- 1State International Joint Research Center for Animal Health Breeding, College of Animal Science and Technology, Shihezi University, Shihezi, China
- 2College of Pharmacy, Shihezi University, Shihezi, China
- 3College of Veterinary, Altai National Agricultural University, Barnaul, Russia
- 4International Sheep Research Center, School of Agriculture and Environment, Massey University, Palmerston North, New Zealand
- 5School of Medicine, Shihezi University, Shihezi, China
Despite the recognized epidemiological importance of ticks as vectors for pathogens that cause numerous zoonotic and veterinary diseases, data regarding the pathogens of pet dogs and their parasitic ticks in the Junggar Basin are scarce. In this study, a total of 178 blood samples and 436 parasitic ticks were collected from pet dogs in Junggar Basin, Xinjiang Uygur Autonomous Region (XUAR), north-western China. All ticks were identified as Rhipicephalus turanicus sensu stricto (s.s.) according to morphological and molecular characteristics. Rh. turanicus s.s. ticks were collected from pet dogs in China for the first time. Seven tick-borne pathogens, such as Ehrlichia chaffeensis, Anaplasma phagocytophilum, Rickettsia massiliae, Candidatus R. barbariae, Brucella spp., Rickettsia sibirica, and Anaplasma ovis, were detected from ticks, whereas the first five bacteria were detected from blood samples of dogs. Brucella spp. was the most predominant pathogen in both blood samples and ticks of pet dogs, with the detection rates of 16.29 and 16.74%, respectively. Moreover, 17 ticks and 1 blood sample were co-infected with two pathogens, and 1 tick was co-infected with three pathogens. This study provided molecular evidence for the occurrence of Anaplasma spp., Ehrlichia spp., Rickettsia spp., and Brucella spp. circulating in pet dogs and their parasitic ticks in Junggar Basin, north-western China. These findings extend our knowledge of the tick-borne pathogens in pet dogs and their parasitic ticks in Central Asia; therefore, further research on these pathogens and their role in human and animal diseases is required.
Introduction
Tick-borne diseases, such as zoonotic and veterinary diseases, represent a serious threat to human and/or animal health (1, 2). Globally, Canis familiaris (domestic dog) is raised as a pet and shares the household environment with humans; they serve as a host for infected ticks that can be carried into the household environment (3). These ticks may represent a threat to health, especially to children, elder individuals, and immunocompromised individuals (4). Since dogs can be considered as “sentinels” for monitoring the risk of disease affecting humans in an endemic area, the investigation of neglected zoonotic pathogens in pet dogs and the vectors that transmit pathogens is important in the prevention and control of zoonotic diseases (5–7).
Many members of the genus Brucella and certain members of the order Rickettsiales (Rickettsia, Anaplasma, and Ehrlichia) are important zoonotic and veterinary pathogens, causing brucellosis, rickettsiosis, anaplasmosis, and ehrlichiosis, which are considered as re-emerging tick-borne diseases worldwide (2, 8, 9). In the past 30 years, at least 13 emerging tick-borne pathogens that infect humans have been identified in the order Rickettsiales and found to be present in mainland China. Among these species, the most important species Rickettsia sibirica, Rickettsia conorii, Rickettsia massiliae, Candidatus R. tarasevichiae, Rickettsia raoultii, Ehrlichia chaffeensis, and Anaplasma phagocytophilum have been confirmed as the causative agents of human rickettsiosis, human monocytic ehrlichiosis, and human granulocytic anaplasmosis (2, 10–14). Other species prevalent in ticks and dogs included Anaplasma bovis, Anaplasma platys, Anaplasma ovis, Ehrlichia canis, Ehrlichia ewingii, and Rickettsia felis (15–17). Brucella spp. can be classically transmitted to humans via inhalation of aerosolized bacteria or via ingestion of, or contact with, contaminated tissues or derived products (18, 19). Considering livestock, brucellosis infection relates to direct contact with infected animals through the exchange of body fluids and via mating (8). The Brucella genus contains 12 valid species, among which Brucellas melitensis, Brucella abortus, B. canis, Brucella suis, and B. ovis have emerged in China and can infect livestock, wildlife, and humans and are transmitted by ticks and their offspring (8, 20, 21).
Xinjiang Uygur Autonomous Region (XUAR) is the largest province in China, which hosts a wide range of natural-focal diseases, among which brucellosis is the most common tick-borne disease of livestock (22); additionally, emerging tick-borne zoonoses caused by R. raoultii, E. chaffeensis, and A. phagocytophilum have been reported from sheep (23, 24). The Junggar Basin is located between the Altay Mountain and Tian Shan Mountain in XUAR and is the second-largest inland basin in China. Currently, tick-borne infections in pets in the Junggar Basin have not been studied. Therefore, the aim of the present study was to determine the prevalence of several tick-borne pathogenic bacteria, particularly Rickettsia, Ehrlichia, Anaplasma, and Brucella, in pet dogs and their ticks in Junggar Basin, XUAR, north-western China.
Materials and Methods
Sample Collection
During the period between 2017 and 2020, late April to mid-May (coinciding with the peak activities of adult ticks), blood and tick samples were collected from pet dogs based on clinical symptoms that include but not limited to depression, fever, lethargy, weakness, weight loss, and anorexia at six veterinary clinics close to pastures in Shihezi City (483 m above sea level; 44°27′N 86°06′E) and Shawan City (797 m above sea level; 44°29′N 85°56′E), Junggar Basin, XUAR, north-western China. A total of 178 blood and 436 tick samples were collected from pet dogs. All samples were collected under the permission of the pet owners, and sample collection was performed by local veterinarians. All tick samples correspond to blood samples according to each individual dog. The blood samples were collected into vacutainer tubes that contained ethylenediaminetetraacetic acid (EDTA) anticoagulant, and the ticks were placed in tubes that contained 75% ethanol and 5% glycerine to keep specimens better preserved and stored at −80°C for further possible virus studies.
DNA Extraction and Identification of Ticks
Total DNA was extracted from 200 μl of whole blood samples using the Blood DNA Extraction Kit (Omega Bio-tek, Norcross, USA) according to the manufacturer's instructions, and genomic DNA from each tick was extracted using the TIANamp Genomic DNA Kit (TIANGEN, Beijing, China). Before DNA extraction, all ticks were identified based on morphology as described previously (25). Subsequently, 45 representative ticks, with 5–8 ticks at each veterinary clinic, were subjected to molecular classification analysis based on partial mitochondrial [12S rRNA and cytochrome c oxidase subunit 1 (COI)] gene sequences to confirm tick species (23).
Detection of Tick-Borne Pathogens and Sequence Analysis
We used a partial 16S rRNA gene to detect Anaplasma spp., A. phagocytophilum, Ehrlichia spp., and E. chaffeensis, as described previously (26–29). The molecular detection of Rickettsia was performed using the citrate synthase (gltA) and outer membrane protein B (ompB) genes (30). A. ovis and E. canis were detected based on the major surface protein 4 (msp4) gene (26) and gltA gene (31), respectively. Brucella spp. were identified using the partial omp22 gene encoding 22-kD outer membrane protein (8). The DNA of Anaplasma, Ehrlichia, Rickettsia, and Brucella amplified in our laboratory was used as positive controls. Double-distilled water was used as a negative control (Dongsheng, Guangzhou, China). The amplified products were cloned into the pGEM-T Easy vector (TransGen Biotech, Beijing, China), according to the instructions, and then sequenced.
The sequence results were compared with the reference sequences available in centralized databases using a basic local alignment search tool (BLAST) (http://www.ncbi.nlm.nih.gov/BLAST/). Phylogenetic trees were constructed using the maximum-likelihood method using MEGA X software (https://www.megasoftware.net).
Statistical Analysis
Statistical analyses were performed using GraphPad Prism 7 software (GraphPad, Inc., La Jolla, CA, USA), one-tailed or t-test was used to determine the differences, and the data were expressed as mean values ± standard deviation (SD). The association of pathogen DNA between dogs and ticks was computed using the MedCalc Statistical Software. p-values <0.05 were considered statistically significant.
Results
All ticks (186 male ticks and 250 female ticks) were identified as Rhipicephalus turanicus sensu stricto (s.s.) based on their morphology. The sequencing data based on BLAST analyses for 12S rRNA and COI of ticks confirmed the morphological identification. Morphological analyses are shown in Supplementary Figure S1, and phylogenetic analyses are shown in Figure 1 and Supplementary Figure S2. The obtained sequences of Rh. turanicus s.s. have been deposited in the GenBank database (12S rRNA: MW067832 and MW995983; COI: MW065551 and MZ026893).
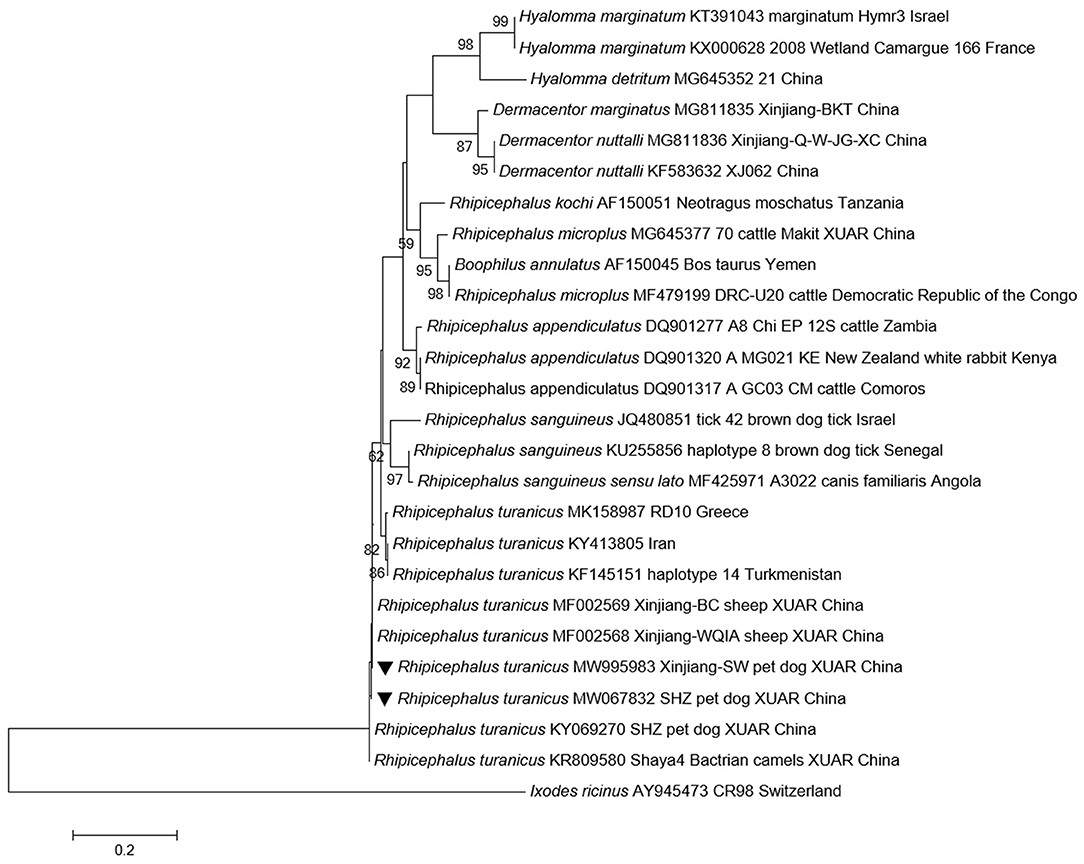
Figure 1. Phylogenetic tree based on 12S rRNA sequences of ticks collected from pet dogs. New sequences obtained in this study are indicated by black triangles.
Seven tick-borne pathogenic bacteria were detected in the ticks, out of which Brucella spp. were the most prevalent pathogens with a detection rate of 16.74%, followed by E. chaffeensis (8.26%), R. massiliae (6.42%), A. phagocytophilum (5.05%), Candidatus R. barbariae (4.13%), R. sibirica (3.21%), and A. ovis (0.92%; Table 1). Among them, 18 ticks were co-infected (17 ticks were co-infected with two pathogens and 1 tick was co-infected with three pathogens; Table 2). Meanwhile, we found that 5 tick-borne pathogens were detected in 178 blood samples; among them, Brucella spp. was most prevalent with a detection rate of 16.29%, followed by E. chaffeensis (5.62%), A. phagocytophilum (4.49%), Candidatus R. barbariae (1.69%), and R. massiliae (1.12%; Table 1). Moreover, E. chaffeensis and Brucella spp. were simultaneously detected in 1 blood sample. The overall detection rate of tick-borne pathogenic bacteria in ticks was significantly higher than that in blood samples of pet dogs (p < 0.0001). All ticks and blood samples were screened for the presence of E. canis; however, none of the samples was infected with this bacterium.
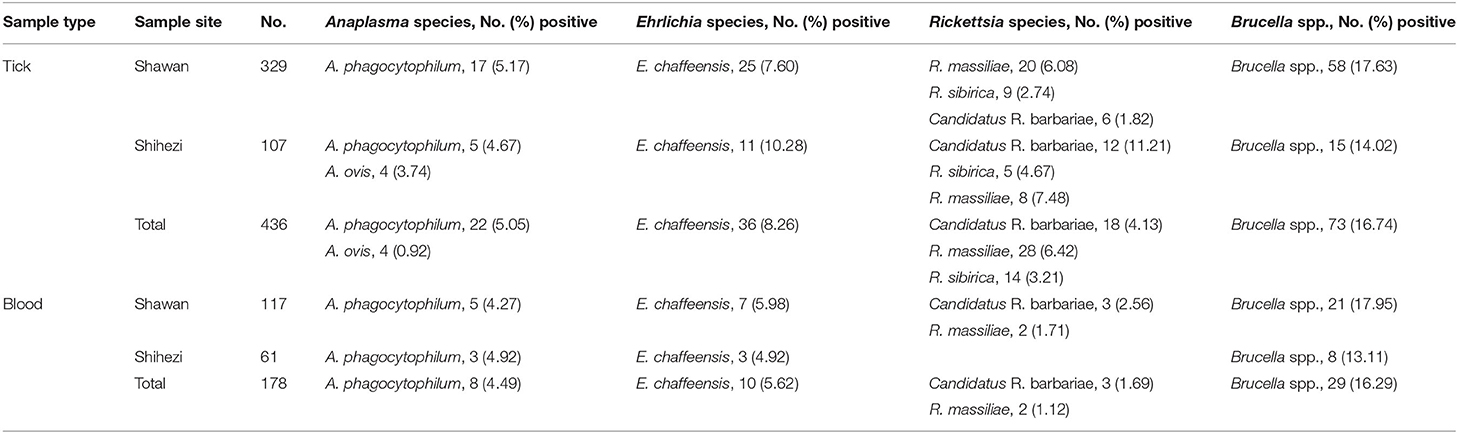
Table 1. Detection of Anaplasma, Ehrlichia, Rickettsia, and Brucella in blood and tick samples collected from pet dogs in Shihezi and Shawan, Xinjiang Uygur Autonomous Region, north-western China.
Among all the positive ticks and blood samples, Anaplasma spp. and Brucella spp. showed 99.23–100% and 99.6–100% identity to the corresponding sequence of Anaplasma sp. BL102-7 (KJ410249) from XUAR, China and Brucella sp. YC31 (MK201679) from XUAR, China, respectively. Ehrlichia spp. showed 99.64–99.65% identity to the corresponding sequence of Ehrlichia sp. QYP9 (KY630175) from Anhui Province, China and Ehrlichia sp. XJ-Eh1 (MF098393) from XUAR, China. A. phagocytophilum and E. chaffeensis showed 99.84% and 99.23–100% identity to the corresponding sequence of A. phagocytophilum (KJ782386) from XUAR, China and E. chaffeensis (MN368552) from Egypt respectively. The msp4 sequences (MW802667) showed 100% identity to the msp4 sequence of A. ovis (MN198191) from China. In addition, based on gltA gene and ompB gene, the sequences of R. massiliae, Candidatus R. barbariae, and R. sibirica achieved 99.83–100, 99.76–100, and 99.38–100% similarities with the corresponding sequences of available in GenBank, respectively. The GenBank accession numbers are shown in Supplementary Table S1.
Discussion
Tick-borne infections, especially zoonotic diseases, have been increasing in humans and pet dog cases (15). However, a few studies have analyzed pet dogs and their ticks in the Junggar Basin, north-western China. Herein, we detected Brucella DNA in pet dog-associated ticks in Junggar Basin. Moreover, we identified three Rickettsia species (R. massiliae, R. sibirica, and Candidatus R. barbariae), two Anaplasma species (A. phagocytophilum and A. ovis), and the Ehrlichia species E. chaffeensis from pet dogs and their Rh. turanicus s.s. ticks. The spread of these pathogens in the human household environment increases the range of vectors and reservoirs of tick-borne pathogens and provides a basis for assessing the risk of infection in humans.
The livestock industry is one of the main sources of its economic growth in XUAR (32). Almost every sheep farm likes to keep one dog for guarding their belongings (17). Most dogs live around the pasture and their owners, so it is a common phenomenon for these dogs to be bitten by ticks from livestock or to be parasitized by free-living ticks from pastures. Rh. turanicus is widely distributed in Central Asia, North Africa, and Europe and is the dominant tick species in XUAR (23, 33). Previous studies have reported that Rh. turanicus is parasitic in dogs and represents a risk for transmission of pathogens, such as Rickettsia, Anaplasma, and Ehrlichia in Israel, Italy, Greece, and Turkmenistan (34, 35), and serves as a bridge vector for humans (30). However, in all previous studies that infested pet dogs in XUAR, only Rh. sanguineus sensu lato has been reported, while Rh. turanicus s.s., the dominant tick species, has not been reported, it may be due to the following reasons: i) tick-borne diseases in pet dogs have not previously received much attention in XUAR and ii) it is possible that earlier misidentification that usually happens in Rh. sanguineus s.l. complex, so it might be found earlier but due to misidentification, it was reported as Rh. sanguineus; however, in fact, it was Rh. turanicus s.s. tick.
Anaplasma phagocytophilum and E. chaffeensis are considered as emerging pathogens of public health importance as they can infect humans; they are naturally maintained in tick-mammal cycles and have been detected in ticks, rodents, deer, domestic animals, and humans (36–40). A. phagocytophilum and E. chaffeensis have been previously detected in domestic animals and their Rh. turanicus s.s. ticks in XUAR (41). In this study, we detected these two pathogens in neglected pet dogs and their parasitic ticks. Phylogenetic relationships showed that A. phagocytophilum and E. chaffeensis detected from pet dogs and their ticks formed a cluster with strains detected from livestock in southern China or other counties/cities in XUAR (Figures 2A,B). Rh. turanicus s.s. is a three-host tick and is characterized by constant host changes during development (42); this suggests that there is a possibility of pathogen transmission from domestic animals to pet dogs via ticks.
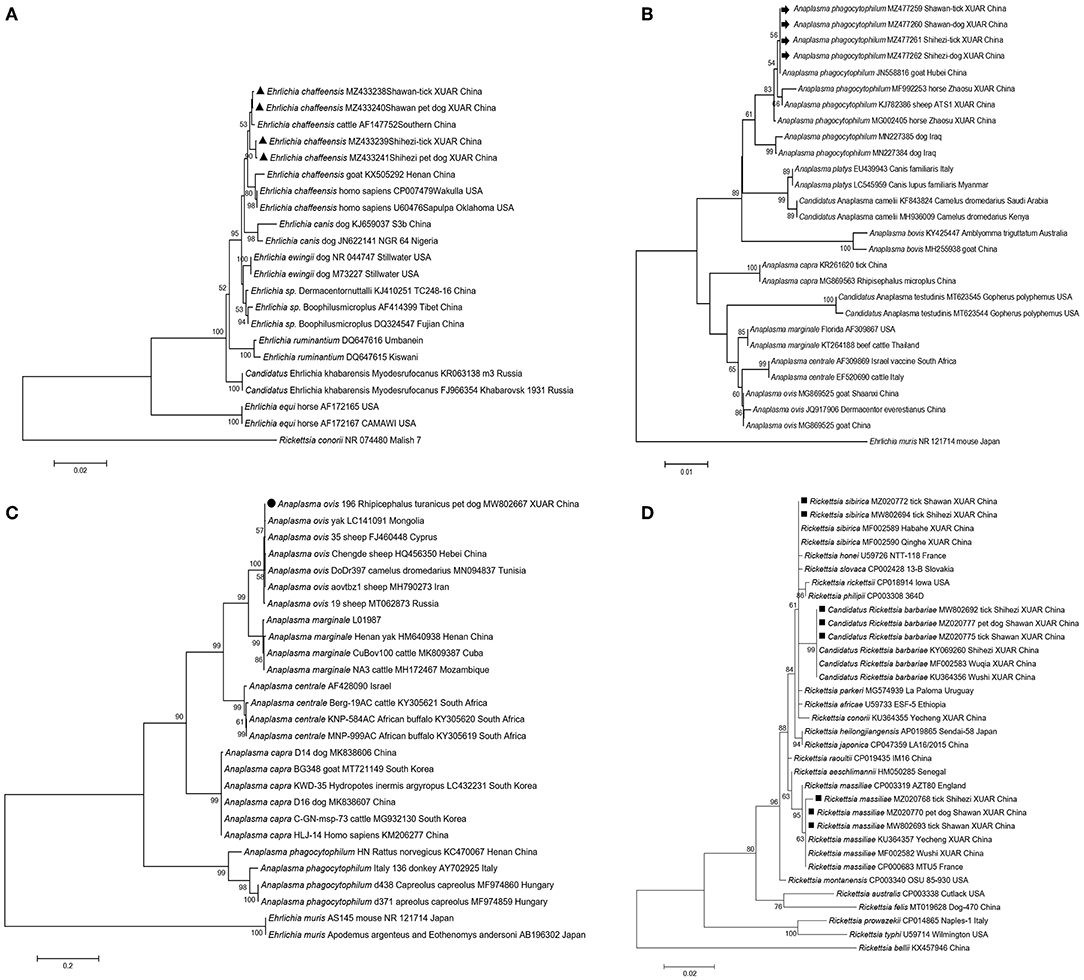
Figure 2. Phylogenetic trees of tick-borne pathogenic bacteria detected from pet dogs and their associated ticks from Shihezi and Shawan, Junggar Basin, Xinjiang Uygur Autonomous Region, north-western China. The evolutionary history was inferred via the maximum-likelihood method (bootstrap replicates: 1,000) using MEGA X. New sequences obtained in this study are indicated in black triangles for Ehrlichia chaffeensis, black arrows for Anaplasma phagocytophilum, black circle for Anaplasma ovis, black squares for three Rickettsia species (Rickettsia massiliae, Rickettsia sibirica, and Candidatus R. barbariae) based on gltA gene respectively. (A) Ehrlichia chaffeensis based on 16S rRNA gene; (B) Anaplasma phagocytophilum based on 16S rRNA gene; (C) Anaplasma ovis based on msp4 gene; (D) Rickettsia massiliae, Rickettsia sibirica, and Candidatus R. barbariae based on gltA gene.
Canine monocytic ehrlichiosis (CME), a pathogen caused by E. canis, has been found in dogs and ticks in North America, Europe, Asia, and Africa since it was first identified in Algeria in 1935 and is now spreading around the world (15, 43–45). One recent study in XUAR reported that the prevalence of E. canis in pet dogs was 12.12% (45), while another study did not detect Ehrlichia spp. in Rh. sanguineus s.l. from XUAR when detecting dogs for vector-borne agents in 10 provinces (46), which is consistent with the detected in this study. These differences in prevalence may be attributed to variations in techniques used, sources, and numbers of samples (34).
Anaplasma ovis has been identified in China and worldwide for many years since its first description (47, 48). A. ovis has been previously detected from ticks or blood samples from livestock and wild animals over ten counties in XUAR (22, 47, 49). In this study, A. ovis was only detected in Rh. turanicus s.s. obtained from pet dogs (Figure 2C), all blood samples of pet dogs were tested negative. However, based on a report that a strain isolated from a stray dog in Henan has been shown to be highly homologous to the A. ovis detected in a human in Cyprus (17), it suggests the possibility that ticks, dogs, and even humans can be infected with A. ovis. Therefore, individuals who have been in contact with animals infected with the pathogen or those caring for dogs may be at a risk.
At present, at least 19 validated spotted fever group (SFG) Rickettsia species have been detected in ticks in China (50). R. sibirica, R. massiliae, and Candidatus R. barbariae, which had previously been detected in Rh. turanicus s.s. ticks obtained from sheep in XUAR (23, 51), were found in the same tick species and/or blood samples obtained from pet dogs in this study (Figure 2D and Supplementary Figure S3). The detection rate of Rickettsia in the ticks obtained from pet dogs was lower than that in the ticks obtained from domestic in XUAR (22, 23). Additionally, the overall detection rate of Rickettsia infection in ticks (13.76%) was significantly higher than that in blood samples of pet dogs (2.81%). As SFG Rickettsia is endemic in north-western China, human rickettsiosis cases have been reported in the recent years in this area, the fact that the Rickettsia species were detected from pet dogs in this study suggests that surveillance of pathogens and ticks in pet dogs is needed to clarify the risk level and prevent human infection.
Brucellosis, also known as Malta fever in humans, can lead to abortion in livestock (52). Its pathogen Brucella spp. may be carried continuously through transstadial transmission of ticks (engorged adult female ticks, eggs, and larvae) and transmitted to healthy animals via blood sucking (8, 53). In spite of evidence showing that Brucella spp. can be transmitted by ticks (53), but no follow-up survey has confirmed such transmission. In 2018, Wang et al. (8) found that B. melitensis and B. abortus can be transmitted vertically in Dermacentor marginatus obtained from sheep. After that, Brucella DNA was successively identified in D. marginatus, Dermacentor nuttalli, Hyalomma asiaticum, Haemaphysalis punctata, Haemaphysalis longicornis, and Rh. turanicus s.s. obtained from livestock and/or free-living ticks in XUAR and Henan Province, China (22, 42, 54). In the present study, Brucella DNA was detected from blood samples and Rh. turanicus s.s. ticks infesting pet dogs. This may suggest the role of Rh. turanicus s.s. ticks in the transmission of Brucella spp. in the Junggar Basin. Moreover, since pet dogs are considered as human companions and share the household environment with humans when ticks parasitize pet dogs, it is suggested that both dogs and their owners are at risk of contracting brucellosis via tick bites. This finding indicates that in addition to the prevention of Brucella transmission through classical routes, we also need to strengthen the prevention of the transmission of Brucella by ticks from pet dogs. Moreover, the most important species to infect dogs are B. melitensis, B. abortus, B. canis, and B. suis in China (20, 55, 56), so further studies will be necessary to confirm the Brucella species by isolation and identification, to improve the understanding of the epidemiology of these tick-borne diseases, and to monitor emerging tick-borne pathogens and factors influencing their prevalence, which will facilitate implementing integrated strategies for controlling ticks and tick-borne pathogens in China.
In addition, we found two pathogens (Brucella and Ehrlichia) that might share common tick vectors and reservoir pet dogs. Interestingly, the two pathogen sequences retrieved from positive ticks were identical to those found in their dog host, which may mean that ticks are vectors for the two pathogens or the presence of the two pathogens in ticks was due to the presence of the pathogens in the blood meal (57). We also detected co-infection of two or three bacteria in pet dogs and/or their parasitic ticks in this study. These bacteria share a common tick vector, and pet dogs may become infected with these pathogens either simultaneously or sequentially (58). Although the influence of co-infection on disease severity remains unclear (59), it may result in more complicated pathogenicity and worse prognosis if humans or pet dogs are parasitized by these ticks (60). Therefore, additional efforts should be made to actively monitor the prevalence of pathogenic and potentially pathogenic tick-borne bacteria in pet dogs and their owners in XUAR and China to assess the risk of infection in pets and humans.
Data Availability Statement
The datasets presented in this study can be found in online repositories. The names of the repository/repositories and accession number(s) can be found in the article/Supplementary Material.
Ethics Statement
The animal study was reviewed and approved by the Animal Health Committee of Shihezi University. Written informed consent was obtained from the owners for the participation of their animals in this study.
Author Contributions
JG, HZ, YW, and ZW conceived and designed the study. JG, SS, and SC critically revised the manuscript. HZ and JG analyzed the data and drafted the manuscript. ZS and QZ conducted the morphological test of dog ticks. CC, XD, TZ, YC, DZ, PB, and HB conducted molecular analyses. All authors contributed to the article and approved the submitted version.
Funding
This research was supported by the Scientific and Technological Tackling Plan for Key Fields of the Corps (grant nos. 2022DB018 and 2021AB012), the National Natural Science Foundation of China (grant nos. 31860691 and 31602080), the International Science and Technology Cooperation Promotion Plan (grant nos. 2015DFR31110 and GJHZ201709), the Training Program for Excellent Young Teachers Colleges and Universities of Corps (grant no. CZ027202), and the Youth Science and Technology Innovation Leading Talent Program of Corps (grant no. 2017CB002).
Conflict of Interest
The authors declare that the research was conducted in the absence of any commercial or financial relationships that could be construed as a potential conflict of interest.
Publisher's Note
All claims expressed in this article are solely those of the authors and do not necessarily represent those of their affiliated organizations, or those of the publisher, the editors and the reviewers. Any product that may be evaluated in this article, or claim that may be made by its manufacturer, is not guaranteed or endorsed by the publisher.
Supplementary Material
The Supplementary Material for this article can be found online at: https://www.frontiersin.org/articles/10.3389/fvets.2022.895140/full#supplementary-material
Supplementary Figure 1. The morphological characteristics of male ticks collected from pet dogs.
Supplementary Figure 2. Phylogenetic tree based on cytochrome c oxidase subunit 1(COI) sequences of ticks collected from pet dogs. New sequences obtained in this study are indicated by white triangles.
Supplementary Figure 3. Phylogenetic tree of Rickettsia species based on ompB gene within pet dogs and their ticks. New sequences obtained in this study are indicated in black diamonds.
Supplementary Table 1. GenBank accession numbers of representative nucleotide sequences.
References
1. Perronne C. Lyme and associated tick-borne diseases: global challenges in the context of a public health threat. Front Cell Infect Microbiol. (2014) 4:74. doi: 10.3389/fcimb.2014.00074
2. Wei F, Song M, Liu H, Wang B, Wang S, Wang Z, et al. Molecular detection and characterization of zoonotic and veterinary pathogens in ticks from northeastern China. Front Microbiol. (2016) 7:1913. doi: 10.3389/fmicb.2016.01913
3. Shao JW, Yao XY, Song XD, Li WJ, Huang HL, Huang SJ, et al. Molecular detection and genetic diversity of Rickettsia spp. in pet dogs and their infesting ticks in Harbin, northeastern China. BMC Vet Res. (2021) 17:113. doi: 10.1186/s12917-021-02823-y
4. Dantas-Torres F, Otranto D. Dogs, cats, parasites, and humans in Brazil: opening the black box. Parasit, Vectors. (2014) 7:22. doi: 10.1186/1756-3305-7-22
5. Cardoso L, Oliveira AC, Granada S, Nachum-Biala Y, Gilad M, Lopes AP, et al. Molecular investigation of tick-borne pathogens in dogs from Luanda, Angola. Parasit Vectors. (2016) 9:1–6. doi: 10.1186/s13071-016-1536-z
6. Olivieri E, Zanzani SA, Latrofa MS, Lia RP, Dantas-Torres F, Otranto D, et al. The southernmost foci of Dermacentor reticulatus in Italy and associated Babesia canis infection in dogs. Parasit Vectors. (2016) 9:213. doi: 10.1186/s13071-016-1502-9
7. Moonga LC, Hayashida K, Nakao R, Lisulo M, Kaneko C, Nakamura I, et al. Molecular detection of Rickettsia felis in dogs, rodents and cat fleas in Zambia. Parasit Vectors. (2019) 12:168–76. doi: 10.1186/s13071-019-3435-6
8. Wang Q, Zhao S, Wureli H, Xie S, Chen C, Wei Q, et al. Brucella melitensis and B. abortus in eggs, larvae and engorged females of Dermacentor marginatus. Ticks Tick Borne Dis. (2018) 9:1045–8. doi: 10.1016/j.ttbdis.2018.03.021
9. Zintl A, Mulcahy G, Skerrett HE, Taylor SM, Gray JS. Babesia divergens, a bovine blood parasite of veterinary and zoonotic importance. Clin Microbiol Rev. (2003) 16:622. doi: 10.1128/CMR.16.4.622-636.2003
10. Jia N, Jiang JF, Huo QB, Jiang BG, Cao WC. Rickettsia sibirica subspecies sibirica BJ-90 as a cause of human disease. N Engl J Med. (2013a) 369:1176–8. doi: 10.1056/NEJMc1303625
11. Jia N, Zheng YC, Jiang JF, Ma L, Cao WC. Human infection with Candidatus Rickettsia tarasevichiae. N Engl J Med. (2013b) 369:1178–1180. doi: 10.1056/NEJMc1303004
12. Li H, Zhang PH, Huang Y, Du J, Cui N, Yang ZD, et al. Isolation and identification of Rickettsia raoultii in human cases: a surveillance study in 3 medical centers in China. Clin Infect Dis. (2018) 66:1109–15. doi: 10.1093/cid/cix917
13. Serban R, Pistol A, Negut M, Cucuiu R. Evolutia febrei butonoase în România 2000-2008 [Rickettsia conorii infection in Romania, 2000-2008]. Bacteriol Virusol Parazitol Epidemiol. (2009) 54:177–83.
14. Zaharia M, Popescu CP, Florescu SA, Ceausu E, Raoult D, Parola P, et al. Rickettsia massiliae infection and SENLAT syndrome in Romania. Ticks Tick Borne Dis. (2016) 7:759–62. doi: 10.1016/j.ttbdis.2016.03.008
15. Zhang J, Liu Q, Wang D, Li W, Beugnet F, Zhou J. Epidemiological survey of ticks and tick-borne pathogens in pet dogs in south-eastern China. Parasite. (2017) 24:35. doi: 10.1051/parasite/2017036
16. Breitschwerdt EB, Hegarty BC, Qurollo BA, Saito TB, Maggi RG, Blanton LS, et al. Intravascular persistence of Anaplasma platys, Ehrlichia chaffeensis, and Ehrlichia ewingii DNA in the blood of a dog and two family members. Parasit Vectors. (2014) 7:298. doi: 10.1186/1756-3305-7-298
17. Cui Y, Yan Y, Wang X, Cao S, Zhang Y, Jian F, et al. First molecular evidence of mixed infections of Anaplasma species in dogs in Henan, China. Ticks Tick Borne Dis. (2017) 8:283–9. doi: 10.1016/j.ttbdis.2016.12.001
18. Pappas G, Akritidis N, Bosilkovski M, Tsianos E. Brucellosis. N Engl J Med. (2005) 352:2325–36. doi: 10.1056/NEJMra050570
19. Atluri VL, Xavier MN, de Jong MF, den Hartigh AB, Tsolis RM. Interactions of the human pathogenic Brucella species with their hosts. Annu Rev Microbiol. (2011) 65:523–41. doi: 10.1146/annurev-micro-090110-102905
20. Liu ZG, Wang M, Zhao HY, Piao DR, Jiang H, Li ZJ. Investigation of the molecular characteristics of Brucella isolates from Guangxi Province, China. BMC Microbiol. (2019) 19:292. doi: 10.1186/s12866-019-1665-6
21. Liu X, Yang M, Song S, Liu G, Zhao S, Liu G, et al. Brucella melitensis in Asian Badgers, Northwestern China. Emerg Infect Dis. (2020) 26:804–6. doi: 10.3201/eid2604.190833
22. Li Y, Wen X, Li M, Moumouni P, Galon EM, Guo Q, et al. Molecular detection of tick-borne pathogens harbored by ticks collected from livestock in the Xinjiang Uygur Autonomous Region, China. Ticks Tick Borne Dis. (2020) 11:101478. doi: 10.1016/j.ttbdis.2020.101478
23. Song S, Chen C, Yang M, Zhao S, Wang B, Hornok S, et al. Diversity of Rickettsia species in border regions of northwestern China. Parasit Vectors. (2018) 11:634. doi: 10.1186/s13071-018-3233-6
24. Chahan B, Jian Z, Xuan X, Sato Y, Kabeya H, Tuchiya K, et al. Serological evidence of infection of Anaplasma and Ehrlichia in domestic animals in Xinjiang Uygur Autonomous Region area, China. Vet Parasitol. (2005) 134:273–8. doi: 10.1016/j.vetpar.2005.07.024
25. Filippova NA. Ixodid Ticks of the Subfamily Amblyomminae. Fauna of Russia and Neighbouring Countries, Vol. 4. St. Petersburg, FL: Nauka Publishing House (1997). p. 436.
26. de la Fuente J, Atkinson MW, Naranjo V, Fernández de Mera IG, Mangold AJ, Keating KA, et al. Sequence analysis of the msp4 gene of Anaplasma ovis strains. Vet Microbiol. (2007) 119:375–81. doi: 10.1016/j.vetmic.2006.09.011
27. Kawahara M, Rikihisa Y, Lin Q, Isogai E, Tahara K, Itagaki A, et al. Novel genetic variants of Anaplasma phagocytophilum, Anaplasma bovis, Anaplasma centrale, and a novel Ehrlichia sp. in wild deer and ticks on two major islands in Japan. Appl Environ Microbiol. (2006) 72:1102–9. doi: 10.1128/AEM.72.2.1102-1109.2006
28. Wen B, Jian R, Zhang Y, Chen R. Simultaneous detection of Anaplasma marginale and a new Ehrlichia species closely related to Ehrlichia chaffeensis by sequence analyses of 16S ribosomal DNA in Boophilus microplus ticks from Tibet. J Clin Microbiol. (2002) 40:3286. doi: 10.1128/JCM.40.9.3286-3290.2002
29. Cao WC, Gao YM, Zhang PH, Zhang XT, Dai QH, Dumler JS, et al. Identification of Ehrlichia chaffeensis by nested PCR in ticks from Southern China. J Clin Microbiol. (2000) 38:2778–80. doi: 10.1128/JCM.38.7.2778-2780.2000
30. Guo LP, Jiang SH, Liu D, Wang SW, Chen CF, Wang YZ. Emerging spotted fever group rickettsiae in ticks, northwestern China. Ticks Tick Borne Dis. (2016) 7:1146–50. doi: 10.1016/j.ttbdis.2016.08.006
31. Inokuma H, Brouqui P, Drancourt M, Raoult D. Citrate synthase gene sequence: a new tool for phylogenetic analysis and identification of Ehrlichia. J Clin Microbiol. (2001) 39:3031–9. doi: 10.1128/JCM.39.9.3031-3039.2001
32. Zhang H, Song S, Wang B, Jiang Y, Wu W, Guo F, et al. Brucella melitensis isolated from aborted cow and sheep fetuses in Northwest of China. Kafkas Univ Vet Fak Derg. (2018) 24:307–10. doi: 10.9775/kvfd.2017.18881
33. Chochlakis D, Ioannou1 I, Papadopoulos B, Tselentis Y, Psaroulaki A. Rhipicephalus turanicus: from low numbers to complete establishment in cyprus. its possible role as a bridge-vector. Parasit Vectors. (2014) 7:P11. doi: 10.1186/1756-3305-7-S1-P11
34. Kamani J, Baneth G, Mumcuoglu KY, Waziri NE, Eyal O, Guthmann Y, et al. Molecular detection and characterization of tick-borne pathogens in dogs and ticks from Nigeria. PLoS Negl Trop Dis. (2013) 7:e2108. doi: 10.1371/journal.pntd.0002108
35. Dantas-Torres F, Latrofa MS, Annoscia G, Giannelli A, Parisi A, Otranto D. Morphological and genetic diversity of Rhipicephalus sanguineus sensu lato from the new and old worlds. Parasit Vectors. (2013) 6:213. doi: 10.1186/1756-3305-6-213
36. Jin H, Wei F, Liu Q, Qian J. Epidemiology and control of human granulocytic anaplasmosis: a systematic review. Vector Borne Zoonotic Dis. (2012) 12:269–74. doi: 10.1089/vbz.2011.0753
37. Bown KJ, Begon M, Bennett M, Woldehiwet Z, Ogden NH. Seasonal dynamics of Anaplasma phagocytophila in a rodenttick (Ixodes trianguliceps) system, United Kingdom. Emerg Infect Dis. (2003) 9:63–70. doi: 10.3201/eid0901.020169
38. Yang J, Li Y, Liu Z, Liu J, Niu Q, Ren Q, et al. Molecular detection and characterization of Anaplasma spp. in sheep and cattle from Xinjiang, northwest China. Parasit Vectors. (2015) 8:108. doi: 10.1186/s13071-015-0727-3
39. Dong T, Qu Z, Zhang L. Detection of A. phagocytophilum and E. chaffeensis in patient and mouse blood and ticks by a duplex real-time PCR assay. PLoS ONE. (2013) 8:e74796. doi: 10.1371/journal.pone.0074796
40. Li H, Cui XM, Cui N, Yang ZD, Hu JG, Fan YD, et al. Human infection with novel spotted fever group Rickettsia genotype, China, 2015. Emerg Infect Dis. (2016) 22:2153–6. doi: 10.3201/eid2212.160962
41. Li H, Zhao S, Zhang L, Zhang K, Chen C, Wang Y. Molecular investigation on the genera of Anaplasma and Borrelia in north region of Xinjiang. J Shihezi University: Nat Sci. (2017) 35:108–12. doi: 10.13880/j.cnki.65-1174/n.2017.01.018
42. Jiang M, Yang M, Song R, Wang Y, Sheng J. Molecular epidemiology analysis of Brucella in ticks in Xinjiang Uygur Autonomous Region. Chin J Endemiol. (2019) 12:947–50.
43. Rotondano TEF, Krawczak FDS, Barbosa WO, Moraes-Filho J, Bastos FN, Labruna MB, et al. Ehrlichia canis and Rickettsia spp. in dogs from urban areas in Paraiba state, northeastern Brazil. Rev Bras Parasitol Vet. (2017) 26:211–5. doi: 10.1590/s1984-29612017030
44. Maekawa N, Konnai S, Balbin MM, Mingala CN, Gicana KRB, Bernando FAEM, et al. Molecular detection and phylogenetic analysis of Ehrlichia canis in a Philippine dog. Ticks Tick Borne Dis. (2018) 9:266–9. doi: 10.1016/j.ttbdis.2017.09.012
45. Qiao MF, Wang LX, Lei Y, Ren Y, Cai KJ, Zhang JS, et al. Molecular detection and genetic variability of Ehrlichia canis in pet dogs in Xinjiang, China. Vet World. (2020) 13:916–22. doi: 10.14202/vetworld.2020.916-922
46. Xu D, Zhang J, Shi Z, Song C, Zheng X, Zhang Y, et al. Molecular detection of vector-borne agents in dogs from ten provinces of China. Parasit Vectors. (2015) 8:501. doi: 10.1186/s13071-015-1120-y
47. Song R, Wang Q, Guo F, Liu X, Song S, Chen C, et al. Detection of Babesia spp., Theileria spp. and Anaplasma ovis in border regions, northwestern China. Transbound Emerg Dis. (2018) 65:1537–44. doi: 10.1111/tbed.12894
48. Ding ZB, Lu FT, Han LJ, Huang JS. The first report of goats Anaplasma in China. Chin. J Vet Sci Technol. (1985) 6:61.
49. Zhao L, Lv J, Li F, Li K, He B, Zhang L, et al. Identification and molecular analysis of Ixodid ticks (Acari: Ixodidae) infesting domestic animals and tick-borne pathogens at the Tarim Basin of Southern Xinjiang, China. Korean J Parasitol. (2020) 58:37–46. doi: 10.3347/kjp.2020.58.1.37
50. Liu G, Zhao S, Tan W, Hornok S, Yuan W, Mi L, et al. Rickettsiae in red fox (Vulpes vulpes), marbled polecat (Vormela peregusna) and their ticks in northwestern China. Parasit Vectors. (2021) 14:204. doi: 10.1186/s13071-021-04718-1
51. Wei QQ, Guo LP, Wang AD, Mu LM, Zhang K, Chen CF, et al. The first detection of Rickettsia aeschlimannii and Rickettsia massiliae in Rhipicephalus turanicus ticks, in northwest China. Parasit Vectors. (2015) 8:631. doi: 10.1186/s13071-015-1242-2
52. Del Vecchio VG, Kapatral V, Redkar RJ, Patra G, Mujer C, Los T, et al. The genome sequence of the facultative intracellular pathogen Brucella melitensis. P Natl Acad Sci. (2002) 99:443–8. doi: 10.1073/pnas.221575398
53. Tovar RM. Infection and transmission of Brucella by ectoparasites. Am J Vet Res. (1947) 8:138–40.
54. Zhang K, Li A, Wang Y, Zhang J, Chen Y, Wang H, et al. Investigation of the presence of Ochrobactrum spp. and Brucella spp. in Haemaphysalis longicornis. Ticks Tick Borne Dis. (2021) 12:101588. doi: 10.1016/j.ttbdis.2020.101588
55. Li GH, Qing Y, Tai XP, Niu XL, Ma JC, Muhetaer. Brucella bovis was isolated from a dog. Bull Endem Dis. (1987) 3:69. doi: 10.13215/j.cnki.jbyfkztb.1987.03.01
56. Shi P, Hao J, He Q, Zhu Z, GUO Z, Zhang Z, et al. Identification of Brucella melitensis mutants isolated from dogs for the first time. Chin J Local Epidemiol. (1992) 4:15–6.
57. Dahmani M, Davoust B, Tahir D, Raoult D, Fenollar F, Mediannikov O. Molecular investigation and phylogeny of Anaplasmataceae species infecting domestic animals and ticks in Corsica, France. Parasit Vectors. (2017) 10:302. doi: 10.1186/s13071-017-2233-2
58. Pesapane R, Foley J, Thomas R, Castro LR. Molecular detection and characterization of Anaplasma platys and Ehrlichia canis in dogs from northern Colombia. Vet Microbiol. (2019) 233:184–9. doi: 10.1016/j.vetmic.2019.05.002
59. Starkey LA, Barrett AW, Chandrashekar R, Stillman BA, Tyrrell P, Thatcher B, et al. Development of antibodies to and PCR detection of Ehrlichia spp. in dogs following natural tick exposure. Vet Microbiol. (2014) 173:379–84. doi: 10.1016/j.vetmic.2014.08.006
Keywords: pet dogs, tick-borne bacteria, Rhipicephalus turanicus sensu stricto, north-western China, Brucella
Citation: Guo J, Song S, Cao S, Sun Z, Zhou Q, Deng X, Zhao T, Chai Y, Zhu D, Chen C, Baryshnikov PI, Blair HT, Wang Z, Wang Y and Zhang H (2022) Molecular Detection of Zoonotic and Veterinary Pathogenic Bacteria in Pet Dogs and Their Parasitizing Ticks in Junggar Basin, North-Western China. Front. Vet. Sci. 9:895140. doi: 10.3389/fvets.2022.895140
Received: 23 March 2022; Accepted: 14 June 2022;
Published: 08 July 2022.
Edited by:
Nicola Pugliese, University of Bari Aldo Moro, ItalyReviewed by:
Valentina Virginia Ebani, University of Pisa, ItalyAbdul Jabbar, The University of Melbourne, Australia
Haytham Senbill, Alexandria University, Egypt
Copyright © 2022 Guo, Song, Cao, Sun, Zhou, Deng, Zhao, Chai, Zhu, Chen, Baryshnikov, Blair, Wang, Wang and Zhang. This is an open-access article distributed under the terms of the Creative Commons Attribution License (CC BY). The use, distribution or reproduction in other forums is permitted, provided the original author(s) and the copyright owner(s) are credited and that the original publication in this journal is cited, in accordance with accepted academic practice. No use, distribution or reproduction is permitted which does not comply with these terms.
*Correspondence: Zhen Wang, 530469380@qq.com; Yuanzhi Wang, wangyuanzhi621@126.com; Hui Zhang, prof.zhang@foxmail.com
†These authors have contributed equally to this work and share first authorship