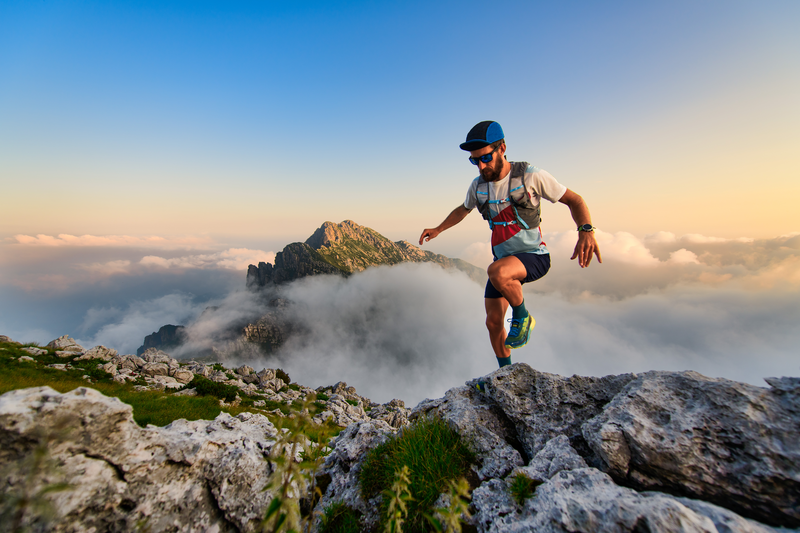
94% of researchers rate our articles as excellent or good
Learn more about the work of our research integrity team to safeguard the quality of each article we publish.
Find out more
ORIGINAL RESEARCH article
Front. Vet. Sci. , 11 July 2022
Sec. Veterinary Humanities and Social Sciences
Volume 9 - 2022 | https://doi.org/10.3389/fvets.2022.897287
This article is part of the Research Topic Women in Veterinary Humanities and Social Sciences: 2021 View all 5 articles
Negative stress due to human handling has been reported for a number of domestic animals, including dogs. Many companion dogs display significant stress during routine care in the veterinary clinic, risking injury to staff and potentially compromising the quality of care that these dogs receive. On the other hand, positive interactions with humans can have a beneficial effect on dogs, particularly in stressful situations such as animal shelters. Research has shown that dogs can detect human emotions through visual, auditory, and chemical channels, and that dogs will exhibit emotional contagion, particularly with familiar humans. This study investigated relationships between emotional states of dogs and unfamiliar human handlers, using simultaneous measures of cardiac activity and behavior, during two sessions of three consecutive routine handling sets. Measures of cardiac activity included mean heart rate (HRmean), and two measures of heart rate variability (HRV): the root mean square of successive differences between normal heartbeats (RMSSD); and the high frequency absolute power component of HRV, log transformed (HFlog). We also assessed human handlers' emotional state during handling sessions following an intervention designed to reduce stress, compared with sessions conducted on a different day and following a control activity. Polar H10 cardiac sensors were used to simultaneously record cardiac activity for both canine and human participants, and behavioral data were collected via digital video. The strongest influence on the dogs' stress levels in our study was found to be increasing familiarity with the setting and the handler; HRmean and SI decreased, and HRV (as RMSSD) increased, significantly from the first to the third handling set. Canine HRV (as HFlog) was also highest in set 3, although the difference was not statistically significant. There were no strong patterns found in the human cardiac data across handling set, session, or by pre-handling activity. We did not find consistent support for emotional contagion between the dogs and their handlers in this study, perhaps due to the brief time that the dogs spent with the handlers. Recommendations for application to dog handling, and limitations of our methods, are described.
Despite their dedication to animal wellbeing, many animal-care and veterinary professionals suffer from high levels of work-related stress, and this can result in compassion fatigue, burn-out, clinical depression and other signs of chronic stress (1–6). Unresolved chronic stress in animal care workers can jeopardize both staff and animals, as staff may be insensitive to animal stress and inured to risks while handling highly-stressed animals, which may compromise the quantity and quality of the care these animals receive (7). Negative stress due to human handling has been documented in numerous domestic and laboratory animals including dogs, Canis lupus familiaris (8–15). Although regular veterinary visits are an essential part of caring for companion dogs, many dogs display significant stress at the veterinary clinic. In one recent survey-based study of >26,500 dog owners, over 50% of dogs were reported to display fear at the veterinary clinic, ranging from mild to intense (16). The environment and human interactions, rather than dog characteristics like breed or age, are likely to be the primary drivers of the prevalence and severity of this issue (16). On the other hand, positive interactions between friendly humans and shelter dogs have been shown to have a beneficial effect on dogs by reducing their stress level (17–20).
When assessing canine stress during human-animal interactions, it is important to note that domestic dogs can detect human emotion through visual, auditory, and chemical channels (21–23). Dogs have been reported to use social referencing with their human companions, with the emotional reaction of the human influencing that of the dog (24); and emotional contagion between humans and dogs has been reported, especially in female dogs and with duration of the relationship playing a role (25). Research suggests that factors such as owner personality, human-animal interactions, and choice of training methods, particularly over extended time periods, can all impact companion animal behavior and welfare (26–34). If, during human-animal interactions of shorter duration (such as in a veterinary clinic or animal shelter), the emotional state of the human can influence the emotional state of the animal, then emotional state of human caretakers may play an indirect role in animal welfare, in addition to any direct (behavior-based) impacts that may occur. The goal of the present study was to assess whether the emotional state of a human handler is associated with the emotional state of a dog during routine handling exercises, when the handler is unfamiliar to the dog, and the duration of the interactions brief. To help assess whether emotional contagion occurs in these circumstances, handling exercises were conducted before and after the addition of a mild verbal (psychological) stressor to the human. If human stress can be detected by the animals during direct interactions such as these, and thus impact the stress levels of these animals under veterinary or shelter care, any steps that could be taken to reduce or mitigate stressors for both humans and non-humans would seem advisable, in order to maintain high standards of care for companion animals (7, 11).
Stress levels of dogs and humans can be assessed in various ways, including behavioral measures (body language, behaviors indicative of stress) and physiological stress responses, such as measurement of stress hormones or changes in cardiac activity. Cardiac activity, particularly heart rate variability (HRV), has frequently been used as an indicator of stress and emotional state in human and non-human animals (9, 35–39).
HRV describes variations of instantaneous heartbeat intervals (i.e., the time intervals between successive heartbeats, aka RR intervals) and reflects changes in activity of the autonomic nervous system (ANS), which (among other functions) is the primary mechanism in control of the “fight-or-flight” response. HRV provides a reliable index of cardiac vagal tone, which represents the contribution of the parasympathetic branch of the ANS (responsible for the “fight-or-flight” response) to cardiac regulation (40) and is, thus, linked to emotion. Research supports that HRV may be a significant indicator of important body functions associated with stress, adaptability and health (41). In general, lower HRV at rest is correlated with stress, anxiety, worry or panic (42). HRV has also been widely used to assess stress and emotional state in animals in response to environmental variables (35). For example, Kuhne et al. (9) used real-time measurement of HRV to document increased emotional stress in dogs (indicated by increased heart rate, HR, and reduced HRV) exposed to certain types of handling. Changes in HRV can occur in the absence of detectable alteration in heart rate (35), and thus are considered a more reliable indicator of emotional state than heart rate alone. For example, in a study of lamb responses to aversive events, learning to control an aversive event was associated with elevated HRV, but was not reflected in heart rate; while lack of control over the environment was associated with a decreased HRV, suggesting greater sympathetic control over cardiac activity (36, 37). Therefore, methods for HRV analysis based on measurement of interbeat intervals, such as those used in this study, allow for a more detailed interpretation of cardiac activity in terms of ANS activity (43). Heart rate is continuously monitored and recorded during study interventions, using non-invasive electrodes placed on the animal's skin which continuously transmit data to the heart rate monitor; HRV parameters are then calculated from the recorded HR data, using specifically-designed software, for comparison between participant states (e.g., baseline vs. intervention).
HRV has been successfully used in the study of emotion in domestic dogs in a number of recent studies [e.g., (8, 9, 44, 45)]. Maros et al. (39) used a harness-mounted telemetric system for ambulatory measurement of RR intervals [ISAX (46)] on dogs, and reported that HR increased during activity; no changes were seen in HRV based on body position or movement. In that study, HRV did increase when dogs oriented toward a favorite toy (and when petting by an unfamiliar individual ceased), leading the authors to conclude that HRV could be a good indicator of the dogs' attentive state (39).
This study investigated relationships between emotional states and stress levels of human handlers and domestic dogs, using simultaneous measures of cardiac activity and behavior of human and dog during routine handling in a veterinary clinic setting, in both non-stressful and stressful handling contexts. In addition, the study assessed the impact of an intervention activity designed to reduce stress on the human handler's emotional state during handling. Cardiac activity and behavior during handling following the intervention were compared to these variables following a control activity not specifically designed to be calming. Our hypotheses were that (1) increased stress in the human handler would be reflected in increased stress in the dog being handled; and (2) an intervention designed to reduce the human handler's stress level would be reflected in lower stress levels in both the human and the dog during the subsequent handling session.
All research protocols for this study were reviewed and approved by the University of California at Davis' Institutional Animal Care and Use Committee (IACUC), protocol approval #20756, and Institutional Research Board (IRB) for human subjects, protocol approval #1313227-1. For this study, 40 adult dogs and their owners were recruited from the university community. Healthy adult (>1 yr old) dogs of any breed and larger than 20 lbs (9.1 kg) were eligible to participate. The minimum size limit was put in place because it was difficult to fit the HR monitor chest strap to dogs <20 lbs in such a way as to allow consistent HR data from these dogs. Dogs were screened prior to inclusion in the study to ensure the safety of our research participants and student research assistants; dogs with a known history of excessive fear or aggression toward humans or other dogs were not included in the study. To maximize generalizability to companion dogs, dogs who have undergone unusually high levels of socialization and obedience training (such as working, assistance, or therapy dogs) were not included in the study. Participants were offered an incentive ($50 Amazon.com gift card) for completing the two data collection sessions.
To investigate potential impacts of the handler's emotional state on the dog's emotional state, during each research session, each handler and dog pair completed three identical sets of handling exercises, with a short (2-min) break between sets (detailed description of these exercises is provided below). Just prior to the start of the third set of handling exercises, a mild verbal stressor was introduced by the researcher (Figure 1); the intent was to influence the emotional state of the handler, by putting the handler on alert for a potentially stressful or aversive event, without the stressor directly impacting the dog [see (47) for a similar experimental design involving horses].
To investigate potential impacts of an intervention designed to reduce animal handler stress on the outcomes of our experiment, each dog/human pair came in for two data collection sessions 1-week apart and at approximately the same time of day (Figure 1). Prior to direct interaction with the unfamiliar dogs, human participants participated in either a brief mindfulness meditation activity, or in a control activity (detailed description of the pre-handling activities is provided below). Mindfulness meditation activities have been shown to improve resilience in workers employed in high stress professions (48), to help with emotion and behavioral regulation (49), and to increase positive emotions (50). Order of participation in the control vs. mindfulness activities was alternated for a balanced cross-over design, such that half the study participants did the mindfulness activity in session 1, followed by the control activity in session 2 (1 week later); the other participants did these activities in reverse order.
Thirty minutes prior to the start of the handling exercise, dogs and humans were fitted with Polar H10 (Polar Electro Oy; Kempele, Finland) non-invasive cardiac sensors on Polar Pro soft straps (containing two electrodes). Polar HR monitors have been validated for use in reliably measuring HRV in stationary dogs (51), although some researchers have expressed concern about their validity and reliability compared to electrocardiogram (ECG) data, particularly when the animal is moving [for pigs (52); for horses (53)]. However, Essner et al. (54) assessed the use of Polar HR monitors on standing dogs and when dogs were in motion (trotting on a treadmill), and concluded that the criterion validity and instrument reliability of the Polar monitors were excellent, and the standard error of measurement was low (54). They noted that the measurement error was comparable to ECG, with the Polars both under- and overestimating HR, which may have particular importance in the clinical setting. Our goal in the present study, using a within-subject research design, was to use the Polar HR monitors to compare cardiac activity parameters of dogs (and humans) during handling. For our purposes, clinical accuracy of the Polar monitors in measuring cardiac activity was less important than instrument reliability, which is reported to be high (54), across our experimental conditions.
For the dogs, a strip of hair approximately 2 inches (5.1 cm) wide x 12 inches (30.5 cm) long was shaved from each dog's chest between the axillae (armpits), and ultrasound gel (Spectra 360; Parker Labs, Fairfield, NJ) applied between sensors and skin to ensure complete contact with the canine subject's skin, and allow continuous HR readings. The HR monitor and strap were then further secured using elastic veterinary bandage material (Vet Wrap; WildCow, Marietta, GA), to minimize electrode shifting during the dog's movement. Low-stress handling techniques were used for HR monitor fitting, to avoid unnecessary stress to the dogs. If any dog showed signs of marked distress and/or defensive aggression during the HR monitor fitting (or later, during handling exercises), the session was immediately ended and the dog returned to his/her owner. For humans, Polar H10 monitors on chest straps were fitted to human volunteers by the researchers, with ultrasound gel applied between the sensors and skin. The HR monitors were connected via Bluetooth® to Apple iPhone (Apple, Inc.; Cupertino, CA) smartphones running the Heart Rate Variability Logger app (Altini, M.; A.S.M.A. B.V.); after the data collection was complete, cardiac data files were uploaded via the file hosting service Dropbox™ (Dropbox, Inc.; San Francisco, CA) for storage and later analysis.
After the HR monitors were securely fitted and connectivity to the smartphones confirmed, both canine and human participants were allowed 10 min to acclimate to wearing the HR sensor equipment; baseline cardiac activity data was collected during this time window. As human and canine participants could be both sitting and standing during the handling sets, the 10-min HR baseline time was divided into two segments; 5 min of participants sitting down, and 5 min standing. Canine participants were separated from their owners and spent 10 min with a trained research assistant, in a quiet area away from the testing room, during baseline data collection.
Prior to beginning the handling exercises, human participants (without their dog present) participated in either a brief (10-min) mindfulness meditation activity, or in a 10-min control activity (either reading a short informational pamphlet on dogs, or engaged in a casual conversation on neutral topics with a trained student research assistant). The reading and conversation activities were briefly introduced by a research assistant, to better match the mindfulness activity setting, and were conducted in the same room as the mindfulness activity. For the mindfulness activity, following a 5-min introduction by a trained instructor, dog handlers were led in a 5-min guided mindfulness meditation that included breath focused attention; open monitoring of the transitory of sensory experiences, thoughts and emotions; and concluding with a mindful loving kindness meditation. All potentially emotion-inducing items (such as pet memorial brochures, artwork, etc.) were removed from the pre-handling activity room prior to use.
Polar H10 HR monitors were used to continuously track HR (allowing calculation of heart rate variability, HRV, using associated software) of both handler and dog, during a session consisting of three short, nearly identical routine handling exercises (see below for differences).
To avoid the confounding effects of the previous relationship between owner/dog on the results (and, to increase applicability to interactions occurring in animal care settings such as shelters and veterinary clinics), two sessions, involving two handler/dog dyads, were conducted simultaneously, in separate rooms. Prior to the handling exercises, dogs were exchanged between participating owners, so that human handlers were not working with their own dog. Rooms used for this study were standard veterinary clinic exam rooms, located at the Center for Companion Animal Health (CCAH; School of Veterinary Medicine, University of California, Davis). All data collection sessions were conducted on weekends when the CCAH was closed, to minimize impacts of other dogs or human activity on the research participants. Data collection took place from April–December 2019, prior to Covid-19 pandemic restrictions and mask mandates.
Once the dog and handler were in the research study room, they were allowed 5 min to acclimate to the room (Figure 1). During the acclimation period, handlers were allowed soft verbal and physical contact with the dogs. After this acclimation period, participants were led (via verbal instructions) through the handling exercises by a trained research assistant. A second research assistant filmed all handling exercises using a Canon Vixia HF R800 HD digital camcorder (Canon, Inc.; Tokyo, Japan) for later analysis of behavioral data. Interactions were synchronized to the HR data by marking the exact (simultaneous) start time for both recordings, and/or by using the “event annotation” function in the HR data logger app. Research assistants present in the testing room could interact verbally with the human participants for the purposes of providing handling protocols, but were instructed to not interact directly with the dogs during data collection.
All handling exercises were conducted on the floor, with the handler seated on a stool or on the floor. Dogs wore a flat buckle collar and a short leash; use of the leash was minimized during handling exercises. Handlers were instructed to use only low-stress handling approaches with the dogs (e.g., no use of force or firm restraint, no punishment of any kind, maintain gentle voice and handling; food treats were available to distract or lure dogs as needed). Handling exercises consisted of items 1 (“Look”), 2 (“Touch Sensitivity”) and 4 (“Squeeze”) from the ASPCA's SAFER behavioral assessment (55) (Table 1); each assessment item takes between 30 s and 2 min. If the handler completed the handling exercises within a given set in <2 min., they were asked to repeat the same exercises until the 2-min set time was reached. Three handling sets were performed during each research session. Following each handling set, there was a 2-min break period for the handler and the dog.
Just prior to the start of the last of the three handling sets, the verbal stressor was introduced; two forms of added psychological stressor were used in this study. For the first 3 months of data collection, the handler was told that a research assistant might bring another unfamiliar dog into the testing room, and, as the dog they were handling was mildly dog reactive, they should be prepared for a negative reaction from the handled dog. This stressor was designed to put the handler on alert for a strong reaction from the dog they were handling, including the possibility of dog-dog aggression in the confined space of the testing room. No additional dog was brought into the room. In order to maintain the handler's belief that a dog might enter the room, the handlers were told that the days on which the additional dog would be brought into the room were randomly assigned (so, they might need to deal with the second dog on one, both, or neither of their two sessions). At the study midpoint, and based on preliminary analysis of behavioral data collected to date (comparison of canine stress levels in handling sets 1 and 2 vs. 3; see “Data analysis,” below), it was decided that this stressor might not be sufficient to significantly increase the stress level of the human participants. This was supported by anecdotal observations by the research team of human participants' lack of marked behavior change following introduction of the initial stressor. For this reason, for the remainder of data collection, an alternate anticipatory stressor was used: the handlers were told (again, just prior to beginning the third handling set) that their performance on the handling exercises in the third and final set would be evaluated by the research team using the video recordings, with the participant who performed the exercises the most accurately (and with no prompting from research assistants) awarded an additional $50 gift card. In both cases, the third handling exercise (post-stressor) was in fact identical to the previous two (pre-stressor), other than the introduction of a verbal stressor designed to influence the attitude/emotional state of the handler via increased psychological stress. It should be noted that the goal of the verbal stressors was not to mimic stressors typically found in the veterinary or shelter setting (although dog-dog aggression could be reasonably anticipated in either setting). The goal was simply to change the emotional state of the human handler, allowing us to compare the emotional state of the dogs under varying emotional states of the handler.
All cardiac (RR interval) data recorded via the Polar H10 sensors were pre-processed for artifact correction and trend removal using Kubios HRV, a device-independent software used for HRV analysis (62). The cardiac activity measures used in this study were HR (mean heart rate for handling set); one time-domain measure of HRV (RMSSD, the root mean square of successive RR interval differences, in ms); and one frequency-domain measure of HRV (HF, absolute power of the high frequency band). HF power was log transformed to increase normality of those data. RMSSD and HF were chosen for analysis as these are recommended for studies of psychophysiological research, as they may best reflect vagal tone (40). For all HRV variables, we used a within-subject approach by calculating the difference from baseline measurement (for each participant and session date). Within-subject designs have been highly recommended for psychophysiological and biobehavioral research, given the complex interactions influencing HRV and high inter-individual variation (40, 63). Difference from baseline was calculated as experimental value minus baseline value (so, if the experimental value was lower than the baseline value for that parameter, difference from baseline would be a negative value; if experimental > baseline, difference would be positive). As the primary position of both human and dog during the handling sets proved to be seated, the 5-min “sitting” baseline measurement was used for comparisons, when available. For some baseline measurements, it was not possible to divide the time equally into “sitting” vs. “standing” baselines (for example, due to a dog's unwillingness to remain seated calmly for 5 full min); in these cases, the mean baseline measurement (for the 10-min baseline) was used. Note that no significant differences were found in the baseline “sitting” and overall mean baseline HR measurements, for either humans or dogs, in either session 1 or 2 (all p > 0.355).
Behavioral data were coded from the digital video recordings using event-logging software [BORIS (56)]. Frequencies and durations of established canine stress-related behaviors [e.g., lip-licking, tucked tail, panting, “whale eye” (57–60)] were tabulated (Table 2A). Times when the dog and/or human were out of view of the camera were excluded from the analysis. Despite attempts to keep set duration consistent, the time it took for a handler to complete each handling exercise varied, as did the amount of time the dog and/or human were “in view” of the digital video recording device for a given handling set. Thus, the behavioral data was converted to rate per minute, to allow comparison between sets and across data collection sessions. For each set, duration (in seconds per minute) was calculated for all state behaviors (e.g., sitting, lying down); frequency (in number of occurrences per minute) was calculated for all event behaviors (e.g., lip lick, yawning). A canine “stress index” (SI; rate of stress behaviors observed/min) was calculated for each handling set as the sum of both state and event behavior rates for that set. Frequencies and durations of human behaviors indicative of stress [e.g., facial expressions such as frowning, furrowed brow; self-directed behaviors such as covering face or mouth; (61)] were also recorded (Table 2B).
Table 2. Ethograms used for tabulating participant behaviors from the digital video recordings canine (A) and human (B).
Initial estimates for sample size for this study were made according to recommendations of Ruxton and Colegrave (64), to meet or exceed sample sizes in published studies successfully able to answer similar research questions, in this case involving HRV analyses in dogs and other mammals [e.g., n = 7 (38); n = 14 (39); n = 20 (18); n = 24 (9, 43); n = 27 (51); n = 30 (36)]. In addition, we ran an a priori power analysis (G*power v.3.1.9.2) for a repeated measures ANOVA (input parameters: effect size = 0.20, α = 0.05, power = 0.80); calculated required sample size was n = 36, supporting our proposed sample size of 40 dogs.
For both human and canine participants, to investigate potential impacts of the introduction of the verbal stressor and/or the pre-handling activity on emotional state, repeated measures ANOVAs were used to look for differences in the cardiac parameters by handling set (1, 2, or 3), with session (1 or 2) and pre-handling activity (mindfulness vs. control) as fixed effects. Although human participants' HR (mean) values were slightly higher, and their HRV variables slightly lower, during handling set 3 following the introduction of the alternate verbal stressor, the differences between the cardiac variables when the original vs. alternate stressor were used were not statistically significant (HR: p = 0.216; RMSSD: p = 0.968; HF: p = 0.608). For this reason, no further distinction was made in the statistical analyses between data collected using the first, vs. second, verbal stressor. Post-hoc pairwise comparisons between groups using Tukey's HSD were conducted to better understand within-subject and between-subject patterns in the data. Standardized effect sizes for the repeated measures ANOVAs were estimated using partial eta-squared (), with 0.01 = small, 0.06 = moderate, and 0.14 = large effect size. Simple effect sizes are demonstrated with mean differences (±standard deviations) between groups. In addition, Pearson's correlations for paired samples were calculated to investigate relationships among and between the human and canine cardiac parameters.
As the behavioral data were non-normal (based on Shapiro-Wilks tests for normality), Friedman tests (a non-parametric alternative to repeated measures ANOVA) and Mann-Whitney U tests were used to compare SI by handling set, by session, and by pre-handling activity. For the non-parametric analyses, post-hoc pairwise comparisons were conducted for the Friedman test using the Nemenyi test (65), and for the Mann-Whitney U tests and correlation matrices using Bonferroni corrections for multiple comparisons. Standardized effect sizes for the Friedman test were estimated using the Kendall's W value (66); the Kendall's W coefficient uses the Cohen's interpretation guidelines (0.1 - <0.3 = small; 0.3- <0.5 = moderate; ≥0.5 = large). Pearson's correlations for paired samples were also run to assess relationships between the behavioral (SI) and cardiac parameters. All statistical analyses were conducted in XLSTAT 2021 (Addinsoft, Inc, New York, NY, USA) for Microsoft Excel (Microsoft Corp., Redmond, WA, USA), with α = 0.05.
Forty healthy adult dogs were recruited for this study; two of the dogs were released from the study due to excessive fearfulness when handled, leaving 38 dogs and their human caretakers participating in the handling sessions. Breed designations were provided by the dogs' owners; half of the dogs (n = 19, 50%) were mixed breed; 11 breeds were represented, including Golden Retriever, Labrador Retriever, Boxer, Beagle, and others. Dogs' ages ranged from 1 to >10 years (mode age category: 1–2 yrs), and weight ranged from 20.1 to >80 lbs (mode category: 40.1–50 lbs, or 18.2–22.7 kg). Sixteen dogs (42.1%) were female/female spayed; the remainder were male/male neutered. All human participants in this study were students or graduate students at the University of California, Davis, ranging in age between 18 and 44 years (mode category: 18–24 yrs). The overwhelming majority of human participants were female (n = 36, 94.7%). Three human participants only completed session 1 of the study protocols; data for these participants were excluded from analyses where appropriate. A number of cardiac activity data files were lost due to equipment issues: temporary losses of Bluetooth® connectivity between the sensors and the smartphones during data collection, and technical issues with uploading for storage and later analysis. Final participant counts used in the cardiac activity data analyses were 30 humans (session 1), 26 humans (session 2), 31 dogs (session 1), and 26 dogs (session 2). Mean HR for canine and human participants during baseline and the handling sets, and for data collection sessions 1 and 2, are shown in Figure 2.
Figure 2. Mean HR for canine and human participants for data collection sessions 1 and 2, during baseline and the three handling sets. As the majority of the handling exercises were conducted while the human and dog were seated, the baseline data when sitting [BL(sit)] were used for calculation of “difference from baseline” when available. When BL(sit) data were not available, baseline data for the entire baseline period (BL), including both sitting and standing, were used. No significant differences were found in the baseline “sitting” and overall mean baseline HR measurements, for either humans or dogs, in either session 1 or 2 (all p > 0.355).
For the dogs, there was a significant difference in mean HR between the handling sets (F(2,101) = 4.203, p = 0.017; = 0.08); mean HR values and differences (± SD) from baseline for each handling set are shown in Table 3A. There were no differences in mean HR by session, or by pre-handling activity, nor any interaction effects. The ANOVA table results are available in the Supplementary Material 1. Overall mean HR was slightly higher than baseline in set 1, progressively declining to near baseline by set 3 (Table 3A). In session 2, mean HR fell below baseline by handling set 3. Although not significant, mean HR was consistently higher during session 1 than session 2, for all three handling sets (Figure 3). Canine cardiac response varied by individual dog, with some dogs exhibiting reduced HR compared to baseline, others increased HR compared to baseline (Figure 4).
Table 3. Descriptive statistics for the canine cardiac activity parameters (raw data, and difference from baseline, BL), by handling set#: (A) mean HR, (B) RMSSD, (C) HF (log).
Figure 3. Canine cardiac activity variables by session number (1 vs. 2) and handling set number (1-3). Statistical significance for individual comparisons (by session, and by handling set) can be found in the text, and in the Supplementary Material.
Figure 4. Scatterplot illustration demonstrating individual variation in canine cardiac responses (HR, difference from baseline) to handling, during the three handling sets. The horizontal line on the graphs represents the mean, and can be compared to the “0” point on the y-axis (representing no difference from baseline HR).
There was a significant difference in canine HRV (as RMSSD) between the handling sets (F(2,95) = 3.219, p = 0.043; = 0.06), with RMSSD markedly higher in handling set 3; mean RMSSD values and differences (±SD) from baseline for each handling set are shown in Table 3B. RMSSD tended to be higher for session 1 than for session 2 for all three handling sets, but was not significantly different by session (p = 0.134) (Figure 3). There was no significant difference in canine RMSSD by pre-handling activity, nor were there any significant interaction effects found (Supplementary Material 1). As with HR, canine cardiac response varied by dog, with some dogs exhibiting reduced HRV (RMSSD) compared to baseline, others increased HRV (RMSSD) compared to baseline.
There were no significant differences in canine HRV (as HF) by set, session, or pre-handling activity. Mean HF(log) values and differences (±SD) from baseline for each handling set are shown in Table 3C. The interaction effect for canine HF between session*pre-handling activity*repetition approached significance (p = 0.065) (Supplementary Material 1). As with RMSSD, HF(log) tended to be slightly higher during session 1 than session 2, but not significantly (Figure 3). As with the other two measures of canine cardiac activity, response to handling varied by individual dog, with some dogs exhibiting decreased HF relative to baseline during handling, while others exhibited increased HF during handling.
For the human participants, HR (mean) tended to be higher relative to baseline during handling set 3 (post-stressor) than in sets 1 or 2 (pre-stressor) (Table 4A), although the difference did not reach statistical significance (p = 0.100). There were no significant differences in HR (mean) by session or pre-handling activity, and no significant interaction effects (Supplementary Material 2). Similarly, there were no significant differences in human HRV (as RMSSD) by set, session, or pre-handling activity; there was one significant interaction effect between session*pre-handling activity*set# (F(2,95) = 3.202, p = 0.044; = 0.06) (Supplementary Material 2; Figure 5). Unlike the dogs, human results indicated consistently elevated HR and decreased HRV relative to baseline throughout the data collection (Tables 4A–C). Means plots of human RMSSD by set, session, and pre-handling activity (Figure 5) illustrate that when handling followed the control activity, human HRV (as RMSSD) was higher during session 2 than session 1; HRV following the mindfulness activity was more similar (and between the control high and low values), regardless of whether the mindfulness activity took place before session 1 or 2. There were no significant differences in human HRV (as HF) between set, session or pre-handling activity, and no significant interaction effects between any of the factors (Supplementary Material 2).
Table 4. Descriptive statistics for the human cardiac activity parameters (raw data, and difference from baseline, BL), by handling set#: (A) mean HR, (B) RMSSD, (C) HF (log).
Figure 5. Means plots for RMSSD (human) for the three handling sets, by session (1 vs. 2) and pre-handling exercise (mindfulness vs. control), illustrating the significant interaction effect between session and pre-handling activity. Statistical significance for the interaction effect can be found in the text, and in the Supplementary Material.
Pearson's correlation analyses for paired samples revealed that the canine cardiac activity measures were significantly correlated, for both session 1 (HR and RMSSD: r = −0.265, p = 0.021; RMSSD and HF: r = 0.528, p = 0.0001), and session 2 (HR and RMSSD: r = −0.554, p = 0.0001; HR and HF: r = −0.446, p = 0.0001; RMSSD and HF: r = 0.548, p = 0.0001) (corrected α = 0.01). Human cardiac activity variables were also significantly correlated, as follows: for session 1 (HR and RMSSD: r = −0.303, p = 0.008; RMSSD and HF: r = 0.637, p = 0.0001), and for session 2 (HR and RMSSD: r = −0.376, p = 0.002). No significant correlations were found between the human and canine cardiac activity parameters during session 1; during session 2, canine RMSSD was significantly correlated with human HF (r = 0.432, p = 0.0001).
For the behavioral data, there was a significant difference between canine SI between handling sets (Q = 19.788, df = 2, p < 0.0001; Kendall's W = 0.14), with SI lower during set 3 than during sets 1 or 2 (Figure 6). As canine SI differed by handling set, comparisons between canine SI in sessions 1 and 2, and between the two pre-handling activities, were run separately for handling sets 1-3, using Mann-Whitney U tests. During handling sets 1 and 2, mean canine SI was slightly higher during session 1 than session 2, and in all three sets, slightly higher following the mindfulness activity; but the differences were not significant for any of the handling sets (all p > 0.20; corrected α = 0.008). There were very few human stress signs recorded in the behavioral data, so it was not possible to calculate a meaningful human stress index (SI) for behavioral analysis of the human participants.
Canine SI was not significantly correlated with any of the cardiac activity variables, in any of the handling sets. The correlation between SI and HF during handling sets 1 and 3 approached significance (set 1: p = 0.049; set 3: p = 0.026), but did not meet the corrected α = 0.017 for this analysis in either case.
In this study, canine cardiac activity parameters HR and RMSSD differed by handling set. Heart rate decreased as sets progressed from 1 to 3, and HRV (as RMSSD) was consistently higher in set 3 than during set 1, suggesting that dogs became less stressed as they became more familiar with the protocols and handler. HRV (as HF) also tended to increase across handling sets, although not significantly. Although the direction of change was similar between the two HRV measures, the reason for this difference in statistical significance between the HF and RMSSD results is not clear. Calculated standardized effect sizes for these comparisons were moderate. We found no evidence that the verbal stressor to the human handler impacted the dogs' stress levels in any way, nor that the handlers' participation in a mindfulness (vs. control) activity impacted the dogs during handling. Rather, our results suggest that familiarity with the handler and/or handling protocols was the factor most strongly influencing the dogs' emotional state during handling (bearing in mind that low-stress handling approaches were used throughout the handling exercises; e.g., no physical force, firm restraint, scolding or punishment were used). This is perhaps most clearly seen in the canine HR (mean) data across successive handling sets: HR was slightly elevated during the first handling set (relative to baseline) but dropped below baseline for handling set 2 and continued to decline for handling set 3. This conclusion is also supported by the behavioral data: dogs' stress levels (SI) were significantly lower during handling set 3 (post-stressor) than during sets 1 or 2 (pre-stressor), although the standardized effect size for the behavior results was small.
Heart rate tended to be higher during the first data collection session than the second, which lends some support to the importance of familiarity on stress reduction in these dogs, although this trend was not statistically significant. Both measures of HRV (RMSSD and HF) also tended to be higher during the first session, although as with HR, these differences were not statistically significant. The HRV results by session are somewhat surprising as, in contrast to the HR results by session, they would seem to suggest that the dogs may be less stressed (i.e., displaying higher HRV) during the first data collection session. However, Zupan et al. (45) studied beagle dogs' reactions to a series of different emotion-inducing stimuli (such as favorite vs. less-preferred food items, or social interactions with a familiar vs. unfamiliar human), and suggested that a combined decrease in RMSSD and HF may actually reflect an increase in the dogs' positive emotions, even when the dog is already in a positively-valenced emotional state. Although not statistically significant, a combined decrease in RMSSD and HF was seen in our study when comparing session 1 to session 2; this combined decrease was not seen for RMSSD and HF when comparing cardiac activity across handling sets within a session (see, for example, data presented in Figure 3). If Zupan et al. (45) conclusions apply here, this could explain the apparent contradiction between the HR and HRV results for the dogs in our study for the session data, and would support that familiarity with the handler and protocols was the strongest influence on the dogs' emotional state (particularly across handling sets within a given session, where the only statistically-significant differences were found).
Behavioral signs of canine stress (SI values) were also slightly lower during session 2 than 1 (although this latter difference was not statistically significant). Reduced stress when working with familiar individuals and with familiar low-stress procedures in the veterinary setting has also been reported for cats (67). For all cardiac activity parameters, there was considerable individual variation in the dogs' response to handling, with some dogs showing signs of increased stress relative to baseline (e.g., increased HR, decreased HRV), while other dogs showed decreased stress levels during handling (e.g., decreased HR, increased HRV). Individual variation in behavior is commonly seen in studies of dogs and many other species, and can play an important role in welfare (68, 69); we suspect (given the identical handling exercises, conducted in the same exam rooms, for all human/dog dyads) that each dog's prior and varying experiences with veterinary care may have been a strong driver of these differences, along with their varying comfort levels when interacting with unfamiliar humans (which in turn is shaped by temperament, previous experiences, etc.).
In addition, there were no strong patterns found in the human cardiac parameters across handling set, session, or pre-handling activity. Although HR (relative to baseline) of the handlers tended to be higher during set 3 (post addition of the verbal stressor) compared to sets 1 and 2, neither HR nor HRV differed significantly by handling set. This suggests that our attempts to increase human handler stress by introducing a verbal stressor prior to set 3 were not sufficiently impactful to be observable over any increased stress associated with participation in the novel study protocols (indicated by the increased HR, and decreased HRV, relative to baseline seen in the human cardiac results). A recent study assessing emotional contagion between dog and human (25) used an established method of causing social stress to the human participant [the Trier social stress test, TSST (70)], requiring participants to engage in a brief public speaking activity, followed by verbal mental arithmetic critiqued by an audience. In comparison to the TSST, our “stressor” was very mild; it may not have been particularly stressful for the human participants, or not stressful enough to cause any changes perceived by the dogs. We recommend that future studies of emotional contagion in a veterinary or shelter setting use an established experimental stressor to the human participants, perhaps increasing the length of time between repetitions, and using the last break between sets to apply the more salient stressor (in the absence of the dogs) to the humans.
A number of studies have reported physiological stress-reduction benefits of the presence of dogs [reviewed in (71)]. The degree to which any stress experienced by our volunteer participants in our study (all dog owners) was moderated by the presence of an unfamiliar pet dog is not known, although mean HR did not decline as the handling sets progressed for the humans as it did for the dogs. The human cardiac values did not differ significantly following the pre-handling mindfulness activity, although the interaction effect for HRV (RMSSD) between session*pre-handling activity suggests that the mindfulness activity may have had a mild stabilizing effect on handler emotional state, reflected in more consistent values of HRV regardless of whether the mindfulness activity preceded session 1 or session 2. As noted above, human participants did not overtly display many behavioral signs of stress, perhaps because of the presence of the two research assistants and the video camera in the testing room. Thus, we were unable to compare human SI across sets, sessions or pre-handling activity.
Although numerous within-species correlations were seen among the cardiac variables, we only found one significant correlation, during session 2, between the canine and human cardiac variables: canine HRV (RMSSD) was positively correlated with human HRV (HF), supporting that the more relaxed the handler, the more relaxed the dog, during the second session. However, given the lack of consistent correlations between the canine and human cardiac variables, combined with the differences between the canine and human patterns by handling set and session (e.g., human HR tended to be highest in handling set 3, post stressor; whereas canine HR was lowest during handling set 3), we could not demonstrate strong support for a relationship between human and canine stress levels in our study. The impact of social referencing between humans and dogs on dog behavior appears to be stronger when the human is familiar to the dog (24), for example when the human is the dog's owner or caretaker vs. a stranger, and perhaps influenced by the dog's learned ability to associate emotions of a familiar human with a given outcome (positive or negative) (72). Synchronization in long-term stress levels has been reported between companion dogs and their human guardians (73). Katayama et al. (25) found evidence of emotional contagion between humans and dogs based on analyses of human and canine HRV parameters (R-R intervals, SDNN and RMSSD), but reported that the strength of contagion was dependent on the length of the relationship (e.g., length of ownership) between the dog and the human. Lack of familiarity between handler and dog in our study may have played a role in the lack of consistent correlation between human and cardiac variables.
Reasons for the lack of correlations between the canine stress index (SI) and the cardiac activity parameters analyzed in this study are unclear. Canine behaviors chosen in this study to indicate stress in the dogs are widely recognized as canine stress signs. The most commonly-observed behaviors in this study were lip licking (constituting 76.7% of total frequency of event behaviors recorded), vocalization (18.0% of event behaviors), panting (82.8% of total duration for state behaviors), and tail tucked (16.6% of duration for state behaviors). Not surprisingly (given our study protocols), other signs of marked stress in the dogs such as attempting to snap or bite were rarely recorded (constituting < 1% of all event behaviors). Some canine behaviors can have multiple meanings depending on context. It is unlikely that the dogs in this study were panting due to heat exertion (given the study protocols, and the climate-controlled rooms used for data collection), and thus the most probable reason for panting was anxiety. Similarly, in this veterinary exam room context, lip licking was likely a reliable sign of stress in the dogs (74). On the other hand, vocalizations could have been a sign of distress in this context (e.g., due to separation from the familiar owner), but could also have been a sign of excitement (for example, due to receiving positive attention from a novel human). In addition, the ability to reliably code all the stress behaviors in the ethogram varied when using the digital video. For example, “whale eye” could only be coded when the dog's face was clearly visible in the video, and the affective state of “rolling on back” (submission vs. playful attention-seeking) was sometimes difficult to discern. Finally, as evident in the marked individual variation seen in the dogs' cardiac activity data, there was undoubtedly a great deal of variation in the dogs' perception of the stressfulness associated with the handling protocols, the study location (a veterinary clinic exam room), and direct physical interaction with an unfamiliar human. Veterinary visits are stressful for many dogs, and dogs' individual reactions to the veterinary setting and associated handling will vary based on a number of factors, as noted above, including (but not limited to) previous experience and environmental factors associated with a particular clinic (16, 75). These issues collectively may have made it difficult to discern clear and consistent associations between the behavioral and cardiac data.
This study was subject to a number of additional limitations. The first was the small sample size given the complexity of our study design and analyses. Although we were confident that our initial planned sample size of 40 human/dog pairs would be sufficient, two dogs were removed from the study due to excessive stress, three human participants only completed the first data collection session, and a number of cardiac activity data files (both human and canine) were lost due to the technical issues involving Bluetooth® connectivity and data upload. The resulting sample sizes were lower than our goal: 26–30 humans, and 26–31 dogs, depending on session. In order to enter all three potential influences (handling set, session, and pre-handling exercise) into our models simultaneously, participant data from session 2 were considered independent from data from session 1; handling sets were designated as repetitions in the repeated-measures ANOVAs. Although there was a 1-week period and a difference in pre-handling activity between the two data collection sessions for any given participant (and corrections for multiple comparisons were used), this means that two sessions from the same participant were entered into the models as independent samples, and thus conclusions from the significant repeated measures ANOVAs on the cardiac parameters should be interpreted with caution. In addition, both humans and dogs moved frequently during the handling sets. Although Polar monitors have performed well on ambulatory animals in some studies [e.g., (58)], many authors have noted that movement can both influence HRV directly, and negatively impact the accuracy of HRV data collection (via production of artifacts). Thus, movement may “cloud the regulation linked to cognitive, emotional, social and health processes” [(40), p. 10]. We did not control for physical activity, such as acceleration or respiration, in this study. While some authors have recommended controlling for respiration to accurately assess vagal function [e.g., (76)], other authors recommend against correcting HRV for respiration in cases of participants' breathing spontaneously (as in our study) [summarized in (40)]. The design of our study meant that activity and respiration rates were likely to be similar across the experimental tasks (sessions and handling sets), given the identical handling exercises conducted throughout (and in the same limited space and layout provided by the two exam rooms used), and as suggested by Laborde et al. (40). Our within-subject study design may also have helped control for individual differences in subjects' innate tendency to move during the handling exercises. Finally, differences in duration of baseline periods used (5 min sitting vs. 10 min complete baseline) in the calculations of “difference from baseline” mean that the time-domain HRV measure (RMSSD) results should be interpreted with caution.
In conclusion, findings from this study support that even short-term familiarity with a previously-unfamiliar handler when low-stress handling protocols are employed (no use of force and only minimal restraint via leash, calm behavior by handler, availability of food treats for interested dogs, exercises conducted on the floor vs. the examination table) is associated with reduced stress in dogs in a veterinary setting. This suggests that, when dogs are in stressful settings such as animal shelters or veterinary clinics, consistency of personnel interacting with the dogs, predictability of handling activities, and use of low stress handling techniques can reduce stress and improve welfare. Given the considerable individual variation in the dogs' physiological and behavioral reactions to handling in this study, staff handling animals regularly need to be well-versed in reading canine body language, particularly signs of stress, and adjust their approach when necessary to reduce stress and improve welfare (69). This finding supports recommendations for optimal care of shelter dogs [e.g., (77)], and aligns with findings reported for cats housed in cages (78). In addition, a short mindfulness activity, conducted immediately prior to working with the dogs, may have had a stabilizing effect on the emotional state of the handlers, although further research is needed to confirm this. We did not find strong evidence of emotional contagion between dogs and unfamiliar handlers in this study, perhaps due to the brief duration of the relationship between human and dog in this setting.
The raw data supporting the conclusions of this article will be made available by the authors, without undue reservation.
The studies involving human participants were reviewed and approved by UC Davis Institutional Research Board. The patients/participants provided their written informed consent to participate in this study. The animal study was reviewed and approved by the UC Davis Institutional Animal Care and Use Committee (IACUC). Written informed consent was obtained from the owners for the participation of their animals in this study.
EG and LH: conceived the study. EG, LH, MB, JS, JB, and AAT: developed the study design protocols for behavioral and cardiac activity data collection. DD, LH, and LK: developed the study design protocols for the mindfulness intervention and comparison. APT: conducted participant recruitments and initial screening. SL: coordinated behavioral data coding using BORIS. JS and RH: completed cardiac data processing in Kubios. EG, SL, APT, KW, and DD: collected the video and cardiac data from dogs and human handlers. EG: conducted the data analysis and drafted the initial manuscript. EG, SL, DD, KW, MB, JS, RH, LK, JB, AAT, APT, and LH: reviewed and revised the final manuscript for submission. All authors contributed to the article and approved the submitted version.
Funding for this study was provided by the Koret Shelter Medicine Program, UC Davis Center for Companion Animal Health (CCAH), School of Veterinary Medicine, Grant#: 2018-64-KSMP.
DD owns company StressReductionPrograms.com.
The remaining authors declare that the research was conducted in the absence of any commercial or financial relationships that could be construed as a potential conflict of interest.
All claims expressed in this article are solely those of the authors and do not necessarily represent those of their affiliated organizations, or those of the publisher, the editors and the reviewers. Any product that may be evaluated in this article, or claim that may be made by its manufacturer, is not guaranteed or endorsed by the publisher.
We are indebted to the CCAH for allowing us to use their facility for the duration of data collection, and to all the volunteer participants and their dogs for making the study possible. We thank S.P. Fitzgerald for assistance with the KUBIOS data processing. Our deepest thanks to all our UC Davis student research assistants for their remarkable hard work, dedication, and enthusiasm for this project: D. Cruz, O. Cunningham, J. Di Bernardo, M. Fairchild, J. Flores, A. Gatesy-Davis, M. Ho, A. Huitrado, S. Lam, A. Nukala, K. Ono, E. Parker, S. Simas, and M. Tani.
The Supplementary Material for this article can be found online at: https://www.frontiersin.org/articles/10.3389/fvets.2022.897287/full#supplementary-material
1. Figley CR, Roop RG. Compassion Fatigue in the Animal-Care Community. Washington, DC: Humane Society Press (2006).
2. Baran BE, Allen JA, Rogelberg SG, Spitzmuller C, Digiacomo NA, Webb JB, et al. Euthanasia-related strain and coping strategies in animal shelter employees. J Am Vet Med Assoc. (2009) 235:83–8. doi: 10.2460/javma.235.1.83
3. Lovell B, Lee R. Burnout and health promotion in veterinary medicine. Can Vet J. (2013) 54:790–1.
4. Spencer T, Behar-Horenstein LS, Alber JM, Bennett SL, Delmoro R, Dolce J, et al. In their own words: what qualitative analysis of online discussions with students reveals about their experiences with compassion fatigue in shelter medicine. IOSR J Agric Vet Sci. (2016) 9:38–55. doi: 10.9790/2380-0905013855
5. Scotney RL, McLaughlin D, Keates HL. A systematic review of the effects of euthanasia and occupational stress in personnel working with animals in animal shelters, veterinary clinics, and biomedical research facilities. J Am Vet Med Assoc. (2015) 247:1121–30. doi: 10.2460/javma.247.10.1121
6. Rohlf V. Interventions for occupational stress and compassion fatigue in animal care professionals – a systematic review. Traumatology. (2018) 24:186–92. doi: 10.1037/trm0000144
7. Brannick EM, DeWilde CA, Frey E, Gluckman TL, Keen JL, Larsen MR, et al. Taking stock and making strides towards wellness in the veterinary workplace. J Am Vet Med Assoc. (2015) 247:739–42. doi: 10.2460/javma.247.7.739
8. Kuhne F, Hößler JC, Struwe R. Behavioral and cardiac responses by dogs to physical human–dog contact. J Vet Behav. (2014) 9:93–7. doi: 10.1016/j.jveb.2014.02.006
9. Kuhne F, Hößler JC, Struwe R. Emotions in dogs being petted by a familiar or unfamiliar person: validating behavioural indicators of emotional states using heart rate variability. Appl Anim Behav Sci. (2014) 161:113–20. doi: 10.1016/j.applanim.2014.09.020
10. Hemsworth P, Ricea M, Karlena MG, Callega L, Barnett JL, Nash J, et al. Human–animal interactions at abattoirs: Relationships between handling and animal stress in sheep and cattle. Appl Anim Behav Sci. (2011) 135:24–33. doi: 10.1016/j.applanim.2011.09.007
11. Moberg G, Mench JA. The Biology of Animal Stress: Basic Principles and Implications for Animal Welfare (CABI). (2000). doi: 10.1079/9780851993591.0000
12. Rushen J, Taylor AA, DePassillé AM. Domestic animals' fear of humans and its effect on their welfare. Appl Anim Behav Sci. (1999) 65:285–303. doi: 10.1016/S0168-1591(99)00089-1
13. Rushen J, Munksgaard L, Marnet PG, DePassillé AM. Human contact and the effects of acute stress on cows at milking. Appl Anim Behav Sci. (2001) 73:1–14. doi: 10.1016/S0168-1591(01)00105-8
14. Grandin T. Assessment of stress during handling and transport. J Anim Sci. (1997) 75:249–57. doi: 10.2527/1997.751249x
15. Balcombe JP, Barnard ND, Sandusky C. Laboratory routines cause animal stress. Contemp Top Lab Anim Sci. (2004) 43:42–51.
16. Edwards PT, Hazel SJ, Browne M, Serpell JA, McArthur ML, Smith BP. Investigating risk factors that predict a dog's fear during veterinary consultations. PLoS ONE. (2019) 14:e0215416. doi: 10.1371/journal.pone.0215416
17. Shiverdecker MD, Schiml PA, Hennessy MB. Human interaction moderates plasma cortisol and behavioral responses of dogs to shelter housing. Physiol Behav. (2013) 109:75–9. doi: 10.1016/j.physbeh.2012.12.002
18. Bergamasco L, Osella MC, Savarino P, Larosa G, Ozella L, et al. Heart rate variability and saliva cortisol assessment in shelter dogs: human-animal interaction effects. Appl Anim Behav Sci. (2010) 125:56–68. doi: 10.1016/j.applanim.2010.03.002
19. Coppola C, Grandin T, Enns R. Human interaction and cortisol: can human contact reduce stress for shelter dogs? Physiol Behav. (2006) 87:537–41. doi: 10.1016/j.physbeh.2005.12.001
20. Hennessy MB, Williams TM, Miller DD, Douglas CW, Voith VL. Influence of male and female petters on plasma cortisol and behaviour: can human interaction reduce the stress of dogs in a public animal shelter? Appl Anim Behav Sci. (1998) 61:63–77. doi: 10.1016/S0168-1591(98)00179-8
21. Albuquerque N, Guo K, Wilkinson A, Savalli C, Otta E, Mills D, et al. Dogs recognize dog and human emotions. Biol Lett. (2016) 12:20150883. doi: 10.1098/rsbl.2015.0883
22. D'Aniello B, Scandurra A, Alterisio A, Valsecchi P, Prato-Previde E. The importance of gestural communication: a study of human–dog communication using incongruent information. Anim Cogn. (2016) 19:1231–5. doi: 10.1007/s10071-016-1010-5
23. D'Aniello B, Semin GR, Alterisio A, Aria M, Scandurra A. Interspecies transmission of emotional information via chemosignals: from humans to dogs (Canis lupus familiaris). Anim Cogn. (2018) 21:67–78. doi: 10.1007/s10071-017-1139-x
24. Merola I, Prato-Previde E, Marshall-Pescini S. Dogs' social referencing towards owners and strangers. PLoS ONE. (2012) 7:e47653. doi: 10.1371/journal.pone.0047653
25. Katayama M, Kubo T, Yamakawa T, Fujiwara K, Nomoto K, Ikeda K, et al. Emotional contagion from humans to dogs Is facilitated by duration of ownership. Front Psychol. (2019) 10:1678. doi: 10.3389/fpsyg.2019.01678
26. Ziv G. The effects of using aversive training methods in dogs – a review. J Vet Behav. (2017) 19:50–60. doi: 10.1016/j.jveb.2017.02.004
27. Hiby EF, Rooney NJ, Bradshaw JWS. Dog training methods: their use, effectiveness and interaction with behaviour and welfare. Anim Welfare. (2004) 13:63–9.
28. Herron ME, Shofer FS, Reisner IR. Survey of the use and outcome of confrontational and non-confrontational training methods in client-owned dogs showing undesired behaviors. Appl Anim Behav Sci. (2009) 117:47–54. doi: 10.1016/j.applanim.2008.12.011
29. Arhant C, Bubna-Littitz H, Bartels A, Futschik A, Troxler J. Behaviour of smaller and larger dogs: Effects of training methods, inconsistency of owner behaviour and level of engagement in activities with the dog. Appl Anim Behav Sci. (2010) 123:131–42. doi: 10.1016/j.applanim.2010.01.003
30. Casey RA, Loftus B, Bolster Richards GJ, Blackwell EJ. Human directed aggression in domestic dogs (Canis familiaris): occurrence in different contexts and risk factors. Appl Anim Behav Sci. (2014) 152:52–63. doi: 10.1016/j.applanim.2013.12.003
31. O'Farrell V. The effect of owner attitudes on behaviour. In: Serpell J, Editor. The Domestic Dog. Cambridge: Cambridge University Press (1995).
32. Lefebvre D, Diederich C, Delcourt M, Giffroy J-M. The quality of the relation between handler and military dogs influences efficiency and welfare of dogs. Appl Anim Behav Sci. (2007) 104:49–60. doi: 10.1016/j.applanim.2006.05.004
33. Dodman N, Brown D, Serpell JA. Associations between owner personality and psychological status and the prevalence of canine behavior problems. PLoS ONE. (2018) 13:e0192846. doi: 10.1371/journal.pone.0192846
34. Höglin A, Van Poucke E, Katajamaa R, Jensen P, Theodorsson E, Roth LSV. Long-term stress in dogs is related to the human–dog relationship and personality traits. Sci Rep. (2021) 11:8612. doi: 10.1038/s41598-021-88201-y
35. Von Borell E, Langbein J, Despres G, Hansen S, Leterrier C, Marchant JN, et al. Heart rate variability as a measure of autonomic regulation of cardiac activity for assessing stress and welfare in farm animals – a review. Physiol Behav. (2007) 92:293–316. doi: 10.1016/j.physbeh.2007.01.007
36. Gacsi M, Maros A, Sernkvist S, Fargo T, Miklósi A. Human analogue safe haven effect of the owner: behavioural and heart rate response to stressful social stimuli in dogs. PLoS ONE. (2013) 8:e58475. doi: 10.1371/journal.pone.0058475
37. Greiveldinger L, Veissier I, Boissy A. Behavioural and physiological responses of lambs to controllable versus uncontrollable aversive events. Psychoneuroendocrinology. (2009) 34:805–14. doi: 10.1016/j.psyneuen.2008.10.025
38. Lanata A, Nardelli M, Valenza G, Baragli P, D'Aniello B, Alterisio A, et al. A case for the interspecific transfer of emotions: a preliminary investigation on how humans' odors modify reactions of the autonomic nervous system in horses. Annu Int Conf IEEE Eng Med Biol Soc. (2018) 2018:522–5. doi: 10.1109/EMBC.2018.8512327
39. Maros K, Doka A, Miklosi A. Behavioural correlation of heart rate changes in family dogs. Appl Anim Behav Sci. (2008) 109:329–41. doi: 10.1016/j.applanim.2007.03.005
40. Laborde S, Mosley E, Thayer JF. Heart rate variability and cardiac vagal tone in psychophysiological research—recommendations for experiment planning, data analysis, and data reporting. Front Psychol. (2017) 8:213. doi: 10.3389/fpsyg.2017.00213
41. Thayer JF, Ahs F, Fredrikson M, Sollers JJ, Wager T. A meta-analysis of heart rate variability and neuroimaging studies: implications for heart rate variability as a marker of stress and health. Neurosci Biobehav Rev. (2012) 36:747–56. doi: 10.1016/j.neubiorev.2011.11.009
42. Shaffer F, Ginsberg JP. An overview of heart rate variability norms and metrics. Front Public Health. (2017) 5:258. doi: 10.3389/fpubh.2017.00258
43. Hagen K, Langbein J, Schmied C, Lexer D, Waiblinger S. Heart rate variability in dairy cows – influences of breed and milking system. Physiol Behav. (2005) 85:195–204. doi: 10.1016/j.physbeh.2005.03.019
44. Katayama M, Kubo T, Mogi K, Ikeda K, Nagasawa M. Heart rate variability predicts the emotional state in dogs. Behav Proc. (2016) 128:108–12. doi: 10.1016/j.beproc.2016.04.015
45. Zupan M, Buskas J, Altimiras J, Keeling LJ. Assessing positive emotional states in dogs using heart rate and heart rate variability. Physiol Behav. (2016) 155:102–11. doi: 10.1016/j.physbeh.2015.11.027
46. Láng E, Szilágyi N. Significance and assessment of autonomic indices in cardiovascular reactions. Acta Physiol Hungary. (1991) 78:241–60.
47. Keeling LJ, Jonare L, Lanneborn L. Investigating horse–human interactions: the effect of a nervous human. Vet J. (2009) 181:70–1. doi: 10.1016/j.tvjl.2009.03.013
48. Jha AP, Rogers SL, Morrison AB. Mindfulness training in high stress professions: Strengthening attention and resilience. In: Mindfulness-Based Treatment Approaches: Clinician's Guide to Evidence Base and Applications. (2014). p. 347–66. doi: 10.1016/B978-0-12-416031-6.00015-3
49. Lutz A, Slagter HA, Dunne JD, Davidson RJ. Attention regulation and monitoring in meditation. Trends Cogn Sci. (2008) 12:163–9. doi: 10.1016/j.tics.2008.01.005
50. Zeng X, Chiu CP, Wang R, Oei TP, Leung FY. The effect of loving-kindness meditation on positive emotions: a meta-analytic review. Front Psychol. (2015) 6:1693. doi: 10.3389/fpsyg.2015.01693
51. Jonckheer-Sheehy VSM, Vinke CM, Ortolani A. Validation of a Polar® human heart rate monitor for measuring heart rate and heart rate variability in adult dogs under stationary conditions. J Vet Behav. (2012) 7:205–12. doi: 10.1016/j.jveb.2011.10.006
52. Marchant-Forde RM, Marlin DJ, Marchant-Forde JN. Validation of a cardiac monitor for measuring heart rate variability in adult female pigs: accuracy, artefacts and editing. Physiol Behav. (2004) 80:449–58. doi: 10.1016/j.physbeh.2003.09.007
53. Parker M, Goodwin D, Eager RA, Redhead ES, Marlin DJ. Comparison of Polar® heart rate interval data with simultaneously recorded ECG signals in horses. Comp Exerc Physiol. (2010) 6:137–42. doi: 10.1017/S1755254010000024
54. Essner A, Sjöström R, Ahlgren E, Lindmark B. Validity and reliability of Polar® RS800CX heart rate monitor, measuring heart rate in dogs during standing position and at trot on a treadmill. Physiol Behav. (2013) 114–5:1–5. doi: 10.1016/j.physbeh.2013.03.002
55. ASPCAPro SAFER Behavioral Assessment. Available online at: http://aspcapro.org/sites/default/files/safer-guide-and-forms.pdf (accessed February 8, 2022).
56. Friard O, Gamba M. BORIS: a free, versatile open-source event-logging software for video/audio coding and live observations. Method Ecol Evol. (2016) 7:1325–30. doi: 10.1111/2041-210X.12584
57. Beerda B, Schilder MBH, van Hooff JA, de Vries HW. Manifestations of chronic and acute stress in dogs. Appl Anim Behav Sci. (1997) 52:307–19. doi: 10.1016/S0168-1591(96)01131-8
58. Beerda B, Schilder MBH, van Hooff JA, de Vries HW, Mol JA, et al. Behavioural, saliva cortisol and heart rate responses to different types of stimuli in dogs. Appl Anim Behav Sci. (1998) 58:365–81. doi: 10.1016/S0168-1591(97)00145-7
59. Part CE, Kiddie JL, Hayes WA, Mills DS, Neville RF, Morton DB, et al. Physiological, physical and behavioral changes in dogs (Canis familiaris) when kennelled: testing the validity of stress parameters. Physiol Behav. (2014) 133:260–71. doi: 10.1016/j.physbeh.2014.05.018
60. Protopopova A. Effects of sheltering on physiology, immune function, behavior, and the welfare of dogs. Physiol Behav. (2016) 159:95–103. doi: 10.1016/j.physbeh.2016.03.020
61. Sharma N, Gedeon T. Objective measures, sensors and computational techniques for stress recognition and classification: a survey. Comput Methods Programs Biomed. (2012) 108:1287–301. doi: 10.1016/j.cmpb.2012.07.003
62. Tarvainen MP, Niskanen JP, Lipponen JA, Ranta-Aho PO, Karjalainen PA. Kubios HRV–heart rate variability analysis software. Comput Methods Programs Biomed. (2014) 113:210–20. doi: 10.1016/j.cmpb.2013.07.024
63. Quintana DS, Heathers JA. Considerations in the assessment of heart rate variability in biobehavioral research. Front Psychol. (2014) 5:805. doi: 10.3389/fpsyg.2014.00805
64. Ruxton GD, Colegrave N. Experimental Design for the Life Sciences, 2nd ed. Oxford: Oxford University Press (2006). p. 162.
65. Nemenyi PB. Distribution-free Multiple Comparisons. (PhD thesis). Princeton, NJ: Princeton University (1963).
66. Tomczak M, Tomczak E. The need to report effect size estimates revisited: an overview of some recommended measures of effect size. Trend Sport Sci. (2014) 1:19–25.
67. Nibblett B, Ketzis JK, Grigg EK. Comparison of stress exhibited by cats examined in a clinic versus a home setting. Appl Anim Behav Sci. (2014) 173:68–75. doi: 10.1016/j.applanim.2014.10.005
68. Richter SH, Hintze S. From the individual to the population – and back again? Emphasising the role of the individual in animal welfare science. Appl Anim Behav Sci. (2019) 212:1–8. doi: 10.1016/j.applanim.2018.12.012
69. Broom DM. Welfare assessment and relevant ethical decisions: key concepts. Annu Rev Biomed Sci. (2008) 10:79–90. doi: 10.5016/1806-8774.2008.v10pT79
70. Buske-Kirschbaum A, von Auer K, Krieger S, Weis S, Rauh W, Hellhammer D. Blunted cortisol responses to psychosocial stress in asthmatic children: a general feature of atopic disease? Psychosom Med. (2003) 65:806–10. doi: 10.1097/01.PSY.0000095916.25975.4F
71. Beetz A, Uvnas-Moberg K, Julius H, Kotrschal K. Psychosocial and psychophysiological effects of human-animal interactions: the possible role of oxytocin. Front Psychol. (2012) 3:234. doi: 10.3389/fpsyg.2012.00234
72. Merola I, Prato-Previde E, Lazzaroni M, Marshall-Pescini S. Dogs' comprehension of referential emotional expressions: familiar people and familiar emotions are easier. Anim Cogn. (2014) 17:373–85. doi: 10.1007/s10071-013-0668-1
73. Sundman A-S, Van Poucke E, Svensson Holm A-C, Faresjö Å, Theodorsson E, Jensen P, et al. Long-term stress levels are synchronized in dogs and their owners. Sci Rep. (2019) 9:7391. doi: 10.1038/s41598-019-43851-x
74. Csoltova E, Martineau M, Boissy A, Gilbert C. Behavioral and physiological reactions in dogs to a veterinary examination: owner-dog interactions improve canine well-being. Physiol Behav. (2017) 177:270–81. doi: 10.1016/j.physbeh.2017.05.013
75. Edwards PT, Smith BP, McArthur ML, Hazel SJ. Fearful Fido: investigating dog experience in the veterinary context in an effort to reduce stress. Appl Anim Behav Sci. (2019) 213:14–25. doi: 10.1016/j.applanim.2019.02.009
76. Grossman P. Respiratory and cardiac rhythms as windows to central and autonomic biobehavioral regulation: selection of window frames, keeping the panes clean and viewing the neural topography. Biol Psychol. (1992) 34:131–61. doi: 10.1016/0301-0511(92)90013-K
77. Newbury S, Blinn MK, Bushby PA, Barker Cox C, Dinnage JD, Griffin B. The Association of Shelter Veterinarians Guidelines for Standards of Care in Animal Shelters. The Association of Shelter Veterinarians (ASV), Apex, NC (2010). Available online at: https://www.sheltervet.org/assets/docs/shelter-standards-oct2011-wforward.pdf (accessed February 14, 2022).
Keywords: human-animal interactions, domestic dogs, familiarity, veterinary clinic, emotional contagion, low stress handling, heart rate variability, welfare
Citation: Grigg EK, Liu S, Dempsey DG, Wong K, Bain M, Sollers JJ, Haddock R, Kogan LR, Barnhard JA, Tringali AA, Thigpen AP and Hart LA (2022) Assessing the Relationship Between Emotional States of Dogs and Their Human Handlers, Using Simultaneous Behavioral and Cardiac Measures. Front. Vet. Sci. 9:897287. doi: 10.3389/fvets.2022.897287
Received: 15 March 2022; Accepted: 14 June 2022;
Published: 11 July 2022.
Edited by:
Federica Pirrone, University of Milan, ItalyReviewed by:
Michael B. Hennessy, Wright State University, United StatesCopyright © 2022 Grigg, Liu, Dempsey, Wong, Bain, Sollers, Haddock, Kogan, Barnhard, Tringali, Thigpen and Hart. This is an open-access article distributed under the terms of the Creative Commons Attribution License (CC BY). The use, distribution or reproduction in other forums is permitted, provided the original author(s) and the copyright owner(s) are credited and that the original publication in this journal is cited, in accordance with accepted academic practice. No use, distribution or reproduction is permitted which does not comply with these terms.
*Correspondence: Emma K. Grigg, ZWtncmlnZ0B1Y2RhdmlzLmVkdQ==
Disclaimer: All claims expressed in this article are solely those of the authors and do not necessarily represent those of their affiliated organizations, or those of the publisher, the editors and the reviewers. Any product that may be evaluated in this article or claim that may be made by its manufacturer is not guaranteed or endorsed by the publisher.
Research integrity at Frontiers
Learn more about the work of our research integrity team to safeguard the quality of each article we publish.