 10.1111/asj.13584
82. Badman J, Daly K, Kelly J, Moran AW, Cameron J, Watson I, et al. The effect of milk replacer composition on the intestinal microbiota of pre-ruminant dairy calves. Front Vet Sci. (2019) 6:371. doi: 10.3389/fvets.2019.00371
83. Garrido D, Dallas DC, Mills DA. Consumption of human milk glycoconjugates by infant-associated bifidobacteria: mechanisms and implications. Microbiology. (2013) 159:649–64. doi: 10.1099/mic.0.064113-0
84. Khachatryan AR. Mechanisms responsible for the maintenance of high prevalence of antimicrobial drug resistant Escherichia coli in dairy calves. Diss Abstr Int. (2005) 66–12:6472.
85. Mataseje LF, Baudry PJ, Zhanel GG, Morck DW, Read RR, Louie M, et al. Comparison of CMY-2 plasmids isolated from human, animal, and environmental Escherichia coli and Salmonella spp. from Canada Diagn Microbiol Infect Dis. (2010) 67:387–91. doi: 10.1016/j.diagmicrobio.2010.02.027
86. Feng X, Littier HM, Knowlton KF, Garner E, Pruden A. The impacts of feeding milk with antibiotic on the fecal microbiome and antibiotic resistance genes in dairy calves. Can J Anim Sci. (2019) 100:2038–117. doi: 10.1139/cjas-2018-0202
87. Lalles JP. Long term effects of pre- and early postnatal nutrition and environment on the gut. J Anim Sci. (2012) 90:421. doi: 10.2527/jas.53904
88. Mao S, Zhang R, Wang D, Zhu W. The diversity of the fecal bacterial community and its relationship with the concentration of volatile fatty acids in the feces during subacute rumen acidosis in dairy cows. BMC Vet Res. (2012) 8:237. doi: 10.1186/1746-6148-8-237
89. Bryan A, White BA, Yeoman CJ. Gut microbiomes and probiotics in production animals. Ann Rev Animal Biosci. (2014) 2:469–86. doi: 10.1146/annurev-animal-022513-114149
90. Aqib AI, Saqib M, Khan SR, Ahmad T, Yasmeen K. Nonsteroidal antiinflammatory drugs, plant extracts, and characterized microparticles to modulate antimicrobial resistance of epidemic mecA positive S. aureus of dairy origin. Appl Nanosci. (2021) 11:345–78. doi: 10.1007/s13204-020-01628-z
91. Horton RA, Uncan DD, Randall LP, Chappell S, Brunton LA, Warner R, et al. Longitudinal study of CTX-M ESBL-producing E. coli strains on a UK dairy farm. Res Vet Sci. (2016) 109:107–13. doi: 10.1016/j.rvsc.2016.09.018
92. Randall L, Heinrich K, Horton R, Brunton L, Sharman M, Bailey-Horne V, et al. Detection of antibiotic residues and association of cefquinome residues with the occurrence of Extended-Spectrum β-Lactamase (ESBL)-producing bacteria in waste milk samples from dairy farms in England and Wales in 2011. Res Vet Sci. (2014) 96:15–24. doi: 10.1016/j.rvsc.2013.10.009
93. Springer HR, Denagamage TN, Fenton GD, Haley BJ, Van Kessel JAS, Hovingh EP. Antimicrobial resistance in fecal Escherichia coli and Salmonella enterica from dairy calves: a systematic review. Foodborne Pathog Dis. (2019) 16:23–34. doi: 10.1089/fpd.2018.2529
94. Awosile BB, Smith BA. Risk assessment modelling of fecal shedding caused by extended-spectrum cephalosporin-resistant Escherichia coli transmitted through waste milk fed to dairy pre-weaned calves. J Dairy Sci. (2017) 100:9667–73. doi: 10.3168/jds.2017-13196
95. Penati M, Sala G, Biscarini F, Boccardo A, Bronzo V, Castiglioni B, et al. Feeding pre-weaned calves with waste milk containing antibiotic residues is related to a higher incidence of diarrhea and alterations in the fecal microbiota. Front Vet Sci. (2021) 8:650150. doi: 10.3389/fvets.2021.650150
96. Zhang X, Yi X, Zhuang H, Deng Z, Ma C. Invited review: antimicrobial use and antimicrobial resistance in pathogens associated with diarrhea and pneumonia in dairy calves. Animals (Basel). (2022) 12:771. doi: 10.3390/ani12060771
97. Bernier Gosselin V, Visschers VHM, Bodmer M, Meylan M. Swiss dairy farmers' perceptions surrounding the disposal of waste milk containing antibiotic residues and antibiotic resistance. Front Vet Sci. (2021) 8:787828. doi: 10.3389/fvets.2021.787828
98. Pereira R, Carroll LM, Lima S, Foditsch C, Warnick LD. Impacts of feeding preweaned calves milk containing drug residues on the functional profile of the fecal microbiota. Sci Rep. (2018) 8:554. doi: 10.1038/s41598-017-19021-2
99. Firth CLL, Kremer K, Werner T, Käsbohrer A. The effects of feeding waste milk containing antimicrobial residues on dairy calf health. Pathogens. (2021) 10:112. doi: 10.3390/pathogens10020112
100. Tetens JL, Billerbeck S, Schwenker JA, Hölzel CS. Short communication: selection of extended-spectrum β-lactamase-producing Escherichia coli in dairy calves associated with antibiotic dry cow therapy-A cohort study. J Dairy Sci. (2019) 102:11449–52. doi: 10.3168/jds.2019-16659
101. Wickramasinghe H, Anast JM, Schmitz-Esser S, Serão NVL, Appuhamy J. Beginning to offer drinking water at birth increases the species richness and the abundance of Faecalibacterium and Bifidobacterium in the gut of preweaned dairy calves. J Dairy Sci. (2020) 103:4262–74. doi: 10.3168/jds.2019-17258
102. Stone B. Waste milk, milk replacer or pasteurized waste milk Which one of these makes the most sense for your dairy's calves? J dairy sci. (2012) 8:217–83.
103. Maynou G, Chester-Jones H, Bach A, Terré M. Feeding pasteurized waste milk to preweaned dairy calves changes fecal and upper respiratory tract microbiota. Res Vet Sci. (2019) 6:159. doi: 10.3389/fvets.2019.00159
104. Godden S, Fetrow J, Feirtag J, Wells S, Green L. A review of issues surrounding the feeding of pasteurized nonsaleable milk and Colostrum. Animal. (2015) 21:67–89.
105. Maryana D, Malaka R, Maruddin F. Antibacterial activity of pasteurized milk supplemented with binahong leaf extract (Anredera cordifolia (Ten) Steenis) and sukrose toward Escherichia coli and Staphylococcus aureus. IOP Conf Ser Earth Environ Sci. (2019) 247:012065. doi: 10.1088/1755-1315/247/1/012065
106. Garcia JA, Edrington TS, Hagevoort GR, Farrow RF, Nisbet DJ. The effect of feeding pasteurized or non-pasteurized waste milk on fecal populations and prevalence of Salmonella in dairy calves. ADSA-ASAS Joint Annu Meet. (2015) 11:3472–875.
107. Al Mawly J, Grinberg A, Prattley D, Moffat J, Marshall J, French N. Risk factors for neonatal calf diarrhoea and enteropathogen shedding in New Zealand dairy farms. Vet J. (2015) 203:155–60. doi: 10.1016/j.tvjl.2015.01.010
108. Magrin L, Brscic M, Cozzi G, Armato L, Gottardo F. Prevalence of gastrointestinal, liver and claw disorders in veal calves fed large amounts of solid feed through a cross-sectional study. Res Vet Sci. (2020) 133:318–25. doi: 10.1016/j.rvsc.2020.10.022
109. Opgenorth J, Sordillo LM, Gandy JC, VandeHaar MJ. Colostrum supplementation with n-3 fatty acids does not alter calf outcome on a healthy commercial farm. J Dairy Sci. (2020) 103:11689–96. doi: 10.3168/jds.2019-18046
110. Yousif M, Li JH, Li ZQ, Alugongo GM, Ji SK, Li YX, et al. Low concentration of antibiotics modulates gut microbiota at different levels in pre-weaning dairy calves. Microorganisms. (2018) 6:118. doi: 10.3390/microorganisms6040118
111. Xie G, Duff GC, Hall LW, Allen JD, Burrows CD, Bernal-Rigoli JC, et al. Alteration of digestive tract microbiome in neonatal Holstein bull calves by bacitracin methylene disalicylate treatment and scours. J Anim Sci. (2013) 91:4984–90. doi: 10.2527/jas.2013-6304
112. Weese JS, Jelinski M. Assessment of the fecal microbiota in beef calves. J Inter Med. (2017) 31:176–85. doi: 10.1111/jvim.14611
113. Friedman ND, Kaye KS, Stout JE, McGarry SA, Trivette SL, Briggs JP, et al. Health care—associated bloodstream infections in adults: a reason to change the accepted definition of community-acquired infections. Ann Intern Med. (2002) 137:791–7. doi: 10.7326/0003-4819-137-10-200211190-00007
114. López E, Blázquez J. Effect of subinhibitory concentrations of antibiotics on intrachromosomal homologous recombination in Escherichia coli. Antimicrob Agents Chemother. (2009) 53:3411–5. doi: 10.1128/AAC.00358-09
115. Thi TD, López E, Rodríguez-Rojas A, Rodríguez-Beltrán J, Couce A, Guelfo JR, et al. Effect of recA inactivation on mutagenesis of Escherichia coli exposed to sublethal concentrations of antimicrobials. J Antimicrob Chemother. (2011) 66:531–8. doi: 10.1093/jac/dkq496
116. WHO. Antimicrobial resistance: Global report on surveillance. Australasian Med J. (2014) 7:237.
117. Moore DA, Taylor J, Hartman ML, Sischo WM. Quality assessments of waste milk at a calf ranch. J Dairy Sci. (2009) 92:3503–09. doi: 10.3168/jds.2008-1623
118. Schaffer LV, Mcguffey RK. Effects of feeding fermented mastitic milk to calves. Meet Natl Mastitis Council. (1980) 23:372–457.
119. Parker AM, House JK, Hazelton MS, Bosward KL, Mohler VL, Maunsell FP, et al. Milk acidification to control the growth of Mycoplasma bovis and Salmonella Dublin in contaminated milk. J dairy sci. (2016) 99:9875–84. doi: 10.3168/jds.2016-11537
120. Godden S, McMartin S, Feirtag J, Stabel J, Bey R, Goyal S, et al. Heat-treatment of bovine colostrum. II: effects of heating duration on pathogen viability and immunoglobulin G. J Dairy Sci. (2006) 89:3476–83. doi: 10.3168/jds.S0022-0302(06)72386-4
121. Butler JA, Sickles SA, Johanns CJ, Rosenbusch RF. Pasteurization of discard mycoplasma mastitic milk used to feed calves: thermal effects on various mycoplasma. J dairy sci. (2008) 83:2285–8. doi: 10.3168/jds.S0022-0302(00)75114-9
122. Baumgartener L, Olson C, Onuma M. Effect of pasteurization and heat treatment on bovine leukemia virus. J Am Vet Med Assoc. (1976) 169:1189–91.
123. Fayer. Effect of high temperature on infectivity of Cryptosporidium parvum oocysts in water. Appl Environ Microbiol. (1994) 60:2732–5. doi: 10.1128/aem.60.8.2732-2735.1994
124. Morrill KM, Polo J, Lago A, Campbell J, Quigley J, Tyler H. Estimate of serum immunoglobulin G concentration using refractometry with or without caprylic acid fractionation. J dairy sci. (2013) 96:4535–41. doi: 10.3168/jds.2012-5843
125. Deelen SM, Ollivett TL, Haines DM, Leslie KE. Evaluation of a Brix refractometer to estimate serum immunoglobulin G concentration in neonatal dairy calves. J Dairy Sci. (2014) 97:3838–44. doi: 10.3168/jds.2014-7939
126. Yi Z, Qiyu D, Yan T, Qiang Y. Effects of Yeast β-glucan on Gastrointestinal Development in Early-weaning Calves. Chinese J Anim Nutr. (2009) 0:846–52. Available online at: http://www.chinajan.com/CN/Y0/V/I/846
127. Robblee ED, Erickson PS, Whitehouse NL, McLaughlin AM, Schwab CG, Rejman JJ, et al. Supplemental lactoferrin improves health and growth of Holstein calves during the preweaning phase. J Dairy Sci. (2003) 86:1458–64. doi: 10.3168/jds.S0022-0302(03)73729-1
128. Yuangklang C, Wensing T, Van D, Jittakhot S, Beynen AC. Fat digestion in veal calves fed milk replacers low or high in calcium and containing either casein or soy protein isolate. J dairy sci. (2004) 87:1051–6. doi: 10.3168/jds.S0022-0302(04)73251-8
129. Egli CP, Blum JW. Clinical, haematological, metabolic and endocrine traits during the first three months of life of suckling simmentaler calves held in a cow-calf operation. Zentralbl Veterinarmed A. (1998) 45:99–118. doi: 10.1111/j.1439-0442.1998.tb00806.x
130. De Almeida Santana MH, Junior GA, Cesar AS, Freua MC, da Costa Gomes R, da Luz ESS, et al. Copy number variations and genome-wide associations reveal putative genes and metabolic pathways involved with the feed conversion ratio in beef cattle. J Appl Genet. (2016) 57:495–504. doi: 10.1007/s13353-016-0344-7
131. Liu Q, Wang C, Guo G, Huo WJ, Zhang YL, Pei CX, et al. Effects of isovalerate supplements on morphology and functional gene expression of rumen mucosa in pre- and post-weaning dairy calves. Animal. (2018) 12:491–500. doi: 10.1017/S175173111700194X
132. Drackley JK. Calf Nutrition from Birth to Breeding. Vet Clin N Am Food Animal Pract. (2008) 24:55–86. doi: 10.1016/j.cvfa.2008.01.001
133. Yang M, Zou Y, Wu ZH, Li SL, Cao ZJ. Colostrum quality affects immune system establishment and intestinal development of neonatal calves. J Dairy Sci. (2015) 98:7153–63. doi: 10.3168/jds.2014-9238
134. Bai H, Ukita H, Kawahara M, Mitani T, Furukawa E, Yanagawa Y, et al. Effect of summer heat stress on gene expression in bovine uterine endometrial tissues. Anim Sci J. (2020) 91:e13474. doi: 10.1111/asj.13474
135. Li Y, Zou S, Ding H, Hao N, Huang Y, Tang J, et al. Low expression of sirtuin 1 in the dairy cows with mild fatty liver alters hepatic lipid metabolism. Animals (Basel). (2020) 10:560–77. doi: 10.3390/ani10040560
136. Jiang ZY, Jiang SQ, Lin YC, Xi PB, Yu DQ, Wu TX. Effects of soybean isoflavone on growth performance, meat quality, and antioxidation in male broilers. Poult Sci. (2007) 86:1356–62. doi: 10.1093/ps/86.7.1356
137. Wang YZ, Xu CL, An ZH, Liu JX, Feng J. Effect of dietary bovine lactoferrin on performance and antioxidant status of piglets. Anim Feed Sci Technol. (2008) 140:326–36. doi: 10.1016/j.anifeedsci.2007.02.006
138. Halliwell B, Chirico S. Lipid peroxidation: Its mechanism, measurement, and significance. Am J Clin Nutr. (1993) 57:715S–24S. doi: 10.1093/ajcn/57.5.715S
139. Sumida S, Tanaka K, Kitao H, Nakadomo F. Exercise-induced lipid peroxidation and leakage of enzymes before and after vitamin E supplementation. Int J Biochem. (1989) 21:835–8. doi: 10.1016/0020-711x(89)90280-2
140. Chang-Wen W, Wei-Dong Y, Wen-Yan Y, Wen-Fa LV, Lian-Yu Y. Effect of Acanthopanax Synbiotics on Serum TAOC,IgG and IgA of Lactation Calves. Chinese J Veter Med. (2015) 51:48–50.
141. Maizels R. Immune regulation by helminth parasites : cellular and molecular mechanisms. Nat Rev Immunol. (2003) 3:733–44. doi: 10.1038/nri1183
142. Nizar S, Copier J, Meyer B, Bodman-Smith M, Galustian C, Kumar D, et al. T-regulatory cell modulation: the future of cancer immunotherapy?. Br J Cancer. (2009) 100:1697–703. doi: 10.1038/sj.bjc.6605040
143. Bensaude E, Turner JLE, Wakeley PR, Sweetman DA, Pardieu C, Drew TW, et al. Classical swine fever virus induces proinflammatory cytokines and tissue factor expression and inhibits apoptosis and interferon synthesis during the establishment of long-term infection of porcine vascular endothelial cells. J Gen Virol. (2004) 85:1029–37. doi: 10.1099/vir.0.19637-0
144. Tarradas J, Argilaguet JM, Rosell R, Nofrarías M, Crisci E, Córdoba L, et al. Interferon-gamma induction correlates with protection by DNA vaccine expressing E2 glycoprotein against classical swine fever virus infection in domestic pigs. Vet Microbiol. (2010) 142:51–8. doi: 10.1016/j.vetmic.2009.09.043
145. Perdijk O, van Splunter M, Savelkoul HFJ, Brugman S, van Neerven RJJ. Cow's milk and immune function in the respiratory tract: potential mechanisms. Front Immunol. (2018) 9:143. doi: 10.3389/fimmu.2018.00143
146. Yin S, Yongli QU, Gao Y, Xin X, Wang L, Zhang S. Effects of acidified milk on growth performance and serum immune indexes of holstein calves. Chinese J Anim Nutri. (2019) 31:1655–65.
147. Chardavoyne JR, Ibeawuchi JA, Kesler EM, Borland KM. Waste Milk from Antibiotic Treated Cows as Feed for Young Calves. J dairy sci. (1979) 62:1285–9. doi: 10.3168/jds.S0022-0302(79)83413-X
148. Pearce LE, Truong HT, Crawford RA, Yates GF, Cavaignac S, de Lisle GW. Effect of turbulent-flow pasteurization on survival of Mycobacterium avium subsp. paratuberculosis added to raw milk. Appl Environ Microbiol. (2001) 67:3964–9. doi: 10.1128/AEM.67.9.3964-3969.2001
149. Hammer P, Kiesner C, Walte HG, Knappstein K, Teufel P. Heat resistance of Mycobacterium avium ssp. paratuberculosis in raw milk tested in a pilot plant pasteurizer. Kieler Milchwirtschaftliche Forschungsberichte. (2002) 54:275–303.
150. McDonald WL, O'Riley KJ, Schroen CJ, Condron RJ. Heat inactivation of Mycobacterium avium subsp. paratuberculosis in milk. Appl Environ Microbiol. (2005) 71:1785–9. doi: 10.1128/AEM.71.4.1785-1789.2005
151. Grant IR, Ball HJ, Rowe MT. Effect of higher pasteurization temperatures, and longer holding times at 72°C, on the inactivation of Mycobacterium paratuberculosis in milk. Lett Appl Microbiol. (1999) 28:461–5. doi: 10.1046/j.1365-2672.1999.00557.x
152. Wilson DJ, Goodell GM, Kelly T. On-farm pasteurized milk fed to dairy calves—association of bacteria counts following pasteurization with season, temperature and time until feeding. J Veter Sci Tech. (2012) 3:3347–574. doi: 10.4172/2157-7579.1000124
153. Jamaluddin AA, Carpenter TE, Hird DW, Thurmond MC. Economics of feeding pasteurized colostrum and pasteurized waste milk to dairy calves. J amer veter med ass. (1996) 209:751–6.
154. Todd CG, Leslie KE, Millman ST, Bielmann V, Anderson NG, Sargeant JM, et al. Clinical trial on the effects of a free-access acidified milk replacer feeding program on the health and growth of dairy replacement heifers and veal calves. J dairy sci. (2017) 100:713–25. doi: 10.3168/jds.2016-11401
155. Jaster EH, McCoy GC, Tomkins T, Davis CL. Feeding acidified or sweet milk replacer to dairy calves. J dairy sci. (1990) 73:3563–6. doi: 10.3168/jds.S0022-0302(90)79056-X
156. Trujillo AJ, Castro N, Quevedo JM, Argüello A, Capote J, Guamis B. Effect of heat and high-pressure treatments on microbiological quality and immunoglobulin G stability of caprine colostrum. J Dairy Sci. (2007) 90:833–9. doi: 10.3168/jds.S0022-0302(07)71567-9
157. Rubino MJ, Donham KJ. Inactivation of bovine leukemia virus-infected lymphocytes in milk. Am J Vet Res. (1984) 45:1553–6.
158. Harp JA, Fayer R, Pesch BA, Jackson GJ. Effect of pasteurization on infectivity of Cryptosporidium parvum oocysts in water and milk. Appl Environ Microbiol. (1996) 62:2866–8. doi: 10.1128/aem.62.8.2866-2868.1996
159. Meylan M, Rings DM, Shulaw WP, Kowalski JJ, Bechnielsen S, Hoffsis GF. Survival of Mycobacterium paratuberculosis and preservation of immunoglobulin G in bovine colostrum under experimental conditions simulating pasteurization. Am J Vet Res. (1996) 57:1580–5.
160. Millar D, Ford J, Sanderson J, Withey S, Tizard M, Doran T, et al. IS900 PCR to detect Mycobacterium paratuberculosis in retail supplies of whole pasteurized cows' milk in England and Wales. Appl Environ Microbiol. (1996) 62:3446–52. doi: 10.1128/aem.62.9.3446-3452.1996
161. Grant IR, Hitchings EI, McCartney A, Ferguson F, Rowe MT. Effect of commercial-scale high-temperature, short-time pasteurization on the viability of Mycobacterium paratuberculosis in naturally infected cows' milk. Appl Environ Microbiol. (2002) 68:602–7. doi: 10.1128/AEM.68.2.602-607.2002
162. Jorgensen MA, Hoffman PC, Nytes AJ. A field survey of on-farm milk pasteurization efficacy. Prof Animal Scientist. (2006) 22:472–6. doi: 10.15232/S1080-7446(15)31149-9
163. Chirollo C, Vassallo A, Dal Piaz F, Lamagna B, Tortora G, Neglia G, et al. Investigation of the persistence of Penicillin G and dihydrostreptomycin residues in milk of lactating buffaloes (Bubalus bubalis) using ultra-high-performance liquid chromatography and tandem mass spectrometry. J Agric Food Chem. (2018) 66:6388–93. doi: 10.1021/acs.jafc.8b00229
164. Fechner K, Dreymann N, Schimkowiak S, Czerny CP, Teitzel J. Efficacy of dairy on-farm high-temperature, short-time pasteurization of milk on the viability of Mycobacterium avium ssp. paratuberculosis J Dairy Sci. (2019) 102:11280–90. doi: 10.3168/jds.2019-16590
Keywords: waste milk, microbiota, antibiotic residues, pasteurization, growth performance, rumen fermentation
Citation: Ma Y, Khan MZ, Xiao J, Alugongo GM, Chen X, Li S, Wang Y and Cao Z (2022) An Overview of Waste Milk Feeding Effect on Growth Performance, Metabolism, Antioxidant Status and Immunity of Dairy Calves. Front. Vet. Sci. 9:898295. doi: 10.3389/fvets.2022.898295
Received: 20 March 2022; Accepted: 19 April 2022;
Published: 17 May 2022.
Edited by:
Xuezhao Sun, Jilin Agricultural Science and Technology University, ChinaReviewed by:
Lorenzo Fraile, Universitat de Lleida, SpainCopyright © 2022 Ma, Khan, Xiao, Alugongo, Chen, Li, Wang and Cao. This is an open-access article distributed under the terms of the Creative Commons Attribution License (CC BY). The use, distribution or reproduction in other forums is permitted, provided the original author(s) and the copyright owner(s) are credited and that the original publication in this journal is cited, in accordance with accepted academic practice. No use, distribution or reproduction is permitted which does not comply with these terms.
*Correspondence: Zhijun Cao, Y2FvemhpanVuQGNhdS5lZHUuY24=
†These authors have contributed equally to this work
Disclaimer: All claims expressed in this article are solely those of the authors and do not necessarily represent those of their affiliated organizations, or those of the publisher, the editors and the reviewers. Any product that may be evaluated in this article or claim that may be made by its manufacturer is not guaranteed or endorsed by the publisher.
Research integrity at Frontiers
Learn more about the work of our research integrity team to safeguard the quality of each article we publish.