- 1Department of Poultry Science, University of Arkansas Agricultural Experiment Station, Fayetteville, AR, United States
- 2Facultad de Estudios Superiores Cuautitlan, Universidad Nacional Autonoma de Mexico, Cuautitlan Izcalli, Mexico
Histomonas meleagridis, the etiological agent of histomonosis, is a poultry parasite primarily detrimental to turkeys. Characteristic lesions occur in the liver and ceca, with mortalities in turkey flocks often reaching 80–100%. Chickens and other gallinaceous birds can be susceptible but the disease was primarily considered sub-clinical until recent years. Treating and preventing H. meleagridis infection have become more difficult since 2015, when nitarsone was voluntarily removed from the market, leaving the poultry industry with no approved prophylactics, therapeutics, or vaccines to combat histomonosis. Phytogenic compounds evaluated for chemoprophylaxis of histomonosis have varied results with in vitro and in vivo experiments. Some recent research successes are encouraging for the pursuit of antihistomonal compounds derived from plants. Turkeys and chickens exhibit a level of resistance to re-infection when recovered from H. meleagridis infection, but no commercial vaccines are yet available, despite experimental successes. Safety and stability of live-attenuated isolates have been demonstrated; furthermore, highly efficacious protection has been conferred in experimental settings with administration of these isolates without harming performance. Taken together, these research advancements are encouraging for vaccine development, but further investigation is necessary to evaluate proper administration age, dose, and route. A summary of the published research is provided in this review.
Introduction
The first known histomonosis outbreak was described by Cushman (1) and occurred in a Rhode Island turkey flock. Smith (2) further characterized histomonosis and attributed it to the protozoan Amoeba meleagridis obtained from liver lesions. Shortly thereafter, Tyzzer (3) more appropriately renamed this protozoon as Histomonas meleagridis. Further studies confirmed H. meleagridis as the etiological agent, although the mode of cecal invasion was still uncertain (4). Common synonyms for the disease have included blackhead disease, infectious enterohepatitis, histomoniasis, and typhlohepatitis (5–7). Blackhead disease is an unfortunate misnomer as a cyanotic head is neither pathognomonic nor common (8, 9); therefore, histomonosis will be the preferred terminology used throughout this review based on the Standardized Nomenclature of Animal Parasitic Diseases (10). Turkeys are especially susceptible to H. meleagridis infection, although other gallinaceous birds such as chickens, pheasants, and peafowls can be affected (7, 11, 12). Annual economic losses to the turkey industry have been estimated to exceed 2 million USD, and a 2020 survey listed histomonosis in position #11 of current issues facing the industry (9, 13).
Graybill and Smith (14) implicated Heterakis spp. in the role of transmitting H. meleagridis as they were unable to initiate the disease in absence of cecal worms. Further research showed that unprotected histomonads did not survive long periods outside the host, although duration in the environment when protected by feces or other materials was not well-characterized (15, 16). The separate rearing of poultry species is critical as chickens are considered partially resistant to histomonosis, frequently serving as asymptomatic carriers and reservoirs of H. meleagridis-infected heterakid eggs [Figure 1; (11, 17–21)]. Direct transmission within a flock is considered to occur through cloacal drinking which transfers materials from the vent region into the ceca through waves of reverse peristalsis (22–25). Horizontal transmission of H. meleagridis has occurred by comingling and contact of infected with uninfected turkeys, regardless of floor type and in absence of H. gallinarum (26, 27). The breed of turkeys or chickens may affect susceptibility to H. meleagridis infection, although male and female turkeys appear to be similarly susceptible; however, research is limited on the possible influence on disease development (28–31).
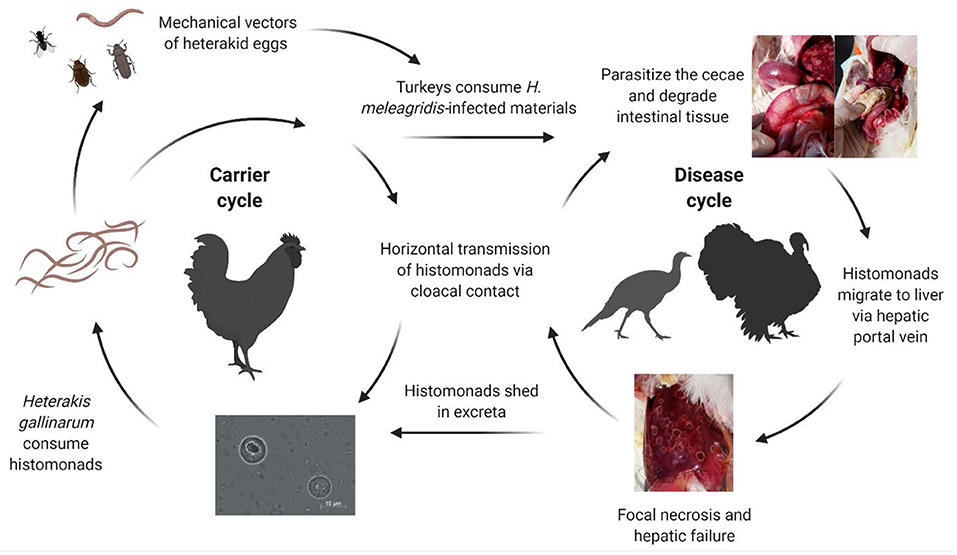
Figure 1. Complex transmission of Histomonas meleagridis. Histomonads can be consumed by Heterakis gallinarum and can be subsequently incorporated into the nematode ova. Carrier birds such as chickens can harbor the cecal worms and shed infected heterakid eggs into the environment. Earthworms, flies, and other invertebrates can serve as intermediate mechanical vectors of infected heterakid ova. Turkeys may ingest infected materials such as excreta or invertebrates contaminated with the protozoa. Once inside the intestine, the histomonads will migrate to the ceca, replicating and degrading the cecal lining. Direct transmission can occur rapidly from turkey-to-turkey due to cloacal drinking and reverse peristalsis movement of materials into the vent region (Created with BioRender.com).
Biology of H. meleagridis
H. meleagridis is a unicellular parasite belonging to the phylum Parabasalia, class Tritrichomonadea, order Tritrichomonadida, and family Dientamoebidae (32, 33). Interestingly, the morphology can change between flagellated and amoeboid forms depending on location within the ceca or liver, respectively, with an average histomonad size of 10–14 μm [Figure 2; (3, 5, 9, 34–36)]. The cell morphology and associated phenotypic changes have been mimicked experimentally in vitro (37). H. meleagridis typically exhibits a single-flagellated form within the cecal lumen, but this flagellum is lost upon mucosal invasion with the development of pseudopods (38). H. wenrichi (alternatively Parahistomonas wenrichi), a non-pathogenic but separate species, appears as 4-flagellated or amoeboid in form with a larger size of 20–30 μm (16, 39–41). H. meleagridis reproduce via binary fission; lacking mitochondria, these protozoa rely on hydrogenosomes as modified organelles for energy metabolism (3, 42–44).
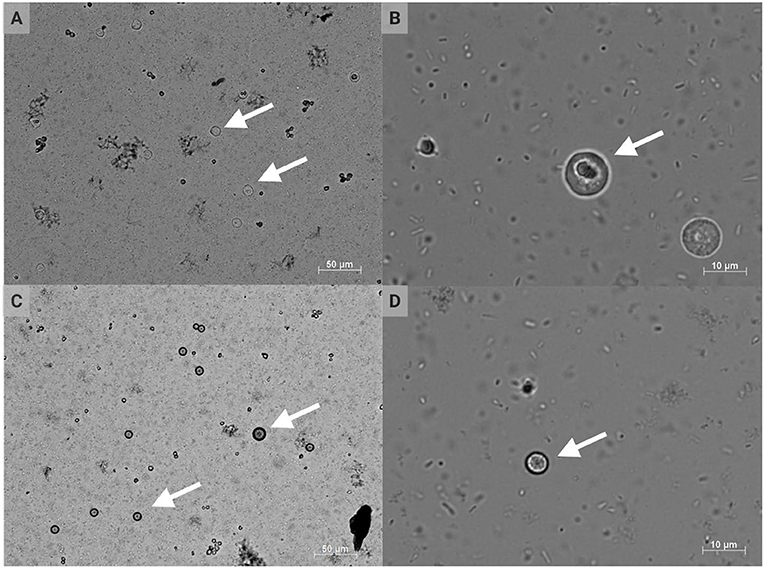
Figure 2. Conventional DIC photomicrographs of Histomonas meleagridis. Amoeboid or irregular morphology of histomonads at 200 × (A) and 1,000 × (B) total magnification; spherical morphology of histomonads at 200 × (C) and 1,000 × (D) total magnification. The arrows indicate selected histomonads (Created with BioRender.com).
Early in vitro work indicated that histomonads can be grown at temperatures of 36.5–37°C but not when reduced to 18–22°C for 48 h or 5°C for 24 h, suggesting that environmental survival of protozoa shed from infected birds is not likely to be culpable in mass infectivity (35). Currently, in vitro propagation occurs anaerobically at 40–41°C with a Medium 199-based cell culture and bacterial co-culture to simulate the body temperature and environment of a healthy turkey (44, 45). Dwyer's medium comprised of Medium 199, chick embryo extract, horse sera, and rice powder has been utilized, although other cell culture media such as L-15, MEM, or RPMI have been substituted effectively for Medium 199 (46). Modified Dwyer's medium, which removes the chick embryo extract and increases rice powder from 0.096 (w/v) to 0.8% (w/v), improved histomonad growth following revival of aliquots from liquid nitrogen and serial passage (47). Further increasing rice powder from 0.8 (w/v) to 4–8% (w/v) resulted in a nearly 10-fold growth increase, but this was not sustained longer than 2 days as the remaining nutrients became exhausted (48). Cholesterol supplementation has improved H. meleagridis growth in vitro, even in the absence of serum, which is typically required for adequate growth (49). Chute and Chute (50) cryogenically preserved H. meleagridis isolates in combination with 8% dimethylsulfoxide for up to 345 days and demonstrated viability of infection to birds following thaw. Honigberg and Dwyer (51) demonstrated that either 5 or 10% dimethylsulfoxide effectively preserved the protozoa in cryogenic storage as observed after 7 weeks; therefore, isolates could be maintained for future studies.
Field isolates of H. meleagridis can be cultivated from infected carcasses, particularly cecal samples, if shipment to a laboratory occurs soon after bird mortality with greater recovery potential if temperatures are maintained above 30°C (52). In vitro growth from cecal samples can usually be confirmed between 1 and 4 days after inoculation into culture media; intracloacal inoculation back into live birds can be used to further diagnose H. meleagridis as the original cause of infection in field outbreaks (53). Histomonads have also been isolated effectively from liver lesions, but in vitro propagation attempts in absence of bacteria have been unsuccessful (16, 34, 53, 54). Attempts to culture the protozoa in absence of live bacteria and serum were achieved with difficulty, but supplementation of palmitic acid or cholesterol was required along with antibiotic-killed bacteria and hamster liver extract; however, these results have not been easily replicated (45, 55–57). In vitro growth of H. meleagridis was better sustained with undefined populations of turkey cecal bacteria than with mixed chicken cecal bacteria (58). Moreover, histomonads have been grown with supplementation of single species of bacteria and monoxenic cultures have been established (59, 60).
Pathogenesis
After parasitizing and degrading the cecal tissue, histomonads migrate to the liver via the hepatic portal blood; the resulting pathognomonic lesions are exhibited as target-like liver lesions and caseous cecal cores [Figures 3, 4; (44, 61, 62)]. Histomonads have been observed in the bursa of Fabricius of 6-week-old commercial chickens diagnosed with histomonosis, further implicating the intracloacal route for natural infection (63). Although less common, H. meleagridis has also been shown to infect areas including the brain, pancreas, heart, lungs, kidneys, and spleen (64–68). Turkeys are especially vulnerable to histomonosis, and chickens (Figure 5) are less susceptible but function to serve as reservoirs and can develop the disease (17). Cloacal transmission seems less important to chickens than turkeys for transfer of histomonosis, as horizontal transmission did not occur in the absence of vectors and was not exacerbated with Eimeria adenoeides challenge, which is not surprising as this Eimeria spp. is turkey-specific (69). While cloacal drinking is a well-known occurrence in chickens and turkeys, species differences in horizontal transmission could result from higher litter moisture and huddling behavior in turkeys than chickens, allowing greater survival and subsequent transmission of H. meleagridis in the absence of vectors (22, 69). Mortalities in turkey flocks can reach 80–100%; organic farms co-rearing turkeys and broilers have struggled with series of outbreaks with broiler and turkey mortalities reaching 100 and 67.2%, respectively, possibly due to co-infection with Eimeria spp. (19, 44, 70). Susceptibility of different poultry species and genetic lines has only been evaluated briefly, but infection incidence and severity do appear different (28, 29, 68, 71, 72). In chickens, sex-related variations and environmental differences have influenced intestinal structure and function; therefore, it seems reasonable that these differences could factor into the incidence and severity of histomonosis (73). In addition to age and genetic line of poultry, variations in mortality rate and lesion severity could result from strain-specific differences in virulence of H. meleagridis or exposure dose (23, 74, 75). Although chickens were previously regarded as sub-clinically affected by histomonosis, outbreaks have occurred recently in broiler breeder and free-range flocks (76, 77). Interestingly, recent research has indicated that H. meleagridis infection and replication are similar regardless of chicken genetic line, further suggesting that chickens may be asymptomatic or sub-clinically infected but not actually resistant to infection (78).
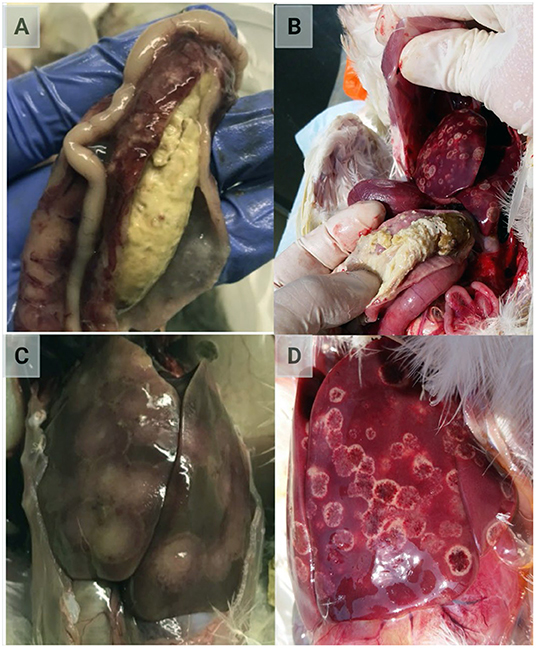
Figure 3. Classic lesions resulting from Histomonas meleagridis infection. (A,B) Caseous cheese-like cecal core; (C,D) focal necrosis resulting in target-like liver lesions (Created with BioRender.com).
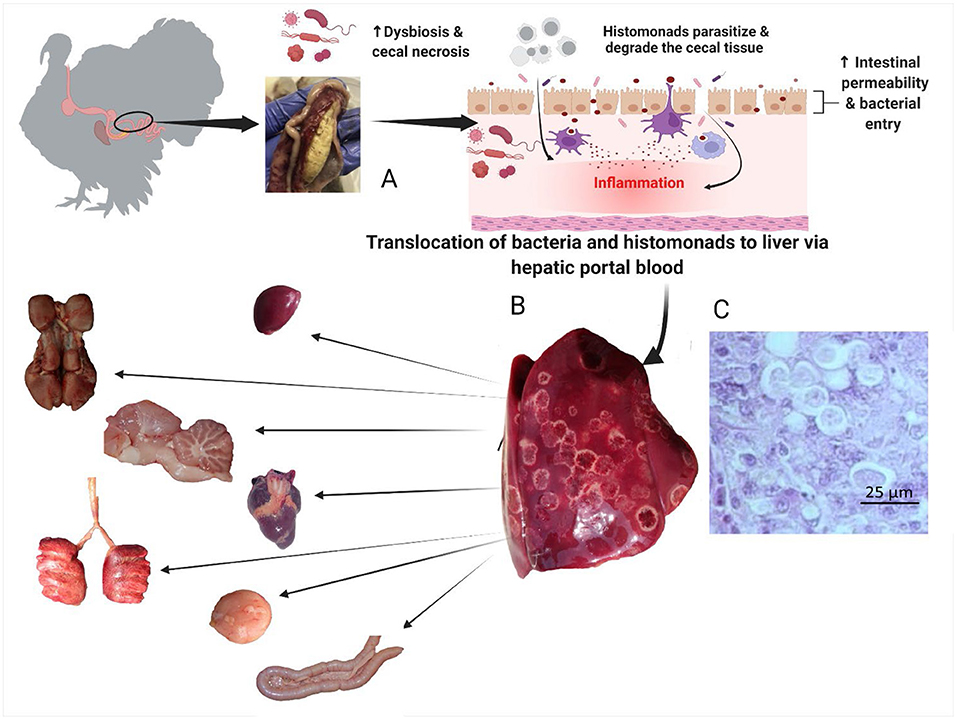
Figure 4. Pathogenesis of histomonosis. The parasite induces a severe inflammatory reaction in the ceca. The inflammatory reaction is followed by necrosis, with dysbiosis causing increased permeability in the ceca (B). This allows bacterial and parasitic translocation to the liver via hepatic portal blood; the resulting pathognomonic lesions are exhibited as target-like liver lesions and caseous cecal cores (A). Histomonas meleagridis in the liver of a turkey, Periodic acid–Schiff (PAS), 40 × (C). From the liver, bacteria and histomonads migrate to other parenchymal organs (spleen, heart, kidneys, pancreas, lungs, brain, bursa of Fabricius) causing chronic systemic inflammation and multiple organ failure (Created with BioRender.com).
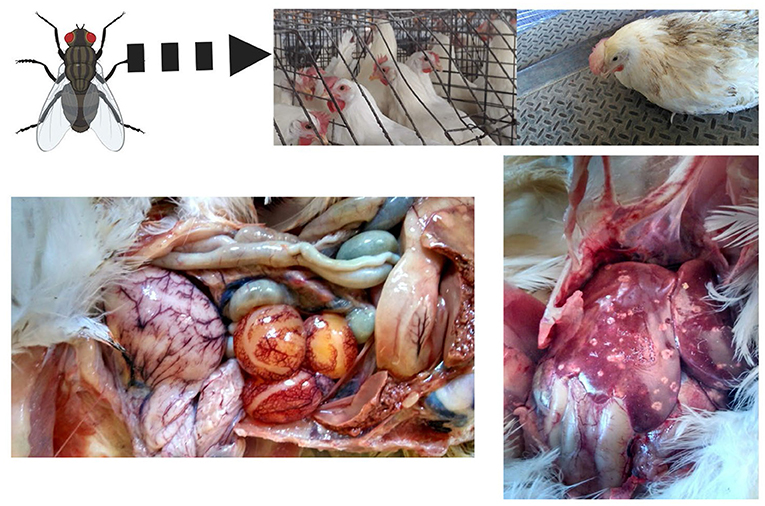
Figure 5. Field case of histomonosis in a layer operation. Turkeys are especially susceptible to Histomonas meleagridis infection, although other gallinaceous birds such as chickens, pheasants, and peafowls can be affected. In this figure, a cage layer hen in a commercial operation with multiple ages and a fly problem developed clinical signs and lesions of histomonosis without the presence of Heterakis spp. (Created with BioRender.com).
A virulent clonal strain of H. meleagridis induced similar mortality and pathology in turkeys regardless of age, sex, or dose (31). A low dose of 3,162 histomonads induced 100% mortality in British United turkeys (BUT-Big6) by 2-weeks post-infection (30). Three different genetic lines of turkeys showed similar susceptibility to histomonosis, although wild Canadian turkeys exhibited higher mortality rates and lower liver lesions than BUT-Big6 or Kelly-Bronze lines (72). Concurrent infection with E. tenella can aggravate the development of histomonosis in broiler chickens, specifically increasing liver lesions (79). Conversely, turkeys co-infected with E. adenoeides and H. meleagridis resulted in significantly reduced cases of histomonosis (24). The dosage and timing of Eimeria vaccination of chickens will influence the severity of aggravation due to histomonosis, although further co-infection studies are necessary to conclude effects of combined pathogens to severity in chickens and turkeys (80).
Bradley and Reid (81) inoculated gnotobiotic (bacteria-free) turkeys with H. meleagridis in combination with either Escherichia coli, Bacillus subtilis, or Clostridium perfringens and suggested that a combination of the protozoa and bacteria populations was required to initiate histomonosis. Incidence of H. meleagridis infection in gnotobiotic chickens and turkeys increased when concurrently challenged with a mixture of E. coli and C. perfringens, whereas histomonosis was lessened with administration of a single bacteria species (82). Healthy turkey ceca contain predominantly (>50%) anaerobic Lactobacillus spp. and relatively low (<1%) coliforms and Enterococcus spp. (61). Salmonella typhimurium, E. coli, and H. meleagridis infections have been found concurrently in broiler chicken flocks (83). Cultures of H. meleagridis were identified to favor obligate anaerobes of the Clostridiaceae family, aerotolerant anaerobes of the Bacteriodaceae family, or facultative to obligate anaerobes of the Baccillaceae family (84). The Proteobacteria phylum increased in relative abundance in birds with severe histomonosis, but E. coli populations were maintained at the same level in turkeys regardless of the level of gut inflammation (85). E. coli mutually benefited histomonad growth in vitro and increased cecal involvement in vivo (60, 86). Co-infection of laying chickens with H. meleagridis and E. coli produced severe dysbiosis, increased microscopic lesions, and enhanced colonization of the cecal tissue (86). Recently however, the gastrointestinal pathology and E. coli load were not associated with severity of histomonosis, while microbiota composition and dysbiosis were directly attributed to the severity of inflammation (85). In addition to providing direct nutrients, bacteria appear to serve a mutualistic role with the protozoa by supplying essential proteins and metabolites during replication, as well as regulating in vitro environmental conditions (87).
Histomonosis has been produced in experimental settings with the intracloacal inoculation of infected liver, cecal tissues, or with a suspension of in vitro cultivated H. meleagridis (15, 62). Variations in host resistance, challenge dose, pathogen virulence, and frequency of exposure are some factors influencing disease severity (88). A case reproductive rate of 8.4 was estimated in a horizontal transmission study and turkeys recovered from histomonosis were shown to remain infectious for 5.7 days after recovery (89). A retrospective data analysis implicated an increased relative risk of male commercial turkey grow-out flocks to contracting histomonosis when located within 1 mile of a broiler breeder flock (90). Lund (74) reported a positive correlation between infective dose (102-105 histomonads/birds) and mortality; conversely, a low dose of 10 histomonads induced 100% mortality in turkeys (91). Liebhart and Hess (92) administered a virulent isolate via oral administration to 1-day-old turkeys with successful initiation of histomonosis, but the oral route of infection remains controversial. Presumably, histomonads cannot survive the low pH in the ventriculus unless protected by a vector such as Heterakis spp. or with a neutral to alkaline pH in the gastrointestinal tract to allow survival of the protozoa (67, 93). H. meleagridis has been shown to persist up to 9 h in non-chlorinated water and fecal droppings and up to 6 h on materials such as feathers and feed (94). Histomonads are fragile when shed unprotected into the environment, but not much is known about the methods for disinfection (95). Consequently, the importance of H. meleagridis-infected water as a possible source of involvement for cloacal transmission has been suggested as an important risk factor (94, 96). Although previously disregarded to form resistant structures, cyst-like forms have recently been described in vitro, but the importance of these structures to pathogenesis is not yet understood (95, 97–100).
Oral challenge with virulent histomonads on day-of-hatch has previously induced histomonosis in turkeys (31), although the oral route in absence of vectors remains somewhat controversial in older birds. Recently, challenge with wild-type H. meleagridis before feeding on day-of-hatch induced disease regardless of oral or cloacal route, presumably due to the near-neutral pH in the proventriculus-ventriculus region allowing the histomonads to survive and parasitize the ceca (101). Interestingly, oral challenge with virulent H. meleagridis at day 21 did not induce histomonosis, further suggesting that the cloacal route rather than the oral route is the primary method for transmitting unprotected histomonads in older birds; however, the oral route should not be disregarded for young birds (101).
Tyzzer (102) indicated the survival of H. meleagridis within heterakid ova for 2 months during winter temperatures. Heterakids can thereby serve as primary transmitters for initial introduction of disease due to infected ova withstanding environmental conditions for long durations (18, 103–105). Histomonads are released when the infected Heterakis spp. larvae hatch in poultry (106, 107). Lifetime fecundity of H. gallinarum is regulated by both inverse density and density-dependent mechanisms (108). Alphitobius diaperinus (darkling beetle or lesser mealworm) function as environmental contaminants for accidental introduction of H. meleagridis into a flock rather than serving as a primary transmitter like Heterakis spp. (109). The importance of A. diaperinus as a reservoir is uncertain due to the persistence of H. gallinarum and H. meleagridis DNA within dead beetles and litter from depopulated houses even after long periods (109, 110). Lumbricus spp. (earthworms) are not required for completion of the heterakid larvae or histomonad life cycles, serving rather as paratenic hosts and mechanical vectors if consumed by poultry (12, 20, 21, 111).
Phylogenetic and Molecular Characterizations
Indirect and blocking ELISAs have been developed for detection of H. meleagridis but have not yet been rigorously tested for specificity or cross-reactivity to other related protozoa commonly found in field isolates (112, 113). An indirect sandwich ELISA has been used successfully to identify H. meleagridis infections in pullet and layer flocks (114). Other parasites such as Tetratrichomonas gallinarum and Blastocystis spp. may be present in field outbreaks and potentially confused with H. meleagridis (42, 115). Polymerase chain reaction (PCR) has been successfully utilized to detect H. meleagridis in samples and infected birds, as well as to differentiate from T. gallinarum and Blastocystis spp. (89, 116–120). DNA presence does not necessarily indicate active infection; therefore, diagnosis of histomonosis is recommended to include microscopy to confirm presence of the protozoa (78, 110, 115). H. meleagridis conforms similarly to other trichomonad parasites in structure and division; close phylogenetic relationships to D. fragilis and Tritrichomonas foetus were identified based on gene sequencing analysis of β-tubulin and small subunit rRNA genes (40, 121–123). Analysis of 18S rRNA and internal transcribed spacer (ITS)-1 sequences has demonstrated a clear distinction between H. meleagridis isolates and other trichomonads such as D. fragilis (98). Genetic sequencing and phylogenetic analysis of 5.8S rRNA and the flanking ITS-1 and ITS-2 regions revealed marked genetic diversity of H. meleagridis isolates (33). Furthermore, combinations of data obtained from Nanopore and Illumina sequencing platforms resulted in the assembly of genome sequences exhibiting gene deletions and truncations for two phenotypically different H. meleagridis isolates, indicating a difference in attenuated and virulent strains (124).
Analysis of 18S rRNA, α-actinin1, and rpb1 genetic loci revealed two different phylogenetic clusters of H. meleagridis isolates in Europe and further identified two genotypes; in contrast, a probed sequence and partial 18S rRNA have displayed genetic similarity of six purportedly different isolates (33, 125). Biological relevance and incidence of these two distinct genotypes have not yet been fully elucidated (19, 125); although Grafl et al. (126) described a field outbreak of male turkeys with H. meleagridis genotype 2 infection as having severe typhlitis with limited hepatic lesions. Using micromanipulation, clonal cultures of H. meleagridis and other protozoa have been established which enable researchers to better understand pathogenicity, morphology, and genetic differences between species (75). Mono-eukaryotic cultures have also been established from mixed field samples containing H. meleagridis, T. gallinarum, and Blastocystis spp., and these monocultures could potentially better mimic field strains as opposed to clonal cultures while removing the interference of other protozoa (127). Thirty-seven unique surface and intracellular antigens were identified through analysis of a cDNA library generated from a monoculture and screened against polyclonal anti-H. meleagridis rabbit sera (128). A cDNA library generated from a non-clonal culture resulted in the identification of 3,425 putative genes belonging to H. meleagridis (84). Hydrogenosome protein-coding sequences and three different α-actinin proteins (α-actinin1, α-actinin2, α-actinin3) were identified and shown to be immunogenic to turkeys and chickens (128, 129). Humoral immune response to H. meleagridis α-actinin1 and α-actinin3 was higher and induced sooner in specific-pathogen-free layer-type chickens as compared to meat-type chickens (68). Shotgun proteomics has been utilized to compare virulent and attenuated mono-eukaryotic monoxenic H. meleagridis; cysteine proteases were the predominant lytic molecules in the virulent exoproteome as compared to the attenuated isolate (130, 131). Mazumdar et al. (132) completed a de novo transcriptome sequencing study utilizing single-cell cloned virulent and attenuated isolates, demonstrating different gene families. Proteomic comparisons have detected expression differences including upregulation of stress response, peptidase, and metabolic proteins in a low-passaged virulent H. meleagridis isolate; whereas an attenuated strain had higher expression of cellular division proteins (133, 134).
Chemotherapy and Prophylaxis
Tyzzer (135) tested several trivalent arsenicals (including arsenious acid, atoxyl, neoarsphenamine, and tryparsamide) as chemotherapeutics against histomonosis, but with inconsistent results. Pentavalent arsenicals such as nitarsone (4-nitrophenyl-arsonic acid; Histostat-50TM), carbasone (4-carbamylamino-phenylarsonic acid), and roxarsone (3-nitro-phenylarsonic acid) offered fewer toxicity concerns than the trivalent compounds for poultry but also exhibited a narrower chemotherapeutic index (16, 136). Carbasone was highly effective in prevention of a field isolate of H. meleagridis (136). Nitroimidazole compounds (including dimetridazole, metronidazole, ornidazole, and tinidazole) were effective in vitro at concentrations of ≥10 μg/ml and in vivo at 200 ppm in the feed, but were toxic if overdosed (137–141). Dimetridazole was highly effective for treating histomonosis and was rapidly metabolized and eliminated by turkeys with no detectable tissue residue (<0.02 ppm) following 3-day post-administration (142). Enheptin-T (2-amino 5-nitrothiazole) was used at 0.05% in the feed with effective prophylaxis against histomonosis, but average weights of turkeys were suppressed in direct proportion to drug inclusion (143). Nithiazide [1-ethyl-3-(5-nitro-2-thiazolyl) urea] was an effective therapeutic in turkeys when administered at 3-day post-infection and was somewhat better tolerated than enheptin-T (144). Benzimidazole compounds, such as albendazole and fenbendazole, were effective in vivo when provided prophylactically and mechanism of action was attributed to damage of heterakid larvae or histomonads residing in the cecal lumen (145).
Research with H. meleagridis waned around the 1970s, partly due to effective antihistomonal compounds alleviating disease outbreak, but research increased again in the early 2000s following the removal of effective drugs and feed additives from poultry production in the European Union and the United States which resulted in a re-emergence of disease due to lack of treatment options (6, 67, 146–148). The nitroimidazoles and nitrofurans were banned in the United States in 1987 and 1991, respectively (90, 149). Nitarsone was the last-remaining prophylactic drug for the treatment of histomonosis until the voluntary removal from the US market in late 2015 because of consumer carcinogenic concerns (147, 149–151). Despite occasional success with antihistomonal candidates in vitro, subsequent in vivo evaluations have failed to conclusively prevent or treat histomonosis (150, 152–156). Boric acid, deoxycholic acid, sodium chlorate, and sodium nitrate are among just a few chemoprophylaxis candidates with antimicrobial or antifungal properties that have been recently tested with in vitro evaluation showing significant antihistomonal properties but with no effective prophylaxis in vivo (154–156). The antibacterial properties of some candidate antihistomonal compounds are known to impact effectiveness in vitro, but histomonads can survive 48 h after destruction of xenic bacterial populations (16, 62, 70, 157). Further complicating the problem, H. meleagridis isolates have varied in susceptibility to candidate compounds in vitro and in vivo (30, 62, 70, 152, 158). Drug resistance was not previously known to occur with H. meleagridis; however, some isolates have developed partial resistance to nitarsone and metronidazole, further emphasizing the necessity of new solutions to prevent histomonosis and supporting the likelihood of different populations of protozoa and corresponding drug susceptibility (38, 159, 160). A comparatively reliable compound to replace the previously used dimetridazole and nitarsone drugs is critically needed, but mitigation of histomonosis remains elusive and inconsistent (6, 16). Adaptations likely need to occur for concentration and administration of compounds for in vivo protection, but effective in vitro evaluation is the initial key step to determining whether to devote resources toward a live animal study (150, 161). In vitro methods are useful for initially evaluating candidate chemoprophylactics, but emphasis is placed on in vivo evaluation against more than one isolate of H. meleagridis before concluding effectiveness.
Paromomycin, an aminoglycoside antibiotic that inhibits protein synthesis, has been effective prophylactically against histomonosis with the target site of action identified as a small subunit rRNA (162–164). Inclusion of paromomycin in the feed at 200 and 400 ppm also reduced Clostridium perfringens counts in excreta while reducing H. meleagridis-related mortalities under experimental conditions (163). Unfortunately, paromomycin seems limited to prophylactic rather than therapeutic properties, as three commercial turkey flocks in Canada were not successful in reducing mortalities with paromomycin sulfate treatment in the feed (165). Taken together, paromomycin sulfate should be further evaluated as a prophylactic compound for in-feed or in-water administration to prevent H. meleagridis infection.
In absence of approved effective drugs or vaccines for histomonosis, the prevailing measure for disease prevention is to minimize exposure to H. meleagridis. Worm treatment programs and flock management to prevent H. gallinarum and accessory hosts such as earthworms and darkling beetles will help to reduce histomonosis incidence, since histomonads cannot survive for long durations if shed unprotected directly into the environment (15, 16). Limiting exposure to mechanical vectors such as rodents, insects, or contaminated litter is critical to reducing potential contamination. Prompt removal of infected birds and utilization of migration barriers are additional control strategies to prevent rapid horizontal transmission in turkey flocks, while de-worming options would be more appropriate to control histomonosis in chickens based on the differences in bird-to-bird transmission (26, 41, 69).
Phytochemicals for Prevention of Histomonosis
Phytogenic compounds offer great potential as alternatives to mitigate histomonosis and improve poultry health since the exclusion of antibiotics (166). Herbal products have received much interest for antihistomonal properties, but in vitro results are often encouraging while in vivo trials yield unsuccessful protection (30, 152, 158). ProtophytTM and NatustatTM, plant-derived proprietary combinations of herbal extracts, were successful antihistomonal products in vitro but generated only limited success in field trials when provided prophylactically (30, 158, 167–169). Further complicating the search and development of antihistomonal drugs, different monoculture strains of H. meleagridis have exhibited varied susceptibilities to natural organic compounds (70). Two proprietary blends of plant extract products containing unspecified amounts of Capsicum essential oils exhibited antihistomonal and antibacterial effects after only 48 h in vitro; furthermore, mode of action was suggested as cell membrane disruption directly on the histomonads rather than attributed to indirect effects of antibacterial reduction, but in vivo studies have not yet been conducted (170). Recently, a dietary supplement (adiCoxSOLPF) comprised of a proprietary mixture of herbal extracts was effective prophylactically and therapeutically against histomonosis in a turkey breeder flock (171). With increasing demand for organic-raised poultry, naturally derived plant compounds offer a certain attraction as they could potentially be utilized in both organic and traditional production facilities. Plant-based compounds are often relatively cheap to produce, leading to a greater likelihood for industry application (161).
Quinine, an alkaloid obtained from Cinchona tree bark, has been successfully utilized to combat malaria (172). Early researchers postulated its potential for treating histomonosis; however, researchers hypothesized that an antihistomonal compound would have to be active more than just locally within the intestines because H. meleagridis embeds within the cecal lining and migrates to hepatic tissue (2, 103). Tyzzer (135) observed no reduction in histomonosis following injection of unspecified levels of quinine into the veins or muscles of turkeys. Delaplane and Stuart (173) reported quinine sulfate to be ineffective against H. meleagridis infection but did not specify the dose or route of administration. Farmer (174) injected 0.1 ml of 10% quinine iodobismuthate with no apparent protection against histomonosis. Tyzzer and Fabyan (103) suggested that a possible reason for the failure of compounds utilized in human amebic infections to protect poultry from histomonosis could be due to histomonads exhibiting a predominantly flagellated form rather than solely an amoebic form, leading to some products being amebicidal but not antihistomonal. Ensuring delivery of chemoprophylactic candidates directly to the ceca is a challenge, and quinine, although recently shown to be an effective antihistomonal in vitro, may not have reached the ceca in sufficient concentration to impair the protozoa when evaluated in vivo (175). Previously, chickens recognized the bitter taste of quinine and reduced feed intake of diets containing more than 0.2% quinine, but threshold levels have not been established for turkeys (176). A 0.2% dietary inclusion of quinine was hypothesized to be maximum for turkeys as well; however, the days 0–10 body weight gain in the quinine diets was not different (p > 0.05) as compared to the basal diet (175). Turkeys may perceive the bitter taste of quinine differently from chickens and subsequently have higher threshold levels than 0.2%, but the impact to performance at higher inclusion levels is unknown. Other antimalarial compounds such as the herb Artemisia annua and plant extracts have been tested against H. meleagridis with limited success in vitro but no protection was transferred to birds when tested in vivo (152, 170).
Immune Response to H. meleagridis Infection
Turkeys and chickens recovered from H. meleagridis infection have shown a degree of natural resistance, although both species may retain histomonads sub-clinically and thereby serve as carriers (5, 177). Joyner (178) administered 0.05% dimetridazole in the water to H. meleagridis-infected turkeys, and the recovered turkeys were resistant to re-infection which suggested a level of acquired immunity. Protective immunity was observed in birds that recovered from histomonosis and were then subsequently re-infected with H. meleagridis, but further attempts with immunization have been inconsistent (18, 102, 103, 177, 179, 180). Sera recovered from immune birds failed to confer robust protection to histomonosis when injected into the peritoneum of naïve poultry that were subsequently challenged intracloacally with H. meleagridis-infected liver homogenate (180–182). Passive immunity (via peritoneal injection of antisera) or active immunity (via intramuscular or intraperitoneal injection of lysed clonal H. meleagridis) failed to protect against wild-type challenge (183, 184). Turkeys surviving H. meleagridis infection have exhibited resistance to re-infection while still maintaining populations of the protozoa within the ceca (182). Humoral immunity does not seem to be the primary component of protective immunity to histomonosis, although antibodies may work in combination with local immunity initiated by leukocytes in the ceca (182).
Clarkson (181) reported that turkeys exhibited decreased albumin and elevated globulin concentrations at 12-day post-infection as compared to the non-challenged controls. Similarly, albumin concentrations greatly decreased by 9-day post-infection in chickens subjected to H. meleagridis infection, with normal levels of albumin and globulin fractions restored by 12-day post-infection, suggesting disease recovery (185). The immune barrier in purportedly histomonosis-resistant chickens was suggested to be limited to cecal epithelial tissue as H. gallinarum could disrupt and overcome any developed immunity (74). Natural and experimental H. meleagridis infection produced antibodies in both chickens and turkeys but transfer of antibodies to naïve birds did not successfully confer protection (180, 184). Subsequently, Clarkson (180) suggested that antibody production alone was not a good indicator of histomonosis recovery or immunity to re-infection. Antibody titers of passively immunized birds were increased compared to pre-immunized groups; however, no protection was induced against intracloacal infection with 3 × 105 H. meleagridis, possibly due to the experimental challenge dose not accurately mimicking a natural challenge, antibody levels lower than needed for protection, or more likely, serum antibodies not primarily responsible for protection against H. meleagridis infection (184). Immunoglobulin A (IgA) levels have been shown to increase throughout the intestine, while immunoglobulin G (IgG) levels particularly increased in the ceca following infection with an established clonal H. meleagridis isolate (186).
Heterophils begin to accumulate around histomonads following initial infection, but the protozoa secrete tissue-degrading enzymes to phagocytose leukocytes (44). Total numbers of heterophils increase throughout the body as H. meleagridis migrates to parasitize other tissues; other leukocytes involved include macrophages, giant cells, and plasma cells (44, 64, 119, 187). Once the histomonads invade the cecal submucosa or enter the portal blood, degenerating H. meleagridis can be observed within the gut-associated lymphoid tissue (44). Plasma levels of glutamic oxaloacetic transaminase can indicate cellular damage and this enzyme can increase in turkeys with liver and cecal damage from histomonosis (28, 29). CD4+ and CD8α+ T cells have been implicated in the immune response to histomonosis (188–190). Recently, populations of CD4+, CD8α+, and non-CD4+CD8α+ T cells in the liver and spleen of turkeys were induced following administration of attenuated H. meleagridis as a putative vaccine and subsequent virulent infection (191). Comparative study of chickens and turkeys indicated that vaccination with a monoxenic, clonal culture of live-attenuated H. meleagridis resulted in higher systemic immune response in turkeys as compared to chickens, with increased levels of interferon (IFN)-γ producing CD4+ T cells confirmed in the spleens of infected chickens as compared to turkeys (191). Increased T-helper cell type-1 (Th1) and type-2 (Th2) cytokine responses of IFN-γ and IL-13 occurred in chickens which were co-infected with H. gallinarum and H. meleagridis (192). Chickens developed a stronger pro-inflammatory innate immune response than turkeys, along with higher antibody levels, with specific increase in the Th2 response in cecal and liver tissues to mitigate infection (188). Despite the extracellular nature of H. meleagridis which would be expected to stimulate differentiation of Th2 cells, immune response to this pathogen was suggested to be dominated by Th1 rather than Th2 cells (190–193). Turkeys appeared to have a delayed and uncontrolled immune response as compared to chickens when infected with H. meleagridis, allowing greater tissue destruction and ultimately higher mortality in turkeys (194).
Attempted Vaccination With Attenuated Isolates
Tyzzer (102) evaluated avirulent field strains of H. meleagridis for immunization against histomonosis, but inoculation of turkeys was required at a young age and constant re-infection was necessary to maintain a level of effective protection. Partial protection was conferred with an attenuated isolate against subsequent cloacal challenge with a virulent isolate; however, administration of histomonads as an immunization incorporated into Heterakis spp. ova and likewise challenged did not satisfactorily confer protection (195, 196). The resulting conclusion was that the low-virulent histomonads were not introduced in sufficient numbers via heterakid ova to successfully initiate immune response to protect against virulent challenge (195, 196). Tyzzer (102, 179) reported attenuation of H. meleagridis following repeated passage in vitro but attempts with immunization did not produce consistent protection. An isolate repeatedly passaged in vitro for 6 years resulted in loss of immunizing ability to chickens and turkeys (196). Further study observed a steady decline of immunizing ability of attenuated histomonads after 730, 766, and 1,000 passages in vitro (197). Specifically, passage 1,000 was non-pathogenic and had lost nearly all ability to confer protection to either chickens or turkeys against virulent challenge (197).
Long-term serial passaging in vitro places selective pressures on H. meleagridis and co-cultured bacterial populations. Freshly obtained field samples of histomonads could not grow in the limited bacterial populations of attenuated culture media; similarly, the attenuated protozoa were unable to survive with the field isolates of cecal bacteria (196). Importantly, in vitro attenuation of H. meleagridis occurred independently of bacterial populations in culture media (60). In vitro growth of H. meleagridis Hm-L1 strain at 41.5°C for 9 weeks resulted in low pathogenicity while histomonads stored in liquid nitrogen maintained their original virulence (198, 199). Serial in vivo passaging of the Hm-L1 attenuated strain from chicken-to-chicken or turkey-to-turkey restored the strain to original virulence (198, 199). Differences in virulence have been found within H. meleagridis isolates obtained from different geographical locations, in addition to varied loss of pathogenicity following repeated passaging (131). Furthermore, subpopulations of serially passaged monocultures originating from the same parental isolate have shown a marked difference in virulence, supporting the idea of genetic mutation through repeated serial passaging in vitro (131). Long-term passaging in vitro (>290 serial passages) resulted in a phenotype shift toward greater tenacity of histomonad survival at lower temperatures and improved growth rates (37). Gross lesion scoring and histology samples have demonstrated the lowered pathogenicity and reduced ability of attenuated isolates to invade host tissues (200). After 295 serial passages in vitro, an avirulent strain of H. meleagridis parasitized only the cecal region with no translocation to other tissues in chickens or turkeys, while a virulent strain could be identified in cecal, hepatic, and lung tissues (200).
Vaccination attempts for histomonosis have yielded some success in controlled experimental conditions, but a histomonosis vaccine has not yet been developed for commercial application (91, 101, 164, 183, 194, 201–203). A clonal in vitro attenuated strain of H. meleagridis administered cloacally as a vaccine at day 14 protected turkeys which were subsequently challenged on day 42 with a virulent strain; in-contact turkeys from the vaccination were also resistant to subsequent infection (183). Furthermore, birds which were administered an attenuated clonal strain as a vaccine were negative for H. meleagridis DNA in the liver (183). Oral administration of in vitro attenuated H. meleagridis to turkeys at day-of-hatch has protected against subsequent wild-type challenge with no adverse effects to performance data during the vaccination phase; the oral route would be a preferable administration route for the poultry industry (91). Under experimental conditions, vaccination of layer chickens with attenuated histomonads prevented a drop in egg production upon virulent challenge and pathological histomonosis lesions were also reduced (203). In vivo serial passaging five times in chickens and turkeys did not revert virulence to an in vitro attenuated strain, demonstrating stability and safety of attenuated histomonads as vaccine candidates (204). An attenuated clonal strain (passage 295) induced cross-protective immunity in turkeys against subsequent challenge with heterologous virulent isolates; however, vaccination occurred at 1 day of age and a booster vaccination occurred at day 14, with challenge administration at 6 weeks of age (205). Repeated intracloacal passaging of H. meleagridis in turkeys produced an isolate of low virulence which was successfully used to induce protection against a virulent strain (206). Candidate vaccination isolates have been shown distinctly attenuated as indicated by lowered mortalities (p < 0.05), lowered lesion scores (p < 0.05), and similar body weight gain (BWG) (p > 0.05) as the non-challenged controls during vaccination phases (101). This information is consistent with previous research indicating attenuation of H. meleagridis following repeated in vitro passage (102, 131, 179, 196). Importantly, administration of non-clonal vaccination isolates on day 14 has conferred protection against challenge with homologous and heterologous virulent isolates; moreover, these conditions potentially better portrayed the field environment where turkeys are exposed to multiple isolates (101). More research remains necessary for histomonosis vaccine development and to elucidate practical methods for industry application.
Final Remarks
Biosecurity measures to prevent exposure to H. meleagridis or vectors of this protozoa are important to reduce histomonosis incidence due to the absence of vaccines or approved drugs. Proper management practices are critical to reducing disease incidence, as birds experimentally reared in a non-challenged environment do not contract histomonosis. Although separate rearing of poultry (e.g., turkeys raised separately from chickens) can reduce disease incidence by limiting contact between asymptomatic carriers and susceptible hosts, an effective prophylactic or vaccination program is still greatly needed. Pairing in vitro and in vivo experiments is necessary to ensure effectiveness of candidate antihistomonal compounds.
Despite immunological research advancements, a histomonosis vaccine has not been developed for commercial application (164, 194, 202). Clonal in vitro attenuated histomonads have been administered orally or cloacally with efficacious protection in experimental settings against virulent challenge without negative performance impacts; however, evaluations have not yet occurred in field conditions against heterologous, multi-isolate challenges (91, 183, 203). Day-of-hatch oral vaccination with live-attenuated histomonads was previously reported as effective, but a booster vaccination was recommended at day 14 for established protection (91, 205). Recent vaccination experiments demonstrated that day-of-hatch administration of attenuated isolates either orally or cloacally did not protect turkeys against subsequent wild-type challenge (101), contrary to previously reported success with oral vaccination at this age (91, 205). Unfortunately, utilizing live histomonads would be difficult for industry application due to the required intracloacal administration, as well as the additional concerns of attenuation stability and inconsistent protective immunity (6, 9). In practicality, the administration of live-attenuated histomonads on a commercial scale with the current methodologies seems unlikely due to the high cost of cell culture propagation and application complexities, although the benefit to further develop a histomonosis vaccine would be tremendous (5, 202).
The overall review of literature reflects the difficulties in mitigating histomonosis, especially in recent years. Dietary inclusion of antihistomonal compounds such as quinine alone was not encouraging for prevention of H. meleagridis infection in turkeys, but vaccination appeared somewhat efficacious when live-attenuated histomonads were administered at day 14 via the cloacal route. Unfortunately, the protection against subsequent wild-type challenge of vaccinated turkeys was neither consistent nor robust throughout the literature. Further research should be conducted with phytochemicals as these compounds may offer a natural remedy for histomonosis that could be both economical for the industry and acceptable to the consumer. Vaccination should be pursued further, especially to elucidate the administration route, dose, and age of bird. Taken together, this information is encouraging for immunity to histomonosis, but the administration of a vaccine and possible requirement for booster vaccination with the live-attenuated method is more experimentally interesting rather than industry applicable.
Author Contributions
LB and CV developed the conceptualization and wrote the first draft of the manuscript. LB, VP-G, and GT-I conceptualized and created the figures. LB, GT-I, BG, BH, and CV participated in the design, analysis, presentation, and writing of the manuscript. All authors contributed to the article and approved the submitted version.
Funding
This research was supported in part by the funds provided by the USDA-NIFA Sustainable Agriculture Systems, Grant No. 2019-69012-29905. Title of the project: Empowering US Broiler Production for Transformation and Sustainability USDA-NIFA (Sustainable Agriculture Systems): No. 2019-69012-29905.
Conflict of Interest
The authors declare that the research was conducted in the absence of any commercial or financial relationships that could be construed as a potential conflict of interest.
Publisher's Note
All claims expressed in this article are solely those of the authors and do not necessarily represent those of their affiliated organizations, or those of the publisher, the editors and the reviewers. Any product that may be evaluated in this article, or claim that may be made by its manufacturer, is not guaranteed or endorsed by the publisher.
References
1. Cushman S. The Production of Turkeys. Kingston, RI: Agricultural Experiment Station, Rhode Island College of Agriculture and Mechanical Arts (1893).
2. Smith T. An infectious disease among turkeys caused by protozoa (infectious entero-hepatitis). USDA Bur Anim Ind Bull. (1895) 8:3–27.
3. Tyzzer EE. The flagellate character and reclassification of the parasite producing “Blackhead” in turkeys: Histomonas (gen. nov.) meleagridis (Smith). J Parasitol. (1920) 6:124–31. doi: 10.2307/3271065
4. Farmer RK, Hughes DL, Whiting G. Infectious enterohepatitis (blackhead) in turkeys: a study of the pathology of the artificially induced disease. J Comp Path. (1951) 61:251–62. doi: 10.1016/S0368-1742(51)80025-0
5. Joyner LP, Davies SFM, Kendall SB. Chemotherapy of histomoniasis. In: Experimental Chemotherapy, Vol. 1. New York, NY: Academic Press (1963). doi: 10.1016/B978-1-4832-3178-5.50013-7
6. Hess M, Liebhart D, Bilic I, Ganas P. Histomonas meleagridis—New insights into an old pathogen. Vet. Parasitol. (2015) 208:67–76. doi: 10.1016/j.vetpar.2014.12.018
7. Clarke LL, Beckstead RB, Hayes JR, Rissi DR. Pathologic and molecular characterization of histomoniasis in peafowl (Pavo cristatus). J Vet Diagn Invest. (2017) 29:237–41. doi: 10.1177/1040638716687002
8. Davidson WR, Doster GL. Blackhead Disease Does Not Really Cause Black Heads. Edgefield, SC: National Wild Turkey Federation (1994).
9. Hess M, McDougald L. Histomoniasis (blackhead) and other protozoan diseases of the intestinal tract. In: Glisson JR, McDougald LR, Nolan LK, Suarez DL, Nair VL, Swayne E, editors. Diseases of Poultry. 13th edn. Ames, IA: Wiley-Blackwell (2013).
10. Kassai T, Cordero del Campillo M, Euzeby J, Gaafar S, Hiepe T, Himonas CA. Standardized nomenclature of animal parasitic diseases (SNOAPAD). Vet Parasitol. (1988) 29:299–326. doi: 10.1016/0304-4017(88)90148-3
11. Lund EE, Chute AM. Heterakis and histomonas infections in young peafowl, compared to such infections in pheasants, chickens, and turkeys. J Wildl Dis. (1972) 8:352–8. doi: 10.7589/0090-3558-8.4.352
12. Friend M, Franson JC, Ciganovich EA. Field Manual of Wildlife Diseases: General Field Procedures and Diseases of Birds. Madison, WI: USGS (1999).
13. Clark SR, Froebel L. Turkey Industry Annual Report—Current Health Industry Issues Facing the US Turkey Industry. (2020). Retrieved from https://huvepharma.com/news/article/2020-turkey-industry-annual-report-current-health-and-industry-issues-facing-the-us-turkey-industry/
14. Graybill HW, Smith T. Production of fatal blackhead in turkeys by feeding embryonated eggs of Heterakis papillosa. J Exp Med. (1920) 31:647–55. doi: 10.1084/jem.31.5.647
15. Tyzzer EE, Collier J. Induced and natural transmission of blackhead in the absence of Heterakis. J Infect Dis. (1925) 37:265–76. doi: 10.1093/infdis/37.3.265
16. McDougald LR. Blackhead disease (histomoniasis) in poultry: a critical review. Avian Dis. (2005) 49:462–76. doi: 10.1637/7420-081005R.1
17. Curtice C. Further Experiments in Connection With the Blackhead Disease of Turkeys. Agricultural Experiment Station of the Rhode Island College of Agriculture and Mechanic Arts (1907).
18. Tyzzer EE. Problems and observations concerning the transmission of blackhead infection in turkeys. Proc Am Philos Soc. (1932) 71:407–10.
19. Popp C, Hauck R, Balczulat S, Hafez HM. Recurring histomonosis on an organic farm. Avian Dis. (2011) 55:328–30. doi: 10.1637/9596-110810-Case.1
20. Cupo KL, Beckstead RB. Heterakis gallinarum, the cecal nematode of gallinaceous birds: a critical review. Avian Dis. (2019) 63:381–8. doi: 10.1637/0005-2086-63.3.381
21. Cupo KL, Beckstead RB. PCR detection of heterakis gallinarum in environmental samples. Vet Parasitol. (2019) 271:1–6. doi: 10.1016/j.vetpar.2019.05.011
22. Sorvari R, Naukkarinen A, Sorvari TE. Anal sucking-like movements in the chicken and chick embryo followed by the transportation of environmental material to the bursa of fabricius, caeca, caecal tonsils. Poult Sci. (1977) 56:1426–9. doi: 10.3382/ps.0561426
23. Hu J, Fuller L, McDougald LR. Infection of turkeys with Histomonas meleagridis by the cloacal drop method. Avian Dis. (2004) 48:746–50. doi: 10.1637/7152
24. McDougald LR, Fuller L. Blackhead disease in turkeys: direct transmission of Histomonas meleagridis from bird to bird in a laboratory model. Avian Dis. (2005) 49:328–31. doi: 10.1637/7257-081004R.1
25. Scanes CG, Pierzchala-Koziec K. Biology of the gastro-intestinal tract in poultry. Avian Biol Res. (2014) 7:193–222. doi: 10.3184/175815514X14162292284822
26. Hu J, McDougald LR. Direct lateral transmission of Histomonas meleagridis in turkeys. Avian Dis. (2003) 47:489–92. doi: 10.1637/0005-2086(2003)047<0489:DLTOHM>2.0.CO;2
27. Armstrong PL, McDougald LR. The infection of turkey poults with Histomonas meleagridis by contact with infected birds or contaminated cages. Avian Dis. (2011) 55:48–50. doi: 10.1637/9568-100710-Reg.1
28. Al-Khateeb GH, Hansen MF. Plasma glutamic oxalacetic transaminase as related to liver lesions from histomoniasis in turkeys. Avian Dis. (1973) 17:269–73. doi: 10.2307/1589208
29. Al-Khateeb GH, Hansen MF. Plasma enzymes as a measure of susceptibility of chickens and turkeys to infection with Histomonas meleagridis. Avian Dis. (1974) 18:507–14. doi: 10.2307/1589006
30. van der Heijden HMJF, Landman WJM. In vivo effect of herbal products against Histomonas meleagridis in turkeys. Avian Pathol. (2008) 37:45–50. doi: 10.1080/03079450701784883
31. Liebhart D, Grabensteiner E, Hess M. A virulent mono-eukaryotic culture of Histomonas meleagridis is capable of inducing fatal histomonosis in different aged turkeys of both sexes, regardless of the infective dose. Avian Dis. (2008) 52:168–72. doi: 10.1637/8107-090707-ResNote
32. Cepicka I, Hampl V, Kulda J. Critical taxonomic revision of parabasalids with description of one new genus and three new species. Protistology. (2010) 161:400–33. doi: 10.1016/j.protis.2009.11.005
33. Lollis L, Gerhold R, McDougald L, Beckstead R. Molecular characterization of Histomonas meleagridis and other parabasalids in the United States using the 5.8S, ITS-1, and ITS-2 rRNA regions. J Parasitol. (2011) 97:610–5. doi: 10.1645/GE-2648.1
34. Bayon HP, Bishop A. Cultivation of Histomonas meleagridis from the liver lesions of a hen. Nature. (1937) 139:370–1. doi: 10.1038/139370b0
35. Bishop A. Histomonas meleagridis in domestic fowls (Gallus gallus). Cultivation and experimental infection. Parasitology. (1938) 30:181–94. doi: 10.1017/S0031182000025749
36. Hess M, McDougald LR. Histomoniasis (histomonoasis, blackhead disease). In: Swayne DE., Boulianne M, Logue CM, McDougald LR, Nair V, Suarez DL, editors. Diseases of Poultry. 14th edn. Ames, IA: Wiley-Blackwell (2020). p. 1223–30.
37. Gruber J, Ganas P, Hess M. Long-term in vitro cultivation of Histomonas meleagridis coincides with the dominance of a very distinct phenotype of the parasite exhibiting increased tenacity and improved cell yields. Parasitology. (2017) 144:1253–63. doi: 10.1017/S0031182017000646
38. Long PL, Current WL, Noble GP. Parasites of the christmas turkey. Parasitol Today. (1987) 3:360–6. doi: 10.1016/0169-4758(87)90241-9
39. Lund EE. Histomonas wenrichi n. sp. (Madtigophora: Mastigamoebidae), a nonpathogenic parasite of gallinaceous birds. J Protzool. (1963) 10:401–4. doi: 10.1111/j.1550-7408.1963.tb01696.x
40. Honigberg BM, Bennett CJ. Lightmicroscopic observations on structure and division of Histomonas-meleagridis (Smith). J Protozool. (1971) 18:687–97. doi: 10.1111/j.1550-7408.1971.tb03398.x
41. Fine PEM. Quantitative studies on the transmission of Parahistomonas wenrichi by ova of Heterakis gallinarum. Parasitology. (1975) 70:407–17. doi: 10.1017/S0031182000052185
42. Tyzzer EE. Developmental phases of the protozoon of “blackhead” in turkeys. J Med Res. (1919) 40:1–30.
43. Mielewczik M, Mehlhorn H, Al-Quraishy S, Grabensteiner E, Hess M. Transmission electron microscopic studies of stages of Histomonas meleagridis from clonal cultures. Parasitol Res. (2008) 103:745–50. doi: 10.1007/s00436-008-1009-1
44. Bleyen N, Mast J, De Gussem K, De Guseem J, De Gussem M, Godderis BM. Histomonas meleagridis: a new focus on a re-emerging protozoan parasite. In: LaMann GV, editor. Veterinary Parasitology. New York, NY: Nova Science Publishers (2010). p. 1–47.
45. Lesser E. Cultivation of Histomonas meleagridis in a modified tissue culture medium. J Parasitol. (1960) 6:686. doi: 10.2307/3275512
46. Hauck R, Armstrong PL, McDougald LR. Histomonas meleagridis (Protozoa: Trichomonadidae): analysis of growth requirements in vitro. J Parasitol. (2010) 96:1–7. doi: 10.1645/GE-1985.1
47. van der Heijden HMJF, Landman WJM. Improved culture of Histomonas meleagridis in a modification of dwyer medium. Avian Dis. (2007) 51:986–88. doi: 10.1637/8018-051007-REVIEWR.1
48. van der Heijden HMJF, McDougald LR, Landman WJM. High yield of parasites and prolonged in vitro culture of Histomonas meleagridis. Avian Pathol. (2005) 34:505–8. doi: 10.1080/03079450500368474
49. Gruber J, Pletzer A, Hess M. Cholesterol supplementation improves growth rates of Histomonas meleagridis in vitro. Exp Parasitol. (2018) 185:53–61. doi: 10.1016/j.exppara.2018.01.007
50. Chute MB, Chute AM. Freeze preservation of Histomonas meleagridis. Poult Sci. (1969) 48:2189–91. doi: 10.3382/ps.0482189
51. Honigberg BM, Dwyer DM. Maintenance of Histomonas meleagridis in liquid nitrogen. J Protozool. (1969) 16:18–9.
52. Gerhold RW, Lollis LA, Beckstead RB, McDougald LR. Establishment of culture conditions for survival of Histomonas meleagridis in transit. Avian Dis. (2010) 54:948–50. doi: 10.1637/9222-122209-ResNote.1
53. McDougald LR, Galloway RB. Blackhead disease: in vitro isolation of Histomonas meleagridis as a potentially useful diagnostic aid. Avian Dis. (1973) 17:847–50. doi: 10.2307/1589053
54. Hirsch DS. Attempts at Axenic Cultivation of Histomonas meleagridis. (M.S. Thesis), Kansas State University, Manhattan, KS (1975).
55. Lesser E. Replacement of serum in the cultivation of Histomonas meleagridis. J Parasitol. (1960) 46:271. doi: 10.2307/3275188
56. Lesser E. In vitro cultivation of Histomonas meleagridis free of demonstrable bacteria. J Protozool. (1961) 8:228–30. doi: 10.1111/j.1550-7408.1961.tb01208.x
57. Lesser E. Effect of amphotericin-B on in vitro growth of Histomonas meleagridis. J Parasitol. (1963) 49:329. doi: 10.2307/3276007
58. Lesser E. Studies on the in vitro growth of Histomonas meleagridis with three different mixed bacterial flora. Proc Helm Soc Wash. (1964) 31:263–4.
59. Lesser E. Studies on the in vitro growth of Histomonas meleagridis with single species of bacteria. Proc Helm Soc Wash. (1964) 31:365–6.
60. Ganas P, Liebhart D, Glösmann M, Hess C, Hess M. Escherichia coli strongly supports the growth of Histomonas meleagridis, in a monoxenic culture, without influence on its pathogenicity. Int J Parasitol. (2012) 42:893–901. doi: 10.1016/j.ijpara.2012.07.007
61. Harrison AP Jr, Hansen PA. The bacterial flora of the cecal feces of healthy turkeys. J Bacteriol. (1950) 59:197–210. doi: 10.1128/jb.59.2.197-210.1950
62. Berks G, Neal R. The effect of some drugs upon Histomonas meleagridis in vitro. Ann Trop Med Parasitol. (1952) 46:68–71. doi: 10.1080/00034983.1952.11685507
63. Cortes PL, Chin RP, Bland MC, Crespo R, Shivaprasad HL. Histomoniasis in the bursa of fabricius of chickens. Avian Dis. (2004) 48:711–5. doi: 10.1637/7175-030404R
65. Singh A, Weissenbock H, Hess M. Histomonas meleagridis: immunohistochemical localization of parasitic cells in formalin-fixed, paraffin-embedded tissue sections of experimentally infected turkeys demonstrates the wide spread of the parasite in its host. Exp Parasitol. (2008) 118:505–13. doi: 10.1016/j.exppara.2007.11.004
66. Sentíes-Cué G, Chin R, Shivaprasad HL. Systemic histomoniasis associated with high mortality and unusual lesions in the bursa of fabricius, kidneys, and lungs in commercial turkeys. Avian Dis. (2009) 53:231–8. doi: 10.1637/8363-051508-Reg.1
67. Spencer T. Parasite Management for Natural Organic Poultry: Blackhead in Turkeys. ATTRA (2010). Available online at: https://citeseerx.ist.psu.edu/viewdoc/download?doi=10.1.1.676.4349&rep=rep1&type=pdf
68. Lotfi A, Hauck R, Olias P, Hafez HM. Pathogenesis of histomonosis in experimentally infected specific-pathogen-free (SPF) layer-type chickens and SPF meat-type chickens. Avian Dis. (2014) 58:427–32. doi: 10.1637/10782-012814-Reg.1
69. Hu J, Fuller L, Armstrong PL, McDougald LR. Histomonas meleagridis in chickens: attempted transmission in the absence of vectors. Avian Dis. (2006) 50:277–9. doi: 10.1637/7431-090605R.1
70. Grabensteiner E, Arshad N, Hess M. Differences in the in vitro susceptibility of mono-eukaryotic cultures of Histomonas meleagridis, Tetratrichomonas gallinarum and Blastocystis sp. to natural organic compounds. Parasitol Res. (2007) 101:193–9. doi: 10.1007/s00436-007-0459-1
71. Chute AM, Lund EE, Wilkins GC. Comparative responses of white leghorn and new hampshire chickens to experimental infections with Histomonas meleagridis and Heterakis gallinarum. Poult Sci. (1976) 55:710–3. doi: 10.3382/ps.0550710
72. Lotfi A, Hafez HM. Susceptibility of different turkey lines to Histomonas meleagridis after experimental infection. Parasitol Res. (2009) 105:113–6. doi: 10.1007/s00436-009-1369-1
73. Metzler-Zebeli BU, Magowan E, Hollmann M, Ball MEE, Molnár A, Witter K, et al. Differences in intestinal size, structure, and function contributing to feed efficiency in broiler chickens reared at geographically distant locations. Poult Sci. (2018) 97:578–91. doi: 10.3382/ps/pex332
74. Lund EE. The progress of histomoniasis (blackhead) in turkeys as related to the size of the infective dose. Poult Sci. (1955) 34:127–30. doi: 10.3382/ps.0340127
75. Hess M, Kolbe T, Grabensteiner E, Prosl H. Clonal cultures of Histomonas meleagridis, Tetratrichomonas gallinarum and a Blastocystis sp. established through micromanipulation. Parasitology. (2006) 133:547–54. doi: 10.1017/S0031182006000758
76. Dolka B, Zbikowski A, Dolka Szeleszczuk IP. Histomonosis – an existing problem in chicken flocks in Poland. Vet Res Commun. (2015) 39:189–95. doi: 10.1007/s11259-015-9637-2
77. Nguyen DT, Bilic I, Jaskulska B, Hess M, Le DQ, Hua LNL, et al. Prevalence and genetic characterization of Histomonas meleagridis in chickens in Vietnam. Avian Dis. (2015) 59:309–14. doi: 10.1637/10964-102414-Reg
78. Daş G, Wachter L, Stehr M, Bilic I, Grafl B, Wernsdorf P, et al. Excretion of Histomonas meleagridis following experimentall co-infection of distinct chicken lines with Heterakis gallinarum and Ascaridia galli. Parasit Vect. (2021) 14:1–15. doi: 10.1186/s13071-021-04823-1
79. McDougald LR, Hu J. Blackhead disease (Histomonas meleagridis) aggravated in broiler chickens by concurrent infection with cecal coccidiosis (Eimeria tenella). Avian Dis. (2001) 45:307–12. doi: 10.2307/1592969
80. Chadwick E, Malheiros R, Oviedo E, Noboa HAC, Ospina GAQ, Wisaquillo MCA, et al. Early infection with Histomonas meleagridis has limited effects on broiler breeder hens' growth and egg production and quality. Poult Sci. (2020) 99:4242–8. doi: 10.1016/j.psj.2020.05.020
81. Bradley RE, Reid WM. Histomonas meleagridis and several bacteria as agents of infectious enterohepatitis in gnotobiotic turkeys. Exp Parasitol. (1966) 19:91–101. doi: 10.1016/0014-4894(66)90057-9
82. Springer WT, Johnson J, Reid WM. Histomoniasis in gnotobiotic chickens and turkeys: biological aspects of the role of bacteria in the etiology. Exp Parasitol. (1970) 28:383–92. doi: 10.1016/0014-4894(70)90106-2
83. Ganapathy K, Salamat MH, Lee CC, Johara MY. Concurrent occurrence of salmonellosis, colibaccillosis and histomoniasis in a broiler flock fed with antibiotic-free commercial feed. Avian Pathol. (2000) 29:639–42. doi: 10.1080/03079450020016000
84. Klodnicki ME, McDougald LR, Beckstead RB. A genomic analysis of Histomonas meleagridis through sequencing of a cDNA library. J Parasitol. (2013) 99:264–9. doi: 10.1645/GE-3256.1
85. Abdelhamid KM, Rychlik I, Hess C, Hatfaludi T, Crhanova M, Karasova D, et al. Typhlitis induced by Histomonas meleagridis affects relative but not the absolute Escherechia coli counts and invasion in the gut in turkeys. Vet Res. (2021) 52:1–12. doi: 10.1186/s13567-021-00962-6
86. Abdelhamid MK, Quijada NM, Dzieciol M, Hatfaludi T, Bilic I, Selberherr E, et al. Co-infection of chicken layers with Histomonas meleagridis and avian pathogenic Escherichia coli is associated with dysbiosis, cecal colonization and translocation of the bacteria from the gut lumen. Front Microbiol. (2020) 11:586437. doi: 10.3389/fmicb.2020.586437
87. Bilic I, Hess M. Interplay between Histomonas meleagridis and bacteria: mutualistic or predator-prey? Trends Parasitol. (2020) 36:232–5. doi: 10.1016/j.pt.2019.12.015
88. Collett SR. Principles of disease prevention, diagnosis, and control. In: Swayne E, Glisson JR, McDougald LR, Nolan LK, Suarez DL, Nair VL, editors. Diseases of Poultry. 13th ed. Ames, IA: Wiley-Blackwell (2013). p. 3–60.
89. Landman WJM, ter Veen C, van der Heijden HMJF, Klinkenberg D. Quantification of parasite shedding and horizontal transmission parameters in Histomonas meleagridis-infected turkeys determined by real-time quantitative PCR. Avian Pathol. (2015) 44:358–65. doi: 10.1080/03079457.2015.1058483
90. Jones RE, Rives DV, Fletcher OJ, Martin MP. Histomoniasis outbreaks in commercial turkeys in the southeastern United States: proximity of broiler breeder farms as a potential risk factor in disease development. J Appl Poult Res. (2020) 29:496–501. doi: 10.1016/j.japr.2019.12.006
91. Liebhart D, Windisch M, Hess M. Oral vaccination of 1-day-old turkeys with in vitro attenuated Histomonas meleagridis protects against histomonosis and has no negative effect on performance. Avian Pathol. (2010) 39:399–403. doi: 10.1080/03079457.2010.506906
92. Liebhart D, Hess M. Oral infection of turkeys with in vitro-cultured Histomonas meleagridis results in high mortality. Avian Pathol. (2009) 38:223–7. doi: 10.1080/03079450902912192
93. Reid WM. Etiology and dissemination of the blackhead disease syndrome in turkeys and chickens. Exp Parasitol. (1967) 21:249–75. doi: 10.1016/0014-4894(67)90087-2
94. Lotfi A, Abdelhab EM, Hafez HM. Persistence of Histomonas meleagridis in or on materials used in poultry houses. Avian Dis. (2012) 56:224–6. doi: 10.1637/9519-090910-ResNote.1
95. Daugschies A, Bangoura B, Lendner M. Inactivation of exogenous endoparasite stages by chemical disinfectants: current state and perspectives. Parasitol Res. (2013) 112:917–32. doi: 10.1007/s00436-013-3324-4
96. Badparva E, Kheirandish F. Epidemiology of pathogenic parasite Histomonas meleagridis in poultry in Lorestan province, western Iran. J Parasit Dis. (2017) 41:1040–3. doi: 10.1007/s12639-017-0931-5
97. Munsch M, Lotfi A, Hafez HM, Al-Quraishy S, Mehlhorn H. Light and transmission electron microscopic studies on trophozoites and cyst-like stages of Histomonas meleagridis from cultures. Parasitol Res. (2009) 104:683–9. doi: 10.1007/s00436-008-1246-3
98. Munsch M, Mehlhorn H, Al-Quraishy S, Lotfi AR, Hafez HM. Molecular biological features of strains of Histomonas meleagridis. Parasitol Res. (2009) 104:1137–40. doi: 10.1007/s00436-008-1299-3
99. Zaragatzki E, Hess M, Grabensteiner E, Abdel-Ghaffar F, Al-Rasheid KAS, Mehlhorn H. Light transmission electron microscopic studies on the encystation of Histomonas meleagridis. Parasitol Res. (2010) 106:977–83. doi: 10.1007/s00436-010-1777-2
100. Zaragatzki E, Mehlhorn H, Abdel-Ghaffar F, Rasheid KS, Grabensteiner E, Hess M. Experiments to produce cysts in cultures of Histomonas meleagridis – The agent of histomonosis in poultry. Parasitol Res. (2010) 106:1005–7. doi: 10.1007/s00436-010-1776-3
101. Beer LC, Graham BDM, Barros TL, Latorre JD, Tellez-Isaias G, Fuller AL, et al. Evaluation of live-attenuated Histomonas meleagridis isolates as vaccine candidates against wild-type challenge. Poult Sci. (2022) 101:101656. doi: 10.1016/j.psj.2021.101656
102. Tyzzer EE. Studies on histomoniasis, or “blackhead” infection, in the chicken and the turkey. Daedalus. (1934) 69:189–264. doi: 10.2307/20023041
103. Tyzzer EE, Fabyan M. A further inquiry into the source of the virus in blackhead of turkeys, together with observations on the administration of ipecac and of sulfur. J Exp Med. (1922) 35:791–812. doi: 10.1084/jem.35.6.791
105. McDougald LR. Intestinal protozoa important to poultry. Poult Sci. (1998) 77:1156–8. doi: 10.1093/ps/77.8.1156
106. Lee DL. The structure and development of Histomonas meleagridis (Mastigamoebidae: Protozoa) in the female reproductive tract of its intermediate host, Heterakis gallinarum (Nematoda). Parasitology. (1969) 59:877–84. doi: 10.1017/S0031182000070384
107. Waters CV III, Hall LD, Davidson WR, Rollor III EA, Lee KA. Status of commercial and noncommercial chickens as potential sources of histomoniasis among wild turkeys. Wildl Soc Bull. (1994) 22:43–9.
108. Daş G, Gauly M. Density related effects on lifetime fecundity of Heterakis gallinarum in chickens. Parasit Vect. (2014) 7:1–9. doi: 10.1186/1756-3305-7-334
109. Huber K, Gouilloud L, Zenner L. A preliminary study of natural and experimental infection of the lesser mealworm Alphitobius diaperinus (Coleoptera: Tenebrionidae) with Histomonas meleagridis (Protozoa: Sarcomastigophora). Avian Pathol. (2007) 36:279–82. doi: 10.1080/03079450701447317
110. Beckmann JF, Dormitorio T, Oladipupo SO, Terra MTB, Lawrence K, Macklin KS, et al. Heterakis gallinarum and Histomonas meleagridis DNA persists in chicken houses years after depopulation. Vet Parasitol. (2021) 298:109536. doi: 10.1016/j.vetpar.2021.109536
111. Lund EE, Wehr EE, Ellis DJ. Earthworm transmission of heterakis and histomonas to turkeys and chickens. J Parasitol. (1966) 52:899–902. doi: 10.2307/3276528
112. Windisch M, Hess M. Establishing an indirect sandwich enzyme-linked-immunosorbent-assay (ELISA) for the detection of antibodies against Histomonas meleagridis from experimentally infected specific pathogen-free chickens and turkeys. Vet Parasitol. (2009) 161:25–30. doi: 10.1016/j.vetpar.2008.12.014
113. van der Heijden HMJF, Stegeman A, Landman WJM. Development of a blocking-ELISA for the detection of antibodies against Histomonas meleagridis in chickens and turkeys. Vet Parasitol. (2010) 171:216–22. doi: 10.1016/j.vetpar.2010.03.028
114. Grafl B, Liebhart D, Windisch M, Ibesich C, Hess M. Seroprevalence of Histomonas meleagridis in pullets and laying hens determined by ELISA. Vet Rec. (2011) 168:160. doi: 10.1136/vr.c6479
115. Sulejmanovic T, Turblin V, Bilic I, Jaskulska B, Hess M. Detection of Histomonas meleagridis DNA in dust samples obtained from apparently healthy meat turkey flocks without effect on performance. Avian Pathol. (2019) 48:329–33. doi: 10.1080/03079457.2019.1599819
116. Hafez HM, Hauck R, Lüschow D, McDougald LR. Comparison of the specificity and sensitivity of PCR. nested PCR, and real-time PCR for the diagnosis of histomoniasis. Avian Dis. (2005) 49:366–70. doi: 10.1637/7341-020805R.1
117. Huber K, Chauve C, Zenner L. Detection of Histomonas meleagridis in turkeys cecal droppings by PCR amplification of the small subunit ribosomal DNA sequence. Vet Parasitol. (2005) 131:311–6. doi: 10.1016/j.vetpar.2005.05.012
118. Grabensteiner E, Hess M. PCR for identification and differentiation of Histomonas meleagridis, Tetratrichomonas gallinarum and Blastocystis spp. Vet Parasitol. (2006) 142:223–30. doi: 10.1016/j.vetpar.2006.07.011
119. Reis JL Jr, Beckstead RB, Brown CC, Gerhold RW. Histomonas meleagridis and capillarid infection in a captive chukar (Alectoris chukar). Avian Dis. (2009) 53:637–9. doi: 10.1637/8678-030409-Case.1
120. Liu W, Peng J, Li F, Sun H, Ding Y, He J, et al. Identification of Histomonas meleagridis by in vitro microculture and polymerase chain reaction. Rep Parasitol. (2011) 1:1–6. doi: 10.2147/RIP.S18259
121. Gerbod D, Edgcomb VP, Noel C, Zenner L, Wintjens R, Delgado-Viscogliosi P, et al. Phylogenetic position of the trichomonad parasite of turkeys Histomonas meleagridis (Smith) Tyzzer, inferred from small subunit rRNA sequence. J Eukaryot Microbiol. (2001) 48:498–504. doi: 10.1111/j.1550-7408.2001.tb00185.x
122. Hauck R, Hafez HM. Partial sequence of the beta-tubulin of Histomonas meleagridis and the activity of benzimidazoles against H. meleagridis in vitro. Parasitol Res. (2009) 104:1183–9. doi: 10.1007/s00436-008-1309-5
123. Lynn EC, Beckstead RB. Identification of gene expression elements in Histomonas meleagridis using splinkerette PCR a variation of ligated adaptor PCR. J Parasitol. (2012) 98:135–41. doi: 10.1645/GE-2916.1
124. Palmieri N, Ramires MJ, Hess M, Bilic I. Complete genomes of the eukaryotic poultry parasite Histomonas meleagridis: Linking sequence analysis with virulence/attenuation. BMC Genomics. (2021) 22:753. doi: 10.1186/s12864-021-08059-2
125. Bilic I, Jaskulska B, Souillard R, Liebhart D, Hess M. Multi-locus typing of Histomonas meleagridis isolates demonstrates the existence of two different genotypes. PLoS ONE. (2014) 9:e92438. doi: 10.1371/journal.pone.0092438
126. Grafl B, Weise H, Bris JL, Liebart D, Bilic I, Hess M. Aberrant clinical appearance and pathomorphology noticed during an outbreak of histomonosis indicates a different pathogenesis of Histomonas meleagridis genotype 2. Avian Dis. (2015) 59:452–8. doi: 10.1637/11093-041715-Case.1
127. Pham ADN, Mast J, De Gussem JK, McDougald LR, Goddeeris BM. Establishing mono-eukaryotic Histomonas meleagridis cultures from in vivo infection contaminated with Tetratrichomonas gallinarum and Blastocystis spp. Parasitology. (2013) 140:1266–74. doi: 10.1017/S0031182013000723
128. Bilic I, Leberl M, Hess M. Identification and molecular characterization of numerous Histomonas meleagridis proteins using a cDNA library. Parasitology. (2009) 136:379–91. doi: 10.1017/S0031182008005477
129. Leberl M, Hess M, Bilic I. Histomonas meleagridis possesses three alpha-actinins immunogenic to its hosts. Mol Biochem Parasitol. (2010) 169:101–7. doi: 10.1016/j.molbiopara.2009.10.007
130. Mazumdar R, Nöbauer K, Hummel K, Hess M, Bilic I. Molecular characterization of Histomonas meleagridis exoproteome with emphasis on protease secretion and parasite-bacteria interaction. PLoS ONE. (2019) 14:e0212429. doi: 10.1371/journal.pone.0212429
131. Wei Z, Abraham M, Chadwick EV, Beckstead RB. Histomonas meleagridis isolates compared by virulence and gene expression. Vet Parasitol. (2020) 286:109233. doi: 10.1016/j.vetpar.2020.109233
132. Mazumdar R, Endler L, Monoyios A, Hess M, Bilic I. Establishment of a de novo reference transcriptome of Histomonas meleagridis reveals basic insights about biological functions and potential pathogenic mechanisms of the parasite. Protist. (2017) 168:663–85. doi: 10.1016/j.protis.2017.09.004
133. Monoyios A, Hummel K, Nöbauer K, Patzl M, Schlosser S, Hess M, et al. An alliance of gel-based and gel-free proteomic techniques displays substantial insight into the proteome of a virulent and an attenuated Histomonas meleagridis strain. Front Cell Infect Microbiol. (2018) 8:1–23. doi: 10.3389/fcimb.2018.00407
134. Monoyios A, Patzl M, Schlosser S, Hess M, Bilic I. Unravelling the differences: Comparative proteomic analysis of a clonal virulent and an attenuated Histomonas meleagridis strain. Int J Parasitol. (2018) 48:145–57. doi: 10.1016/j.ijpara.2017.08.017
135. Tyzzer EE. Arsenical compounds in the treatment of blackhead in turkeys. J Exp Med. (1923) 37:851–73. doi: 10.1084/jem.37.6.851
136. McDougald LR. Efficacy and compatibility of amprolium and carbarsone against coccidiosis and blackhead in turkeys. Poult Sci. (1979) 58:76–80. doi: 10.3382/ps.0580076
137. Lindquist WD. Some effects of paromomycin sulfate on blackhead in turkeys. Am J Vet Res. (1962) 23:1053–6.
138. McGuire WC, Moeller MW, Morehouse NF. The effect of dimetridazole on the growth and prevention of histomoniasis in poultry. Poult Sci. (1964) 43:864–71. doi: 10.3382/ps.0430864
139. Mitrovic M, Hoffer M, Schilknecht EG. Antihistomonal activity of 1,2-disubstituted 5-nitroimidazoles. Antimicrob Agents Chemother. (1968) 8:445–8.
140. Doneley B. Treating liver disease in the avian patient. Semin Avian Exotic Pet Med. (2004) 13:8–15. doi: 10.1053/S1055-937X(03)00053-7
141. Hu J, McDougald LR. The efficacy of some drugs with known antiprotozoal activity against Histomonas meleagridis in chickens. Vet Parasitol. (2004) 121:233–8. doi: 10.1016/j.vetpar.2004.02.022
142. Law GL, Mansfield GP, Muggleton DF, Parnell EW. Dimetridazole: absorption, excretion and metabolism in turkeys. Nature. (1963) 197:1024–5. doi: 10.1038/1971024a0
143. Seeger KC, Lucas WC, Tomhave AE. The use of enheptin-T in the control of enterohepatitis in turkeys. Poult Sci. (1950) 29:610–1. doi: 10.3382/ps.0290610
144. Cuckler AC, Malanga CM. Nithiazide II. Effect on enterohepatitis in turkeys. Proc Soc Exp Biol Med. (1956) 92:485–8. doi: 10.3181/00379727-92-22520
145. Hegngi FN, Doerr J, Cummings TS, Schwartz RD, Saunders G, Zajac A, et al. The effectiveness of benzimidazole derivatives for the treatment and prevention of histomonosis (blackhead) in turkeys. Vet Parasitol. (1999) 81:29–37. doi: 10.1016/S0304-4017(98)00233-7
146. Hauck R, Hafez HM. Experimental infections with the protozoan parasite Histomonas meleagridis: a review. Parasitol Res. (2013) 112:19–34. doi: 10.1007/s00436-012-3190-5
147. Regmi PR, Shaw AL, Hungerford LL, Messenheimer JR, Zhou T, Pillai P, et al. Regulatory considerations for the approval of drugs against histomoniasis (blackhead disease) in turkeys, chickens, and game birds in the United States. Avian Dis. (2016) 60:725–30. doi: 10.1637/11451-061516-Review.1
148. Liebhart D, Hess M. Spotlight on histomonosis (blackhead disease): a re-emerging disease in turkeys and chickens. Avian Pathol. (2020) 49:1–4. doi: 10.1080/03079457.2019.1654087
149. Clark S, Bailey A. Turkey industry annual report – current health and industry issues facing the US turkey industry. In: Proceeding 119th Annual Meeting of the United States Animal Health Association Providence, Rhode Island. (2015). p. 433–42. Available online at: http://www.usaha.org/upload/Proceedings/2015_Proceedings_web2.pdf
150. Grabensteiner E, Liebhart D, Arshad N, Hess M. Antiprotozoal activities determined in vitro and in vivo of certain plant extracts against Histomonas meleagridis, Tetratrichomonas gallinarum, Blastocystis sp. Parasitol Res. (2008) 103:1257–64. doi: 10.1007/s00436-008-1122-1
151. Hafez HM, Shehata AA. Turkey production and health: current challenges. Ger J Vet Res. (2021) 1:3–14. doi: 10.51585/gjvr.2021.0002
152. Thøfner ICN, Liebhart D, Hess M, Schou TW, Hess C, Ivarsen E, et al. Antihistomonal effects of artemisinin and artemisia annua extracts in vitro could not be confirmed by in vivo experiments in turkeys and chickens. Avian Pathol. (2012) 41:487–96. doi: 10.1080/03079457.2012.714459
153. Clark S, Kimminau E. Critical review: future control of blackhead disease (histomoniasis) in poultry. Avian Dis. (2017) 61:281–8. doi: 10.1637/11593-012517-ReviewR
154. Barros TL, Beer LC, Tellez G, Fuller AL, Hargis BM, Vuong CN. Research note: evaluation of dietary administration of sodium chlorate and sodium nitrate for Histomonas meleagridis prophylaxis in turkeys. Poult Sci. (2020) 99:1983–7. doi: 10.1016/j.psj.2019.11.055
155. Beer LC, Latorre JD, Rochell SJ, Sun X, Tellez G, Fuller AL, et al. Research note: evaluation of deoxycholic acid for antihistomonal activity. Poult Sci. (2020) 99:3481–6. doi: 10.1016/j.psj.2020.03.049
156. Beer LC, Vuong CN, Barros TL, Latorre JD, Tellez G, Fuller AL, et al. Research note: evaluation of boric acid as a chemoprophylaxis candidate to prevent histomoniasis. Poult Sci. (2020) 99:1978–82. doi: 10.1016/j.psj.2019.12.003
157. Callait MP, Granier C, Chauve C, Zenner L. In vitro activity of therapeutic drugs against Histomonas meleagridis (2). Poult Sci. (2002) 81:1122–7. doi: 10.1093/ps/81.8.1122
158. van der Heijden HMJF, Landman WJM. In vitro effect of herbal products against Histomonas meleagridis in turkeys. Vet Parasitol. (2008) 154:1–7. doi: 10.1016/j.vetpar.2008.02.033
159. Abraham M, McDougald LR, Beckstead RB. Blackhead disease: reduced sensitivity of Histomonas meleagridis to nitarsone in vitro and in vivo. Avian Dis. (2014) 58:60–3. doi: 10.1637/10635-080913-Reg.1
160. Umar S, Khan MI, Ahmed S, Usman M, Younus M, Sarwar F. In vitro in vivo sensitivity of a flagellated protozoan, Histomonas meleagridis, to metronidazole nitarsone. Pak. J Zool. (2016) 48:209–14. Available online at: https://www.proquest.com/scholarly-journals/vitro-vivo-sensitivity-flagellated-protozoan/docview/2010348719/se-2?accountid=8361
161. Zenner L, Callait MP, Granier C, Chauve C. In vitro effect of essential oils from Cinnamomum aromaticum, Citrus limon and Allium sativum on two intestinal flagellates of poultry, Tetratrichomonas gallinarum and Histomonas meleagridis. Parasite. (2003) 10:153–7. doi: 10.1051/parasite/2003102153
162. Bleyen N, De Gussem K, Pham ADN, Ons E, Gercen NV, Goddeeris BM. Non-curative, but prophylactic effects of paromomycin in Histomonas meleagridis-infected turkeys and its effect on performance in non-infected turkeys. Vet Parasitol. (2009) 165:248–55. doi: 10.1016/j.vetpar.2009.07.007
163. Hafez HM, Hauck R, Gad W, Gussem KD, Lotfi A. Pilot study on the efficacy of paromomycin as a histomonostatic feed additive in turkey poults experimentally infected with Histomonas meleagridis. Arch Anim Nutr. (2010) 64:77–84. doi: 10.1080/17450390903478851
164. Liebhart D, Ganas P, Sulejmanovic T, Hess M. Histomonosis in poultry: previous and current strategies for prevention and therapy. Avian Pathol. (2017) 46:1–18. doi: 10.1080/03079457.2016.1229458
165. Lin GW. Paromomycin sulfate treatment in histomoniasis outbreaks in three commercial turkey flocks in the fraser valley of British Columbia, Canada. Avian Dis. (2021) 65:592–8. doi: 10.1637/aviandiseases-D-21-00076
166. Puvača N, Brkić I, Jahić M, Nikolić SR, Radović G, et al. The effect of using natural or biotic dietary supplements in poultry nutrition on the effectiveness of meat production. Sustainability. (2020) 12:4373. doi: 10.3390/su12114373
167. Duffy CF, Sims MD, Power RF. Preliminary evaluation of dietary Natustat™ versus Histostat® (nitarsone) for control of Histomonas meleagridis in broiler chickens on infected litter. Int J Poult Sci. (2004) 3:753–57. doi: 10.3923/ijps.2004.753.757
168. Duffy CF, Sims MD, Power RF. Evaluation of dietary Natustat™ for control of Histomonas meleagridis in male turkeys on infected litter. Avian Dis. (2005) 49:423–5. doi: 10.1637/7344-022105R2.1
169. Hafez HM, Hauck R. Efficacy of a herbal product against Histomonas meleagridis after experimental infection of turkey poults. Arch Anim Nutr. (2006) 60:436–42. doi: 10.1080/17450390600884500
170. Hauck R, Hafez HM. Effect of coated plant extracts on Histomonas meleagridis and growth of bacteria in vitro. Avian Dis. (2007) 51:880–3. doi: 10.1637/7957-022707-REGR.1
171. Tykałowski B, Smiałek M, Kowalczyk J, Dziewulska D, Stenzel T, Koncicki A. Phytoncides in the prevention and therapy of blackhead disease and their effect on the turkey immune system. J Vet Res. (2021) 65:79–85. doi: 10.2478/jvetres-2021-0010
172. Uzor PE. Alkaloids from plants with antimalarial activity: a review of recent studies. Evid Based Complement Alternat Med. (2020) 2020:1–17. doi: 10.1155/2020/8749083
173. Delaplane JP, Stuart HO. (1935) Blackhead (Entero-Hepatitis) Investigations. State College, RI: Agricultural experiment station.
174. Farmer RK. Infectious enterohepatitis (blackhead) in turkeys: a study of the prophylactic and therapeutic value of certain compounds. J Comp Pathol. (1950) 60:294–310. doi: 10.1016/S0368-1742(50)80027-9
175. Beer LC, Hargis BM, Vuong CN. Research note: evaluation of quinine as a chemoprophylactic candidate against histomoniasis in turkeys. Poult Sci. (2021) 100:101469. doi: 10.1016/j.psj.2021.101469
176. Ueda H, Kaidou S. Aversion to quinine is associated with taste sensation in chicks. J Poult Sci. (2005) 42:254–62. doi: 10.2141/jpsa.42.254
177. Tyzzer EE, Fabyan M, Foot NC. Further observations on “blackhead” in turkeys. J Infect Dis. (1921) 29:268–86. doi: 10.1093/infdis/29.3.268
178. Joyner LP. Immunity to histomoniasis in turkeys following treatment with dimetridazole. J Comp Pathol Ther. (1963) 73:201–7. doi: 10.1016/S0368-1742(63)80023-5
179. Tyzzer EE. A study of immunity produced by infection with attenuated culture-strains of Histomonas meleagridis. J Comp Patho Ther. (1936) 49:285–303. doi: 10.1016/S0368-1742(36)80025-3
180. Clarkson MJ. Immunological responses to Histomonas meleagridis in the turkey and fowl. Immunology. (1963) 6:156–68.
181. Clarkson MJ. Progressive serum protein changes in turkeys infected with Histomonas meleagridis. J Comp Path. (1966) 76:387–97. doi: 10.1016/0021-9975(66)90059-4
182. Cuckler A. Coccidiosis and histomoniasis in avian hosts. In: Jackson GJ, Herman R, Singer I, editors. Immunity to Parasitic Animals. New York, NY: Appleton-Century-Crofts, Educational Division (1970). p. 371–97.
183. Hess M, Liebhart D, Grabensteiner E, Singh A. Cloned Histomonas meleagridis passaged in vitro resulted in reduced pathogenicity and is capable of protecting turkeys from histomonosis. Vaccine. (2008) 26:4187–93. doi: 10.1016/j.vaccine.2008.05.071
184. Bleyen N, Ons E, De Gussem M, Goddeeris BM. Passive immunization against Histomonas meleagridis does not protect turkeys from an experimental infection. Avian Pathol. (2009) 38:71–6. doi: 10.1080/03079450802641255
185. McDougald LR, Hansen MF. Research note: serum protein changes in chickens subsequent to infection with Histomonas meleagridis. Avian Dis. (1969) 13:673–7. doi: 10.2307/1588543
186. Windisch M, Hess M. Experimental infection of chickens with Histomonas meleagridis confirms the presence of antibodies in different parts of the intestine. Parasite Immunol. (2010) 32:29–35. doi: 10.1111/j.1365-3024.2009.01159.x
187. McGuire WC, Cavett JW. Blood studies on histomoniasis in turkeys. Poult Sci. (1952) 31:610–7. doi: 10.3382/ps.0310610
188. Powell FL, Rothwell L, Clarkson MJ, Kaiser P. The turkey, compared to the chicken, fails to mount an effective early immune response to Histomonas meleagridis in the gut. Parasite Immunol. (2009) 31:312–27. doi: 10.1111/j.1365-3024.2009.01113.x
189. Mitra T, Gerner W, Kidane FA, Wernsdorf P, Hess M, Saalmüller A, et al. Vaccination against histomonosis limits pronounced changes of B cells and T-cell subsets in turkeys and chickens. Vaccine. (2017) 35:4184–96. doi: 10.1016/j.vaccine.2017.06.035
190. Lagler J, Mitra T, Schmidt S, Pierron A, Vatzia E, Stadler M, et al. Cytokine production and phenotype of Histomonas meleagridis-specific T cells in the chicken. Vet Res. (2019) 50:107. doi: 10.1186/s13567-019-0726-z
191. Lagler J, Schmidt S, Mitra T, Stadler M, Wernsdorf P, Grafl B, et al. Comparative investigation of IFN-γ-producing T cells in chickens and turkeys following vaccination and infection with the extracellular parasite Histomonas meleagridis. Dev Comp Immunol. (2021) 116:103949. doi: 10.1016/j.dci.2020.103949
192. Schwarz A, Gauly M, Abel H, Daş G, Humburg J, Weiss ATA, et al. Pathobiology of Heterakis gallinarum mono-infection and co-infection with Histomonas meleagridis in layer chickens. Avian Pathol. (2011) 40:277–87. doi: 10.1080/03079457.2011.561280
193. Sharma JM, Rautenschlein S. Host factors for disease resistance. In: Swayne E., Glisson JR, McDougald LR, Nolan LK, Suarez DL, Nair VL, editors. Diseases of Poultry. 13th ed. Ames, IA: Wiley-Blackwell (2013). p. 47–74.
194. Mitra T, Kidane FA, Hess M, Liebhart D. Unravelling the immunity of poultry against the extracellular protozoan parasite Histomonas meleagridis is a cornerstone for vaccine development: a review. Front Immunol. (2018) 9:2518. doi: 10.3389/fimmu.2018.02518
195. Lund EE. Immunizing action of a nonpathogenic strain of histomonas against blackhead in turkeys. J Protozool. (1959) 6:182–5. doi: 10.1111/j.1550-7408.1959.tb03948.x
196. Lund EE, Augustine PC, Ellis DJ. Immunizing action of in vitro-attenuated Histomonas meleagridis in chickens and turkeys. Exp Parasitol. (1966) 18:403–7. doi: 10.1016/0014-4894(66)90041-5
197. Lund EE, Augustine PC, Chute AM. Histomonas meleagridis after one thousand in vitro passages. J Eukaryot Microbiol. (1967) 14:349–51. doi: 10.1111/j.1550-7408.1967.tb02007.x
198. Dwyer DM, Honigberg BM. Effect of in vitro cultivation on the virulence of a pathogenic strain of Histomonas meleagridis. J Protozool. (1969) 16:18–9.
199. Dwyer DM, Honigberg BM. Effect of certain laboratory procedures on the virulence of Histomonas meleagridis for turkeys and chickens. J Parasitol. (1970) 56:694–700. doi: 10.2307/3277714
200. Liebhart D, Zahoor M, Prokofieva I, Hess M. Safety of avirulent histomonads to be used as a vaccine determined in turkeys and chickens. Poult Sci. (2011) 90:996–1003. doi: 10.3382/ps.2010-01255
201. EFSA. Event report: Technical meeting on histomonosis in turkeys. EFSA Supporting Pub. (2013) 10:464. doi: 10.2903/sp.efsa.2013.EN-464
202. McAllister MM. Successful vaccines for naturally occurring protozoal diseases of animals should guide human vaccine research: a review of protozoal vaccines and their designs. Parasitology. (2014) 141:624–40. doi: 10.1017/S0031182013002060
203. Liebhart D, Sulejmanovic T, Grafl B, Tichy A, Hess M. Vaccination against histomonosis prevents a drop in egg production in layers following challenge. Avian Pathol. (2013) 42:79–84. doi: 10.1080/03079457.2012.760841
204. Sulejmanovic T, Liebhart D, Hess M. In vitro attenuated Histomonas meleagridis does not revert to virulence, following serial in vivo passages in turkeys or chickens. Vaccine. (2013) 31:5443–50. doi: 10.1016/j.vaccine.2013.08.098
205. Sulejmanovic T, Bilic I, Hess M, Liebhart D. An in vitro attenuated strain of Histomonas meleagridis provides cross-protective immunity in turkeys against heterologous virulent isolates. Avian Pathol. (2016) 45:46–53. doi: 10.1080/03079457.2015.1117057
Keywords: Histomonas meleagridis, protozoa, parasite, turkey, histomonosis
Citation: Beer LC, Petrone-Garcia VM, Graham BD, Hargis BM, Tellez-Isaias G and Vuong CN (2022) Histomonosis in Poultry: A Comprehensive Review. Front. Vet. Sci. 9:880738. doi: 10.3389/fvets.2022.880738
Received: 21 February 2022; Accepted: 09 March 2022;
Published: 06 May 2022.
Edited by:
Nicola Pugliese, University of Bari Aldo Moro, ItalyReviewed by:
Ruediger Hauck, Auburn University, United StatesMichael Hess, University of Veterinary Medicine Vienna, Austria
Copyright © 2022 Beer, Petrone-Garcia, Graham, Hargis, Tellez-Isaias and Vuong. This is an open-access article distributed under the terms of the Creative Commons Attribution License (CC BY). The use, distribution or reproduction in other forums is permitted, provided the original author(s) and the copyright owner(s) are credited and that the original publication in this journal is cited, in accordance with accepted academic practice. No use, distribution or reproduction is permitted which does not comply with these terms.
*Correspondence: Lesleigh C. Beer, bGNiZWVyQHVhcmsuZWR1