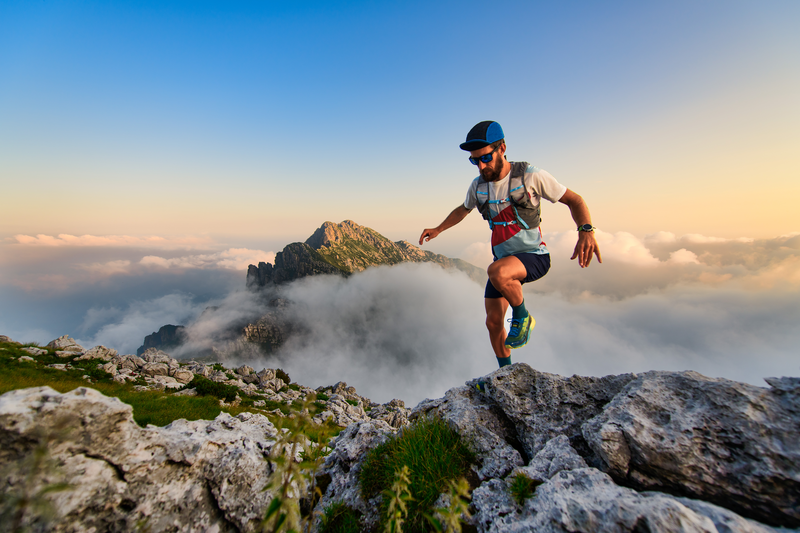
94% of researchers rate our articles as excellent or good
Learn more about the work of our research integrity team to safeguard the quality of each article we publish.
Find out more
ORIGINAL RESEARCH article
Front. Vet. Sci. , 06 June 2022
Sec. Animal Nutrition and Metabolism
Volume 9 - 2022 | https://doi.org/10.3389/fvets.2022.884945
This article is part of the Research Topic Inflammation and Immunomodulation in Veterinary Medicine View all 11 articles
This study was to investigate effects of zinc oxide nanoparticle (Nano-ZnO) on growth, immunity, intestinal morphology and function of intrauterine growth retardation (IUGR) finishing pigs. Six normal birth weight (NBW) and 12 IUGR male piglets were obtained and weaned at 21 d. NBW-weaned piglets fed basal diets (NBW group), IUGR-weaned piglets allocated to two groups fed basal diets (IUGR group) and basal diets further supplemented 600 mg Zn/kg from Nano-ZnO (IUGR+Zn group), respectively. All pigs were slaughtered at 163 d. Results showed: (1) IUGR pigs showed no difference in body weight at 77d and 163d (P > 0.05), while had increased villus height (VH) and villus surface area in jejunum (P < 0.05) and enhanced interleukin-6, TNF-α and NF-κB mRNA expression (P < 0.05) as compared to NBW group; Compared with IUGR group, dietary Nano-ZnO did not affect the body weight (P > 0.05), but increased VH to crypt depth ratio and IgA concentration (P < 0.05) and decreased TNF-α and NF-κB mRNA expression in jejunum (P < 0.05). (2) IUGR increased the number of swollen mitochondria and autolysosomes, and protein expressions of sequestosome-1 (P62) and microtubule-associated protein light chain 3 B/A (LC3B/A) in jejunum as compared to NBW group (P < 0.05); Compared with IUGR group, Nano-ZnO decreased the number of swollen mitochondria and autolysosomes, and P62 and LC3B/A protein expression (P < 0.05). (3) IUGR increased mucosal contents of malondialdehyde and protein carbonyl (PC) and Keap1 protein expression (P < 0.05) as compared to NBW group; Compared with IUGR group, dietary Nano-ZnO increased activities of total antioxidant capacity, catalase, glutathione peroxidase, and glutathione content (P < 0.05), and enhanced nuclear respiratory factor 2 (Nrf2), glutamate-cysteine ligase modifier subunit and glutathione peroxidase 1 mRNA expression, and increased total and nuclear Nrf2 protein expression (P < 0.05), and decreased malondialdehyde and PC content, and Keap1 protein expression (P < 0.05) in jejunum. Results suggested that IUGR pigs showed postnatal catch-up growth and improved intestinal morphology, and dietary Nano-ZnO may further improve intestinal morphology, reduce inflammation, decrease autophagy and alleviate oxidative stress via Nrf2/Keap1 pathway in jejunum of IUGR pigs.
Intrauterine growth retardation (IUGR) is a severe obstetric syndrome mainly caused by a fetal lack of nutrition or oxygen supply in the womb during pregnancy resulting in the hindrance of fetal growth and development in utero (1, 2). The incidence of IUGR in human fetuses is about 5%, and patients with IUGR usually have insulin resistance in the clinic, and may subsequently develop metabolic syndrome, such as type 2 diabetes mellitus and obesity, in adulthood (3–5). The natural incidence of IUGR in pigs can be as high as 15–20% in modern highly intensive farming patterns (6), and IUGR results in a low survival rate and has a significant negative impact on the growth performance of the piglets. However, IUGR pigs may show postnatal catch-up growth in body weight, but with oxidative stress, inflammation, obesity, and a higher risk of cardiovascular disease (7–9).
The jejunum is the principal place for nutrient digestion and absorption, and it is also one of the main organs of the body to participate in immune and inflammatory responses. Many studies have reported that IUGR can damage the intestinal morphology, reduce the immune ability and cause inflammation, and decrease in intestinal weight, villus height (VH), and the villus height to crypt depth ratio (VH/CD) in piglets, as well as increase crypt depth (CD) (10, 11). IUGR can also cause intestinal inflammation and decrease immunity, and induce oxidative stress in growing pigs (12). However, few studies have examined the effects of IUGR on the immune status and intestinal function of finishing pigs, despite the urgency of finding effective nutritional guidelines for IUGR pigs.
One nutrient that can maintain normal life activities of animals and is indispensable for all living organisms is zinc, a mineral element that needs to be consumed regularly to keep the animal healthy (13). The absorption rate of ordinary zinc oxide added to pig feed is very low, and most of the dietary zinc oxide will be excreted in the feces, with potential environmental pollution issues and resource waste (14). The rise of nanotechnology has led to the emergence of a variety of nanomaterials, including those containing zinc, in a historic moment. Zinc oxide nanoparticles (Nano-ZnOs) have high biological activity, antibacterial action and antioxidant properties and may be used as a source of zinc in feed. As the name implies, the size of Nano-ZnO is in the range of 1–100nm, and this size affects the physical and chemical properties through surface effects, quantum size effects, volume effects and macroscopic tunneling effects (15). Dietary supplementation of 400–800 mg/kg Nano-ZnO can improve growth performance, reduce diarrhea and regulate the intestinal microbiota of weaned piglets, and show similar positive effects to 3,000 mg/kg traditional zinc oxide (16, 17). The beneficial effects of dietary 1,200 mg/kg Nano-ZnO on weaned piglets are similar to those of high dose of traditional zinc oxide plus antibiotics (3000 mg/kg ZnO + 20 mg/kg colistin sulfate) (18). Therefore, Nano-ZnO shows substantial promise for applications in the animal husbandry industry.
Given these anticipated potential positive effects of Nano-ZnO, we speculated that Nano-ZnO might show beneficial effects in IUGR pigs. Therefore, this study was conducted to investigate the effects of Nano-ZnO on the growth, immune status, jejunal morphology, antioxidant status, inflammation-related mRNA expression, mitochondrial ultrastructure, and autophagy in IUGR pigs.
This study was approved and conducted under the supervision of the Institutional Animal Care and Use Committee of Nanjing Agricultural University (Jiangsu, China. Permit number SYXK-2019-00142).
The NBW and IUGR piglets were selected according to previous studies (19, 20). A total of 18 male piglets [Duroc × (Landrace × Yorkshire)] were selected from 6 sows with similar parity and litter size. The 6 NBW piglets (1.52 ± 0.01 kg) were separated into an NBW group and the 12 IUGR piglets (0.96 ± 0.02 kg) were randomly assigned to an IUGR group and an IUGR+Zn group. All piglets were weaned at 21 days of age and piglets in the NBW group and the IUGR group were fed a basal diet, whereas piglets in the IUGR+Zn group was fed the same basal diet supplemented with 600 mg Zn/kg from Nano-ZnO. Each treatment had six replicates (pens), and each replicate had 1 piglet. The effective supplemental level of Nano-ZnO was selected according to our previous study (16). The Nano-ZnO (95% purity) used in present study was the same as that used in our previous study (16) and was provided by Zhangjiagang Bonded Area Hualu Nanometer Material Co., Ltd. (Jiangsu, China).
After weaning, all piglets were fed in single slatted-floor cages at the same temperature (nursery period temperature was 26–30°C, fattening period was 20–24°C) and humidity (50–60%). Pigs were provided ad libitum access to feed and water throughout the trial period. Water supply, cage temperature and humidity, and the pig's physiology were checked twice a day. The piggery was cleaned and disinfected regularly, and the usual immunization program was maintained. The weight of each pig at days 1, 21, 77, and 163 was recorded, and the feed intake of each pig was accurately recorded to calculate the average daily gain (ADG), average daily feed intake (ADFI) and the gain-to-feed ratio (G:F) during 21 to 163 d.
After fasting for 12 h at the end of the feeding period (at 163 days of age), blood samples were obtained from each pig's precaval vein and centrifuged at 3,000 g for 10 min at 4°C to collect serum, which was then stored at −80°C for analysis. Subsequently, the pigs were euthanized by electrical stunning and exsanguinated. The jejunum samples (near the midpoint of jejunum) were collected from each pig for intestinal morphology analysis (fixed in 4% paraformaldehyde) and transmission electron microscope index (fixed in 2.5% glutaraldehyde solution), respectively. The jejunal mucosa was scraped, quickly frozen in liquid nitrogen and then stored at −80°C for further analysis.
The content of serum growth hormone (GH, MM-039701), insulin like growth factor 1 (IGF-1, MM-259701), immunoglobulin A (IgA, MM-090501), immunoglobulin G (IgG, MM-040301), immunoglobulin M (IgM, MM-040201) were determined via the enzyme-linked immunosorbent assay (ELISA) kits purchased from Jiangsu MeiMian Industrial Co., LTD (Jiangsu, China).
The jejunal morphology was analyzed according a previous method reported by Dong et al. (21). Briefly, the fixed jejunum in 4% paraformaldehyde was dehydrated and impregnated with wax to prepare the section in thickness of 5 μm, and stained with hematoxylin and eosin (HE). All sections were captured by optical binocular microscope (Olympus BX5, Olympus Optical Co. Ltd, Japan) and digital camera (Nikon H550L). The VH, CD and villous width (VW) were measured with Image-Pro Plus soft-ware. The VH/CD and villous surface area (VSA) were calculated.
The fixed jejunum sample in 2.5% glutaraldehyde solution (24 h, 4°C) was further fixed in 1% osmium for 1 h, dehydrated with a series of hierarchical ethanol solutions and then fixed in epoxy resin. The sections were cut with ultrafine slicing machine (Boeckeler, Tucson, AZ, USA), photographed using a transmission electron microscope (Hitachi H-7650, Tokyo, Japan). The swollen mitochondria and autolysosome number was analyzed according to the methods in previous studies (22–24).
With corresponding commercial kits purchased from Nanjing JianCheng Bioengineering Institute (Jiangsu, China), the concentrations of malondialdehyde (MDA, A003-1-2), protein carbonyl (PC, A087-1), total protein (A045-3-2), and glutathione (GSH, A005-1-2), as well as the activities of total antioxidant capacity (T-AOC, A015-2-1), catalase (CAT, A007-1-1), total superoxide dismutase (T-SOD, A001-1-1) and glutathione peroxidase (GSH-Px, A005-1-2) were determined. T-AOC, SOD, CAT, and GSH-Px activities were expressed in units (U) per milligram protein. MDA and PC concentrations were expressed in nanomole per milligram of protein. The GSH concentration was expressed in milligrams per gram of protein. The contents of IgG and IgA in intestinal mucosa were determined with corresponding commercial kits (Jiangsu MeiMian Industrial Co., Ltd, Jiangsu, China).
With the previous methods (16), we extracted the total RNA from jejunal mucosa, obtained the cDNA samples, and then reverse transcription polymerase chain reaction (RT-PCR) and the 2−ΔΔCt method (25) were used to determine the relative mRNA expression. The specific primers of selected genes used in present study have been listed in Table 1, which are related to the inflammation [interleukin-1β (IL-1β), interleukin-4 (IL-4), interleukin-6 (IL-6), interleukin-10 (IL-10), tumor necrosis factor-α (TNF-α), interferon-γ (IFN-γ), nuclear factor kappa-B (NF-κB)], and antioxidant capacity [nuclear respiratory factor 2 (Nrf2), kelch like ECH associated protein 1 (Keap1), glutamate-cysteine ligase catalytic subunit (GCLC), glutamate-cysteine ligase modifier subunit (GCLM), superoxide dismutase 1 (SOD1), glutathione peroxidase 1 (GPx1)]. These primers were used in our previous studies (9, 12, 16), or designed and synthesized in a commercial company (Beijing Qingke Biological Technology Co., Ltd., Beijing, China). The β-actin was used as an internal reference gene (12, 20).
The western blot analysis in jejunal mucosa was conducted according to previous studies (19, 26). The primary antibodies including Nrf2 (1:1,500), Keap1 (1:6,000), and P62 (1:4,000) were provided by Proteintech Group, Inc., (Rosemont, USA) and antibodies including microtubule-associated protein light chain 3 (LC3, 1:1,000) and β-actin (1:1,000) were provided by Cell Signaling (Danvers, Massachusetts, USA). The secondary antibody (1:5,000; Proteintech Group, Inc., Rosemount, IL, USA) was horseradish peroxidase labeled goat anti-rabbit immunoglobulin G in present study. The β-actin was used as the internal reference protein. For the protein expression of nuclear Nrf2, the protein used for western blot analysis was first extracted from the nuclei of jejunal mucosa with the nuclear extraction kit (SN0020, Beijing Solarbio Science&Technology Co., Ltd, China).
The data were processed with SPSS software (SPSS, ver. 22.0 for Windows, SPSS Inc., Chicago, USA), and statistical differences between groups were assessed using one-way analysis of variance and Tukey's post-hoc test for pairwise comparison. The data is presented as means and standard error of the mean (SEM), with a P-value of <0.05 indicating a statistically significant difference.
As shown in Table 2, body weights of pigs in the IUGR group were significantly lower at 1 d and 21 d (P < 0.05), but showed no significant difference at 77d and 163 d as compared with the NBW group (P > 0.05); body weights of pigs in the IUGR+Zn group were not significant different from that in the IUGR group (P > 0.05), but were significantly lower than that in the NBW group during this whole feeding experiment (P < 0.05). The ADG of pigs in the IUGR and IUGR+Zn group was lower than that of the NBW group during 1 to 21d (P < 0.05). During 21 to 77 d and 77 to 163 d, no significant differences in ADG, ADFI and G:F were observed between the IUGR and NBW groups (P > 0.05). Compared to the IUGR group, dietary 600 mg from Zn/kg Nano-ZnO did not affect the ADG, and G:F (P > 0.05), but significantly decreased ADFI of IUGR pigs during 77 to 163 d (P < 0.05). Moreover, pigs in the IUGR+Zn group had significantly lower ADG and ADFI during 77 to 163 d than that of the NBW group (P < 0.05).
As shown in Table 3 and Figure 1, pigs in the IUGR group showed significantly higher VH, CD and VSA (P < 0.05), but had no significant difference in VW and VH/CD as compared to the NBW group (P > 0.05). Compared with the IUGR group, dietary added 600 mg Zn/kg from Nano-ZnO significantly lowered CD and increased VH/CD (P < 0.05), but did not affect the VH and VW of jejunum (P > 0.05). Compared to the NBW group, pigs in IUGR+Zn group had significantly higher VH, VH/CD and VSA (P < 0.05), and showed no significant difference in VW and CD (P > 0.05).
Figure 1. Effects of dietary Nano-ZnO supplementation on the jejunal histomorphology in IUGR finishing pigs. All samples were stained with hematoxylin and eosin (H&E). Scale bar represents 500 μm. NBW, images from normal birth weight pigs; IUGR, images from intrauterine growth restriction pigs; IUGR+Zn, images from IUGR pigs fed with diets supplemented with 600 mg Zn/kg from Nano-ZnO.
As shown in Figure 2, the mitochondria of jejunal enterocytes in the NBW group were clear, intact, and regular cristae. By contrast, the IUGR pigs had quite a few abnormal mitochondria in morphology, with poor integrity, and significantly increased the number of swollen mitochondria and autolysosomes (P < 0.05). However, compared with the IUGR group, dietary added 600 mg Zn/kg from Nano-ZnO effectively improved mitochondrial abnormalities, decreased the number of swollen mitochondria and autolysosomes in jejunal enterocytes of IUGR pig (P < 0.05). Moreover, pigs in the IUGR+Zn group showed significantly reduced number of autolysosomes (P < 0.05), but no significant differences in number of swollen mitochondria (P > 0.05) as compared to pigs in the NBW group.
Figure 2. Effects of dietary Nano-ZnO supplementation on the jejunal mitochondrial ultrastructure of IUGR finishing pigs. (A) Representative image of jejunum using transmission electron microscopy, red arrows indicated autolysosome, blue arrows indicated the swollen mitochondria. (B) Quantitative analysis of swollen mitochondria and autolysosome of pigs' jejunal mucosa under transmission electron microscopy images (based on 3,000 times magnification, analysis of 4 images in each group, n = 4). Results were normalized by the mean value for the NBW group set to 1 unit. a,bMeans for the same parameter with different superscripts are significantly different (P < 0.05). NBW, normal birth weight pigs; IUGR, intrauterine growth restriction pigs; IUGR+Zn, IUGR pigs fed with diets supplemented with 600 mg Zn/kg from Nano-ZnO.
No significant changes were obtained in concentrations of IGF-1, GH, IgA, IgG and IgM among the NBW, IUGR and IUGR+Zn groups (P > 0.05, Table 4).
Table 4. Effects of dietary Nano-ZnO supplementation on serum hormones parameters and immune status of the IUGR finishing pigs.
As shown in Table 5, IUGR finishing pigs exhibited significantly increased MDA and PC content (P < 0.05), but no significant difference in CAT, T-AOC, T-SOD, GSH-Px activity and GSH level in the jejunal mucosa compared with pigs in the NBW group (P > 0.05). Compared to the IUGR group, dietary added 600 mg Zn/kg from Nano-ZnO significantly lowered the MDA and PC content (P < 0.05) and enhanced the CAT, T-AOC, GSH-Px activity and GSH content (P < 0.05) in the jejunal mucosa of IUGR pigs, but did not significantly alter the T-SOD activity (P > 0.05).
Table 5. Effects of dietary Nano-ZnO supplementation on the antioxidant status of jejunal mucosa of the IUGR finishing pigs.
Results of Table 6, showed that no significant changes in mucosal concentration of IgG among the NBW, IUGR, and IUGR+Zn groups (P > 0.05), while dietary added 600 mg Zn/kg from Nano-ZnO significantly decreased the IgA content in jejunal mucosa of IUGR pigs (P < 0.05). No significant change in IgA in jejunal mucosa was obtained between the NBW and IUGR+Zn group (P > 0.05).
Table 6. Effects of dietary Nano-ZnO supplementation on immune status of jejunal mucosa in IUGR finishing pigs.
As showed in Figure 3, compared with the NBW group, IUGR finishing pigs had significantly enhanced mRNA expression of IL-6, TNF-α and NF-κB (P < 0.05), and no significant differences in the mRNA expression of Nrf2, Keap1, HO-1, GCLC, GCLM, SOD1, GPx1, IL-1β, IL-4, IL-10 and IFN-γ (P > 0.05). Compared with the IUGR group, dietary added 600 mg Zn/kg from Nano-ZnO significantly enhanced the mRNA expression of Nrf2, GCLM, GPx1 and IL-10 (P < 0.05), and reduce the mRNA expression of TNF-α and NF-κB in jejunal mucosa of IUGR pigs (P < 0.05), while did not significantly alter the mRNA expression of Keap1, HO-1, GCLC, SOD1, IL-1β, IL-4, IL-6 and IFN-γ (P > 0.05). The mRNA expression of GCLM and GPx1 significantly enhanced in the IUGR+Zn group (P < 0.05) as compared with the NBW group, while no significant difference in the other selected antioxidant and inflammation related mRNA expression was observed (P > 0.05).
Figure 3. Effects of dietary Nano-ZnO supplementation on the mRNA expressions in jejunal mucosa of finishing pigs. (A) Inflammation related mRNA expression. (B) Antioxidant related mRNA expression. Data are normalized to the NBW group and expressed as means with their SEM (n = 6). a,bMeans for the same parameter with different superscripts are significantly different (P < 0.05). IL-1β, interleukin-1β; IL-4, interleukin-4; IL-6, interleukin-6; IL-10, interleukin-10; TNF-α, tumor necrosis factor-α; IFN-γ, interferon-γ; NF-κB, nuclear factor kappa-B; Nrf2, nuclear respiratory factor 2; GCLC, glutamate-cysteine ligase catalytic subunit; GCLM, glutamate-cysteine ligase modifier subunit; SOD1, superoxide dismutase 1; GPx1, glutathione peroxidase 1. NBW, normal birth weight pigs; IUGR, intrauterine growth restriction pigs; IUGR+Zn, IUGR pigs fed with diets supplemented with 600 mg Zn/kg from Nano-ZnO.
As shown in Figure 4, compared with the NBW finishing pigs, the protein expressions of Keap1, P62 and LC3B/A were upregulated in the jejunal mucosa of IUGR finishing pigs (P < 0.05), while no significant changes in protein expressions of total Nrf2 and nuclear Nrf2 of jejunal mucosa were observed between the NBW and IUGR groups (P > 0.05). Dietary added 600 mg Zn/kg from Nano-ZnO significantly decreased the protein expression of Keap1, P62 and LC3B/A, and increased the protein expressions of total Nrf2 and nuclear Nrf2 in jejunal mucosa of IUGR finishing pigs as compared with the IUGR group (P < 0.05). Pigs in IUGR+Zn group had significantly enhanced the protein expression of total Nrf2 and nuclear Nrf2 as compared with the NBW group (P < 0.05), and no significant differences in protein expression of Keap1, P62 and LC3B/A were observed (P > 0.05).
Figure 4. Effects of dietary Nano-ZnO supplementation on the protein expression in jejunal mucosa of IUGR finishing pigs. Data were expressed relative to β-actin and normalized to the NBW group, and expressed as means with their SEM (n = 4). a,bMeans for the same parameter with different superscripts are significantly different (P < 0.05). Nrf2, nuclear respiratory factor 2; P62, Sequestosome 1; LC3B/A, microtubule-associated protein light chain 3 B/A. NBW, normal birth weight pigs; IUGR, intrauterine growth restriction pigs; IUGR+Zn, IUGR pigs fed with diets supplemented with 600 mg Zn/kg from Nano-ZnO.
Many studies have reported that IUGR can lead to low birth weight in piglets, but the body weight of IUGR pigs will show a catch-up in growth and can be equal to that of normal pigs (8, 9, 27). In this study, IUGR reduced the body weight and ADG of piglets at 1 d and 21d, while IUGR pigs had no significant difference in body weight (at 77 and 163 d), and in ADG (during 21 to 77 d and 77 to 163 d) as compared to the NBW group, suggesting that IUGR pigs showed catch-up growth, which is in agreement with the previous studies (8, 9, 27). Dietary supplementation with 600 mg Zn/kg from Nano-ZnO had no significant effects on the body weight and ADG of IUGR pigs, but the catch-up growth was not observed. This might be partially due to the decreased ADFI (during 77 to 163 d) by the dietary 600 mg Zn/kg from Nano-ZnO. In line with our present study, Sandoval et al. (28) and Miller et al. (29) found that high dietary zinc could decrease the feed intake of animals, which is related to the duration, dosage and bioavailability of zinc sources (30). These results indicated that more researches might be needed to further explore the appropriate duration and dosage of dietary Nano-ZnO in IUGR pigs, especially for the fattening stage.
One important reason for the inhibited growth of IUGR pigs might be the lack of circulating concentration of IGF-1, which is mainly regulated by GH (31–33). Plasma IGF-1 concentration of the IUGR piglets was about 30–35% lower than the NBW piglets (34), while increasing IGF-1 level (via injection or gene therapy) could improve the growth and development of IUGR animals (35, 36). In our present study, there was no significant difference in the serum GH and IGF-1 concentration between NBW and IUGR groups, which is in line with the similar body weight at 77 and 163 d between these two groups. Moreover, dietary 600 mg Zn/kg from Nano-ZnO also did not significantly affect the serum GH and IGF-1 concentration of IUGR pigs, which is in agreement with no significant effect on the growth of IUGR pigs. However, there was also no significant difference in serum GH and IGF-1 between NBW and IUGR+Zn groups, suggesting that the reason for pigs in IUGR+Zn group did not show catch-up growth might be related to other reasons instead of circulating IGF-1 concentration.
The intestinal tract is an important organ for digestion, absorption and immunity. The VH, VW, CD, VH/CD and VSA are critical indicators for evaluating intestinal morphology, and nutrient absorption capacity (37, 38). Many studies support the idea that damage occurs to the intestinal morphology and integrity in piglets with IUGR (19, 20). Interestingly, in the present study, we observed that IUGR increased the jejunal VH, CD, and VSA of the finishing pigs, indicating that IUGR finishing pigs achieved improved jejunal morphology during the fattening stage, which might improve their digestion and absorption abilities. We speculated that the improvement in the jejunal morphology may be related to the later catch-up growth of the IUGR pigs. The Nano-ZnO supplementation significantly decreased CD and increased the VH/CD radio of the IUGR finishing pigs, indicating that Nano-ZnO could further improve the IUGR pig jejunal intestinal morphology. This is consistent with our previous results of the effects of Nano-ZnO on the intestinal morphology of weaned piglets (16, 18).
The content of IgG, IgM and IgA in animals can reflect the state of immune response and can be used to diagnose immune diseases. Many studies have reported decreased immunoglobulin content and induced inflammatory reactions in the serum and intestine of IUGR piglets (20, 21, 39). In our study, no significant difference was observed in the contents of IgG, IgM and IgA in the serum or jejunal mucosa between the NBW and IUGR groups, indicating restoration of the immunoglobulin levels in IUGR pigs during the fattening period. IgA in mucosal tissues is the first line of defense for preventing the invasion of harmful bacteria from invading the body and it is involved in intestinal mucosal inflammation. Dietary Nano-ZnO reduced IgA content in the jejunal mucosa but did not change any other immune indexes. Similar results were found in piglets by Cui et al. (40) who reported that the IgA content in the jejunum of piglets decreased with the increase in the levels of supplemental Nano-ZnO in the feed (maximum 500 mg/kg). We suspect that the antibacterial properties of Nano-ZnO may inhibit the immune stress caused by intestinal harmful bacteria, thereby reducing the IgA content in intestinal mucosa (41, 42).
We further investigated the intestinal inflammatory response induced by IUGR and the beneficial effects of dietary Nano-ZnO supplementation by examining the expression of inflammation-related mRNA in jejunal mucosa. IL-4 and IL-10 are anti-inflammatory cytokines, and IL-1β, IL-6, TNF-α and IFN-γ are pro-inflammatory cytokines (43). TNF-α can bind to the corresponding receptors and activate the NF-κB signaling pathway, resulting in the release of other pro-inflammatory cytokines (e.g., TNF-β, IL-1, IL-2), and triggering of the inflammatory response (44). In the present study, the up-regulation of mRNA expression of IL-6, TNF-α and NF-κB suggested that IUGR-induced inflammatory responses may still exist in jejunal mucosa of finishing pigs. Importantly, the expression of TNF-α and NF-κB mRNA were decreased, whereas the expression of IL-10 mRNA was increased by dietary 600 mg Zn/kg from Nano-ZnO, suggesting that Nano-ZnO can inhibit the activation of the NF-κB signal in jejunal mucosa of IUGR finishing pigs, and thereby alleviate the inflammatory response of the jejunal mucosa. Long et al. (43) also reported that Nano-ZnO has a significant anti-inflammatory effect on weaned piglets, while Xia et al. (45) reported that dietary Nano-ZnO significantly reduced the relative mRNA expression levels of IFN-γ, IL-1β, TNF-α and NF-κB in jejunum of piglets. These observations support the possibility that IUGR-affected finishing pigs fed a diet supplemented with Nano-ZnO might mitigate jejunal mucosa inflammation by transcriptional regulation of TNF-α, NF-κB, and IL-10 expression.
Many studies have confirmed that IUGR can induce oxidative stress in piglets, including oxidative stress in serum (46), leg muscle (9), longissimus dorsi muscle (47), liver (48), and intestinal tract (20). Oxidative stress is caused by the imbalance between the antioxidant system and peroxides in the organisms, and eventually leads to lipid peroxidation and protein oxidation due to excessive production of reactive oxygen species (ROS) in the tissues. The main markers of lipid peroxidation and protein oxidation are MDA and PC, respectively (49, 50). The T-AOC activity and GSH content can reflect non-enzymatic antioxidant system, GSH is a polypeptide that can eliminate peroxides and also serve as the active substrate of GSH-Px. CAT, T-SOD, and GSH-Px are all important antioxidant enzymes in the antioxidant system of organisms and can reflect enzymatic antioxidant systems. In the current study, we observed that the IUGR finishing pigs had increased concentrations of MDA and PC, and that the levels of the CAT, T-AOC, T-SOD, GSH-Px, and GSH were restored to the normal level of jejunal mucosa, indicating that IUGR-induced oxidative stress still exists in the jejunum of pigs during the fattening stage, but the antioxidant capacity was partly improved. Fortunately, supplementation with Nano-ZnO could alleviate the oxidative stress in the jejunum of IUGR finishing pigs by reducing the content of MDA and PC and by increasing the CAT, GSH-Px and T-AOC activity, and the GSH contents. Nano-ZnO has potent antioxidant power both in cell-free systems and under intracellular conditions (51). In agreement with the trend of our results, Bai et al. (52) found that adding Nano-ZnO to the diet could enhance the antioxidant capacity of growing pigs. Zhao et al. (53) reported that adding appropriate concentration of Nano-ZnO to diets can improve the antioxidant capacity and reduce MDA production of broilers.
The Nrf2/Keap1 signaling pathway is one of the most important mechanisms for resistance to oxidative stress damage. Nrf2 can regulate the expression of antioxidant genes and induce the production of related antioxidant proteins. Nrf2 separated from Keap1 protein in the cytoplasm can enter the nucleus to induce the expression of related antioxidant genes and protect the tissue from oxidative stress damage (54–56). HO-1, GCLC, GCLM, SOD1 and GPx1 are common downstream genes activated by Nrf2 in the antioxidant system, and their expression can improve the antioxidant capacity of tissues (57). IUGR has been confirmed to inhibit the gene and protein expression of Nrf2 and to down-regulate the expression of related antioxidant genes of the intestinal tract in piglets (58). In the current study, we saw significantly upregulation of Keap1 protein expression in the jejunal mucosa of IUGR pigs, indicating that IUGR induced oxidative stress in the jejunal mucosa by promoting expression of Keap1 protein. However, Nano-ZnO upregulated the expression of GCLM and GPx1 by decreasing the Keap1 expression, and increasing the Nrf2 expression and protein level in the nucleus, which was consistent with the results of the antioxidant-related enzyme activities. A recent study in rainbow trout reported that dietary supplementation of Nano-ZnO upregulated the expression of SOD1, SOD2, CAT and GPx1 by increasing Nrf2 gene expression (59), and we saw the same effect on IUGR finishing pigs. These results suggested that Nano-ZnO may enhance the antioxidant capacity of IUGR pigs by promoting Nrf2/Keap1 signal pathway in jejunal mucosa, improving the activity of related antioxidant enzymes, and reducing the generation of MDA and PC to alleviate oxidative stress.
Mitochondria play an important role in cellular respiration, but they are also the main sites of energy metabolism and endogenous reactive oxygen species (ROS) production (22). Oxidative stress still existed in the jejunal mucosa of IUGR finishing pigs, which may further cause changes in mitochondrial structure. Mitochondrial swelling is a hallmark of mitochondrial dysfunction and is one of the most important indicators of the opening of the mitochondrial permeability transition (MPT) pore (60). Oxidative stress can affect the activity of related mitochondrial respiratory chain complexes, and the MPT pores may be further activated, greatly increasing the probability of pore opening, aggravating the loss of mitochondrial matrix components, and ultimately leading to mitochondrial swelling (61, 62). Autophagy is an organism's defense mechanism in response to oxidative stress by removing damaged proteins and organelles, while over-autophagy may further induce apoptosis or tissue damage (63, 64). Excessive ROS can lead to mitochondrial damage, which further leads to selective autophagy of damaged mitochondria (65). P62 is a multifunctional ubiquitin-binding scaffold protein that has a variety of cellular functions ranging from cell signaling to autophagy (66). LC3 is a marker of autophagy and is mainly involved in the formation of autophagosomes. It is a structural protein of the autophagosome, and its C-terminal 5-peptide is cut off by ATG4B and lysed to form the cytoplasmic form LC3A, which is then activated by APG7L/ATG7 and transferred to ATG3 and coupled with lipoacylethanolamine to form the membrane-bound form, LC3B, which can be attached to the membranes of autophagosomes, and further form autolysosomes with the lysosome (67, 68). The ratio of LC3B to LC3A can reflect the degree of autophagy. When oxidative stress occurs, P62 increases in number and aggregates, and P62 helps identify autophagy substrates through its ubiquitin-binding domain and promotes interaction with LC3 (69). The results of the present study showed that the number of swollen mitochondria and autolysosomes, and the protein expressions of P62 and ratio of LC3B to LC3A were increased in the jejunum of IUGR finishing pigs, and mitochondria were mostly vacuolated and disorganized. These results suggested that IUGR enhanced the jejunal mucosal autophagy of pigs. Similarly, previous studies have shown that IUGR increases the number of abnormal mitochondrial and autophagy protein expression in the intestinal mucosa of piglets (20, 70). However, dietary supplementation with Nano-ZnO reduced the number of swollen mitochondria and autolysosomes, improved the shape and structure of mitochondria and down-regulated the protein expression of P62 and the ratio of LC3B to LC3A in jejunal mucosa of IUGR finishing pigs, suggesting that Nano-ZnO could reduce IUGR-induced autophagy in jejunal mucosa. This is consistent with our above results that Nano-ZnO might alleviate jejunal oxidative stress through the Nrf2/Keap1 regulatory pathway.
Our results indicated that IUGR pigs showed postnatal catch-up growth and improved intestinal morphology in the fattening stage, but still existed oxidative stress and inflammation in jejunum. Dietary supplemental 600 mg Zn/kg from Nano-ZnO may further improve intestinal morphology, reduce inflammatory response, decrease autophagy and alleviate oxidative stress via enhancing the Nrf2/Keap1 signaling pathway in jejunum of IUGR pigs.
The author selected the following statement: The datasets presented in this study can be found in online repositories. The names of the repository/repositories and accession number(s) can be found below: https://www.ncbi.nlm.nih.gov/.
The animal study protocol was approved by the Institutional Animal Care and Use Committee of Nanjing Agricultural University (Jiangsu, China. Permit number SYXK-2019-00142).
CW and TW conceived and designed the experiments. BZ, JZ, HL, and SC prepared material and collected data. BZ analyzed the data and prepared and drafted the manuscript. CW and BZ revised the manuscript. All authors have read and agreed to the published version of the manuscript.
This study was financially supported by the National Natural Science Foundation of China (No.31972598).
The authors declare that the research was conducted in the absence of any commercial or financial relationships that could be construed as a potential conflict of interest.
All claims expressed in this article are solely those of the authors and do not necessarily represent those of their affiliated organizations, or those of the publisher, the editors and the reviewers. Any product that may be evaluated in this article, or claim that may be made by its manufacturer, is not guaranteed or endorsed by the publisher.
The technical assistance of colleagues in our laboratories and the technicians and teachers from the College of Animal Sciences and Technology, National Experimental Teaching Demonstration Centre of Animal Science, Nanjing Agricultural University are gratefully acknowledged.
1. Wu G, Bazer FW, Wallace JM, Spencer TE. Board-invited review: intrauterine growth retardation: implications for the animal sciences. J Anim Sci. (2006) 84:2316–37. doi: 10.2527/jas.2006-156
2. Brodsky D, Christou H. Current concepts in intrauterine growth restriction. J Intensive Care Med. (2004) 19:307–19. doi: 10.1177/0885066604269663
3. Barker D. The developmental origins of insulin resistance. Horm Res. (2005) 64:2–7. doi: 10.1159/000089311
4. Briana DD, Malamitsi-Puchner A. Intrauterine growth restriction and adult disease: the role of adipocytokines. Eur J Endocrinol. (2009) 160:337–47. doi: 10.1530/EJE-08-0621
5. Xie XM, Gao HJ, Lin TL, Liao LH, Ning Q. IUGR with infantile overnutrition programs an insulin-resistant phenotype through DNA methylation of peroxisome proliferator-activated receptor-gamma coactivator-1 alpha in rats. Pediatr Res. (2015) 77:625–32. doi: 10.1038/pr.2015.32
6. Wang J, Feng CP, Liu T, Shi MS, Wu G, Bazer FB. Physiological alterations associated with intrauterine growth restriction in fetal pigs: causes and insights for nutritional optimization. Mol Reprod Dev. (2017) 84:897–904. doi: 10.1002/mrd.22842
7. Fabricius-Bjerre S, Jensen RB, Frch K, Larsen T, Mlgaard C, Michaelsen KF, et al. Impact of birth weight and early infant weight gain on insulin resistance and associated cardiovascular risk factors in adolescence. PLoS ONE. (2011) 6:e20595. doi: 10.1371/journal.pone.0020595
8. Myrie SB, Mcknight LL, King JC, Mcguire JJ, Vliet BV, Cheema SK, et al. Intrauterine growth-restricted Yucatan miniature pigs experience early catch-up growth leading to greater adiposity and impaired lipid metabolism as young adults. Appl Physiol Nutr Metab. (2017) 42:1322–9. doi: 10.1139/apnm-2017-0311
9. Zhang L, Zhang J, Yan E, He J, Zhong X, Zhang L, et al. Dietary supplemented curcumin improves meat quality and antioxidant status of intrauterine growth retardation growing pigs via Nrf2 signal pathway. Animals. (2020) 10:539. doi: 10.3390/ani10030539
10. Su W, Zhang H, Ying Z, Li Y, Zhou L, Wang F, et al. Effects of dietary L-methionine supplementation on intestinal integrity and oxidative status in intrauterine growth-retarded weanling piglets. Eur J Nutr. (2018) 57:2735–45. doi: 10.1007/s00394-017-1539-3
11. Huang S, Li N, Liu C, Li T, Wang W, Jiang L, et al. Characteristics of the gut microbiota colonization, inflammatory profile, and plasma metabolome in intrauterine growth restricted piglets during the first 12 hours after birth. J Microbiol. (2019) 57:748–58. doi: 10.1007/s12275-019-8690-x
12. Yan E, Zhang J, Han H, Wu J, Gan Z, Wei C, et al. Curcumin alleviates IUGR jejunum damage by increasing antioxidant capacity through Nrf2/Keap1 pathway in growing pigs. Animals. (2020) 10:41. doi: 10.3390/ani10010041
13. Hassan AM, Sada K, Ketheeswaran S, Dubey AK, Bhat MS. Role of zinc in mucosal health and disease: a review of physiological, biochemical, and molecular processes. Cureus. (2020) 12:e8197. doi: 10.7759/cureus.8197
14. Jondreville C, Revy PS, Dourmad JY. Dietary means to better control the environmental impact of copper and zinc by pigs from weaning to slaughter. Livest Prod Sci. (2003) 84:147–56. doi: 10.1016/j.livprodsci.2003.09.011
15. Hosseini-Sarvari M, Tavakolian M. Preparation, characterization, and catalysis application of nano-rods zinc oxide in the synthesis of 3-indolyl-3-hydroxy oxindoles in water. Appl Catal A-Gen. (2012) 441–2:65–71. doi: 10.1016/j.apcata.2012.07.009
16. Wang C, Zhang L, Ying Z, He J, Zhou L, Zhang L, et al. Effects of dietary zinc oxide nanoparticles on growth, diarrhea, mineral deposition, intestinal morphology, and barrier of weaned piglets. Biol Trace Elem Res. (2018) 185:364–74. doi: 10.1007/s12011-018-1266-5
17. Sun YB, Xia T, Wu H, Zhang WJ, Zhu YH, Xue JX, et al. Effects of nano zinc oxide as an alternative to pharmacological dose of zinc oxide on growth performance, diarrhea, immune responses, and intestinal microflora profile in weaned piglets. Anim Feed Sci Technol. (2019) 258:114312. doi: 10.1016/j.anifeedsci.2019.114312
18. Wang C, Zhang L, Su W, Ying Z, He J, Zhang L, et al. Zinc oxide nanoparticles as a substitute for zinc oxide or colistin sulfate: effects on growth, serum enzymes, zinc deposition, intestinal morphology and epithelial barrier in weaned piglets. PLoS ONE. (2017) 12:e0181136. doi: 10.1371/journal.pone.0181136
19. Zhang H, Li Y, Chen Y, Zhang L, Wang T. N-Acetylcysteine protects against intrauterine growth retardation-induced intestinal injury via restoring redox status and mitochondrial function in neonatal piglets. Eur J Nutr. (2019) 58:3335–47. doi: 10.1007/s00394-018-1878-8
20. Zhang H, Chen Y, Li Y, Wang T. Protective effect of polydatin on jejunal mucosal integrity, redox status, inflammatory response, and mitochondrial function in intrauterine growth-retarded weanling piglets. Oxid Med Cell Longev. (2020) 2020:1–14. doi: 10.1155/2020/7178123
21. Dong L, Zhong X, He J, Zhang LL, Bai KW, Xu W, et al. Supplementation of tributyrin improves the growth and intestinal digestive and barrier functions in intrauterine growth-restricted piglets. Clin Nutr. (2016) 35:399–407. doi: 10.1016/j.clnu.2015.03.002
22. Bhansali S, Bhansali A, Walia R, Saikiia UN, Dhawan V. Alterations in mitochondrial oxidative stress and mitophagy in subjects with prediabetes and type 2 diabetes mellitus. Front Endocrinol. (2017) 8:347. doi: 10.3389/fendo.2017.00347
23. Seo BJ, Choi J, La H, Habib O, Choi Y, Hong K, et al. Role of mitochondrial fission-related genes in mitochondrial morphology and energy metabolism in mouse embryonic stem cells. Redox Biol. (2020) 36:2213–317. doi: 10.1016/j.redox.2020.101599
24. Wang XD, Yu WL, Sun Y. Activation of AMPK restored impaired autophagy and inhibited inflammation reaction by up-regulating SIRT1 in acute pancreatitis. Life Sci. (2021) 277:119435. doi: 10.1016/j.lfs.2021.119435
25. Livak KJ, Schmittgen TD. Analysis of relative gene expression data using real-time quantitative PCR and the 2−ΔΔcT method. Methods. (2002) 25:402–8. doi: 10.1006/meth.2001.1262
26. Cheng K, Yu CY, Li ZH, Li S, Yan EF, Song ZH, et al. Resveratrol improves meat quality, muscular antioxidant capacity, lipid metabolism and fiber type composition of intrauterine growth retarded pigs. Meat Sci. (2020) 170:108237. doi: 10.1016/j.meatsci.2020.108237
27. Poore KR, Fowden AL. The effects of birth weight and postnatal growth patterns on fat depth and plasma leptin concentrations in juvenile and adult pigs. J Physiol. (2010) 558:295–304. doi: 10.1113/jphysiol.2004.061390
28. Sandoval M, Henry PR, Luo XG, Littell RC, Miles RD, Ammerman CB. Performance and tissue zinc and metallothionein accumulation in chicks fed a high dietary level of zinc. Poult Sci. (1998) 77:1354–63. doi: 10.1093/ps/77.9.1354
29. Miller WJ, Amos HE, Gentry RP, Blackmon DM, Durrance RM, Crowe CT, et al. Long-term feeding of high zinc sulfate diets to lactating and gestating dairy cows. J dairy sci. (1989) 72:1499–508. doi: 10.3168/jds.S0022-0302(89)79260-2
30. Poulsen HD. Zinc oxide for weanling piglets. Acta Agric Scand. (1995) 45:159–67. doi: 10.1080/09064709509415847
31. Setia S, Sridhar MG. Changes in GH/IGF-1 axis in intrauterine growth retardation: consequences of fetal programming? Horm Metab Res. (2009) 41:791–8. doi: 10.1055/s-0029-1231026
32. Ju D, Teng T, Bai G, Fu H, Qiu S, Zhao X, et al. The role of protein restriction and interaction with antibiotics in the regulation of compensatory growth in pigs: growth performance, serum hormone concentrations, and messenger RNA levels in component tissues of the endocrine growth axis. Domesti Anim Endocrinol. (2021) 74:106524. doi: 10.1016/j.domaniend.2020.106524
33. Martín-Estal I, De La Garza R G, Castilla-Cortázar I. Intrauterine growth retardation (IUGR) as a novel condition of insulin-like growth factor-1 (IGF-1) deficiency. Rev Physiol Biochem Pharmacol. (2016) 170:1–35. doi: 10.1007/112_2015_5001
34. Schoknecht PA, Ebner S, Skottner A, Burrin DG, Davis TA, Ellis K, et al. Exogenous insulin-like growth factor-I increases weight gain in intrauterine growth-retarded neonatal pigs. Pediatr Res. (1997) 42:201–7. doi: 10.1203/00006450-199708000-00012
35. Eremia SC, Boo HA, Bloomfield FH, Oliver MK, Harding JE. Fetal and amniotic insulin-like growth factor-I supplements improve growth rate in intrauterine growth restriction fetal sheep. Comparative study. (2007) 148:2963–72. doi: 10.1210/en.2006-1701
36. Keswani SG, Balaji S, Katz AB, King A, Omar K, Habli M, et al. Intraplacental gene therapy with Ad-IGF-1 corrects naturally occurring rabbit model of intrauterine growth restriction. Hum gene ther. (2015) 26:172–82. doi: 10.1089/hum.2014.065
37. Li BT, Van Kessel AG, Caine WR, Huang SX, Kirkwood RN. Small intestinal morphology and bacterial populations in ileal digesta and feces of newly weaned pigs receiving a high dietary level of zinc oxide. Can J Anim Sci. (2001) 81:511–6. doi: 10.4141/A01-043
38. Dong L, Zhong X, Ahmad H, Li W, Wang Y, Zhang L, et al. Intrauterine growth restriction impairs small intestinal mucosal immunity in neonatal piglets. J Histochem Cytochem. (2014) 62:510–8. doi: 10.1369/0022155414532655
39. Hu L, Peng X, Chen H, Yan C, Liu Y, Xu Q, et al. Effects of intrauterine growth retardation and Bacillus subtilis PB6 supplementation on growth performance, intestinal development and immune function of piglets during the suckling period. Eur J Nutr. (2017) 56:1753–65. doi: 10.1007/s00394-016-1223-z
40. Cui YY, Tian ZM, Lu HJ, Deng D, Liu ZC, Rong T, et al. Zinc oxide nanoparticles improve gut health and reduce faecal zinc excretion in piglets. Livest Sci. (2021) 251:104610. doi: 10.1016/j.livsci.2021.104610
41. Dizaj SM, Lotfipour F, Barzegarjalali M, Zarrintan MH, Adibkia K. Antimicrobial activity of the metals and metal oxide nanoparticles. Mater Sci Eng C Mater Biol Appl. (2014) 44:278–84. doi: 10.1016/j.msec.2014.08.031
42. Sirelkhatim A, Mahmud S, Seeni A, Kaus NHM, Ann LC, Bakhori SKM, et al. Review on zinc oxide nanoparticles: antibacterial activity and yoxicity mechanism. Nanomicro Lett. (2015) 7:219–42. doi: 10.1007/s40820-015-0040-x
43. Long L, Chen J, Zhang Y, Xiao L, Yin Y. Comparison of porous and nano zinc oxide for replacing high-dose dietary regular zinc oxide in weaning piglets. PLoS ONE. (2017) 12:e0188587. doi: 10.1371/journal.pone.0188587
44. Wulczyn FG, Krappmann D, Scheidereit C. The NF-κB/Rel and IκB gene families: mediators of immune response and inflammation. J Mol Med. (1996) 74:749–69. doi: 10.1007/s001090050078
45. Xia T, Lai WQ, Han MM, Han M, Ma X, Zhang LY. Dietary ZnO nanoparticles alters intestinal microbiota and inflammation response in weaned piglets. Oncotarget. (2017) 8:64878–91. doi: 10.18632/oncotarget.17612
46. Niu Y, He J, Ahmad H, Shen M, Zhao Y, Gan Z, et al. Dietary curcumin supplementation increases antioxidant capacity, upregulates Nrf2 and hmox1 levels in the liver of piglet model with intrauterine growth retardation. Nutrients. (2019) 11:2978. doi: 10.3390/nu11122978
47. Cheng K, Wang T, Li S, Song Z, Wang T. Effects of early resveratrol intervention on skeletal muscle mitochondrial function and redox status in neonatal piglets with or without intrauterine growth retardation. Oxid Med Cell Longev. (2020) 2020:1–12. doi: 10.1155/2020/4858975
48. Cheng K, Jia PL Ji SL, Song ZH, Zhang H, Zhang LL, et al. Improvement of the hepatic lipid status in intrauterine growth retarded pigs by resveratrol is related to the inhibition of mitochondrial dysfunction, oxidative stress and inflammation. Food Funct. (2021) 12:278–90. doi: 10.1039/D0FO01459A
49. Del RD, Stewart AJ, Pellegrini N. A review of recent studies on malondialdehyde as toxic molecule and biological marker of oxidative stress. Nutr Metab Cardiovasc Dis. (2005) 15:316–28. doi: 10.1016/j.numecd.2005.05.003
50. Caperna TJ, Shannon AE, Le AB, Garrett WM, Ramsay TG. Identification of protein carbonyls in serum of the fetal and neonatal pig. Comp Biochem Physiol B Biochem Mol Biol. (2010) 156:189–96. doi: 10.1016/j.cbpb.2010.03.006
51. Singh TA, Sharma AE, Tejwan N, Ghosh N, Das J, Sil PC, et al. state of the art review on the synthesis, antibacterial, antioxidant, antidiabetic and tissue regeneration activities of zinc oxide nanoparticles. Adv Colloid Interface Sci. (2021) 295:102495. doi: 10.1016/j.cis.2021.102495
52. Bai MMM, Liu H NN, Xu K, Wen CYY, Yu R, Deng JPP, et al. Use of coated nano zinc oxide as an additive to improve the zinc excretion and intestinal morphology of growing pigs. J Anim Sci. (2019) 97:1772–83. doi: 10.1093/jas/skz065
53. Zhao C, Tan S, Xiao X, Qiu X, Pan J, Tang Z. Effects of dietary zinc oxide nanoparticles on growth performance and antioxidative status in broilers. Biol Trace Elem Res. (2014) 160:361–7. doi: 10.1007/s12011-014-0052-2
54. Motohashi H, Yamamoto M. Nrf2-Keap1 defines a physiologically important stress response mechanism. Trends Mol Med. (2004) 10:549–57. doi: 10.1016/j.molmed.2004.09.003
55. Kaspar JW, Niture SK, Jaiswal AK. Nrf2:INrf2 (Keap1) signaling in oxidative stress. Free Radic Biol Med. (2009) 47:1304–9. doi: 10.1016/j.freeradbiomed.2009.07.035
56. Kobayashi M, Li L, Iwamoto N, Nakajima-Takagi Y, Kaneko H, Nakayama Yu, et al. The antioxidant defense System Keap1-Nrf2 comprises a multiple sensing mechanism for responding to a wide range of chemical compounds. Mol Cell Biol. (2009) 29:493–502. doi: 10.1128/MCB.01080-08
57. Sykiotis GP, Habeos IG, Samuelson AV, Bohmann D. The role of the antioxidant and longevity-promoting Nrf2 pathway in metabolic regulation. Curr Opin Clin Nutr Metab Care. (2011) 14:41–8. doi: 10.1097/MCO.0b013e32834136f2
58. Niu Y, He JT, Zhao YW, Gan ZD, Shen MM, Zhang LL, et al. Dietary enzymatically treated Artemisia annua L. supplementation improved growth performance and intestinal antioxidant capacity of weaned piglets. Livest Sci. (2020) 232:103937. doi: 10.1016/j.livsci.2020.103937
59. Kazemi E, Nazari S, Sourinejad I, Pourkazemi M, Paknejad H, Eslamloo K. Effect of different dietary zinc sources on seminal plasma enzymatic activity, antioxidant, and immune-related gene expression in rainbow trout (Oncorhynchus mykiss). Aquacult Int. (2021) 29:2731–50. doi: 10.1007/s10499-021-00778-3
60. Formanowicz D, Malińska A, Nowicki M, Kowalska K, Gruca-Stryjak K, Brborowicz G, et al. Preeclampsia with intrauterine growth restriction generates morphological changes in endothelial cells associated with mitochondrial swelling—an in vitro study. J Clin Med. (2019) 8:1994. doi: 10.3390/jcm8111994
61. Gardner PR, Nguyen DD, White CW. Aconitase is a sensitive and critical target of oxygen poisoning in cultured mammalian cells and in rat lungs. Proc Natl Acad Sci USA. (1994) 91:12248–52. doi: 10.1073/pnas.91.25.12248
62. Zhang H, Li Y, Su W, Ying Z, Zhou L, Zhang L, et al. Resveratrol attenuates mitochondrial dysfunction in the liver of intrauterine growth retarded suckling piglets by improving mitochondrial biogenesis and redox status. Mol Nutr Food Res. (2016) 61:1600653. doi: 10.1002/mnfr.201600653
63. Kiffin R, Bandyopadhyay U, Cuervo AM. Oxidative stress and autophagy. Antioxid Redox Signal. (2006) 8:152. doi: 10.1089/ars.2006.8.152
64. Scherz-Shouval R, Elazar Z. ROS mitochondria and the regulation of autophagy. Trends Cell Biol. (2007) 17:422–7. doi: 10.1016/j.tcb.2007.07.009
65. Lemasters JJ. Selective mitochondrial autophagy, or mitophagy, as a targeted defense against oxidative stress, mitochondrial dysfunction, and aging. Rejuvenation Res. (2005) 8:3–5. doi: 10.1089/rej.2005.8.3
66. Nezis IP, Stenmark H. p62 at the interface of autophagy, oxidative stress signaling, and cancer. Antioxid Redox Signal. (2012) 17:786–93. doi: 10.1089/ars.2011.4394
67. Pankiv S, Clausen TH, Lamark T, Brech A, Bruun J, Outzen H, et al. p62/SQSTM1 binds directly to Atg8/LC3 to facilitate degradation of ubiquitinated protein aggregates by autophagy. J Biol Chem. (2007) 282:24131–45. doi: 10.1074/jbc.M702824200
68. Klionsky DJ, Eskelinen E, Deretic V. Autophagosomes, phagosomes, autolysosomes, phagolysosomes, autophagolysosomes wait, I'm confused. Autophagy. (2014) 10:549–51. doi: 10.4161/auto.28448
69. Bjorkoy G, Lamark T, Brech A, Outzen H, Perander M, Overvatn A, et al. p62/SQSTM1 forms protein aggregates degraded by autophagy and has a protective effect on huntingtin-induced cell death. J Cell Biol. (2005) 171:603–14. doi: 10.1083/jcb.200507002
Keywords: intrauterine growth retardation finishing pigs, zinc oxide nanoparticle, immunity, inflammation, antioxidant capacity, autophagy
Citation: Zhou B, Zhang J, Liu H, Chen S, Wang T and Wang C (2022) Zinc Oxide Nanoparticle Improves the Intestinal Function of Intrauterine Growth Retardation Finishing Pigs via Regulating Intestinal Morphology, Inflammation, Antioxidant Status and Autophagy. Front. Vet. Sci. 9:884945. doi: 10.3389/fvets.2022.884945
Received: 27 February 2022; Accepted: 10 May 2022;
Published: 06 June 2022.
Edited by:
Tarique Hussain, Nuclear Institute for Agriculture and Biology, PakistanReviewed by:
Zhiwei Kong, Institute of Subtropical Agriculture (CAS), ChinaCopyright © 2022 Zhou, Zhang, Liu, Chen, Wang and Wang. This is an open-access article distributed under the terms of the Creative Commons Attribution License (CC BY). The use, distribution or reproduction in other forums is permitted, provided the original author(s) and the copyright owner(s) are credited and that the original publication in this journal is cited, in accordance with accepted academic practice. No use, distribution or reproduction is permitted which does not comply with these terms.
*Correspondence: Chao Wang, d2FuZ2NoYW8xMjFAbmphdS5lZHUuY24=
Disclaimer: All claims expressed in this article are solely those of the authors and do not necessarily represent those of their affiliated organizations, or those of the publisher, the editors and the reviewers. Any product that may be evaluated in this article or claim that may be made by its manufacturer is not guaranteed or endorsed by the publisher.
Research integrity at Frontiers
Learn more about the work of our research integrity team to safeguard the quality of each article we publish.