- 1Laboratory of Manipulation of Oocytes and Preantral Follicles, Faculty of Veterinary, State University of Ceara, Fortaleza, Brazil
- 2Natural Product Chemistry Laboratory, State University of Ceara, Fortaleza, Brazil
- 3Superior Institute of Biomedical Science, State University of Ceará, Fortaleza, Brazil
- 4Schothorst Feed Research, Lelystad, Netherlands
This study evaluated the effects of different concentrations (10, 20, or 40 μM) of eugenol (EUG 10, EUG 20, or EUG 40), ascorbic acid (50 μg/mL; AA) or anethole (300 μg/mL; ANE 300) on the in-vitro survival and development of goat preantral follicles and oxidative stress in the cultured ovarian tissue. Ovarian fragments from five goats were cultured for 1 or 7 days in Alpha Minimum Essential Medium (α-MEM+) supplemented or not with AA, ANE 300, EUG 10, EUG 20 or EUG 40. On day 7 of culture, when compared to MEM, the addition of EUG 40 had increased the rate of follicular development, as observed by a decrease in the proportion of primordial follicles alongside with an increase in the rate of normally developing follicles. Furthermore, EUG 40 significantly increased both follicular and oocyte diameters. Subsequently, ovarian fragments from three goats were cultured for 1 or 7 days in α-MEM+ supplemented or not with AA, ANE 300 or EUG 40. All tested antioxidants, except ANE 300, were able to significantly decrease the levels of reactive oxygen species in the ovarian tissue, but EUG 40 could most efficiently neutralize free radicals. All ovarian tissues cultured in the presence of antioxidants, especially EUG 40, presented a significant decrease in H3K4me3 labeling, indicating a silencing of genes that play a role in the inhibition of follicular activation and apoptosis induction. When compared to cultured control tissues, both EUG 40 and ANE 300 significantly increased the intensity of calreticulin labeling in growing follicles. The mRNA relative expression of ERP29 and KDM3A was significantly increased when the culture medium was supplemented with EUG 40, indicating a response to ER stress experienced during culture. In conclusion, EUG 40 improved in-vitro follicle survival, activation and development and decreased ROS production, ER stress and histone lysine methylation in goat ovarian tissue.
Introduction
The mammalian ovary contains thousands to millions of immature oocytes, of which most (circa 90%) are enclosed in ovarian preantral follicles (PAFs—primordial, primary and secondary ones). Despite this large oocyte population, only around 0.1% will be ovulated; the remaining ones will suffer atresia (1). To safeguard part of the follicular population before degeneration, the in-vitro culture of PAFs has been developed. Such a procedure supports the preservation of genetic material from the farm and endangered animals (2, 3), the treatment of infertility in humans (4) and for the testing of chemical compounds (3, 5–7). Preantral follicles can be cultured in situ (enclosed in ovarian tissue) or in isolated form (8). In-situ culture aims to study the initial development of primordial and primary follicles (2, 8), whereas the culture of isolated follicles can be applied for the study of secondary follicles (8).
In-vitro culture of PAFs enclosed in the ovarian tissue may result in remarkable follicular losses (50–70%), which may be related to the high production of reactive oxygen species (ROS), leading to oxidative stress (9, 10). The ROS are substances normally produced through cellular metabolism and some cellular processes such as oocyte maturation (11), follicular steroidogenesis and ovulation (12). However, in stressful situations, such as under in-vitro culture conditions, the exceeding ROS can cause DNA damage, peroxidation of membrane phospholipids and cell death (13, 14). Oxidative stress will interfere with protein folding, which can be assessed by the activation of chaperones such as calreticulin and endoplasmic reticulum (ER) (15). Epigenetically, the methylation of histone residues plays an important role during folliculogenesis. For instance, oxidative stress interferes with histone methylation (16, 17), and such an effect is also observed during the in-vitro maturation of oocytes (18). The methylation of the histone 3 (H3), such as the methylation of H3K4me3 and H3K9me3, is associated with the activation and the silencing of gene expression, respectively (19, 20), and their demethylation is controlled by the lysine demethylases KDM1AX1 and KDM3A.
To avoid the negative impacts of ROS, antioxidants are added to the culture media. These antioxidants are classified as enzymatic when they are produced by cells (e.g., glutathione) or as non-enzymatic when they are obtained via the diet (e.g., ascorbic acid, anethole). Both ascorbic acid and anethole have previously been tested in the in-vitro culture of PAFs, resulting in a survival rate ranging from 40 to 60% and in follicular activation of around 65% (9, 10). A promising antioxidant that may increase the rate of viable follicles during in-vitro culture is the phenolic compound eugenol, due to its positive results obtained with other cell types (20, 21).
Eugenol is a phenolic compound, chemically designated as 4-allyl-2-methoxy-phenol, commonly known as clove essence because it is present in large quantities in clove essential oil (Eugenia aromatica), which has antimicrobial, anti-inflammatory, antiseptic, antipyretic and antioxidant properties (22). As an antioxidant, eugenol added to the in-vitro culture medium improves the viability (90%) of neuronal stem cells (23) and increases the expression of antioxidant enzymes (reduced glutathione, peroxidase, catalase and superoxide dismutase) in human mononuclear cells (20). Furthermore, eugenol is chemically stable during culture, with direct and indirect effects against ROS (24). Based on a previous study, eugenol supports the viability of bovine large secondary follicles in vitro (25). However, to the best of our knowledge, the effect of eugenol on in-vitro early folliculogenesis, i.e., survival and activation of the primordial follicle and initial growth of these follicles, is still unknown.
Although in-vitro culture of preantral follicles is a promising alternative to understand early folliculogenesis, oxidative stress during culture remains a challenge (9, 10). Based on this, the present study evaluated the effects of adding different concentrations of eugenol on the in-vitro development of goat preantral follicles. Additionally, in-vitro culture was also performed in the presence of the antioxidants commonly added to the medium for the culture of preantral follicles, i.e., anethole 300 μg/mL and ascorbic acid 50 μg/mL. The following endpoints were evaluated: follicular survival, activation, viability methylation profile (H3K4me3 and Calreticulin), gene expression (CAT; ERP29; KDM1AX1 and KDM3A) and antioxidant capacity.
Materials and Methods
Chemicals and Media
Unless otherwise mentioned, the culture media, ascorbic acid, anethole, eugenol and other chemicals used in the present experiments were purchased from Sigma-Aldrich (USA).
Source of Ovaries
All procedures were approved by the Ethics and Animal Use Committee of State University of Ceara, Brazil (Protocol N. 05498214/2019). A total of eight ovarian pairs from eight adult crossbred goats (1–3 years old) were collected at a local slaughterhouse. The surrounding fat tissue and ligaments were removed, and the ovaries were washed in 70% alcohol, followed by two washes in minimum essential medium (MEM) plus HEPES (MEM-HEPES). The ovaries were placed into tubes containing 15 mL of MEM-HEPES, supplemented with penicillin (100 μg/mL) and streptomycin (100 μg/mL) and then transported to the laboratory at 4°C within 1 h.
Culture Medium
The basic medium used was α-MEM+ (M5650, pH 7.2 −7.4) supplemented with 1.25 mg/mL bovine serum albumin (BSA), ITS (10 μg/mL insulin, 5.5 μg/mL transferrin, 5 ng/mL selenium), 2 mM glutamine, and 2 mM hypoxanthine, which was also named α-MEM+. Incubation was carried out at 38.5 °C in 7.5% CO2 in air for 1 or 7 days. Fresh media were prepared immediately before use and incubated for 1 h prior to use, with 1 mL in each well. The fragments were individually cultured in a 24-well plate containing 1 mL α-MEM+ culture medium. The culture medium was replaced with fresh medium every 2 days. Each treatment was replicated five times.
Experimental Design
In the laboratory, the ovarian cortex of five ovarian pairs was removed with a sterile scalpel and divided into 14 fragments (3 x 3 x 1 mm). Two fragments were randomly taken and immediately fixed for the non-cultured control treatment as described below. The remaining fragments were randomly distributed (two fragments per treatment) into the six treatments as follows: α-MEM+ (cultured control treatment) either or not supplemented with ascorbic acid 50 μg/mL (AA treatment), anethole 300 μg/mL (ANE 300 treatment), or eugenol at three different concentrations of 10, 20 and 40 μM, which were termed as EUG 10, EUG 20, EUG 40 treatments, respectively. The concentration of ascorbic acid (9), anethole (10), and eugenol (10 μM/mL, 20 μM /mL or 40 μM/mL; 25) were chosen based on previous studies. At the end of each culture period (days 1 and 7), ovarian fragments from each treatment were evaluated for morphology and development (follicular class and growth of follicle and oocyte diameters). In addition, spent culture media at the end of 24 h of culture at day 1 and at day 7 of culture (after medium replacement at day 6) were stored at −80°C for ROS analysis and quantification of thiol groups.
Based on the results of the morphologic analysis, the viability, immunofluorescence detection of histone H3 methylated and calreticulin assays were performed only in the treatments with the concentration of eugenol that maintained follicular morphology and increased follicular activation and diameter. For this, three ovarian pairs collected at a slaughterhouse were prepared in the laboratory as aforementioned and then cut into fragments. One fragment was immediately processed for follicle isolation (fresh control) or fixed, and the remaining fragments were cultured for 7 d in basic culture medium (α-MEM+) in the absence or presence AA, ANE 300 or in the eugenol treatment that provided the best outcome, i.e., a significantly higher percentage of morphologically normal follicles at day 7 of culture when compared with the other treatments.
Morphological Analysis and Assessment of in vitro Follicular Growth
Tissues from all treatments (fresh control or cultured for 1 and 7 days) were fixed in Carnoy's solution for 12 h and were processed for histology. The follicle stage and survival were assessed microscopically on serial sections. Coded anonymized slides were then examined by microscopy (Nikon, Sendai, Japan) at 400 × magnification.
The developmental stages of follicles have been defined as primordial (oocyte surrounded by a few flattened granulosa cells) or developing, i.e., intermediate (oocyte surrounded by flattened and at least one cuboidal granulosa cell), primary (oocyte surrounded by a complete layer of cuboidal granulosa cells), or secondary (oocyte surrounded by two or more complete layers of cuboidal granulosa cells). These follicles were classified as morphologically normal (follicles containing an intact oocyte and granulosa cells well-organized in layers without a pyknotic nucleus) and degenerated follicles (oocyte with a pyknotic nucleus, retracted cytoplasm, or disorganized granulosa cells detached from the basement membrane), as described Silva et al. (26).
Overall, 150 follicles were evaluated for each treatment (30 follicles per treatment in one repetition x 5 repetitions). To calculate follicular activation and growth, only morphologically normal follicles with a visible oocyte nucleus were recorded, and the proportion of primordial and growing follicles was calculated at day 0 and after day 7 of the culture in all treatments. In addition, from the basement membrane, the diameter of each normal oocyte and follicle were measured using a light microscope fitted with an eyepiece micrometer (Zeiss, Cologne, Germany) under 400 × magnification.
Assessment of Preantral Follicle Viability by Trypan Blue
Each ovarian pair of ovaries (n = 3) collected at a slaughterhouse was cut into fragments. Two fragments were immediately processed for follicle isolation (non-cultured tissue), and the remaining fragments were cultured for 7 days in basic culture medium (α-MEM+), and ANE 300 or in the treatment group (40 μM/mL eugenol treatment) that provided the best outcome, i.e., a significantly higher percentage of morphologically normal follicles at day 7 of culture when compared with the other treatments. Goat preantral follicles were isolated from ovarian fragments using the mechanical method and stained with trypan blue as previously described (27). The viability of the isolated preantral follicles was assessed by the trypan blue dye exclusion test. The follicles were examined with an inverted microscope (Nikon, Tokyo, Japan) and classified as nonviable or viable if they were positively or negatively stained with trypan blue, respectively.
Immunofluorescence Detection of Histone H3 Methylated and Calreticulin
The ovarian fragments were fixed with 4% paraformaldehyde in PBS (pH 7.2) for 4 h and later dehydrated, diaphanized, and included in paraffin. Briefly, tissue sections (5 μm) were mounted on Superfrost Plus slides (KnittelGlass, Bielefeld, Germany), dewaxed with Citrisolve (Fisher Scientific, Ottawa, Canada), and rehydrated with increasing ethanol concentration. Antigen recovery was performed by incubating tissue sections in 0.01 M sodium citrate buffer (pH 6) for 5 min under a pressure cooker. After cooling to 37°C, the tissue sections were washed in PBS and blocked for 1 h at room temperature using PBS containing 1% (w/v) BSA. After antigenic recovery, the slides were incubated overnight at 4°C with polyclonal primary antibodies H3K4me3 (Abcam ab8580) and calreticulin (Abcam ab2907). The negative control was obtained by omitting the primary antibodies. Subsequently, the slides were incubated with secondary IgG antibody labeled (Alexa Fluor® 488; Abcam ab150077) for 1 h at room temperature and mounted with Fluoroshield Mounting Medium With DAPI® (Abcam ab104139). Finally, the slides were viewed using a fluorescence microscope (Nikon, Tokyo, Japan) with 20 × magnification. The images were individually saved using a monochrome digital camera to measure fluorescence intensities (FIs). Exposure gains and rates were consistent across samples. The FIs were quantified only in the limited area of normal (n = 230) preantral follicles using the software Image J 1.4.7 (NIH, Bethesda, MD, US). All evaluations were performed by a single operator.
RNA Extraction and Real-Time PCR (QPCR)
Total RNA was isolated from frozen-thawed samples (non-cultured and cultured) using the Trizol® reagent, according to the manufacturer's recommendations (Invitrogen, Carlsbad, CA, USA). Isolated RNA was further purified (PureLink™ RNA Mini Kit; Ambion®, Carlsbad, CA, USA), treated with DNase I, and RNA samples were quantified with a spectrophotometer. Isolated RNA served as a template to synthesize the complementary DNA using the Superscript™ II RNase H-reverse Transcriptase (Invitrogen, São Paulo, SP, Brazil), followed by quantitative polymerase chain reaction (qPCR) in a final volume of 20 μL containing 1 μL of each complementary DNA, one x Power SYBR Green PCR Master Mix (10 μL), 7.4 μL of ultra-purewater, and 0.5 μL (final concentration) of both sense and antisense primers. Primer pairs (sense and antisense) and sequences for target genes are shown in Table 1. Peptidylprolyl isomerase A (PPIA) gene was selected as the endogenous control for normalizing gene expression among samples. The PCR conditions consisted of an initial step for denaturation and polymerase activation for 15 min at 94°C, followed by 40 cycles of 15 s at 94°C, 30 s at 60°C, and 45 s at 72°C. A final extension was performed for 10 min at 72°C. The specificity of each primer set was tested with melting curves that were carried out between 60°C and 95°C. All amplifications were carried out in a Bio-Rad iQ5 (Bio-Rad, Hercules, CA). Data were analyzed using the efficiency-corrected Delta-Delta-Ct method (28). The fold-change values of the genes of interest were normalized using the geometric mean of the fold-change values of a housekeeping gene.
Evaluation of the Antioxidant Potential Through Concentration of Reactive Oxygen Species and Quantification of Thiol Groups in the Medium After in vitro Culture
For the quantification of ROS and thiol groups, 60 μL of each spent culture medium was centrifuged (720 × g, 4°C) for 10 min to eliminate cells and cell debris before quantification. The supernatant was used for the analyses.
The concentration of ROS was assessed through the Amplex Red/HRP (Molecular Probes) method which detects the clotting of fluorescent oxidized substances in the reacting mixture. 10 μL of the medium were incubated with SOD (SOD 100 IU/mL; Sigma-Aldrich, St. Louis, MO, USA), the horseradish peroxidase (PRF 0.5 U/ml; Sigma-Aldrich, St. Louis, MO, USA) and AmplexRed (50 M; Sigma-Aldrich, St. Louis, MO, USA). The fluorescence was measured through a microdishes reader (Victor3, Perkin Elmer) at 595 nm of excitation. The levels of ROS were expressed in micromoles of hydrogen peroxide (H2O2).
For the analysis of thiol groups, 60 μL the supernatant of the centrifuged medium was added mixed with 930 μL of 10 mM Tris-HCl, 0.9% NaCl (w/v), pH 7.4 buffer and methanol with an ultra-turrax homogenizer (Jamke & Kunkel IKA Labortechnik, Germany). The quantification of free thiol groups in the medium was performed using 10 μL of dithiolnitrobenzoic acid (DTNB) in a spectrophotometer (Hitachi U-3300). Thiol groups react with DTNB releasing the disulfide joined to the molecule, leading to the formation of 2-nitro-5-benzoato (NTB-) which ionizes the c in aqueous medium with neutral or alkaline pH. The NTB2- is quantified with spectrophotometer at a wavelength of 412 nm.
Evaluation of Antioxidant Capacity by Reducing ABTS and DPPH Free Radicals in the Medium After in vitro Culture
Antioxidant activity was measured in 96-well flat-bottom plates using BIOTEK Elisa reader (model ELX 800, software “Gen5 V2.04.11”). For the ABTS method, ABTS+ solution (7 mM, 5 mL) was mixed with 88 μL of potassium persulfate (140 mM). The mixture was stirred and kept in the dark at room temperature for 16 h. Then, 1 mL of this solution was added to 99 mL of ethanol. In 96-well plates, 300 μL of ABTS solution and 3 μL of the culture medium (sample) were used per well. For the DPPH analysis, the following solutions were used per well: 180 μL of DPPH (2,2-diphenyl-1-picrylhydrazyl) methanolic solution and 20 μL of the culture medium (sample).
The dilutions of samples and positive standards used in the quantitative microplate evaluations, starting from a stock solution with a concentration of 20 mg/mL were: 200 μg/mL, 100 μg/mL, 50 μg/mL, 25 μg/mL, 12.5 μg/mL, 6.25 μg/mL, 3.12 μg/mL, 1.56 μg/mL, and 0.78 μg/mL.
The absorbance for the ABTS method was measured at 630 nm up to a total of 10 min of incubation. As a negative standard, all solutions except the sample were used. For DPPH, the absorbance was measured at 490 nm for a total of 60 min of incubation.
The results were expressed as a percentage of inhibition and calculated according to the following formula:
AC: Absorbance of the ABTS or DPPH control at time 0.
AS: Absorbance of the sample containing ABTS at time 6 and 10 min or DPPH at time 60 min.
Statistical Analysis
Statistical analysis was carried out using Sigma Plot 11 (Systat Software Inc., USA). The normality (Shapiro-Wilk test) and homogeneity of variance (Levene's test) were initially evaluated. All parameters were analyzed with one-way ANOVA followed by Kruskal-Wallis and Mann-Whitney tests, when appropriate. Data are reported as mean (±SEM). Values with P < 0.05 were considered statistically significant.
Results
Follicle Survival Before and After Culture
A total of 2,632 preantral follicles were analyzed by classical histology. Representative images of the follicles after 7 days of culture are shown in Figure 1. The percentages of morphologically normal preantral follicles in each follicular category (primordial, intermediate and primary follicles) in the non-cultured control and after 1 or 7 days of in-vitro culture in all treatments are shown in Table 2. After 1-day culture, a significant reduction in the percentage of normal follicles was observed when compared to the control, except for primordial follicles cultured in the presence of EUG 20 or EUG 40, intermediate follicles cultured in the presence of ANE 300 or EUG 40 and growing follicles cultured in the presence of EUG 40. A significant decrease in the percentage of normal primary follicles was observed only when culture was performed for 1-day in the presence of ANE 300. On day-7 of in-vitro culture, except for the culture in the presence of ANE 300, a significant decrease in the percentage of normal primordial follicles was observed, whereas a decrease in the percentage of normal primary follicles was observed only when culture was performed in the presence of EUG 10. Although in-vitro culture decreased the morphology of growing follicles, the significantly highest percentages of normal growing follicles were observed after culture in the presence of EUG 40 and ANE 300. Moreover, a lower rate of normal primordial follicles was observed at day 7 when compared with day 1 when culture was performed in the presence of EUG, regardless of the tested concentration.
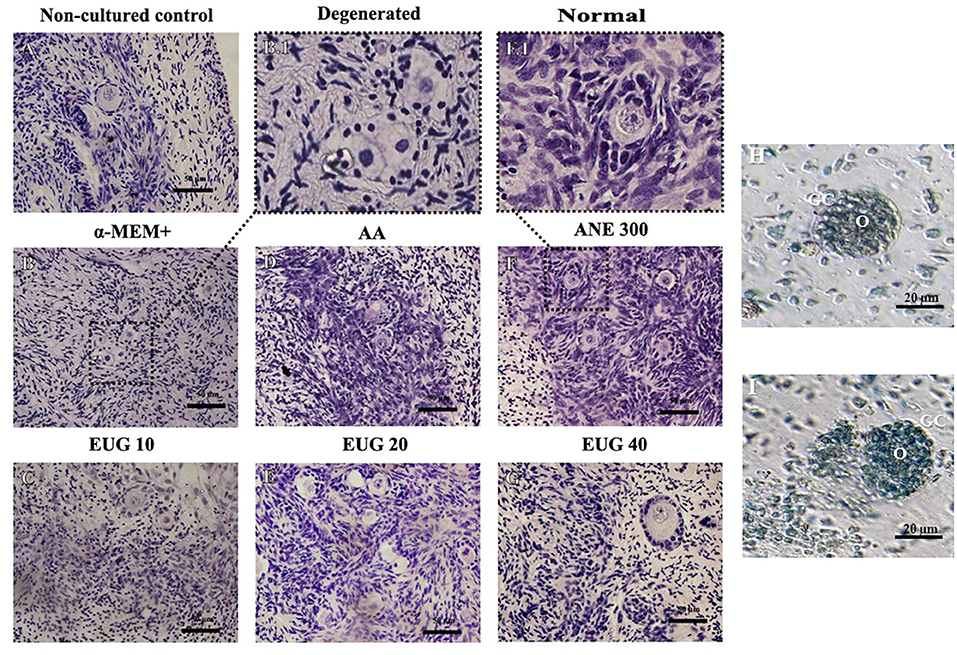
Figure 1. Representative images showing histological aspects of morphological normal preantral follicles before culture (A) and after culture in the absence of antioxidants (B), degenerated follicles when cultured in the absence of antioxidants (B.1), and normal follicles when cultured in a medium supplemented with EUG 10 (C), or AA (D), or EUG 20 (E), or ANE 300 (F,F.1), or EUG 40 (G). Bar 50 μm. Staining: Periodic Acid-Schiff (PAS) and hematoxylin. Assessment of the viability of preantral follicles using trypan blue staining after 7-days culture. Viable (H) and non-viable (I) isolated preantral follicle after in vitro culture in α-MEM+. O, Oocyte; GC, Granulosa cells. Scale bar 20 μm.
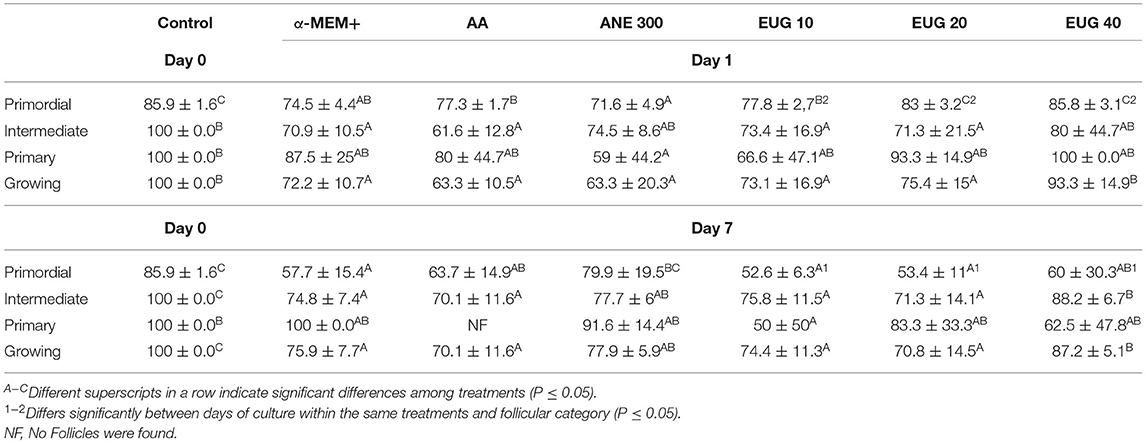
Table 2. Mean (±SEM) percentage of morphologically normal preantral follicles in each follicular category non-cultured ovarian tissue (control) and tissue cultured for 1 or 7 days in control medium without or with ascorbic acid 50 μg/mL (AA), anethole 300 μg/mL (ANE 300) or eugenol at 10 (EUG 10), 20 (EUG 20) or 40 μM/mL (EUG 40).
Distribution Rates of Morphologically Normal and Degenerated Preantral Follicles (Primordial and Growing) Before and After in vitro Culture
The proportions of morphologically normal primordial (Figure 2A) and growing follicles (intermediate + primary—Figure 2B) as well as degenerated primordial (Figure 2C) and growing (Figure 2D) follicles were determined in non-cultured ovarian tissue or in tissues cultured for 1 or 7 days. Regardless of the cultured treatment, from day 1 to day 7, there was a significant reduction in the percentage of normal primordial follicles, with a concomitant increase in the percentage of normally growing follicles. On days 1 and 7 of in-vitro culture, a decrease in the percentage of normal preantral follicles was observed in all treatments, except on day 1, when tissue was cultured in the presence of EUG 40. On day 7, medium supplementation with EUG 40 resulted in a decreased rate of normal primordial follicles together with the highest percentages of normally developing follicles.
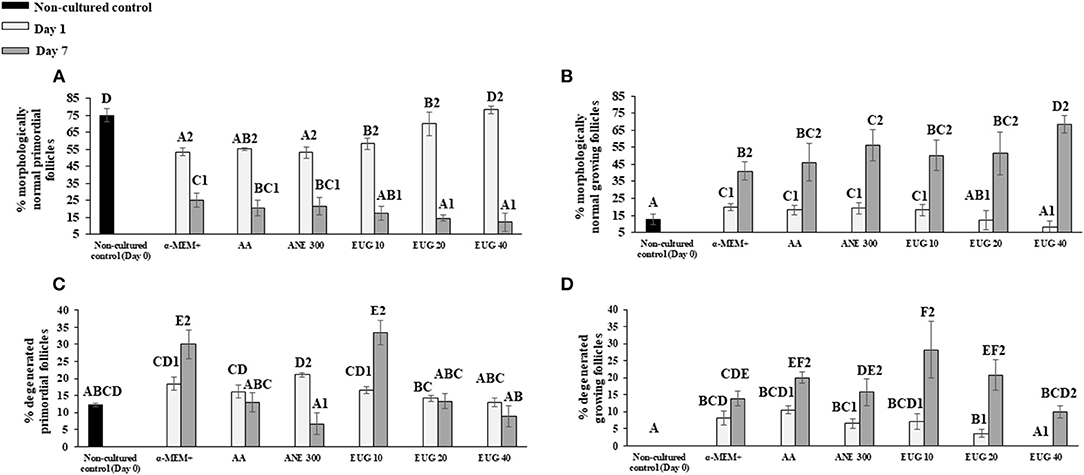
Figure 2. Mean (±SEM) percentage of normal (A,B) and degenerated (C,D) primordial and growing follicles in non-cultured ovarian tissue (control) and tissue cultured for 1 or 7 days in control medium with or without supplementation with ascorbic acid 50 μg/mL (AA), anethole 300 μg/mL (ANE 300), or eugenol at 10 (EUG 10), 20 (EUG 20) or 40 μM/mL (EUG 40). A−FDiffers significantly among treatments (P ≤ 0.05). 1−2Differs significantly between days of culture within the same treatments (P ≤ 0.05).
In vitro Growth of Preantral Follicles and Oocytes
The follicular and oocyte diameters in the non-cultured control treatment and after 1 or 7 days of culture are shown in Tables 3, 4, respectively. When compared to the non-cultured control, smaller follicular diameters were obtained when cultured in the presence of MEM alone (primordial—day 7, intermediate—days 1 and 7) or supplemented with AA (primordial and primary—day 7, and intermediate—days 1 and 7), ANE 300 and EUG 10 (primordial, intermediate and primary—day 7), EUG 20 (primordial, intermediate and primary—days 1 and 7) and EUG 40 (primordial—day 1). Moreover, the intermediate follicles from the EUG 40 presented a greater diameter than the non-cultured control group (Table 3). Regarding the oocyte diameter, regardless of the follicular category and the day of culture, the lowest diameters were obtained in groups grown in vitro in the absence or presence of antioxidants, except for the EUG 40 treatment. In this treatment, regardless of the follicular category, oocyte diameter was significantly higher than in the non-cultured control (Table 4). In general, in the tested treatments, a reduction in follicular and oocyte diameter was observed from day 1 to day 7 only in primordial and intermediate follicles. However, the EUG 40 treatment was the only treatment in which both follicular and oocyte diameters were increased significantly throughout the culture, regardless of the follicular category (Tables 3, 4). When compared to MEM, after 7 days of culture, except for the primordial follicle category in AA treatment, the addition of AA, ANE and EUG (regardless of the concentration) resulted in primordial, intermediate and primary follicles with significantly greater diameters. Concerning oocyte diameter, the addition of AA (only for intermediate follicle), ANE (primordial and intermediate follicles), EUG 10 and 20 (only for intermediate follicles) and EUG 40 (primordial, intermediate and primary follicles) significantly increased oocyte diameter after 7 days of culture. When the tested antioxidants were compared, except for the oocyte diameter of primary follicles, greater follicular and oocyte diameters were observed after 7 days of culture when EUG at 40 μM was added to the culture medium. Considering primary follicles, after 7 days of culture, a greater oocyte diameter was observed in the EUG 40 treatment when compared to the AA treatment (P < 0.05).
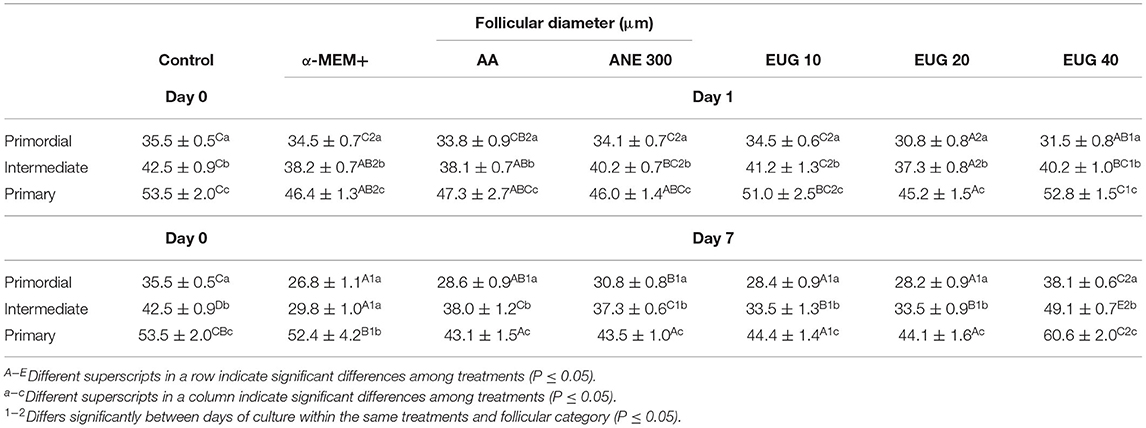
Table 3. Mean (±SEM) follicular in non-cultured ovarian tissue (control) and tissue cultured for 1 or 7 days in control medium without or with ascorbic acid 50 μg/mL (AA), anethole 300 μg/mL (ANE 300) or eugenol at 10 (EUG 10), 20 (EUG 20) or 40 μM/mL (EUG 40).
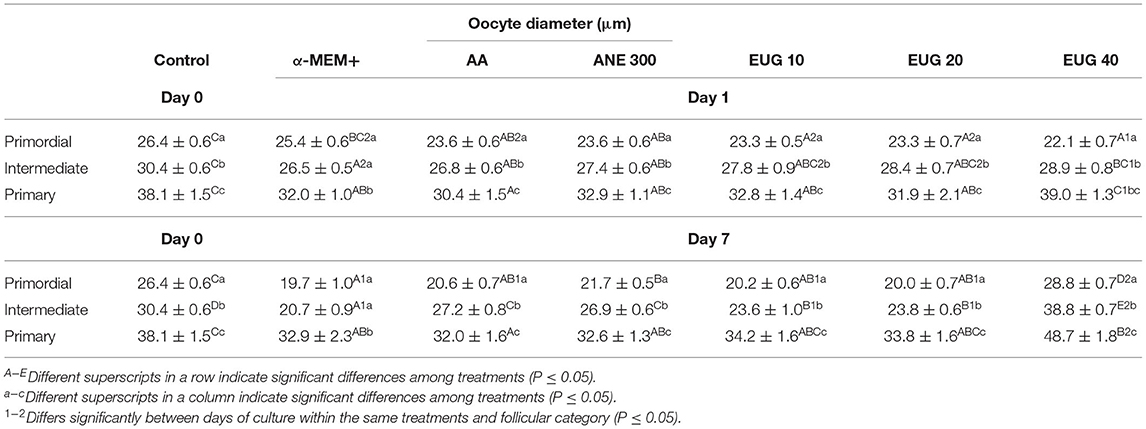
Table 4. Mean (±SEM) oocyte diameter in non-cultured ovarian tissue (control) and tissue cultured for 1 or 7 days in control medium without or with ascorbic acid 50 μg/mL (AA), anethole 300 μg/mL (ANE 300) or eugenol at 10 (EUG 10), 20 (EUG 20) or 40 μM/mL (EUG 40).
In the present study, the follicular and oocyte diameters were compared among the follicular categories, i.e., primordial, intermediate and primary follicles within the same treatment and day of culture (Table 3). Except for the MEM treatment, when similar (P < 0.05) follicular (day 7) and oocyte (days 1 and 7) diameters were observed between primordial and intermediate follicles, a significant and progressive increase in follicular and oocyte diameter was observed from the primordial to the primary follicle category both in the non-cultured control and in the cultured treated groups, regardless of the day of culture.
Assessment of Follicle Viability After Culture
Follicles were classified as viable (Figure 1H) or non-viable (Figure 1I) if they were negatively or positively stained with trypan blue, respectively. After 7 days of culture, the percentages of viable follicles were similar among MEM (68.6%) ANE 300 (75.0%), AA (65.7%), and EUG 40 (83.9%) treatments. However, lower rates of viable follicles were observed in MEM and AA treatments than in the non-cultured control (88.2%) (Figure 3).
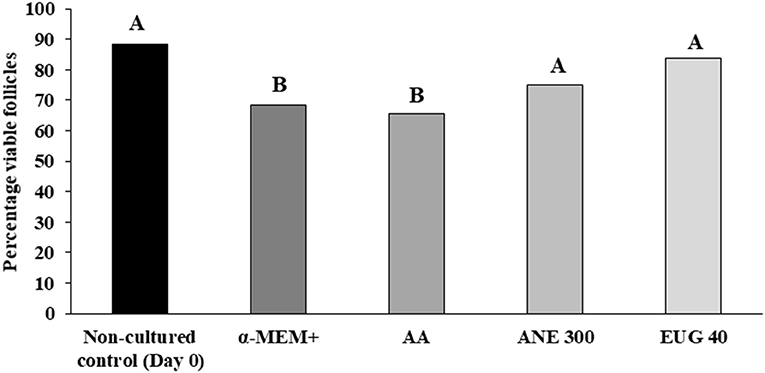
Figure 3. Percentages of viable goat preantral follicles in the non-cultured control group and after 7 days in control medium with or without ascorbic acid 50 μg/mL (AA), anethole 300 μg/mL (ANE 300) or eugenol 40 μM /mL (EUG 40). A, BDifferent superscripts in a row indicate significant differences among treatments (P ≤ 0.05).
Antioxidants Test
The quantification of H2O2 production and thiol groups was performed after 7 days of culture (Figure 4). In all treatments, H2O2 production was similar, except when EUG 40 was added to the medium, which resulted in a decrease in H2O2 production. No differences were observed when comparing thiol levels.
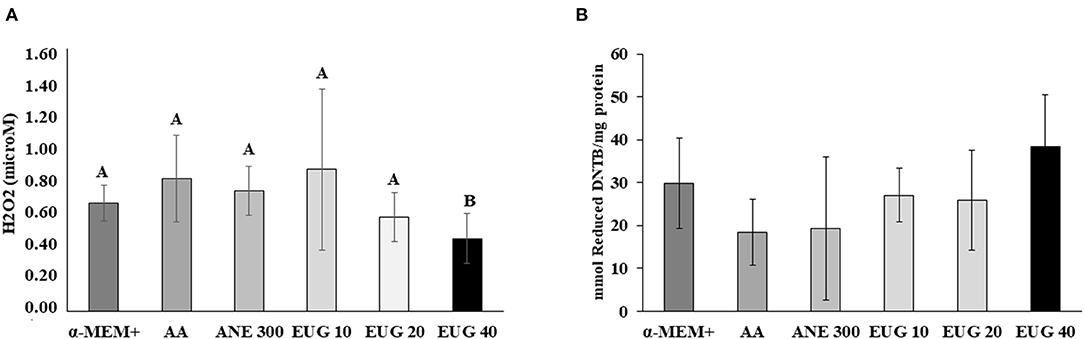
Figure 4. Mean (±SEM) levels of H2O2 production [microM; (A)] and reactive protein thiol (mmol reduced DTNB/mg protein) (B) in ovarian tissue cultured for 7 days. A, BDifferent superscripts in a row indicate significant differences among treatments (P ≤ 0.05).
The neutralizing capacity against ABTS and DPPH on day 7 is shown in Figure 5. All tested antioxidants, except ANE 300, were able to significantly decrease the levels of ABTS and DPPH when compared to the control. The highest capacity to neutralize free radicals was observed with EUG 40, whereas AA was similar to EUG 20 and 40.
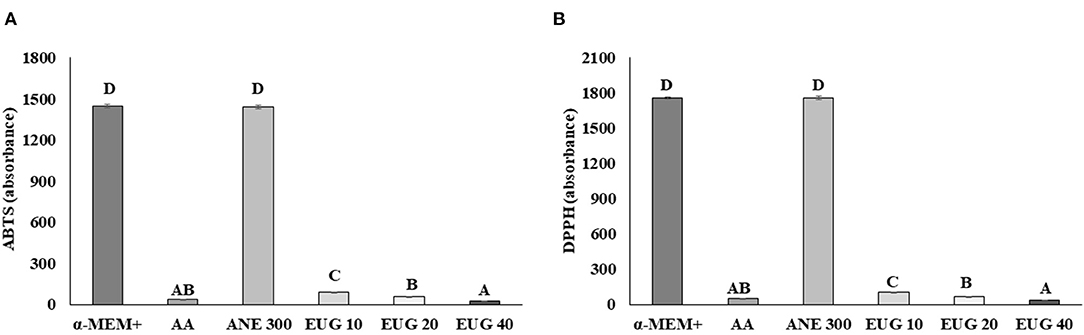
Figure 5. Mean (±SEM) reduction of ABTS (A) and DPPH free radical (B) levels after exposure to culture medium without or with supplementation with ascorbic acid 50 μg/mL (AA), anethole 300 μg/mL (ANE 300) or eugenol 40 μM /mL (EUG 40). Culture medium collected at day 7 of culture. A−DValues with different superscripts differ among treatments (P ≤ 0.05).
Immunofluorescence Detection of H3K4me3 and Calreticulin
Representative staining images of H3K4me3 (Figures 6A–F) and calreticulin (Figures 6G–L) follicles after 7 days of culture are shown. The labeling intensity of H3K4me3 after 7 days of in-vitro culture is shown in Figure 6M. All cultured tissues presented a similar intensity of H3K4me3 labeling, regardless of the follicular class, except when the ovarian tissue was cultured in the presence of EUG 40, resulting in a significant decrease of H3K4me3 labeling. All ovarian tissues cultured in the presence of antioxidants, especially EUG 40, presented a significant decrease in H3K4me3 labeling.
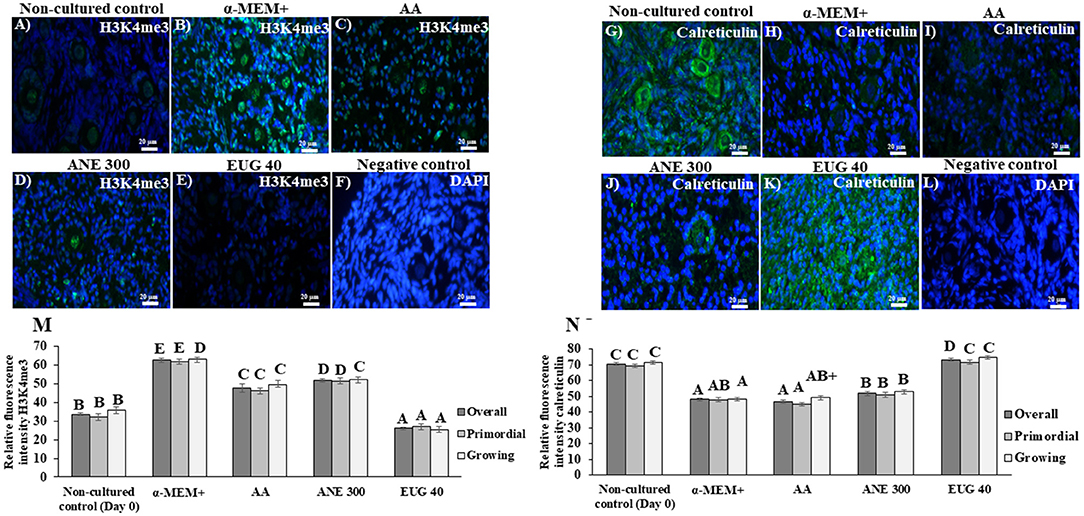
Figure 6. Immunofluorescence results showing the mean (±SEM) levels of H3K4me3 (A–E) and calreticulin (G–K), negative control with DAPI (F,L), quantification of H3K9me3 (M) and of calreticulin (N) signals in non-cultured ovarian tissue and tissue cultured for 7 days in medium without or with ascorbic acid 50 μg/mL (AA), anethole at 300 μg/mL (ANE 300) or eugenol 40 μM/mL (EUG 40). (Scale bar = 20 μm). A−FDifferent superscripts indicate significant differences among treatments (P ≤ 0.05). +Differs significantly between follicular category within in the same treatments (P ≤ 0.05).
Figure 6N shows the labeling intensity for calreticulin after 7 days of in-vitro culture. Only culturing of primordial and growing follicles in the presence of EUG 40 resulted in a labeling intensity similar to that observed in the non-cultured tissue. When compared to cultured control tissues, both EUG 40 and ANE 300 significantly increased the labeling intensity of calreticulin in growing follicles. Such effect was observed in all follicular categories when EUG 40 was added to the culture medium.
Relative Gene Expression
Figure 7 depicts the mRNA expression of the markers CAT (7A), ERP29 (7B), KDM1AX1 (7C) and KDM3A (7D). The mRNA expression of CAT was not affected by the treatments, whereas the mRNA relative expression of ERP29 and KDM3A was significantly increased when the culture medium was supplemented with EUG 40. The mRNA relative expression of KDM1AX1 was significantly increased when the culture medium was supplemented with EUG 40 or ANE 300.
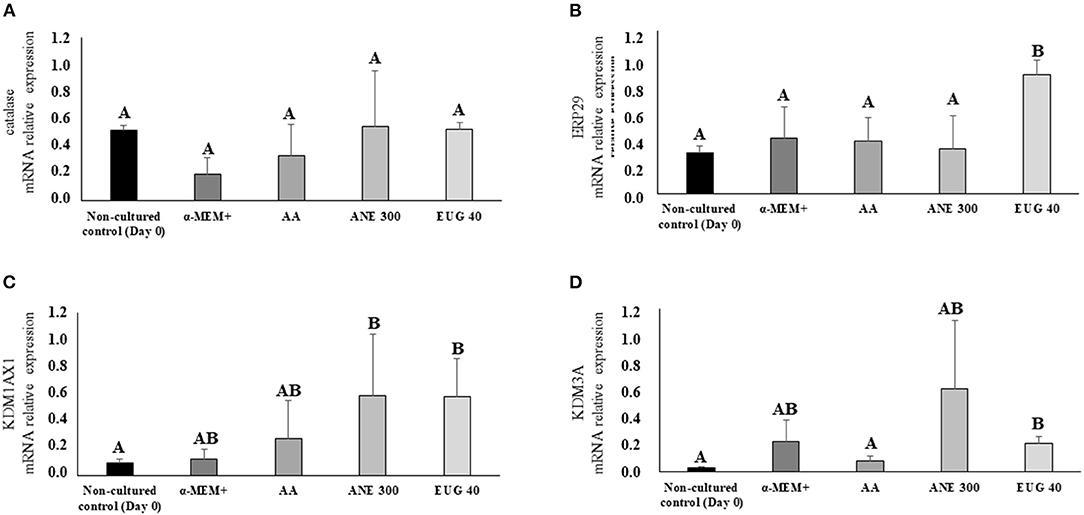
Figure 7. Mean (±SEM) relative mRNA expression of genes involved in cellular stress [CAT (A) and ERP29 (B)] and methylation [KDM1AX1 (C) and KDM3A (D)] in goat preantral follicles in the non-cultured control group and after 7 days in control medium without or with supplementation of 50 μg/mL of ascorbic acid (AA) or anethole at 300 μg/mL (ANE 300) or eugenol 40 ng/mL (EUG 40). A, BDifferent superscripts indicate significant differences among treatments (P ≤ 0.05).
Discussion
The present study investigated the effects of eugenol (10, 20, and 40 uM), ascorbic acid (50 μg/mL) and anethole (300 μg/mL) on the in-vitro culture of caprine preantral follicles enclosed in ovarian tissue. In general, EUG 40 improved follicle survival, activation, and both follicular and oocyte growth and decreased ROS production and histone methylation in ovarian tissue after 7 days of culture.
During the first day of culture, only EUG 40 maintained follicular survival similar to non-culturedtissue, regardless of the follicular class, whereas ANE 300 maintained the morphology of intermediate follicles. Regardless of the treatment, 7-day in-vitro culture decreased the survival rates of the primordial, intermediate and growing follicles, except for ANE 300, which preserved the morphology of primordial and primary follicles. Only medium supplementation with EUG 40 or ANE 300 enhanced the survival rates of growing follicles. In-vitro culture of ovarian follicles enclosed in ovarian tissue requires a medium that supports the maintenance of the ovarian cortex, because stromal cells support the follicular development (29, 30). Sá et al. (10) demonstrated that anethole can support the maintenance of follicular morphology after 7 days of culturing by decreasing the ROS production. The antioxidant capacity of eugenol was confirmed by its ability to decrease the H2O2 production. Eugenol not only decreases the excess of ROS (24) but also enhances the activity of enzymatic antioxidants (25). Ighodaro and Akinloye (31) showed that eugenol mitigates the effects of H2O2 via the regulation of glutathione peroxidase levels. Furthermore, eugenol inhibits iron oxidation, regulating superoxide and hydroxyl levels (22, 23). In the present study, eugenol was able to decrease the levels of both ABTS and DPPH after a 7-day culture, indicating that this antioxidant has a long-term effect in culture. Chemically, eugenol is more stable and has a more long-term activity than anethole because of the presence of a –OH group in its aromatic ring (24). Such chemical stability is also found in ascorbic acid, and its antioxidant activity was previously compared with that of eugenol (32). However, when culturing ovarian tissue, we could not observe similar effects when comparing both antioxidants.
The mRNA relative expression of ERP29 and the increased expression of the protein calreticulin were observed in the tissue cultured in the presence of EUG 40, indicating that this antioxidant was protecting the follicles from ER stress. This was confirmed by a decrease in ROS production. The EPR29 regulates the connexin 43 in the Golgi complex, stabilizing these monomers (33). Connexins are membrane proteins that play a role in intercellular communication, allowing the transfer of ions, such as AMPc (34, 35). Tokuhiro et al. (36) demonstrated that calreticulin regulates the folding of the proteins Growth and Differentiation Factor 9 (GDF9) and Bone Morphogenetic Protein 15 (BMP15) in the ER. Both GDF9 and BMP15 are involved in the survival and growth of oocytes and follicles (17, 37, 38).
The addition of EUG 40 to the control medium improved follicular development after a 7-day culture. Besides the ability of eugenol to stimulate the production of antioxidant substances (glutathione peroxidase, superoxide dismutase and glutathione-S-transferase), this compound also enhances the synthesis of the protein zona occludens (39) and decreases the expression of interleukin-6 (IL-6) and Tumor Necrosis Factor-alpha (TNF-alpha) (21). The zona occludens proteins are present in developing follicles, favoring the antrum formation and the subsequent follicular development (36). Silva et al. (40) showed that during the initial folliculogenesis, TNF-α increases the number of apoptotic cells in cultured bovine ovarian tissue, and Manabe et al. (41) observed an impaired survival of primordial follicles.
The H3 is one of the constituents of the nucleosome protecting the DNA; the methylation of these proteins is associated with a decreased or increased transcription (42). In the present study, we evaluated the expression of markers for the two enzymes lysine demethylase, responsible for the removal of methyl radicals from the H3, KDM3A, and KDM1AX1. The KDM3A decreases gene silencing, including those related to the inhibition of primordial follicle activation and the induction of apoptosis (43–45). The relative mRNA expression levels for KDM3A and KDM1AX1 were increased in anethole and EUG 40 treatments when compared to the non-cultured control. However, EUG 40 was the only treatment that decreased H3K4me3 expression. Therefore, we suggest that the higher rates of follicle survival and activation observed in the EUG 40 may be explained by the silencing of pro-apoptotic genes as well as genes related to the maintenance of primordial follicle quiescence. To date, studies have shown that decreased H3K4me3 levels lead to a reduction in the expression levels of pro-apoptotic genes [Bax, Bad and Bid (44, 45)].
In conclusion, eugenol at 40 μM improved follicle survival, regardless of the follicular class, at day 1 of culture. At day 7, EUG 40 was the most efficient supplement to improve the survival of growing follicles and protected the ovarian tissue against ROS, ER stress as well as histone lysine methylation.
Data Availability Statement
The original contributions presented in the study are included in the article/supplementary material, further inquiries can be directed to the corresponding author.
Author Contributions
RSi, SM, AR, and JF: conceptualization. RSi, LL, AF, AS, DA, BA, and AO: methodology. RSi, RSa, and JF: validation and writing—original draft preparation. RSi, RSa, BA, and JF: formal analysis. RSi, LL, AF, AS, DA, and BA: investigation. AO, SM, AR, and JF: resources. RSi, RSa, AO, BA, and JF: writing—review and editing. RSi: project administration. JF: funding acquisition. All authors have read and agreed to the published version of the manuscript.
Conflict of Interest
The authors declare that the research was conducted in the absence of any commercial or financial relationships that could be construed as a potential conflict of interest.
Publisher's Note
All claims expressed in this article are solely those of the authors and do not necessarily represent those of their affiliated organizations, or those of the publisher, the editors and the reviewers. Any product that may be evaluated in this article, or claim that may be made by its manufacturer, is not guaranteed or endorsed by the publisher.
References
1. Van Der Hurk R, Zhao J. Formation of mammalian oocytes and their growth, differentiation and maturation within ovarian follicles. Theriogenology. (2005) 63:1717–51. doi: 10.1016/j.theriogenology.2004.08.005
2. Figueiredo JR, Rodrigues AP, Silva JR, Santos RR. Cryopreservation and in vitro culture of caprine preantral follicles. Reprod Fertil Dev. (2011) 23:40–7. doi: 10.1071/RD10227
3. Figueiredo JR, Cadenas J, Lima LF, Santos RR. Advances in vitro folliculogenesis in domestic ruminants. Anim Reprod. (2019) 16:52–65. doi: 10.21451/1984-3143-AR2018-0123
4. Picton HM, Harris SE, Muruvi W, Chambers EL. The in vitro growth and maturation of follicles. Reproduction. (2008) 136:703–15. doi: 10.1530/REP-08-0290
5. Xu G, Lin S, Law WC, Roy I, Lin X, Mei S, et al. The invasion and reproductive toxicity of QDs-Transferrin bioconjugates on preantral follicles in vitro. Theranostics. (2012) 2:734–45. doi: 10.7150/thno.4290
6. Silva TES, de Brito DCC, de Sá NAR, da Silva RF, Ferreira ACA, da Silva JYG, et al. Equol: a microbiota metabolite able to alleviate the negative effects of zearalenone during in vitro culture of ovine preantral follicles. Toxins. (2019) 11:652. doi: 10.3390/toxins11110652
7. Brito DCC, Silva IP, Ferreira ACA, Sá NAR, Guedes MIF, Rodrigues APR, et al. Effects of in vitro exposure of sheep ovarian tissue to zearalenone and matairesinol on preantral follicles. Zygote. (2021) 25:1–4. doi: 10.1017/S0967199421000794
8. Telfer EE, Binnie JP, Mc Caffery FH, Campbell BK. In vitro development of oocytes from porcine and bovine primary follicles. Mol Cell Endocrinol. (2000) 163:117–23. doi: 10.1016/S0303-7207(00)00216-1
9. Rossetto R, Lima-Verde IB, Matos MH, Saraiva MV, Martins FS, Faustino LR, et al. Interaction between ascorbic acid and follicle-stimulating hormone maintains follicular viability after long-term in vitro culture of caprine preantral follicles. Domest Anim Endocrinol. (2009) 37:112–23. doi: 10.1016/j.domaniend.2009.04.003
10. Sá NAR, Bruno JB, Guerreiro DD, Cadenas J, Alves BG, Cibin FWS, et al. Anethole reduces oxidative stress and improves in vitro survival and activation of primordial follicles. Braz J Med Biol Res. (2018) 51:e7129. doi: 10.1590/1414-431x20187129
11. Lonergan P, Fair T. Maturation of oocytes in vitro. Annu Rev Anim Biosci. (2016) 4:255–68. doi: 10.1146/annurev-animal-022114-110822
12. Zhu J, Moawad AR, Wang CY Li HF, Ren JY Dai YF. Advances in in vitro production of sheep embryos. Int J Vet Sci Med. (2018) 6:15–26. doi: 10.1016/j.ijvsm.2018.02.003
13. He L, He T, Farrar S, Ji L, Liu T, Ma X. Antioxidants maintain cellular redox homeostasis by elimination of reactive oxygen species. Cell Physiol Biochem. (2017) 44:532–53. doi: 10.1159/000485089
14. Shaban S, El-Husseny MWA, Abushouk AI, Salem AMA, Mamdouh M, Abdel-Daim MM. Effects of antioxidant supplements on the survival and differentiation of stem cells. Oxid Med Cell Longev. (2017) 2017:5032102. doi: 10.1155/2017/5032102
15. Torres-Ramírez N, Ortiz-Hernández R, Escobar-Sánchez ML, Echeverría-Martínez OM, Vázquez-Nin GH. Endoplasmic reticulum stress during mammalian follicular atresia. Endoplasmic Reticulum Intech Open. (2019). doi: 10.5772/intechopen.82687
16. Yu XX, Liu YH, Liu XM, Wang PC, Liu S, Miao JK, et al. Ascorbic acid induces global epigenetic reprogramming to promote meiotic maturation and developmental competence of porcine oocytes. Sci Rep. (2018) 8:1–12. doi: 10.1038/s41598-018-24395-y
17. Saeedabadi S, Abazari-Kia AH, Rajabi H, Parivar K, Salehi M. Melatonin improves the developmental competence of goat oocytes. Int J Fertil Steril. (2018) 12:157. doi: 10.22074/ijfs.2018.5204
18. Liu M, Kun Z, Tian-min Xu. The role of BMP15 and GDF9 in the pathogenesis of primary ovarian insufficiency. Hum Fertil. (2019) 24:325–32. doi: 10.1080/14647273.2019.1672107
19. Seneda MM, Godmann M, Murphy BD, Kimmins S, Bordignon V. Developmental regulation of histone H3 methylation at lysine 4 in the porcine ovary. Reproduction. (2008) 135:829–38. doi: 10.1530/REP-07-0448
20. Sha QQ, Jiang Y, Yu C, Xiang Y, Dai XX, Jiang JC, et al. CFP1-dependent histone H3K4 trimethylation in murine oocytes facilitates ovarian follicle recruitment and ovulation in a cell-nonautonomous manner. Cell Mol Life Sci. (2020) 77:2997–012. doi: 10.1007/s00018-019-03322-y
21. Mateen S, Rehman MdT, Shahzad S, Naeem SS, Faizy AF, Khand AQ, et al. Antioxidant and anti-inflammatory effects of cinnamaldehyde and eugenol on mononuclear cells of rheumatoid arthritis patients. Eur J Pharmacol. (2019) 852:14–24. doi: 10.1016/j.ejphar.2019.02.031
22. Marchese A, Barbieri R, Coppo E, Orhan IE, Daglia M, Nabavi SF, et al. Antimicrobial activity of eugenol and essential oils containing eugenol: a mechanistic viewpoint. Crit Rev Microbiol. (2017) 43:668–89. doi: 10.1080/1040841X.2017.1295225
23. Wie MB, Won MH, Lee KH, Shin JH, Lee JC, Suh HW, et al. Eugenol protects neuronal cells from excitotoxic and oxidative injury in primary cortical cultures. Neurosci Lett. (1997) 225:93–6. doi: 10.1016/S0304-3940(97)00195-X
24. Silva FFM, Monte FJQ, Lemos TLG, Nascimento PGG, Medeiros CAK, Paiva LMM. Eugenol derivatives: synthesis, characterization, and evaluation of antibacterial and antioxidant activities. Chem Cent J. (2018) 12:1–9. doi: 10.1186/s13065-018-0407-4
25. Vasconcelos EM, Costa FC, Azevedo AVN, Barroso PAA, De Assis EIT, Paulino LRFM, et al. Eugenol influences the expression of messenger RNAs for superoxide dismutase and glutathione peroxidase 1 in bovine secondary follicles cultured in vitro. Zygote. (2021) 29:301–6. doi: 10.1017/S0967199420000908
26. Silva JRV, Lucci CM, Carvalho FCA, Bao SN, Costa SHF, Santos RR, et al. Effect of coconut water and Braun-Collins solutions at different temperatures and incubation times on the morphology of goat preantral follicles preserved in vitro. Theriogenoloy. (2000) 54:809–22. doi: 10.1016/S0093-691X(00)00392-7
27. Rodrigues APR, Costa SHF, Santos RR, Amorim CA, Lucci CM, Báo SN, et al. In vitro culture of cryopreserved caprine ovarian tissue pieces and isolated follicles. Cell Preserv Technol. (2006) 4:290–98. doi: 10.1089/cpt.2006.9998
28. Pfaffl MW. A new mathematical model for relative quantification in real-time RT-PCR. Nucleic Acids Res. (2001) 29:e45. doi: 10.1093/nar/29.9.e45
29. Donfack NJ, Alves KA, Alves BG, Rocha RMP, Bruno JB, Bertolini M, et al. Stroma cell-derived factor 1 and connexins (37 and 43) are preserved after vitrification and in vitro culture of goat ovarian cortex. Theriogenology. (2018) 116:83–8. doi: 10.1016/j.theriogenology.2018.05.001
30. Faustino LR, Santos RR, Silva CMG, Pinto LC, Celestino JJH, Campelo CC, et al. Goat and sheep ovarian tissue cryopreservation: Effects on the morphology and development of primordial follicles and density of stromal cell. Anim Reprod Sci. (2010) 122:90–7. doi: 10.1016/j.anireprosci.2010.08.001
31. Ighodaro OM, Akinloye AO. First line defence antioxidants-superoxide dismutase (SOD), catalase (CAT) and glutathione peroxidase (GPX): Their fundamental role in the entire antioxidant defence grid. Alex J Med. (2018) 54:287–93. doi: 10.1016/j.ajme.2017.09.001
32. Kim DY, Won KJ, Hwang DI, Park SM, Kim B, Lee HM. Chemical composition, antioxidant and anti-melanogenic activities of essential oils from Chrysanthemum boreale Makino at different harvesting stages. Chem Biodivers. (2018) 15:170–06. doi: 10.1002/cbdv.201700506
33. Das S, Smith TD, Sarma JD, Ritzenthaler JD, Maza J, Kaplan BE, et al. ERp29 restricts Connexin43 oligomerization in the endoplasmic reticulum. Mol Biol Cell. (2009) 20:2593–604. doi: 10.1091/mbc.e08-07-0790
34. Han C, Liu B, Yang T, Yang Y, Xu J, Wei Z, et al. Involvement of PKCε in FSH-induced connexin43 phosphorylation and oocyte maturation in mouse. Biol Open. (2018) 7:bio034678. doi: 10.1242/bio.034678
35. Bus A, Szymańska-Vandendriessche K, Pintelon I, Leroy JLMR, Leybaert L, Bols PEJ. Preservation of connexin 43 and transzonal projections in isolated bovine pre-antral follicles before and following vitrification. J Assist Reprod Genet. (2021) 38:479–92. doi: 10.1007/s10815-020-01993-2
36. Tokuhiro K, Satouh Y, Nozawa K, Isotani A, Fujihara Y, Hirashima Y, et al. Calreticulin is required for development of the cumulus oocyte complex and female fertility. Sci Rep. (2015) 5:14254. doi: 10.1038/srep14254
37. Silva JRV, Tharasanit T, Taverne MAM, Van der Weijden GC, Santos RR, Figueiredo JR, et al. The activin-follistatin system and in vitro early follicle development in goats. J Endocrinol. (2006) 189:113–25. doi: 10.1677/joe.1.06487
38. Ahmad HI, Liu G, Jiang X, Edallew SG, Wassie T, Tesema B, et al. Maximum-likelihood approaches reveal signatures of positive selection in BMP15 and GDF9 genes modulating ovarian function in mammalian female fertility. Ecol Evol. (2017) 7:8895–902. doi: 10.1002/ece3.3336
39. Hui Q, Ammeter E, Liu S, Yang R, Lu P, Lahaye L, et al. Eugenol attenuates inflammatory response and enhances barrier function during lipopolysaccharide-induced inflammation in the porcine intestinal epithelial cells. J Anim Sci. (2020) 98:245. doi: 10.1093/jas/skaa245
40. Silva AWB, Ribeiro RP, Menezes VG, Barberino RS, Passos JRS, Dau AMP, et al. Expression of TNF-αsystem members in bovine ovarian follicles and the effects of TNF-α or dexamethasone on preantral follicle survival, development and ultrastructure in vitro. Anim Reprod Sci. (2017) 182:56–68. doi: 10.1016/j.anireprosci.2017.04.010
41. Manabe N, Matsuda-Minehata F, Goto Y, Maeda A, Cheng Y, Nakagawa S, et al. Role of cell death ligand and receptor system on regulation of follicular atresia in pig ovaries. Reprod Domest Anim. (2008) 43:268–72. doi: 10.1111/j.1439-0531.2008.01172.x
43. Howe FS, Fischl H, Murray SC, Mellor J. Is H3K4me3 instructive for transcription activation? Bioessays. (2017) 39:1–12. doi: 10.1002/bies.201600095
44. Lu C, Yang D, Sabbatini ME, Aaron H, Colby MW, Grinstaff NH, et al. Contrasting roles of H3K4me3 and H3K9me3 in regulation of apoptosis and gemcitabine resistance in human pancreatic cancer cells. BMC Cancer. (2018) 18:149. doi: 10.1186/s12885-018-4061-y
Keywords: goat, in-vitro culture, eugenol, antioxidant, preantral follicles
Citation: Silva RF, Lima LF, Ferreira ACA, Silva AFB, Alves DR, Alves BG, Oliveira AC, Morais SM, Rodrigues APR, Santos RR and Figueiredo JR (2022) Eugenol Improves Follicular Survival and Development During in vitro Culture of Goat Ovarian Tissue. Front. Vet. Sci. 9:822367. doi: 10.3389/fvets.2022.822367
Received: 25 November 2021; Accepted: 04 April 2022;
Published: 28 April 2022.
Edited by:
Sebastián Demyda-Peyrás, Universidad Nacional de La Plata, ArgentinaReviewed by:
Ayman Abdel-Aziz Swelum, Zagazig University, EgyptRolando Pasquariello, University of Milan, Italy
Copyright © 2022 Silva, Lima, Ferreira, Silva, Alves, Alves, Oliveira, Morais, Rodrigues, Santos and Figueiredo. This is an open-access article distributed under the terms of the Creative Commons Attribution License (CC BY). The use, distribution or reproduction in other forums is permitted, provided the original author(s) and the copyright owner(s) are credited and that the original publication in this journal is cited, in accordance with accepted academic practice. No use, distribution or reproduction is permitted which does not comply with these terms.
*Correspondence: R. F. Silva, anJmLmxhbW9mb3BhcGFwZXJzQGdtYWlsLmNvbQ==