- 1Department of Animal Biosciences, University of Guelph, Guelph, ON, Canada
- 2Department of Food Science, University of Guelph, Guelph, ON, Canada
- 3Hybrid Turkeys, Suite C, Kitchener, ON, Canada
- 4School of Veterinary Science, University of Queensland, Gatton, QLD, Australia
- 5Vetsuisse Faculty, Institute of Genetics, University of Bern, Bern, Switzerland
Wing flapping and body movement can occur during the slaughter of poultry. Wing movement and flapping are driven primarily by the breast muscles (Pectoralis major and minor), and this muscle activity may have implications for meat quality. The objective of this study was to evaluate turkey post mortem activity during slaughter at a commercial poultry processing plant. Post mortem activity (during bleeding) was scored on 5,441 male turkeys, from six different genetic lines, using a 1–4 scale from none to severe wing flapping. Meat quality was measured on these birds in terms of pH (initial, ultimate, delta or change), color (L*, a*, b*), and physiochemical traits (drip loss, cooking loss, shear force). Linear mixed models were used to analyze the effect of activity (score 1–4), genetic line (A–F), and season (summer vs. autumn) on the nine meat quality traits. Post mortem activity influenced a*, drip loss, and shear force although the magnitude of the effects was small. There was an effect (P < 0.05) of genetic line on all the meat quality traits except for L*, cooking loss, and shear force. In general, larger, faster-growing lines had higher pH, but the relationship between the lines for the other traits is not as clear. Season affected all the meat quality traits, except for pHdelta, with meat having a higher pH, L*, b*, drip loss, cooking loss, and shear force in the summer. This study provides an exploratory assessment of post mortem activity in turkeys and identifies meat quality traits which are most affected while also accounting for the effects of genetic line and season. Although identified effect sizes are small, the cumulative effect on turkey meat quality may be more substantial.
Introduction
During slaughter, electrical stunning is a common practice in poultry processing plants to produce an instantaneous unconsciousness before bleeding, which may or may not be reversible, as part of humane slaughter practices (1, 2). After stunning, the bird is bled by severing vessels of the neck which stops the supply of oxygen to the brain and results in death (1). Muscle activity can occur during slaughter, both pre- and post-mortem, which has consequences for the carcass and meat quality. Muscle activity, usually observed as wing flapping, can occur in both under-stunned and adequately stunned birds (3, 4). This is an issue of particular importance in turkey flocks, since variability in bird size or body weight may cause birds to be stunned unevenly. When birds are not stunned sufficiently to induce irreversible unconsciousness they may be more likely to flap or move during the slaughter process (4). Even in adequately stunned broiler chickens (leading to cardiac arrest), wing flapping has been shown to occur in 50% of birds, likely due to cardiac fibrillations during stunning (3).
This muscle activity shown during wing flapping can cause carcass damage and negatively affect meat quality, which reduces the value of the carcass (5, 6). The most common defects associated with these convulsions are hemorrhaging, blood spots, broken bones, and red wing tips (7). Since wing flapping involves use of the breast muscles, birds that flap during slaughter may experience changes in the acid/base balance and quality of their breast meat compared to immobile birds. On a physiological level, wing flapping increases plasma corticosterone, creatine kinase, lactate dehydrogenase, AMPK-activated protein kinase, acetyl-CoA carboxylase phosphorylation in broilers (8). This results in rapid glycolysis and accumulation of lactic acid, which rapidly reduces meat pH (8). Rapid drops in meat pH soon after slaughter, in combination with a warm carcass, is implicated in a number of meat quality defects. This is because pH is tightly linked to color, water-holding capacity, and other sensory properties (9, 10). In this case, meat is usually more red, lighter in color, with higher moisture loss and this has negative implications for value and consumer acceptance (8, 11, 12). However, most studies assessing muscle activity and meat quality are concerned with pre-slaughter movement.
Like pre-slaughter muscle activity, convulsions during bleeding are believed to accelerate the use of adenosine triphosphate (ATP) in the muscle and this increases the rate of pH decline (13). This effect has been shown in turkeys which were either restrained or allowed to struggle freely during exsanguination, and although struggling birds had significantly shorter rigor times, there was no apparent effect on cooking loss or shear force (13). In broilers, the impact of muscle activity during bleeding on carcass damage has been studied. While there was no discernable effect of post mortem activity on red wing tips, number of broken bones or on meat quality measures (e.g., pH, L*, b*, cook yield, shear force), breast meat redness (a*) was higher in decapitated birds that had higher activity (e.g., wing flapping) than conventionally killed birds with lower activity after slaughter (5).
While muscle activity (pre- or post-slaughter) may affect turkey meat quality, there are other important factors to consider, such as the effect of the environment and genetics. The effect of environmental conditions, such as ambient temperature, are well-documented on poultry meat quality (14, 15). In particular, high ambient temperatures can accelerate pH decline in the meat post mortem which typically reduces water-holding capacity and degrades meat pigment (6, 16). For this reason, several studies have found that certain meat quality attributes (e.g., color, pH, water-holding capacity) can be affected depending on the season (i.e., summer vs. winter) the birds were processed (17, 18). Given the distinct seasonal changes that occur in Canada, it is reasonable to assume that this variable should be accounted for when data collection spans many months. Aside from environmental considerations, different poultry genotypes differ in their meat quality attributes which represents another source of variation that should be accounted for in studies containing multiple genetic lines (12, 19–22). Additionally, chickens with different genotypes were shown to behave differently while shackled, which could influence their post mortem activity levels (23). For these reasons, it has been suggested for future research to investigate the influence of genotype and slaughter conditions on turkey meat quality (22).
Several older studies have considered pre-slaughter (24, 25) and post-mortem (13) activity on meat quality in turkeys. However, the prevalence of post mortem muscle activity in commercial turkey processing plants has not often been reported in modern turkey lines and it is still unclear how different levels of post mortem activity may affect meat quality. The primary objectives of this study were (a) to assess the level of post mortem muscle activity in turkeys during conventional slaughter procedures, (b) determine if varying levels of muscle activity influence meat quality traits, and (c) evaluate the effects of season and genetic line.
Materials and Methods
All protocols complied with the guidelines of the Canadian Council on Animal Care and were approved by the University of Guelph Animal Care Committee (AUP 3782).
Animals
Data was collected from 5,441 male turkeys from six different genetic lines (lines A–F), from June to November 2019. The turkeys were raised under identical housing and management conditions at two farms and transported (115–130 km) to a commercial poultry processing facility in Ontario, Canada. Fasting, transport, and lairage conditions were consistent across the genetic lines. Lines A and B were dam-line birds that were selected for body weight and reproductive traits. Line C was a dam line selected for reproductive traits. Line D was a small, slow-growing line. Lines E and F were sire lines selected predominately for body weight, meat yield, and feed efficiency. Birds were slaughtered between 20 and 24 weeks of age across all genetic lines.
During processing, the birds were electrically stunned and then exsanguinated. Stunning voltage was based on average liveweight for birds <9 kg (24–28 V), 9–13 kg (28–32 V), and >13 kg (32–36 V). Birds were then scalded, defeathered, and eviscerated before moving to a water chiller (2 h) and kept refrigerated for 24 h (4°C) before deboning and further meat quality measurements were collected.
Post Mortem Activity
Videos of the birds on the slaughter line were recorded (Hero 5, GoPro, San Mateo, CA, USA). Activity was scored from these videos by the same observer using a four-category scoring system adapted from McNeal et al. (5). Activity scores (Table 1) were determined for each bird once they reached the same point on the slaughter line (post-exsanguination, immediately before scalding).
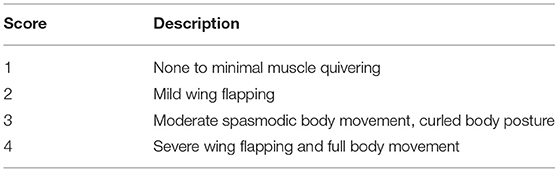
Table 1. Scoring scheme for post mortem carcass activity in turkeys; adapted from McNeal et al. (5).
Intra-observer reliability was calculated to ensure reliable consistent scores. The observer scored videos from five different slaughter days (~500 birds/day) in two separate sessions which were several days apart. Spearman correlations, simple and weighted kappa statistics were calculated to assess reliability of the scores between the two sessions. Kappa values were interpreted following the suggested arbitrary classifications where κ ≤ 0.20 is “poor,” 0.21 ≤ κ ≤ 0.40 is “fair,” 0.41 ≤ κ ≤ 0.60 is “moderate,” 0.61 ≤ κ ≤ 0.80 is “substantial,” and κ > 0.80 is “good” (26, 27).
Meat Quality Measurements
Measurements were obtained during processing at the slaughter line and after chilling. Due to the nature of the study and capacity of the abattoir, meat quality measurements were only collected on a proportion of the birds that were scored for post mortem activity (N = 817–895 depending on the trait). Measurements included pH, color, and physiochemical characteristics which are explained in more detail in the following section. The initial pH of breast meat samples was taken at 45 min post mortem, from the cranial portion of the breast filet (pHinitial, Portable pH meter, Hanna Instruments, Woonsocket, RI, USA). Carcasses were then water chilled (2–5°C) for ~2 h followed by storage in a commercial chiller at 4°C for 24 h before recording the other meat quality measurements.
The ultimate pH measurement was taken from the Pectoralis major (breast filet) at 24 hr post mortem (pHultimate). The pHultimate was subtracted from the pHinitial to calculate the difference between the two time points (pHdelta). Trichromatic coordinates [L*, a*, and b*, (28)] were measured using D50 illumination on the dorsal side of the deboned breast muscle (Nix Pro Colorimeter, Hamilton, ON, CA).
The physiochemical traits, drip loss (%), cooking loss (%), and shear force (N), were also analyzed. In brief, drip loss was determined by placing a 13 ± 1 g Pectoralis major sample inside a drip loss collection tube and measuring the initial and 72 h weight of the sample after refrigerated storage. For cooking loss and shear force, 200 g samples were cut from the breast muscle at 24 h post mortem and frozen at −20°C until they were defrosted and heated to an internal temperature of 72°F (determined via a thermocouple) while wrapped in aluminum foil to prevent crust formation. Cooking loss was calculated as the percent difference between the initial weight of the raw sample and final weight after cooking. To measure shear force, the cooked samples were wrapped and refrigerated for 24 h, after which they were allowed to reach room temperature. Shear force was measured using the Meullenet-Owens Razor Shear (MORS) blade method (29) with a texture analyser (TA-XT2, Texture Technologies Corp., Hamilton, MA, USA) using the cooked sample with an original dimension (raw samples) of 7.5 × 4.5 × 4.5 cm. Each sample was sheared at six locations on the sample surface (perpendicular to the muscle fiber direction) and then averaged.
Statistical Analyses
Linear mixed models were used to analyze the effect of post mortem activity on turkey meat quality. The models for the nine meat quality traits (pHinitial, pHultimate, pHdelta, L*, a*, b*, drip loss, cooking loss, and shear force) included the fixed effects of activity (scores 1–4), genetic line (A–F), and season (summer or autumn). To prevent overfitting of the model and provide insight into meat quality differences between different seasons, the slaughter date (N = 28) of the birds was categorized into seasons. Slaughter dates in June, July, and August were categorized as “Summer” (N = 3,897) and dates in September, October, and November (N = 1,545) were categorized as “Autumn.” Interactions between fixed effects were not considered due to the limited data available for possible two-way and three-way interactions.
All traits were modeled using a Gaussian distribution, except for drip loss and cooking loss for which a lognormal distribution and the back-transformed least-square means (LSmeans) presented. The α level for determination of significance was 0.05, and tendencies are reported between 0.05 and 0.10. All analyses were performed using SAS (Studio, version 9.4., SAS Institute Inc., Cary, NC, USA).
Results
Post Mortem Activity Scores
For intra-observer reliability, the Spearman correlation between the activity scores for the two sessions was 0.83. The simple and weighted kappa values were 0.80 ± 0.039 and 0.81 ± 0.039, respectively, indicating good reliability. The activity of most birds across all seasons and genetic lines was scored as 1 (86.9%, none to mild, Table 2). Scores 2, 3, and 4 made up 3.8, 7.2, and 2.0% of the birds, respectively.
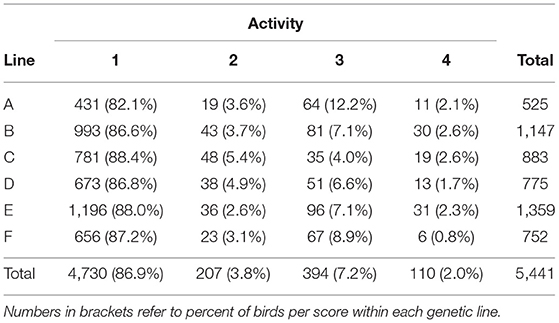
Table 2. Number of birds (NBirds) with each post-mortem activity score (1, none to minimal; 2, mild; 3, moderate; 4, severe) for male turkeys from six genetic lines (A–F).
Effect of Post Mortem Activity on Meat Quality Traits
There was an effect of post mortem activity on a*, drip loss, and shear force (P < 0.05), however no effect of post mortem activity was detected for the remaining color measures (L* and b*), pH, and physiochemical meat quality traits (P > 0.05, Table 3).
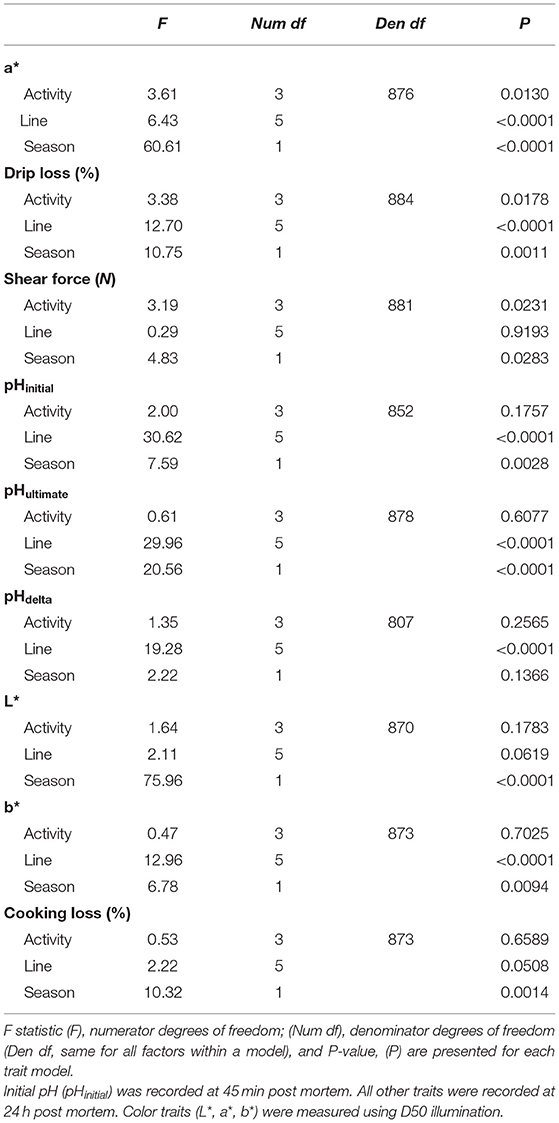
Table 3. Linear models for the effect of post mortem activity (score 1–4), genetic line (A–F), and season (summer vs. autumn) on turkey meat quality traits (NBirds= 817–895 depending on trait).
The studied pH traits (pHinitial, pHultimate, and pHdelta) were unaffected by post mortem activity (P > 0.05). The pHinitial of the meat from score 4 birds was lower than the other scores (e.g., −0.15, score 1 vs. score 4), however this was not significantly different as shown in Table 4.
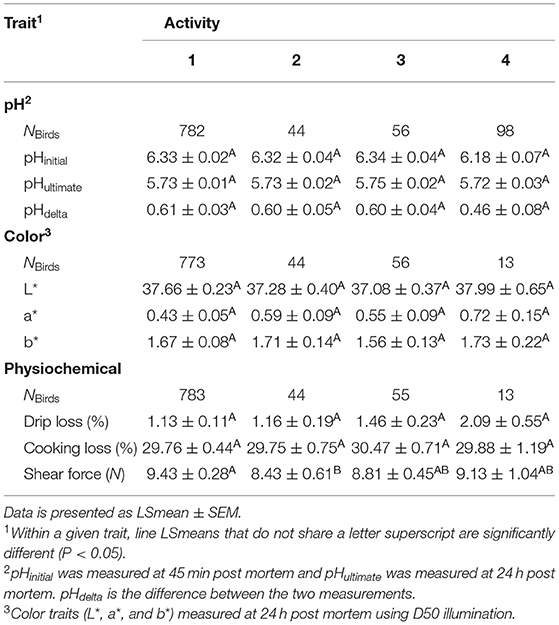
Table 4. Differences in meat quality attributes between turkeys (NBirds= 817–895 depending on the trait) with different post mortem activity scores 1–4.
Of the color traits (L*, a*, b*), a* was affected by post mortem activity. While the results from the ANOVA for the linear model for a* indicated that there was an effect of activity (F3, 876 = 3.61, P = 0.0130, Table 3), the adjusted P-values for the ad-hoc pairwise comparisons between the scores were not significant (P>0.05). Birds with an activity score >1 (none-minimal activity, Table 1) had lower a* values compared to the other scores (Table 4). As the activity score increased, the a* value increased by 32% (score 2), 26% (score 3), 51% (score 4) from score 1.
Within the physiochemical traits, both drip loss and shear force were affected by post mortem activity (P < 0.05). Shear force was affected by activity (F3, 881 = 3.19, P = 0.0231). Birds with score 1 produced meat with higher shear force compared to score 2 (t3, 881 = 2.63, P = 0.0425) but all other comparisons were not different (P > 0.05). Shear force was ~11% higher in meat from score 1 birds compared to score 2 (Table 4). For drip loss, the adjusted P-values for the comparisons indicated that the scores were not significantly different from one another (P > 0.05). However, birds with activity score 4 tended to have higher drip loss (~1%) than birds with score 1 (t884 = −2.49, P = 0.0618, Table 4).
Effect of Genetic Line on Meat Quality Traits
There was an effect (P < 0.05) of genetic line on all the meat quality traits except for L*, cooking loss, and shear force (Table 3). Genetic line influenced all the studied pH traits (Table 5). Lines E and F demonstrated higher pHinitial compared to all other lines (P < 0.05), except for line B. Line A had lower pHultimate compared to lines C, E, and F. The largest difference in pHultimate was observed between line A and line F (+0.14, t5, 878 = −3.32, P = 0.0122). Line E also demonstrated a higher pHultimate compared to line C (+0.04, t5, 878 = −4.97, P < 0.0001). For the difference between pHinitial and pHultimate (pHdelta), line E showed a greater pHdelta compared to line A (+0.15, t5, 807 = −5.45, P < 0.0001), line C (+0.19, t5, 807 = −9.26, P < 0.0001), and line D (+0.32, t5, 807 = −3.30, P = 0.0129). There was also a tendency for birds from line F to have a greater pHdelta compared to line D (+0.37, t5, 807 = −2.64, P = 0.0883).
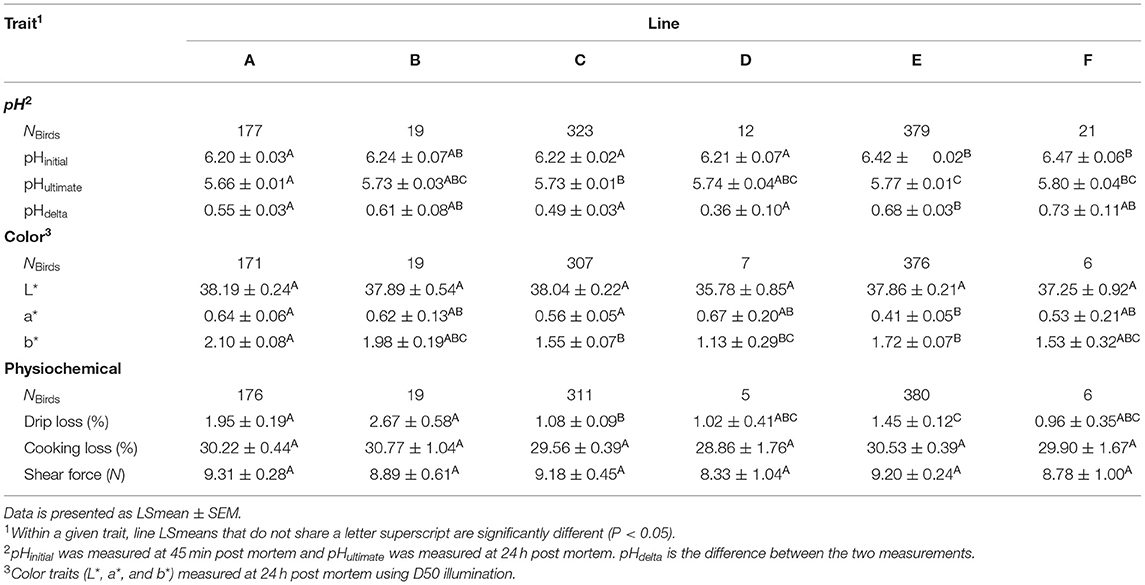
Table 5. Differences in meat quality attributes between six genetic lines (A–F) of turkeys (NBirds= 817–895 depending on trait).
There was a tendency for L* to be influenced by genetic line at the factor level (F5, 870 = 1.64, P = 0.0619), although the adjusted P-values from the pairwise comparisons revealed no differences between the lines at P > 0.05 (Table 5). For a*, line E had lower a* values than lines A (t5, 876 = 5.02, P < 0.0001) and C (t5, 876 = 4.02, P = 0.0009). There were also some differences in b* between the lines. Line A had a higher b* value than lines C (+0.55, t5, 873 = 7.60, P < 0.0001), D (+0.97, t5, 873 = 3.34, P = 0.0114), and E (+0.38, t5, 873 = 5.41, P < 0.0001). Additionally, line C had a lower b* value than line E (−0.17, t5, 873 = −2.73, P = 0.0406), although the magnitude of the difference was not as large.
There was an effect of genetic line on the amount of drip loss (F5, 884 = 12.70, P < 0.0001), but not cooking loss (F5, 873 = 2.22, P = 0.0508) or shear force (F5, 881 = 0.29, P = 0.9193, Table 5). Lines A and B had the highest mean amount of drip loss (1.95 ± 0.188% and 2.67 ± 0.583%, respectively). Line A had greater drip loss than line C (+0.87%, t5, 884 = 7.04, P < 0.0001) and line E (+0.50%, t5, 884 = 3.67, P = 0.0035). Line B also had greater drip loss than line C (+1.59%, t5, 884 = 4.33, P = 0.0002) and line E (+1.22%, t5, 884 = 2.94, P = 0.0390). Lastly, line C had lower drip loss compared to line E (−0.37%, t5, 884 = −4.33, P = 0.0002). Although line F had numerically the lowest drip loss, there was no statistical difference between the other lines (P > 0.05), possibly due to the relatively large standard error. The mean cooking loss (30.0%) was consistent between the six lines with a standard deviation of 0.70%. The same was also observed for the mean shear force (9.0 N) of the lines which had a standard deviation of 0.36 N.
Effect of Season on Meat Quality Traits
Season (summer vs. autumn) affected all the studied meat quality traits except for pHdelta (Table 3). In general, meat pH (pHinitial and pHultimate) was higher during the summer season (Table 6). Although for both pHinitial and pHultimate, the magnitude of the difference between the LSmeans is small (pHinitial: +0.05, t1, 861 = −3.00, P = 0.0028; pHultimate: +0.03, t1, 861 = −4.53, P < 0.0001). In the summer, meat had greater mean L* (+1.39, t1, 870 = −8.72, P < 0.001), a* (+0.29, t1, 876 = −7.79, P < 0.001), and b* (+0.06, t1, 873 = −2.60, P = 0.0123) values compared to autumn. Drip loss, cooking loss, and shear force all increased during the summer (P < 0.05, Table 6). The mean drip loss and cooking loss were higher by 0.30% (t1, 884 = −3.28, P = 0.0011) and 0.93% (t1, 873 = −3.21, P = 0.0014) in the summer, respectively (Table 6). The shear force for the summer (LSmean: 9.14 ± 0.347 N) was greater than in autumn (LSmean: 8.75 ± 0.355 N, t1, 881 = −2.20, P = 0.0283, Table 6).
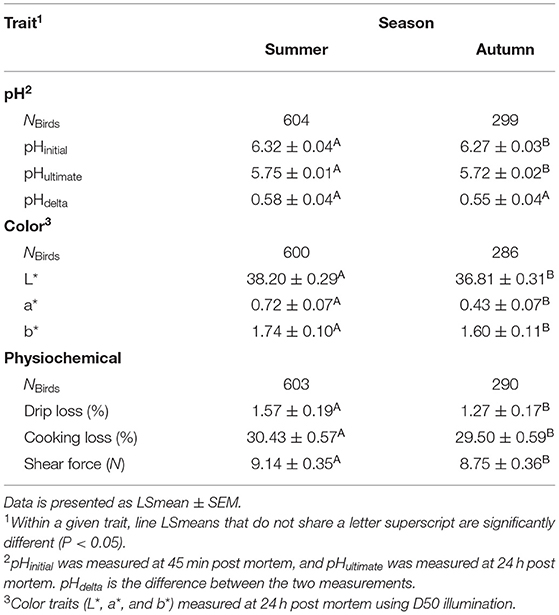
Table 6. Differences in meat quality attributes in male turkeys (NBirds= 817–895 depending on trait) between the summer (June–August) and autumn (September–November) seasons.
Discussion
The goal of this study was to examine the prevalence of post mortem activity in turkeys at a commercial processing plant and analyze the relationship between activity and meat quality, while accounting for differences between genetic lines (A–F) and seasons (summer vs. autumn). Post mortem activity was scored on a 1–4 scale adapted from McNeal et al. (5) after evaluating intra-observer reliability. Most birds displayed none/minimal post mortem activity (score 1, 87%) with lesser percentages having mild (score 2, 4%), moderate (score 3, 7%), and severe (score 4, 2%) muscle activity.
Post Mortem Activity and Meat Quality
Meat redness (a*), drip loss (%), and shear force (N) were associated with activity score. Birds exhibiting severe flapping behavior had breast meat that was numerically redder than the other scores. A connection between meat redness and wing flapping was reported in broiler (5, 8, 30) and turkey studies (24, 25). While it has yet to be fully determined why vigorous wing flapping tends to increase breast meat redness, it is possible that these movements cause damage to the breast muscle as it is the main muscle responsible for wing movement. Reduced muscle tension during slaughter reduces blood spots, hemorrhaging and small blood vessels rupturing in the muscle so it is logical to assume that vigorous movement during bleeding increases muscle tension and subsequently redness (31). Similar to what was noted by McNeal et al. (5), although the differences between these values are numerically significant, they may not be of practical significance or able to be discerned visually by the average consumer.
Breast meat drip loss increased as activity score increased with the highest average drip loss being from birds that exhibited severe body movement and flapping (score 4). Vigorous muscle movement is known to deplete glycogen stores and more quickly increase muscle lactate which speeds up the rate of post mortem pH decline (14, 32, 33). This effect was documented by Berri et al. (30) who found that initial meat pH was strongly and negatively correlated with the duration of wing-flapping before slaughter. In turkeys, rapid declines in muscle pH are well-documented to decrease water-holding capacity of meat and result in greater drip loss and cooking loss (34–36). To provide further support for this hypothesis, Landes et al. (37), found that turkeys that were anesthetized with phenobarbital before slaughter (no struggle) had a slower rate of post mortem pH decline and subsequently lower shear force values. We were unable to discern an effect of post mortem activity on any of the studied pH traits. However, the average pHinitial for birds with activity score 4 was the lowest of all the scores. The lack of discernable effect may be due to the low number of birds who displayed vigorous post mortem activity. It is also possible that the ante mortem activity has a more substantial effect on meat pH than post mortem activity. Future studies should assess both ante and post mortem activity on turkey meat quality to determine their relative importance and relationship. It is unclear whether turkeys exhibiting post mortem activity are also more likely to be active before slaughter.
The toughness of cooked poultry meat (measured by shear force) has been demonstrated to increase with activity during slaughter (24). In our study, we found that birds with no/minimal activity (score 1) had higher average shear force compared to birds with mild activity (score 2), however this difference was small (1 N). Average shear force then increased between scores 2, 3, and 4 as post mortem activity increased, although these differences were not statistically significant. Other turkey studies have found that pre-slaughter activity increases the shear force of breast meat and the opposite is found if birds are anesthetized before slaughter to eliminate struggling (24, 25). This is likely due to the close relationship of shear force with pH and water-holding capacity. Rapid drops in initial pH reduces water-holding capacity which can result in a tougher meat product (higher shear force) (38).
Overall, the results of the present study indicate that post mortem activity may influence some meat quality traits. Although, the magnitude of these differences are not large, their cumulative effect on breast meat quality may be more substantial. Future work should consider how various stunning methods or settings influence the degree of post mortem activity in turkeys.
Effect of Genetic Line
Genetic line influenced pHinitial, pHultimate, pHdelta, a*, b*, and drip loss. There was no effect of genetic line observed for L*, cooking loss, and shear force. The genetic lines used in this study are purebred lines selected for different breeding goals. The most relevant difference for meat quality is the difference in average body weight at slaughter between the lines. The largest lines are sire lines F (25.2 ± 1.8 kg) and E (24.1 ± 1.6 kg), followed by dam lines B (21.9 ± 1.4 kg) and A (21.1 ± 1.4 kg). The two smallest lines are line C (18.8 ± 1.2 kg) and line D (14.6 ± 1.0 kg).
Differences in meat quality between genetic lines has been demonstrated in broiler chickens (12, 39), but consistent differences are not reported in literature for turkeys (21, 40–42). A study looking at fast- and slow-growing turkey lines was unable to detect any effect of genetic line on breast meat color (L*, a*, b*), drip loss, and shear force (40). Zampiga et al. (42) compared a variety of meat quality traits between two commercial turkey hybrids and found that the majority of traits (pHultimate, L*, a*, marinade uptake, cooking loss, and shear force) were not influenced by genotype. However, some traits such as b* and drip loss were different between the groups. Another study by Updike et al. (41) found no differences in the cooking loss, however shear force was significantly lower in the random bred control line compared to modern lines selected for bodyweight and breast yield. Similarly, we did not observe any difference in cooking loss between the six lines. Although we found that the heaviest lines (E and F; 8.8–9.2 N) had numerically higher shear force compared to the lightest line (line D; 8.3 N) these differences were not statistically significant. This could be because line D may be the lightest line in terms of weight, however it is still a modern turkey line with specific breeding goals, whereas Updike et al. (41) they were using a line which had been randomly bred and is more representative of a 1960's commercial turkey line. However, a different study of fast- and slow-growing turkey lines found that slow-growing turkeys had similar pH measurements as the fast-growing line, but were paler and had greater drip loss (21). In our study, the smallest and slowest growing line (line D) had a lower pHinitial compared to the fastest growing lines (lines E and F). Additionally, we found that the slowest growing line (line D), demonstrated the lowest average lightness and highest average redness numerically compared to the faster growing lines. This follows an opposite trend to paler meat observed in slow-growing turkeys by Fernandez et al. (21). However, there were no other differences between line D and lines E and F, which is in line with the lack of differences between fast- and slow-growing turkeys observed in Werner et al. (40).
Poultry meat quality may be influenced by body size and growth rate due to differences in fiber size, although there seems to be substantial conflict in the literature as to whether fast growth rate negatively impacts turkey meat quality (40). Faster growing chicken strains have a larger fiber cross-sectional area (43–46). In broiler chickens, genetic lines with greater breast muscle mass (larger fiber area) revealed lower glycogen stores and subsequently lighter colored meat that was less red and had higher ultimate pH (39). Given the differences in the literature, it may be helpful for future studies to incorporate a serial slaughter experimental design with different genetic lines to better assess how these meat quality attributes change over the different growth trajectories in turkeys. It should also be acknowledged that more data was available for lines A, C, and E compared to lines B, D, and F which may make it a challenge to draw conclusions about meat quality differences between certain genetic lines. Due to the observational nature of the study, and its collaborative nature as part of a larger project (47), the collection of meat quality data was concentrated on some lines more than others.
Effect of Season
Season has a well-documented relationship with poultry meat quality and exposure to high ambient temperature before slaughter tends to produce meat that is lighter with decreased water-holding capacity (15, 48). We expected that turkeys slaughtered during the summer season would be exposed to higher temperature, especially during transportation, which may influence their meat quality. Indeed, season influenced all the studied meat quality traits (except for pHdelta) in the current study, albeit to a limited extent.
Breast meat from birds slaughtered during summer has been reported to be lighter in color, less red, with a lower pH, and greater moisture loss (17, 18). Some of these results were shown in our study as we found the average L* to be greater during summer, as well as a higher b*, indicating that meat is paler and more yellow in color. These effects have been demonstrated in another study of Canadian turkeys where the authors found that the mean L* measurement was highest during the summer, lowest in the winter, with intermediate values in the spring and autumn (48). This aligns with increased incidence of pale, soft, and exudative (PSE) meat reported during summer compared to cooler seasons (18, 48). In Brazil, de Carvalho et al. (18) reported a 22% increase in the occurrence of PSE meat from turkeys processed during the summer compared to winter; i.e., which corresponded to a higher L* and lower pH reported in the summer. To provide further support, turkey hens exposed to cold (−18°C) had breast meat that was darker (lower L*) compared to birds exposed to control temperatures (49). Interestingly, no effect of cold exposure on meat color was observed for turkey toms in this study which indicates that there may be an influence of sex on susceptibility to temperature stress.
Furthermore, we also report that juice loss (drip loss and cooking loss) and shear force were greater during summer months. It is believed that these effects on color and water-holding capacity occur because heat stress increases the conversion of glycogen to lactic acid in the muscle which causes rapid declines in muscle pH (6, 16). The fast decline in pH denatures the muscle proteins and pigments and results in a paler color and increased moisture loss in the raw and cooked products (34, 35).Conversely, meat that is characterized as dark, firm, dry (DFD), which is more often reported during the colder seasons (higher muscle glycogen use to keep the birds warm during transport), tends to have a higher pH and consequently is darker, redder, with decreased moisture loss (50).
However, contrary to expectations, meat pH was higher during the summer compared to autumn. Although the magnitude of this difference was very small (+0.03–0.05 units). As discussed above, we expected that higher summer temperatures would result in lower pH because of the faster conversion of glycogen to lactic acid (51), but also because cold exposure limits muscle glycogen which reduces the potential lactic acid formation in the meat (35). Turkey breasts classified as “fast glycolytic” (pHinitial < 6.2) have been reported to have a warmer internal temperature compared to “slow glycolytic” breasts and one can possibly expect that warmer muscles would be more likely in the summer season (51). It is possible that the pH results from our study do not align with other reports (17, 18) because we are comparing summer and autumn, which may be more similar in environmental conditions compared to the Canadian summer and winter. For example, the average temperature near the processing plant in 2019 for the summer months was 25.2°C compared to 12.9°C in autumn and −0.9°C in winter. This effect was somewhat demonstrated by McCurdy et al. (48) who found that the largest difference in L* values for turkey breast meat samples was between the summer and winter seasons whereas L* values in the spring and autumn were intermediate. Moreover, the majority of data in this study was collected during the summer due to the observational nature of the study and collaboration with the abattoir as part of a larger project (47). Future experimental (i.e., non-observational) studies with a more balanced data distribution may be beneficial to gain further insight into seasonal meat quality differences in Canadian turkeys.
It is also possible that some of the observed differences in meat quality are due to differences in growth between the seasons. After accounting for the effect of genetic line, the LSmean slaughter weight in autumn was 138 grams heavier than the summer and the LSmean breast weight was approximately 88 grams heavier in autumn (data not shown). Cooler temperatures are typically beneficial for poultry growth because the risk of heat stress, which has an inhibitory effect on feed intake and growth, is much lower (52). As mentioned previously, differences in growth and growth rate have the potential to affect meat quality. In particular, faster-growing broiler chicken strains have been shown to have lighter meat with higher ultimate pH (39). It is possible that the differences in growth between the two seasons may influence the effect on meat quality.
There are many points throughout the turkey production cycle where season may influence growth and meat quality. This is a factor of critical importance in many turkey-producing areas that experience distinct seasonal changes. While it should be acknowledged that the observed effects of season on the meat quality traits were small, they may be important when considering the cumulative impact of seasonal changes on turkey production. In the summer season, heat stress can be more likely due to higher ambient temperatures. Heat stress can negatively impact feed intake and growth (52) and result in health and wellbeing issues as well as death during transport (53). The occurrence of dead-on-arrivals at the processing plant was shown to be 79% higher for turkeys slaughtered during the summer compared to autumn (0.52 vs. 0.29%) (54). Therefore, even though the effects on most meat quality traits in this study were relatively small, combined with the effects of temperature on growth and mortality these impacts may be more significant to turkey producers than currently estimated.
Conclusion
This study provides an initial assessment of the prevalence of post mortem activity during conventional turkey slaughter and the effect of this activity on meat quality traits. It is important to acknowledge that although we found associations between activity and several meat quality traits (a*, drip loss, and shear force), the magnitude of the effects was not necessarily large; however, their cumulative effect on profitability of a large turkey operation could be significant. Furthermore, the prevalence of birds exhibiting post mortem activity (score > 1) was low which may have influenced the results. In any case, this study indicates that vigorous post mortem activity may negatively impact certain meat quality traits in male turkeys. There was also a discernable effect of genetic line and season on most studied traits which identifies several areas of future research into the effects of different growth trajectories during different seasons on turkey meat quality.
Data Availability Statement
The raw data supporting the conclusions of this article will be made available by the authors, without undue reservation.
Ethics Statement
The animal study was reviewed and approved by the University of Guelph Animal Care Committee (AUP 3782).
Author Contributions
CB, BW, SB, and EL conceived and designed the experiment. CB and BW secured funding for the experiment. EL, RV, NS, and JM conducted the experiment. EL analyzed the data and wrote the original draft. CB, BW, SB, NS, RV, and EL reviewed and approved the final manuscript.
Funding
This project was funded by the Government of Canada through Genome Canada and the Ontario Genomics Institute (OGI-133). This study was part of the project entitled “Application of genomic selection in turkeys for health, welfare, efficiency, and production traits” funded by the Government of Canada through the Genome Canada Genomic Application Partnership Program and administered by Ontario Genomics [Recipients: BW (Industry) and CB (Academic)].
Conflict of Interest
JM and BW were employees of Hybrid Turkeys at the time of the study. The funders had no role in the design of the study; in the collection, analyses, or interpretation of data; in the writing of the manuscript, or in the decision to publish the results.
The remaining authors declare that the research was conducted in the absence of any commercial or financial relationships that could be construed as a potential conflict of interest.
Publisher's Note
All claims expressed in this article are solely those of the authors and do not necessarily represent those of their affiliated organizations, or those of the publisher, the editors and the reviewers. Any product that may be evaluated in this article, or claim that may be made by its manufacturer, is not guaranteed or endorsed by the publisher.
Acknowledgments
The authors would like to acknowledge Sarah Adams, Elizah McFarland, Emma Hyland, and Michelle Yahiro for assisting with data collection. The authors extend their gratitude to the managers and personnel of Hayter's Farm (Dashwood, Ontario) and Hybrid Turkeys pedigree farm (Kitchener, Ontario) for collaborating on this study. The authors would also like to acknowledge NSERC and Hybrid Turkeys for financial support.
References
1. Raj ABM. Recent developments in stunning and slaughter of poultry. Worlds Poult Sci J. (2006) 62:467. doi: 10.1079/WPS2005109
2. European Community. Directive 93/119/EC on the protection of animals at the time of slaughter or killing. Eur Community Off J. (1993) 340:21–34.
3. Prinz S, van Oijen G, Ehinger F, Coenen A, Bessei W. Electroencephalograms and physical reflexes of broilers after electrical waterbath stunning using an alternating current. Poult Sci. (2010) 89:1265–74. doi: 10.3382/ps.2009-00135
4. Berg C, Raj M. A review of different stunning methods for poultry—animal welfare aspects (stunning methods for poultry). Animals. (2015) 5:1207–19. doi: 10.3390/ani5040407
5. McNeal WD, Fletcher DL, Buhr RJ. Effects of stunning and decapitation on broiler activity during bleeding, blood loss, carcass, and breast meat quality. Poult Sci. (2003) 82:163–8. doi: 10.1093/ps/82.1.163
6. Barbut S. The Science of Poultry Meat Processing. University of Guelph (2015). Available online at: www.poultryandmeatprocessing.com
7. Gregory NG, Wilkins LJ. Effect of ventricular fibrillation at stunning and ineffective bleeding on carcase quality defects in broiler chickens. Br Poult Sci. (1989) 30:825–9. doi: 10.1080/00071668908417208
8. Huang JC, Yang J, Huang M, Zhu ZS, Sun XB, Zhang BH, et al. Effect of pre-slaughter shackling and wing flapping on plasma parameters, postmortem metabolism, AMPK, and meat quality of broilers. Poult Sci. (2018) 97:1841–7. doi: 10.3382/ps/pey019
9. Min B, Ahn DU. Sensory properties of packaged fresh and processed poultry meat. In: Advances in Meat, Poultry and Seafood Packaging. Sawston: Woodhead Publishing Ltd (2012). p. 112–53. doi: 10.1533/9780857095718.1.112
10. Barbut S. Colour measurements for evaluating the pale soft exudative (PSE) occurrence in turkey meat. Food Res Int. (1993) 26:39–43. doi: 10.1016/0963-9969(93)90103-P
11. Schneider BL, Renema RA, Betti M, Carney VL, Zuidhof MJ. Effect of holding temperature, shackling, sex, and age on broiler breast meat quality. Poult Sci. (2012) 91:468–77. doi: 10.3382/ps.2010-00952
12. Debut M, Berri C, Baéza E, Sellier N, Arnould C, Guémené D, et al. Variation of chicken technological meat quality in relation to genotype and preslaughter stress conditions. Poult Sci. (2003) 82:1829–38. doi: 10.1093/ps/82.12.1829
13. Ma RT, Addis PB. The association of struggle during exsanguination to glycolysis, protein solubility and shear in turkey pectoralis muscle. J Food Sci. (1973) 38:995–7. doi: 10.1111/j.1365-2621.1973.tb02132.x
14. Ali MS, Kang GH, Joo ST. A review: influences of pre-slaughter stress on poultry meat quality. Asian Austral J Anim Sci. (2008) 21:912–6. doi: 10.5713/ajas.2008.r.06
15. Leishman EM, Ellis J, van Staaveren N, Barbut S, Vanderhout RJ, Osborne VR, et al. Meta-analysis to predict the effects of temperature stress on meat quality of poultry. Poult Sci. (2021) 100:101471. doi: 10.1016/j.psj.2021.101471
16. Owens CM, Alvarado CZ, Sams AR. Research developments in pale, soft, and exudative turkey meat in North America. Poult Sci. (2009) 88:1513–7. doi: 10.3382/ps.2009-00008
17. Bianchi M, Petracci M, Sirri F, Folegatti E, Franchini A, Meluzzi A. The influence of the season and market class of broiler chickens on breast meat quality traits. Poult Sci. (2007) 86:959–63. doi: 10.1093/ps/86.5.959
18. de Carvalho RH, Soares AL, Guarnieri PD, Oba A, Ida EI, Shimokomaki M. Turkey Meat. Seasonal effect on meat quality and on dead on arrival index in a commercial plant. Braz Arch Biol Technol. (2018) 61:e18180106. doi: 10.1590/1678-4324-2018180106
19. Bianchi M, Petracci M, Cavani C. The influence of genotype, market live weight, transportation, and holding conditions prior to slaughter on broiler breast meat color. Poult Sci. (2006) 85:123–8. doi: 10.1093/ps/85.1.123
20. Tang H, Gong YZ, Wu CX, Jiang J, Wang Y, Li K. Variation of meat quality traits among five genotypes of chicken. Poult Sci. (2009) 88:2212–8. doi: 10.3382/ps.2008-00036
21. Fernandez X, Sante V, Baeza E, Lebihan-Duval E, Berri C, Remignon H, et al. Post mortem muscle metabolism and meat quality in three genetic types of turkey. Br Poult Sci. (2001) 42:462–9. doi: 10.1080/00071660120070604
22. Zampiga M, Soglia F, Baldi G, Petracci M, Strasburg GM, Sirri F. Muscle abnormalities and meat quality consequences in modern turkey hybrids. Front Physiol. (2020) 11:554. doi: 10.3389/fphys.2020.00554
23. Debut M, Berri C, Arnould C, Guemene D, Sante-Lhoutellier V, Sellier N, et al. Behavioural and physiological responses of three chicken breeds to pre-slaughter shackling and acute heat stress. Br Poult Sci. (2005) 46:527–35. doi: 10.1080/00071660500303032
24. Froning GW, Babji AS, Mather FB. The effect of preslaughter temperature, stress, struggle and anesthetization on color and textural characteristics of turkey muscle. Poult Sci. (1978) 57:630–3. doi: 10.3382/ps.0570630
25. Ngoka DA, Froning GW, Lowry SR, Babji AS. Effects of sex, age, preslaughter factors, and holding conditions on the quality characteristics and chemical composition of turkey breast muscles. Poult Sci. (1982) 61:1996–2003. doi: 10.3382/ps.0611996
26. Landis JR, Koch GC. The measurement of observer agreement for categorical data. Biometrics. (1977) 33:159–74. doi: 10.2307/2529310
27. Petrie A, Watson P. Statistics for Veterinary and Animal Science. 3rd Ed. Chichester: Wiley-Blackwell (2013).
28. CIE. Colorimetry: Official Recommendations of the International Commission on Illumination. Paris: Boreau Central de la CIE (1976).
29. Lee YS, Owens CM, Meullenet JF. The meullenet-owens razor shear (mors) for predicting poultry meat tenderness: its applications and optimization. J Texture Stud. (2008) 39:655–72. doi: 10.1111/j.1745-4603.2008.00165.x
30. Berri C, Debut M, Santé-Lhoutellier V, Arnould C, Boutten B, Sellier N, et al. Variations in chicken breast meat quality: implications of struggle and muscle glycogen content at death. Br Poult Sci. (2005) 46:572–9. doi: 10.1080/00071660500303099
31. Gregory NG. Recent concerns about stunning and slaughter. Meat Sci. (2005) 70:481–91. doi: 10.1016/j.meatsci.2004.06.026
32. Ngoka DA, Froning GW. Effect of free struggle and preslaughter excitement on color of turkey breast muscles. Poult Sci. (1982) 61:2291–2293. doi: 10.3382/ps.0612291
33. Papinaho PA, Fletcher DL, Buhr RJ. Effect of electrical stunning amperage and peri-mortem struggle on broiler breast rigor development and meat quality. Poult Sci. (1995) 74:1533–9. doi: 10.3382/ps.0741533
34. Pietrzak M, Greaser ML, Sosnicki AA. Effect of rapid rigor mortis processes on protein functionality in pectoralis major muscle of domestic turkeys. J Anim Sci. (1997) 75:2106–16. doi: 10.2527/1997.7582106x
35. King NJ, Whyte R. Does it look cooked? A review of factors that influence cooked meat color. J Food Sci. (2006) 71:31–40. doi: 10.1111/j.1750-3841.2006.00029.x
36. Carvalho RH, Soares AL, Honorato DCB, Guarnieri PD, Pedrao MR, Paiao FG, et al. The incidence of pale, soft, and exudative (PSE) turkey meat at a Brazilian commercial plant and the functional properties in its meat product. LWT Food Sci Technol. (2014) 59:883–8. doi: 10.1016/j.lwt.2014.07.019
37. Landes DR, Dawson LE, Price JF. Protein extractability of turkey breast muscle exhibiting different rates of post-mortem glycolysis. J Food Sci. (1971) 36:122–4. doi: 10.1111/j.1365-2621.1971.tb02051.x
38. Warriss PD. Meat quality. In: Meat Science: An Introductory Text. Oxon: CABI Pub (2001). p. 106–30. doi: 10.1079/9780851994246.0106
39. Berri C, Wacrenier N, Millet N, Le Bihan-Duval E. Effect of selection for improved body composition on muscle and meat characteristics of broilers from experimental and commercial lines. Poult Sci. (2001) 80:833–8. doi: 10.1093/ps/80.7.833
40. Werner C, Riegel J, Wicke M. Slaughter performance of four different turkey strains, with special focus on the muscle fiber structure and the meat quality of the breast muscle. Poult Sci. (2008) 87:1849–59. doi: 10.3382/ps.2007-00188
41. Updike MS, Zerby HN, Sawdy JC, Lilburn MS, Kaletunc G, Wick MP. Turkey breast meat functionality differences among turkeys selected for body weight and/or breast yield. Meat Sci. (2005) 71:706–12. doi: 10.1016/j.meatsci.2005.05.014
42. Zampiga M, Tavaniello S, Soglia F, Petracci M, Mazzoni M, Maiorano G, et al. Comparison of 2 commercial turkey hybrids: productivity, occurrence of breast myopathies, and meat quality properties. Poult Sci. (2019) 98:2305–15. doi: 10.3382/ps/pey607
43. Dransfield E, Sosnicki AA. Relationship between muscle growth and poultry meat quality. Poult Sci. (1999) 78:743–6. doi: 10.1093/ps/78.5.743
44. Yalcin S, Sahin K, Tuzcu M, Bilgen G, Ozkan S, Izzetoglu GT, et al. Muscle structure and gene expression in pectoralis major muscle in response to deep pectoral myopathy induction in fast- and slow-growing commercial broilers. Br Poult Sci. (2019) 60:195–201. doi: 10.1080/00071668.2018.1430351
45. Koomkrong N, Theerawatanasirikul S, Boonkaewwan C, Jaturasitha S, Kayan A. Breed-related number and size of muscle fibres and their response to carcass quality in chickens. Ital J Anim Sci. (2015) 14:638–42. doi: 10.4081/ijas.2015.4145
46. Remignon H, Gardahaut MF, Marche G, Ricard FH. Selection for rapid growth increases the number and the size of muscle fibres without changing their typing in chickens. J Muscle Res Cell Motil. (1995) 16:95–102. doi: 10.1007/BF00122527
47. Malchiodi F, Wood B, Barbut S, Harlander A, Baes C. Application of genomic selection for enhancing health, welfare, efficiency and production traits in turkeys. J Anim Sci. (2019) 96:116–8. doi: 10.1093/jas/sky404.255
48. McCurdy RD, Barbut S, Quinton M. Seasonal effect on pale soft exudative (PSE) occurrence in young turkey breast meat. Food Res Int. (1996) 29:363–6. doi: 10.1016/0963-9969(96)00020-8
49. Henrikson ZA, Vermette CJ, Schwean-Lardner K, Crowe TG. Effects of cold exposure on physiology, meat quality, and behavior of turkey hens and toms crated at transport density. Poult Sci. (2018) 97:347–57. doi: 10.3382/ps/pex227
50. Mallia JG, Barbut S, Vaillancourt JP, Martin SW, McEwen SA. A dark, firm dry-like condition in turkeys condemned for cyanosis. Poult Sci. (2000) 79:281–5. doi: 10.1093/ps/79.2.281
51. Fraqueza MJ, Cardoso AS, Ferreira MC, Barreto AS. Incidence of pectoralis major turkey muscles with light and dark color in a portuguese slaughterhouse. Poult Sci. (2006) 85:1992–2000. doi: 10.1093/ps/85.11.1992
52. Lara LJ, Rostagno MH. Impact of heat stress on poultry production. Animals. (2013) 3:356–69. doi: 10.3390/ani3020356
53. Broom DM. Welfare of transported animals: welfare assessment factors affecting welfare. In: Grandin T, editor. Livestock Handling Transport. Oxfordshire: CAB International (2019). p. 12–29. doi: 10.1079/9781786399151.0012
Keywords: breast meat, genetics, Meleagris gallopavo, poultry, season, slaughter, wing flapping
Citation: Leishman EM, Vanderhout RJ, van Staaveren N, Barbut S, Mohr J, Wood BJ and Baes CF (2022) Influence of Post Mortem Muscle Activity on Turkey Meat Quality. Front. Vet. Sci. 9:822447. doi: 10.3389/fvets.2022.822447
Received: 25 November 2021; Accepted: 20 January 2022;
Published: 21 February 2022.
Edited by:
Edward Narayan, The University of Queensland, AustraliaReviewed by:
Massimiliano Petracci, University of Bologna, ItalyNuh Ocak, Ondokuz Mayıs University, Turkey
Aristide Maggiolino, University of Bari Aldo Moro, Italy
Copyright © 2022 Leishman, Vanderhout, van Staaveren, Barbut, Mohr, Wood and Baes. This is an open-access article distributed under the terms of the Creative Commons Attribution License (CC BY). The use, distribution or reproduction in other forums is permitted, provided the original author(s) and the copyright owner(s) are credited and that the original publication in this journal is cited, in accordance with accepted academic practice. No use, distribution or reproduction is permitted which does not comply with these terms.
*Correspondence: Christine F. Baes, Y2JhZXMmI3gwMDA0MDt1b2d1ZWxwaC5jYQ==