- 1College of Animal Science and Technology, Nanjing Agricultural University, Nanjing, China
- 2State Key Laboratory for Managing Biotic and Chemical Threats to the Quality and Safety of Agro-products, Institute of Animal Science and Veterinary, Zhejiang Academy of Agricultural Science, Hangzhou, China
- 3Huzhou Lvchang Ecoagriculture Co., Ltd., Huzhou, China
- 4Animal Disease Prevention and Control Center, Huzhou Wuxing District Agricultural and Rural Bureau, Huzhou, China
Poultry as a large-scale intensive farming is vulnerable to oxidative stress. Resveratrol and apigenin are recognized to have many beneficial bioactive functions. This study tested the hypothesis that dietary resveratrol and apigenin supplementation alleviates oxidative stress in the small intestine of diquat-challenged pullets. A total of 200 healthy pullets were randomly divided into four treatment groups: control group fed with a basal diet (CON), diquat group fed with a basal diet (DIQ), resveratrol group fed with a basal diet containing 500 mg/kg resveratrol (RES), and an apigenin group fed with a basal diet containing 500 mg/kg apigenin (API) and injected intraperitoneally with either 1 ml of saline (CON) or 8 mg/kg body weight of diquat (DIQ, RES, and API) to induce oxidative stress. The day of the injection was considered as day 0. The results indicated that resveratrol and apigenin were able to decrease the malondialdehyde (MDA) level and upregulate total antioxidant capacity (T-AOC), superoxide dismutase (SOD), and glutathione peroxidase (GSH-PX) levels in serum on day 1 and 10 after being diquat-challenged. In addition, resveratrol increased mRNA expression of NQO1 (NAD(P)H dehydrogenase quinone 1) and HO-1 (heme oxygenase-1) in ileum and jejunum on day 10, while apigenin upregulated nuclear factor erythroid 2-related factor 2 (NRF2), NQO1, and HO-1 in ileum and jejunum on day 10. Both resveratrol and apigenin increased the mRNA expression of CLAUDIN-1 in ileum and jejunum on day 1 and that of ZO-1 (zonula occludens-1) in ileum on day 10 post-diquat-injection. These findings indicate that dietary supplementation with resveratrol and apigenin attenuates oxidative stress involving NRF2 signaling pathways in diquat-challenged pullets to some extent. These observations are valuable for the chicken industry and resveratrol and apigenin applications in animal husbandry.
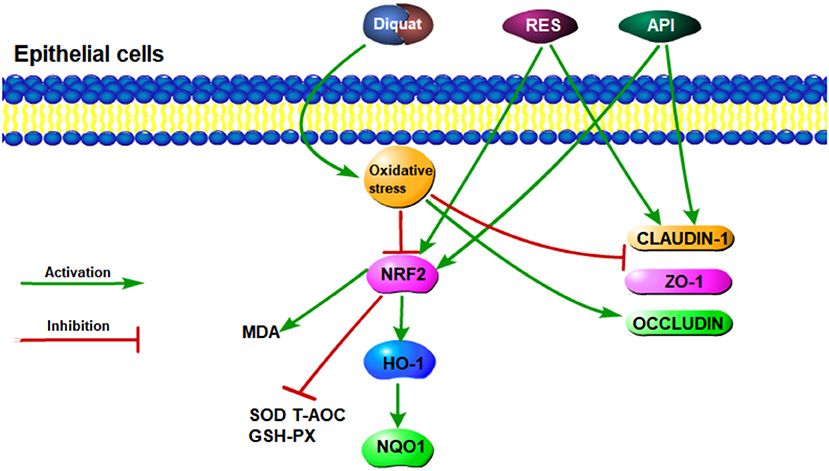
Graphical Abstract. The process of resveratrol and apigenin to alleviate diquat-induced oxidative stress in epithelial cells.
Introduction
Intensive modern farming significantly boosts livestock productivity and economic benefit and increases the risk of animals' exposure to oxidative stress (1). Various factors such as physics/chemistry, nutrition, temperature, and local environment can induce oxidative stress, which represents the imbalance between reactive oxygen species (ROS) production and defense responses of animals (2). When this balance is disrupted, increased ROS will further alter antioxidant defense capacities, including changes in malondialdehyde (MDA), superoxide dismutase (SOD), catalase (CAT), total antioxidant capacity (T-AOC), and glutathione peroxidase (GSH-PX) levels (3–5). Excessive ROS generation could damage cellular macromolecules, DNA, and proteins to impair cellular functions; thus, affecting animal survival and resulting in economic losses in animal farming (6). As a natural barrier between the internal and external environments of pullets, the intestine is susceptible to oxidative stress. Therefore, it is imperative to establish appropriate nutrition strategies to decrease the risk of intestinal oxidative damage in pullets.
Recent studies have shown that oxidative stress can disturb cellular functions by influencing transcription factors and the redox-sensitive signaling pathway, nuclear factor erythroid 2-related factor 2 (NRF2), NAD(P)H dehydrogenase quinone 1 (NQO1), and heme oxygenase-1 (HO-1) regarded as transcription factors exerting critical regulatory effects on the oxidative status via induced expression of the antioxidant and phase-2 detoxifying enzymes (7–9). Normal intestinal functioning depends on the initiation and conservation of a mucosal barrier, and this intestinal mucosal barrier is indispensable for preventing intestinal injury due to certain microorganisms or undesirable substances (10). Several studies have demonstrated that oxidative stress and disruption of cellular redox status impaired intestinal functioning and intestinal turnover (11–13). The formation of tight junctions creates the significant components of the intestinal barrier: OCCLUDIN is the first tight junction protein to be identified, whereas CLAUDINS and ZO-1 (zonula occludens-1) are the main proteins contributing to the physiological and structural paracellular barrier function (14).
Diquat, a bipyridyl herbicide, is able to convert molecular oxygen into superoxide anion radical and stimulate cellular production of free radical species via cyclic reduction-oxidation processes and is often used to induce oxidative stress (15–19). Diquat-challenging oxidative stress has been reported to affect intestinal morphology and disrupt intestinal function (17–20). The maintenance of the intestinal epithelial redox environment is essential for the activities of pivotal physiological processes, such as digestion and absorption, cell proliferation and apoptosis, and immune response (21). Many factors lead to oxidative stress (22, 23) and ultimately affect intestinal health. Resveratrol is a nutraceutical that has garnered much attention because of its antioxidant and anti-apoptosis potential, being a phytoalexin polyphenolic compound found in many kinds of plants, such as grapes and peanuts, among others. Evidence suggest that that dietary resveratrol supplementation enhances the antioxidant status of the animal body and/or animal products (24, 25). Besides this, resveratrol exerts a strong inhibitory effect on the production of ROS in many experimental systems, with anti-inflammatory, anti-senescence, and anti-obesity among its various biological functions (26, 27). For poultry, resveratrol can be used as a feed additive to improve the meat quality of broilers, an outcome that may be associated with an improved muscle antioxidative status and mitochondrial biogenesis (28, 29). Apigenin is a natural phytochemical, a flavonoid, which is present in several dietary plant foods, namely, vegetables and fruits (30). A few studies conducted in recent years have shown that apigenin is a potential antioxidant, anti-apoptosis, and anti-inflammatory agent (30–33). In this context, surprisingly, limited information is available on the effects of resveratrol and apigenin to ameliorate diquat-induced oxidative stress and intestinal barrier dysfunction in pullets. Hence, the objective of this study is to evaluate the influence of resveratrol and apigenin for mitigating oxidative stress-induced impairment of serum antioxidative capacity, intestinal morphology, and mRNA expression levels of the NRF2 pathway and tight junctions-related genes in pullets.
Materials and Methods
Animals, Diets, and Management
This experimental trial was conducted at Huzhou Lvchang Ecoagriculture Co., Ltd., in Zhejiang Province. A total of 200 healthy, 13-week-old Jingfen NO.1, pullets (1.17 kg ± 0.24) were recruited and randomly assigned to four dietary treatments with five replicates (n = 10 pullets per replicate) as follows: (1) control group (CON), pullets fed with a basal diet and injected with sterile saline; (2) diquat-challenged group (DIQ), pullets fed with a basal diet and injected with diquat; (3) resveratrol group (RES) + diquat, pullets fed with a basal diet containing 500 mg/kg resveratrol and injected with diquat; (4) apigenin group (API) + diquat, pullets fed with a basal diet containing 500 mg/kg apigenin and injected with diquat. The diquat was purchased from Shangdong Baishiwei Crop Protection Co., Ltd; the resveratrol came from Chengdu Huagao Biological Products Co. Ltd, and the apigenin was bought from Changsha Shanghe Biological Technology Co. Ltd. The supplemental level of resveratrol was based on a previous study (34). The supplemental level of apigenin is the same as that of resveratrol. All the chickens were fed different diets for 7 days and then injected intraperitoneally with either 1 ml of saline or 8 mg/kg, which was according to the previous report (19), diquat dissolved in 1 ml of saline to induce oxidative stress. Five pullets were caged in a single pen (40 × 50 × 40 cm), with 3-tiered battery cages and diet and freshwater offered ad libitum. The photoperiod regimen was set to 16-h-light: 8-h-dark during the experimental period. The temperature and light conditions were the same across the different treatment groups. The basal diets provided to meet the nutritional requirements of hens are shown in Table 1. According to the Local Experimental Animal Care Committee, all procedures were implemented and approved by Nanjing Agricultural University.
Sample Collection
Day 0 corresponded to when the intraperitoneal injection was administered; then, on day 1 and 10 of the trial, one pullet was randomly selected from each group replicate (40 pullets in total). Blood samples were drawn from the axillary vein into vacuum tubes (5 ml) containing coagulant and then centrifuged at 3,000 × g for 10 min, after which the ensuing serum was stored at −20°C until further analysis. The pullets were slaughtered by intracardial administration of sodium pentobarbital (30 mg/kg of body weight, Sinopharm, China) and jugular exsanguination after overnight feed deprivation according to a previous article (35). Samples of the jejunum and ileum were removed from the middle of the jejunum segment and ileum segment and then rinsed with ice-cold phosphate-buffered saline (PBS, Solarbio, China). One section of the jejunum and ileum samples were immediately frozen in liquid nitrogen, then transferred to storage at −80°C until further analysis. Other sections of intestinal samples (3 cm, taken on day 10) were fixed in 4% para form (Biosharp, Shanghai, China) and stored at room temperature for morphological analysis.
Estimation of Serum Antioxidant Parameters
The activities of the total antioxidant capacity (T-AOC), the superoxide dismutase (SOD), the glutathione peroxidase (GSH-PX) activity, and the malondialdehyde (MDA) in the serum were determined to estimate the oxidative status of the pullets. In brief, the T-AOC level was evaluated by the FRAP method. The SOD activity was detected by the WST method. The activity of GSH-PX was determined by the 5,5'-dithiobis- (2-nitrobenzoic acid) method. The MDA level was measured by the TBA reaction method. The T-AOC (HY-60021), SOD (HY-M0001), GSH-PX (HY-60005), and MDA (HY-60003) assay kits purchased from the Beijing Sino-UK Institute of Biological Technology were used. All experiments were performed according to the manufacturer's instructions.
Histological Assay
Small intestine samples, i.e., jejunum and ileum, were prepared for histological analysis. First, these samples in para form solution were embedded in paraffin and cut into 5-μm-thick sections. Next, hematoxylin–eosin staining was carried out using this sequence of procedures: dehydration, embedding, sectioning, and staining. Villous height and crypt depth were measured from three discontinuous sections of each sample were made for observation, and six complete, typical fields of view were selected for each sample using an optical binocular microscope (Olympus BX5; Olympus Optical Co. Ltd, Tokyo, Japan) equipped with a digital camera (Nikon Eclipse Ci-L; Nikon, Tokyo, Japan) and an image analyzer (Image-Pro Plus 6.0; Media Cybernetics, Bethesda, MD, U.S.A.) (35).
Total RNA Isolation and Quantitative Real-Time PCR
The total RNA was extracted from different animal tissues with the E.Z.N.A total RNA Kit II (OMEGA Bio-Tek, Norcross, USA), was then stored at −80°C until the cDNA synthesis. The RNA quality was measured using a Nanodrop spectrophotometer (Thermo Fisher, Waltham, USA) at 260 and 280 nm. Approximately, 1 μg of RNA was reverse transcribed into cDNA in a total volume of 10 μl by using the HiScript RII Q RT SuperMix for qPCR (+gDNA wiper [Vazyme, R223-01, Nanjing, China]). The levels of SOD-1, CAT, GPX-1, NRF2, NQO1, HO-1, CLAUDIN-1, OCCLUDIN, and ZO-1 expression were determined in jejunum and ileum samples. All primer sequences (Table 2) were designed in Primer 5.0 software. The PCR reactions were performed in a LightCycler96 (Roche, Basel, Switzerland), using the SYBR Green PCR Master Mix (Vazyme, Q711-02/03, Nanjing, China) to the manufacturer's protocol. The cycle threshold was collected from each reaction, and the relative expression level of different genes' mRNA to the β-actin mRNA was evaluated using the 2−ΔΔCT method. β-actin is used as an internal control to normalize target gene transcript levels.
Statistical Analysis
This analysis was implemented in SPSS 15.0 (Statistical Product and Service Solutions, Inc., USA) and plotted to utilize GraphPad Prism 8 (GraphPad, CA, USA). One-way analysis of variance (ANOVA) followed by the least significant difference (LSD) test was used for multiple comparisons with each pullet as the experimental unit. Results were presented as means ± the standard error of the mean (SEM). p < 0.05 were considered statistically significant.
Results
Serum Antioxidative Capacity
According to the results given in Table 3, on day 1 post-injection, the serum MDA concentration of pullets was higher in the DIQ group than in CON groups injected with normal saline (p < 0.05). Meanwhile, the serum MDA concentration of the DIQ group supplemented with the basal diet was higher than that of samples supplemented with resveratrol and apigenin (p < 0.05). The diquat treatment tended to block serum SOD activity (p < 0.05), but resveratrol and apigenin markedly restored (p < 0.05) that inhibited function when compared with the DIQ group. The GSH-PX concentration significantly decreased after exposure to diquat (p < 0.05). In contrast, dietary supplementation with resveratrol and apigenin increased the serum GSH-PX level when compared with the DIQ group (p < 0.05). The serum T-AOC level was more remarkable in pullets fed a diet with 500 mg/kg of resveratrol or apigenin with the diquat treatment than that in the DIQ group. The antioxidant status of serum was examined on day 10 after the diquat injection (Table 3). Compared with the CON group, the diquat significantly increased the serum MDA concentration and decreased the concentrations of SOD, GSH-PX, and T-AOC. On the contrary, resveratrol and apigenin attenuated the enhanced MDA levels induced by diquat and augmented the SOD, GSH-PX, and T-AOC levels compared with the DIQ group.
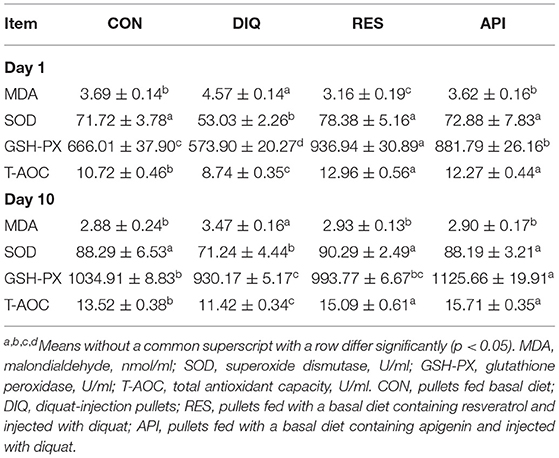
Table 3. Serum antioxidative status activity of pullets fed with resveratrol and apigenin on day 1 and day 10 after their injection with diquat.
Expression of Antioxidant Enzyme Genes in Ileum Tissue and in Jejunum Tissue
As shown in Figure 1A, the expression levels of SOD-1 and CAT in ileum tissue of pullets on day 10 were lower in the DIQ group than those in the CON group (p < 0.05). Resveratrol and apigenin supplementation elevated the expression level of SOD-1 in ileum tissue compared to the CON group (p < 0.05). Moreover, on day 10, the expression levels of SOD-1 and CAT in jejunum tissue of pullets were lower in the DIQ group than those in the CON group (p < 0.05) (Figure 1B). On the contrary, the expression levels of SOD-1 and CAT in jejunum tissue were significantly increased in the API group, as compared to those in the DIQ group (p < 0.05). The expression level of GPX-1 in ileum tissue and jejunum tissue was upregulated in the DIQ group than those in the CON group (p < 0.05), and resveratrol and apigenin supplementation could reverse in jejunum tissue (p < 0.05).
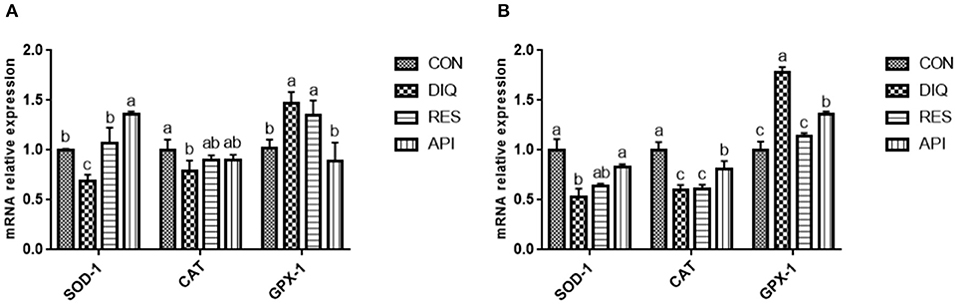
Figure 1. Effects of dietary resveratrol and apigenin supplementation on mRNA expression of antioxidant genes in ileum and jejunum on day 10. (A) Related genes expression level in the ileum of pullets. (B) Related genes expression level in the jejunum of pullets. SOD-1, superoxide dismutase 1; CAT, catalase; GPX-1, glutathione peroxidase 1. CON, pullets fed basal diet; DIQ, diquat-injection pullets; RES, pullets fed with a basal diet containing resveratrol and injected with diquat; API, pullets fed with a basal diet containing apigenin and injected with diquat. Data are means ± standard error; mean values sharing different lowercase letters differ significantly (p < 0.05).
Intestinal Morphology
Morphology, villus height, and crypt depth of the jejunum and ileum on day 10 are shown in Figure 2 and Table 4. In the ileum tissue, the structure of the CON group gut was clear, the mucosal layer epithelium was complete, and the intestinal villi were arranged regularly. In the DIQ group, the villus height was higher (p < 0.05) compared with those of the CON group, whereas in both RES and API groups, the villus height of the RES and API group was higher than that of the DIQ group (p < 0.05). Similarly, for the morphology of jejunum tissue, compared with CON, the villus height of the jejunum was significantly decreased in DIQ (p < 0.05). However, the dietary supplement containing resveratrol alleviated this abnormal condition by increasing the height of the intestinal villi of pullets under stress (p < 0.05).
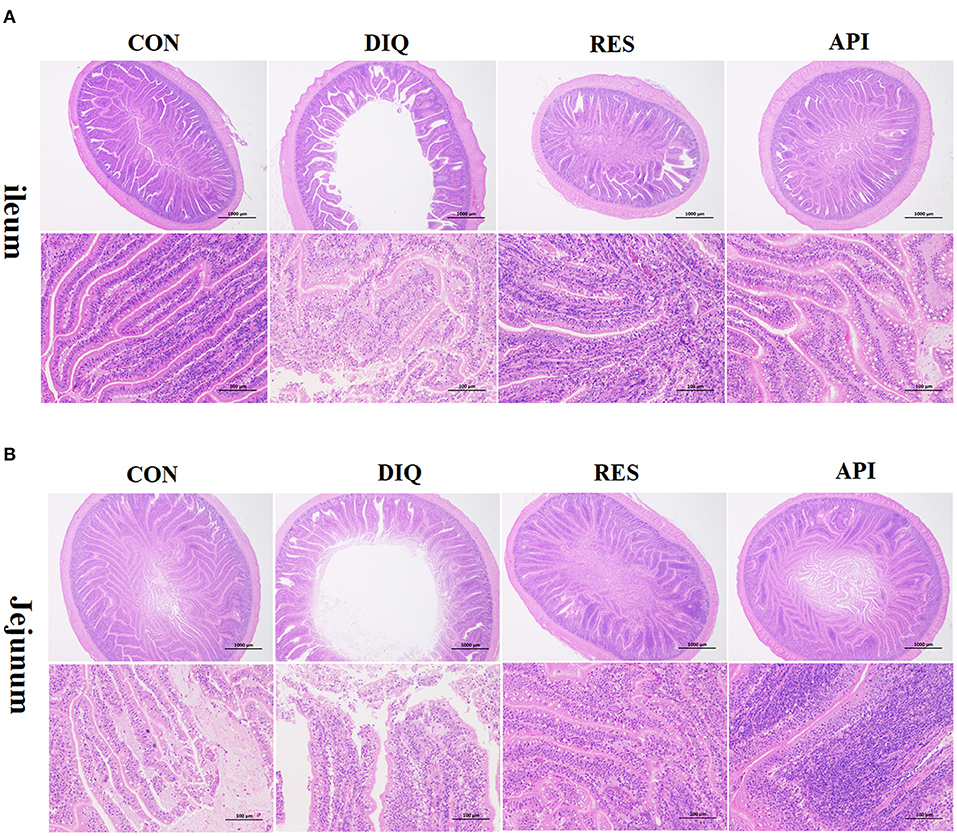
Figure 2. Effect of resveratrol and apigenin on intestinal morphological structure in diquat-induce oxidative stress with chickens on day 10 after injection with diquat (HE, ×20 and ×200). (A) The images of the ileum morphology. (B) The images of the jejunum morphology. CON, pullets fed basal diet; DIQ, diquat-injection pullets; RES, pullets fed with a basal diet containing resveratrol and injected with diquat; API, pullets fed with a basal diet containing apigenin and injected with diquat.
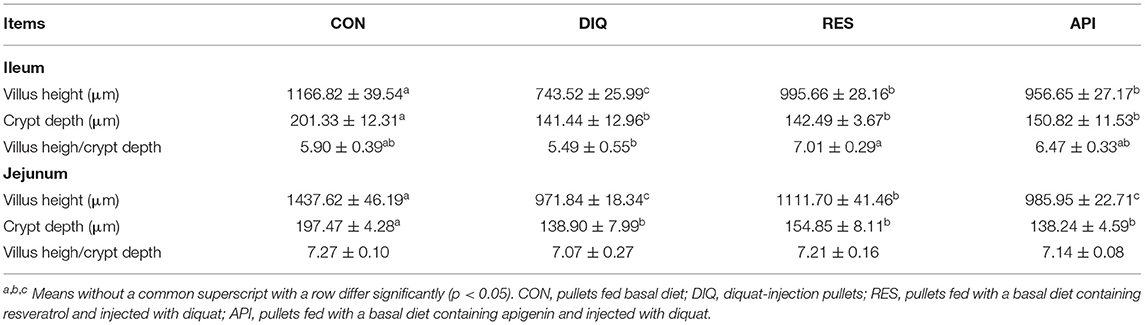
Table 4. Effects of resveratrol and apigenin on intestinal morphology in ileum and jejunum of pullets with injected diquat on day 10.
Relative Expression of the NRF2 Signaling Pathway and Tight Junction mRNAs in Ileum Tissue
The relative expression levels of mRNAs involved in the NRF2 signaling pathway on day 1 are shown in Figures 3A–C. When compared with the saline-treated pullets, diquat-treated ones fed the basal diet had lower mRNA abundances of NRF2, NQO1, and HO-1 in their ileum (p < 0.05). In contrast, the diquat-challenged chickens fed the diet supplemented with resveratrol and apigenin did not differ from the DIQ group in their NRF2 and HO-1 mRNA expression (p > 0.05). The levels NRF2, NQO1, and HO-1 mRNA expression of the API group were similar to those of the DIQ group. The mRNA expression levels of CLAUDIN-1, OCCLUDIN, and ZO-1 in the ileum are presented in Figures 3D–F. Pullets in the RES and API groups exhibited higher CLAUDIN-1 mRNA levels in the ileum than it in the DIQ group (p < 0.05). In addition, both resveratrol and apigenin supplementation groups tended to undergo greater ZO-1 mRNA expression than the DIQ group (p > 0.05). Concerning the mRNA expression of OCCLUDIN, its level significantly increased in the DIQ group compared with the CON group, but this phenomenon was partly relieved in the supplementation groups. Next, we performed qPCR to test the validity of expressed mRNA of NRF2, NQO1, HO-1, CLAUDIN-1, OCCLUDIN, and ZO-1 in the ileum of the four groups on day 10 (Figure 4). Compared with the CON group, the expression levels of NRF2, NQO1, and HO-1 were significantly decreased in the DIQ group but increased in the RES group and API group (p < 0.05). Further, the mRNA expression level of OCCLUDIN was downregulated, and the ZO-1 mRNA expression level was upregulated in the RES and API groups compared with those in the DIQ group (p < 0.05).
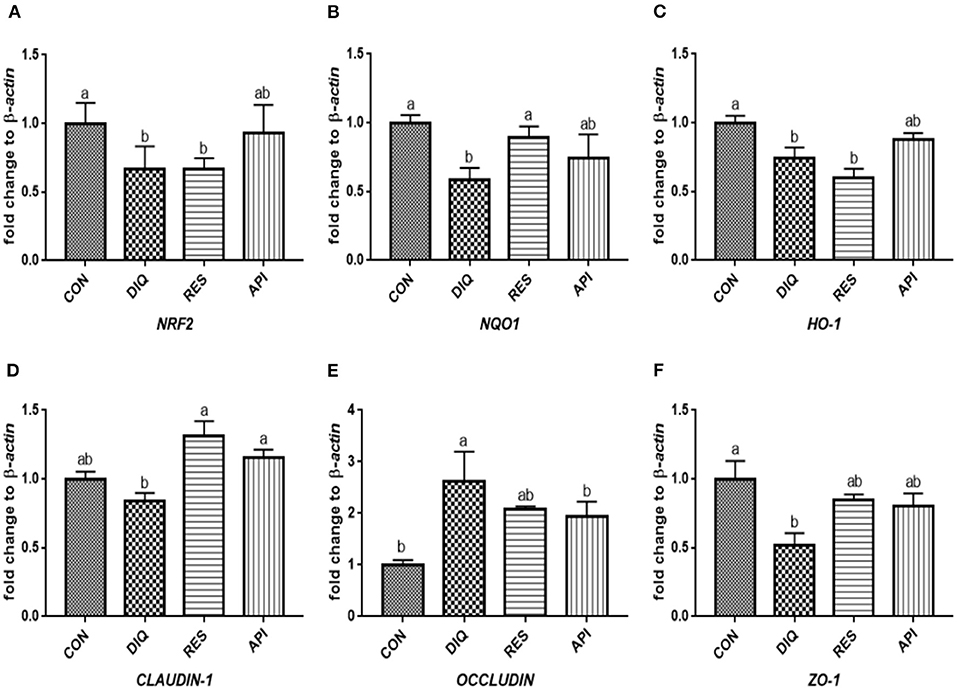
Figure 3. Effects of dietary resveratrol and apigenin supplementation on mRNA expression of antioxidant genes and tight junction RNAs in ileum on day 1. (A) The expression level of NRF2 gene in ileum from different groups. (B) The expression level of NQO1 gene in ileum from different groups. (C) The expression level of HO-1 gene in ileum from different groups. (D) The expression level of CLAUDIN-1 gene in ileum from different groups. (E) The expression level of OCCLUDIN gene in ileum from different groups. (F) The expression level of ZO-1 gene in ileum from different groups. NRF2, nuclear factor erythroid 2-related factor 2; NQO1, NAD (P) H dehydrogenase quinone 1; HO-1, heme oxygenase-1; ZO1, zonula occludens-1. CON, pullets fed basal diet; DIQ, diquat-injection pullets; RES, pullets fed with a basal diet containing resveratrol and injected with diquat; API, pullets fed with a basal diet containing apigenin and injected with diquat. Data are means ± standard error; mean values sharing different lowercase letters differ significantly (p < 0.05).
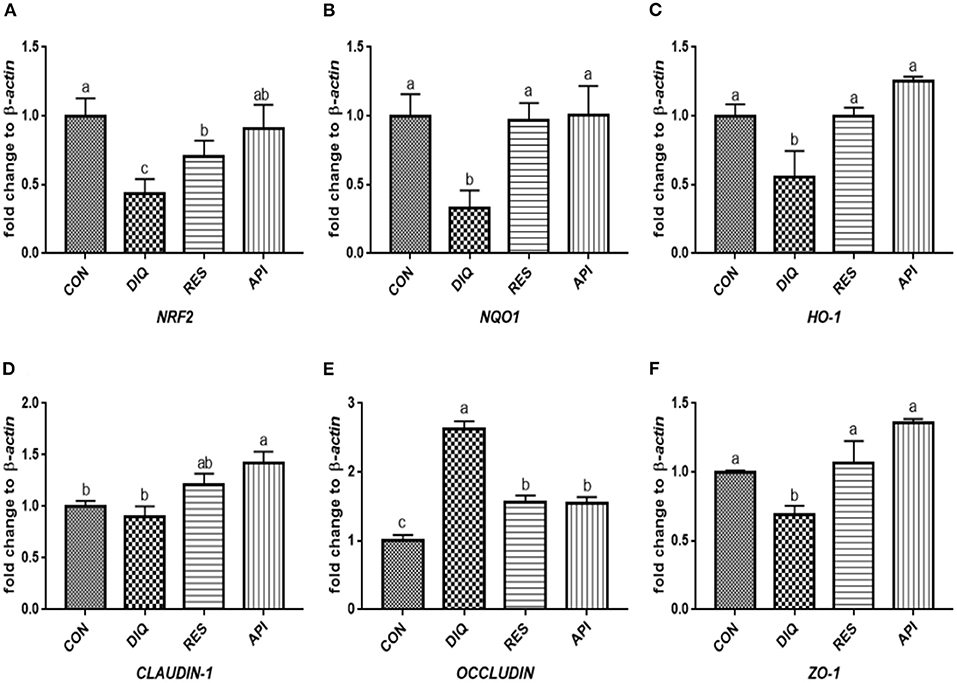
Figure 4. Effects of dietary resveratrol and apigenin supplementation on mRNA expression of antioxidant genes and tight junction RNAs in ileum on day 10. (A) The expression level of NRF2 gene in ileum from different groups. (B) The expression level of NQO1 gene in ileum from different groups. (C) The expression level of HO-1 gene in ileum from different groups. (D) The expression level of CLAUDIN-1 gene in ileum from different groups. (E) The expression level of OCCLUDIN gene in ileum from different groups. (F) The expression level of ZO-1 gene in ileum from different groups. NRF2, nuclear factor erythroid 2-related factor 2; NQO1, NAD (P) H dehydrogenase quinone 1; HO-1, heme oxygenase-1; ZO1, zonula occludens-1. CON, pullets fed basal diet; DIQ, diquat-injection pullets; RES, pullets fed with a basal diet containing resveratrol and injected with diquat; API, pullets fed with a basal diet containing apigenin and injected with diquat. Data are means ± standard error; mean values sharing different lowercase letters differ significantly (p < 0.05).
Relative Expression of the NRF2 Signaling Pathway and Tight Junction mRNAs in Jejunum Tissue
The day 1 detection results (Figure 5) revealed a trend of downregulated NRF2, NQO1, and HO-1 mRNA levels when exposed to diquat (p < 0.05). The NRF2 and HO-1mRNA levels of the DIQ group were on par with those of the API group (p > 0.05). Compared with the CON group, the diquat injection lowered the mRNA abundance of CLAUDIN-1 (p < 0.05). At the same time, resveratrol and apigenin increased the CLAUDIN-1 gene levels (p < 0.05), and vice versa for the OCCLUDIN gene. ZO-1 expression of the DIQ group was not significantly different from the RES group (p > 0.05), whereas it was significantly lower than that of the API group (p < 0.05). The relative mRNA expression levels of the Nrf2 signaling pathway and tight junction genes (NRF2, NQO1, HO-1, CLAUDIN-1, OCCLUDIN, and ZO-1) on day 10 are shown in Figure 6. Unlike the trends on day 1, the NQO1 and HO-1 mRNA levels of both RES and API groups were significantly those of the DIQ group (p < 0.05). CLAUDIN-1 and ZO-1 mRNA expression levels of the DIQ group were downregulated compared to the CON group (p < 0.05). Moreover, ZO-1 mRNA expression has no significant difference in the RES or API group than in the DIQ group (p > 0.05).
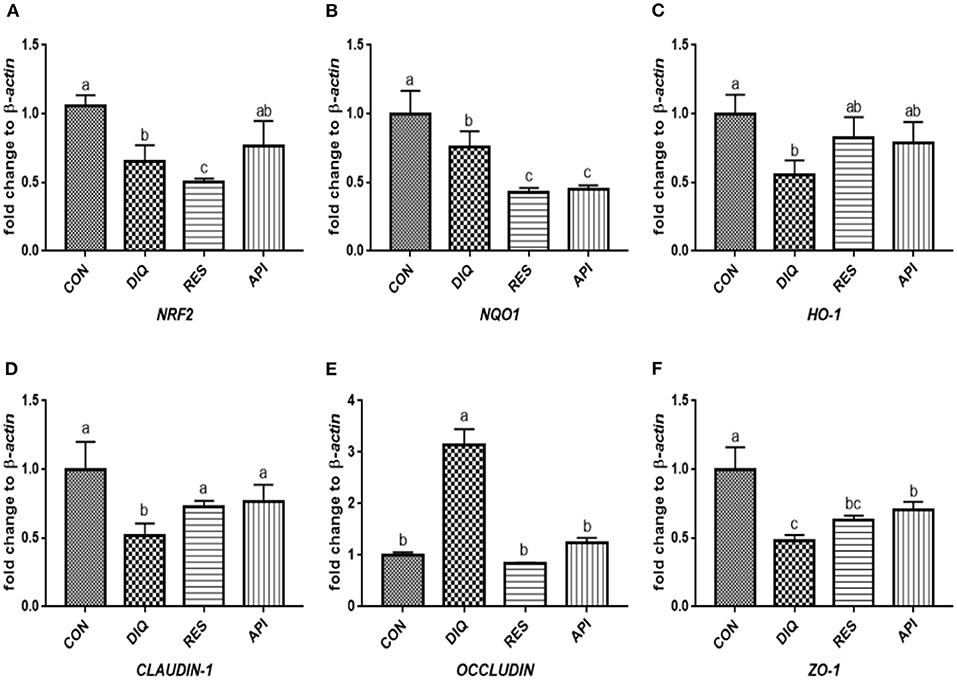
Figure 5. Effects of dietary resveratrol and apigenin supplementation on mRNA expression of antioxidant genes and tight junction RNAs in jejunum on day 1. (A) The expression level of NRF2 gene in jejunum from different groups. (B) The expression level of NQO1 gene in jejunum from different groups. (C) The expression level of HO-1 gene in jejunum from different groups. (D) The expression level of CLAUDIN-1 gene in jejunum from different groups. (E) The expression level of OCCLUDIN gene in jejunum from different groups. (F) The expression level of ZO-1 gene in jejunum from different groups. NRF2, nuclear factor erythroid 2-related factor 2; NQO1, NAD (P) H dehydrogenase quinone 1; HO-1, heme oxygenase-1; ZO1, zonula occludens-1. CON, pullets fed basal diet; DIQ, diquat-injection pullets; RES, pullets fed with a basal diet containing resveratrol and injected with diquat; API, pullets fed with a basal diet containing apigenin and injected with diquat. Data are means ± standard error; mean values sharing different lowercase letters differ significantly (p < 0.05).
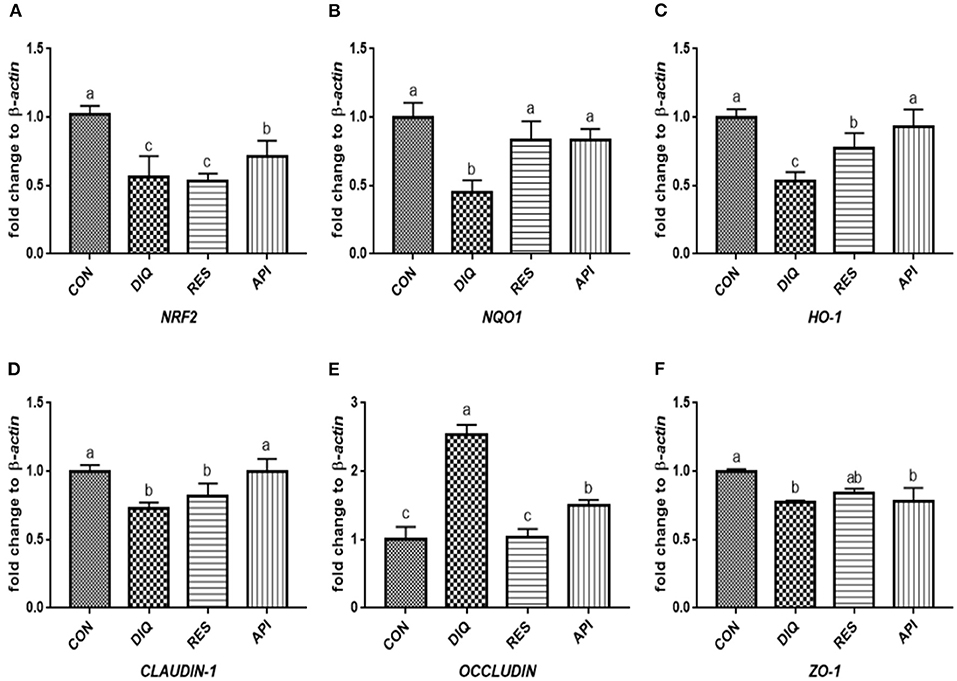
Figure 6. Effects of dietary resveratrol and apigenin supplementation on mRNA expression of antioxidant genes and tight junction RNAs in jejunum on day 10. (A) The expression level of NRF2 gene in jejunum from different groups. (B) The expression level of NQO1 gene in jejunum from different groups. (C) The expression level of HO-1 gene in jejunum from different groups. (D) The expression level of CLAUDIN-1 gene in jejunum from different groups. (E) The expression level of OCCLUDIN gene in jejunum from different groups. (F) The expression level of ZO-1 gene in jejunum from different groups. NRF2, nuclear factor erythroid 2-related factor 2; NQO1, NAD (P) H dehydrogenase quinone 1; HO-1, heme oxygenase-1; ZO1, zonula occludens-1. CON, pullets fed basal diet; DIQ, diquat-injection pullets; RES, pullets fed with a basal diet containing resveratrol and injected with diquat; API, pullets fed with a basal diet containing apigenin and injected with diquat. Data are means ± standard error; mean values sharing different lowercase letters differ significantly (p < 0.05).
Discussion
In the present study, we relied on diquat to induce intestinal oxidative stress injury in pullets and examined the protective function of resveratrol and apigenin toward mitigating that process. Our results showed that the serum concentration of MDA rose after diquat injection on the pullets fed the basal diet treatment on day 1 and 10, while the main antioxidative parameters SOD, GSH-PX, and T-AOC were lower in the CON group than those of the DIQ group, which supports previous results (17, 18, 36). Similarly, the previous result showed that diquat down-regulated the expression levels of SOD-1 and GPX-1 in rats' spleen (37), but Chen et al. found that diquat increased the expression level of GPX-1 in the liver of broilers (38). These results confirmed that the antioxidative capabilities of pullets were impaired after injection with diquat. This was due to the excessive production of ROS with an increase in the level of MDA, finally, the product of peroxidation of polyunsaturated fatty acids, and related esters, and cells can be protected from oxidative damage with SOD, which converts superoxide anion to hydrogen peroxide that is removed by GSH-Px (36). Resveratrol, a plant polyphenol, appears to have a potent antioxidant property via increasing levels of GSH and modulating antioxidant enzymes (MDA, SOD, and CAT) (24, 39). A study in mice proved that the level of SOD in serum was improved by dietary resveratrol supplementation, whereas the MDA concentration in the serum was downregulated (40). Furthermore, The levels of SOD and GSH-PX in the plasma of sows were increased, meanwhile, the MDA level was decreased by resveratrol supplementation (41). As for the studies on chickens, dietary supplementation of resveratrol was found to protect against heat stress by increasing the muscle GSH-PX and T-AOC activities and decreasing muscle MDA levels (42). Moreover, resveratrol could ameliorate heat stress in chickens by enhancing their antioxidant capacity and reducing their MDA content (34, 43). Previous studies showed that resveratrol increased the expression level of SOD-1 in tissues to resist oxidative stress and heat stress in rats (44, 45). In vitro, the expression level of SOD-1 was upregulated by resveratrol in cells under H2O2-induced (46, 47). Apigenin is a flavonoid compound abundantly present in common fruits and vegetables, increasingly noted for its various pharmacological effects, especially its anti-oxidation property (48). Studies about the antioxidant effect of apigenin are mainly based on in vitro cellular experiments, and only a few studies have investigated the antioxidant effect of apigenin in vivo on chickens. Our results revealed that the activity of several essential enzymes participating in the antioxidant defense system, namely SOD, T-AOC, and GSH-PX, was significantly enhanced by apigenin post-diquat treatment. Conversely, the MDA level was inhibited by apigenin on days 1 and 10, which is in accordance with previous studies (49, 50). Therefore, the improved antioxidative capacity induced by resveratrol and apigenin may partly explain why resveratrol and apigenin are beneficial to the pullets under oxidative stress.
The intestinal epithelial barrier is the first line of defense against a hostile environment within the intestinal lumen. We found that administering a diquat injection to chickens disrupted their intestinal morphology, with large amounts of intestinal villi shed and large quantities of epithelial cells appearing necrotic, findings similar to a few studies (18, 20, 51). Other research works showed that resveratrol treatment partly improved the histological morphology of jejunum in heat-stressed rats (44); a diet supplemented with resveratrol countered the impairment of intestinal morphology in heat-stressed broilers (42); and resveratrol supplementation significantly protected against intestinal morphological damage and weakened intestinal integrity in weaning piglets (52). Thus, these studies suggest that resveratrol supplementation can benefit intestinal health. Similarly, we found that supplementation with resveratrol developed the morphology of jejunum and ileum. Moreover, the present study is the first to explore the ameliorative effect of apigenin supplementation against oxidative stress and intestinal morphological changes induced by diquat in pullets. As expected, administration of apigenin reduced the oxidative stress response induced by diquat and relieved the injury of ileum and jejunal morphology in pullets.
As a pivotal sensor of oxidative stress, nuclear factor erythroid 2-related factor 2 (NRF2) plays a central role in the regulation of antioxidant and phase 2 detoxifying enzymes and related proteins (53). GSH is one of the most versatile cellular antioxidants, and all enzymes involved in GSH biosynthesis are controlled by NRF2 (54). Previous studies have shown that NRF2 can regulate the antioxidant response and represents the underlying mechanism that provides a pivotal defense in animals against diquat toxicity (55). Once stimulated by inducers, NRF2 activates downstream enzymes, including NQO1 and HO-1, to prevent oxidative stress damage from occurring. In this study, the diquat challenge downregulated the intestinal NRF2 expression and its downstream target genes NQO1 and HO-1. This could be inferred as an acute response to diquat-induced oxidative stress, which was in agreement with the results (56, 57). Resveratrol could not increase the expression of the NRF2 gene in the liver of rats exposed to high temperature (45), akin to our result that resveratrol was unable to upregulate the expression of the NRF2 gene, except in ileum on day 10. However, a previous study did show that the NRF2 protein and HO-1 gene expression levels are increased in pigs by dietary resveratrol supplementation (41). Moreover, a resveratrol treatment considerably increased the HO-1 protein levels in the heart tissue of rats, suggesting resveratrol greatly improved their antioxidant ability (58). In vitro, an H2O2 treatment markedly decreased the expression of NRF2 and HO-1 in cells, whereas resveratrol significantly reversed the H2O2-induced downregulation of NRF2 and HO-1 (47, 59). Apigenin has been capable of augmenting the expression of HO-1, NQO1, and GCLM at both the mRNA and protein levels (60). Similarly, apigenin dramatically raised the mRNA and protein expression of NRF2, HO-1, and NQO1 to higher levels in a dose-dependent manner (61). The present study showed that resveratrol and apigenin supplementation for 10 days markedly promoted the mRNA expressions of HO-1 and NQO1 in the ileum and jejunum of diquat-induced pullets. These results suggest that resveratrol and apigenin have a time-dependent effect on the anti-oxidative stress of the small intestine involving the Nrf2 pathway caused by diquat.
The intestinal barrier is physically composed of epithelial cells connected by tight junction proteins, such as ZO-1, CLAUDIN-1, and OCCLUDIN, which regulate epithelial cell-selective permeability. Therefore, the expression of genes is crucial for maintaining a functionally intact intestinal epithelial barrier (62). The family of ZO is a part of the cytoplasmic plaque of the tight junction proteins, and the occludin and clauding families are markers of tight junction integrity found in the epithelial barrier; their presence or absence could reflect the permeability of the intestinal epithelium (63). The integrity of the intestinal mucosal barrier prevents and defends against the invasion of stimulating factors and bacteria and further mitigates intestinal inflammation and oxidative stress (64). Tight junction mRNAs and proteins expression can vary according to the exposure to diquat (18, 20), for example, diquat challenge decreased the mRNA and protein levels of OCCLUDIN, CLAUDIN-1, and ZO-1 in the jejunal mucosa of piglets compared with the control group (65), which matched our results. However, we noticed that oxidative stress had negative effects on CLAUDIN-1 gene expression in the ileum, which is consistent with the results of a previous study (20). Whether this phenomenon is induced by diquat still requires further investigation, and it may be related to a compensatory effect. According to other research, resveratrol could prevent diquat from causing a decline in the CLAUDIN-1 expression level in jejunal mucosa of piglets (66). However, there was no significant difference in the mRNA expressions of OCCLUDIN and CLAUDIN-1 between the resveratrol groups and the control group in jejunal mucosa of piglets (52). This phenomenon suggests that resveratrol and apigenin supplementation may result in different expression patterns of tight junction genes in the ileum and jejunum. The current results collectively provide essential evidence of the potential protective effects of resveratrol and apigenin against diquat-induced dysfunction in the intestinal barrier.
Conclusions
Collectively, the results of this study demonstrated that dietary supplementation of resveratrol and apigenin conferred relieved the intestinal oxidative stress by modulating the NRF2 pathway and tight junction mRNAs in diquat-induced pullets, indicating that resveratrol and apigenin could be promising antioxidant additives for use in mitigating oxidative stress and protecting the intestinal barrier of farmed poultry. However, further investigation is needed for a deeper understanding of the action mechanisms of resveratrol and apigenin in diquat-induced animal models.
Data Availability Statement
The datasets presented in this study can be found in online repositories. The names of the repository/repositories and accession number(s) can be found in the article/supplementary material.
Ethics Statement
The animal study was reviewed and approved by the Local Experimental Animal Care Committee and all procedures were implemented and approved by Nanjing Agricultural University.
Author Contributions
NZ conducted the animal experiments and wrote the manuscript. YT, WX, and TG contributed to the study design. WL and BT executed the lab analysis and performed the statistical analysis. KZ and LL revised the paper. All authors carefully read and approved the final revision of the manuscript.
Funding
This work was supported by Zhejiang Provincial Key Research and Development Plan (No. 2021C02034) and Zhejiang Provincial Special Commissioner Team Projects of Science & Technology (No. Xianju Chicken Industry, 2020–2024).
Conflict of Interest
WL was employed by Huzhou Lvchang Ecoagriculture Co., Ltd.
The remaining authors declare that the research was conducted in the absence of any commercial or financial relationships that could be construed as a potential conflict of interest.
Publisher's Note
All claims expressed in this article are solely those of the author and do not necessarily represent those of their affiliated organizations, or those of the publisher, the editors and the reviewers. Any product that may be evaluated in this article, or claim that may be made by its manufacturer, is not guaranteed or endorsed by the publisher.
References
1. Frankič T, Salobir K, Salobir J. The comparison of in vivo antigenotoxic and antioxidative capacity of two propylene glycol extracts of calendula officinalis (marigold) and vitamin E in young growing pigs. J Anim Physiol Anim Nutr. (2010) 93:688–94. doi: 10.1111/j.1439-0396.2008.00855.x
2. Cap M, Vachova L, Palkova Z. Reactive oxygen species in the signaling and adaptation of multicellular microbial communities. Oxid Med Cell Longev. (2012) 2012:976753. doi: 10.1155/2012/976753
3. Kumar S, Pandey AK. Free radicals: health implications and their mitigation by herbals. Br J Med Med Res. (2015) 7:438–57. doi: 10.9734/BJMMR/2015/16284
4. Bhat AH, Dar KB, Anees S, Zargar MA, Masood A, Sofi MA, et al. Oxidative stress, mitochondrial dysfunction and neurodegenerative diseases; a mechanistic insight. Biomed Pharmacother. (2015) 74:101–10. doi: 10.1016/j.biopha.2015.07.025
5. Han JY, Shuvaev VV, Muzykantov VR. Catalase and SOD conjugated with PECAM antibody distinctly alleviate abnormal endothelial permeability caused by exogenous ROS and vascular endothelial growth factor. J Pharmacol Exp Ther. (2011) 378:82–91. doi: 10.1124/jpet.111.180620
6. Zanichelli F, Capasso S, Bernardo GD, Cipollaro M, Pagnotta E, Cartenì M, et al. Low concentrations of isothiocyanates protect mesenchymal stem cells from oxidative injuries, while high concentrations exacerbate DNA damage. Apoptosis. (2012) 17:964–74. doi: 10.1007/s10495-012-0740-3
7. Song DG, Cheng YZ, Li XX, Wang FQ, Lu ZQ, Xiao X, et al. Biogenic nanoselenium particles effectively attenuate oxidative stress-induced intestinal epithelial barrier injury by activating the Nrf2 antioxidant pathway. ACS Appl Mater Interfaces. (2017) 9:14724–40. doi: 10.1021/acsami.7b03377
8. Wang Y, Wu YP, Wang YB, Fu AK, Gong L, Li WF, et al. Bacillus amyloliquefaciens SC06 alleviates the oxidative stress of IPEC-1 via modulating Nrf2/Keap1 signaling pathway and decreasing ROS production. Appl Microbiol Biotechnol. (2017) 101:3015–26. doi: 10.1007/s00253-016-8032-4
9. Guo QP, Li FN, Duan YH, Wen CY, Wang WL, Zhang LY, et al. Oxidative stress, nutritional antioxidants and beyond. Sci China. (2020) 63:78–86. doi: 10.1007/s11427-019-9591-5
10. Guilloteau P, Zabielski R, Hammon HM, Metges CC. Nutritional programming of gastrointestinal tract development. Is the pig a good model for man? Nutr Res Rev. (2010) 23:4–22. doi: 10.1017/S0954422410000077
11. Rahal A, Kumar A, Singh V, Yadav B, Tiwari R, Chakraborty S, et al. Oxidative stress, prooxidants, and antioxidants: the interplay. Biomed Res. Int. (2014) 2014:761264. doi: 10.1155/2014/761264
12. Rosero DS, Odle J, Moeser AJ, Boyd RD, Van Heugten E. Peroxidised dietary lipids impair intestinal function and morphology of the small intestine villi of nursery pigs in a dose-dependent manner. Br J Nutr. (2015) 114:1985–92. doi: 10.1017/S000711451500392X
13. Li Y, Hansen SL, Borst LB, Spears JW, Moeser AJ. Dietary iron deficiency and oversupplementation increase intestinal permeability, ion transport, and inflammation in pigs. J Nutr. (2016) 146:1499–505. doi: 10.3945/jn.116.231621
14. Elkouby-Naor L, Ben-Yosef T. Functions of claudin tight junction proteins and their complex interactions in various physiological systems. Int Rev Cell Mol Biol. (2010) 279:1–32. doi: 10.1016/S1937-6448(10)79001-8
15. Sun ZW, Olanders K, Lasson A, Dib M, Annborn M, Andersson K, et al. Effective treatment of gut barrier dysfunction using an antioxidant, a PAF inhibitor, and monoclonal antibodies against the adhesion molecule PECAM-1. J Surg Res. (2002) 105:220–33. doi: 10.1006/jsre.2001.6342
16. Yin J, Ren WK, Wu XS, Yang G, Wang J, Li TJ. Oxidative stress-mediated signaling pathways: a review. J Food Agric Environ. (2013) 11:132–9. Available online at: https://www.researchgate.net/publication/286003969
17. Yuan D, Tarique H, Tan B, Liu YH, Ji P, Yin YL. The evaluation of antioxidant and anti-Inflammatory effects of eucommia ulmoides flavones using diquat-challenged piglet models. Oxid Med Cell Longev. (2017) 2017:8140962. doi: 10.1155/2017/8140962
18. Cao ST, Wu H, Wang CC, Zhang QH, Jiao LF, Lin FH, et al. Diquat-induced oxidative stress increases intestinal permeability, impairs mitochondrial function and triggers mitophagy in piglets. J Anim Sci. (2018) 96:1795–805. doi: 10.1093/jas/sky104
19. Li M, Yuan DX, Liu YH, Jin H, Tan B. Dietary puerarin supplementation alleviates oxidative stress in the small intestines of diquat-challenged piglets. Animals. (2020) 10:631. doi: 10.3390/ani10040631
20. Wen C, Guo Q, Wang W, Duan Y, Zhang L, Li J, et al. Taurine alleviates intestinal injury by mediating tight junction barriers in diquat-challenged piglet models. Front Physiol. (2020) 11:449. doi: 10.3389/fphys.2020.00449
21. Tsunada S, Iwakiri R, Fujimoto K, Aw TY. Chronic lipid hydro peroxide stress suppresses mucosal proliferation in rat intestine: potentiation of ornithine decarboxylase activity by epidermal growth factor. Dig Dis Sci. (2003) 48:2333–41. doi: 10.1023/B:DDAS.0000007872.66693.6c
22. Selman C, McLaren JS, Himanka MJ, Speakman JR. Effect of long-term cold exposure on antioxidant enzyme activities in a small mammal. Free Radic Biol Med. (2000) 28:1279–85. doi: 10.1016/S0891-5849(00)00263-X
23. Finkel T, Holbrook NJ. Oxidants, oxidative stress and the biology of ageing. Nature. (2000) 408:239–47. doi: 10.1038/35041687
24. Bitterman JL, Chung JH. Metabolic effects of resveratrol: addressing the controversies. Cell Mol Life Sci. (2015) 72:1473–88. doi: 10.1007/s00018-014-1808-8
25. Zhang C, Luo JQ, Yu B, Zheng P, Huang ZQ, Mao XB, et al. Dietary resveratrol supplementation improves meat quality of finishing pigs through changing muscle fiber characteristics and antioxidative status. Meat Sci. (2015) 102:15–21. doi: 10.1016/j.meatsci.2014.11.014
26. Xia N, Daiber A, Frstermann U, Li HG. Antioxidant effects of resveratrol in the cardiovascular system. Br J Pharmacol. (2017) 174:1633–46. doi: 10.1111/bph.13492
27. Zhang HZ, Chen DW, He J, Zheng P, Yu J, Mao XB, et al. Long-term dietary resveratrol supplementation decreased serum lipids levels, improved intramuscular fat content, and changed the expression of several lipid metabolism-related miRNAs and genes in growing-finishing pigs. J Anim Sci. (2019) 97:1745–56. doi: 10.1093/jas/skz057
28. Zhang C, Chen KK, Zhao XH, Geng. ZY. Protective effects of resveratrol against high ambient temperature-induced spleen dysplasia in broilers through modulating splenic redox status apoptosis. J Sci Food Agric. (2018a) 98:5409–17. doi: 10.1002/jsfa.9084
29. Zhang C, Yang L, Zhao XH, Chen XY, Wang L, Geng ZY. Effect of dietary resveratrol supplementation on meat quality, muscle antioxidative capacity and mitochondrial biogenesis of broilers. J Sci Food Agric. (2018b) 98:1216–21. doi: 10.1002/jsfa.8576
30. Madunić J, Madunić IV, Gajski G, Popić J, Garaj-Vrhovac V. Apigenin: a dietary flavonoid with diverse anticancer properties. Cancer Lett. (2018) 413:11–22. doi: 10.1016/j.canlet.2017.10.041
31. Li F, Lang FF, Zhang HL, Xu LD, Wang YD, Zhai CX, et al. Apigenin alleviates endotoxin-induced myocardial toxicity by modulating inflammation, oxidative stress, and autophagy. Oxid Med Cell Longev. (2017) 2017:2302896. doi: 10.1155/2017/2302896
32. Sánchez-Marzo N, Pérez-Sánchez A, Ruiz-Torres V, Martínez-Tébar A, Castillo J, Herranz-López M, et al. Antioxidant and photoprotective activity of apigenin and its potassium salt derivative in human keratinocytes and absorption in caco-2 cell monolayers. Int J Mol Sci. (2019) 20:2148. doi: 10.3390/ijms20092148
33. Wang DT, Yang YJ, Zou XH, Zhang J, Zheng ZN, Wang ZW. Antioxidant apigenin relieves age-related muscle atrophy by inhibiting oxidative stress and hyperactive mitophagy and apoptosis in skeletal muscle of mice. J Gerontol A Biol Sci Med Sci. (2020) 75:2081–8. doi: 10.1093/gerona/glaa214
34. He SP, Li S, Arowolo MA, Yu QF, Chen F, Hu RZ, et al. Effect of resveratrol on growth performance, rectal temperature and serum parameters of yellow-feather broilers under heat stress. Anim Sci J. (2019) 90:401–11. doi: 10.1111/asj.13161
35. Dong YY, Lei JQ, Zhang BK. Effects of dietary quercetin on the antioxidative status and cecal microbiota in broiler chickens fed with oxidized oil. Poult Sci. (2020) 99:4892–903. doi: 10.1016/j.psj.2020.06.028
36. Chen YN, Chen YP, Zhang H, Wang T. Pterostilbene as a protective antioxidant attenuates diquat-induced liver injury and oxidative stress in 21-day-old broiler chickens. Poult Sci. (2020) 99:3158–67. doi: 10.1016/j.psj.2020.01.021
37. Mo WW, Wu XJ, Jia G, Zhao H, Chen XL, Tang JY, et al. Roles of dietary supplementation with arginine or N-carbamylglutamate in modulating the inflammation, antioxidant property, and mRNA expression of antioxidant-relative signaling molecules in the spleen of rats under oxidative stress. Anim Nutr. (2018) 4:322–8. doi: 10.1016/j.aninu.2018.02.003
38. Chen YP, Gu YF, Zhao HR, Zhou YM. Dietary squalene supplementation alleviates diquat-induced oxidative stress and liver damage of broiler chickens. Poult Sci. (2021) 100:100919. doi: 10.1016/j.psj.2020.12.017
39. Yen GC, Duh PD, Lin CW. Effects of resveratrol and 4-hexylresorcinol on hydrogen peroxide-induced oxidative DNA damage in human lymphocytes. Free Radic Res Commun. (2003) 37:509–14. doi: 10.1080/1071576031000083099
40. Zhu W, Chen SF, Li ZL, Zhao XH, Li WX, Sun YS, et al. Effects and mechanisms of resveratrol on the amelioration of oxidative stress and hepatic steatosis in KKAy mice. Nutr Metab. (2014) 11:35. doi: 10.1186/1743-7075-11-35
41. Meng QW, Guo T, Li GQ, Sun SS, He SQ, Cheng BJ, et al. Dietary resveratrol improves antioxidant status of sows and piglets and regulates antioxidant gene expression in placenta by Keap1-Nrf2 pathway and Sirt1. J Anim Sci Biotechnol. (2018) 9:639–51. doi: 10.1186/s40104-018-0248-y
42. Zhang C, Zhao XH, Yang L, Chen XY, Jiang RS, Jin SH, et al. Resveratrol alleviates heat stress-induced impairment of intestinal morphology, microflora, and barrier integrity in broilers. Poult Sci. (2017) 96:4325–32. doi: 10.3382/ps/pex266
43. Liu LL, He JH, Xie HB, Yang YS, Zou Y. Resveratrol induces antioxidant and heat shock protein mRNA expression in response to heat stress in black-boned chickens. Poult Sci. (2014) 93:54–62. doi: 10.3382/ps.2013-03423
44. Cheng K, Song ZH, Li SM, Yan EF, Zhang H, Zhang LL, et al. Effects of resveratrol on intestinal oxidative status and inflammation in heat-stressed rats. J Therm Biol. (2019) 85:102415. doi: 10.1016/j.jtherbio.2019.102415
45. Cheng K, Yan EF, Song ZH, Li SM, Zhang H, Zhang LL, et al. Protective effect of resveratrol against hepatic damage induced by heat stress in a rat model is associated with the regulation of oxidative stress and inflammation. J Therm Biol. (2019) 82:70–5. doi: 10.1016/j.jtherbio.2019.03.012
46. Ido Y, Duranton A, Lan F, Weikel KA, Breton L, Ruderman NB. Resveratrol prevents oxidative stress-induced senescence and proliferative dysfunction by activating the ampk-foxo3 cascade in cultured primary human keratinocytes. PLoS ONE. (2015) 10:e0115341. doi: 10.1371/journal.pone.0115341
47. Zhuang Y, Wu HR, Wang XX, He JY, He SP, Yin YL. Resveratrol attenuates oxidative stress-induced intestinal barrier injury through PI3K/Akt-mediated Nrf2 signaling pathway. Oxid Med Cell Longev. (2019) 2019:7591840. doi: 10.1155/2019/7591840
48. Andueza A, García-Garzón A, Galarreta MR, Ansorena E, Iraburu MJ, López-Zabalza MJ, et al. Oxidation pathways underlying the pro-oxidant effects of apigenin. Free Radic Biol Med. (2015) 87:169–80. doi: 10.1016/j.freeradbiomed.2015.06.003
49. Guo H, Li M, Xu LJ. Apigetrin treatment attenuates LPS-induced acute otitis media though suppressing inflammation and oxidative stress. Biomed Pharmacother. (2019) 109:1978–87. doi: 10.1016/j.biopha.2018.07.022
50. Zare MFR, Rakhshan K, Aboutaleb N, Nikbakht F, Naderi N, Bakhshesh M, et al. Apigenin attenuates doxorubicin induced cardiotoxicity via reducing oxidative stress and apoptosis in male rats. Life Sci. (2019) 232:116623. doi: 10.1016/j.lfs.2019.116623
51. Xiao L, Cao W, Liu GM, Fang TT, Wu XJ, Jia G, et al. Arginine, N-carbamylglutamate, and glutamine exert protective effects against oxidative stress in rat intestine. Animal Nutrition. (2016) 2:242–8. doi: 10.1016/j.aninu.2016.04.005
52. Chen XL, Zeng ZY, Huang Z Q, Chen DW, He J, Chen H, et al. Effects of dietary resveratrol supplementation on immunity, antioxidative capacity and intestinal barrier function in weaning piglets. Anim Biotechnol. (2019) 32:240–5. doi: 10.1080/10495398.2019.1683022
53. Suzuki T, Motohashi H, Yamamoto M. Toward clinical application of the Keap1-Nrf2 pathway. Trends Pharmacol Sci. (2013) 34:340–6. doi: 10.1016/j.tips.2013.04.005
54. Escartin C, Won SJ, Malgorn C, Auregan G, Berman AE, Chen PC, et al. Nuclear factor erythroid 2-related factor 2 facilitates neuronal glutathione synthesis by upregulating neuronal excitatory amino acid transporter 3 expression. J Neurosci. (2011) 31:7392–401. doi: 10.1523/JNEUROSCI.6577-10.2011
55. Lau WL, Liu SM, Pahlevan S, Yuan J, Khazaeli M, Ni ZM, et al. Role of Nrf2 dysfunction in uremia-associated intestinal inflammation and epithelial barrier disruption. Dig Dis Sci. (2015) 60:1215–22. doi: 10.1007/s10620-014-3428-4
56. Hao LH, Cheng YZ, Su WF, Wang C, Lu ZQ, Jin ML, et al. Pediococcus pentosaceus ZJUAF-4 relieves oxidative stress and restores the gut microbiota in diquat-induced intestinal injury. Appl Microbiol Biotechnol. (2021) 105:1657–68. doi: 10.1007/s00253-021-11111-6
57. Liu L, Wu CM, Chen DW, Yu B, Huang ZQ, LuoYH, et al. Selenium-enriched yeast alleviates oxidative stress-induced intestinal mucosa disruption in weaned pigs. Oxid Med Cell Longev. (2020) 2020:5490743. doi: 10.1155/2020/5490743
58. Sun ZM, Guan P, Luo LF, Qin LY, Wang N, Zhao YS, et al. Resveratrol protects against CIH-induced myocardial injury by targeting Nrf2 and blocking NLRP3 inflammasome activation. Life Sci. (2020) 245:117362. doi: 10.1016/j.lfs.2020.117362
59. Zhou YF, Jin YC, Yu H, Shan AS, Shen JL, Zhou CH, et al. Resveratrol inhibits aflatoxin B1-induced oxidative stress and apoptosis in bovine mammary epithelial cells and is involved the Nrf2 signaling pathway. Toxicon. (2019) 164:10–5. doi: 10.1016/j.toxicon.2019.03.022
60. Xu XR, Li M, Chen WW, Yu HT, Yang Y, Hang L. Apigenin attenuates oxidative injury in ARPE-19 cells thorough activation of Nrf2 pathway. Oxid Med Cell Longev. (2016) 2016:4378461. doi: 10.1155/2016/4378461
61. Zhang BX, Wang J, Zhao GD, Lin M, Lang Y, Zhang DC, et al. Apigenin protects human melanocytes against oxidative damage by activation of the Nrf2 pathway. Cell Stress Chaperones. (2020) 25:277–85. doi: 10.1007/s12192-020-01071-7
62. Capaldo CT, Powell DN, Kalman D. Layered defense: how mucus and tight junctions seal the intestinal barrier. J Mol Med. (2017) 95:927–34. doi: 10.1007/s00109-017-1557-x
63. Jin L, Wang W, Degroote J, Noten NV, Yan H, Majdeddin M, et al. Mycotoxin binder improves growth rate in piglets associated with reduction of toll-like receptor-4 and increase of tight junction protein gene expression in gut mucosa. J Anim Sci Biotechnol. (2017) 8:80. doi: 10.1186/s40104-017-0210-4
64. Pan HH, Zhou XX, Ma YY, Pan WS, Zhao F, Yu MS, et al. Resveratrol alleviates intestinal mucosal barrier dysfunction in dextran sulfate sodium-induced colitis mice by enhancing autophagy. World J Gastroenterol. (2020) 26:4945–59. doi: 10.3748/wjg.v26.i33.4945
65. Liu JB, Zhang Y, Li Y, Yan HL, Zhang HF. L-Tryptophan Enhances intestinal integrity in diquat-challenged piglets associated with improvement of redox status and mitochondrial function. Animals. (2019) 9:266. doi: 10.3390/ani9050266
Keywords: resveratrol, apigenin, diquat, oxidative stress, mRNA expression, small intestine, pullets
Citation: Zhou N, Tian Y, Liu W, Tu B, Xu W, Gu T, Zou K and Lu L (2022) Protective Effects of Resveratrol and Apigenin Dietary Supplementation on Serum Antioxidative Parameters and mRNAs Expression in the Small Intestines of Diquat-Challenged Pullets. Front. Vet. Sci. 9:850769. doi: 10.3389/fvets.2022.850769
Received: 08 January 2022; Accepted: 29 April 2022;
Published: 30 May 2022.
Edited by:
Shourong Shi, Poultry Institute (CAAS), ChinaReviewed by:
Mohammad H. Abukhalil, Al-Hussein Bin Talal University, JordanRiaz Hussain, Islamia University of Bahawalpur, Pakistan
Copyright © 2022 Zhou, Tian, Liu, Tu, Xu, Gu, Zou and Lu. This is an open-access article distributed under the terms of the Creative Commons Attribution License (CC BY). The use, distribution or reproduction in other forums is permitted, provided the original author(s) and the copyright owner(s) are credited and that the original publication in this journal is cited, in accordance with accepted academic practice. No use, distribution or reproduction is permitted which does not comply with these terms.
*Correspondence: Kang Zou, a2FuZ3pvdUBuamF1LmVkdS5jbg==; Lizhi Lu, bHVsaXpoaWJveEAxNjMuY29t