- 1Department of Medicine and Epidemiology, School of Veterinary Medicine, University of California, Davis, Davis, CA, United States
- 2Department of Otolaryngology, Center for Voice and Swallowing, School of Medicine, University of California, Davis, Davis, CA, United States
- 3The Vatche and Tamar Manoukian Division of Digestive Diseases, Department of Medicine, UCLA Robert G. Kardashian Center for Esophageal Health, David Geffen School of Medicine, University of California, Los Angeles, Los Angeles, CA, United States
- 4Division of Gastroenterology and Hepatology, Department of Medicine, Northwestern Medicine, Feinberg School of Medicine, Northwestern University, Chicago, IL, United States
Swallowing impairment is a highly prevalent and clinically significant problem affecting people and dogs. There are myriad causes of swallowing impairment of which gastroesophageal reflux is the most common in both species. Similarities in anatomy and physiology between humans and canines results in analogous swallowing disorders including cricopharyngeus muscle achalasia, esophageal achalasia, hiatal herniation, and gastroesophageal reflux with secondary esophagitis and esophageal dysmotility. Accordingly, the diagnostic approach to human and canine patients with swallowing impairment is similar. Diagnostic procedures such as swallowing fluoroscopy, high-resolution manometry, pH/impedance monitoring, and endolumenal functional luminal imaging probe can be performed in both species; however, nasofacial conformation, increased esophageal length, and the difficulty of completing several of these procedures in awake dogs are inherent challenges that need to be considered. Human patients can convey their symptoms and respond to verbal cues, whereas veterinarians must rely on clinical histories narrated by pet owners followed by comprehensive physical examination and observation of the animal eating different food consistencies and drinking water. Dogs may also be unwilling to drink or eat in the hospital setting and may be resistant to physical restraint during diagnostic procedures. Despite the species differences and diagnostic challenges, dogs are a natural animal model for many oropharyngeal and esophageal disorders affecting people, which presents a tremendous opportunity for shared learnings. This manuscript reviews the comparative aspects of esophageal anatomy and physiology between humans and canines, summarizes the diagnostic assessment of swallowing impairment in both species, and discusses future considerations for collaborative medicine and translational research.
Introduction
Difficulty swallowing is a prevalent problem in both people and dogs (1–3) that can cause malnutrition (4), dehydration (5), aspiration pneumonia (6, 7), and negatively impact overall quality of life (8). The exact prevalence of swallowing impairment in dogs is unknown, but at the University of California, Davis, nearly 1% of 105,000 dogs presenting to the Small Animal Clinic between 2003 and 2013 were evaluated for a swallowing abnormality. In humans, 1 in 6 adults in the US report symptoms of dysphagia (9) and contribute to nearly 600,000 outpatient visits yearly (1). Dysphagia is even more common in the elderly population with a prevalence of 15% (10). The prevalence of difficulty swallowing is more easily documented in human patients because they can report their symptoms of dysphagia. The term “dysphagia” denotes symptoms that canine patients cannot convey. Thus, although dysphagia is still conventionally used in veterinary medicine to describe swallowing impairment in dogs and cats, the authors have elected to use the term “swallowing impairment” over “dysphagia” in this manuscript to more accurately portray this important phenomenon.
Swallowing impairment can be categorized anatomically into oropharyngeal or esophageal disorders and further classified into structural, motility, or functional disorders (11). Functional disorders are unique to human patients because they can report their symptoms, including pain while swallowing (odynophagia) or a sensation of food sticking in the throat or chest. Extensive diagnostic testing excludes structural and motility disorders to diagnose a functional disorder (12).
Functional and motility disorders can involve not just the oropharynx and esophagus, but the entire gastrointestinal tract (13). For example, systemic scleroderma (14–16) and dysautonomia (17, 18) can cause esophageal and gastrointestinal dysmotility (14–18). Thus, a holistic assessment of the gastrointestinal tract is valuable when evaluating patients with swallowing impairment.
Evaluating gastrointestinal motility is specifically important in patients with gastroesophageal reflux disease (GERD). Delayed gastric emptying from an outflow obstruction (19), gastroparesis (20), or ileus (21) can exacerbate gastroesophageal reflux (GER) and esophageal dysmotility (20, 22, 23). Methods such as gastric emptying scintigraphy (24, 25), ultrasound (26, 27), or wireless motility capsules (28, 29) can be employed to assess gastrointestinal motility. However, an extensive review of gastrointestinal motility and its assessment is beyond the scope of this manuscript.
Prior to pursuing advanced diagnostic tests in patients with swallowing impairment, the clinician should obtain a thorough clinical history and patient examination to help distinguish the anatomic location and cause of the swallowing impairment. For example, dropping of food from the mouth in dogs is characteristic of oral dysfunction that may be secondary to poor dentition, glossal disease, or oral tumors. Pharyngeal and cricopharyngeus muscle impairment often cause gagging and retching within seconds of food or water consumption whereas regurgitation is more delayed with esophageal or gastroesophageal disorders (30). Humans may localize concerns to a specific area of discomfort. Examples include globus (31), which is a non-painful sensation of tightness in the pharynx; heartburn, a burning sensation in the retrosternal region; or dyspepsia, characterized by epigastric pain (32, 33). A history of a stroke or signs such as muscle atrophy, generalized weakness, tremors, or cognitive decline suggest neuromuscular pathology. Nevertheless, in light of many overlapping clinical signs and the breadth of possible differential diagnoses in both dogs and people, further diagnostic tests are usually needed to accurately localize and confirm the underlying etiology of the swallowing impairment.
There is a plethora of causes of swallowing impairment, but due to similarities in pharyngeal and esophageal anatomy and function between humans and canines (34–37), many of the same diseases occur. Some of the most common causes of swallowing dysfunction in both canines and humans include cricopharyngeus muscle achalasia (38, 39), esophageal achalasia (40, 41), sliding (Type I) hiatal herniation (42–44), GER (32, 43, 45) with secondary esophagitis (46–48), esophageal strictures (49), and esophageal dysmotility (50, 51). Thus, many diagnostic procedures utilized to assess human patients can be used in dogs. Examples include swallowing fluoroscopy (2, 52), high-resolution manometry (HRM) (53), pH/impedance monitoring (45, 54), and endolumenal functional luminal imaging probe (EndoFLIP) (40, 55–58). However, there are differences between the two species in patient conformation, neuromuscular anatomy, and compliance that impact performing and interpreting these tests in canine patients. Furthermore, there are disparities between the human and veterinary fields in research progress, funding, and equipment availability that curb the widespread use of these diagnostic modalities in veterinary medicine. For example, HRM and endoFLIP hardware and software currently cost $70,000 and $81,000, respectively, which can be cost-prohibitive for veterinary clinics to purchase. In addition, the HRM esophageal catheters are extremely fragile, have a limited number of usages, and cost $16,000 to replace.
This review article will highlight the comparative aspects of esophageal anatomy and physiology between humans and canines, summarize the procedures and applications of swallowing fluoroscopy, HRM, pH/impedance, and EndoFLIP to assess swallowing impairment in both species, explain the limitations and roadblocks to using these tests in canines, and discuss future directions and considerations for collaborative medicine and translational research.
Comparative Esophageal Anatomy and Physiology Between Humans and Dogs
Functions of the Esophagus
The esophagus is an essential neuromuscular tubular structure that functions to transport food or liquid from the pharynx to stomach. The upper (UES) and lower esophageal sphincters (LES) relax to allow ingesta into the esophagus and stomach, respectively. The UES and LES are otherwise tonically contracted to block laryngopharyngeal and gastroesophageal reflux, respectively, and prevent aspiration (34–37, 59). The UES also prevents entry of excess air into the digestive tract (60). The conservation of these esophageal and sphincter functions across species explains the similarities in anatomy and physiology between humans and canines.
Anatomical and Physiological Similarities
The basic anatomy of the human and canine esophagus is organized into two zones of high pressure at the UES and LES with an esophageal body between that is divided into cervical, thoracic, and abdominal components (Figure 1A). The wall of the esophagus consists of 4 separate tissue layers: mucosa, submucosa, muscularis, and the outermost adventitia (Figures 1B,C) (16, 17, 19). The mucosa is composed of stratified squamous epithelial cells linked together by tight junctions, desmosomes, claudins, occludins, and other fortifying proteins to create a protective barrier (61). Atop the epithelial surface rests a pre-epithelial layer of bicarbonate, mucin, and water that neutralizes swallowed or refluxed acidic contents. The components of the pre-epithelial layer are secreted by submucosal mucous glands. Other elements of the submucosa are blood vessels, nerves, and collagenous connective tissue (35, 37, 59).
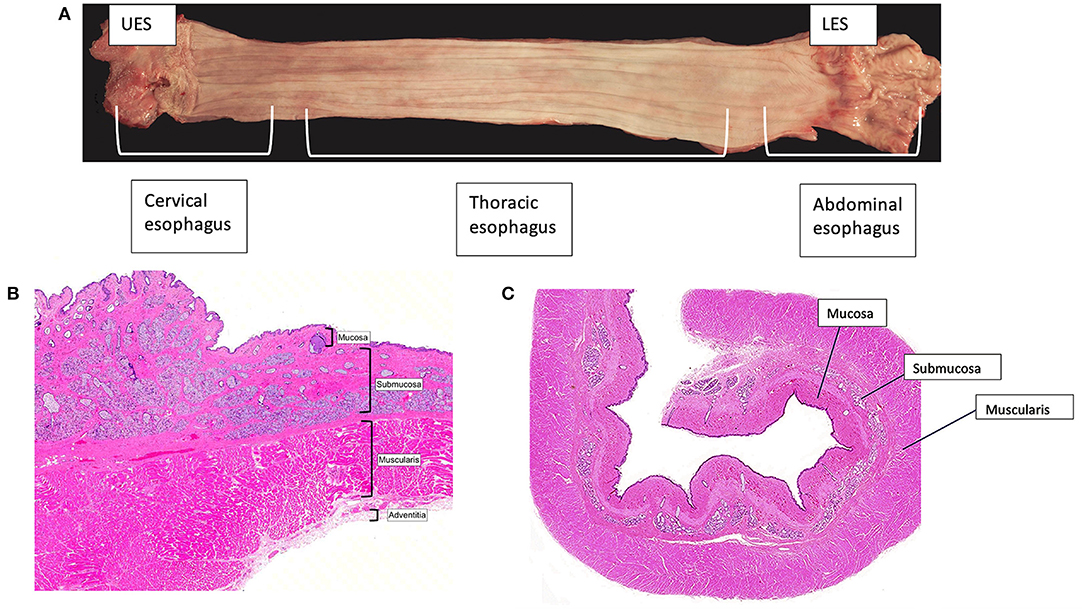
Figure 1. Esophageal anatomy. (A) The canine esophagus is shown with the proximal esophagus on the left and the distal on the right. The canine and human esophagus are composed of the upper esophageal sphincter, the esophageal body, which is segmented into the cervical, thoracic, and abdominal esophagus, and the lower esophageal sphincter. (B,C) A transverse image from the cervical portion of the canine esophagus (B) and the thoracic portion of the canine esophagus (C). Images were obtained with light microscopy and stained with hematoxylin and eosin. The inner folded mucosal layer is surrounded by submucosa, muscularis, and the outermost external adventitia. Note the absence of a serosal layer in the esophagus. This makes the esophagus reliant upon the holding strength of the submucosa.
The integrity and coordination of these anatomical components enables normal deglutition in humans and canines. Deglutition (Figures 2A–C) (2) begins with the oral preparatory phase, which is voluntary and is associated with mastication and lubrication of the food bolus in preparation for swallowing. The oral phase consists of the muscular events responsible for movement of the bolus from the tongue to the pharynx, and is facilitated by the tongue, jaw, and hyoid muscle movements. The pharyngeal phase begins as the bolus reaches the tonsils, and is characterized by elevation of the soft palate to prevent the bolus from entering the nasopharynx, elevation and forward movement of the larynx and hyoid, retroflexion of the epiglottis and closure of the vocal folds to close the entrance into the larynx, synchronized contraction of the middle and inferior constrictor muscles of the pharynx, and relaxation of the cricopharyngeus muscle, which makes up much of the UES, to allow passage of the bolus into the esophagus. Respiration is briefly halted (apneic moment) during the pharyngeal phase. The esophageal phase follows during which peristaltic contractions drive contents down the esophageal body, across the esophagogastric junction (EGJ), and into the stomach (59, 62–64). Primary peristalsis is triggered in the swallowing center by activation of vagal lower motor neurons, which interact with neuromuscular elements of the esophageal wall. Peristaltic contractions then spearhead bolus transit. Secondary peristalsis, stimulated by mechanical distension of the esophagus and enhanced by chemosensory triggers (65), assists to clear ingested material not cleared by primary peristalsis (66). Multiple rapid swallows induce a period of latency called deglutitive inhibition that terminates with an accentuated peristaltic contraction. This physiologic pattern has been observed in both humans and dogs (53, 67). Transient lower esophageal sphincter relaxation (tLESR) is a vagally-mediated reflex that also occurs in both species. Gastric distension stimulates stretch receptors, which activate vagal sensory neurons that synapse on interneurons in the nucleus tractus solitarius of the brainstem. These interneurons then excite vagal motor neurons in the dorsal motor nucleus of the vagus, which travel in the vagus to stimulate myenteric neurons that innervate LES muscle. The myenteric neurons make nitric oxide, which causes LES relaxation. This is a major mechanism of GER and is the genesis of the gastric belch (68, 69).
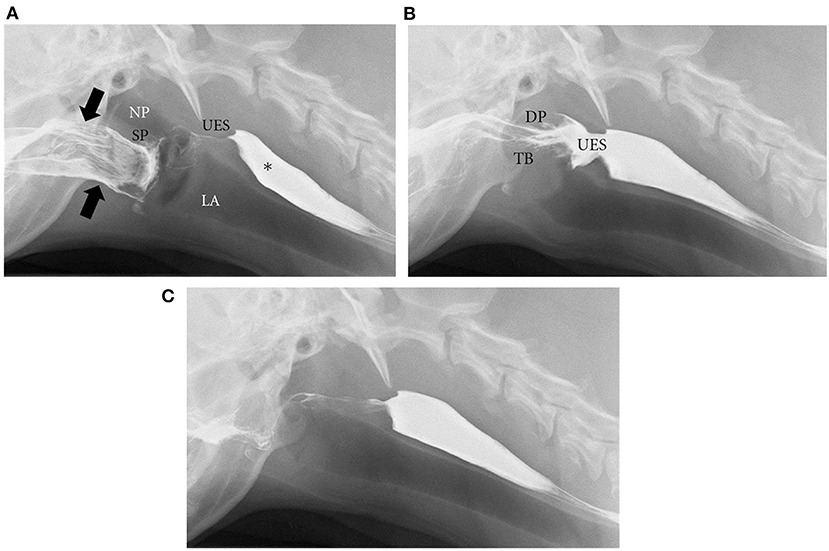
Figure 2. Phases of deglutition. Digital images from a videofluoroscopic swallow study in a healthy dog show the phases of deglutition. (A) Oral phase: Liquid barium contrast in the oral cavity (designated by black arrows). There is also remaining barium from a previous swallow in the cervical esophagus (*). At the start of the pharyngeal phase, the soft palate will rise to close the nasopharynx while the epiglottis closes the larynx to prevent nasopharyngeal reflux and laryngeal penetration, respectively. NP, nasopharynx; LA, larynx; SP, soft palate; UES, upper esophageal sphincter. (B) As a continuation of the pharyngeal phase, the pharyngeal muscles contract and the dorsal pharyngeal wall (DP) meets the tongue base (TB) while the cricopharyngeus muscle relaxes to open the upper esophageal sphincter (UES). Liquid barium contrast can then pass through the open UES into the proximal esophagus. (C) After the contrast reaches the esophagus, esophageal peristalsis (primary and secondary) can occur to move the bolus through the lower esophageal sphincter (LES) to the stomach. (A–C) were reprinted from International Scholarly Research Network Veterinary Science, Volume 2012, Pollard RE, Imaging evaluation of dogs and cats with dysphagia, Copyright 2012 Rachel E. Pollard. Reprinted with permission from Dr. Rachel E Pollard.
Similarities in Pathology
Given the resemblance in esophageal anatomy and physiology between humans and canines, it is logical that the two species share common pathologies. The most common anatomic locations involved in swallowing impairment are oropharyngeal and esophageal pathologies.
Oropharyngeal Swallowing Impairment
Oropharyngeal swallowing impairment can be the result of oral, palatal, pharyngeal or pharyngoesophageal pathology. In humans, oropharyngeal swallowing impairment is particularly common amongst geriatric patients (70), secondary to aging or associated neurologic conditions. Elderly human patients have reduced lingual propulsion and delayed swallow response (70). They also have a smaller UES diameter, which could be due to reduced UES compliance or webbing, weak pharyngeal drive, and decreased hyolaryngeal traction (71, 72). In addition to aging, several neurologic conditions such as stroke (73), Alzheimer's disease (74), Parkinson's disease (75), neuromuscular diseases, and dementia (76) can cause pharyngeal weakness, discoordination, and/or UES dysfunction in humans. Similarly, pharyngeal weakness in dogs mainly occurs secondary to neuromuscular disorders [myasthenia gravis (77), muscular dystrophy (78), polymyositis (79), and polyneuropathies (80)]. Pharyngeal weakness typically occurs in middle-aged to older dogs, and can cause delayed propulsion of the bolus to the UES, with subsequent asynchrony between pharyngeal contraction and relaxation of the UES (81).
Esophageal Swallowing Impairment
Esophageal swallowing impairment in canines is mainly caused by gastroesophageal disease with consequent esophagitis, structural lesions, or motility disorders. In both dogs and people, gastroesophageal disease due to GER ± hiatal herniation is the most common etiology (42–45). Brachycephalic (short-muzzled) breeds (French bulldogs, English bulldogs, pugs, and Boston terriers, boxers, shih-tzus) are frequently affected (Figures 3A–C) (82). Due to their nasofacial conformation and unique respiratory anatomy, they often have an upper airway tract obstruction, brachycephalic obstructive airway syndrome (BOAS), which increases negative intrathoracic pressure and causes subsequent hiatal herniation and GER (42, 50, 83). GER in dogs also frequently occurs during anesthesia secondary to reduction of LES tone (84, 85), and can lead to reflux esophagitis, esophageal dysmotility, and esophageal strictures (86) identical to peptic strictures in humans (Figures 4A,B,D) (32, 82). Other structural lesions such as tumors or vascular ring anomalies (87) occur in both species although dogs are at greater risk for esophageal foreign bodies (88) (Figure 4C) (82). Motility disorders in canine patients are frequently found in association with megaesophagus, which can be a congenital or acquired disease in dogs (Figure 4E) (82). Approximately 50% of dogs with megaesophagus have an acquired and idiopathic form, but there are many secondary causes of megaesophagus associated with polyneuropathies, polymyopathies, junctionopathies (myasthenia gravis, botulism, tick paralysis, tetanus, organophosphate poisoning), myopathies (inflammatory myopathies, dermatomyositis), and polyneuropathies (polyradiculoneuritis, dysautonomia) (89) in dogs. In humans, a sigmoid megaesophagus can develop as an end result of late-stage achalasia (90) (Figure 4F). Similarly, megaesophagus secondary to LES achalasia has been described in dogs and at one academic institution's teaching hospital, comprised 60% (14/23) of the megaesophagus cases seen over a 2-year span (40, 41). Esophageal motility disorders in the absence of megaesophagus such as juvenile esophageal dysmotility (51) or dysmotility secondary to GER have been identified in dogs (3, 42) but esophageal motility disorders in dogs are poorly characterized compared to those in humans in light of the limited application of HRM in animals (91).
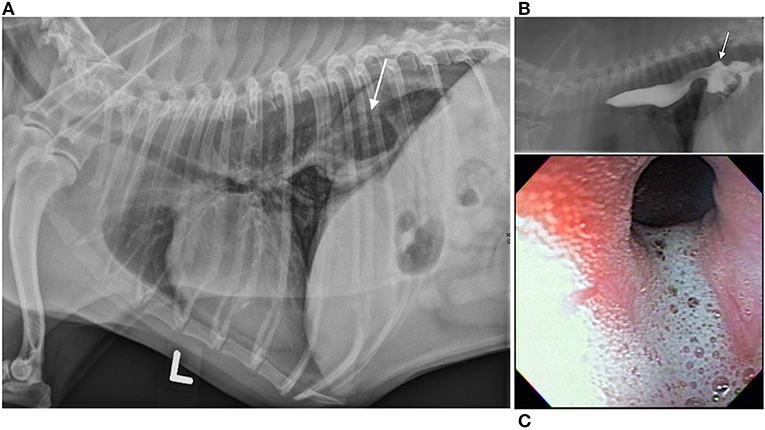
Figure 3. Hiatal herniation, gastroesophageal reflux, and esophagitis in a brachycephalic dog. Hiatal herniation in a brachycephalic dog. (A) Left lateral thoracic radiograph of a 5-year old brachycephalic Boston Terrier with a chronic history of regurgitation. The stomach is seen extending through the diaphragm into the craniodorsal thorax in this image (arrow). Although not pictured, the stomach returns to a normal position on subsequent views, which is suggestive of a sliding or type I hiatal hernia. (B) Contrast videofluoroscopic swallow study of the same patient. This image documents gastroesophageal reflux of barium contrast as a result of hiatal herniation (arrow points to hiatal herniation and stream of white contrast extending cranial is evidence of gastroesophageal reflux). (C) Endoscopic image from the same patient showing foamy gastroesophageal reflux cranial to the lower esophageal sphincter and secondary esophagitis [reddened hyperemic area in the upper left of the image (denoted with arrow)]. (A–C) Images were published in Textbook of veterinary internal medicine: diseases of the dog and the cat, Vol 2, 8th edition, Marks SL, Chapter 273: Diseases of the Pharynx and Esophagus, 8501–8576, Copyright 2017 by Elsevier, Inc, Reprinted with permission from Elsevier.
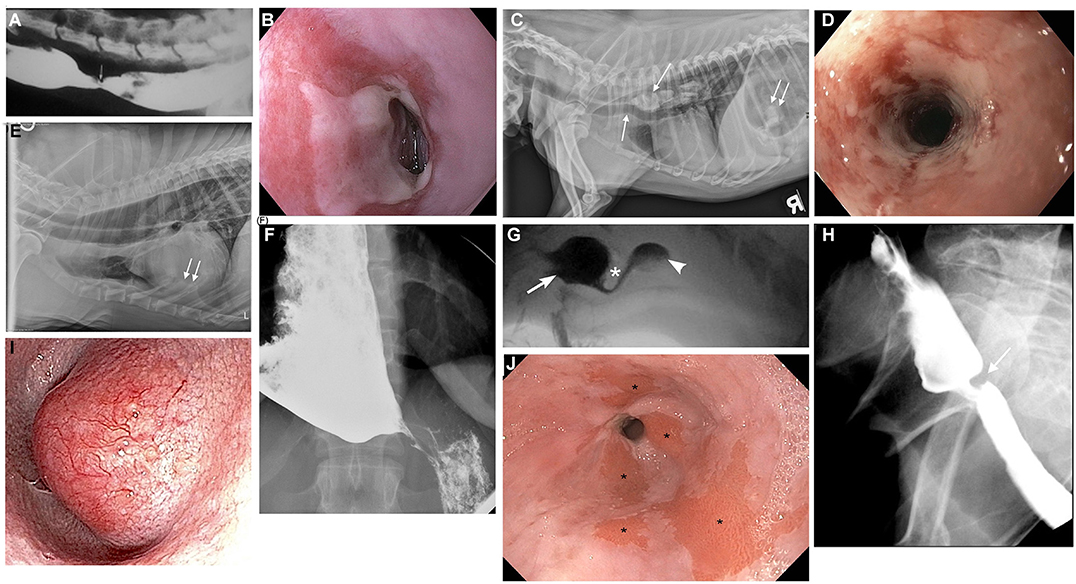
Figure 4. Canine and human esophageal disorders. (A) Contrast esophagram study performed in lateral recumbency in a 2-year old mixed breed dog documenting a focal esophageal stricture (arrow) secondary to severe gastroesophageal reflux. (B) Endoscopic image of a peptic stricture secondary to gastroesophageal reflux in a 58-year-old human patient. (C) Survey lateral thoracic radiograph of a 7-year old mixed breed dog with multiple fragments of a pork bone lodged in the thoracic esophagus (downward arrow). The mineral fragments are distending the esophagus and even ventrally deviate the trachea (upward arrow). There are also a few mineral fragments seen in the gas-dilated stomach (double arrow). (D) Severe ulcerative esophagitis and an esophageal stricture in a 48-year old human patient with a history of gastroesophageal reflux. (E) Right lateral survey thoracic radiograph of a 3-year old male Viszla with a 3-week history of regurgitation, ptyalism, and dysphonia. The esophagus is diffusely gas-distended (arrow) and there are ventral interstitial to alveolar infiltrates within the left cranial and right middle lung lobes (double arrows) consistent with aspiration pneumonia. The dog was diagnosed with focal myasthenia gravis and the megaesophagus resolved with pyridostigmine treatment of the myasthenia gravis. (F) Anterior-posterior contrast radiographic image of a 35-year old human patient with a sigmoid megaesophagus secondary to achalasia. The distal esophagus is distended with barium contrast, but the contrast column narrows into a classic bird's beak shape at the esophagogastric junction due to failed relaxation of the lower esophageal sphincter. (G) Videofluoroscopic still image from a 7-month-old spayed female miniature Dachshund with severe dysphagia secondary to cricopharyngeal achalasia. A hypertrophied cricopharyngeus muscle (cricopharyngeal bar) is seen (asterisk), which obstructs bolus passage of the barium liquid from the pharynx (arrow) into the proximal esophagus (arrowhead). The barium column seen below the asterisk is attenuated as it flows through the narrow opening of the upper esophageal sphincter (UES). (H) Videofluoroscopic still image from a 78-year-old human patient with a cricopharyngeal bar. A fibrotic cricopharyngeus muscle (cricopharyngeal bar) is seen (arrow) that obstructs bolus passage of barium liquid from the pharynx into the proximal esophagus. (I) Endoscopic image of a distal esophageal mass in a 13-year-old male West Highland White Terrier with a history of lip-smacking and regurgitation. The mass had a broad-based attachment to the esophageal mucosa on esophagoscopy, but was surgically resected with marginal excision to confirm a well-differentiated leiomyosarcoma. (J) Endoscopic image of a 62-year-old human patient with a history of chronic gastroesophageal reflux and subsequent Barrett's esophagus. The salmon-colored patches of mucosa (asterisks) in the distal esophagus are areas where squamous epithelium has converted to metaplastic columnar epithelium as a result of chronic esophageal mucosal injury. This patient is at an increased risk of developing esophageal cancer. (A,C,E,G) Images were published in Textbook of veterinary internal medicine: diseases of the dog and the cat, Vol 2, 8th edition, Marks SL, Chapter 273: Diseases of the Pharynx and Esophagus, 8501–8576, Copyright 2017 by Elsevier, Inc, Reprinted with permission from Elsevier.
Differences in Pathology and Anatomy
Despite the similarities, there are numerous differences in pathology and anatomy between the two species. Firstly, although cricopharyngeus muscle dysfunction can affect older dogs, it is far more commonly recognized in young puppies as a congenital anomaly in the form of cricopharyngeus muscle asynchrony (delayed UES opening) or achalasia (ineffective UES opening) (71, 72) (Figure 4G) (82). A hereditary cause for cricopharyngeus muscle dysfunction has been identified in Golden Retrievers, and results of complex segregation analysis suggest that a single recessive allele of large effect contributed to the expression of this disease in the breed (92). In addition, miniature dachshunds, Maltese, toy poodles, and spaniels are predisposed to the congenital development of cricopharyngeus muscle dysfunction (Table 1) (92–95).
Abnormal clinical signs of nasal reflux of milk or food, gagging, and retching immediately upon swallowing manifest shortly after birth, and are exacerbated when swallowing thin liquids compared to thicker liquids or solids. In contrast, cricopharyngeus muscle dysfunction in humans is a rare cause of pediatric swallowing impairment (96). Interestingly, cricopharyngeus muscle dysfunction is well-documented in elderly human patients and symptoms do not appear to be exacerbated following the consumption of liquids. The development of a cricopharyngeus bar, a radiologic descriptor of a posterior impression at the pharyngoesophageal segment, in elderly human patients as a compensatory mechanism to help prevent aspiration of refluxed material is an intriguing consideration; (97, 98) however, a cricopharyngeus bar is commonly observed during swallow fluoroscopy in young puppies diagnosed with cricopharyngeus muscle achalasia (92). Further research is warranted to elucidate the role of this radiologic descriptor in humans and dogs (Figure 4H).
Another difference in disease manifestation between the two species is in the prevalence of Barrett's esophagus (Figure 4J). Despite the high prevalence of GER in brachycephalics since birth and homology in LES anatomy, Barrett's esophagus and neoplastic transformation to adenocarcinoma rarely occurs spontaneously in dogs (99). Instead, the most common esophageal neoplasia in dogs is esophageal sarcomas (osteosarcoma, fibrosarcoma, chondrosarcoma, and undifferentiated sarcoma) secondary to the carcinogenic canine nematode, Spirocerca lupi (100, 101). Esophageal leiomyomas represent the most common benign tumor of the canine esophagus (Figure 4I). Disorders such as eosinophilic esophagitis and megaesophagus also affect the two species with differing prevalence. Eosinophilic esophagitis is far more prevalent in humans and is often diagnosed during childhood (102), however, there are only rare case reports in canines, despite the relatively high prevalence of food allergy, eosinophilic gastroenteritis, and atopy in dogs (48). This is juxtaposed by the high frequency of megaesophagus in dogs, which is diagnosed far more commonly in canines possibly due to differences in neuromuscular anatomy between the two species, and because of the far higher prevalence of myasthenia gravis in dogs. The canine esophageal body is composed entirely of striated muscle (35) whereas in humans, the proximal esophagus (up to one-third), including the UES, is striated and the distal third is smooth muscle with a transition zone between (37) (Figures 5A,B) (53). The peristalsis in the striated muscle of both species is controlled by vagal efferents arising from the nucleus ambiguus in the brainstem (66, 103). These vagal efferents synapse directly on striated muscle motor endplates, and release acetylcholine. Acetylcholine stimulates nicotinic cholinergic receptors, which causes striated muscle contraction. Peristalsis in the striated segment occurs because a pattern generator in the nucleus ambiguus sequentially activates vagal efferents such that the striated muscle motor units are activated in a craniocaudal sequence along the length of the esophagus (104, 105). Control of peristalsis in the smooth muscle esophagus is quite different. Vagal efferents that arise in the dorsal motor nucleus of the vagus innervate myenteric neurons present between the circular and longitudinal muscle layers. Myenteric neurons are the terminal motor innervation of esophageal circular smooth muscle. These neurons are either excitatory or inhibitory. The excitatory neurons release acetylcholine, which activates muscarinic cholinergic receptors to produce contraction. Inhibitory myenteric neurons contain nitric oxide synthase. Their activation causes the release of nitric oxide, which relaxes the lower esophageal sphincter and inhibits contraction of the esophageal circular smooth muscle (106). Nitric oxide plays a key role in the generation of peristalsis in the smooth muscle esophagus, since blocking its production abolishes peristalsis and LES relaxation, and achalasia in humans is the result of loss of nitric oxide synthase neurons (106–108). Despite these differences, manometrically recorded esophageal motor function in canines and humans is remarkably similar (53).
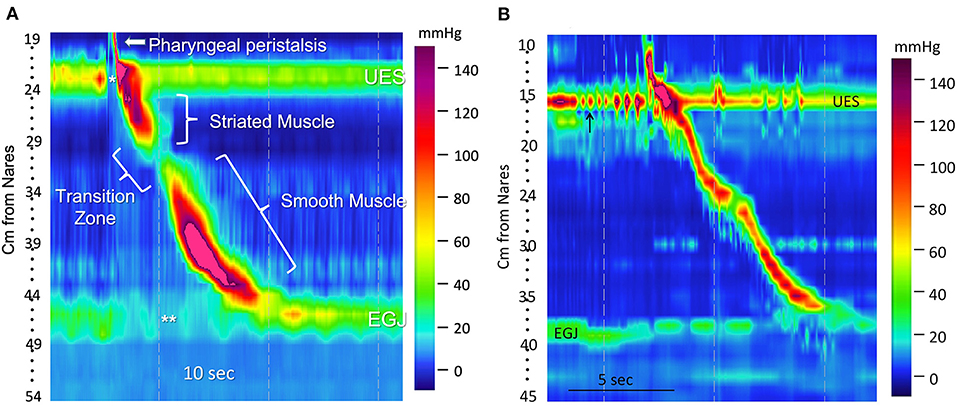
Figure 5. A transition zone is present in humans, but lacking in dogs. (A) A high-resolution color topographical pressure plot of esophageal motor function produced by a 5-milliliter water swallow in a human. It was obtained with a high-resolution manometry (HRM) catheter placed to simultaneously record pressures from the pharynx to the stomach. Pressure is represented by color coding (interpreted on the basis of the color bar on the right), sensor location (distance from the nares in cm) is on the y-axis, and time is on the x-axis. Resting UES (upper esophageal sphincter) and EGJ (esophagogastric junction) pressures are seen as horizontal bands of color that are several centimeters in width. Their hues indicate pressures that are greater than those in the adjacent portion of the pharynx, esophagus, or stomach. Opening of the UES (*) and LES (**) are depicted as changes of color to hues that represent a lower pressure. The narrow, diagonal bar of color above the UES in the pharynx (arrow) represents a pharyngeal contraction. A diagonal band of color running from the UES to 30 cm from the nares represents peristalsis of the striated muscle esophagus, and the diagonal band from 32 cm to the EGJ represents peristalsis in the smooth muscle esophagus. The area of diminished pressure separating these two bands denotes the transition zone over which the muscle is transitioning from striated to smooth. (B) A high-resolution manometry esophageal topography plot showing a pharyngeal contraction and esophageal peristaltic pressure wave generated by the swallow of a 5 g canned food bolus in a 7.2 kg terrier mixed breed dog. There is a continuous diagonal color band from UES to LES representing an uninterrupted peristaltic wave. This continuous peristaltic wave occurs because, except for the LES, the dog esophagus is striated muscle and lacks a transition zone. There are rhythmic contractions of the UES just prior to the swallow (arrow). The genesis of this contractile pattern is unclear, but might represent mastication. (B) was reprinted from American Journal of Veterinary Research, Volume 77, Ullal TV, Kass PH, Conklin JL, Belafsky PC, Marks SL, High-resolution manometric evaluation of the effects of cisapride on the esophagus during administration of solid and liquid boluses in awake healthy dogs, Copyright 2016 American Journal of Veterinary Research. Reprinted with permission from American Veterinary Medical Association.
Nonetheless, neuromuscular differences impact disease phenotype. For example, acquired secondary megaesophagus is more commonly diagnosed in dogs, particularly secondary to myasthenia gravis that is diagnosed in 25% of dogs with megaesophagus (77). This may be because myasthenic autoantibodies preferentially target nicotinic receptors in striated muscle, which is found throughout the canine esophagus, but only the cervical esophagus in humans (109). In contrast, peristaltic defects of the transition zone uniquely occur in humans because dogs lack this anatomic region (110, 111). Similarly, myenteric plexopathies that cause smooth muscle disorders such as distal esophageal spasm or hypercontractile esophagus in humans have not been diagnosed in dogs to date (36, 112, 113).
Other important anatomical differences between humans and canines include esophageal length and nasofacial conformation. Esophageal length in dogs ranges widely with the size and breed of the dog (114), ranging in length from ~20 to 70 cm compared to the consistent range of 18–26 cm in adult humans (115). Nasofacial structure also varies in dogs. Dolicocephalic or mesaticephalic breeds have longer noses and skulls and wider nares compared to brachycephalic breeds (116) (Figures 6A,B). This brachycephalic conformation makes placement of transnasal endoscopes, feeding tubes, and manometric catheters more challenging because their nares are small and their foreshortened muzzles are difficult to grasp during placement. However, the increased nasal length in dolicocephalic breeds can also complicate such procedures because the dead-space of the nasal passage can limit the endoscope or catheter from reaching the LES of the animal. Dogs also have more nasal turbinates compared to humans (117), which makes placing catheters and scopes transnasally more challenging.
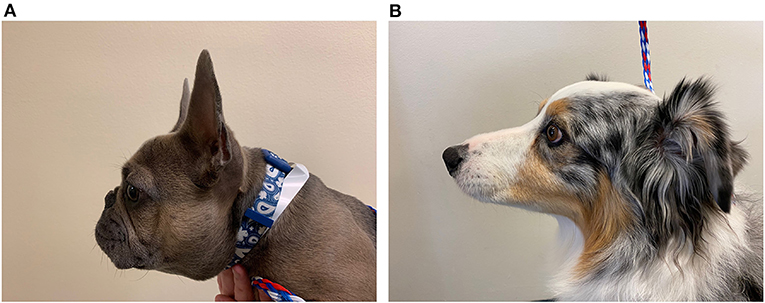
Figure 6. Brachycephalic vs. dolicocephalic conformation. (A) Picture of a brachycephalic 9-month-old French Bulldog showing the foreshortened muzzle, round face, and nasal folds in comparison to (B). (B) Picture of a dolicocephalic breed dog, 7-year-old Australian Shepherd, with an elongated muzzle.
Finally, the anatomy of a bipedal human predisposes to greater GER compared to quadrupedal dogs. Because humans stand upright, gravity assists with esophageal transit, but organs in the chest cavity apply a greater pressure on the diaphragmatic crura, which can compromise the gastroesophageal junction (GEJ). Additionally, the stomach in humans is positioned such that the antrum and pylorus are superior to the fundus whereas in dogs, the antrum is below the fundus to facilitate gastric emptying and minimize reflux (118). However, dogs appear predisposed to hiatal herniation compared to humans because they often lack an intra-abdominal esophageal segment. This exposes the thoracic esophagus and gastric cardia to intrathoracic pressures, which can result in hiatal herniation and reflux (119).
Diagnostic Assessment of Swallowing Disorders in Canines and Humans
Clinical Assessment
The approach to the human or canine patient with swallowing impairment begins with a careful review of the patient's signalment which is particularly important in the canine in light of hereditary and breed-related predispositions (Table 1) (7, 42, 51, 78, 89, 92–95, 120–141).
Congenital causes of swallowing impairment are commonly seen in young pure-bred dogs and several of these disorders are self-limiting in nature or can resolve spontaneously. Specific examples of the latter include juvenile esophageal dysmotility, a self-limiting disorder documented in terrier breeds <1-year of age that is thought to reflect delayed maturation of esophageal neuromuscular function (51). Congenital megaesophagus can resolve spontaneously although the probability of complete recovery is only 20–40% (142) despite higher rates of remission reported in miniature schnauzers (143). In contrast, swallowing impairment in adult or geriatric dogs is typically due to acquired neuropathies, myopathies, or junctionopathies. Clinical signs of regurgitation, hypersalivation, cough secondary to aspiration pneumonia, and emaciation are seen secondary to primary esophageal involvement, however, systemic manifestations of polyneuropathy (geriatric-onset laryngeal paralysis polyneuropathy) or polymyopathy can also be associated with weakness, ataxia, and fever (144).
The common etiologies of dysphagia differ depending on age group for humans as well. Congenital disease is more common in infancy. Traumatic brain injury and neck infections occur more often in pediatric patients (145). GER or immunologic causes (eosinophilic esophagitis or inflammatory myopathy) are more likely in children and adults. Esophageal achalasia more commonly affects the middle-aged. Neurodegenerative disease (Parkinson's disease, dementia, stroke) and neoplasia typically affect the elderly (145).
After considering patient signalment, obtaining a thorough medical history is essential in the assessment of a human or canine patient with swallowing impairment. However, obtaining a history from a human patient is more straightforward. Human patients can describe whether they experience regurgitation, reflux or heartburn, dyspepsia, globus, coughing, choking, drooling, aspiration, or concerning alarm symptoms (vomiting, gastrointestinal bleeding, weight loss) that will expedite diagnostic testing. They can convey the time course of their symptoms as chronic and intermittent (suggestive of a motility disorder) or rapidly progressive, which in combination with weight loss would raise concern for esophageal malignancy. They can indicate whether symptoms worsen with solid foods over liquids, which would suggest a mechanical obstruction caused by a stricture, ring, or web. They can also convey whether their symptoms occur within a second or two of swallowing, which would be suggestive of oropharyngeal swallowing impairment or a proximal esophageal web because the entire pharyngeal swallow occurs in this time domain. Difficulty swallowing that occurs in 5–10 s or more is consistent with esophageal dysfunction, because this is the time over which peristalsis travels to the esophagogastric junction (EGJ). Human patients can point to the area of their discomfort (subxiphoid, mid chest, or cervical) to help localize the problem. Finally, they can report medication and food/seasonal allergy history, which may reveal causative agents of pill-induced esophagitis or triggers for eosinophilic esophagitis, respectively (146). In contrast, canine patients cannot verbally communicate their history and clinical signs. Furthermore, pet owners can misreport regurgitation as vomiting or overlook subtle behaviors such as hard swallowing, lip smacking, and burping. Most pet owners do not closely observe their pets eating or drinking, and mild swallowing abnormalities can easily be missed. In addition, pets are often left unobserved at home during the day when owners are working, further increasing the challenges of obtaining a comprehensive and accurate history from the owner. Thus, veterinarians and pediatricians have similar challenges in assessing their dysphagic patients. To overcome these hurdles in communication, veterinarians must ensure they elicit comprehensive histories from the pet owner. An example list of history and clinical details a veterinarian should inquire about in the assessment of a canine with swallowing impairment is summarized below (147).
1. Age of onset (congenital vs. acquired)
2. Onset of swallowing problem (sudden vs. gradual)
3. Duration of signs (acute vs. subacute vs. chronic)
4. Frequency of signs (intermittent vs. persistent)
5. Progression of signs (static vs. progressive)
6. Temporal pattern (oropharyngeal swallowing impairment will occur within seconds of food or water consumption; esophageal swallowing impairment will occur seconds to hours following food or water consumption)
7. Associations with meals, activity (exacerbates hiatal herniation), or sleep (nocturnal GER)
8. Difficulty with solids, liquids, or both (canine patients with cricopharyngeus muscle achalasia typically experience exacerbation with liquids whereas patients with esophageal strictures experience exacerbation with solid foods)
9. Weight loss (weight loss from chronic regurgitation or reduced food intake) or weight gain (obesity can worsen GER)
10. Weakness, painful or stiff gait, exercise intolerance (suggestive of polymyopathy, polyneuropathy, or junctionopathy)
11. Dysphonia and dyspnea, history of laryngeal paralysis (suggestive of polyneuropathy, polymyopathy, or junctionopathy)
12. Recent administration of medications (pill-esophagitis or stricture formation secondary to clindamycin, doxycycline, tetracycline, ampicillin or non-steroidal anti-inflammatory drug administration)
13. Recent general anesthesia (causing GER and subsequent esophagitis or stricture formation)
14. Historical episodes of aspiration pneumonia (suggestive of aerodigestive disorders)
15. Change in diet (to identify dietary triggers of inflammatory bowel disease or eosinophilic esophagitis or to recognize increased dietary fat content that could precipitate delayed gastric emptying)
16. If brachycephalic dog breed, severity of brachycephalic obstructive airway syndrome (BOAS) and history of previous airway surgery (impacts management of hiatal herniation and GER).
Dysphagia questionnaires (148), such as the Eating Assessment Tool (EAT-10) (149), are used in humans to obtain history, score severity of disease, measure quality of life, and monitor treatment response. A Dog Swallowing Assessment Tool (Dog SAT) is a similar questionnaire currently being validated in dogs to assess the severity of swallowing impairment and help classify the anatomic localization of disease (43) (Supplementary Figure 1). However, the survey still depends greatly on the recognition of pet owners to accurately gauge the signs of swallowing impairment in their pets.
Given the challenges of obtaining comprehensive and accurate histories, a thorough physical examination of the canine patient with swallowing impairment is critical to augment the history and yield important clues. The physical examination should include an assessment of the oral cavity, throat and neck palpation, neurologic evaluation with cranial nerve tests and gag reflex, facial symmetry, muscle atrophy, body condition, and nutritional status. Finally, a critical aspect of the examination is observing the canine patient swallow, which can help characterize and localize the swallowing impairment.
In humans, physical exams may reveal weight loss and frailty, muscle atrophy, neurologic abnormalities, or specific dermatologic abnormalities indicative of connective tissue diseases (scleroderma). Bedside swallow tests (150) can be useful to assess which consistencies a human patient can tolerate (rheology assessment). They can also help screen at-risk neurologic or elderly patients for choking and aspiration. Specifically, poor hyoid elevation during a dry swallow and repeated throat clearing or a wet vocal quality after a wet swallow are suggestive of pharyngoesophageal dysfunction (98). Cognitive assessments and evaluations of social and emotional health can be especially important in the elderly with dementia (76). Psychological evaluations may also be indicated to investigate psychogenic or functional dysphagia if patients are suffering from globus or choking despite normal anatomy and swallow function (151, 152).
Even if there are no reported signs of swallowing impairment and swallow exam is normal, a history of recurrent aspiration pneumonia or chronic cough should alert both veterinarians and physicians to screen for aerodigestive disease (153, 154) and silent (subclinical) aspiration (155). Thus, although history and physical exam are important initial steps of the evaluation, further diagnostic testing with imaging or endoscopy are typically needed to identify aspiration and characterize the swallowing impairment accurately (146, 156).
Contrast Radiography
Imaging in the canine patient typically begins with plain survey radiographs of the cervical region and thorax (3-views) to screen for anatomical and structural abnormalities, including megaesophagus (7, 157) (Figure 4E) (82), vascular ring anomalies (138), hiatal herniation (42, 43) (Figure 3A) (82), foreign bodies (Figure 4C) (82) or intra- or extra-esophageal masses. Radiography with contrast material can delineate strictures, esophageal mass lesions, perforations, and vascular ring anomalies. However, contrast enhanced swallowing fluoroscopy is the gold standard to diagnose swallowing disorders in dogs because it provides a real-time assessment of deglutition (2).
Swallowing Fluoroscopy
Indications
The primary objectives of swallowing fluoroscopy are to localize the swallowing impairment and diagnose its etiology. Swallowing fluoroscopy can also detect tracheal aspiration or laryngeal penetration and guide the management of at-risk patients by modifying the diet consistency or specifically for human patients, teaching compensatory maneuvers.
In both dogs and people, structural abnormalities including strictures, vascular ring anomalies, foreign bodies, esophageal malignancy, and hiatal hernias or pharyngeal weakness, cricopharyngeus muscle achalasia, delayed opening of the upper esophageal sphincter, esophageal achalasia, esophageal dysmotility, and GERD can be diagnosed with swallowing fluoroscopy (3). Fluoroscopy can also confirm aerodigestive disorders in dogs and people with a respiratory history or signs (153, 154). However, there are several other esophageal disorders more commonly identified in humans. Examples include esophageal webs (thin, eccentric squamous epithelium membranes typically found in the proximal esophagus) or rings (thin extensions of tissue causing narrowing typically in the distal esophagus). Tissue in webs and rings is <3 mm in width compared to >3 mm in strictures (158). Other examples include spastic esophageal motility disorders such as distal esophageal spasm (112) or hypercontractile esophagus (113), viral and fungal infectious esophagitis (159), and numerous other causes of oropharyngeal (160) and esophageal swallowing impairment [diabetes mellitus (161), Alzheimer's disease (162), Parkinson's disease (163), Huntington's chorea (164), multiple sclerosis (165), and scleroderma] (16).
Swallowing fluoroscopy informs diet recommendations and safer feeding practices that can enhance swallow function and reduce aspiration risk in dogs and humans (3, 166). Duration of time spent upright in a Bailey chair to facilitate gravity-assisted feeding and treatment modifications can be advised for dogs with megaesophagus (41, 166). Follow-up fluoroscopy can also assess treatment responses or outcomes in dogs or humans that undergo hiatal hernia surgery or achalasia interventions (43, 167, 168).
Procedure
During the fluoroscopic study, patients consume liquid and food boluses of various consistencies mixed with radio-opaque contrast material. In dogs, liquid contrast followed by soft canned food and kibble mixed with barium or iohexol are given, which is analogous to barium liquid, barium pudding, and barium biscuits used in humans. As patients swallow, images are captured as digitized recordings by a fluoroscopy unit at a preferred rate of 30 frames per second. Each frame is then analyzed frame-by-frame to assess swallow kinematics (2, 3).
Although the basic protocol is the same in humans, adjunctive techniques are used in people to minimize aspiration, expose impairments, and formulate therapeutic recommendations (57, 169–172). Firstly, patients are evaluated in both the lateral and anterior-posterior views to maximize visualization and diagnostic yield. Secondly, the patient is asked to perform multiple swallow tasks with varying bolus volumes (5 mL amounts, clinician-directed sips, self-directed swallows), viscosities (thin liquid, thick liquid nectar, thicker liquid honey), food textures (pudding and shortbread cookie), and delivery mechanisms (cup and spoon) to improve diagnostic yield (169, 170). Also, different temperatures, carbonation, and flavors are sometimes altered to change the sensory experience (173–176). Each distinct swallow type provides unique and complementary information for a holistic assessment of the patient. The shortbread cookie is the ideal method to assess oral clearance whereas large volume thin liquid boluses tend to reveal abnormalities in oropharyngeal dynamics (170). Higher viscosity barium or the addition of thickening gum-based agents may reduce the risk of aspiration and penetration. However, several studies show thickening agents can lead to increased post-swallow pharyngeal residues (177–180). Sour liquids can improve pharyngeal delays in swallows (174). The protocol can be further tailored to the particular patient. Larger, thicker boluses can be avoided if pharyngeal clearance of thin 5 mL liquid boluses already appears poor (169). Infants can be bottle-fed with different temperature milk-formulations at varying paces (175, 181). To evaluate the esophagus in more detail, double contrast examination can be performed where the human patient ingests gas producing effervescent tablets followed by barium. This technique fills the esophagus with both air and contrast to delineate mucosal irregularities (182, 183). A barium tablet (e.g., E-Z-DISK contains 700 mg of barium sulfate and is 13 mm in diameter) may also be used to better elucidate a stricture (184, 185).
Compensatory treatment strategies can then be trialed during the study (186). Patients may be asked to wait 3 s before swallowing (187, 188) or to hold their breath while swallowing (supraglottic swallow) to improve coordination and protect the airway from aspiration, respectively (186, 189). Patients may also be instructed to engage in a more forceful swallow (190) or adjust their head or neck postures to facilitate a stronger pharyngeal contraction (191). Specific lingual exercises may be tested during the videofluoroscopy to rehabilitate patients with stroke or traumatic brain injury (192, 193). Provocative maneuvers such as the water siphon test (patient asked to drink water while rolling into a right posterior oblique position) (194), cough stimulation, or Valsalva maneuvers can increase the detection of GER (195).
Challenges and Limitations
Although swallowing fluoroscopy is the gold standard diagnostic procedure to evaluate swallowing impairment in dogs, there are many challenges to conducting the study in this species. Variables such as body position (196), bolus size, bolus type (197), use of physical restraint (198), sedation (199), and equipment can impact the study (172). Positioning of the dog in lateral recumbency is associated with delayed cervical esophageal transit and fewer primary esophageal contractions compared to a standing or seated position (196) (Figure 7A). Seated positions increase the hydrostatic pressure against the lower esophageal sphincter (41) and upright feeding protocols accelerate bolus transit time due to gravity (200) (Figures 7B,C). Solid boluses increase primary peristalsis, but delay pharyngeal contraction and slow esophageal transit compared to liquid boluses (197). Larger boluses shorten the time to UES opening compared to medium sized boluses (197). However, it is virtually impossible to have a dog swallow a consistent bolus volume despite best efforts to facilitate this practice. Even if specified volumes or amounts are administered, dogs may intentionally fragment the bolus into several swallows, particularly if doing so minimizes their signs of swallowing impairment. Large and giant-breed dogs are more likely to swallow the entire solid bolus rapidly without chewing, whereas toy-breed dogs are more likely to chew and fragment the bolus before swallowing resulting in marked variation in bolus size.
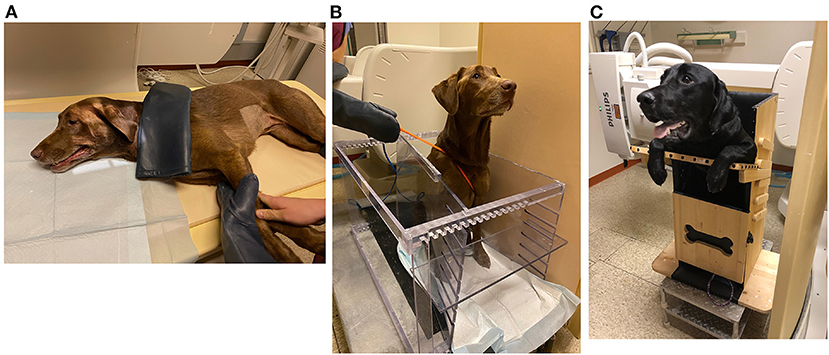
Figure 7. Positioning of dog in swallowing fluoroscopy study. (A) A 5-year-old Labrador retriever undergoing a videofluoroscopic swallow study examination in lateral recumbency with physical restraint by trained personnel. (B) The same patient in (A) undergoing a videofluoroscopic swallow study examination in a polycarbonate kennel restraint device. (C) An 11-month-old Labrador retriever undergoing videofluoroscopic swallow study examination in a Bailey Chair due to history of regurgitation. The Bailey Chair acts as a restraining device and maintains the dog in an upright position, enabling gravity to assist with passage of boluses down the esophagus.
Patient factors such as the dog's size, temperament, severity of disease, and willingness to eat or drink can also affect study quality and accuracy. Non-compliant or larger breed dogs are difficult to physically restrain, which introduces motion artifact and exposes personnel to radiation. Polycarbonate kennel devices and Bailey chairs can restrain the dog and limit radiation exposure to personnel. These restraint devices can also facilitate free-feeding protocols during which the dog voluntarily consumes liquid and solid boluses (41, 166, 198). However, sedation could still be required to calm anxious dogs to facilitate the study, which can potentially alter esophageal motility (201) and sphincter tone (199). Furthermore, stressed, anorexic, or severely ill dogs may refuse to voluntarily prehend the bolus, requiring force-feeding practices that increase the risk of aspiration pneumonia or pre-empt a complete evaluation. Additionally, the compensatory treatment strategies used in humans require complex verbal directions that cannot be relayed to dogs. Dynamic disorders that occur intermittently such as GER or sliding hiatal hernia might also be missed given the limited duration of the swallow fluoroscopy study.
Due to these enumerable factors, swallowing fluoroscopy procedures are challenging to perform in dogs and difficult to standardize. Study protocols vary between patients and veterinary institutions (Table 2) (3, 41, 43, 51, 81, 153, 166, 168, 196–198, 202, 203). A standardization initiative amongst veterinary institutions and practices would improve reproducibility, but even with a uniform methodology, variability exists amongst healthy dogs and interpreting radiologists (172, 198). Optimally, objective swallow metrics such as inter-swallow interval, time to UES opening, time to maximum pharyngeal contraction, pharyngeal constriction ratio (204), and esophageal transit time would be used (198). However, further research of these parameters is needed to establish normative data and prove their diagnostic validity in dogs. In humans, there are a multitude of established swallow metrics in healthy individuals with normative data (205, 206). The effects of age (207), gender (208), bolus volume (209, 210), viscosity (173, 177, 178, 180, 209–211), carbonation, and palatability (176, 212) on these parameters are much better understood in humans. The swallowing reflex and UES opening delays with age (207) and larger bolus volumes (209, 210), higher viscosity (178), and carbonation decrease the risk of penetration and aspiration in some populations (176, 212). There are also many standardized study protocols and training systems such as the Modified Barium Swallow Impairment Profile (MBSImp) protocol (169) to minimize interoperator and interrater variability. However, even for humans, a comprehensive, evidence-based set of practice guidelines that is used globally is lacking (213).
Future Directions in Veterinary Medicine
Swallowing fluoroscopy methodology should be more standardized in veterinary practice. This will help establish quantitative normative data in dogs. Separate reference ranges for swallow metrics should also be established for specific age groups from juvenile to mature adult to geriatric dogs. This would optimize evaluation of oropharyngeal and esophageal function in animals of different ages. Although standardization is essential, study methodology should also be tailored to specific patients and clinical scenarios. In patients with cricopharyngeus muscle dysfunction, smaller volumes or thicker consistencies can minimize aspiration and improve swallow safety although larger volume, thinner consistencies can reveal abnormalities and improve diagnostic yield (178, 180, 210, 211). For dogs with esophageal achalasia, timed barium esophagrams can be performed in which barium retention is assessed at specified time intervals following ingestion (214, 215). For patients with suspected hiatal herniation or GERD, provocative maneuvers akin to those used in humans could be performed to improve detection (83). The stomach can be maximally distended with food or air to stimulate TLESR and subsequent GER. Patients can be rotated into different body positions to elicit abnormalities. Lateral recumbency will apply more pressure to the fundus to encourage hiatal herniation (114) whereas a seated position will increase hydrostatic pressure against a hypertonic LES in patients with esophageal achalasia (41). Thus, both standardization and individualization are important to improve the diagnostic utility of swallowing fluoroscopy in veterinary medicine.
The clinical applications of swallowing fluoroscopy extend beyond diagnosis. It can be utilized to assess outcomes of medical (sildenafil, botulinum toxin, pneumatic dilation) (167, 216) or surgical management (myotomy with fundoplication) (167) of esophageal achalasia, prokinetic and proton pump inhibition or surgical treatment of GER or hiatal herniation (43, 168), and laser or surgical myectomy for cricopharyngeus muscle achalasia (39).
Fiberoptic Endoscopic Evaluation of Swallowing (FEES)
Indications
Fiberoptic endoscopic evaluation of swallowing (FEES) is a procedure commonly used in humans to evaluate oropharyngeal swallow function. FEES eliminates the use of specialized fluoroscopy equipment, contrast agents, and radiation required for swallowing fluoroscopy (217). Systematic reviews have shown an advantage of FEES over swallowing fluoroscopy to detect aspiration, penetration, and laryngopharyngeal residue in humans (218, 219). Guided observation of swallowing in the esophagus (GOOSE) can also be performed afterwards to identify a structural abnormality or evidence of delayed esophageal transit (220).
Procedure
The nasal passage is routinely anesthetized with 4–5 drops of topical anesthetic in dogs, and the tip of a fiberoptic endoscope (2.9 mm outer diameter in dogs) is lubricated before being passed transnasally in an awake patient. The endoscope is advanced until the scope is positioned between the soft palate and tip of the epiglottis, facilitating observation of the base of the tongue, vallecula, larynx, and both pyriform sinuses. The patient is then offered boluses of liquid and food stained with food coloring. During each swallow, laryngeal and pharyngeal anatomy is directly observed to evaluate the integrity of pharyngeal function and document evidence of penetration or aspiration (217, 221).
Challenges and Limitations
Disadvantages of the procedure are that the endoscope can interfere with deglutition and pharyngeal contraction can cause transient image white-out. Patients may also experience excessive gagging, coughing, or anxiety with scope placement. FEES was successfully performed without sedation in 6 healthy dogs; however, the procedure warrants further assessment in dogs with swallowing impairment. Additionally, passage of the 2.9 mm diameter scope may not be feasible in smaller dogs or brachycephalic breeds with narrow nares (221).
High-Resolution Manometry
FEES or fluoroscopy is optimal to assess oropharyngeal dysphagia, but high-resolution manometry (HRM) is preferred to evaluate esophageal motility in humans (222). Esophageal manometry measures esophageal pressure profiles using an intraesophageal catheter lined with pressure sensors (223). Data captured at rest and during swallows are digitally converted to contoured line tracings or color topographical plots of pressure that depict esophageal motor function (Figures 5A,B) (53, 224). Compared to the 3–5 pressure sensors in conventional manometry catheters, high-resolution catheters have 36 pressure sensors spaced at 1-cm interval along the catheter, giving a 35-cm sensing segment. Each pressure sensor measures pressure at 12 positions around it circumference, which substantially improves the quantity and quality of data captured (225) (Figures 8A–C).
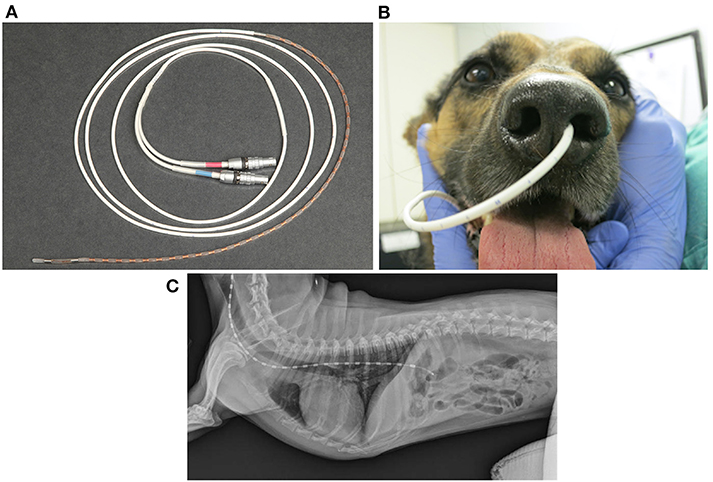
Figure 8. Implementation of high-resolution manometry in dogs. (A) A coiled 8Fr high resolution manometric (HRM) solid-state catheter with 36 circumferential pressure sensors spaced 1 cm apart, lining the end of the catheter. The red and blue labeled connectors plug into the manometry hardware module, which transmits information to the manometric data acquisition software that runs on a computer. (B) A picture of the HRM catheter successfully placed transnasally into the left nasal passage of a 4-year-old, 18-kg, mixed breed dog. (C) Survey lateral thoracic radiographic view of a 7.2-kg terrier cross after placement of a high-resolution manometry probe. In this dog, the probe traverses both the UES and LES. The brighter rectangular regions spaced equally along the probe represent each of the 36 probe sensors. (C) was reprinted from American Journal of Veterinary Research, Volume 77, Ullal TV, Kass PH, Conklin JL, Belafsky PC, Marks SL, High-resolution manometric evaluation of the effects of cisapride on the esophagus during administration of solid and liquid boluses in awake healthy dogs, Copyright 2016 American Journal of Veterinary Research. Reprinted with permission from American Veterinary Medical Association.
Indications
HRM is utilized to diagnose esophageal motility disorders in humans after obstructing lesions have been ruled out. Motility disorders can be classified into disorders of EGJ outflow including esophageal achalasia (type I, II, or III) and EGJ outflow obstruction or disorders of peristalsis such as absent contractility, distal esophageal spasm, hypercontractile esophagus, and ineffective esophageal motility (91, 226). The key metrics analyzed with HRM are integrated relaxation pressure (IRP), distal contractile integral (DCI), and distal latency (Figure 9A) (222) to assess LES relaxation, strength of esophageal peristalsis, and latency of deglutitive inhibition, respectively, which help characterize the type of major or minor motility disorder. For example, an elevated IRP denotes an esophageal outflow obstruction or esophageal achalasia (Figure 9B) (222). An increased DCI is supportive of a hypercontractile disorder such as jackhammer esophagus (hypercontractile esophagus). A short distal latency indicates premature contractions as seen in distal esophageal spasm (91). Interpreting IRP, DCI, and distal latency in combination aids in subtyping esophageal achalasia as type I, II, or III (Figure 9C) (222). Type I (classic) achalasia is marked by the absence of peristalsis in 100% of swallows, an elevated median integrated relaxation pressure (IRP > 15 mm Hg) and distal contractile integral (DCI) <100 mm Hg/s/cm; type II by panesophageal pressurization in ≥20% of swallows (most common subtype) and elevated median IRP (>15 mm Hg), and type III as elevated median IRP (>15 mm Hg) and premature, contractions in ≥20% of swallows with DCI > 450 mm Hg/s/cm (227).
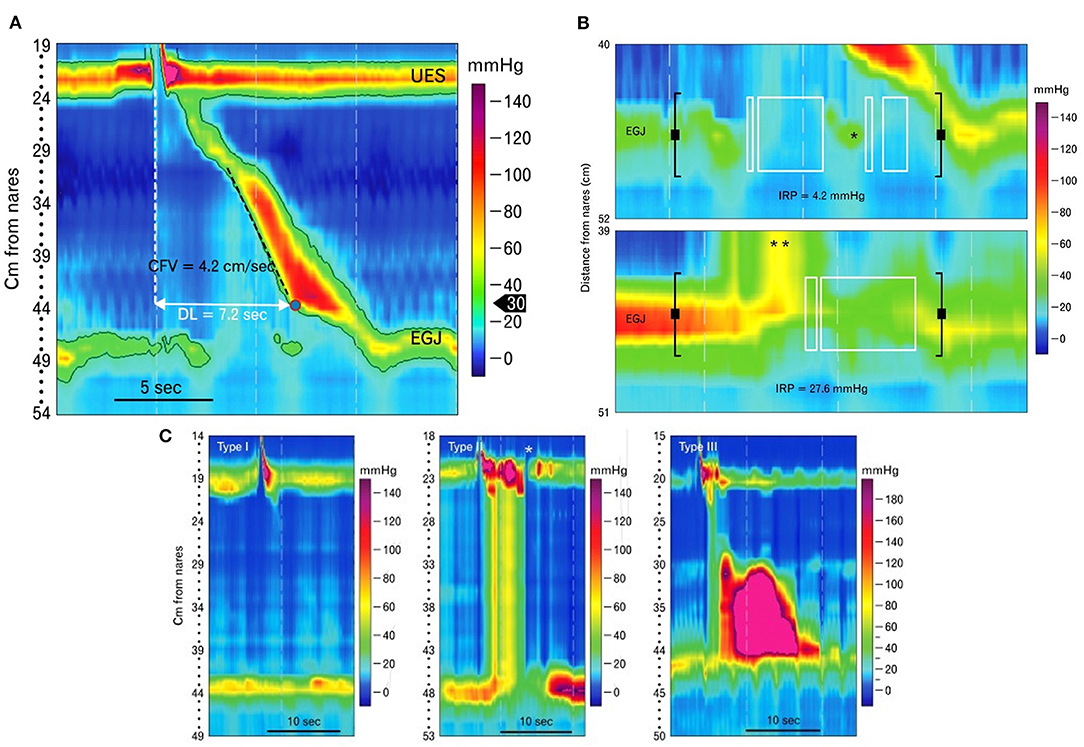
Figure 9. Esophageal pressure topography plots generated using high-resolution manometry in human patients. (A) High-resolution manometry catheters span and simultaneously measure pressures from pharynx to stomach and measure pressure throughout the esophagus. The results are graphically depicted in color contoured esophageal pressure topography plots as seen here. Metrics such as contraction front velocity (CFV) and distal latency (DL) can then be measured and calculated to evaluate esophageal peristalsis. CFV is a measure of peristaltic velocity in the smooth muscle portion of the esophagus. Distal latency is the time from upper esophageal sphincter (UES) opening to the contractile deceleration point (CDP), when peristalsis terminates at the esophagogastric junction (EGJ). (B) The top image shows the pressure topography of the esophagogastric junction (EGJ) following a liquid swallow in a normal human patient. Integrated relaxation pressure (IRP) is measured during a time window (bounded by black brackets) that occurs after upper esophageal sphincter (UES) opening. An eSleeve tool determines the highest pressure at each point in time within this window. An algorithm is then used to average the lowest of those pressures over four continuous or discontinuous seconds (marked by the white boxes). This average is the IRP. The example below is the EGJ pressure topography of a patient with achalasia. Due to failure of lower esophageal sphincter (LES) relaxation, excessive pressurization of the swallowed bolus occurs above the LES (**) and IRP is elevated. (C) Esophageal pressure topography plots showing the three different types of achalasia from left to right: Type I, II, and III. Achalasia is defined by a failure of normal peristalsis and lower esophageal sphincter opening and can be further classified into 3 types. Type I is characterized by absence of peristaltic activity in the esophagus without esophageal pressurization, type II by panesophageal pressurization and type III by premature esophageal contractions. The asterisk in the middle panel points to a brief opening of the UES that is not associated with a pharyngeal contraction or swallow and is therefore an example of the UES opening to vent. The images in this figure were published in Journal of Neurogastroenterology Motility; Volume 19, Conklin JL, Evaluation of esophageal motor function with high-resolution manometry, 281–294, Copyright 2013 by The Korean Society of Neurogastroenterology and Motility, Reprinted with permission from Editorial Office of Journal of Neurogastroenterology and Motility.
An accurate diagnosis and classification optimizes treatment and informs prognosis. Esophagogastric junction (EGJ) outflow obstruction may resolve spontaneously or with discontinuation of opioid medications (58). Spastic disorders including distal esophageal spasm and type III (spastic) achalasia benefit from peroral endoscopic myectomy (POEM) surgery while type I or II achalasia have better outcomes with interventions focused on the LES (pneumatic dilation or Heller's myotomy of the LES) (228–231). HRM can also diagnose hiatal hernias (232) or evaluate esophageal motility before (233) and after anti-reflux fundoplication surgery (234, 235).
In contrast to human medicine, HRM is still a relatively novel procedure in veterinary medicine and has been utilized predominantly in healthy dogs. However, the procedure has been successfully conducted in awake dogs (53, 236, 237) and results showed vigorous peristaltic contractions with solid compared to liquid boluses and a significant increase in LES pressure induced by cisapride compared to metoclopramide (53, 236). Thus, with further study, HRM could improve the characterization and treatment of esophageal motility disorders in dogs.
Procedure
HRM can be conducted in both humans and dogs with a similar protocol except for a series of provocative maneuvers. The procedure begins with a temperature and pressure calibration. The calibrated manometric catheter is then placed transnasally in the awake patient. Humans should be positioned supine (12–30 degree angle) and dogs restrained in sternal recumbency or seated. The nasal passage is topically anesthetized with lidocaine jelly (238) ± a combination nasal spray of 1% tetracaine and 0.05% oxymetazoline (239) and the catheter is lubricated to facilitate passage of the flexible catheter into the nasopharynx and down the esophagus (Figures 8B,C) (53). Two to 3 mL of water can be administered orally to trigger peristalsis and advance the catheter past the LES. The distal 3–4 sensors are positioned intragastrically and the UES and LES should be visible on the image display as two bands of higher pressure above and below (Figures 5A,B) (53). Once the catheter position is set and the patient has acclimated to the catheter, baseline recording can begin. After baseline data is obtained, 5 mL boluses of water at room temperature are given and a swallow is recorded. This process is repeated until 10 consistent, intact swallows are obtained (227). In larger sized dogs and tall human patients with an esophageal length >30-cm, the manometry catheter may not span the entire esophagus, and the catheter is placed distally to span the distal esophagus and LES for acquisition of topographic data before being pulled proximally to repeat the procedure at the proximal esophagus, UES, and pharynx (53).
After the standard 10 water swallows, “provocative maneuvers” (240) are attempted in human patients to further test esophageal function. A multiple rapid swallow test (241) is performed by asking the person to swallow 5 times in quick successions. This helps reveal impairments in deglutitive inhibition or weak peristaltic reserve to diagnose achalasia (242) or ineffective esophageal motility (243), respectively. The individual is then moved to an upright position where at least 5 liquid swallows and another rapid swallow test are performed. The upright posture better replicates normal eating and improves detection of motility disorders (200) and hiatal herniation (244). If findings are equivocal, solid foods may be administered (245, 246) or post-prandial monitoring (247) is performed to improve diagnostic yield.
Challenges and Limitations
HRM has great potential in veterinary medicine, but there are many challenges to its implementation in dogs. Even with appropriate physical restraint of the patient, placement and retention of the manometric catheter is variably successful in the awake dog due to operator experience, patient non-compliance, and nasopharyngeal and esophageal anatomy. Dogs can be challenging to restrain during passage of the catheter transnasally, and are extremely sensitive to catheter insertion, particularly brachycephalic breeds with their shorter muzzle, upper airway obstruction, and respiratory distress. The catheter can be forcefully sneezed out due to nasal irritation, causing transient epistaxis and potential damage to the fragile pressure sensors along the catheter. In patients with megaesophagus or a sigmoid esophagus from esophageal achalasia, the catheter can coil and loop back on itself in the distended esophagus or meet physical resistance at the LES. Even if the catheter is placed successfully, it can be challenging to maintain in place, particularly while the dog is swallowing liquid or food boluses. Sedation can calm the dog to facilitate placement and retention, but sedatives or tranquilizers such as butorphanol and acepromazine can affect manometric parameters (237), and chronic opiate administration is known to affect esophageal function in humans (248).
Additionally, the provocative maneuvers applied in humans can be imitated in dogs, but are more challenging to replicate. For example, dogs can be kept in an upright position in a Bailey chair if they are amenable, but movement of their heads is uncontrolled and dogs with osteoarthrosis may be uncomfortable sitting on their haunches. Furthermore, this position does not emulate the physiologic feeding position of dogs as quadrupeds. Pre-determined bolus weights or volumes can be syringe-fed at specific intervals to perform a multiple rapid swallow test, but dogs may partially swallow the bolus at irregular intervals or retain material from multiple boluses in their mouths before swallowing at unpredictable times.
Furthermore, as with swallowing fluoroscopy, HRM methodology and data interpretation has not been standardized in dogs, whereas in humans there is a standardized protocol and consensus (the Chicago Classification v4.0) to diagnose and categorize esophageal disorders (91). In addition to the challenges of performing and interpreting HRM in dogs, the cost of manometry probes, hardware modules, and software costs is excessive for most veterinary practices and pet owners, and the procedures are not covered by veterinary insurance. The procedure is thus restricted to a few veterinary academic institutions in which it is predominantly utilized as a research tool.
Future Directions in Veterinary Medicine
Further evaluation of HRM in dogs with swallowing impairment is needed to develop a system analogous to the Chicago Classification. However, the many challenges encountered with HRM could impede its widespread application in veterinary practice. Meanwhile, the role of HRM in human medicine is expanding. Emerging applications in humans include the implementation of three-dimensional (3-D) HRM, in which there is a 9-cm segment lined with 12 pressure sensing loci and 8 radially dispersed pressure sensors at each locus, to evaluate pressure distributions at the pharynx, UES, and EGJ (249) or combination HRM-impedance technology to evaluate post-prandial TLESRs (250) or belching disorders (247). HRM-impedance technology is also being trialed in humans to assess bolus transit and post-residue swallows in patients with oropharyngeal (251) and esophageal dysmotility (252, 253).
Once metrics and normal reference ranges are established, HRM should be performed in dogs with swallowing abnormalities to appreciate the spectrum of esophageal dysfunction. Performing HRM in clinical patients could confirm esophageal motility disorders similar to those found in humans including ineffective esophageal motility, hypercontractile esophagus, distal esophageal spasm, and esophageal achalasia. HRM could also help differentiate the causes of oropharyngeal dysfunction or assist in the diagnosis of hiatal herniation and GER in dogs. Improved detection of these disorders will invariably improve treatment and patient outcomes.
Intraesophageal pH Testing
Esophageal pH-metry and combined pH-impedance monitoring are the optimal procedures to diagnose and monitor GER. Esophagography, swallowing fluoroscopy (254), endoscopy and esophageal biopsies can reveal esophagitis (183), Barrett's esophagus, or peptic strictures secondary to reflux (255), but these methods lack sensitivity (256, 257) to detect reflux compared to pH monitoring (148, 149). pH-metry collects data over ≥24 h to survey acid reflux and the addition of impedance technology helps detect non-acidic and weak acid reflux (258, 259). In addition, multi channel intraluminal impedance and pH monitoring (MII-pH) measures changes in electrical impedance to determine composition, direction, and movement of the refluxate (260) to track gastric content moving orad or esophageal content moving aborad.
Indications
pH-metry is used in humans to diagnose GERD, classify phenotype as erosive or non-erosive reflux disease (NERD), and direct treatment. Human patients symptomatic for GER are initially treated with a proton pump inhibitor (PPI), but up to 33% of patients do not respond to a 2-week PPI course (261, 262). For PPI-refractory patients, upper endoscopy and ambulatory pH monitoring are recommended to evaluate for confirmatory evidence of GER. pH monitoring can also be used in conjunction with methods that assess gastric motility to assess whether delayed gastric emptying is exacerbating GER (20, 263).
In contrast, ambulatory reflux monitoring is understudied and underutilized in veterinary medicine. pH monitoring in dogs has mainly been performed under anesthesia with catheter-based techniques (29, 164–185). Numerous factors such as age (84, 264), sex (265, 266), breed (267), body size (268), type of surgery (84, 269), length of pre-operative fasting (85, 264, 270), body position (84, 269), anesthetic agents (morphine, acepromazine, inhalant gases) (271–275), and use of maropitant (276, 277), acid suppressant (omeprazole and esomeprazole) and prokinetic medications (metoclopramide and cisapride) (54, 276, 278–281) have been evaluated in association with peri-anesthetic GER. However, results have been variable and often conflicting possibly due to differing anesthetic protocols, definitions of reflux, and methods of pH measurement (Table 3) (45, 54, 84, 85, 264–283). For example, in some studies, increasing age and prolonged pre-anesthetic fasting were identified as risk factors for GER (84, 270), but were found to be protective in others (264). Changes in body position were associated with acid reflux in one study (269), but had minimal effect on GER in other studies (84, 275). Medications such as metoclopramide and omeprazole (280, 281) were initially found to reduce reflux under anesthesia, but subsequent publications refuted this claim (276). pH/impedance technology has now shown that PPIs only raise esophageal pH enough to mitigate acid reflux (54, 279), but cisapride significantly decreases both acid and non-acid reflux (54).
Procedure
The multichannel intraluminal impedance/pH catheter (MII-pH) is a 2.13 mm (6.4Fr) diameter catheter made of polyurethane. It has 6–8 impedance sensors, spaced 2-cm apart, and 1–2 pH sensors. After calibration in the appropriate pH buffer solutions, the catheter is placed transnasally or transorally (for assessment of GER in anesthetized canine patients) into the esophagus such that the proximal pH sensor is 5-cm above the LES or 6-cm proximal to the EGJ (Figures 10A,B). The catheter is affixed to the patient's face or around the dog's muzzle and kept in place for the duration of the study. Catheter-free ambulatory pH monitoring can be performed with a wireless Bravo pH capsule. Although impedance data cannot be obtained, pH data can be collected for up to 96 h, which minimizes variance and increases diagnostic sensitivity (284). The pH capsule is deployed with endoscopic guidance and tethered to the esophageal mucosa 6-cm proximal to the EGJ with a suction and locking pin mechanism (Figure 10C). The capsule measures pH every 6 s and transmits data to a receiver every 12 s using radio telemetry. Within 5–7 days, the capsule naturally detaches from the esophagus and passes through the intestinal tract. Data from the receiver can then be uploaded to a computer software program for analysis (285, 286). The primary outcome measure assessed is distal esophageal acid exposure time (AET). Acid exposure time >6% denotes pathologic GER, and <4% is considered physiologic in humans. Symptom association indices are also evaluated to determine the correlation of reflux events and patient reported symptoms of heartburn and regurgitation (255).
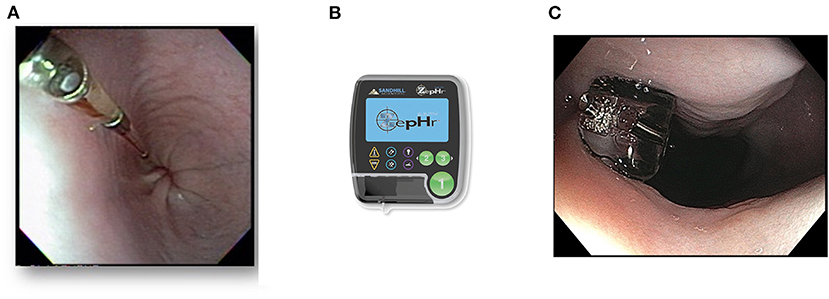
Figure 10. Multichannel intraluminal impedance/pH (MII-pH) catheter and ambulatory pH capsule. (A) Photographed is a 6.4-French (2.13 mm) esophageal multi-use impedance/pH probe in the esophagus of a dog patient. (B) The recording device (ZepHr) that the impedance/pH probe connects to. (C) A wireless BravoTM Calibration-Free Reflux pH Capsule tethered to the esophageal mucosa after placement in a 2-year-old French Bulldog with a diagnosis of hiatal herniation and gastroesophageal reflux.
Challenges and Limitations
pH testing should be utilized more frequently in veterinary medicine to evaluate GER in non-anesthetized canine patients. Unfortunately, transnasal placement in an awake dog and retention of the probe for 24 h is challenging. However, ambulatory pH monitoring utilizing the wireless pH capsule (Bravo) is catheter-free and enables continuous pH data collection in the awake patient for up to 96 h. Results can show daily fluctuations in pH in association with the patient's symptoms, meal or water intake, body position, and activity (284, 287). As previously mentioned, the major limitation of wireless pH capsule monitoring is that it lacks impedance technology and therefore cannot diagnose non-acid reflux events or belching/rumination (repetitive regurgitation of undigested food due to a learned behavior) disorders. Additionally, minor technical complications such as breaks in data transmission, early dislodgement, or capsule retention can occur. However, non-trivial complications including esophageal wall trauma or capsule aspiration are rare (288). Another drawback to placing pH capsules in dogs is that the procedure requires general anesthesia and optimal positioning for placement is reliant on human studies. In humans, the capsule is consistently placed 6-cm proximal to the EGJ, but dogs have variable esophageal and sphincter lengths (114). Thus, a standard 6-cm distance may not be applicable to all dogs and could contribute to variation in pH readings. Another significant limitation to using pH monitoring in dogs is that normative data and metrics for analysis have not been established. In a study that used nuclear scintigraphy to evaluate reflux in healthy dogs, reflux events occurred on average twice every 5 min, however, pH monitoring was not performed to discern whether these were acid reflux events (289). A study that performed ambulatory pH monitoring in 7 healthy dogs documented a median of 10 acid reflux events (range 1–65) over a median study duration of 45 h (45).
In contrast to canines, there are conclusive criteria to diagnose GERD in humans. These have been summarized and published in the Lyon Consensus of 2018. They include both endoscopic criteria and metrics such as number of reflux episodes per 24 h, AET, SI (symptom index), and SAP (symptom association probability) (255). These metrics can even be assimilated into a DeMeester composite score (290) which directly correlates with endoscopic findings of erosive esophagitis. Endoscopic findings are even graded with established classification schemes such as the Los Angeles Classification system (291).
For example, if advanced grade erosive esophagitis (Los Angeles classification grade C and D) (Figure 4D), long-segment Barrett's mucosa, or peptic strictures are seen on endoscopy or distal esophageal AET > 6% on ambulatory pH or pH-impedance monitoring in a human patient, GERD is confirmed (255). However, <50% of human patients have endoscopic evidence of esophagitis (292, 293). These patients may have non-erosive reflux disease (NERD) or functional esophageal disorders.
If endoscopic findings are normal, but AET is >6%, NERD is diagnosed. If endoscopy and AET are both normal, further testing with pH-impedance is warranted to document non-acid reflux. Additionally, symptom-reflux association metrics SI (294) and SAP (295) should be analyzed. SI is the percentage of symptom events preceded by reflux episodes and SAP is the probability that symptoms and reflux events are associated. Patients with SI > 50% and SAP > 95% are predictive of better responses to medical and anti-reflux surgical therapy (296, 297). pH monitoring can also be repeated after anti-reflux surgery to assess surgical response. Symptom-reflux association is also used to diagnose functional esophageal disorders of altered nociception. Human patients with normal endoscopy and AET, but high symptom-reflux association may have reflux hypersensitivity. Alternatively, if symptom-reflux correlation is poor, functional heartburn is possible and cognitive behavioral therapy may be necessary (255).
The Lyon consensus also recognizes the heterogeneity of GERD in humans. For example, although an AET <4% is considered normal and > 6% defines pathologic reflux, patients may be diagnosed with borderline or inconclusive GERD based on low-grade esophagitis on endoscopy, equivocal AET between 4 and 6%, or normal AET but positive symptom-reflux associations.
In such cases, further testing with impedance and HRM may be warranted to interrogate for non-acid reflux, evaluate esophageal mucosal permeability, assess LES tone, and screen for poor esophageal contractility and delayed acid clearance (255). Novel impedance metrics including baseline impedance (298, 299) and post-reflux swallow-induced peristaltic wave (PSPW) index (300) can be used to assess esophageal mucosal integrity and peristalsis following a reflux episode, respectively. Although normative values for these impedance metrics are not yet available in humans, low baseline impedance is indicative of alterations in intercellular space and tight junctions secondary to reflux, and abnormal PSPW reflects diminished peristalsis and prolonged acid clearance (298, 301).
Future Directions in Veterinary Medicine
Additional pH/impedance studies should be performed in awake, healthy dogs to establish normative reference ranges for key metrics. This will provide a contextual basis to perform studies in clinical canine patients that help differentiate physiologic reflux, pathologic reflux, and functional/hypersensitivity conditions. A relevant patient population to study would be brachycephalic dogs given the high prevalence of GER and hiatal herniation (42, 50). Dogs with signs of aerodigestive disease would also be a pertinent patient group. The wireless Bravo pH capsule could help evaluate whether cough or nasal symptoms were correlated to reflux events. Once a classification system is developed, pH monitoring can be used in dogs, as it is in humans, to diagnose GERD phenotype, direct therapy, and assess response to medical or surgical treatments.
Esophageal Histopathology
Further confirmation of GERD can be obtained by identifying esophageal histopathologic alterations secondary to GER. Examples include Barrett's esophagus (columnar metaplastic change) (302), dilation and edema of intercellular spaces, infiltration of mononuclear cells, proliferative basal cell hyperplasia, and papillary elongation of the squamous epithelium. Acute or healed erosions may also be seen (303, 304). Identification of such pathology can support the diagnosis of GERD (305) and serial biopsies can confirm treatment response.
In human patients, microscopic esophagitis significantly improves in response to administration of PPIs (306) and anti-reflux surgery (307). Similar findings have been observed in dogs (308, 309). Seventeen of 65 (26%) dogs clinical for reflux had evidence of hyperregeneratory esophagopathy (HRE), characterized by basal cell hyperplasia and papillary elongation, of which 12/17 (71%) responded positively to PPI treatment (46). Thus, esophageal histology not only aids in the diagnosis of GER, but justifies therapy. However, challenges to performing and interpreting esophageal biopsies in dogs should be recognized. Firstly, it is difficult to obtain endoscopic biopsies of the esophageal mucosa in dogs because the tissue is incredibly resilient. As a result, veterinary laboratories rarely receive adequate tissue samples for analysis. Even if a sufficient sample is obtained, normal esophageal histology does not exclude GERD (310, 311). Finally, there are no standardized criteria or established scoring systems to evaluate esophagitis in dogs.
In human patients with cricopharyngeus muscle dysfunction, biopsy of the cricopharyngeus muscle can be informative. Myositis of the cricopharyngeus muscle may occur secondary to polymyositis, dermatomyositis, or inclusion body myositis. Concurrent fibrosis indicates chronicity of disease (312, 313). Cricopharyngeus muscle histology in 5 dogs diagnosed with cricopharyngeus muscle dysfunction showed myofiber degeneration and atrophy suggestive of an underlying neuropathy, but larger sample sizes are needed (39). Severe ganglionic cell depletion of the LES in human patients with esophageal achalasia indicates progressive, end-stage disease, characteristic of type I achalasia (314); however, histopathology of the LES in dogs with esophageal achalasia-like syndrome has not been performed to date.
Electrodiagnostics
Indications
Electrodiagnostic testing, including electromyography, nerve conduction velocity testing, and repetitive nerve stimulation, can confirm neuromuscular causes of swallowing impairment (315–317). Results can also guide selection of muscle and nerve biopsy sites (318). In humans, electromyography can also be used as a screening tool for dysphagia (319) and as biofeedback to guide swallowing rehabilitation (320). In dogs, electrodiagnostics have elucidated esophageal physiology (321) and explored pathology in patients with laryngeal paralysis (322) and megaesophagus (323–325). This has led to the hypotheses that vagal afferent dysfunction and secondary alterations in biomechanical properties explain the pathogenesis of idiopathic megaesophagus in dogs (323–326). Electromyography has also played an integral role in facilitating the diagnosis of inflammatory myopathies, including polymyositis, an immune-mediated disorder well-documented in Boxers and Newfoundlands (134).
Procedure
Electromyography is performed by inserting electrodes into skeletal muscles of interest such as pharyngeal, laryngeal, esophageal, thoracic, and pelvic limb musculature. Abnormal spontaneous electrical activity, characterized by scattered fibrillation potentials, positive sharp waves, and complex repetitive discharges, is consistent with a myopathy or neuropathy. Motor and sensory nerve conduction velocity testing and repetitive nerve stimulation measure electrical activity in muscles following nerve stimulation (316). Muscle biopsies can then be obtained to analyze affected myofiber types and identify features of inflammation, atrophy, or necrosis. Immunofluorescence staining on muscle biopsies can also characterize antibodies, major histocompatibility complexes, or T-lymphocytes to suggest polymyositis or identify protein deficiencies associated with muscular dystrophy (134). Nerve biopsies can be analyzed for inflammatory infiltrate, axonal degeneration, axonal dystrophies, demyelination, and nerve regeneration (318).
Challenges and Limitations
In humans, conventional electromyography electrodes can be placed intramuscularly in awake patients. Surface electromyography with adhesive electrodes (319) or skin patches can also be performed (320) in awake patients and can characterize swallowing disorders (327). In dogs, general anesthesia is required, which makes the procedure more costly and labor-intensive. Furthermore, procedural technique must be adhered to strictly to limit confounding variables. Electrodes must be grounded properly and inserted at various depths and locations in the muscles. Motion artifact must be minimized and the temperature of the muscle should be held constant because varying temperatures can affect readings (316). These requirements and specifications make these procedures complex and challenging to perform in veterinary practice.
Endolumenal Functional Lumen Imaging Probe (EndoFLIP)
Endolumenal functional lumen imaging probe (EndoFLIP) employs high-resolution impedance planimetry to analyze the cross-sectional area and distensibility of the esophagus and EGJ (328, 329). The EndoFLIP balloon catheter has 16 pairs of impedance electrodes and a single pressure sensor at the distal end (Figure 11A). The balloon portion of the catheter can be volumetrically distended to measure cross-sectional area and pressure at a given location. If that location is centered on the LES, an esophagogastric junction distensibility index (EGJ-DI) can be calculated by dividing the narrowest cross-sectional area by the intra-balloon pressure (330, 331). FLIP 1.0 measures EGJ-DI, but second generation FLIP 2.0 also evaluates esophageal motility with pressure topography. The distension of the balloon catheter triggers secondary peristalsis. The peristaltic contractions that occur can be classified into four different patterns: normal repetitive anterograde contractions, abnormal repetitive retrograde contractions, absent contractility, or other/diminished contractions (332, 333).
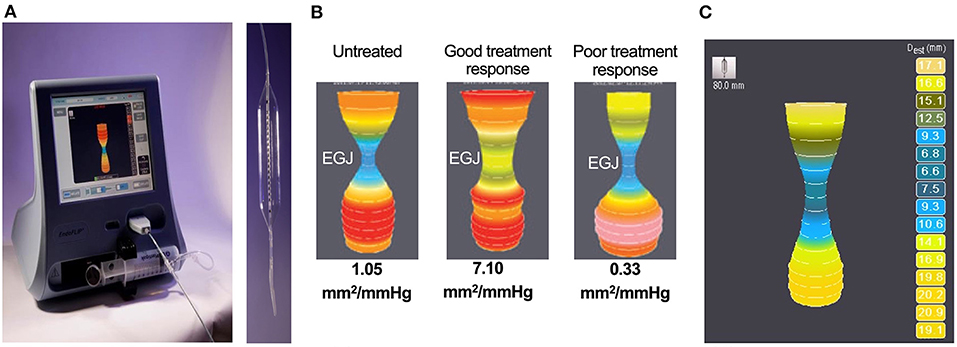
Figure 11. Endoluminal Functional Lumen Imaging Probe (EndoFLIP). (A) Image of the Endoluminal Functional Lumen Imaging Probe (EndoFLIP) machine and catheter with a soft balloon at the distal end. The EndoFLIP system uses impedance planimetry to map out the geometry of cross-sectional areas of the esophagus and esophagogastric junction (EGJ). (B) Intra-operative EndoFLIP images assessing the EGJ in human patients with achalasia pre and post Heller myotomy. The left image shows a patient pre-treatment with a narrowed EGJ. The middle image shows a patient with a good response to myotomy with an improved EGJ diameter. The right image shows a patient with a poor response to myotomy given the persistently narrowed EGJ. (C) Hourglass shape image generated by the EndoFLIP balloon catheter spanning the EGJ in a brachycephalic dog with a history of regurgitation. The numbers on the right indicate the diameter (in millimeters) read out at each 1 cm mark along the length of the 8 cm balloon.
Indications
EndoFLIP has numerous indications in humans, but the primary use is to evaluate distensibility of the EGJ for pathology. EndoFLIP can complement or further support HRM and esophagography, particularly for patients in whom results are mismatched or equivocal (333, 334). An abnormally low EGJ-DI is expected in human patients with EGJ outflow obstruction or achalasia as compared to an elevated EGJ-DI in patients with GERD (331, 335). However, FLIP can also be used intra-operatively or post-operatively in achalasia and GERD patients to tailor surgical procedures. Real-time intra-operative data can guide surgeons in adjusting the extent of the myotomy or tightness of the fundoplication wrap (Figure 11B). Post-operative DI can then be compared to pre-op and intra-operative data to assess surgical outcome and predict clinical outcome (218–221). FLIP can also be used to measure stricture dimensions and guide balloon dilation in patients (336). This is particularly helpful for strictures in the pharyngoesophageal region because this area can be difficult to visualize endoscopically (337). In human patients with eosinophilic esophagitis, FLIP can monitor disease activity and track fibrotic remodeling that can occur with chronic inflammation (338). A less common indication of FLIP is to diagnose hiatal herniation by identifying a double-sphincter image caused by the separation of the LES from the crural diaphragm with the hiatal hernia in between (339). EndoFLIP could theoretically be used for the same indications in dogs; however, only EndoFLIP 1.0 has been evaluated in dogs to date in brachycephalic breeds following hiatal hernia surgery (Figure 11C) (43, 168).
Procedure
The EndoFLIP balloon catheter is placed transorally under sedation or light anesthesia. The catheter is positioned with a couple sensors in the stomach and the mechanical pump fills the balloon to a volume of 20 mL with an electrolyte solution of known conductance. At this point, the EGJ should be visualized as a narrowing of the hourglass shape on the image display. After 15–30 s, additional 10 mL aliquots of volume are instilled with wait periods of 30–60 s between until the balloon is distended to the recommended volume (70 mL). The volumetric distension should trigger secondary peristalsis for the contractile pattern to be observed with EndoFLIP 2.0. EGJ-DI can also be assessed once the volume is at least 60 mL and pressure is at least 15 mmHg. After all measurements are obtained, the balloon is deflated and the catheter is removed (329).
Challenges and Limitations
Although EndoFLIP is an attractive technological modality, there are a few barriers to entry in veterinary medicine. Firstly, the cost of EndoFLIP 2.0 equipment costs ~$70,000, excluding the cost of the single-use catheters ($350 each) (329). In the study of brachycephalics undergoing open hiatal hernia surgery, significant changes to EGJ geometry and DI were not found. Furthermore, the characteristic double-sphincter view indicative of a hiatal hernia was not observed in any of the dogs pre-operatively (43). Brachycephalic breeds most commonly have type I hiatal herniation, which is intermittent and dynamic (43, 168). Thus, measuring EGJ-DI at static, set volumes and time-points may not be adequate to detect sliding herniation. The mechanics of the EGJ are also incredibly complex, involving the hiatus, phrenoesophageal ligament, crural diaphragm, angle of His, and the LES (36). By obtaining measurements intraluminally, EndoFLIP may not be capturing all the relevant exatraluminal components and pathophysiology. It is also unclear whether distension of the EndoFLIP 2.0 balloon will stimulate the same contractile patterns in dogs because the musculature of the distal esophagus is different from that in humans (35). Another potential drawback to EndoFLIP is the patient must be anesthetized for the procedure, which alters LES pressure profiles and puts patients at heightened risk for aspiration pneumonia and GER. Additionally, variables including body position (244), obesity (340), and brachycephalic conformation (341) can alter the anatomy and mechanics of the EGJ junction. Finally, a logistical hurdle may be providing adequate training to personnel to ensure that EndoFLIP is conducted consistently and interpreted properly.
Future Directions in Veterinary Medicine
If the safety and feasibility of EndoFLIP is proven in dogs, the technology may be useful to characterize EGJ outflow obstruction, esophageal achalasia, or GERD disorders in dogs. It is also possible that EndoFLIP could document hiatal herniation in brachycephalic breeds with a higher intra-balloon pressure, a different anesthetic protocol, or the newer EndoFLIP 2.0 module. EndoFLIP could also be used in the operating room to assess GEJ distensibility and predict patient outcomes following fundoplication. EndoFLIP could potentially be used to evaluate dogs with cricopharyngeal disease, but first requires further study of the UES in humans. Furthermore, a study in healthy dogs showed greater variability in UES measurements and significant effects of body position (55). Other potential confounding variables such as patient size and body condition may also significantly affect measurements.
Models for Translational Research and Medicine
A variety of animal models (rodent, canine, feline, opossum, porcine, and non-human primate) have been utilized to study human esophageal disorders, particularly GERD and the progression to Barrett's esophagus and esophageal adenocarcinoma (342, 343). While there are many advantages to using animal models, there are limitations as well. Rodent models are widely available, ideal for laboratory maintenance, and suitable for genetic modification, but are different to humans in various ways. Rodents have keratinized esophageal epithelium, lack submucosal glands and a squamocolumnar EGJ, and do not experience spontaneous reflux (343, 344). Furthermore, their esophageal musculature differs to that in humans and other animal species. Both the rodent and canine esophagus (35) consist of primarily striated muscle whereas cats (345), opossums (346), pigs (347), and non-human primates (348) have both striated and smooth muscle, as found in humans (349). Additionally, rodents and most animal species except for non-human primates, rarely develop Barrett's esophagus secondary to GER (350). Thus, surgical procedures (351–356) or intraesophageal infusions (357, 358) are required to induce reflux and esophageal injury, which raises concerns and questions regarding the ethics and utility of these representative models.
Nevertheless, due to the homology at the EGJ and shared esophageal physiology between humans and canines, there is opportunity for translational research. Many different canine models of GER already exist that have helped in understanding the pathogenesis of Barrett's esophagus in humans (302). Most are uncontrolled, artificial reflux models with surgically configured paraesophageal hiatal hernias, esophago-intestinal anastomoses, or biliary diversions that induce reflux (308, 343, 359). There are sporadic cases of Barrett's esophagus and adenocarcinoma in dogs (360, 361), but the process and progression may take longer than the median lifespan of a dog (362). It is also plausible that Barrett's esophagus is underrecognized because endoscopic esophageal biopsies are rarely obtained in dogs. Although Barrett's esophagus is infrequent in dogs, spontaneous canine models of GER in brachycephalics with hiatal herniation do occur. Many of these dogs also have BOAS making them optimal models of aerodigestive disease to study the effects of sleep apnea syndrome (363), evaluate the relationships of intrathoracic pressure alterations and reflux (364), and interrogate the response to corrective airway surgery (168, 365, 366). The role of acid and airway reflux in aspiration pneumonia (367), laryngeal dysfunction or spasm (368, 369), chronic cough and bronchoconstrictive airway disease (370) could also be studied in dogs and related back to in humans (371).
Canine models of GER also enable pilot testing of novel diagnostic and therapeutic approaches. Procedures such as CT (341), nuclear scintigraphy (289), or acoustic interrogation devices have been used to detect GER in dogs (372). Nuclear scintigraphy can also be used to detect silent, post-prandial, and extraesophageal reflux (289). Measurement of biomarkers like gastric pepsin in saliva (373) or bile acids in airway samples could confirm extraesophageal reflux and microaspiration (370). However, pepsin may not be a useful biomarker in dogs because concentrations are very low in canine gastric fluid. Furthermore, pepsin has not been detected in oropharyngeal swabs from dogs with a known history of vomiting or regurgitation (374). Bioacoustic recordings of respiratory sounds can be performed and synchronized with reflux testing to establish cough-reflux associations in aerodigestive patients (375). In addition to testing innovative diagnostic methods in dogs, novel treatments such as baclofen to reduce tLESr (376), injectable bulking agents for the LES (377), electrical microstimulation of the LES (378), extracellular matrix hydrogel to mitigate Barrett's esophagus (379), tissue engineering with autologous cells and/or bioscaffolds to prevent esophageal strictures (380) and repair esophageal defects (381–384), and surgical procedures, such as endoscopic fundoplication (385, 386), have already been trialed in canine models and are paving the way for usage in human patients.
Apart from GER models, there are spontaneous and induced canine models for cricopharyngeus muscle dysfunction and esophageal achalasia as well. Canine breeds such as the golden retrievers, miniature dachshunds, Maltese, and spaniels are highly predisposed to cricopharyngeus muscle dysfunction (92–94). Genomic analysis in affected breeds could elucidate hereditary mutations that develop genetic models of disease. For example, golden retrievers, Rottweilers, German shorthaired pointers, Welsh corgis, Cavalier King Charles spaniels, Cockers spaniels, Tibetan terriers, and Labrador retrievers are genetically predisposed to the X-linked recessive disorder, muscular dystrophy, due to a heritable mutation that depletes muscular dystrophin (Table 1) (387). Studying these canine models has helped isolate the culprit mutation and implement gene editing technology to cure the disease (388). Similar genomic analyses could be fruitful in patients with esophageal achalasia and secondary megaesophagus. Further study in dogs could also uncover environmental factors associated with esophageal achalasia and/or secondary megaesophagus, such as toxins (aflatoxin) (389) or dietary ingredients (390). Esophageal achalasia can even be experimentally induced in canines by injecting a surfactant, benzyldimethyltetradecylammonium chloride into the LES. Novel interventions or procedures for achalasia such as retrievable self-expanding cardia stents or esoFLIP (endoFLIP with balloon dilation) for esophageal achalasia (391) or cricopharyngeus per oral endoscopic myectomy (c-POEM) for cricopharyngeus achalasia can then be tested (392).
Diagnostics such as swallowing fluoroscopy, HRM, pH/impedance, and endoFLIP will be valuable in accurately assessing the therapeutic effects of such interventions. Incorporating these diagnostic tools into translational research and veterinary practice will require interdisciplinary collaboration between veterinary clinicians, human health care professionals, researchers, and biomedical device companies.
Conclusion
Difficulty swallowing is a highly prevalent symptom that significantly affects quality of life and shortens life expectancy in both humans and dogs. Assessment of oropharyngeal and esophageal function is critical to appropriately diagnose and manage swallowing disorders. Although not discussed in detail in this manuscript, careful consideration of delayed gastric emptying in precipitating GER is important. Due to the shared features in pharyngeal and esophageal anatomy, physiology, and pathology between humans and canines, diagnostic tests such as swallowing fluoroscopy, FEES, HRM, pH/impedance, and endoFLIP can be utilized in both species. However, there are notable challenges and limitations to performing and interpreting these tests in dogs. Although further research of these modalities in canines is necessary, there is significant potential for translational research and clinical application in veterinary and human medicine.
Author Contributions
TU primarily authored the manuscript and completed multiple revisions of the draft. SM helped with the outline of the manuscript, assisted with revisions of the manuscript, and provided several figures. PB edited the manuscript, provided guidance on the outline of the manuscript, and provided several figures. JC and JP edited the manuscript and provided several figures. All authors reviewed and approved the final draft of the manuscript.
Funding
The authors received Open Access Funds from UC Davis to cover a portion of publication costs.
Conflict of Interest
The authors declare that the research was conducted in the absence of any commercial or financial relationships that could be construed as a potential conflict of interest.
Publisher's Note
All claims expressed in this article are solely those of the authors and do not necessarily represent those of their affiliated organizations, or those of the publisher, the editors and the reviewers. Any product that may be evaluated in this article, or claim that may be made by its manufacturer, is not guaranteed or endorsed by the publisher.
Acknowledgments
TU appreciates Nestlé Purina for their support of her current UC Davis Purina Gastroenterology and Hepatology Fellowship.
Supplementary Material
The Supplementary Material for this article can be found online at: https://www.frontiersin.org/articles/10.3389/fvets.2022.889331/full#supplementary-material
References
1. Peery AF, Crockett SD, Murphy CC, Lund JL, Dellon ES, Williams JL, et al. Burden and cost of gastrointestinal, liver, and pancreatic diseases in the united states: update 2018. Gastroenterology. (2019) 156:254–72.e11. doi: 10.1053/j.gastro.2018.08.063
2. Pollard RE. Imaging evaluation of dogs and cats with dysphagia. ISRN Vet Sci. (2012) 2012:238505. doi: 10.5402/2012/238505
3. Pollard RE, Marks SL, Cheney DM, Bonadio CM. Diagnostic outcome of contrast videofluoroscopic swallowing studies in 216 dysphagic dogs. Vet Radiol Ultrasound. (2017) 58:373–380. doi: 10.1111/vru.12493
4. Ueshima J, Momosaki R, Shimizu A, Motokawa K, Sonoi M, Shirai Y, et al. Nutritional assessment in adult patients with dysphagia: a scoping review. Nutrients. (2021) 13:778. doi: 10.3390/nu13030778
5. Reber E, Gomes F, Dahn IA, Vasiloglou MF, Stanga Z. Management of dehydration in patients suffering swallowing difficulties. J Clin Med. (2019) 8:1923. doi: 10.3390/jcm8111923
6. Lo WL, Leu HB, Yang MC, Wang DH, Hsu ML. Dysphagia and risk of aspiration pneumonia: a nonrandomized, pair-matched cohort study. J Dent Sci. (2019) 14:241–247. doi: 10.1016/j.jds.2019.01.005
7. McBrearty AR, Ramsey IK, Courcier EA, Mellor DJ, Bell R. Clinical factors associated with death before discharge and overall survival time in dogs with generalized megaesophagus. J Am Vet Med Assoc. (2011) 238:1622–8. doi: 10.2460/javma.238.12.1622
8. Gonçalves BFdT, Bastilha GR, Costa CdC, Mancopes R. Utilização de protocolos de qualidade de vida em disfagia: revisão de literatura. Revista CEFAC. (2015) 17:1333–40. doi: 10.1590/1982-0216201517418014
9. Adkins C, Takakura W, Spiegel BMR, Lu M, Vera-Llonch M, Williams J, et al. Prevalence and characteristics of dysphagia based on a population-based survey. Clin Gastroenterol Hepatol. (2020) 18:1970–9.e2. doi: 10.1016/j.cgh.2019.10.029
10. Madhavan A, LaGorio LA, Crary MA, Dahl WJ, Carnaby GD. Prevalence of and risk factors for dysphagia in the community dwelling elderly: a systematic review. J Nutr Health Aging. (2016) 20:806–15. doi: 10.1007/s12603-016-0712-3
11. McCarty EB, Chao TN. Dysphagia and swallowing disorders. Med Clin North Am. (2021) 105:939–54. doi: 10.1016/j.mcna.2021.05.013
12. Drossman DA. Functional gastrointestinal disorders: history, pathophysiology, clinical features, and rome IV. Gastroenterology. (2016) 150:1262–79.e2. doi: 10.1053/j.gastro.2016.02.032
13. Bashashati M, Hejazi RA. Overlap between gastric and esophageal motility disorders: a contractual arrangement? Digest Dis Sci. (2018) 63:3164–6. doi: 10.1007/s10620-018-5258-2
14. Di Ciaula A, Covelli M, Berardino M, Wang DQH, Lapadula G, Palasciano G, et al. Gastrointestinal symptoms and motility disorders in patients with systemic scleroderma. BMC Gastroenterol. (2008) 8:7. doi: 10.1186/1471-230X-8-7
15. Wegener M, Adamek RJ, Wedmann B, Jergas M, Altmeyer P. Gastrointestinal transit through esophagus, stomach, small and large intestine in patients with progressive systemic sclerosis. Dig Dis Sci. (1994) 39:2209–15. doi: 10.1007/BF02090373
16. Ntoumazios SK, Voulgari PV, Potsis K, Koutis E, Tsifetaki N, Assimakopoulos DA. Esophageal involvement in scleroderma: gastroesophageal reflux, the common problem. Semin Arthritis Rheum. (2006) 36:173–81. doi: 10.1016/j.semarthrit.2006.08.002
17. Krausz Y, Maayan C, Faber J, Marciano R, Mogle P, Wynchank S. Scintigraphic evaluation of esophageal transit and gastric emptying in familial dysautonomia. Eur J Radiol. (1994) 18:52–6. doi: 10.1016/0720-048X(94)90367-0
18. Clarke KE, Sorrell S, Breheny C, Jepson R, Adamantos S, Milne EM, et al. Dysautonomia in 53 cats and dogs: retrospective review of clinical data and outcome. Vet Record. (2020) 187:e118. doi: 10.1136/vr.105258
19. Takeuchi S, Tamate S, Nakahira M, Kadowaki H. Esophagitis in infants with hypertrophic pyloric stenosis: a source of hematemesis. J Pediatr Surg. (1993) 28:59–62. doi: 10.1016/S0022-3468(05)80356-2
20. Jehangir A, Parkman HP. Reflux symptoms in gastroparesis: correlation with gastroparesis symptoms, gastric emptying, and esophageal function testing. J Clin Gastroenterol. (2020) 54:428–38. doi: 10.1097/MCG.0000000000001190
21. Husnik R, Gaschen F. Gastric motility disorders in dogs and cats. Vet Clin North Am Small Anim Pract. (2021) 51:43–59. doi: 10.1016/j.cvsm.2020.09.002
22. McCallum RW, Berkowitz DM, Lerner E. Gastric emptying in patients with gastroesophageal reflux. Gastroenterology. (1981) 80:285–91. doi: 10.1016/0016-5085(81)90716-2
23. Gourcerol G, Benanni Y, Boueyre E, Leroi AM, Ducrotte P. Influence of gastric emptying on gastro-esophageal reflux: a combined ph-impedance study. Neurogastroenterol Motil. (2013) 25:800–e634. doi: 10.1111/nmo.12181
24. Lester NV, Roberts GD, Newell SM, Graham JP, Hartless CS. Assessment of barium impregnated polyethylene spheres (BIPS®) as a measure of solid-phase gastric emptying in normal dogs-comparison to scintigraphy. Vet Radiol Ultrasound. (1999) 40:465–71. doi: 10.1111/j.1740-8261.1999.tb00376.x
25. Gardella R, Silver PJ, Shahsavari D, Maurer AH, Parkman HP. Gastric half emptying time (t(½)) for 4-h gastric emptying scintigraphy simplifies reporting but reduces detection of gastroparesis. Neurogastroenterol Motil. (2021) 34:e14261. doi: 10.1111/nmo.14261
26. Shi J, Shen H, Gao Q, Mulmi Shrestha S, Tan J, Lu T, et al. Evaluation of gastric emptying in patients with gastroparesis by three-dimensional ultrasound. Ann Transl Med. (2021) 9:1343. doi: 10.21037/atm-21-3972
27. Sanderson JJ, Boysen SR, McMurray JM, Lee A, Stillion JR. The effect of fasting on gastrointestinal motility in healthy dogs as assessed by sonography. J Vet Emerg Crit Care. (2017) 27:645–50. doi: 10.1111/vec.12673
28. Lee AA, Rao S, Nguyen LA, Moshiree B, Sarosiek I, Schulman MI, et al. Validation of diagnostic and performance characteristics of the wireless motility capsule in patients with suspected gastroparesis. Clin Gastroenterol Hepatol. (2019) 17:1770–9.e2. doi: 10.1016/j.cgh.2018.11.063
29. Boillat CS, Gaschen FP, Gaschen L, Stout RW, Hosgood GL. Variability associated with repeated measurements of gastrointestinal tract motility in dogs obtained by use of a wireless motility capsule system and scintigraphy. Am J Vet Res. (2010) 71:903–8. doi: 10.2460/ajvr.71.8.903
30. Watrous BJ. Clinical presentation and diagnosis of dysphagia. Vet Clin North Am Small Anim Pract. (1983) 13:437–59. doi: 10.1016/S0195-5616(83)50052-1
31. Manabe N, Tsutsui H, Kusunoki H, Hata J, Haruma K. Pathophysiology and treatment of patients with globus sensation–from the viewpoint of esophageal motility dysfunction. J Smooth Muscle Res. (2014) 50:66–77. doi: 10.1540/jsmr.50.66
32. Richter JE, Rubenstein JH. Presentation and epidemiology of gastroesophageal reflux disease. Gastroenterology. (2018) 154:267–76. doi: 10.1053/j.gastro.2017.07.045
33. Suzuki H. The application of the rome IV criteria to functional esophagogastroduodenal disorders in asia. J Neurogastroenterol Motil. (2017) 23:325–33. doi: 10.5056/jnm17018
34. Gelberg HB. Comparative anatomy, physiology, and mechanisms of disease production of the esophagus, stomach, and small intestine. Toxicol Pathol. (2014) 42:54–66. doi: 10.1177/0192623313518113
35. Watson AG. Structure of the canine oesophagus. N Z Vet J. (1973) 21:195–200. doi: 10.1080/00480169.1973.34106
36. Mittal R, Vaezi MF. Esophageal motility disorders and gastroesophageal reflux disease. N Engl J Med. (2020) 383:1961–72. doi: 10.1056/NEJMra2000328
37. Patti MG, Gantert W, Way LW. Surgery of the esophagus. Surgical Clinics of North America. (1997) 77:959–70. doi: 10.1016/S0039-6109(05)70600-9
38. Dewan K, Santa Maria C, Noel J. Cricopharyngeal achalasia: management and associated outcomes-a scoping review. Otolaryngol Head Neck Surg. (2020) 163:1109–13. doi: 10.1177/0194599820931470
39. Warnock JJ, Marks SL, Pollard R, Kyles AE, Davidson A. Surgical management of cricopharyngeal dysphagia in dogs: 14 cases (1989-2001). J Am Vet Med Assoc. (2003) 223:1462–8. doi: 10.2460/javma.2003.223.1462
40. Vaezi MF, Pandolfino JE, Yadlapati RH, Greer KB, Kavitt RT. Acg clinical guidelines: diagnosis and management of achalasia. Am J Gastroenterol. (2020) 115:1393–11. doi: 10.14309/ajg.0000000000000731
41. Grobman ME, Schachtel J, Gyawali CP, Lever TE, Reinero CR. Videofluoroscopic swallow study features of lower esophageal sphincter achalasia-like syndrome in dogs. J Vet Intern Med. (2019) 33:1954–63. doi: 10.1111/jvim.15578
42. Reeve EJ, Sutton D, Friend EJ, Warren-Smith CMR. Documenting the prevalence of hiatal hernia and oesophageal abnormalities in brachycephalic dogs using fluoroscopy. J Small Anim Pract. (2017) 58:703–8. doi: 10.1111/jsap.12734
43. Mayhew PD, Marks SL, Pollard R, Culp WTN, Kass PH. Prospective evaluation of surgical management of sliding hiatal hernia and gastroesophageal reflux in dogs. Vet Surg. (2017) 46:1098–109. doi: 10.1111/vsu.12684
44. Siegal SR, Dolan JP, Hunter JG. Modern diagnosis and treatment of hiatal hernias. Langenbecks Arch Surg. (2017) 402:1145–51. doi: 10.1007/s00423-017-1606-5
45. Kook PH, Kempf J, Ruetten M, Reusch CE. Wireless ambulatory esophageal ph monitoring in dogs with clinical signs interpreted as gastroesophageal reflux. J Vet Intern Med. (2014) 28:1716–23. doi: 10.1111/jvim.12461
46. Münster M, Kook P, Araujo R, Hörauf A, Vieth M. Determination of hyperregeneratory esophagopathy in dogs with clinical signs attributable to esophageal disease. Tierarztl Prax. (2015) 43:147–55. doi: 10.15654/TPK-140765
47. Kook PH. Esophagitis in cats and dogs. Vet Clin North Am Small Anim Pract. (2021) 51:1–15. doi: 10.1016/j.cvsm.2020.08.003
48. Mazzei MJ, Bissett SA, Murphy KM, Hunter S, Neel JA. Eosinophilic esophagitis in a dog. J Am Vet Med Assoc. (2009) 235:61–5. doi: 10.2460/javma.235.1.61
49. Leib MS, Dinnel H, Ward DL, Reimer ME, Towell TL, Monroe WE. Endoscopic balloon dilation of benign esophageal strictures in dogs and cats. J Vet Intern Med. (2001) 15:547–52. doi: 10.1111/j.1939-1676.2001.tb01589.x
50. Eivers C, Chicon Rueda R, Liuti T, Salavati Schmitz S. Retrospective analysis of esophageal imaging features in brachycephalic versus non-brachycephalic dogs based on videofluoroscopic swallowing studies. J Vet Intern Med. (2019) 33:1740–6. doi: 10.1111/jvim.15547
51. Bexfield NH, Watson PJ, Herrtage ME. Esophageal dysmotility in young dogs. J Vet Intern Med. (2006) 20:1314–8. doi: 10.1111/j.1939-1676.2006.tb00744.x
52. Pollard RE. Videofluoroscopic evaluation of the pharynx and upper esophageal sphincter in the dog: a systematic review of the literature. Front Vet Sci. (2019) 6:117. doi: 10.3389/fvets.2019.00117
53. Ullal TV, Kass PH, Conklin JL, Belafsky PC, Marks SL. High-resolution manometric evaluation of the effects of cisapride on the esophagus during administration of solid and liquid boluses in awake healthy dogs. Am J Vet Res. (2016) 77:818–27. doi: 10.2460/ajvr.77.8.818
54. Zacuto AC, Marks SL, Osborn J, Douthitt KL, Hollingshead KL, Hayashi K, et al. The influence of esomeprazole and cisapride on gastroesophageal reflux during anesthesia in dogs. J Vet Intern Med. (2012) 26:518–25. doi: 10.1111/j.1939-1676.2012.00929.x
55. Pitt KA, Mayhew PD, Barter L, Pollard R, Kass PH, Marks SL. Consistency and effect of body position change on measurement of upper and lower esophageal sphincter geometry using impedance planimetry in a canine model. Dis Esophagus. (2017) 30:1–7. doi: 10.1093/dote/dow031
56. Gyawali CP, de Bortoli N, Clarke J, Marinelli C, Tolone S, Roman S, et al. Indications and interpretation of esophageal function testing. Ann N Y Acad Sci. (2018) 1434:239–2. doi: 10.1111/nyas.13709
57. Expert Expert Panel on Gastrointestinal I, Levy AD, Carucci LR, Bartel TB, Cash BD, Chang KJ, et al. Acr appropriateness criteria((r)) dysphagia. J Am Coll Radiol. (2019) 16:S104–15. doi: 10.1016/j.jacr.2019.02.007
58. Kahrilas PJ, Bredenoord AJ, Carlson DA, Pandolfino JE. Advances in management of esophageal motility disorders. Clin Gastroenterol Hepatol. (2018) 16:1692–700. doi: 10.1016/j.cgh.2018.04.026
59. Venker-van-Haagen A. Esophagus. In: Washabau RJ, Day MJ, editors. Canine Feline Gastroenterology. St. Louis, MO: W.B. Saunders (2013). p. 570–605. doi: 10.1016/B978-1-4160-3661-6.00055-9
60. Sivarao DV, Goyal RK. Functional anatomy and physiology of the upper esophageal sphincter. Am J Med. (2000) 108:27–37. doi: 10.1016/S0002-9343(99)00337-X
61. Blevins CH, Iyer PG, Vela MF, Katzka DA. The esophageal epithelial barrier in health and disease. Clin Gastroenterol Hepatol. (2018) 16:608–17. doi: 10.1016/j.cgh.2017.06.035
62. Sasegbon A, Hamdy S. The anatomy and physiology of normal and abnormal swallowing in oropharyngeal dysphagia. Neurogastroenterol Motil. (2017) 29. doi: 10.1111/nmo.13100
63. Matsuo K, Palmer JB. Anatomy and physiology of feeding and swallowing: normal and abnormal. Phys Med Rehabil Clin N Am. (2008) 19:691–707, vii. doi: 10.1016/j.pmr.2008.06.001
64. Sanmiguel CP, Ito Y, Hagiike M, Conklin JL, Lalezari D, Soffer EE. The effect of eating on lower esophageal sphincter electrical activity. Am J Physiol Gastrointest Liver Physiol. (2009) 296:G793–7. doi: 10.1152/ajpgi.90369.2008
65. Liu TT, Yi CH, Lei WY, Hung XS, Yu HC, Chen CL. Influence of repeated infusion of capsaicin-contained red pepper sauce on esophageal secondary peristalsis in humans. Neurogastroenterol Motil. (2014) 26:1487–93. doi: 10.1111/nmo.12414
66. Goyal RK, Chaudhury A. Physiology of normal esophageal motility. J Clin Gastroenterol. (2008) 42:610–9. doi: 10.1097/MCG.0b013e31816b444d
67. Sifrim D, Jafari J. Deglutitive inhibition, latency between swallow and esophageal contractions and primary esophageal motor disorders. J Neurogastroenterol Motil. (2012) 18:6–12. doi: 10.5056/jnm.2012.18.1.6
68. Pauwels A, Altan E, Tack J. The gastric accommodation response to meal intake determines the occurrence of transient lower esophageal sphincter relaxations and reflux events in patients with gastro-esophageal reflux disease. Neurogastroenterol Motil. (2014) 26:581–8. doi: 10.1111/nmo.12305
69. Patrikios J, Martin CJ, Dent J. Relationship of transient lower esophageal sphincter relaxation tai post randial gastroesophageal reflex and belching in dogs. Gastroenterology. (1986) 90:545–51. doi: 10.1016/0016-5085(86)91107-8
70. Rofes L, Arreola V, Romea M, Palomera E, Almirall J, Cabré M, et al. Pathophysiology of oropharyngeal dysphagia in the frail elderly. Neurogastroenterol Motil. (2010) 22:851–8.e230. doi: 10.1111/j.1365-2982.2010.01521.x
71. Kern M, Bardan E, Arndorfer R, Hofmann C, Ren J, Shaker R. Comparison of upper esophageal sphincter opening in healthy asymptomatic young and elderly volunteers. Ann Otol Rhinol Laryngol. (1999) 108:982–9. doi: 10.1177/000348949910801010
72. Williams RB, Wallace KL, Ali GN, Cook IJ. Biomechanics of failed deglutitive upper esophageal sphincter relaxation in neurogenic dysphagia. Am J Physiol Gastrointest Liver Physiol. (2002) 283:G16–26. doi: 10.1152/ajpgi.00189.2001
73. Lee T, Park JH, Sohn C, Yoon KJ, Lee YT, Park JH, et al. Failed deglutitive upper esophageal sphincter relaxation is a risk factor for aspiration in stroke patients with oropharyngeal dysphagia. J Neurogastroenterol Motil. (2017) 23:34–40. doi: 10.5056/jnm16028
74. Suh MK, Kim H, Na DL. Dysphagia in patients with dementia: Alzheimer versus vascular. Alzheimer Dis Assoc Disord. (2009) 23:178–84. doi: 10.1097/WAD.0b013e318192a539
75. Ali GN, Wallace KL, Schwartz R, DeCarle DJ, Zagami AS, Cook IJ. Mechanisms of oral-pharyngeal dysphagia in patients with parkinson's disease. Gastroenterology. (1996) 110:383–92. doi: 10.1053/gast.1996.v110.pm8566584
76. Rösler A, Pfeil S, Lessmann H, Höder J, Befahr A, von Renteln-Kruse W. Dysphagia in dementia: influence of dementia severity and food texture on the prevalence of aspiration and latency to swallow in hospitalized geriatric patients. J Am Med Dir Assoc. (2015) 16:697–701. doi: 10.1016/j.jamda.2015.03.020
77. Shelton GD, Willard MD, Cardinet GH 3rd, Lindstrom J. Acquired myasthenia gravis. Selective involvement of esophageal, pharyngeal, and facial muscles. J Vet Intern Med. (1990) 4:281–4. doi: 10.1111/j.1939-1676.1990.tb03124.x
78. Peeters ME, Venker-van Haagen AJ, Goedegebuure SA, Wolvekamp WT. Dysphagia in bouviers associated with muscular dystrophy; evaluation of 24 cases. Vet Q. (1991) 13:65–73. doi: 10.1080/01652176.1991.9694287
79. Ryckman LR, Krahwinkel DJ, Sims MH, Donnell RL, Moore PF, Shelton GD. Dysphagia as the primary clinical abnormality in two dogs with inflammatory myopathy. J Am Vet Med Assoc. (2005) 226:1519–23. doi: 10.2460/javma.2005.226.1519
80. Braund KG. Encephalitis and meningitis. Vet Clin North Am Small Anim Pract. (1980) 10:31–56. doi: 10.1016/S0195-5616(80)50002-1
81. Pollard RE, Marks SL, Davidson A, Hornof WJ. Quantitative videofluoroscopic evaluation of pharyngeal function in the dog. Vet Radiol Ultrasound. (2000) 41:409–12. doi: 10.1111/j.1740-8261.2000.tb01862.x
82. Marks SL. Chapter 273: Diseases of the Pharynx and Esophagus. In: Feldman EC, Côté E, Ettinger SJ, editors. Textbook of Veterinary Internal Medicine: Diseases of the Dog and the Cat. 8th ed. St. Louis, MO: Elsevier, Inc. (2017). p. 8501–76.
83. Broux O, Clercx C, Etienne AL, Busoni V, Claeys S, Hamaide A, et al. Effects of manipulations to detect sliding hiatal hernia in dogs with brachycephalic airway obstructive syndrome. Vet Surg. (2018) 47:243–51. doi: 10.1111/vsu.12735
84. Galatos AD, Raptopoulos D. Gastro-oesophageal reflux during anaesthesia in the dog: the effect of age, positioning and type of surgical procedure. Vet Rec. (1995) 137:513–6. doi: 10.1136/vr.137.20.513
85. Galatos AD, Raptopoulos D. Gastro-oesophageal reflux during anaesthesia in the dog: the effect of preoperative fasting and premedication. Vet Rec. (1995) 137:479–83. doi: 10.1136/vr.137.19.479
86. Wilson DV, Walshaw R. Postanesthetic esophageal dysfunction in 13 dogs. J Am Anim Hosp Assoc. (2004) 40:455–60. doi: 10.5326/0400455
87. Backer CL, Mongé MC, Popescu AR, Eltayeb OM, Rastatter JC, Rigsby CK. Vascular rings. Semin Pediatr Surg. (2016) 25:165–75. doi: 10.1053/j.sempedsurg.2016.02.009
88. Burton AG, Talbot CT, Kent MS. Risk factors for death in dogs treated for esophageal foreign body obstruction: a retrospective cohort study of 222 cases (1998-2017). J Vet Intern Med. (2017) 31:1686–90. doi: 10.1111/jvim.14849
90. Hammad A, Lu VF, Dahiya DS, Kichloo A, Tuma F. Treatment challenges of sigmoid-shaped esophagus and severe achalasia. Ann Med Surg. (2021) 61:30–34. doi: 10.1016/j.amsu.2020.11.077
91. Yadlapati R, Kahrilas PJ, Fox MR, Bredenoord AJ, Prakash Gyawali C, Roman S, et al. Esophageal motility disorders on high-resolution manometry: Chicago classification version 4.0((c)). Neurogastroenterol Motil. (2021) 33:e14058. doi: 10.1111/nmo.14058
92. Davidson AP, Pollard RE, Bannasch DL, Marks SL, Hornof WJ, Famula TR. Inheritance of cricopharyngeal dysfunction in golden retrievers. Am J Vet Res. (2004) 65:344–9. doi: 10.2460/ajvr.2004.65.344
93. Heilman RM, Marks SL. Cricopharyngeal achalasia. In: Mott J, Morrison JA, editors. Blackwell's Five-Minute Veterinary Consult Clinical Companion: Small Animal Gastrointestinal Diseases. 1st ed. Ames, IA: John Wiley & Sons (2019). p. 187–94. doi: 10.1002/9781119376293.ch27
94. Weaver AD. Cricopharyngeal achalasia in cocker spaniels. J Small Anim Prac. (1983) 24:209–14. doi: 10.1111/j.1748-5827.1983.tb00433.x
95. Elliott RC. An anatomical and clinical review of cricopharyngeal achalasia in the dog. J S Afr Vet Assoc. (2010) 81:75–9. doi: 10.4102/jsava.v81i2.108
96. Huoh KC, Messner AH. Cricopharyngeal achalasia in children: indications for treatment and management options. Curr Opin Otolaryngol Head Neck Surg. (2013) 21:576–80. doi: 10.1097/01.moo.0000436789.29814.62
97. Nativ-Zeltzer N, Rameau A, Kuhn MA, Kaufman M, Belafsky PC. The relationship between hiatal hernia and cricopharyngeus muscle dysfunction. Dysphagia. (2019) 34:391–6. doi: 10.1007/s00455-018-9950-3
98. Kuhn MA, Belafsky PC. Management of cricopharyngeus muscle dysfunction. Otolaryngol Clin North Am. (2013) 46:1087–99. doi: 10.1016/j.otc.2013.08.006
99. Chambers JK, Saito T, Fukushima K, Kakuta S, Nakayama J, Ohno K, et al. Adenocarcinoma of barrett's esophagus in a dog. J Toxicol Pathol. (2017) 30:239–43. doi: 10.1293/tox.2017-0009
100. Pazzi P, Kavkovsky A, Shipov A, Segev G, Dvir E. Spirocerca lupi induced oesophageal neoplasia: predictors of surgical outcome. Vet Parasitol. (2018) 250:71–7. doi: 10.1016/j.vetpar.2017.11.013
101. Rojas A, Dvir E, Farkas R, Sarma K, Borthakur S, Jabbar A, et al. Phylogenetic analysis of spirocerca lupi and spirocerca vulpis reveal high genetic diversity and intra-individual variation. Parasit Vect. (2018) 11:639. doi: 10.1186/s13071-018-3202-0
102. Kidambi T, Toto E, Ho N, Taft T, Hirano I. Temporal trends in the relative prevalence of dysphagia etiologies from 1999-2009. World J Gastroenterol. (2012) 18:4335–41. doi: 10.3748/wjg.v18.i32.4335
103. Hudson LC, Cummings JF. The origins of innervation of the esophagus of the dog. Brain Research. (1985) 326:125–36. doi: 10.1016/0006-8993(85)91391-5
104. Park H, Conklin JL. Neuromuscular control of esophageal peristalsis. Curr Gastroenterol Rep. (1999) 1:186–97. doi: 10.1007/s11894-999-0033-3
105. Andrew BL. The nervous control of the cervical oesophagus of the rat during swallowing. J Physiol. (1956) 134:729–40. doi: 10.1113/jphysiol.1956.sp005679
106. Murray J, Du C, Ledlow A, Bates JN, Conklin JL. Nitric oxide: mediator of nonadrenergic noncholinergic responses of opossum esophageal muscle. Am J Physiol. (1991) 261:G401–6. doi: 10.1152/ajpgi.1991.261.3.G401
107. Yamato S, Spechler SJ, Goyal RK. Role of nitric oxide in esophageal peristalsis in the opossum. Gastroenterology. (1992) 103:197–204. doi: 10.1016/0016-5085(92)91113-I
108. De Giorgio R, Di Simone MP, Stanghellini V, Barbara G, Tonini M, Salvioli B, et al. Esophageal and gastric nitric oxide synthesizing innervation in primary achalasia. Am J Gastroenterol. (1999) 94:2357–62. doi: 10.1111/j.1572-0241.1999.01357.x
109. Gilhus NE, Tzartos S, Evoli A, Palace J, Burns TM, Verschuuren JJGM. Myasthenia gravis. Nat Rev Dis Primers. (2019) 5:30. doi: 10.1038/s41572-019-0079-y
110. Ghosh SK, Janiak P, Schwizer W, Hebbard GS, Brasseur JG. Physiology of the esophageal pressure transition zone: separate contraction waves above and below. Am J Physiol Gastrointest Liver Physiol. (2006) 290:G568–76. doi: 10.1152/ajpgi.00280.2005
111. Ghosh SK, Pandolfino JE, Kwiatek MA, Kahrilas PJ. Oesophageal peristaltic transition zone defects: real but few and far between. Neurogastroenterol Motil. (2008) 20:1283–90. doi: 10.1111/j.1365-2982.2008.01169.x
112. Gorti H, Samo S, Shahnavaz N, Qayed E. Distal esophageal spasm: update on diagnosis and management in the era of high-resolution manometry. World J Clin Cases. (2020) 8:1026–32. doi: 10.12998/wjcc.v8.i6.1026
113. Savarino E, Smout A. The hypercontractile esophagus: still a tough nut to crack. Neurogastroenterol Motil. (2020) 32:e14010. doi: 10.1111/nmo.14010
114. Waterman AE, Hashim MA. Measurement of the length and position of the lower oesophageal sphincter by correlation of external measurements and radiographic estimations in dogs. Vet Rec. (1991) 129:261–4. doi: 10.1136/vr.129.12.261
115. Staller K, Kuo B. Development, anatomy, and physiology of the esophagus. In: Shaker R, Belafsky PC, Postma GN, Easterling C, editors. Principles of Deglutition: A Multidisciplinary Text for Swallowing and Its Disorders. New York, NY: Springer New York. (2013). p. 269–86.
116. O'Neill DG, Pegram C, Crocker P, Brodbelt DC, Church DB, Packer RMA. Unravelling the health status of brachycephalic dogs in the uk using multivariable analysis. Sci Rep. (2020) 10:17251. doi: 10.1038/s41598-020-73088-y
117. Chamanza R, Wright JA. A review of the comparative anatomy, histology, physiology and pathology of the nasal cavity of rats, mice, dogs and non-human primates. Relevance to inhalation toxicology and human health risk assessment. J Compar Pathol. (2015) 153:287–314. doi: 10.1016/j.jcpa.2015.08.009
118. Mansfield LE. Embryonic origins of the relation of gastroesophageal reflux disease and airway disease. Am J Med. (2001) 111:3–7. doi: 10.1016/S0002-9343(01)00846-4
119. Pratschke KM, Fitzpatrick E, Campion D, McAllister H, Bellenger CR. Topography of the gastro-oesophageal junction in the dog revisited: possible clinical implications. Res Vet Sci. (2004) 76:171–7. doi: 10.1016/j.rvsc.2003.12.001
120. Shelton GD, Cardinet III GH, Bandman E. Canine masticatory muscle disorders: a study of 29 cases. Muscle Nerve. (1987) 10:753–66. doi: 10.1002/mus.880100812
121. Mayhew PD, Bush WW, Glass EN. Trigeminal neuropathy in dogs: a retrospective study of 29 cases (1991-2000). J Am Anim Hosp Assoc. (2002) 38:262–70. doi: 10.5326/0380262
122. Haley AC, Platt SR, Kent M, Schatzberg SJ, Durham A, Cochrane S, et al. Breed-specific polymyositis in hungarian vizsla dogs. J Vet Internal Med. (2011) 25:393–7. doi: 10.1111/j.1939-1676.2010.0660.x
123. Bedu AS, Labruyère JJ, Thibaud JL, Barthélémy I, Leperlier D, Saunders JH, et al. Age-related thoracic radiographic changes in golden and labrador retriever muscular dystrophy. Vet Radiol Ultrasound. (2012) 53:492–500. doi: 10.1111/j.1740-8261.2012.01948.x
124. Cooper BJ, Valentine BA, Wilson S, Patterson DF, Concannon PW. Canine muscular dystrophy: confirmation of x-linked inheritance. J Hered. (1988) 79:405–8. doi: 10.1093/oxfordjournals.jhered.a110543
125. Barthélémy I, Calmels N, Weiss RB, Tiret L, Vulin A, Wein N, et al. X-linked muscular dystrophy in a labrador retriever strain: phenotypic and molecular characterisation. Skelet Muscle. (2020) 10:23. doi: 10.1186/s13395-020-00239-0
126. Walmsley GL, Arechavala-Gomeza V, Fernandez-Fuente M, Burke MM, Nagel N, Holder A, et al. A duchenne muscular dystrophy gene hot spot mutation in dystrophin-deficient cavalier king charles spaniels is amenable to exon 51 skipping. PLoS ONE. (2010) 5:e8647. doi: 10.1371/journal.pone.0008647
127. McGreevy JW, Hakim CH, McIntosh MA, Duan D. Animal models of duchenne muscular dystrophy: from basic mechanisms to gene therapy. Dis Model Mech. (2015) 8:195–13. doi: 10.1242/dmm.018424
128. Mickelson JR, Minor KM, Guo LT, Friedenberg SG, Cullen JN, Ciavarella A, et al. Sarcoglycan a mutation in miniature dachshund dogs causes limb-girdle muscular dystrophy 2d. Skelet Muscle. (2021) 11:2. doi: 10.1186/s13395-020-00257-y
129. Ito D, Kitagawa M, Jeffery N, Okada M, Yoshida M, Kobayashi M, et al. Dystrophin-deficient muscular dystrophy in an alaskan malamute. Vet Rec. (2011) 169:127. doi: 10.1136/vr.d2693
130. Giannasi C, Tappin SW, Guo LT, Shelton GD, Palus V. Dystrophin-deficient muscular dystrophy in two lurcher siblings. J Small Anim Pract. (2015) 56:577–80. doi: 10.1111/jsap.12331
131. Cox ML, Evans JM, Davis AG, Guo LT, Levy JR, Starr-Moss AN, et al. Exome sequencing reveals independent sgcd deletions causing limb girdle muscular dystrophy in boston terriers. Skeletal Muscle. (2017) 7:15. doi: 10.1186/s13395-017-0131-0
132. Shelton GD, Engvall E. Canine and feline models of human inherited muscle diseases. Neuromuscul Disord. (2005) 15:127–38. doi: 10.1016/j.nmd.2004.10.019
133. Hong HP, Thomovsky SA, Lewis MJ, Bentley RT, Shelton GD. Clinical characteristics of non-infectious inflammatory myopathy in the boxer dog. J Small Anim Pract. (2021) 62:765–74. doi: 10.1111/jsap.13350
134. Evans J, Levesque D, Shelton GD. Canine inflammatory myopathies: a clinicopathologic review of 200 cases. J Vet Intern Med. (2004) 18:679–91. doi: 10.1111/j.1939-1676.2004.tb02606.x
135. Toyoda K, Uchida K, Matsuki N, Sakai H, Kitagawa M, Saito M, et al. Inflammatory myopathy with severe tongue atrophy in pembroke welsh corgi dogs. J Vet Diagn Invest. (2010) 22:876–85. doi: 10.1177/104063871002200605
136. Shelton GD, Schule A, Kass PH. Risk factors for acquired myasthenia gravis in dogs: 1,154 cases (1991-1995). J Am Vet Med Assoc. (1997) 211:1428–31.
137. Patterson DF. Epidemiologic and genetic studies of congenital heart disease in the dog. Circul Res. (1968) 23:171–202. doi: 10.1161/01.RES.23.2.171
138. Buchanan JW. Tracheal signs and associated vascular anomalies in dogs with persistent right aortic arch. J Vet Intern Med. (2004) 18:510–4. doi: 10.1111/j.1939-1676.2004.tb02576.x
139. Krebs IA, Lindsley S, Shaver S, MacPhail C. Short- and long-term outcome of dogs following surgical correction of a persistent right aortic arch. J Am Anim Hosp Assoc. (2014) 50:181–6. doi: 10.5326/JAAHA-MS-6034
140. Gunby JM, Hardie RJ, Bjorling DE. Investigation of the potential heritability of persistent right aortic arch in greyhounds. J Am Vet Med Assoc. (2004) 224:1120–2:1111. doi: 10.2460/javma.2004.224.1120
141. Guiot LP, Lansdowne JL, Rouppert P, Stanley BJ. Hiatal hernia in the dog: a clinical report of four chinese shar peis. J Am Anim Hosp Assoc. (2008) 44:335–41. doi: 10.5326/0440335
142. Johnson BM, Denovo RC, Mears EA. Web chapter 47: Canine Megaesophagus. In: Bonagura JD, Twedt DC, editors. Kirk's Current Veterinary Therapy, 15th ed. St. Louis, MO: Elsevier Saunders (2014). p. 4050–82.
143. Cox VS, Wallace LJ, Anderson VE, Rushmer RA. Hereditary esophageal dysfunction in the miniature schnauzer dog. Am J Vet Res. (1980) 41:326–30.
144. Stanley BJ, Hauptman JG, Fritz MC, Rosenstein DS, Kinns J. Esophageal dysfunction in dogs with idiopathic laryngeal paralysis: a controlled cohort study. Vet Surg. (2010) 39:139–49. doi: 10.1111/j.1532-950X.2009.00626.x
145. Roden DF, Altman KW. Causes of dysphagia among different age groups: a systematic review of the literature. Otolaryngol Clin North Am. (2013) 46:965–87. doi: 10.1016/j.otc.2013.08.008
146. Kim JP, Kahrilas PJ. How i approach dysphagia. Curr Gastroenterol Rep. (2019) 21:49. doi: 10.1007/s11894-019-0718-1
147. Marks SL. Chapter 121: Oropharyngeal Dysphagia. In: Bonagura JD, Twedt DC, editor. Kirk's Current Veterinary Therapy, XV 15th ed. St. Louis, MO: Elsevier/Saunders (2014). p. 3450-3475.
148. Patel DA, Sharda R, Hovis KL, Nichols EE, Sathe N, Penson DF, et al. Patient-reported outcome measures in dysphagia: a systematic review of instrument development and validation. Dis Esophagus. (2017) 30:1–23. doi: 10.1093/dote/dow028
149. Belafsky PC, Mouadeb DA, Rees CJ, Pryor JC, Postma GN, Allen J, et al. Validity and reliability of the eating assessment tool (eat-10). Ann Otol Rhinol Laryngol. (2008) 117:919–24. doi: 10.1177/000348940811701210
150. Taft TH, Kern E, Starkey K, Craft J, Craven M, Doerfler B, et al. The dysphagia stress test for rapid assessment of swallowing difficulties in esophageal conditions. Neurogastroenterol Motil. (2019) 31:e13512. doi: 10.1111/nmo.13512
151. Thottam PJ, Silva RC, McLevy JD, Simons JP, Mehta DK. Use of fiberoptic endoscopic evaluation of swallowing (fees) in the management of psychogenic dysphagia in children. Int J Pediatr Otorhinolaryngol. (2015) 79:108–10. doi: 10.1016/j.ijporl.2014.11.007
152. Baumann A, Katz PO. Functional disorders of swallowing. Handb Clin Neurol. (2016) 139:483–8. doi: 10.1016/B978-0-12-801772-2.00039-4
153. Grobman ME, Masseau I, Reinero CR. Aerodigestive disorders in dogs evaluated for cough using respiratory fluoroscopy and videofluoroscopic swallow studies. Vet J. (2019) 251:105344. doi: 10.1016/j.tvjl.2019.105344
154. Boesch RP, Balakrishnan K, Acra S, Benscoter DT, Cofer SA, Collaco JM, et al. Structure and functions of pediatric aerodigestive programs: a consensus statement. Pediatrics. (2018) 141:e20171701. doi: 10.1542/peds.2017-1701
155. Velayutham P, Irace AL, Kawai K, Dodrill P, Perez J, Londahl M, et al. Silent aspiration: who is at risk? Laryngoscope. (2018) 128:1952–7. doi: 10.1002/lary.27070
156. Liu LWC, Andrews CN, Armstrong D, Diamant N, Jaffer N, Lazarescu A, et al. Clinical practice guidelines for the assessment of uninvestigated esophageal dysphagia. J Can Assoc Gastroenterol. (2018) 1:5–19. doi: 10.1093/jcag/gwx008
157. Haines JM. Survey of owners on population characteristics, diagnosis, and environmental, health, and disease associations in dogs with megaesophagus. Res Vet Sci. (2019) 123:1–6. doi: 10.1016/j.rvsc.2018.11.026
158. Tobin RW. Esophageal rings, webs, and diverticula. J Clin Gastroenterol. (1998) 27:285–95. doi: 10.1097/00004836-199812000-00003
159. Rosolowski M, Kierzkiewicz M. Etiology, diagnosis and treatment of infectious esophagitis. Prz Gastroenterol. (2013) 8:333–7. doi: 10.5114/pg.2013.39914
160. Jaffer NM, Ng E, Au FW, Steele CM. Fluoroscopic evaluation of oropharyngeal dysphagia: anatomic, technical, and common etiologic factors. AJR Am J Roentgenol. (2015) 204:49–58. doi: 10.2214/AJR.13.12374
161. George NS, Rangan V, Geng Z, Khan F, Kichler A, Gabbard S, et al. Distribution of esophageal motor disorders in diabetic patients with dysphagia. J Clin Gastroenterol. (2017) 51:890–5. doi: 10.1097/MCG.0000000000000894
162. Boccardi V, Ruggiero C, Patriti A, Marano L. Diagnostic assessment and management of dysphagia in patients with alzheimer's disease. J Alzheimers Dis. (2016) 50:947–55. doi: 10.3233/JAD-150931
163. Lopez-Liria R, Parra-Egeda J, Vega-Ramirez FA, Aguilar-Parra JM, Trigueros-Ramos R, Morales-Gazquez MJ, et al. Treatment of dysphagia in parkinson's disease: a systematic review. Int J Environ Res Public Health. (2020) 17. doi: 10.3390/ijerph17114104
164. Pizzorni N, Pirola F, Ciammola A, Schindler A. Management of dysphagia in huntington's disease: a descriptive review. Neurol Sci. (2020) 41:1405–17. doi: 10.1007/s10072-020-04265-0
165. Ansari NN, Tarameshlu M, Ghelichi L. Dysphagia in multiple sclerosis patients: diagnostic and evaluation strategies. Degener Neurol Neuromuscul Dis. (2020) 10:15–28. doi: 10.2147/DNND.S198659
166. Haines JM, Khoo A, Brinkman E, Thomason JM, Mackin AJ. Technique for evaluation of gravity-assisted esophageal transit characteristics in dogs with megaesophagus. J Am Anim Hosp Assoc. (2019) 55:167–77. doi: 10.5326/JAAHA-MS-6711
167. Grobman ME, Hutcheson KD, Lever TE, Mann FA, Reinero CR. Mechanical dilation, botulinum toxin a injection, and surgical myotomy with fundoplication for treatment of lower esophageal sphincter achalasia-like syndrome in dogs. J Vet Intern Med. (2019) 33:1423–33. doi: 10.1111/jvim.15476
168. Mayhew PD, Balsa IM, Marks SL, Pollard RE, Case JB, Culp WTN, et al. Clinical and videofluoroscopic outcomes of laparoscopic treatment for sliding hiatal hernia and associated gastroesophageal reflux in brachycephalic dogs. Vet Surg. (2021) 50:O67–77. doi: 10.1111/vsu.13622
169. Martin-Harris B, Brodsky MB, Michel Y, Castell DO, Schleicher M, Sandidge J, et al. Mbs measurement tool for swallow impairment–mbsimp: establishing a standard. Dysphagia. (2008) 23:392–405. doi: 10.1007/s00455-008-9185-9
170. Hazelwood RJ, Armeson KE, Hill EG, Bonilha HS, Martin-Harris B. Identification of swallowing tasks from a modified barium swallow study that optimize the detection of physiological impairment. J Speech Lang Hear Res. (2017) 60:1855–63. doi: 10.1044/2017_JSLHR-S-16-0117
171. Martin-Harris B, Logemann JA, McMahon S, Schleicher M, Sandidge J. Clinical utility of the modified barium swallow. Dysphagia. (2000) 15:136–41. doi: 10.1007/s004550010015
172. Martin-Harris B, Canon CL, Bonilha HS, Murray J, Davidson K, Lefton-Greif MA. Best practices in modified barium swallow studies. Am J Speech Lang Pathol. (2020) 29:1078–93. doi: 10.1044/2020_AJSLP-19-00189
173. Bisch EM, Logemann JA, Rademaker AW, Kahrilas PJ, Lazarus CL. Pharyngeal effects of bolus volume, viscosity, and temperature in patients with dysphagia resulting from neurologic impairment and in normal subjects. J Speech Hear Res. (1994) 37:1041–59. doi: 10.1044/jshr.3705.1041
174. Logemann JA, Pauloski BR, Colangelo L, Lazarus C, Fujiu M, Kahrilas PJ. Effects of a sour bolus on oropharyngeal swallowing measures in patients with neurogenic dysphagia. J Speech Hear Res. (1995) 38:556–63. doi: 10.1044/jshr.3803.556
175. Gosa MM, Dodrill P. Effect of time and temperature on thickened infant formula. Nutr Clin Pract. (2017) 32:238–44. doi: 10.1177/0884533616662991
176. Turkington L, Ward EC, Farrell AM. An investigation of taste intensity, palatability, effervescence, and sip volumes of carbonated liquid stimuli for use in videofluoroscopic studies. Am J Speech Lang Pathol. (2019) 28:1248–56. doi: 10.1044/2019_AJSLP-19-0045
177. Steele CM, Alsanei WA, Ayanikalath S, Barbon CE, Chen J, Cichero JA, et al. The influence of food texture and liquid consistency modification on swallowing physiology and function: a systematic review. Dysphagia. (2015) 30:2–26. doi: 10.1007/s00455-014-9578-x
178. Newman R, Vilardell N, Clave P, Speyer R. Effect of bolus viscosity on the safety and efficacy of swallowing and the kinematics of the swallow response in patients with oropharyngeal dysphagia: white paper by the european society for swallowing disorders (essd). Dysphagia. (2016) 31:232–49. doi: 10.1007/s00455-016-9696-8
179. Kuhlemeier KV, Palmer JB, Rosenberg D. Effect of liquid bolus consistency and delivery method on aspiration and pharyngeal retention in dysphagia patients. Dysphagia. (2001) 16:119–22. doi: 10.1007/s004550011003
180. Leonard RJ, White C, McKenzie S, Belafsky PC. Effects of bolus rheology on aspiration in patients with dysphagia. J Acad Nutr Diet. (2014) 114:590–4. doi: 10.1016/j.jand.2013.07.037
181. McGrattan KE, McGhee HC, McKelvey KL, Clemmens CS, Hill EG, DeToma A, et al. Capturing infant swallow impairment on videofluoroscopy: timing matters. Pediatr Radiol. (2020) 50:199–206. doi: 10.1007/s00247-019-04527-w
182. Gilchrist AM, Levine MS, Carr RF, Saul SH, Katzka DA, Herlinger H, et al. Barrett's esophagus: diagnosis by double-contrast esophagography. AJR Am J Roentgenol. (1988) 150:97–102. doi: 10.2214/ajr.150.1.97
183. Dibble C, Levine MS, Rubesin SE, Laufer I, Katzka DA. Detection of reflux esophagitis on double-contrast esophagrams and endoscopy using the histologic findings as the gold standard. Abdom Imaging. (2004) 29:421–5. doi: 10.1007/s00261-003-0128-5
184. Novak SH, Shortsleeve MJ, Kantrowitz PA. Effective treatment of symptomatic lower esophageal (schatzki) rings with acid suppression therapy: confirmed on barium esophagography. AJR Am J Roentgenol. (2015) 205:1182–7. doi: 10.2214/AJR.15.14704
185. Levine MS, Carucci LR, DiSantis DJ, Einstein DM, Hawn MT, Martin-Harris B, et al. Consensus statement of society of abdominal radiology disease-focused panel on barium esophagography in gastroesophageal reflux disease. AJR Am J Roentgenol. (2016) 207:1009–15. doi: 10.2214/AJR.16.16323
186. Lazarus CL. History of the use and impact of compensatory strategies in management of swallowing disorders. Dysphagia. (2017) 32:3–10. doi: 10.1007/s00455-016-9779-6
187. Daniels SK, Schroeder MF, DeGeorge PC, Corey DM, Rosenbek JC. Effects of verbal cue on bolus flow during swallowing. Am J Speech Lang Pathol. (2007) 16:140–7. doi: 10.1044/1058-0360(2007/018)
188. Johnson DN, Herring HJ, Daniels SK. Dysphagia management in stroke rehabilitation. Curr Phys Med Rehabil Rep. (2014) 2:207–18. doi: 10.1007/s40141-014-0059-9
189. Donzelli J, Brady S. The effects of breath-holding on vocal fold adduction: implications for safe swallowing. Arch Otolaryngol Head Neck Surg. (2004) 130:208–10. doi: 10.1001/archotol.130.2.208
190. Huckabee ML, Steele CM. An analysis of lingual contribution to submental surface electromyographic measures and pharyngeal pressure during effortful swallow. Arch Phys Med Rehabil. (2006) 87:1067–72. doi: 10.1016/j.apmr.2006.04.019
191. Lee SM, Lee BH, Kim JW, Jang JY, Jang EG, Ryu JS. Effects of chin-down maneuver on pharyngeal pressure generation according to dysphagia and viscosity. Ann Rehabil Med. (2020) 44:493–501. doi: 10.5535/arm.20016
192. Robbins J, Kays SA, Gangnon RE, Hind JA, Hewitt AL, Gentry LR, et al. The effects of lingual exercise in stroke patients with dysphagia. Arch Phys Med Rehabil. (2007) 88:150–8. doi: 10.1016/j.apmr.2006.11.002
193. Smaoui S, Langridge A, Steele CM. The effect of lingual resistance training interventions on adult swallow function: a systematic review. Dysphagia. (2020) 35:745–61. doi: 10.1007/s00455-019-10066-1
194. Dane B, Doshi A, Khan A, Megibow A. Utility of water siphon maneuver for eliciting gastroesophageal reflux during barium esophagography: correlation with histologic findings. AJR Am J Roentgenol. (2018) 211:335–9. doi: 10.2214/AJR.17.19063
195. Thompson JK, Koehler RE, Richter JE. Detection of gastroesophageal reflux: value of barium studies compared with 24-hr ph monitoring. AJR Am J Roentgenol. (1994) 162:621–6. doi: 10.2214/ajr.162.3.8109509
196. Bonadio CM, Pollard RE, Dayton PA, Leonard CD, Marks SL. Effects of body positioning on swallowing and esophageal transit in healthy dogs. J Vet Intern Med. (2009) 23:801–5. doi: 10.1111/j.1939-1676.2009.0325.x
197. Cheney DM, Marks SL, Pollard RE. Effect of bolus size on deglutition and esophageal transit in healthy dogs. Vet Radiol Ultrasound. (2016) 57:359–65. doi: 10.1111/vru.12362
198. Harris RA, Grobman ME, Allen MJ, Schachtel J, Rawson NE, Bennett B, et al. Standardization of a videofluoroscopic swallow study protocol to investigate dysphagia in dogs. J Vet Intern Med. (2017) 31:383–93. doi: 10.1111/jvim.14676
199. Strombeck DR, Harrold D. Effects of atropine, acepromazine, meperidine, and xylazine on gastroesophageal sphincter pressure in the dog. Am J Vet Res. (1985) 46:963–5.
200. Zhang X, Xiang X, Tu L, Xie X, Hou X. Esophageal motility in the supine and upright positions for liquid and solid swallows through high-resolution manometry. J Neurogastroenterol Motil. (2013) 19:467–72. doi: 10.5056/jnm.2013.19.4.467
201. Tariq H, Makker J, Chime C, Kamal MU, Rafeeq A, Patel H. Revisiting the reliability of the endoscopy and sedation-assisted high-resolution esophageal motility assessment. Gastroenterology Res. (2019) 12:157–65. doi: 10.14740/gr1185
202. Pollard RE, Johnson LR, Marks SL. The prevalence of dynamic pharyngeal collapse is high in brachycephalic dogs undergoing videofluoroscopy. Vet Radiol Ultrasound. (2018) 59:529–34. doi: 10.1111/vru.12655
203. Bexfield NH, Buxton RJ, Vicek TJ, Day MJ, Bailey SM, Haugland SP, et al. Breed, age and gender distribution of dogs with chronic hepatitis in the united kingdom. Vet J. (2012) 193:124–8. doi: 10.1016/j.tvjl.2011.11.024
204. Pollard RE, Marks SL, Leonard R, Belafsky PC. Preliminary evaluation of the pharyngeal constriction ratio (pcr) for fluoroscopic determination of pharyngeal constriction in dysphagic dogs. Vet Radiol Ultrasound. (2007) 48:221–6. doi: 10.1111/j.1740-8261.2007.00232.x
205. Lee JW, Randall DR, Evangelista LM, Kuhn MA, Belafsky PC. Subjective assessment of videofluoroscopic swallow studies. Otolaryngol Head Neck Surg. (2017) 156:901–5. doi: 10.1177/0194599817691276
206. Dharmarathna I, Miles A, Allen J. Twenty years of quantitative instrumental measures of swallowing in children: a systematic review. Eur J Pediatr. (2020) 179:203–23. doi: 10.1007/s00431-019-03546-x
207. Nishikubo K, Mise K, Ameya M, Hirose K, Kobayashi T, Hyodo M. Quantitative evaluation of age-related alteration of swallowing function: videofluoroscopic and manometric studies. Auris Nasus Larynx. (2015) 42:134–8. doi: 10.1016/j.anl.2014.07.002
208. Alves LM, Cassiani Rde A, Santos CM, Dantas RO. Gender effect on the clinical measurement of swallowing. Arq Gastroenterol. (2007) 44:227–9. doi: 10.1590/S0004-28032007000300009
209. Clave P, De Kraa M, Arreola V, Girvent M, Farre R, Palomera E, et al. The effect of bolus viscosity on swallowing function in neurogenic dysphagia. Alimentary Pharmacol Ther. (2006) 24:1385–94. doi: 10.1111/j.1365-2036.2006.03118.x
210. Butler SG, Stuart A, Case LD, Rees C, Vitolins M, Kritchevsky SB. Effects of liquid type, delivery method, and bolus volume on penetration-aspiration scores in healthy older adults during flexible endoscopic evaluation of swallowing. Ann Otol Rhinol Laryngol. (2011) 120:288–95. doi: 10.1177/000348941112000502
211. Sia I, Crary MA, Kairalla J, Carnaby GD, Sheplak M, McCulloch T. Bolus volume and viscosity effects on pharyngeal swallowing power-how physiological bolus accommodation affects bolus dynamics. Neurogastroenterol Motil. (2018) 30:e13481. doi: 10.1111/nmo.13481
212. Turkington L, Ward EC, Farrell A, Porter L, Wall LR. Impact of carbonation on neurogenic dysphagia and an exploration of the clinical predictors of a response to carbonation. Int J Lang Commun Disord. (2019) 54:499–513. doi: 10.1111/1460-6984.12458
213. Boaden E, Nightingale J, Bradbury C, Hives L, Georgiou R. Clinical practice guidelines for videofluoroscopic swallowing studies: a systematic review. Radiography. (2020) 26:154–62. doi: 10.1016/j.radi.2019.10.011
214. Blonski W, Kumar A, Feldman J, Richter JE. Timed barium swallow for assessing long-term treatment response in patients with achalasia: absolute cutoff versus percent change - a cross-sectional analytic study. Neurogastroenterol Motil. (2021) 33:e14005. doi: 10.1111/nmo.14005
215. Kachala SS, Rice TW, Baker ME, Rajeswaran J, Thota PN, Murthy SC, et al. Value of routine timed barium esophagram follow-up in achalasia after myotomy. J Thorac Cardiovasc Surg. (2018) 156:871–877.e2. doi: 10.1016/j.jtcvs.2018.03.001
216. Mehain SO, Haines JM, Guess SC. A randomized crossover study of compounded liquid sildenafil for treatment of generalized megaesophagus in dogs. Am J Vet Res. (2022) 83:317–23. doi: 10.2460/ajvr.21.02.0030
217. Langmore SE, Schatz K, Olsen N. Fiberoptic endoscopic examination of swallowing safety: a new procedure. Dysphagia. (1988) 2:216–9. doi: 10.1007/BF02414429
218. Giraldo-Cadavid LF, Leal-Leano LR, Leon-Basantes GA, Bastidas AR, Garcia R, Ovalle S, et al. Accuracy of endoscopic and videofluoroscopic evaluations of swallowing for oropharyngeal dysphagia. Laryngoscope. (2017) 127:2002–10. doi: 10.1002/lary.26419
219. Espitalier F, Fanous A, Aviv J, Bassiouny S, Desuter G, Nerurkar N, et al. International consensus (icon) on assessment of oropharyngeal dysphagia. Eur Ann Otorhinolaryngol Head Neck Dis. (2018) 135:S17–S21. doi: 10.1016/j.anorl.2017.12.009
220. Belafsky PC, Rees CJ. Functional oesophagoscopy: endoscopic evaluation of the oesophageal phase of deglutition. J Laryngol Otol. (2009) 123:1031–4. doi: 10.1017/S0022215109004472
221. Marks SL, Douthitt KL, Belafsky PC. Feasibility of flexible endoscopic evaluation of swallowing in healthy dogs. Am J Vet Res. (2016) 77:294–9. doi: 10.2460/ajvr.77.3.294
222. Conklin JL. Evaluation of esophageal motor function with high-resolution manometry. J Neurogastroenterol Motil. (2013) 19:281–94. doi: 10.5056/jnm.2013.19.3.281
223. Fox MR, Bredenoord AJ. Oesophageal high-resolution manometry: moving from research into clinical practice. Gut. (2008) 57:405–23. doi: 10.1136/gut.2007.127993
224. Pandolfino JE, Fox MR, Bredenoord AJ, Kahrilas PJ. High-resolution manometry in clinical practice: utilizing pressure topography to classify oesophageal motility abnormalities. Neurogastroenterol Motil. (2009) 21:796–806. doi: 10.1111/j.1365-2982.2009.01311.x
225. Fox M, Hebbard G, Janiak P, Brasseur JG, Ghosh S, Thumshirn M, et al. High-resolution manometry predicts the success of oesophageal bolus transport and identifies clinically important abnormalities not detected by conventional manometry. Neurogastroenterol Motil. (2004) 16:533–42. doi: 10.1111/j.1365-2982.2004.00539.x
226. Yadlapati R. High-resolution esophageal manometry: interpretation in clinical practice. Curr Opin Gastroenterol. (2017) 33:301–9. doi: 10.1097/MOG.0000000000000369
227. Fox MR, Sweis R, Yadlapati R, Pandolfino J, Hani A, Defilippi C, et al. Chicago classification version 4.0(©) technical review: update on standard high-resolution manometry protocol for the assessment of esophageal motility. Neurogastroenterol Motil. (2021) 33:e14120. doi: 10.1111/nmo.14120
228. Oude Nijhuis RAB, Prins LI, Mostafavi N, van Etten-Jamaludin FS, Smout A, Bredenoord AJ. Factors associated with achalasia treatment outcomes: systematic review and meta-analysis. Clin Gastroenterol Hepatol. (2020) 18:1442–53. doi: 10.1016/j.cgh.2019.10.008
229. Andolfi C, Fisichella PM. Meta-analysis of clinical outcome after treatment for achalasia based on manometric subtypes. Br J Surg. (2019) 106:332–41. doi: 10.1002/bjs.11049
230. Kahrilas PJ, Katzka D, Richter JE. Clinical practice update: the use of per-oral endoscopic myotomy in achalasia: expert review and best practice advice from the aga institute. Gastroenterology. (2017) 153:1205–11. doi: 10.1053/j.gastro.2017.10.001
231. Jung HK, Hong SJ, Lee OY, Pandolfino J, Park H, Miwa H, et al. 2019 seoul consensus on esophageal achalasia guidelines. J Neurogastroenterol Motil. (2020) 26:180–203. doi: 10.5056/jnm20014
232. Li L, Gao H, Zhang C, Tu J, Geng X, Wang J, et al. Diagnostic value of x-ray, endoscopy, and high-resolution manometry for hiatal hernia: a systematic review and meta-analysis. J Gastroenterol Hepatol. (2020) 35:13–8. doi: 10.1111/jgh.14758
233. Rengarajan A, Gyawali CP. High-resolution manometry can characterize esophagogastric junction morphology and predict esophageal reflux burden. J Clin Gastroenterol. (2020) 54:22–7. doi: 10.1097/MCG.0000000000001205
234. Triantafyllou T, Theodoropoulos C, Georgiou G, Kalles V, Chrysikos D, Filis K, et al. Long-term outcome of myotomy and fundoplication based on intraoperative real-time high-resolution manometry in achalasia patients. Ann Gastroenterol. (2019) 32:46–51. doi: 10.20524/aog.2018.0326
235. Siegal SR, Dunst CM, Robinson B, Dewey EN, Swanstrom LL, DeMeester SR. Preoperative high-resolution manometry criteria are associated with dysphagia after nissen fundoplication. World J Surg. (2019) 43:1062–7. doi: 10.1007/s00268-018-4870-9
236. Kempf J, Lewis F, Reusch CE, Kook PH. High-resolution manometric evaluation of the effects of cisapride and metoclopramide hydrochloride administered orally on lower esophageal sphincter pressure in awake dogs. Am J Vet Res. (2014) 75:361–6. doi: 10.2460/ajvr.75.4.361
237. Kempf J, Heinrich H, Reusch CE, Kook PH. Evaluation of esophageal high-resolution manometry in awake and sedated dogs. Am J Vet Res. (2013) 74:895–900. doi: 10.2460/ajvr.74.6.895
238. McCulloch TM, Knigge MA. High-resolution Manometry. In: Weissbrod PA, Francis DO, editors. Neurologic and Neurodegenerative Diseases of the Larynx. Cham: Springer International Publishing (2020). p. 97–106.
239. Belafsky PC. Transnasal Esophagoscopy. In: Belafsky PC, editor. Clinical Esophagology and Transnasal Esophagoscopy. San Diego, CA: Plural Publishing, Incorporated (2019). p. 15–28.
240. Rogers BD, Gyawali CP. Enhancing high-resolution esophageal manometry: use of ancillary techniques and maneuvers. Gastroenterol Clin North Am. (2020) 49:411–26. doi: 10.1016/j.gtc.2020.04.001
241. Fornari F, Bravi I, Penagini R, Tack J, Sifrim D. Multiple rapid swallowing: a complementary test during standard oesophageal manometry. Neurogastroenterol Motil. (2009) 21:718.e41. doi: 10.1111/j.1365-2982.2009.01273.x
242. Kushnir V, Sayuk GS, Gyawali CP. Multiple rapid swallow responses segregate achalasia subtypes on high-resolution manometry. Neurogastroenterol Motil. (2012) 24:1069.e561. doi: 10.1111/j.1365-2982.2012.01971.x
243. Min YW, Shin I, Son HJ, Rhee P-L. Multiple rapid swallow maneuver enhances the clinical utility of high-resolution manometry in patients showing ineffective esophageal motility. Medicine. (2015) 94:e13100. doi: 10.1097/MD.0000000000001669
244. Hashmi S, Rao SS, Summers RW, Schulze K. Esophageal pressure topography, body position, and hiatal hernia. J Clin Gastroenterol. (2014) 48:224–30. doi: 10.1097/MCG.0000000000000057
245. Ang D, Misselwitz B, Hollenstein M, Knowles K, Wright J, Tucker E, et al. Diagnostic yield of high-resolution manometry with a solid test meal for clinically relevant, symptomatic oesophageal motility disorders: serial diagnostic study. Lancet Gastroenterol Hepatol. (2017) 2:654–61. doi: 10.1016/S2468-1253(17)30148-6
246. Araujo IK, Roman S, Napoléon M, Mion F. Diagnostic yield of adding solid food swallows during high-resolution manometry in esophageal motility disorders. Neurogastroenterol Motil. (2021) 33:e14060. doi: 10.1111/nmo.14060
247. Yadlapati R, Tye M, Roman S, Kahrilas PJ, Ritter K, Pandolfino JE. Postprandial high-resolution impedance manometry identifies mechanisms of nonresponse to proton pump inhibitors. Clin Gastroenterol Hepatol. (2018) 16:211–8.e1. doi: 10.1016/j.cgh.2017.09.011
248. Snyder DL, Crowell MD, Horsley-Silva J, Ravi K, Lacy BE, Vela MF. Opioid-induced esophageal dysfunction: differential effects of type and dose. Am J Gastroenterol. (2019) 114:1464–9. doi: 10.14309/ajg.0000000000000369
249. Omari TI, Ciucci M, Gozdzikowska K, Hernández E, Hutcheson K, Jones C, et al. High-resolution pharyngeal manometry and impedance: protocols and metrics-recommendations of a high-resolution pharyngeal manometry international working group. Dysphagia. (2020) 35:281–95. doi: 10.1007/s00455-019-10023-y
250. He S, Jell A, Hüser N, Kohn N, Feussner H. 24-hour monitoring of transient lower esophageal sphincter relaxation events by long-term high-resolution impedance manometry in normal volunteers: the “mirror phenomenon”. Neurogastroenterol Motil. (2019) 31:e13530. doi: 10.1111/nmo.13530
251. Lee TH, Lee JS, Park JW, Cho SJ, Hong SJ, Jeon SR, et al. High-resolution impedance manometry facilitates assessment of pharyngeal residue and oropharyngeal dysphagic mechanisms. Dis Esophagus. (2014) 27:220–9. doi: 10.1111/dote.12101
252. Singendonk MJ, Lin Z, Scheerens C, Tack J, Carlson DA, Omari TI, et al. High-resolution impedance manometry parameters in the evaluation of esophageal function of non-obstructive dysphagia patients. Neurogastroenterol Motil. (2019) 31:e13505. doi: 10.1111/nmo.13505
253. Kou W, Carlson DA, Patankar NA, Kahrilas PJ, Pandolfino JE. Four-dimensional impedance manometry derived from esophageal high-resolution impedance-manometry studies: a novel analysis paradigm. Therap Adv Gastroenterol. (2020) 13:1756284820969050. doi: 10.1177/1756284820969050
254. Ott DJ. Gastroesophageal reflux: what is the role of barium studies? AJR Am J Roentgenol. (1994) 162:627–9. doi: 10.2214/ajr.162.3.8109510
255. Gyawali CP, Kahrilas PJ, Savarino E, Zerbib F, Mion F, Smout AJPM, et al. Modern diagnosis of gerd: the lyon consensus. Gut. (2018) 67:1351. doi: 10.1136/gutjnl-2017-314722
256. Dent J, Becher A, Sung J, Zou D, Agréus L, Bazzoli F. Systematic review: patterns of reflux-induced symptoms and esophageal endoscopic findings in large-scale surveys. Clin Gastroenterol Hepatol. (2012) 10:863–73.e3. doi: 10.1016/j.cgh.2012.02.028
257. Chen MY, Ott DJ, Sinclair JW, Wu WC, Gelfand DW. Gastroesophageal reflux disease: correlation of esophageal ph testing and radiographic findings. Radiology. (1992) 185:483–6. doi: 10.1148/radiology.185.2.1410359
258. Hila A, Agrawal A, Castell DO. Combined multichannel intraluminal impedance and ph esophageal testing compared to ph alone for diagnosing both acid and weakly acidic gastroesophageal reflux. Clin Gastroenterol Hepatol. (2007) 5:172–7. doi: 10.1016/j.cgh.2006.11.015
259. Bredenoord AJ, Weusten BL, Timmer R, Conchillo JM, Smout AJ. Addition of esophageal impedance monitoring to ph monitoring increases the yield of symptom association analysis in patients off ppi therapy. Am J Gastroenterol. (2006) 101:453–9. doi: 10.1111/j.1572-0241.2006.00427.x
260. Bredenoord AJ. Impedance-ph monitoring: new standard for measuring gastro-oesophageal reflux. Neurogastroenterol Motil. (2008) 20:434–9. doi: 10.1111/j.1365-2982.2008.01131.x
261. Bytzer P, Jones R, Vakil N, Junghard O, Lind T, Wernersson B, et al. Limited ability of the proton-pump inhibitor test to identify patients with gastroesophageal reflux disease. Clin Gastroenterol Hepatol. (2012) 10:1360–6. doi: 10.1016/j.cgh.2012.06.030
262. Oh TH. Accuracy of the diagnosis of gord by questionnaire, physicians and a trial of proton pump inhibitor treatment: the diamond study (gut 2010;59:714-721). J Neurogastroenterol Motil. (2011) 17:98–9. doi: 10.5056/jnm.2011.17.1.98
263. Emerenziani S, Sifrim D. Gastroesophageal reflux and gastric emptying, revisited. Curr Gastroenterol Rep. (2005) 7:190–5. doi: 10.1007/s11894-005-0033-x
264. Viskjer S, Sjöström L. Effect of the duration of food withholding prior to anesthesia on gastroesophageal reflux and regurgitation in healthy dogs undergoing elective orthopedic surgery. Am J Vet Res. (2017) 78:144–50. doi: 10.2460/ajvr.78.2.144
265. Anagnostou TL, Savvas I, Kazakos GM, Ververidis HN, Psalla D, Kostakis C, et al. The effect of the stage of the ovarian cycle (anoestrus or dioestrus) and of pregnancy on the incidence of gastro-oesophageal reflux in dogs undergoing ovariohysterectomy. Vet Anaesth Analg. (2015) 42:502–11. doi: 10.1111/vaa.12234
266. Anagnostou TL, Savvas I, Kazakos GM, Ververidis HN, Haritopoulou MR, Rallis TS, et al. Effect of endogenous progesterone and oestradiol-17beta on the incidence of gastro-oesophageal reflux and on the barrier pressure during general anaesthesia in the female dog. Vet Anaesth Analg. (2009) 36:308–18. doi: 10.1111/j.1467-2995.2009.00462.x
267. Shaver SL, Barbur LA, Jimenez DA, Brainard BM, Cornell KK, Radlinsky MG, et al. Evaluation of gastroesophageal reflux in anesthetized dogs with brachycephalic syndrome. J Am Anim Hosp Assoc. (2017) 53:24–31. doi: 10.5326/JAAHA-MS-6281
268. Anagnostou TL, Kazakos GM, Savvas I, Kostakis C, Papadopoulou P. Gastro-oesophageal reflux in large-sized, deep-chested versus small-sized, barrel-chested dogs undergoing spinal surgery in sternal recumbency. Vet Anaesth Analg. (2017) 44:35–41. doi: 10.1111/vaa.12404
269. Torrente C, Vigueras I, Manzanilla EG, Villaverde C, Fresno L, Carvajal B, et al. Prevalence of and risk factors for intraoperative gastroesophageal reflux and postanesthetic vomiting and diarrhea in dogs undergoing general anesthesia. J Vet Emerg Crit Care. (2017) 27:397–408. doi: 10.1111/vec.12613
270. Savvas I, Raptopoulos D, Rallis T. A “light meal” three hours preoperatively decreases the incidence of gastro-esophageal reflux in dogs. J Am Anim Hosp Assoc. (2016) 52:357–63. doi: 10.5326/JAAHA-MS-6399
271. Wilson DV, Evans AT, Miller R. Effects of preanesthetic administration of morphine on gastroesophageal reflux and regurgitation during anesthesia in dogs. Am J Vet Res. (2005) 66:386–90. doi: 10.2460/ajvr.2005.66.386
272. Wilson DV, Boruta DT, Evans AT. Influence of halothane, isoflurane, and sevoflurane on gastroesophageal reflux during anesthesia in dogs. Am J Vet Res. (2006) 67:1821–5. doi: 10.2460/ajvr.67.11.1821
273. Roush JK, Keene BW, Eicker SW, Bjorling DE. Effects of atropine and glycopyrrolate on esophageal, gastric, and tracheal ph in anesthetized dogs. Vet Surg. (1990) 19:88–92. doi: 10.1111/j.1532-950X.1990.tb01147.x
274. Wilson DV, Tom Evans A, Mauer WA. Pre-anesthetic meperidine: associated vomiting and gastroesophageal reflux during the subsequent anesthetic in dogs. Vet Anaesth Analg. (2007) 34:15–22. doi: 10.1111/j.1467-2995.2006.00295.x
275. Favarato ES, de Souza MV, Costa PR, Pompermayer LG, Favarato LS, Ribeiro Júnior JI. Ambulatory esophageal phmetry in healthy dogs with and without the influence of general anesthesia. Vet Res Commun. (2011) 35:271–82. doi: 10.1007/s11259-011-9471-0
276. Jones CT, Fransson BA. Evaluation of the effectiveness of preoperative administration of maropitant citrate and metoclopramide hydrochloride in preventing postoperative clinical gastroesophageal reflux in dogs. J Am Vet Med Assoc. (2019) 255:437–45. doi: 10.2460/javma.255.4.437
277. Johnson RA. Maropitant prevented vomiting but not gastroesophageal reflux in anesthetized dogs premedicated with acepromazine-hydromorphone. Vet Anaesth Analg. (2014) 41:406–10. doi: 10.1111/vaa.12120
278. Favarato ES, Souza MV, Costa PR, Favarato LS, Nehme RC, Monteiro BS, et al. Evaluation of metoclopramide and ranitidine on the prevention of gastroesophageal reflux episodes in anesthetized dogs. Res Vet Sci. (2012) 93:466–7. doi: 10.1016/j.rvsc.2011.07.027
279. Lotti F, Twedt D, Warrit K, Bryan S, Vaca C, Krause L, et al. Effect of two different pre-anaesthetic omeprazole protocols on gastroesophageal reflux incidence and ph in dogs. J Small Anim Pract. (2021) 62:677–82. doi: 10.1111/jsap.13328
280. Panti A, Bennett RC, Corletto F, Brearley J, Jeffery N, Mellanby RJ. The effect of omeprazole on oesophageal ph in dogs during anaesthesia. J Small Anim Pract. (2009) 50:540–4. doi: 10.1111/j.1748-5827.2009.00818.x
281. Wilson DV, Evans AT, Mauer WA. Influence of metoclopramide on gastroesophageal reflux in anesthetized dogs. Am J Vet Res. (2006) 67:26–31. doi: 10.2460/ajvr.67.1.26
282. Lambertini C, Pietra M, Galiazzo G, Torresan F, Pinna S, Pisoni L, et al. Incidence of gastroesophageal reflux in dogs undergoing orthopaedic surgery or endoscopic evaluation of the upper gastrointestinal tract. Vet Sci. (2020) 7. doi: 10.3390/vetsci7040144
283. Tarvin KM, Twedt DC, Monnet E. Prospective controlled study of gastroesophageal reflux in dogs with naturally occurring laryngeal paralysis. Vet Surg. (2016) 45:916–21. doi: 10.1111/vsu.12539
284. Scarpulla G, Camilleri S, Galante P, Manganaro M, Fox M. The impact of prolonged ph measurements on the diagnosis of gastroesophageal reflux disease: 4-day wireless ph studies. Off J Am Coll Gastroenterol. (2007) 102:2642–7. doi: 10.1111/j.1572-0241.2007.01461.x
285. Pandolfino JE, Vela MF. Esophageal-reflux monitoring. Gastrointest Endosc. (2009) 69:917–30. doi: 10.1016/j.gie.2008.09.022
286. Ghoshal U, Singh R. Chapter 9: Catheter-based 24h- pH-metry and Impedance: Technique, Interpretation, and Clinical Application. In: Ghoshal UC, editor. Evaluation of Gastrointestinal Motility and Its Disorders. New Delhi: Springer India (2016). p. 95–106.
287. Sweis R, Fox M, Anggiansah A, Wong T. Prolonged, wireless ph-studies have a high diagnostic yield in patients with reflux symptoms and negative 24-h catheter-based ph-studies. Neurogastroenterol Motil. (2011) 23:419–26. doi: 10.1111/j.1365-2982.2010.01663.x
288. Kessels SJM, Newton SS, Morona JK, Merlin TL. Safety and efficacy of wireless ph monitoring in patients suspected of gastroesophageal reflux disease: a systematic review. J Clin Gastroenterol. (2017) 51:777–88. doi: 10.1097/MCG.0000000000000843
289. Grobman ME, Maitz CA, Reinero CR. Detection of silent reflux events by nuclear scintigraphy in healthy dogs. J Vet Intern Med. (2020) 34:1432–9. doi: 10.1111/jvim.15798
290. Johnson LF, DeMeester TR. Development of the 24-hour intraesophageal ph monitoring composite scoring system. J Clin Gastroenterol. (1986) 8(Suppl. 1):52–8. doi: 10.1097/00004836-198606001-00008
291. Lundell LR, Dent J, Bennett JR, Blum AL, Armstrong D, Galmiche JP, et al. Endoscopic assessment of oesophagitis: clinical and functional correlates and further validation of the los angeles classification. Gut. (1999) 45:172–80. doi: 10.1136/gut.45.2.172
292. Savarino E, Zentilin P, Savarino V. Nerd: an umbrella term including heterogeneous subpopulations. Nat Rev Gastroenterol Hepatol. (2013) 10:371–80. doi: 10.1038/nrgastro.2013.50
293. Muthusamy VR, Lightdale JR, Acosta RD, Chandrasekhara V, Chathadi KV, Eloubeidi MA, et al. The role of endoscopy in the management of gerd. Gastrointest Endosc. (2015) 81:1305–10. doi: 10.1016/j.gie.2015.02.021
294. Wiener GJ, Richter JE, Copper JB, Wu WC, Castell DO. The symptom index: a clinically important parameter of ambulatory 24-hour esophageal ph monitoring. Am J Gastroenterol. (1988) 83:358–61. doi: 10.1007/BF01535789
295. Weusten BL, Roelofs JM, Akkermans LM, Van Berge-Henegouwen GP, Smout AJ. The symptom-association probability: an improved method for symptom analysis of 24-hour esophageal ph data. Gastroenterology. (1994) 107:1741–5. doi: 10.1016/0016-5085(94)90815-X
296. Aanen MC, Weusten BL, Numans ME, de Wit NJ, Samsom M, Smout AJ. Effect of proton-pump inhibitor treatment on symptoms and quality of life in gerd patients depends on the symptom-reflux association. J Clin Gastroenterol. (2008) 42:441–7. doi: 10.1097/MCG.0b013e318074dd62
297. Desjardin M, Luc G, Collet D, Zerbib F. 24-hour ph-impedance monitoring on therapy to select patients with refractory reflux symptoms for antireflux surgery. a single center retrospective study. Neurogastroenterol Motil. (2016) 28:146–52. doi: 10.1111/nmo.12715
298. Zhong C, Duan L, Wang K, Xu Z, Ge Y, Yang C, et al. Esophageal intraluminal baseline impedance is associated with severity of acid reflux and epithelial structural abnormalities in patients with gastroesophageal reflux disease. J Gastroenterol. (2013) 48:601–10. doi: 10.1007/s00535-012-0689-6
299. Kandulski A, Weigt J, Caro C, Jechorek D, Wex T, Malfertheiner P. Esophageal intraluminal baseline impedance differentiates gastroesophageal reflux disease from functional heartburn. Clin Gastroenterol Hepatol. (2015) 13:1075–81. doi: 10.1016/j.cgh.2014.11.033
300. Frazzoni M, Bertani H, Manta R, Mirante VG, Frazzoni L, Conigliaro R, et al. Impairment of chemical clearance is relevant to the pathogenesis of refractory reflux oesophagitis. Dig Liver Dis. (2014) 46:596–602. doi: 10.1016/j.dld.2014.03.005
301. Frazzoni M, Savarino E, de Bortoli N, Martinucci I, Furnari M, Frazzoni L, et al. Analyses of the post-reflux swallow-induced peristaltic wave index and nocturnal baseline impedance parameters increase the diagnostic yield of impedance-ph monitoring of patients with reflux disease. Clin Gastroenterol Hepatol. (2016) 14:40–6. doi: 10.1016/j.cgh.2015.06.026
302. Naini BV, Souza RF, Odze RD. Barrett's esophagus: a comprehensive and contemporary review for pathologists. Am J Surg Pathol. (2016) 40:e45–66. doi: 10.1097/PAS.0000000000000598
303. Tripathi M, Streutker CJ, Marginean EC. Relevance of histology in the diagnosis of reflux esophagitis. Ann N Y Acad Sci. (2018) 1434:94–101. doi: 10.1111/nyas.13742
304. Schneider NI, Plieschnegger W, Geppert M, Wigginghaus B, Hoess GM, Eherer A, et al. Validation study of the esohisto consensus guidelines for the recognition of microscopic esophagitis (histogerd trial). Hum Pathol. (2014) 45:994–1002. doi: 10.1016/j.humpath.2013.12.013
305. Mastracci L, Spaggiari P, Grillo F, Zentilin P, Dulbecco P, Ceppa P, et al. Microscopic esophagitis in gastro-esophageal reflux disease: individual lesions, biopsy sampling, and clinical correlations. Virchows Arch. (2009) 454:31–9. doi: 10.1007/s00428-008-0704-8
306. Calabrese C, Bortolotti M, Fabbri A, Areni A, Cenacchi G, Scialpi C, et al. Reversibility of gerd ultrastructural alterations and relief of symptoms after omeprazole treatment. Am J Gastroenterol. (2005) 100:537–42. doi: 10.1111/j.1572-0241.2005.40476.x
307. Fiocca R, Mastracci L, Engström C, Attwood S, Ell C, Galmiche JP, et al. Long-term outcome of microscopic esophagitis in chronic gerd patients treated with esomeprazole or laparoscopic antireflux surgery in the lotus trial. Am J Gastroenterol. (2010) 105:1015–23. doi: 10.1038/ajg.2009.631
308. Gillen P, Keeling P, Byrne PJ, West AB, Hennessy TP. Experimental columnar metaplasia in the canine oesophagus. Br J Surg. (1988) 75:113–5. doi: 10.1002/bjs.1800750208
309. Van Nieuwenhove Y, Willems G. Gastroesophageal reflux triggers proliferative activity of the submucosal glands in the canine esophagus. Dis Esophagus. (1998) 11:89–93. doi: 10.1093/dote/11.2.89
310. Vakil N, Vieth M, Wernersson B, Wissmar J, Dent J. Diagnosis of gastro-oesophageal reflux disease is enhanced by adding oesophageal histology and excluding epigastric pain. Aliment Pharmacol Ther. (2017) 45:1350–7. doi: 10.1111/apt.14028
311. Vieth M, Mastracci L, Vakil N, Dent J, Wernersson B, Baldycheva I, et al. Epithelial thickness is a marker of gastroesophageal reflux disease. Clin Gastroenterol Hepatol. (2016) 14:1544–51.e1. doi: 10.1016/j.cgh.2016.06.018
312. Laurikainen E, Aitasalo K, Halonen P, Falck B, Kalimo H. Muscle pathology in idiopathic cricopharyngeal dysphagia. Enzyme histochemical and electron microscopic findings. Eur Arch Otorhinolaryngol. (1992) 249:216–23. doi: 10.1007/BF00178473
313. Labeit B, Pawlitzki M, Ruck T, Muhle P, Claus I, Suntrup-Krueger S, et al. The impact of dysphagia in myositis: a systematic review and meta-analysis. J Clin Med. (2020) 9. doi: 10.3390/jcm9072150
314. Sodikoff JB, Lo AA, Shetuni BB, Kahrilas PJ, Yang GY, Pandolfino JE. Histopathologic patterns among achalasia subtypes. Neurogastroenterol Motil. (2016) 28:139–45. doi: 10.1111/nmo.12711
315. Perlman A. Electromyography in oral and pharyngeal motor disorders. GI Motility Online. (2006). doi: 10.1038/gimo32
316. Cuddon PA. Electrophysiology in neuromuscular disease. Vet Clin North Am Small Anim Pract. (2002) 32:31–62. doi: 10.1016/S0195-5616(03)00079-2
317. van Nes JJ. Clinical application of neuromuscular electrophysiology in the dog: a review. Vet Q. (1986) 8:240–50. doi: 10.1080/01652176.1986.9694048
318. Dickinson PJ, LeCouteur RA. Muscle and nerve biopsy. Vet Clin North Am Small Anim Pract. (2002) 32:63–102, vi. doi: 10.1016/S0195-5616(03)00080-9
319. Vaiman M, Eviatar E. Surface electromyography as a screening method for evaluation of dysphagia and odynophagia. Head Face Med. (2009) 5:9. doi: 10.1186/1746-160X-5-9
320. Kantarcigil C, Kim MK, Chang T, Craig BA, Smith A, Lee CH, et al. Validation of a novel wearable electromyography patch for monitoring submental muscle activity during swallowing: a randomized crossover trial. J Speech Lang Hear Res. (2020) 63:3293–3310. doi: 10.1044/2020_JSLHR-20-00171
321. Hellemans J, Vantrappen G. Electromyographic studies on canine esophageal motility. Am J Dig Dis. (1967) 12:1240–55. doi: 10.1007/BF02233925
322. Andrade N, Kent M, Howerth EW, Radlinsky MG. Evaluation of pharyngeal function in dogs with laryngeal paralysis before and after unilateral arytenoid lateralization. Vet Surg. (2015) 44:1021–8. doi: 10.1111/vsu.12410
323. Holland CT, Satchell PM, Farrow BR. Vagal afferent dysfunction in naturally occurring canine esophageal motility disorder. Dig Dis Sci. (1994) 39:2090–8. doi: 10.1007/BF02090355
324. Holland CT, Satchell PM, Farrow BR. Vagal esophagomotor nerve function and esophageal motor performance in dogs with congenital idiopathic megaesophagus. Am J Vet Res. (1996) 57:906–13.
325. Rogers WA, Fenner WR, Sherding RG. Electromyographic and esophagomanometric findings in clinically normal dogs and in dogs with idiopathic megaesophagus. J Am Vet Med Assoc. (1979) 174:181–3.
326. Tan BJ, Diamant NE. Assessment of the neural defect in a dog with idiopathic megaesophagus. Dig Dis Sci. (1987) 32:76–85. doi: 10.1007/BF01296691
327. Yüksel A, Kulan CA, Akçiçek F. The investigation of asymptomatic swallowing disorder through surface electromyography in the geriatric population. Aging Clin Exp Res. (2020) 32:1567–76. doi: 10.1007/s40520-019-01349-6
328. Ahuja NK, Clarke JO. The role of impedance planimetry in the evaluation of esophageal disorders. Curr Gastroenterol Rep. (2017) 19:7. doi: 10.1007/s11894-017-0544-2
329. Savarino E, di Pietro M, Bredenoord AJ, Carlson DA, Clarke JO, Khan A, et al. Use of the functional lumen imaging probe in clinical esophagology. Am J Gastroenterol. (2020) 115:1786–96. doi: 10.14309/ajg.0000000000000773
330. McMahon BP, Frøkjaer JB, Liao D, Kunwald P, Drewes AM, Gregersen H. A new technique for evaluating sphincter function in visceral organs: application of the functional lumen imaging probe (flip) for the evaluation of the oesophago-gastric junction. Physiol Meas. (2005) 26:823–36. doi: 10.1088/0967-3334/26/5/019
331. Pandolfino JE, de Ruigh A, Nicodème F, Xiao Y, Boris L, Kahrilas PJ. Distensibility of the esophagogastric junction assessed with the functional lumen imaging probe (flip™) in achalasia patients. Neurogastroenterol Motil. (2013) 25:496–501. doi: 10.1111/nmo.12097
332. Carlson DA, Lin Z, Rogers MC, Lin CY, Kahrilas PJ, Pandolfino JE. Utilizing functional lumen imaging probe topography to evaluate esophageal contractility during volumetric distention: a pilot study. Neurogastroenterol Motil. (2015) 27:981–9. doi: 10.1111/nmo.12572
333. Carlson DA, Kahrilas PJ, Lin Z, Hirano I, Gonsalves N, Listernick Z, et al. Evaluation of esophageal motility utilizing the functional lumen imaging probe. Am J Gastroenterol. (2016) 111:1726–35. doi: 10.1038/ajg.2016.454
334. Carlson DA, Lin Z, Kahrilas PJ, Sternbach J, Donnan EN, Friesen L, et al. The functional lumen imaging probe detects esophageal contractility not observed with manometry in patients with achalasia. Gastroenterology. (2015) 149:1742–51. doi: 10.1053/j.gastro.2015.08.005
335. Hirano I, Pandolfino JE, Boeckxstaens GE. Functional lumen imaging probe for the management of esophageal disorders: expert review from the clinical practice updates committee of the aga institute. Clin Gastroenterol Hepatol. (2017) 15:325–34. doi: 10.1016/j.cgh.2016.10.022
336. Ng K, Mogul D, Hollier J, Khashab MA. Utility of functional lumen imaging probe in esophageal measurements and dilations: a single pediatric center experience. Surg Endoscopy. (2020) 34:1294–9. doi: 10.1007/s00464-019-06898-5
337. Wu PI, Szczesniak MM, Maclean J, Choo L, Quon H, Graham PH, et al. Clinical utility of a functional lumen imaging probe in management of dysphagia following head and neck cancer therapies. Endoscopy. (2017) 49:848–54. doi: 10.1055/s-0043-110670
338. Carlson DA, Lin Z, Hirano I, Gonsalves N, Zalewski A, Pandolfino JE. Evaluation of esophageal distensibility in eosinophilic esophagitis: an update and comparison of functional lumen imaging probe analytic methods. Neurogastroenterol Motil. (2016) 28:1844–53. doi: 10.1111/nmo.12888
339. Lottrup C, McMahon BP, Ejstrud P, Ostapiuk MA, Funch-Jensen P, Drewes AM. Esophagogastric junction distensibility in hiatus hernia. Dis Esophagus. (2016) 29:463–71. doi: 10.1111/dote.12344
340. Tucker E, Sweis R, Anggiansah A, Wong T, Telakis E, Knowles K, et al. Measurement of esophago-gastric junction cross-sectional area and distensibility by an endolumenal functional lumen imaging probe for the diagnosis of gastro-esophageal reflux disease. Neurogastroenterol Motil. (2013) 25:904–10. doi: 10.1111/nmo.12218
341. Conte A, Morabito S, Dennis R, Murgia D. Computed tomographic comparison of esophageal hiatal size in brachycephalic and non-brachycephalic breed dogs. Vet Surg. (2020) 49:1509–16. doi: 10.1111/vsu.13521
342. Wang RH. From reflux esophagitis to barrett's esophagus and esophageal adenocarcinoma. World J Gastroenterol. (2015) 21:5210–9. doi: 10.3748/wjg.v21.i17.5210
343. Kapoor H, Lohani KR, Lee TH, Agrawal DK, Mittal SK. Animal models of barrett's esophagus and esophageal adenocarcinoma-past, present, and future. Clin Transl Sci. (2015) 8:841–7. doi: 10.1111/cts.12304
344. Su Y, Chen X, Klein M, Fang M, Wang S, Yang CS, et al. Phenotype of columnar-lined esophagus in rats with esophagogastroduodenal anastomosis: similarity to human barrett's esophagus. Lab Investig. (2004) 84:753–65. doi: 10.1038/labinvest.3700079
345. Bremner CG, Shorter RG, Ellis FH Jr. Anatomy of feline esophagus with special reference to its muscular wall and phrenoesophageal membrane. J Surg Res. (1970) 10:327–31. doi: 10.1016/0022-4804(70)90051-X
346. Marklin GF, Krause WJ, Cutts JH. Structure of the esophagus in the adult opossum, didelphis virginiana. Anat Anz. (1979) 145:249–61.
347. Wu M, Majewski M, Wojtkiewicz J, Vanderwinden JM, Adriaensen D, Timmermans JP. Anatomical and neurochemical features of the extrinsic and intrinsic innervation of the striated muscle in the porcine esophagus: evidence for regional and species differences. Cell Tissue Res. (2003) 311:289–97. doi: 10.1007/s00441-002-0696-7
348. Brown FC, Gideon RM, Voelker FA, Castell DO. Muscle function and structure of the esophagus of the baboon (papio anubis). Am J Vet Res. (1978) 39:1209–11.
349. Geboes K, Desmet V. Histology of the esophagus. Front Gastrointest Res. (1978) 3:1–17. doi: 10.1159/000400844
350. Rubio CA, Nilsson JR, Owston M, Dick EJ Jr. The length of the barrett's mucosa in baboons, revisited. Anticancer Res. (2012) 32:3115–8.
351. Bremner CG, Lynch VP, Ellis FH Jr. Barrett's esophagus: congenital or acquired? An experimental study of esophageal mucosal regeneration in the dog. Surgery. (1970) 68:209–16.
352. Kawaura Y, Tatsuzawa Y, Wakabayashi T, Ikeda N, Matsuda M, Nishihara S. Immunohistochemical study of p53, c-erbb-2, and pcna in barrett's esophagus with dysplasia and adenocarcinoma arising from experimental acid or alkaline reflux model. J Gastroenterol. (2001) 36:595–600. doi: 10.1007/s005350170042
353. Narbona-Arnau B, Argente-Navarro P, Lloris-Carsi JM, Calvo-Bermudez MA, Cejalvo-Lapena D. Experimental model of barrett esophagus in dogs. J Chir. (1994) 131:261–5.
354. Kumagai H, Mukaisho K, Sugihara H, Bamba M, Miyashita T, Miwa K, et al. Cell kinetic study on histogenesis of barrett's esophagus using rat reflux model. Scand J Gastroenterol. (2003) 38:687–92. doi: 10.1080/00365520310003435
355. Levrat M, Lambert R, Kirshbaum G. Esophagitis produced by reflux of duodenal contents in rats. Am J Dig Dis. (1962) 7:564–73. doi: 10.1007/BF02236137
356. Kadirkamanathan SS, Yazaki E, Evans DF, Hepworth CC, Gong F, Swain CP. An ambulant porcine model of acid reflux used to evaluate endoscopic gastroplasty. Gut. (1999) 44:782–8. doi: 10.1136/gut.44.6.782
357. Poplawski C, Sosnowski D, Szaflarska-Popławska A, Sarosiek J, McCallum R, Bartuzi Z. Role of bile acids, prostaglandins and cox inhibitors in chronic esophagitis in a mouse model. World J Gastroenterol. (2006) 12:1739–42. doi: 10.3748/wjg.v12.i11.1739
358. Li Y, Wo JM, Ellis S, Ray MB, Jones W, Martin RC. A novel external esophageal perfusion model for reflux esophageal injury. Dig Dis Sci. (2006) 51:527–32. doi: 10.1007/s10620-006-3165-4
359. McMahon RL, Ali A, Chekan EG, Clary EM, Garcia-Oria MJ, Fina MC, et al. A canine model of gastroesophageal reflux disease (gerd). Surg Endosc. (2002) 16:67–74. doi: 10.1007/s004640080153
360. Gibson CJ, Parry NM, Jakowski RM, Cooper J. Adenomatous polyp with intestinal metaplasia of the esophagus (barrett esophagus) in a dog. Vet Pathol. (2010) 47:116–9. doi: 10.1177/0300985809352973
361. Kopke MA, Munday JS, Gal A. Carcinoma in situ within an area of barrett esophagus in a dog with megaesophagus. J Vet Diagn Invest. (2018) 30:752–4. doi: 10.1177/1040638718786330
362. Lewis TW, Wiles BM, Llewellyn-Zaidi AM, Evans KM, O'Neill DG. Longevity and mortality in kennel club registered dog breeds in the uk in 2014. Canine Genet Epidemiol. (2018) 5:10. doi: 10.1186/s40575-018-0066-8
363. Hendricks JC, Kline LR, Kovalski RJ, O'Brien JA, Morrison AR, Pack AI. The english bulldog: a natural model of sleep-disordered breathing. J Appl Physiol. (1987) 63:1344–50. doi: 10.1152/jappl.1987.63.4.1344
364. Bhatia R, Pagala M, Vaynblat M, Marcus M, Kazachkov M. Intrathoracic airway obstruction and gastroesophageal reflux: a canine model. Pediatr Pulmonol. (2012) 47:1097–102. doi: 10.1002/ppul.22510
365. Kaye BM, Rutherford L, Perridge DJ, Ter Haar G. Relationship between brachycephalic airway syndrome and gastrointestinal signs in three breeds of dog. J Small Animal Prac. (2018) 59:670–3. doi: 10.1111/jsap.12914
366. Haimel G, Dupré G. Brachycephalic airway syndrome: a comparative study between pugs and french bulldogs. J Small Animal Prac. (2015) 56:714–9. doi: 10.1111/jsap.12408
367. Darcy HP, Humm K, Ter Haar G. Retrospective analysis of incidence, clinical features, potential risk factors, and prognostic indicators for aspiration pneumonia in three brachycephalic dog breeds. J Am Vet Med Assoc. (2018) 253:869–76. doi: 10.2460/javma.253.7.869
368. Lux CN, Archer TM, Lunsford KV. Gastroesophageal reflux and laryngeal dysfunction in a dog. J Am Vet Med Assoc. (2012) 240:1100–3. doi: 10.2460/javma.240.9.1100
369. Duke SG, Postma GN, McGuirt WF Jr, Ririe D, Averill DB, et al. Laryngospasm and diaphragmatic arrest in immature dogs after laryngeal acid exposure: A possible model for sudden infant death syndrome. Ann Otol Rhinol Laryngol. (2001) 110:729–33. doi: 10.1177/000348940111000806
370. Määttä OLM, Laurila HP, Holopainen S, Lilja-Maula L, Melamies M, Viitanen SJ, et al. Reflux aspiration in lungs of dogs with respiratory disease and in healthy west highland white terriers. J Vet Intern Med. (2018) 32:2074–81. doi: 10.1111/jvim.15321
371. Gaude GS. Pulmonary manifestations of gastroesophageal reflux disease. Ann Thorac Med. (2009) 4:115–23. doi: 10.4103/1817-1737.53347
372. Lu Q, Zhang L, Zhao C, Jin H, Wang B, Yadid-Pecht O, et al. Catheter-based acoustic interrogation device for real-time monitoring of the dynamics of the lower esophageal sphincter: in vitro and pilot canine studies. Physiol Meas. (2015) 36:2471–82. doi: 10.1088/0967-3334/36/12/2471
373. Guo Z, Wu Y, Chen J, Zhang S, Zhang C. The role of salivary pepsin in the diagnosis of gastroesophageal reflux disease (gerd) evaluated using high-resolution manometry and 24-hour multichannel intraluminal impedance-ph monitoring. Med Sci Monit. (2020) 26:e927381. doi: 10.12659/MSM.927381
374. Grobman M, Rindt H, Reinero CR. Proteomic characterization of canine gastric fluid by liquid chromatography-mass spectrometry for development of protein biomarkers in regurgitation, vomiting, and cough. Front Vet Sci. (2021) 8:687. doi: 10.3389/fvets.2021.670007
375. Smith JA, Decalmer S, Kelsall A, McGuinness K, Jones H, Galloway S, et al. Acoustic cough-reflux associations in chronic cough: potential triggers and mechanisms. Gastroenterology. (2010) 139:754–62. doi: 10.1053/j.gastro.2010.06.050
376. Lehmann A, Hansson-Brändén L, Kärrberg L. Effects of repeated administration of baclofen on transient lower esophageal sphincter relaxation in the dog. Eur J Pharmacol. (2000) 403:163–7. doi: 10.1016/S0014-2999(00)00528-8
377. Marsh RE, Awad ZT, Cornet DA, Tomonaga T, Smyrk T, Filipi CJ. Endoscopic sis injection into the lower oesophageal sphincter in dogs. Ir J Med Sci. (2003) 172:20–3. doi: 10.1007/BF02914780
378. Sanmiguel CP, Hagiike M, Mintchev MP, Cruz RD, Phillips EH, Cunneen SA, et al. Effect of electrical stimulation of the les on les pressure in a canine model. Am J Physiol Gastrointest Liver Physiol. (2008) 295:G389–94. doi: 10.1152/ajpgi.90201.2008
379. Naranjo JD, Saldin LT, Sobieski E, Quijano LM, Hill RC, Chan PG, et al. Esophageal extracellular matrix hydrogel mitigates metaplastic change in a dog model of barrett's esophagus. Sci Adv. (2020) 6:eaba4526. doi: 10.1126/sciadv.aba4526
380. Nieponice A, McGrath K, Qureshi I, Beckman EJ, Luketich JD, Gilbert TW, et al. An extracellular matrix scaffold for esophageal stricture prevention after circumferential emr. Gastrointest Endosc. (2009) 69:289–96. doi: 10.1016/j.gie.2008.04.022
381. Yang GP, Soetikno RM. Treatment of oesophageal ulcerations using endoscopic transplantation of tissue-engineered autologous oral mucosal epithelial cell sheets in a canine model. Gut. (2007) 56:313–4. doi: 10.1136/gut.2006.100073
382. Tan B, Wei RQ, Tan MY, Luo JC, Deng L, Chen XH, et al. Tissue engineered esophagus by mesenchymal stem cell seeding for esophageal repair in a canine model. J Surg Res. (2013) 182:40–8. doi: 10.1016/j.jss.2012.07.054
383. Tan B, Wang M, Chen X, Hou J, Chen X, Wang Y, et al. Tissue engineered esophagus by copper–small intestinal submucosa graft for esophageal repair in a canine model. Sci China Life Sci. (2014) 57:248–55. doi: 10.1007/s11427-013-4603-0
384. Nakase Y, Nakamura T, Kin S, Nakashima S, Yoshikawa T, Kuriu Y, et al. Intrathoracic esophageal replacement by in situ tissue-engineered esophagus. J Thorac Cardiovasc Surg. (2008) 136:850–9. doi: 10.1016/j.jtcvs.2008.05.027
385. Jobe BA, O'Rourke RW, McMahon BP, Gravesen F, Lorenzo C, Hunter JG, et al. Transoral endoscopic fundoplication in the treatment of gastroesophageal reflux disease: the anatomic and physiologic basis for reconstruction of the esophagogastric junction using a novel device. Ann Surg. (2008) 248:69–76. doi: 10.1097/SLA.0b013e31817c9630
386. Dai JG, Liu QX, Den XF, Min JX. Oesophageal flap valvuloplasty and wrapping suturing prevent gastrooesophageal reflux disease in dogs after oesophageal anastomosis. World J Gastroenterol. (2014) 20:17434–8. doi: 10.3748/wjg.v20.i46.17434
387. Kornegay JN, Bogan JR, Bogan DJ, Childers MK, Li J, Nghiem P, et al. Canine models of duchenne muscular dystrophy and their use in therapeutic strategies. Mamm Genome. (2012) 23:85–108. doi: 10.1007/s00335-011-9382-y
388. Wasala NB, Hakim CH, Chen SJ, Yang NN, Duan D. Questions answered and unanswered by the first crispr editing study in a canine model of duchenne muscular dystrophy. Hum Gene Ther. (2019) 30:535–43. doi: 10.1089/hum.2018.243
389. Lin S, Gao P, Li Q, Zhang Y, Hu J, Cai M, et al. Aflatoxin influences achalasia symptomatology. Mol Med Rep. (2020) 21:1276–84. doi: 10.3892/mmr.2020.10914
390. Hurley KJ, Mansfield C, VanHoutan IM, Lacorcia L, Allenspach K, Hebbard G, et al. A comparative analysis of two unrelated outbreaks in latvia and australia of acquired idiopathic megaesophagus in dogs fed two brands of commercial dry dog foods: 398 cases (2014-2018). J Am Vet Med Assoc. (2021) 259:172–83. doi: 10.2460/javma.259.2.172
391. Baumann AJ, Carlson DA. Esoflip for esophageal dilation: proposed advantages. Curr Opin Gastroenterol. (2020) 36:329–35. doi: 10.1097/MOG.0000000000000639
Keywords: esophageal anatomy, physiology, dysphagia, fluoroscopy, manometry, EndoFLIP®, gastroesophageal reflux
Citation: Ullal TV, Marks SL, Belafsky PC, Conklin JL and Pandolfino JE (2022) A Comparative Assessment of the Diagnosis of Swallowing Impairment and Gastroesophageal Reflux in Canines and Humans. Front. Vet. Sci. 9:889331. doi: 10.3389/fvets.2022.889331
Received: 04 March 2022; Accepted: 05 May 2022;
Published: 09 June 2022.
Edited by:
Thomas Schermerhorn, Kansas State University, United StatesReviewed by:
Annamaria Grandis, Università di Bologna, ItalyMegan Grobman, Auburn University, United States
Copyright © 2022 Ullal, Marks, Belafsky, Conklin and Pandolfino. This is an open-access article distributed under the terms of the Creative Commons Attribution License (CC BY). The use, distribution or reproduction in other forums is permitted, provided the original author(s) and the copyright owner(s) are credited and that the original publication in this journal is cited, in accordance with accepted academic practice. No use, distribution or reproduction is permitted which does not comply with these terms.
*Correspondence: Stanley L. Marks, slmarks@ucdavis.edu