- 1Guangdong Provincial Key Laboratory of Animal Nutrition Control, College of Animal Science, South China Agricultural University, Guangzhou, China
- 2College of Animal Science, Anhui Science and Technology University; Anhui Provincial Key Laboratory of Animal Nutritional Regulation and Health, Fengyang, China
- 3College of Animal Science and National Engineering Research Center for Breeding Swine Industry, South China Agricultural University, Guangzhou, China
Artemisia annua L., which is known for its antimalarial compound artemisinin, has commonly been used for its anti-inflammatory and antibacterial functions. Enzymatically treating Artemisia annua L. can improve its bioavailability. The purpose of this study was to investigate the effects of dietary enzymatically treated Artemisia annua L. (EA) supplementation in late gestation and lactation diets on sow performance, serum hormone, inflammatory cytokines, and immunoglobulin level of heat-stressed sows. A total of 135 multiparous sows (Large White × Landrace) on day 85 of gestation were selected and randomly distributed into 3 groups with 45 replicates per group. The control group was reared under standard conditions (temperature: 27.12 ± 0.18°C, THI (temperature-humidity index): 70.90 ± 0.80) and fed with basal diet. The heat stress (HS) and HS + EA groups were raised in heat-stressed conditions (temperature: 30.11 ± 0.16°C, THI: 72.70 ± 0.60) and fed with basal diets supplemented with 0 or 1.0 g/kg EA, respectively. This trial lasted for 50 consecutive days until day 21 of lactation. Compared with the control group, HS increased the concentrations of serum endotoxin and heat shock protein 70 (HSP-70), and inflammatory cytokines in serum, colostrum, and 14 day milk of sows. Meanwhile, the EA supplementation decreased levels of serum endotoxin, HSP-70, and inflammatory cytokines in both sows and offspring and increased serum triiodothyronine (T3) level and average daily feed intake (ADFI) of sows. In addition, EA significantly improved average daily gain (ADG) and altered intestinal morphology with an increased villus height in the duodenum and ileum of piglets. Collectively, EA supplementation at 1.0 g/kg in late gestation and lactation diets alleviated the adverse effects of HS, which were reflected by enhancing ADFI and decreasing endotoxin as well as inflammatory cytokine levels in the serum and colostrum of heat-stressed sows, while promoting ADG and gut development of their offspring.
Introduction
Heat stress (HS) has been reported to seriously affect the production performance (1), immune status (2), plasma hormone profiles (3), intestinal integrity and function (4), oxidative stress (5), and inflammation and heat shock response (6) of livestock, and is estimated to lose over US $450 million annually (7). In addition, global warming worsens the already serious problem of HS for the pig industry in tropical and subtropical regions around the world (8). Due to a lack of functional sweat glands and the existence of a thicker layer of subcutaneous adipose tissue that impedes radiant heat loss, sows are more susceptible to HS (9). HS normally reduces appetite and feed intake in sows which results in a negative energy balance and nutrient supply deficit for sows and impairs lactation performance further (9). Many detrimental effects of HS can be attributed to HS-induced intestinal barrier dysfunction (10). HS exposure directly impairs gut integrity and increases endotoxin concentration, which causes a severe immune inflammatory response and cytokine-induced febrile response in sows (11). The long-term effects of maternal HS exposure might induce intestinal dysfunction of offspring, especially gut permeability and barrier function (12).
Currently, supplementing animal diets with natural phytogenic additives, which can alleviate HS, have received extensive attention in consideration of the safety of animal-origin food (13–15). These extracts can be used as anti-inflammatory agents due to the ability of scavenging free radicals or alleviating inflammatory response (16, 17). Artemisia annua L. (A. annua) is a natural herb belonging to the genus artemisia of Compositae, famous for its antimalarial compound artemisinin (Qinghaosu) (18). Approximately 600 secondary metabolites have been identified and separated in A. annua, including sesquiterpenes, monoterpenes, flavonoids, and phenolic compounds. Among these compounds, artemisinin characterizes the biological action of A. annua (19). In addition, many previous studies have shown that A. annua powder or extract could enhance growth performance (20), anti-inflammation (21–23), redox status, and innate immunity in broilers and rats (24, 25). Nevertheless, the plant cytoderm restricts the release of phytochemicals inside the cytoplasm, which leads to inefficient nutritional ingredient utilization in natural herbs. Previous studies have shown that enzymatic hydrolysis technology has positive effects, such as: (i) promotion in the release and purification of biologically active substances in plant cells; (ii) shorter extraction time, less usage of solvents, and higher yield and quality of product extract than physical and chemical extraction; and (iii) improvement in absorption efficiency to the animal (26).
Our lab recently found that the dietary supplementation of enzymatically treated Artemisia annua L. (EA) at 1.0 g/kg greatly alleviated the oxidative stress in sows, and improved the antioxidant capacity (15). Until now, little information is available regarding the effect of EA on ameliorating the adverse effects caused by HS in sows and offspring. Therefore, we speculated that the EA supplementation in late gestation and lactation diets could ameliorate the adverse effects of HS on the lactation performance, serum hormone secretion, inflammation, and immunoglobulin transfer of multiparous sows and promote intestinal development of their preweaning offspring.
Materials and Methods
Preparation of EA
Artemisia annua was produced in China and harvested during the prosperous period. The dried whole plant (stems and leaves) of A. annua was pulverized and mixed thoroughly with the 0.6% compound enzymatic hydrolysate (50000 U/g cellulase: 30000 U/g pectinase = 4:1) at 55°C and a pH of 4.5 for 4 h. Following enzymatic hydrolysis, filtrate and residue were collected, dried (filtrate of enzymatic hydrolysis of A. annua for 3 h at 60°C; residue of enzymatic hydrolysis of A. annua for 5 h at 90°C), and pulverized separately, then mixed to obtain final products. The EA contained 45.02, 972.96, and 335.17 g/kg of CP (AOAC, 2005, method 991.20), DM (AOAC, 2005, method 926.12), and ether extract (AOAC, 2005, method 920.39), respectively. Total phenolic content and total flavonoid content were measured as previously described (27). In addition, the main active ingredients of EA include 1.68 ± 0.13 mg of rutin equivalents (RE)/g flavonoids, 4.77 ± 0.22 mg of gallic acid equivalents (GAE)/g phenols, 156.2 ± 0.20 mg/kg of arteannuinic acid, 62.0 ± 0.08 mg/kg of deoxyartemisinin, and 26.0 ± 0.06 mg/kg of artemisinin.
Animals and Experimental Design
Overall, 135 sows (Large White × Landrace) of similar parity and weight on day 85 of gestation (G85) were selected and randomly distributed into 3 treatment groups with an initial 45 sows per treatment. The three treatment groups were as follows: control (Con) group, in which sows were raised in normal conditions (temperature: 27.12 ± 0.18°C, THI: 70.90 ± 0.80) and fed the basal diet. The heat-stressed (HS) and HS + EA groups, in which sows were raised in heat-stressed conditions (temperature: 30.11 ± 0.16°C, THI: 72.70 ± 0.60), and fed basal diets supplemented with 0 or 1.0 g/kg EA, respectively. The trial lasted for 50 consecutive days until day 21 of lactation (L21). The ultimate numbers of sows for analysis were 40, 41, and 42 in the Con, HS, and HS + EA groups in the present trial, respectively. The numbers of sows which were abortive, diseased (refer to metritis, lameness, poor health, etc.), deceased, and returned to estrus were 1, 1, 0, and 3 in the Con group, 0, 0, 1, and 3 in the HS group, and 1, 0, 0, and 2 in the HS + EA group, respectively.
This trial was conducted in a subtropical city in Guangdong Province in South China (subtropical climate) from July to October 2018. Comfortable control rooms contained a pad curtain with cycling water on one end of a completely closed room and large fans on the wall of the opposite end, while HS rooms were conventional rearing rooms, which are in a semi-opened building. It is worth noting that the normal adaptation temperature of sows generally should be no more than 25°C (28). In this experiment, the temperature of the Con group was higher than the normal range, although it was 3°C lower than the HS group. However, during our experiment, we did not observe any abnormal behavior of sows under 27°C, which might be due to the possibility that sows in Guangdong have partially adapted to the surrounding environment.
Feeding and Management
The experimental basic diet is a corn-soybean diet that can meet the nutritional needs of pregnant sows and lactating sows (NRC 2012). The composition and nutritional levels of the basal diet are shown in Table 1. During the experiment, the temperature (T) and relative humidity (RH) were recorded hourly using an auto temperature and humidity recorder (W-series, Henan, China). The temperature-humidity index (THI) was calculated according to the formula of Wegner et al. (29).
From the day 85 to day 107 of gestation of the sows, every sow was housed in individual crates (2.10 × 0.6 m2) in the gestation facility, and the sows were allowed to drink freely and were fed twice a day (6:30 and 14:00). On day 108 of gestation, all sows were transferred into respective farrowing rooms, where all experimental settings were held constant during the gestational period. They had ad libitum access to their respective experimental diets, which were fed to them until weaning of their offspring on L21. One week after the piglets were born, they were assisted with creep feeding, and they were free to eat until L21. Sows were housed in individual farrowing crates (2.3 × 2.4 m2). Piglets were cross-fostered only within 48 h postpartum in each treatment group, and the number of litters was standardized to 10 ± 1.
Data and Sample Collection
Sow and Litter Performance
Feed intakes of sows were recorded during lactation. The estrus rate of sows was recorded 7 days post-weaning of offspring. Sow backfat thickness was measured at the P2 position (at the last rib and 66 mm away from spine) using a digital backfat indicator (Renco Lean-Meater®, Renco Corporation, Minneapolis, USA) on G85, G114, and L21, which were used to calculate the backfat loss of lactation. Within the first 24 h after farrowing, the litter size at birth (total, live, healthy, weak, and stillborn) was recorded in this study. Moreover, individual piglet body weight (BW) as well as litter size at weaning and individual piglet BW were recorded on L21 and shown in our published research (15). These data were used to calculate the average daily gain (ADG) and survival rate of piglets during lactation in this study.
Serum Sample of Sows and Piglets
Blood samples (~8 mL per sow) were collected by ear venipuncture from a random subset of sows (n = 10 per treatment) on G85, G114, L14, and L21. Sows were fed at 06:30, the blood samples were taken 3.5 h after morning feeding (around 10:00). The sows that had blood collected at each time point were the same. On L1 and L21, six piglets were randomly selected from each treatment. At the postprandial time, blood samples were collected via jugular venipuncture. Blood samples were collected into sterile vacuum tubes, followed by centrifuged at 3000 × g at 4°C for 10 min. The serum was separated, transferred into micro-tubes, and then frozen immediately at −80°C for subsequent biochemical analysis.
Colostrum and Milk Samples of Sows
Colostrum was collected from all functional teats within 12 h postpartum; 14 day milk was collected after intramuscular injection of 30 IU oxytocin on L14. Approximately 25 mL was collected at each time period, and samples were frozen immediately at −80°C until further analysis.
Small Intestine Samples of Piglets
Whole gastrointestinal tracts were rapidly removed after piglets were sacrificed by exsanguination. The small intestine was peeled from the mesentery and placed on a cold stainless-steel tray. Then, segments of ~2 cm in length of the middle of the duodenum, jejunum, and ileum were immediately isolated, respectively. The intestinal tissues were gently flushed with cold phosphate-buffered saline and then placed in 10% formalin solution for morphological examination.
Laboratory Analysis
Serum Hormone Profile, Endotoxin, and HSP-70 Concentrations
Serum hormone concentrations (corticosterone, insulin, glucagon, T3 (triiodothyronine), T4 (thyroxine), leptin, and prolactin), endotoxin, and heat stress protein 70 (HSP-70) were assayed as described in previous studies (30–32) using commercial radio-immunoassay kits (CUSABIO Biotech Company, Wuhan, China), then read on a spectrophotometer.
The Levels of Cytokines in Serum and Immunoglobulins in Colostrum and Milk
The levels of inflammatory cytokines [IL-1β (interleukin-1β), TNF-α (tumor necrosis factor-α), sCD14 (soluble cluster of differentiation 14), IL-6 (interleukin-6), IL-8 (interleukin-8), and IL-18 (interleukin-18)] in serum, and immunoglobulin G (IgG), IgA, and IgM levels in colostrum and 14 day milk of sows were analyzed as described by a previous method (33) using commercially available porcine ELISA kits (CUSABIO Biotech Company, Wuhan, China). These indices were measured with the corresponding assay kits according to the manufacturer's instructions.
Before analyzing inflammatory cytokines, lipids in colostrum and 14 day milk were removed following centrifugation at 4°C, 3000 × g for 25 min as described by Hu et al. (34). Somatic cell counts (SCC) were determined by a Fossomatic 5000 (Foss Electric, Hillerød, Denmark).
Intestinal Morphology
The collected duodenum, jejunum, and ileum segments were dehydrated, embedded in paraffin, then stained with hematoxylin as well as eosin according to the procedures described by Song et al. (35). Villus height and crypt depth were determined using an image processing and analysis system (Leica Imaging Systems Ltd, Cambridge, UK).
Statistical Analysis
Statistical analyses were conducted using one-way ANOVA in SPSS 17.0 software (SPSS, INC., Chicago, IL, USA), differences among groups were evaluated by Duncan's test. The individual sow and its litter were used as the experimental unit. Hormone and inflammatory cytokines in sow serum and milk were analyzed as repeated measures. The results were expressed as mean and its pooled standard errors. The significance of all data analysis was defined as P < 0.05.
Results
Lactation Performance of Sows
As shown in Table 2, higher ADG during lactation was observed in the HS + EA group compared to the HS group and Con group (P < 0.05). Compared with the Con group, HS decreased the average daily feed intake (ADFI); conversely, a higher ADFI was observed when the HS group was fed an EA-supplemented diet during lactation (P < 0.05). Sow backfat loss and the estrus rate over 7 days post-weaning and piglet survival rate did not differ among treatments (P > 0.05).
Hormone, Endotoxin, and HSP-70 Levels in Serum of Sows
As shown in Tables 3, 4, compared with the Con group, sows in the HS group had lower levels of T3 (L14, L21) and higher levels of endotoxin (G114, L14) and HSP-70 (G114) (P < 0.05). In contrast, dietary EA supplementation resulted in higher levels of T3 (L14, L21) and lower concentrations of endotoxin (G114, L14) and HSP-70 (G114) compared with the Con and HS groups (P < 0.05).
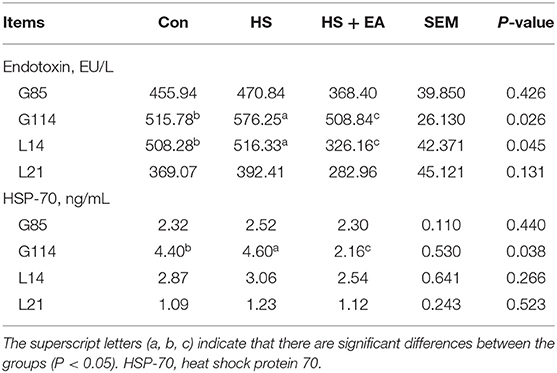
Table 4. Effects of dietary supplementation with EA on endotoxin and HSP-70 levels in the serum of sows.
Inflammatory Cytokines Levels in Serum, Colostrum, and 14 Day Milk
Compared with the Con group, the HS group had higher levels of TNF-α (G114) and IL-6 (G114, L14) in the serum of sows, as well as TNF-α (L1) and IL-6 (L1) levels in the serum of piglets (P < 0.05). Dietary EA supplementation resulted in lower levels of TNF-α and IL-6 levels compared with the Con and HS groups in the serum of sows and their offspring (P < 0.05; Tables 5, 6). Compared with the Con group, the HS group had higher levels of TNF-α, IL-6, and SCC in the colostrum of sows (P < 0.05). Dietary EA supplementation resulted in lower levels of TNF-α, IL-6, and SCC in the colostrum compared with the Con and HS groups (P < 0.05; Table 7).
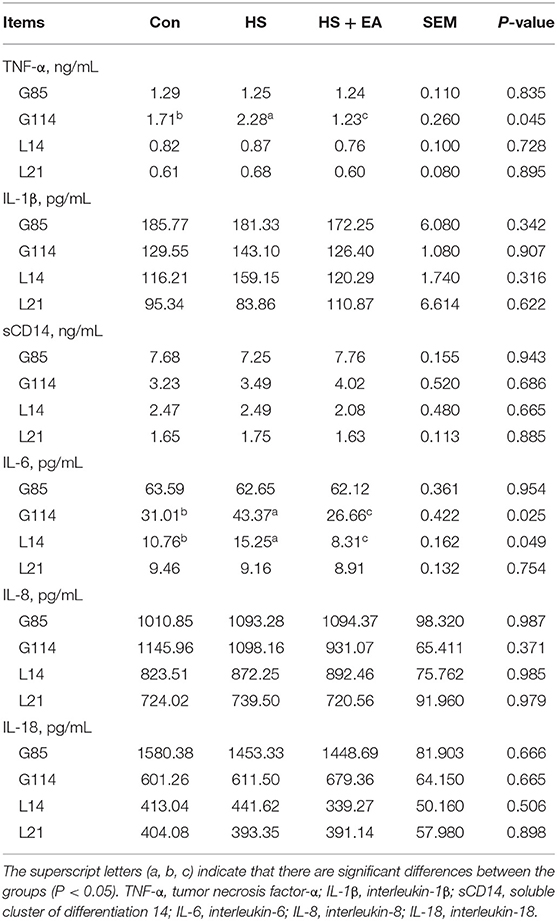
Table 5. Effects of dietary supplementation with EA on the inflammatory cytokine levels in the serum of sows.
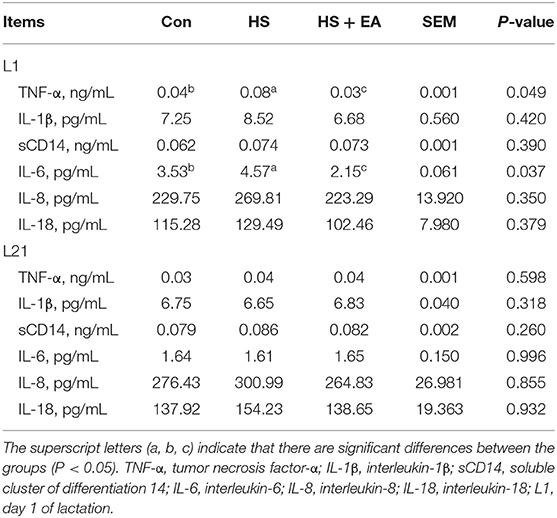
Table 6. Effects of maternal supplementation with EA on the inflammatory cytokine levels in the serum of piglets.
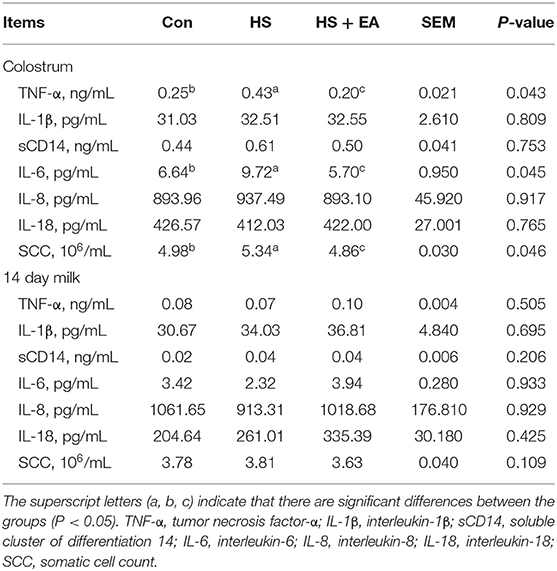
Table 7. Effects of dietary supplementation with EA on the inflammatory cytokine and SCC levels in the colostrum and 14 day milk of sows.
Immunoglobulin Levels in Colostrum, 14 Day Milk of Sows, and Serum of Nursing Piglets
As shown in Tables 8, 9. There were no treatment effects on IgA, IgG, and IgM levels in the colostrum, 14 day milk of sows, and serum of nursing piglets (P > 0.05).
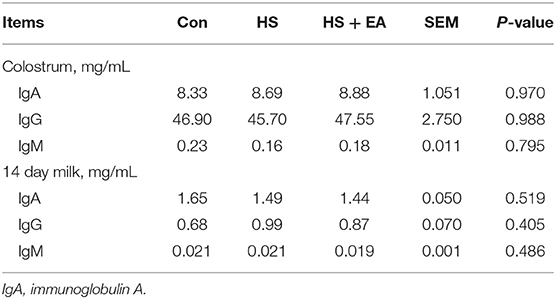
Table 8. Effects of dietary supplementation with EA on immunoglobulin levels in the colostrum and 14 day milk of sows.
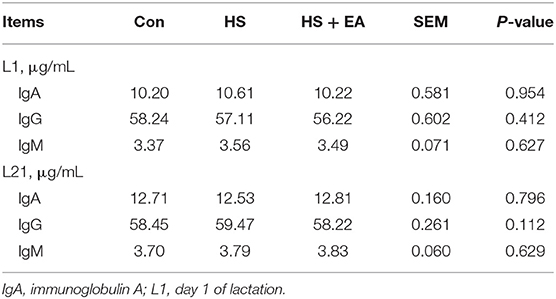
Table 9. Effects of maternal dietary supplementation with EA on immunoglobulin levels in the serum of nursing piglets.
Intestinal Morphology of Piglets
As shown in Table 10. Compared with the Con group, the villus height decreased in the duodenum at birth (L1) and in the ileum at weaning (L21) (P < 0.05) in the HS group. Compared with the Con and HS groups, EA supplementation increased the villus height in the duodenum at birth and in the ileum at weaning (P < 0.05).
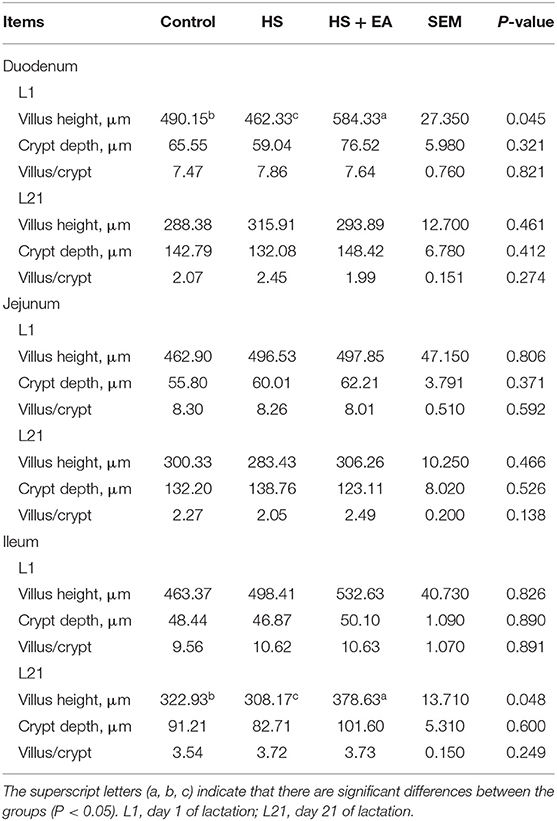
Table 10. Effects of maternal dietary supplementation with EA on small intestinal morphology of nursing piglets.
Discussion
HS exposure in later pregnancy induces oxidative stress (36), reduces digestive enzyme activities and immunity capability (37), causes inflammation and intestinal damage (38), and leads to an insufficient nutrient supply for sows, which have a series of detrimental effects on the reproductive and lactation performance as well as health condition of sows (39). Therefore, it is particularly important to find a suitable method to alleviate the adverse effects of HS on sows and their offspring.
When the ambient temperature exceeds the sow's critical temperature for evaporation, the sow is under HS (40). HS reduced the appetite and feed intake which impairs the compensatory capacity of sows (41) and sows usually consume less feed in order to reduce metabolic heat production (42). Disrupted physiological balance and reduced milk production and feed conversion efficiency of sows under HS have also been observed (43). To date, many previous studies have shown that diets supplemented with A. annua extract or A. annua leaf powder increased ADG and ADFI (14, 23, 44) and alleviated HS-induced body weight reduction and intestinal morphology impairment in broilers (19). Beyond this, emerging evidence also demonstrated that a diet directly supplemented with EA increased ADG and nutrient digestibility in weaned pigs (45, 46). Similar to this trial, the higher ADG in weaned piglets and ADFI in sows fed EA-supplemented diets was observed during lactation compared with the Con and HS groups. The crude protein, essential amino acids, minerals, vitamins, and flavonoids in EA delivered through the colostrum are important for growth and development of piglets, which is probably the reason for the enhancement of ADG (14, 45). The higher ADFI in the sows fed with supplemental EA might be due to the fact that artemisinin and phenolic compounds, including curcumin, resveratrol, and gallate could arouse appetite and reduce the heat from immuno-inflammatory responses.
A balanced physiological state is key for animal health. HS causes endocrine hormone increases in sows during pregnancy and lactation (3). T3 is mainly derived by deiodination of T4 which is known to stimulate lactation. HS could induce decreases in deiodinase activity to decrease T3 expression (47). In addition, published experimental research indicated that HS negatively affected thyroid status, including depression in pituitary-thyroid axis activity and peripheral metabolism of T3 and T4 through LPS-induced pro-inflammatory stimulus (48). In addition, HS injures the barrier of the intestine and induces the increment of endotoxins (i.e., LPS) from bacteria flow across the mesenteric-drained viscera. The transfer of endotoxins to the interior circulation contributes to systemic inflammation (49). A high content of HSP-70 is widely considered as a pointer of various environmental stress responses such as HS (50). Many previous studies have shown that HS reduced T3 concentration (51) and activity and increased endotoxin (33) and HSP-70 levels (4) in the blood of broilers and sows. In this study, HS reduced the T3 level in the serum of sows. Meanwhile, HS significantly increased the expressions of endotoxin and HSP-70 in the serum, which indicated that the HS model was established in sows in the current study. Our results were consistent with the findings reported in previous studies (4, 51). Whereas, EA supplementation effectively increased the T3 level and reduced the endotoxin level, which might be due to the fact that artemisinin and arteannuinic acid have antioxidant activity (24), bactericidal and antibacterial functions (52), and improve deiodinase activity. Thus, EA directly or indirectly alleviated the inflammatory response triggered by HS. The downregulation of HSP-70 level in heat-stressed sows following supplemental EA might be associated with the modulation of reduced endotoxin-induced pro-inflammatory cytokine expression in the present study.
The gastrointestinal tract is predominantly responsive to HS which destroys the intestinal mucosal structure (53). Subsequently, pathogens and toxins enter the damaged mucosal barrier, which stimulates the immune inflammatory response and leads to the flow of inflammatory cytokines into the colostrum and milk via blood circulation (54). The levels of inflammatory cytokines (such as IL-1β, IL-6, and TNF-α) in blood are considered to be an indicator to assess inflammation (23). HS upregulates IL-1β, IL-6, and TNF-α levels in the blood of pigs (55), which manifests as a serious proinflammatory stimulus. Song et al. (4, 56) reported that EA reduced proinflammatory cytokines and restored intestinal mucosal integrity caused by HS in broilers. Niu et al. (45) also showed that EA decreased the concentrations of IL-1β, IL-6, and TNF-α in the small intestine of weaned pigs. Accumulating evidence suggested that artemisinin and phenolic compounds (such as gallate, curcumin, resveratrol, and chlorogenic acids) in EA could exhibit a greater ability to prohibit the inflammatory response induced by lipopolysaccharide (LPS) (21, 57). Our recent studies have shown that EA extractive artemisinin inhibited LPS-induced activation of the mitogen-activated protein kinase (MAPK) and nuclear factor-κB (NF-κB) signaling pathways in porcine mammary epithelial cells (58). Our findings were consistent with the findings of previous studies (21, 56–58). The mechanism has been observed that sesquiterpenes (artemisinin and arteannuinic acid) as well as flavonoids (casticin and chrysosplenol D) from EA can attenuate the NF-κB/ nod-like receptor family pyrin domain containing 3 (NLRP3) pathways as well as toll-like receptor 4 (TLR4)/NF-κB and MAPK signaling pathways to further inhibit the expression of various inflammatory cytokines genes, including TNF-α and IL-1β (59, 60). In the present study, the addition of EA in sow diets effectively reduced the increase in inflammatory cytokine levels in the serum, colostrum, and milk of sows and piglets and further relieved the inflammation caused by HS. Hence, we speculated that EA attenuated the LPS-induced inflammatory pathways in the gut and mammary glands to alleviate inflammation in heat-stressed sows. Furthermore, the reduction resulting from EA in the colostrum might indicate the lower incidence of LPS-induced mastitis in sows during lactation (58).
The small intestine is not only the main place for the body to digest and absorb nutrients, but also the largest immune organ of the body (54). Oxidative and inflammatory stress brought by HS damages the intestinal villus structure, reduces villus height (33), increases intestinal permeability, and subsequently results in diarrhea and growth retardation (61). Small intestine mucosal villus height and crypt depth are important indicators for evaluating intestinal development (46). Intestinal villus height is positively correlated with the surface area in contact with the chyme, which is an indicator of the intestinal absorption capacity (62). Studies have shown that HS affected the structural integrity and barrier function of piglet intestines through maternal effect directly and colostrum intake indirectly (10, 61). In the current study, we investigated the vertically transmitted effect of maternal effect on offspring's small intestinal health. Maternal supplementation with 1.0 g/kg of EA effectively alleviated the damage of HS to the small intestine of offspring by increasing intestinal villus height. However, Niu et al. (46) reported that diet supplemented with 2.0 g/kg of EA (but not 1.0 g/kg of EA) decreased crypt depth and increased villus height and villus height to crypt depth ratio in both the jejunum and ileum of piglets. The different results between these two studies indicates the optimal dose of EA for weaning piglets and sows is controversial. Different from the direct effect of EA on the gut health of sows, EA could indirectly protect mucosal health in piglets by means of reducing oxidative and inflammatory stimulus caused by milk from sows (15). The above results suggested that the decreased oxidative and inflammatory stress in the colostrum and milk in this trial might be the reason why EA protected gut health in piglets.
Conclusion
Our present study demonstrated for the first time that dietary supplementation with enzymatically treated Artemisia annua L. at 1.0 g/kg exhibited beneficial effects on the lactation performance, serum hormone level, and inflammatory response of sows under HS and the gut health of their offspring (Figure 1). The results of this study provided a new perspective for alleviating the inflammatory response of sows, and at the same time supplied a new nutritional management strategy for alleviating the HS response in animal production.
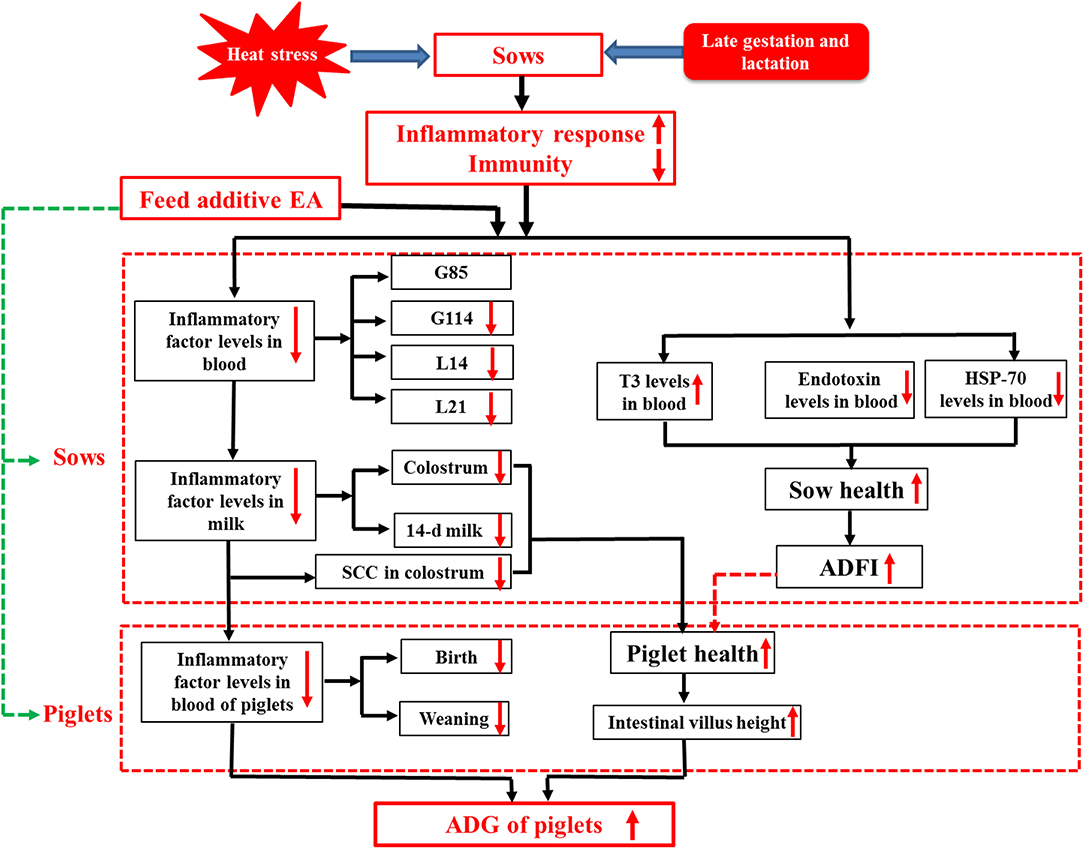
Figure 1. Dietary enzymatically treated Artemisia annua L. (EA) supplementation at 1.0 g/kg during late gestation and lactation increased ADFI, decreased endotoxin and inflammatory factor levels in the serum and colostrum of heat-stressed sows during lactation, and increased ADG and improved the gut development of their offspring.
Data Availability Statement
The original contributions presented in the study are included in the article/supplementary material, further inquiries can be directed to the corresponding authors.
Ethics Statement
The animal study was reviewed and approved by South China Agricultural University Animal Care and Use Committee (Guangzhou, China) (SCAU-AEC-2010-0416).
Author Contributions
LX, WZ, FC, SZ, and WG designed the experiments. WZ, LX, HZ, and ZT performed the experiments. WZ analyzed the data and wrote the manuscript. FC, MR, WG, and SZ conducted the final proofreading. All authors have read and agreed to the published version of the manuscript.
Funding
This study was financially supported by the National Key R&D Program of China (No. 2021YFD1300700), the National Natural Science Foundation of the P. R. of China (Nos. 31872364 and 31802067), Guangdong Basic and Applied Basic Research Foundation (No. 2021A1515010440), Science and Technology Program of Guangzhou (No. 202102020056), and Major Science and Technology Projects in Anhui Province (201903a06020002).
Conflict of Interest
The authors declare that the research was conducted in the absence of any commercial or financial relationships that could be construed as a potential conflict of interest.
Publisher's Note
All claims expressed in this article are solely those of the authors and do not necessarily represent those of their affiliated organizations, or those of the publisher, the editors and the reviewers. Any product that may be evaluated in this article, or claim that may be made by its manufacturer, is not guaranteed or endorsed by the publisher.
Acknowledgments
Special thanks for the Guangzhou Cohoo bio-tech Research & Development Center (Guangzhou, China) for their strong support.
References
1. Prunier A, Messias De Braganca M, Le Dividich J. Influence of high ambient temperature on performance of reproductive sows. Livest Prod Sci. (1997) 52:123–33. doi: 10.1016/S0301-6226(97)00137-1
2. Quinteiro-Filho WM, Ribeiro A, Ferraz-de-Paula V, Pinheiro ML, Sakai M, Sá LR, et al. Heat stress impairs performance parameters, induces intestinal injury, and decreases macrophage activity in broiler chickens. Poult Sci. (2010) 89:1905–14. doi: 10.3382/ps.2010-00812
3. Barb CR, Estienne MJ, Kraeling RR, Marple DN, Rampacek GB, Rahe CH, et al. Endocrine changes in sows exposed to elevated ambient temperature during lactation. Domest Anim Endocrin. (1991) 8:117–27. doi: 10.1016/0739-7240(91)90046-m
4. Song Z, Cheng K, Zhang L, Wang T. Dietary supplementation of enzymatically treated Artemisia annua could alleviate the intestinal inflammatory response in heat-stressed broilers. J Therm Biol. (2017) 69:184–90. doi: 10.1016/j.jtherbio.2017.07.015
5. Yi D, Hou Y, Tan L, Liao M, Xie J, Wang L, et al. N-acetylcysteine improves the growth performance and intestinal function in the heat-stressed broilers. Anim Feed Sci Tech. (2016) 220:83–92. doi: 10.1016/j.anifeedsci.2016.07.014
6. Siddiqui SH, Kang D, Park J, Khan M, Shim K. Chronic heat stress regulates the relation between heat shock protein and immunity in broiler small intestine. Sci Rep. (2020) 10:18872. doi: 10.1038/s41598-020-75885-x
7. St-Pierre NR, Co Ba Nov B, Schnitkey G. Economic losses from heat stress by US livestock industries1. J Dairy Sci. (2003) 86:E52–77. doi: 10.3168/jds.S0022-0302(03)74040-5
8. Gourdine JL, Bidanel JP, Noblet J, Renaudeau D. Effects of breed and season on performance of lactating sows in a tropical humid climate. J Anim Sci. (2006) 84:360–9. doi: 10.2527/2006.842360x
9. Zhang S, Johnson JS, Trottier NL. Effect of dietary near ideal amino acid profile on heat production of lactating sows exposed to thermal neutral and heat stress conditions. J Anim Sci Biotechno. (2020) 11:75. doi: 10.1186/s40104-020-00483-w
10. Baumgard LH, Rhoads RP Jr. Effects of heat stress on postabsorptive metabolism and energetics. Annu Rev Anim Biosci. (2013) 1:311–37. doi: 10.1146/annurev-animal-031412-103644
11. Lucy MC, Safranski TJ. Heat stress in pregnant sows: thermal responses and subsequent performance of sows and their offspring. Mol Reprod Dev. (2017) 84:946–56. doi: 10.1002/mrd.22844
12. Guo H, He J, Yang X, Zheng W, Yao W. Responses of intestinal morphology and function in offspring to heat stress in primiparous sows during late gestation. J Therm Biol. (2020) 89:102539. doi: 10.1016/j.jtherbio.2020.102539
13. Liu HW, Tong JM, Zhou DW. Utilization of Chinese herbal feed additives in animal production. Agr Sci China. (2011) 10:1262–72. doi: 10.1016/s1671-2927(11)60118-1
14. Brisibe EA, Umoren UE, Owai PU, Brisibe F. Dietary inclusion of dried Artemisia annua leaves for management of coccidiosis and growth enhancement in chickens. Afr J Biotechnol. (2008) 7:4083–92. doi: 10.5897/AJB08.490
15. Zhang W, Heng J, Kim SW, Chen F, Guan W. Dietary enzymatically-treated Artemisia annua L. supplementation could alleviate oxidative injury and improve reproductive performance of sows reared under high ambient temperature. J Therm Biol. (2020) 94:102751. doi: 10.1016/j.jtherbio.2020.102751
16. Ozer H, Sokmen M, Gulluce M, Adiguzel A, Sahin F, Sokmen A, et al. Chemical composition and antimicrobial and antioxidant activities of the essential oil and methanol extract of Hippomarathrum microcarpum (Bieb.) from Turkey. J Agric Food Chem. (2007) 55:937–42. doi: 10.1021/jf0624244
17. Zheng W, Wang SY. Antioxidant activity and phenolic compounds in selected herbs. J Agric Food Chem. (2001) 49:5165–70. doi: 10.1021/jf010697n
18. Tu YY. The discovery of artemisinin (qinghaosu) and gifts from Chinese medicine. Nat Med. (2011) 17:1217–20. doi: 10.1038/nm.2471
19. Fatima K, Abbas SR, Zia M, Sabir SM, Khan RT, Khan AA, et al. Induction of secondary metabolites on nanoparticles stress in callus culture of Artemisia annua L. Braz J Biol. (2021) 81:474–83. doi: 10.1590/1519-6984.232937
20. Almeida G, Horsted K, Thamsborg SM, Kyvsgaard NC, Ferreira J, Hermansen JE. Use of Artemisia annua as a natural coccidiostat in free-range broilers and its effects on infection dynamics and performance. Vet Parasitol. (2012) 186:178–87. doi: 10.1016/j.vetpar.2011.11.058
21. Qin DP, Li HB, Pang QQ, Huang YX, Pan DB, Su ZZ, et al. Structurally diverse sesquiterpenoids from the aerial parts of Artemisia annua (Qinghao) and their striking systemically anti-inflammatory activities. Bioorg Chem. (2020) 103:104221. doi: 10.1016/j.bioorg.2020.104221
22. Ferreira JFS, Luthria DL, Sasaki T, Heyerick A. Flavonoids from Artemisia annua L. as antioxidants and their potential synergism with artemisinin against malaria and cancer. Molecules. (2010) 15:3135–70. doi: 10.3390/molecules15053135
23. Wan XL, Song ZH, Niu Y, Cheng K, Zhang JF, Ahmad H, et al. Evaluation of enzymatically treated Artemisia annua L. on growth performance, meat quality, and oxidative stability of breast and thigh muscles in broilers. Poult Sci. (2017) 96:844–50. doi: 10.3382/ps/pew307
24. Wan XL, Niu Y, Zheng XC, Huang Q, Su WP, Zhang JF, et al. Antioxidant capacities of Artemisia annua L. leaves and enzymatically treated Artemisia annua L. in vitro and in broilers. Anim Feed Sci Tech. (2016) 221:27–34. doi: 10.1016/j.anifeedsci.2016.08.017
25. Hwang KE, Kim HW, Choi YS, Lee SY, Yeo EJ, Ham YK, et al. Evaluation of the antioxidant effect of ganghwayakssuk (Artemisia princeps Pamp.) extract alone and in combination with ascorbic acid in raw chicken patties. Polut Sci. (2013) 92:3244–50. doi: 10.3382/ps.2013-03274
26. Puri M, Sharma D, Barrow CJ. Enzyme-assisted extraction of bioactives from plants. Trends Biotechnol. (2012) 30:37–44. doi: 10.1016/j.tibtech.2011.06.014
27. Mahboubi A, Asgarpanah J, Sadaghiyani PN, Faizi M. Total phenolic and flavonoid content and antibacterial activity of Punica granatum L. var. pleniflora flowers (Golnar) against bacterial strains causing foodborne diseases. BMC Complem Altern M. (2015) 15:366. doi: 10.1186/s12906-015-0887-x
28. Muns R, Malmkvist J, Larsen MLV, Sorensen D, Pedersen LJ. High environmental temperature around farrowing induced heat stress in crated sows. J Anim Sci. (2016) 94:377–84. doi: 10.2527/jas.2015-9623.
29. Wegner K, Lambertz C, Das G, Reiner G, Gauly M. Climatic effects on sow fertility and piglet survival under influence of a moderate climate. Animal. (2014) 8:1526–33. doi: 10.1017/s1751731114001219
30. Kraeling RR, Rampacek GB, Cox NM, Kiser TE. Prolactin and luteinizing hormone secretion after bromocryptine (CB-154) treatment in lactating sows and ovariectomized gilts. J Anim Sci. (1982) 54:1212. doi: 10.2527/jas1982.5461212x
31. Koketsu Y, Dial GD, Pettigrew JE, Marsh WE, King VL. Influence of imposed feed intake patterns during lactation on reproductive performance and on circulating levels of glucose, insulin, and luteinizing hormone in primiparous sows. J Anim Sci. (1996) 74:1036. doi: 10.2527/1996.7451036x
32. Brendemuhl JH, Lewis AJ, Peo ER Jr. Effect of protein and energy intake by primiparous sows during lactation on sow and litter performance and sow serum thyroxine and urea concentrations. J Anim Sci. (1987) 64:1060. doi: 10.2527/jas1987.6441060x
33. Chen J, Zhang F, Guan W, Song H, Tian M, Cheng L, et al. Increasing selenium supply for heat-stressed or actively cooled sows improves piglet preweaning survival, colostrum and milk composition, as well as maternal selenium, antioxidant status and immunoglobulin transfer. J Trace Elem Med Biol. (2019) 52:89–99. doi: 10.1016/j.jtemb.2018.11.010
34. Hu CH, Xiao K, Luan ZS, Song J. Early weaning increases intestinal permeability, alters expression of cytokine and tight junction proteins, and activates mitogen-activated protein kinases in pigs. J Anim Sci. (2013) 91:1094–101. doi: 10.2527/jas.2012-5796
35. Song J, Jiao LF, Xiao K, Luan ZS, Hu CH, Shi B, et al. Cello-oligosaccharide ameliorates heat stress-induced impairment of intestinal microflora, morphology and barrier integrity in broilers. Anim Feed Sci Tech. (2013) 185:175–81. doi: 10.1016/j.anifeedsci.2013.08.001
36. Berchieri-Ronchi CB, Kim SW, Zhao Y, Correa CR, Yeum KJ, Ferreira AL. Oxidative stress status of highly prolific sows during gestation and lactation. Animal. (2011) 5:1774–9. doi: 10.1017/S1751731111000772
37. Qu H, Yan H, Lu H, Donkin SS, Ajuwon KM. Heat stress in pigs is accompanied by adipose tissue-specific responses that favor increased triglyceride storage. J Anim Sci. (2016) 94:1884–96. doi: 10.2527/jas.2015-0084
38. Black JL, Mullan BP, Lorschy ML, Giles LR. Lactation in the sow during heat stress. Anim Feed Sci Tech. (1993) 35:153–70. doi: 10.1016/0301-6226(93)90188-N
39. Zhu Y, Johnston LJ, Reese MH, Buchanan ES, Tallaksen JE, Hilbrands AH, et al. Effects of cooled floor pads combined with chilled drinking water on behavior and performance of lactating sows under heat stress. J Anim Sci. (2021) 99:66. doi: 10.1093/jas/skab066
40. Quiniou N, Noblet J. Influence of high ambient temperatures on performance of multiparous lactating sows. J Anim Sci. (1999) 77:2124–34. doi: 10.2527/1999.7782124x
41. Chen J, Zhang Y, You J, Song H, Zhang Y, Lv Y, et al. The effects of dietary supplementation of Saccharomyces Cerevisiae fermentation product during late pregnancy and lactation on sow productivity, colostrum and milk composition, and antioxidant status of sows in a subtropical climate. Front Vet Sci. (2020) 7:71. doi: 10.3389/fvets.2020.00071
42. Mack LA, Felver-Gant JN, Dennis RL, Cheng HW. Genetic variations alter production and behavioral responses following heat stress in 2 strains of laying hens. Poult Sci. (2013) 92:285–94. doi: 10.3382/ps.2012-02589
43. Renaudeau D, Collin A, Yahav S, Basilio VD, Gourdine JL, Collier RJ. Adaptation to hot climate and strategies to alleviate heat stress in livestock production. Animal. (2012) 6:707–28. doi: 10.1017/S1751731111002448
44. Wan X, Jiang L, Zhong H, Lu Y, Zhang L, Wang T. Effects of enzymatically treated Artemisia annua L. on growth performance and some blood parameters of broilers exposed to heat stress. Anim Sci J. (2017) 88:1239–46. doi: 10.1111/asj.12766
45. Niu Y, Zhao Y, He J, Yun Y, Shi Y, Zhang L, et al. Effect of diet supplemented with enzymatically treated Artemisia annua L. on intestinal digestive function and immunity in weaned pigs. Ital J Anim Sci. (2020) 19:1171–80. doi: 10.1080/1828051X.2020.1826364
46. Niu Y, He JT, Zhao YW, Gan ZD, Shen MM, Zhang LL, et al. Dietary enzymatically treated Artemisia annua L. supplementation improved growth performance and intestinal antioxidant capacity of weaned piglets. Livest Sci. (2020) 232:103937. doi: 10.1016/j.livsci.2020.103937
47. Todini L, Salimei E, Malfatti A, Brunetti VL, Fantuz F. Thyroid hormones in donkey blood and milk: correlations with milk yield and environmental temperatures. Ital J Anim Sci. (2015) 14:4089–99. doi: 10.4081/ijas.2015.4089
48. Kahl S, Elsasser TH, Rhoads RP, Collier RJ, Baumgard LH. Environmental heat stress modulates thyroid status and its response to repeated endotoxin challenge in steers. Domest Anim Endocrinol. (2015) 52:43–50. doi: 10.1016/j.domaniend.2015.02.001
49. Wang Z, Zhang L, Li Z, Yu Y, Yang L, Zhang P, et al. Alterations of endotoxin distribution across different biofluids and relevant inflammatory responses by supplementing L-theanine in dairy cows during heat stress. Anim Nutr. (2021) 7:1253–7. doi: 10.1016/j.aninu.2021.03.012
50. He J, Guo H, Zheng W, Xue Y, Zhao R, Yao W. Heat stress affects fecal microbial and metabolic alterations of primiparous sows during late gestation. J Anim Sci Biotechnol. (2019) 10:84. doi: 10.1186/s40104-019-0391-0
51. Messias de Bragan ca M, Mounier AM, Prunier A. Does feed restriction mimic the effects of increased ambient temperature in lactating sows? J Anim Sci. (1998) 76:2017–24. doi: 10.2527/1998.7682017x
52. Engberg RM, Grevsen K, Ivarsen E, Fretté X, Christensen LP, Højberg O, et al. The effect of Artemisia annua on broiler performance, on intestinal microbiota and on the course of a Clostridium perfringens infection applying a necrotic enteritis disease model. Avian Pathol. (2012) 41:369–76. doi: 10.1080/03079457.2012.696185
53. He J, Zheng W, Tao C, Guo H, Xue Y, Zhao R, et al. Heat stress during late gestation disrupts maternal microbial transmission with altered offspring's gut microbial colonization and serum metabolites in a pig model. Environ Pollut. (2020) 266:115111. doi: 10.1016/j.envpol.2020.115111
54. Pearce SC, Sanz Fernandez MV, Torrison J, Wilson ME, Baumgard LH, Gabler NK. Dietary organic zinc attenuates heat stress-induced changes in pig intestinal integrity and metabolism. J Anim Sci. (2015) 93:4702–13. doi: 10.2527/jas.2015-9018
55. Ju XH, Xu HJ, Yong YH, An LL, Jiao PR, Liao M. Heat stress upregulation of Toll-like receptors 2/4 and acute inflammatory cytokines in peripheral blood mononuclear cell (PBMC) of Bama miniature pigs: an in vivo and in vitro study. Animal. (2014) 8:1462–8. doi: 10.1017/S1751731114001268
56. Song ZH, Cheng K, Zheng XC, Ahmad H, Zhang LL, Wang T. Effects of dietary supplementation with enzymatically treated Artemisia annua on growth performance, intestinal morphology, digestive enzyme activities, immunity, and antioxidant capacity of heat-stressed broilers. Poult Sci. (2018) 97:430–7. doi: 10.3382/ps/pex312
57. Abate G, Zhang L, Pucci M, Morbini G, Mac Sweeney E, Maccarinelli G, et al. Phytochemical analysis and anti-inflammatory activity of different ethanolic phyto-extracts of Artemisia annua L. Biomolecules. (2021) 11:975. doi: 10.3390/biom11070975
58. Zhang W, Xiong L, Chen J, Tian Z, Liu J, Chen F, et al. Artemisinin protects porcine mammary epithelial cells against lipopolysaccharide-induced inflammatory injury by regulating the NF-κB and MAPK signaling pathways. Animals. (2021) 11:1528. doi: 10.3390/ani11061528
59. Jiang Y, Du H, Liu X, Fu X, Li X, Cao Q. Artemisinin alleviates atherosclerotic lesion by reducing macrophage inflammation via regulation of AMPK/NF-κB/NLRP3 inflammasomes pathway. J Drug Target. (2020) 28:70–9. doi: 10.1080/1061186X.2019.1616296
60. Li YJ, Guo Y, Yang Q, Weng XG, Yang L, Wang YJ, et al. Flavonoids casticin and chrysosplenol D from Artemisia annua L. inhibit inflammation in vitro and in vivo. Toxicol Appl Pharmacol. (2015) 286:151–8. doi: 10.1016/j.taap.2015.04.005
61. Pearce SC, Mani V, Boddicker RL, Johnson JS, Weber TE, Ross JW, et al. Heat stress reduces barrier function and alters intestinal metabolism in growing pigs. J Anim Sci. (2012) 90 (Suppl. 4):257–9. doi: 10.2527/jas.52339
Keywords: Artemisia annua L., heat stress, hormone level, inflammatory factor, intestinal morphology
Citation: Xiong L, Zhang W, Zhao H, Tian Z, Ren M, Chen F, Guan W and Zhang S (2022) Dietary Supplementation of Enzymatically Treated Artemisia annua L. Improves Lactation Performance, Alleviates Inflammatory Response of Sows Reared Under Heat Stress, and Promotes Gut Development in Preweaning Offspring. Front. Vet. Sci. 9:843673. doi: 10.3389/fvets.2022.843673
Received: 26 December 2021; Accepted: 16 February 2022;
Published: 25 March 2022.
Edited by:
Zongyong Jiang, Guangdong Academy of Agricultural Sciences, ChinaReviewed by:
Shenfei Long, China Agricultural University, ChinaArtabandhu Sahoo, Indian Council of Agricultural Research (ICAR), India
Copyright © 2022 Xiong, Zhang, Zhao, Tian, Ren, Chen, Guan and Zhang. This is an open-access article distributed under the terms of the Creative Commons Attribution License (CC BY). The use, distribution or reproduction in other forums is permitted, provided the original author(s) and the copyright owner(s) are credited and that the original publication in this journal is cited, in accordance with accepted academic practice. No use, distribution or reproduction is permitted which does not comply with these terms.
*Correspondence: WuTai Guan, d3V0YWlndWFuMTk2M0AxNjMuY29t; ShiHai Zhang, emhhbmdzaGloYWlAc2NhdS5lZHUuY24=