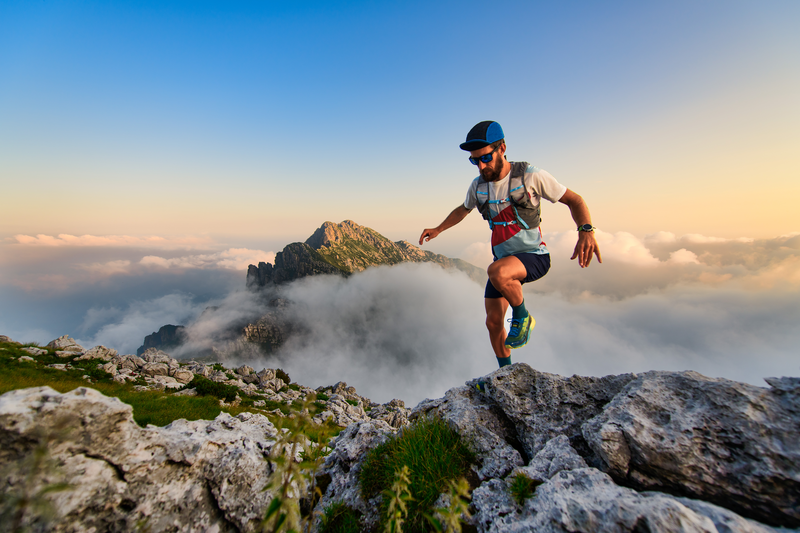
95% of researchers rate our articles as excellent or good
Learn more about the work of our research integrity team to safeguard the quality of each article we publish.
Find out more
ORIGINAL RESEARCH article
Front. Vet. Sci. , 18 April 2022
Sec. Veterinary Infectious Diseases
Volume 9 - 2022 | https://doi.org/10.3389/fvets.2022.877066
This article is part of the Research Topic Antimicrobials Alternatives for the Prevention and Treatment of Veterinary Infectious Diseases View all 16 articles
This study was conducted to investigate the alleviative effects of trans-anethole (TA) on intestinal oxidative stress by enhancing the activities of intestinal antioxidant enzymes and activating the Nrf2 signaling pathway in subclinical necrotic enteritis (NE) infected broilers. A total of 192 1-day-old male Arbor Acres broilers were randomly allocated into three treatment groups: (1) control (CON); (2) subclinical NE challenge (NE); (3) NE challenge + 600 mg/kg TA (NE+TA600). Subclinical NE was induced by oral administration of live coccidiosis vaccine containing 2 × 104 oocysts at 10 days of age and 2 ml of Clostridium perfringens type A solution (3 × 108 CFU/ml) daily from days 14 to 19. The results showed that NE infection led to a severe decline (p < 0.05) in the final body weight (BW) and average daily gain (ADG), but an increase (p < 0.05) in feed/gain (F/G) of broilers at day 10–21 and day 1–21 compared with the control group. TA administration improved (p < 0.05) the growth performance of NE birds. The intestinal villus height (VH) and villus height/crypt depth (VH/CD) were reduced (p < 0.05) by NE challenge as compared with those of the control group, which was elevated by TA administration. Subclinical NE infection decreased (p < 0.05) serum activities of total superoxide dismutase (T-SOD), total antioxidant capacity (T-AOC), and jejunal and ileal glutathione peroxidase (GSH-PX), and T-SOD activity as well as T-AOC in the jejunum, while TA interventions positively elevated that (p < 0.05). Administration of TA protected the intestine against oxidative stress through up-regulation of intestinal nuclear factor erythroid 2-related factor 2 (Nrf2) signaling pathway as compared with the NE group (p < 0.05). In addition, dietary inclusion of TA elevated (p < 0.05) mRNA abundance of c-mesenchymal-epithelial transition factor (c-Met), jejunal epidermal growth factor receptor (EGFR), and transforming growth factor-beta 1 (TGF-β1) in the jejunum and ileum of birds after subclinical NE challenge. In conclusion, 600 mg/kg of TA may be a promising tool to prevent and control subclinical NE by increasing intestinal antioxidant status in broilers.
Necrotic enteritis (NE) is a worldwide high incidence of intestinal disease in poultry induced by Clostridium perfringens type A and C, or Net B (1, 2) and annually results in a global economic loss of 6 billion dollars (3). This infectious disease has been well-controlled for many years by the traditional use of antibiotics. Currently, the use of antibiotics has been banned due to the development of bacterial resistance and the production of antibiotic residues in poultry products (4). Therefore, there has been an increasing concern in exploring new strategies for prevention and treatment of NE other than the use of antibiotics.
Necrotic enteritis infection could result in intestinal lesions, intestinal inflammation, and intestinal oxidative stress (4, 5). Oxidative stress leads to the release of intracellular cytokines and further causes systemic and chronic inflammation (6). Oxidative stress is accompanied by increasing the production of reactive oxygen species (ROS) and is thought to be a trigger for intestinal injury (7, 8). Enhancement of endogenous antioxidant enzymes activities, such as superoxide dismutase (SOD), catalase (CAT), and glutathione peroxidase (GSH-PX), could eliminate the excessive production of ROS, and further ameliorate oxidative stress (9). Accordingly, fortifying the intestinal antioxidant status may be a promising strategy for preventing or treating NE in broilers.
Trans-anethole [TA, 1-methoxy-4-(prop-1-enyl) benzene], a major component (higher than 80%) of the essential oil extracted from the plant of anise and fennel, has been used as a flavoring agent in foods, cosmetics, alcoholic beverage, and perfumes and herbal medicine (10–12). It is a volatile terpenoid with anise flavor, poor water solubility, and easy to be deteriorated when exposed to light and high temperature. It is worth noting that TA is non-toxic with no genotoxic activity recognized by the United States Food and Drug Administration. Several recent studies have confirmed the antioxidant capacity of TA. It was demonstrated that TA sachets improved overall freshness and odor of organic ready-to-eat iceberg lettuce packages due to their antimicrobial and antioxidant activities (13). TA could prevent hydrogen peroxide-induced collagen metabolism alterations and apoptosis in human skin fibroblasts, proving that TA may be beneficial against oxidative stress (10). Moreover, our previous study found that star anise oil enhanced antioxidant status in laying hens (14). However, the mechanism by which the inclusion of TA enhanced antioxidant status of broilers has not been elucidated yet. Nuclear factor E2-related factor 2 (Nrf2), which is a member of the NF-E2 family of the basic leucine zipper of redox-sensitive transcription factors, is well known for scavenging free radicals and preventing oxidative stress (15, 16). It is a classic antioxidant signaling pathway that regulates the expression of phase II antioxidant enzyme genes against oxidative stress. Therefore, the present study aimed to investigate whether TA had alleviative potential on intestinal oxidative stress of subclinical NE challenged broilers through activating the Nrf2 signaling pathway.
This study was reviewed and approved by the Institutional Animal Care and Use Committee of Nanjing Agricultural University (Permit No. SYXK-2017-0027).
Trans-anethole was purchased from Nanjing Dilger Medical Technology Co., Ltd (D105737, Nanjing, China). The analyzed purity of TA was 98.35%. The TA was stored in the dark and at 4°C until use.
Freeze-dried bacteria powder of C. perfringens type A strain (125404) was obtained from BeNa Culture Collection (Xinyang, China). It was cultured anaerobically with fluid thioglycollate (FT) medium (HB5190; Hopebio Biotechnology Co., Ltd, Qingdao, China) in panel for 24 h at 37°C, then aseptically picked single colony into 2 L conical flask with FT medium and anaerobically incubated by shaker for 13 h at 37°C.
A total of 192 1-day-old male Arbor Acres broilers (42.43 ± 0.88 g) were purchased from Yantai Land Animal Husbandry (Shandong, China). On arrival, all birds were weighed and randomly allocated into three groups, with 8 replicates of 8 birds in each group. The basal diets were formulated to meet the bird's nutritional requirements according to NY/T 33-2004 [(17); Supplementary Table 1]. TA was blended with soybean oil and then mixed with other ingredients. All of the diets were pelleted and crumbled. All birds were reared in wire cages and had free access to diet and water throughout the entire experimental period. Room temperature was maintained at 33°C during the first 5 days and then gradually decreased by 0.5°C daily until 22°C. The treatment groups were as follows: (1) CON group (basal diet); (2) NE group (subclinical NE challenge); and (3) NE + TA600 group (subclinical NE challenge + 600 mg/kg of TA). The procedure of subclinical NE challenge was performed with minor modifications by Liu et al. (18) and Zhang et al. (19). Briefly, all chicks in the subclinical NE infected groups were each orally gavaged with live coccidiosis vaccine (Foshan Standard Biotechnology Co., Ltd, Guangdong, China) containing 2 × 104 oocysts suspended in 500 μl of normal saline with coccidia suspension agent at 10 days of age and then with 2 ml of C. perfringens type A solution (3 × 108 CFU/mL) daily from days 14 to 19. Birds in the CON group were orally gavaged with the same amount of normal saline at 10 days of age and sterile FT medium solution during days 14–19. TA was supplemented throughout the whole experimental period.
On the morning of day 22, 8 birds per group with average body weight (BW) of its replicate were selected for sampling. The serum samples were collected from peripheral blood, which was centrifuged at 3,500 × g for 10 min at 4°C, then were stored at −80°C until analysis. After blood sampling, the birds were stunned and sacrificed by cervical dislocation. Approximately 1 cm of middle segments of jejunum and ileum were cut off carefully and fixed in 4% paraformaldehyde solution for histomorphology analysis. Approximately 3 cm of middle jejunum and ileum segments of each bird were dissected and washed with ice-cold sterile saline, then frozen in liquid nitrogen and stored at −80°C for subsequent analysis.
The BW and feed intake of birds of each replicate were recorded weekly to calculate the average daily feed intake (ADFI), average daily gain (ADG), and feed/gain (F/G).
The fixed jejunum and ileum segments were dehydrated, transparentized, and embedded in paraffin. Each sample was sliced into 5–μm cross-sections, deparaffinized in xylene, graded rehydrated, and finally stained with hematoxylin-eosin. With reference to Ekim et al. (20), 10 well-oriented villi and crypts per sample were selected for measuring the villus height (VH) and crypt depth (CD) using light microscope (Olympus CX31, Tokyo, Japan) and Image-Pro Plus 6.0 software (Media Cybernetics, Inc., Rockville, MD, USA).
Frozen jejunum and ileum were weighed, and homogenized (3 min) with ice-cold physiologic saline in the ratio of 1:4 (wt/vol). The homogenates were then centrifuged at 4,000 × g for 10 min at 4°C. The supernatants were then diluted into the optimal content for examining the activities of total SOD (A001-1-1), total antioxidant capacity (T-AOC; A015-1-2), and GSH-PX (A005-1-2), and the concentration of malondialdehyde (MDA; A003-1-2) using the assay kits (Nanjing Jiancheng Bioengineering Institute, Nanjing, China). The total protein concentration of supernatants was detected by bicinchoninic acid (BCA) protein assay kit (P0010; Beyotime Institute of Biotechnology, Nanjing, China). The results were expressed as activities of antioxidant enzyme and concentration of MDA in per mg of protein in the intestinal tissues of broilers. Additionally, the serum activities of T-SOD, T-AOC, GSH-PX, and concentration of MDA were also detected using the same assay kits and presented as that in per ml of serum.
Extraction of total RNA in the jejunum and ileum was performed using Trizol reagent (9108; TaKaRa Biotechnology, Dalian, Liaoning, China). The quality and concentration of total RNA were detected using a NanoDrop-1000 microspectrophotometer (Thermo Fisher Scientific, Waltham, MA, USA), and the integrity of extracted RNA was evaluated with 2.0% agarose gel electrophoresis. Subsequently, the reverse transcription polymerase chain reaction (PCR) was conducted to produce the complementary DNA using the PrimeScriptTMRT reagent Kit (RR036A; TaKaRa Biotechnology Co., Ltd, Dalian, China) by two steps: 37°C for 15 min and 85°C for 5 s. qRT-PCR reactions were conducted to determine the relative mRNA abundance of Nrf2, NAD(P)H quinone dehydrogenase 1 (NQO1), heme oxygenase 1 (HO1), superoxide dismutase 1 (SOD1), glutathione peroxidase (GSH-PX), epidermal growth factor receptor (EGFR), c-mesenchymal epithelial transition factor (c-Met), transforming growth factor-alpha (TGF-α), transforming growth factor-beta 1 (TGF-β1), and beta-actin (β-actin) ChamQ SYBR® qPCR Master Mix Kit (Q311-02; Vazyme Biotechnology, Nanjing, China) based on Applied Biosystems 7500 Real–time PCR System (Life Technologies, CA, USA). The primers were commercially synthesized by Sangon Biotechnology Co., Ltd (Shanghai, China), which are shown in Supplementary Table 2. The amplification program consists of an initial denaturation step at 95°C for 30 s, followed by 40 cycles of 95°C for 10 s and 60°C for 30 s, then 15 s at 95°C, and 60 s at 60°C, with a final step at 95°C for 15 s. The relative mRNA abundance of target genes were analyzed using the 2-ΔΔCt method and normalized against the reference gene (β-actin) expression level.
Total protein extracted from the jejunum and ileum tissues was performed by radioimmunoprecipitation assay lysis buffer and protease inhibitor (P0013B and ST506; Beyotime Institute of Biotechnology, Nanjing, China). Nuclear protein was isolated by Nuclear and Cytoplasmic Protein Extraction Kit (P0027; Beyotime Institute of Biotechnology, Nanjing, China) for detection of Nrf2 protein expression. The concentrations of total cellular protein and nuclear protein were detected by the BCA assay kit (P0010; Beyotime Institute of Biotechnology, Nanjing, China). Equal amounts of protein were separated through sodium dodecylsulfate polyacrylamide gel electrophoresis (SDS-PAGE), transferred onto polyvinylidene difluoride (PVDF) membranes. Subsequently, the membranes were blocked with 5% skimmed milk (w/v) in tris-buffered saline with 0.1% tween (TBST) buffer for 2 h at room temperature, and then incubated with primary antibodies against Nrf2 (16396-1-AP; Proteintech Group, Inc., Wuhan, China), HO1 (10701-1-AP; Proteintech), SOD1 (10269-1-AP; Proteintech), and β-actin (20536-1-AP; Proteintech) overnight at 4°C, and then incubated with secondary goat anti-rabbit IgG horseradish peroxidase-conjugated antibody for 1.5 h at room temperature. The expression of target proteins was determined using ECL chemiluminescence reagents (E412-01; Vazyme Biotechnology) and images were captured by Imager-Bio-Rad (Bio-Rad Laboratories, Inc., Hercules, CA, USA). The band intensities were quantified using Image J software.
All data were presented as mean ± standard error of mean. The Shapiro–Wilk test was used to determine the dataset normality and homogeneity of variances. Data sets were analyzed using one-way analysis of variance (ANOVA), followed by Tukey's HSD test (SAS Institute, 2001). Results were regarded statistically significant with a p < 0.05.
No mortality was observed in the prevention groups during the experimental period. NE infection led to a severe decline (p < 0.05) in the final BW and ADG, but an increase (p < 0.05) in F/G of broilers at day 10–21 and 1–21 compared with control groups (Table 1), but TA administration reversely altered (P < 0.05) those of NE birds. No remarkable difference was found with regards to initial BW, and ADFI at day 0–9 and 1–21 among groups (p > 0.05), but the ADFI at day 10–21 tended to be decreased (p = 0.074) by NE infection. Moreover, the ADG and F/G were not altered (p > 0.05) by TA supplementation before NE infection.
Table 1. Effect of dietary TA supplementation on the growth performance of broilers challenged with subclinical necrotic enteritis1.
Figure 1A revealed that there was some damage to jejunal and ileal villi development after NE infection, as found by broken and shortened villi. Consistent with the histological observations of tissue sections, NE infection significantly reduced the VH and VH/CD in jejunal and ileal tissues as compared with those of control (Figure 1B), which was elevated by TA supplementation (p < 0.05). In addition, the intestinal CD was not affected by NE challenge (p > 0.05).
Figure 1. Effects of TA on intestinal morphology of subclinical NE broilers. (A) Hematoxylin and eosin staining, (B) VH, CD, and VH/CD of the intestine. Values were expressed as mean with standard error represented by vertical bars. a, bMeans with different letters differ significantly among the groups (p < 0.05). VH, villus height; CD, crypt depth.
As revealed by Figure 2, these data indicated that the serum activities of T-SOD, T-AOC, and GSH-PX were decreased (p < 0.05) by subclinical NE infection, but TA interventions elevated that (Figures 2A–D). Although no significant difference was shown with regards to ileal MDA concentration and T-AOC activity among the four groups, the inclusion of TA increased (p < 0.05) the T-SOD activity in the jejunum and ileum as well as T-AOC activity in the jejunum in comparison with subclinical NE group (Figures 2E–G). In addition, the GSH-PX activity in the jejunum (p = 0.068) and ileum (p = 0.062) was tended to be reduced after subclinical NE infection, but elevated by TA administration.
Figure 2. Effects of TA on the activities of antioxidant enzymes in the serum and intestine of subclinical NE broilers. (A–D) The activities of T-SOD, MDA, T-AOC, and GSH-PX of serum; (E–H) The activities of T-SOD, MDA, T-AOC, and GSH-PX in the jejunum and ileum. Values are means with their standard errors represented by vertical bars. a, bMeans within a row with different letters differ significantly (p < 0.05). T-SOD, total superoxide dismutase; MDA, malondialdehyde; T-AOC, total antioxidant capacity; GSH-PX, glutathione peroxidase.
As exhibited in Figure 3, the results showed that subclinical NE challenge downregulated the jejunal and ileal mRNA expression of Nrf2, HO1, NQO1, SOD1, and GSH-PX as compared with control group, while the inclusion of TA upregulated (p < 0.05) the mRNA abundance of these genes.
Figure 3. Effects of TA on the mRNA abundance of Nrf2 pathway and antioxidant enzymes in the jejunum and ileum of subclinical NE broilers. Values are means with their standard errors represented by vertical bars. a, bMeans within a row with different letters differ significantly (p < 0.05). Nrf2, nuclear factor erythroid 2-related factor 2; NQO1, NAD(P)H quinone dehydrogenase 1; HO1, heme oxygenase 1; SOD1, superoxide dismutase 1; GSH-PX, glutathione peroxidase.
Western blot results revealed that the jejunal and ileal nuclear translocation level of Nrf2, and protein abundance of HO1, and SOD1 of subclinical NE infected birds fed with TA was higher (p < 0.05) than those in the NE group (Figure 4).
Figure 4. Effects of TA on the relative protein abundance of Nrf2 and SOD1 in the jejunum and ileum of subclinical NE broilers. (A–D) The protein abundance of Nrf2, HO1, and SOD1 in the jejunum; (E–H) The protein abundance of Nrf2, HO1, and SOD1 in the ileum. Values are means with their standard errors represented by vertical bars. a−cMeans within a row with different letters differ significantly (p < 0.05). Nrf2, nuclear factor erythroid 2-related factor 2; HO1, hemeoxygenase 1; SOD1, superoxide dismutase 1.
Figure 5 presents the results of jejunal and ileal mucosal repair factors gene expression. Dietary inclusion of TA elevated downregulated (p < 0.05) mRNA abundance of jejunal and ileal c-Met, and jejunal EGFR and TGF-β1 in subclinical NE infected birds. The ileal EGFR and TGF-β1, and jejunal and ileal TGF-α did not differ (p > 0.05) among the groups.
Figure 5. Effects of TA on the mRNA abundance of intestinal mucosal repair factor of subclinical NE broilers. Values are means with their standard errors represented by vertical bars. a−cMeans within a row with different letters differ significantly (p < 0.05). EGFR, epidermal growth factor receptor; c-Met, c-mesenchymal epithelial transition factor; TGF-α, transforming growth factor-alpha; TGF-β1, transforming growth factor-beta 1.
Necrotic enteritis caused by C. perfringens destroys the intestinal barrier integrity and leads to intestinal dysfunction in chickens, resulting in a decrease in growth performance (21). NE annually costs up to six billion dollars in the poultry industry (3). Previous studies reported that subclinical NE infection could cause more serious economic loss than clinical NE infection due to mild intestinal damage, poor digestion, and further poor growth performance (22, 23). The results of our study revealed that NE infection led to a severe decline in the final BW and ADG, but an increase in F/G of broilers compared with controls, while TA administration at 600 mg/kg reversely improved the growth performance of NE birds. Accordingly, the VH and VH/CD were improved by TA during the NE challenge. This may be attributed to the previously reported beneficial effects of TA in increasing nutrient digestibility (24), gastroprotector activity (25), antimicrobial (13, 26), and anti-inflammatory (27–29) activities. However, the results of this study revealed that ADG and F/G were not altered by TA supplementation before NE infection. Similar with that, a recent study investigated the effects of different concentrations of TA on the growth performance of broilers and observed that TA inclusion had no distinct effect on ADG, BW, and F/G (17). It was reported that the growth performance of broilers was not affected by the inclusion of essential oils consisting of menthol and anethole (30). Additionally, the experimental conditions, hygiene, animal age, diet type, and altered microbiota may also affect the performance response of broilers to TA (31, 32). Taken together, the effects of TA on growth performance of broilers under normal feeding conditions and subclinical NE challenge require to be further characterized.
Necrotic enteritis infection is usually accompanied by intestinal lesions, intestinal inflammation, and intestinal oxidative stress (4, 5). Rochat et al. (33) also indicated that bacterial infection could cause gastrointestinal inflammation and further lead to oxidative stress. Therefore, we conducted the current study to investigate whether TA could ameliorate subclinical NE in broilers by enhancing the intestinal antioxidant status and intestinal mucous repair factor expression.
The anti-inflammatory activity of Foeniculum vulgare essential oil (81.08% TA) against acetic acid-induced colitis in rats has been reported (27). TA, one of the main active constituents present in essential oils of plants, such as Syzygium anisatum, F. vulgare, Coriandrum sativum, and star anise, has been shown the anti-inflammatory activity by regulation of Th17/Treg function in the mouse model of lipopolysaccharide-induced acute lung injury (28). In another study, TA showed a hepatoprotective effect against hepatic ischemia/reperfusion injury via inhibition of toll-like receptor activation (34). Additionally, treatment with TA exerted a protective effect on the hepatotoxicity induced by acetaminophen via down-regulating the pro-inflammatory mediators (35). These data indicated that TA may ameliorate intestinal injury due to its anti-inflammatory activity. The present study shows that TA inclusion reduces intestinal damage induced by C. perfringens in the experimental model of subclinical NE through the increase of serum and intestinal antioxidant enzymes activities. Previously, TA was reported to play an crucial role in the maintenance of the redox balance through either decreasing ROS levels (36) or enhancing the activities of cellular antioxidant enzymes, such as SOD and GSH-PX (37). Oxidative stress leads to the release of intracellular cytokines and further causes systemic and chronic inflammation (6, 38). Changes in antioxidant enzyme activities can result in oxidative stress. It is well-known that the levels of antioxidant defense can be reflected by the determination of antioxidant activities of T-SOD, T-AOC GSH-PX, and MDA concentration. SOD and GSH-PX are vital intracellular antioxidant enzymes responsible for the antioxidant defense system via converting oxygen radicals to hydrogen peroxide (39). Overall antioxidant defense capacity can be determined by T-AOC. In this study, TA inclusion enhanced serum and intestinal SOD activities as well as the jejunal mRNA and protein levels of SOD1 in subclinical NE-infected birds. Furthermore, TA interventions reversely elevated the serum and jejunal activities of T-AOC and GSH-PX, and mRNA abundance of GSH-PX in the jejunum and ileum of birds infected with subclinical NE. These results indicated that the increased expression and activities of antioxidant enzymes may be the mechanism of action by which TA alleviates the intestinal damage caused by subclinical NE. Similarly, Chaudhari et al. (40) observed that TA exhibited the in vitro free radicals scavenging activity and the inhibiting capacity of lipid oxidation in stored maize samples, confirming the antioxidant activity of TA in preserving maize samples. TA reduced oxidative stress of in vitro primordial follicles and bovine embryos development by decreasing the production of ROS and regulating the redox balance (8, 41). TA also prevented hydrogen peroxide-induced collagen metabolism alterations and apoptosis in human skin fibroblasts, proving that TA may be an effective therapeutic agent for oxidative stress-related skin diseases (10). Besides that, the antioxidant potential of TA has been widely reported (25, 42, 43). The antioxidant activity of TA may be attributed to the conjugated double bonds and phenol group in its chemical structure, which has high reactivity with peroxyl radicals (10, 25, 44, 45). On the other hand, TA increased jejunal and ileal mRNA and protein expression of Nrf2, and downstream target molecules. Nrf2 is a principal transcription factor exerting an antioxidant role and maintaining cellular redox balance (46). Upon activation, Nrf2 moves into cell nucleus after releasing from Keap 1, where it is combined to the antioxidant response element to activate transcription of antioxidant genes. Similarly, it has been demonstrated that star anise oil could reduce the oxidative stress of birds during subclinical Escherichia coli challenges through upregulation of the Nrf2 signaling pathway (47). In addition, we found that NE infection resulted in vacuolization and swelling in the mitochondria, while TA administration improved that. Many lines of evidence indicate that mitochondria play a key role in preventing oxidative damage (48). Therefore, further research on the protective effects of TA on the subclinical NE-induced mitochondria dysfunction requires to be characterized.
Previous studies have widely reported that growth factors, including EGFR, TGF-β, and TGF-α, had positive impacts on epidermal repair and regeneration, inflammation, and proliferation (49–51). Alterations in the endogenous growth factors status are also correlated with poor clinical prognosis (52). In addition, the hepatocyte growth factor (HGF)/c-MeT signaling system may contribute to cell mobilization, tissue regeneration, and repair (53). Our results showed that dietary inclusion of TA elevated down-regulated mRNA abundance of jejunal and ileal c-Met, and jejunal EGFR and TGF-β1 in subclinical NE-infected birds. These data indicated that TA may have substantial repair effects on the damaged intestinal mucosa induced by subclinical NE infection. The specific mechanism requires to be further characterized.
This study indicated that TA has promising potential treatment in subclinical NE in broilers. Prior to that, TA has been proven effective in animal and cell experimental models of a variety of diseases. Therefore, it may be worthwhile to further explore the pharmacological effects of TA in intestinal diseases of humans and animals.
The inclusion of 600 mg/kg of TA may be a promising tool to prevent and control subclinical NE by increasing intestinal antioxidant status and intestinal mucosal repair factor expression in broilers. The mechanisms by which TA exerts its antioxidant activity may be attributed to the activation of Nrf2 signaling pathway. Taken together, TA may be an effective agent to prevent and treat NE in poultry industry.
The original contributions presented in the study are included in the article/Supplementary Material, further inquiries can be directed to the corresponding author/s.
CY contributed to conceptualization, methodology, and writing—original draft. YT and QL contributed to investigation and supervision. TW contributed to supervision, project administration, and funding acquisition. ZY contributed to visualization, funding acquisition, writing, reviewing, and editing the manuscript. All authors contributed to the article and approved the submitted version.
This work was supported by the National Key Research and Development Program of China (Grant No. 2018YFD0501101).
The authors declare that the research was conducted in the absence of any commercial or financial relationships that could be construed as a potential conflict of interest.
All claims expressed in this article are solely those of the authors and do not necessarily represent those of their affiliated organizations, or those of the publisher, the editors and the reviewers. Any product that may be evaluated in this article, or claim that may be made by its manufacturer, is not guaranteed or endorsed by the publisher.
The Supplementary Material for this article can be found online at: https://www.frontiersin.org/articles/10.3389/fvets.2022.877066/full#supplementary-material
1. Engström BE, Fermér C, Lindberg A, Saarinen E, Båverud V, Gunnarsson A. Molecular typing of isolates of Clostridium perfringens from healthy and diseased poultry. Vet Microbiol. (2003) 94:225–35. doi: 10.1016/S0378-1135(03)00106-8
2. Prescott JF, Smyth JA, Shojadoost B, Vince A. Experimental reproduction of necrotic enteritis in chickens: a review. Avian Pathol. (2016) 45:317–22. doi: 10.1080/03079457.2016.1141345
4. Cao L, Wu XH, Bai YL, Wu XY, Gu SB. Anti-inflammatory and antioxidant activities of probiotic powder containing Lactobacillus plantarum 1.2567 in necrotic enteritis model of broiler chickens. Livest Sci. (2019) 223:157–63. doi: 10.1016/j.livsci.2019.03.009
5. Park SS, Lillehoj HS, Allen PC, Park DW, FitzCoy S, Bautista DA, et al. Immunopathology and cytokine responses in broiler chickens coinfected with Eimeria maxima and Clostridium perfringens with the use of an animal model of necrotic enteritis. Avian Dis. (2008) 52:14–22. doi: 10.1637/7997-041707-Reg
6. Mitra S, Natarajan R, Ziedonis D, Fan XD. Antioxidant and anti-inflammatory nutrient status, supplementation, and mechanisms in patients with schizophrenia. Prog Neuro Psychopharmacol Biol Psychiatry. (2017) 78:1–11. doi: 10.1016/j.pnpbp.2017.05.005
7. Su WP, Zhang H, Ying ZX, Li Y, Zhou L, Wang F, et al. Effects of dietary L-methionine supplementation on intestinal integrity and oxidative status in intrauterine growth-retarded weanling piglets. Eur J Nutr. (2018) 57:2735–45. doi: 10.1007/s00394-017-1539-3
8. Sá NAR, Vieira LA, Ferreira ACA, Cadenas J, Bruno JB, Maside C, et al. Anethole supplementation during oocyte maturation improves in vitro production of bovine embryos. Reprod Sci. (2020) 27:1602–8. doi: 10.1007/s43032-020-00190-x
9. He L, He T, Farrar S, Ji LB, Liu TY, Ma X. Antioxidants maintain cellular redox homeostasis by elimination of reactive oxygen species. Cell Physiol Biochem. (2017) 44:532–53. doi: 10.1159/000485089
10. Galicka A, Kretowski R, Nazaruk J, Cechowska-Pasko M. Anethole prevents hydrogen peroxide-induced apoptosis and collagen metabolism alterations in human skin fibroblasts. Mol Cell Biochem. (2014) 394:217–24. doi: 10.1007/s11010-014-2097-0
11. Sheikh B, Pari L, Ayyasamy R, Ramasamy C. Trans-anethole, a terpenoid ameliorates hyperglycemia by regulating key enzymes of carbohydrate metabolism in streptozotocin induced diabetic rats. Biochimie. (2015) 112:57–65. doi: 10.1016/j.biochi.2015.02.008
12. Aprotosoaie AC, Costache II, Miron A. Anethole and its role in chronic diseases. Adv Exp Med Biol. (2016) 929:247–67. doi: 10.1007/978-3-319-41342-6_11
13. Wieczyńska J, Cavoski I. Antimicrobial, antioxidant and sensory features of eugenol, carvacrol and trans-anethole in active packaging for organic ready-to-eat iceberg lettuce. Food Chem. (2018) 259:251–60. doi: 10.1016/j.foodchem.2018.03.137
14. Yu CY, Wei JD, Yang CW, Yang ZB, Yang WR, Jiang SZ. Effects of star anise (Illicium verum Hook.f.) essential oil on laying performance and antioxidant status of laying hens. Poult Sci. (2018) 97:3957–66. doi: 10.3382/ps/pey263
15. Lee MS, Lee B, Park KE, Utsuki T, Shin T, Oh CW, et al. Dieckol enhances the expression of antioxidant and detoxifying enzymes by the activation of Nrf2-MAPK signaling pathway in HepG2 cells. Food Chem. (2015) 174:538–46. doi: 10.1016/j.foodchem.2014.11.090
16. Zhang H, Wang J, Liu Y, Sun B. Wheat bran feruloyl oligosaccharides modulate the phase II detoxifying/antioxidant enzymes via Nrf2 signaling. Int J Biol Macromol. (2015) 74:150–4. doi: 10.1016/j.ijbiomac.2014.12.011
17. Yu CY, Zhang JF, Zhang H, Chen YN, Wang C, Zhang LL, et al. Influence of trans-anethole on the nutrient digestibility and intestinal barrier function in broilers. Poult Sci. (2021) 100:101489. doi: 10.1016/j.psj.2021.101489
18. Liu D, Guo YM, Wang Z, Yuan JM. Exogenous lysozyme influences Clostridium perfringens colonization and intestinal barrier function in broiler chickens. Avian Pathol. (2010) 39:17–24. doi: 10.1080/03079450903447404
19. Zhang BB, Lv ZP, Li HX, Guo SS, Liu D, Guo YM. Dietary larginine inhibits intestinal Clostridium perfringens colonization and attenuates intestinal mucosal injury in broiler chickens. Br J Nutr. (2017) 118:321–32. doi: 10.1017/S0007114517002094
20. Ekim B, Calik A, Ceylan A, Saçakli P. Effects of Paenibacillus xylanexedens on growth performance, intestinal histomorphology, intestinal microflora, and immune response in broiler chickens challenged with Escherichia coli K88. Poult Sci. (2020) 99:214–23. doi: 10.3382/ps/pez460
21. Abudabos AM, Alyemni AH, Dafalla YM, Khan RU. The effect of phytogenics on growth traits, blood biochemical and intestinal histology in broiler chickens exposed to Clostridium perfringens challenge. J Appl Anim Res. (2018) 46:691–5. doi: 10.1080/09712119.2017.1383258
22. Kan LG, Guo FS, Liu Y, Pham VH, Guo YM, Wang Z. Probiotics Bacillus licheniformis improves intestinal health of subclinical necrotic enteritis-challenged broilers. Front Microbiol. (2021) 12:623739. doi: 10.3389/fmicb.2021.623739
23. Emami NK, Dalloul RA. Centennial review: recent developments in host-pathogen interactions during necrotic enteritis in poultry. Poult Sci. (2021) 100:101330. doi: 10.1016/j.psj.2021.101330
24. Jamroz D, Kamel C. Plant extracts enhance broiler performance. In non-ruminant nutrition: antimicrobial agents and plant extracts on immunity, health and performance. J Anim Sci. (2002) 80:41–6.
25. Freire RS, Morais SM, Catunda-Junior FEA, Pinheiro DCSN. Synthesis and antioxidant, anti-inflammatory and gastroprotector activities of anethole and related compounds. Bioorgan Med Chem. (2005) 13:4353–8. doi: 10.1016/j.bmc.2005.03.058
26. Kwiatkowski P, Grygorcewicz B, Pruss A, Wojciuk B, Dołegowska B, Giedrys-Kalemba S, et al. The effect of subinhibitory concentrations of trans-anethole on antibacterial and antibiofilm activity of mupirocin against mupirocin-resistant Staphylococcus aureus strains. Microb Drug Resist. (2019) 25:1424–9. doi: 10.1089/mdr.2019.0101
27. Rezayat SM, Dehpour A-R., Motamed SM, Yazdanparast M, Chamanara M, et al. Foeniculum vulgare essential oil ameliorates acetic acid-induced colitis in rats through the inhibition of NF-κB pathway. Inflammopharmacol. (2018) 26:851–9. doi: 10.1007/s10787-017-0409-1
28. Zhang S, Chen X, Devshilt I, Yun Q, Huang C, An LJ, et al. Fennel main constituent, trans-anethole treatment against LPS-induced acute lung injury by regulation of Th17/Treg function. Mol Med Rep. (2018) 18:1369–76. doi: 10.3892/mmr.2018.9149
29. Yi QY, Liu JX, Zhang YF, Qiao HZ, Chen F, Zhang SH, et al. Anethole attenuates enterotoxigenic escherichia coli-induced intestinal barrier disruption and intestinal inflammation via modification of TLR signaling and intestinal microbiota. Front Microbiol. (2021) 12:647242. doi: 10.3389/fmicb.2021.647242
30. Hafeez A, Männer K, Schieder C, Zentek J. Effect of supplementation of phytogenic feed additives (powdered vs. encapsulated) on performance and nutrient digestibility in broiler chickens. Poult Sci. (2016) 95:622–9. doi: 10.3382/ps/pev368
31. Goel G, Makkar HP, Becker K. Changes in microbial community structure, methanogenesis and rumen fermentation in response to saponin-rich fractions from different plant materials. J Appl Microbiol. (2008) 105:770–7. doi: 10.1111/j.1365-2672.2008.03818.x
32. Attia Y, Al-Harthi M, El-Kelawy M. Utilisation of essential oils as a natural growth promoter for broiler chickens. Italian J Anim Sci. (2019) 18:1005–12. doi: 10.1080/1828051X.2019.1607574
33. Rochat T, Bermúdez-Humarán L, Gratadoux JJ, Fourage C, Hoebler C, Corthier G, et al. Anti-inflammatory effects of Lactobacillus casei BL23 producing or not a manganese-dependant catalase on DSS-induced colitis in mice. Microb. Cell. Fact. (2007) 6, 22. doi: 10.1186/1475-2859-6-22
34. Cho HI, Kim K-M., Kwak JH, Lee SK, Lee, et al. Protective mechanism of anethole on hepatic ischemia/reperfusion injury in mice. J Nat Prod. (2013) 76:1717–23. doi: 10.1021/np4004323
35. da Rocha BA, Ritter AMV, Ames FQ, Gonçalves OH, Leimann FV, Bracht L, et al. Acetaminophen-induced hepatotoxicity: preventive effect of trans-anethole. Biomed Pharmacother. (2017) 86:213–20. doi: 10.1016/j.biopha.2016.12.014
36. Rhee Y, Moon JH, Mo J, Pham T, Chung P. mTOR and ROS regulation by anethole on adipogenic differentiation in human mesenchymal stem cells. BMC Cell Biol. (2018) 19:12. doi: 10.1186/s12860-018-0163-2
37. Dongare V, Kulkarni C, Kondawar M, Magdum C, Haldavnekar V, Arvindekar A. Inhibition of aldose reductase and anti-cataract action of trans-anethole isolated from Foeniculum vulgare mill fruits. Food Chem. (2012) 132:385–90. doi: 10.1016/j.foodchem.2011.11.005
38. Yu ZW, Bao ZJ, Ruan QW, Ma YX. Oxi-inflamm-aging and its association with the polymorphism of ApoE genes. Acta Physiol Sin. (2013) 65:338–46.
39. Fattman CL, Chang LY, Termin TA, Petersen L, Enghild JJ, Oury TD. Enhanced bleomycin-induced pulmonary damage in mice lacking extracellular superoxide dismutase. Free Radic Biol Med. (2003) 35:763–71. doi: 10.1016/S0891-5849(03)00402-7
40. Chaudhari AK, Singh VK, Das S, Deepika SBK, Dubey NK. Antimicrobial, aflatoxin B1 inhibitory and lipid oxidation suppressing potential of anethole-based chitosan nanoemulsion as novel preservative for protection of stored maize. Food Bioprocess Tech. (2020) 13:1462–77. doi: 10.1007/s11947-020-02479-w
41. Sá NAR, Bruno JB, Guerreiro DD, Cadenas J, Alves BG, Cibin FWS, et al. Anethole reduces oxidative stress and improves in vitro survival and activation of primordial follicles. Braz J Med Biol Res. (2018) 51:e7129. doi: 10.1590/1414-431x20187129
42. Singh G, Kapoor IPS, Singh P, de Heluani CS, Catalan CAN. Chemical composition and antioxidant potential of essential oil and oleoresins from anise seeds (Pimpinella anisum L.). Int J Essent Oil Ther. (2008) 2:122–30.
43. Senatore F, Oliviero F, Scandolera E, Taglialatela-Scafati O, Roscigno G, Zaccardelli M, et al. Chemical composition, antimicrobial and antioxidant activities of anethole-rich oil from leaves of selected varieties of fennel [Foeniculum vulgare Mill. ssp. vulgare var. azoricum (Mill.) Thell]. Fitoterapia. (2013) 90:214–9. doi: 10.1016/j.fitote.2013.07.021
44. Chainy GBN, Manna SK, Chaturvedi MM, Aggarwal BB. Anethole blocks both early and late cellular responses transduced by tumor necrosis factor: effect on NF-κB, AP-1, JNK. MAPK and apoptosis. Oncogene. (2000) 19:2943–50. doi: 10.1038/sj.onc.1203614
45. Amorati R, Foti MC, Valgimigli L. Antioxidant activity of essential oils. J Agri Food Chem. (2013) 61:10835–47. doi: 10.1021/jf403496k
46. Johnson JA, Johnson DA, Kraft AD, Calkins MJ, Jakel RJ, Vargas MR, et al. The Nrf2-ARE pathway: an indicator and modulator of oxidative stress in neurodegeneration. Ann N Y Acad Sci. (2008) 1147:61–9. doi: 10.1196/annals.1427.036
47. Ding X, Yang CW, Yang ZB, Ren XJ, Wang PP. Effects of star anise (Illicium verum Hook.f) oil on the nuclear factor E2-related factor 2 signaling pathway of chickens during subclinical Escherichia coli challenge. Poult Sci. (2020) 6:3092–101. doi: 10.1016/j.psj.2019.10.004
48. Lin MT, Beal MF. Mitochondrial dysfunction and oxidative stress in neurodegenerative diseases. Nature. (2006) 443:787–95. doi: 10.1038/nature05292
49. Zieske JD, Takahashi H, Hutcheon AE, Dalbone AC. Activation of epidermal growth factor receptor during corneal epithelial migration. Invest Ophthalmol Vis Sci. (2000) 41:1346–55. doi: 10.1007/s004170050379
50. Repertinger SK, Campagnaro E, Fuhrman J, El-Abaseri T, Yuspa SH, Hansen LA. EGFR enhances early healing after cutaneous incisional wounding. J Invest Dermatol. (2004) 123:982–9. doi: 10.1111/j.0022-202X.2004.23478.x
51. Lorente AF, Brooks SA, Vinageras EN, Alvarez MCB, Brito BW, Concepcion MT, et al. Effect of blockade of the EGF system on wound healing in patients vaccinated with CIMAvax® EGF. World J Surg Oncol. (2013) 11:275. doi: 10.1186/1477-7819-11-275
52. Arteaga CL. The epidermal growth factor receptor: from mutant oncogene in nonhuman cancers to therapeutic target in human neoplasia. J Clin Oncol. (2001) 19:32S−40S. doi: 10.1002/ijc.1442
Keywords: Trans-anethole, broiler, subclinical necrotic enteritis, intestinal oxidative stress, intestinal mucosal repair factor
Citation: Yu C, Tong Y, Li Q, Wang T and Yang Z (2022) Trans-anethole Ameliorates Intestinal Injury Through Activation of Nrf2 Signaling Pathway in Subclinical Necrotic Enteritis-Induced Broilers. Front. Vet. Sci. 9:877066. doi: 10.3389/fvets.2022.877066
Received: 16 February 2022; Accepted: 21 March 2022;
Published: 18 April 2022.
Edited by:
Amjad Islam Aqib, Cholistan University of Veterinary and Animal Sciences, PakistanReviewed by:
Mughees Aizaz Alvi, Lanzhou Veterinary Research Institute (CAAS), ChinaCopyright © 2022 Yu, Tong, Li, Wang and Yang. This is an open-access article distributed under the terms of the Creative Commons Attribution License (CC BY). The use, distribution or reproduction in other forums is permitted, provided the original author(s) and the copyright owner(s) are credited and that the original publication in this journal is cited, in accordance with accepted academic practice. No use, distribution or reproduction is permitted which does not comply with these terms.
*Correspondence: Tian Wang, dGlhbndhbmduamF1QDE2My5jb20=; Zaibin Yang, eWFuZ3piQHNkYXUuZWR1LmNu
Disclaimer: All claims expressed in this article are solely those of the authors and do not necessarily represent those of their affiliated organizations, or those of the publisher, the editors and the reviewers. Any product that may be evaluated in this article or claim that may be made by its manufacturer is not guaranteed or endorsed by the publisher.
Research integrity at Frontiers
Learn more about the work of our research integrity team to safeguard the quality of each article we publish.