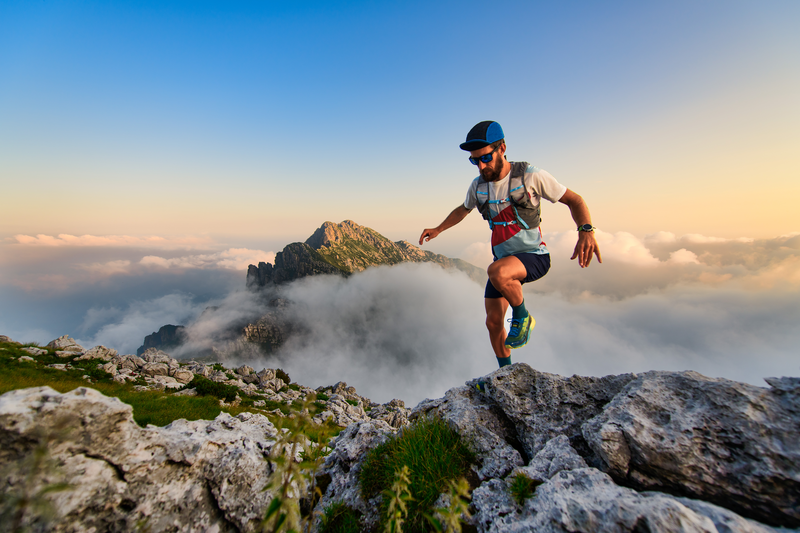
94% of researchers rate our articles as excellent or good
Learn more about the work of our research integrity team to safeguard the quality of each article we publish.
Find out more
ORIGINAL RESEARCH article
Front. Vet. Sci. , 10 March 2022
Sec. Veterinary Infectious Diseases
Volume 9 - 2022 | https://doi.org/10.3389/fvets.2022.816135
Bovine paratuberculosis (PTB) is a chronic enteritis caused by Mycobacterium avium subspecies paratuberculosis (Map) that causes a heavy economic impact worldwide. Map infected animals can remain asymptomatic for years while transmitting the mycobacteria to other members of the herd. Therefore, accurate detection of subclinically infected animals is crucial for disease control. In a previous RNA-Seq study, we identified several mRNAs that were overexpressed in whole blood of cows with different PTB-associated histological lesions compared with control animals without detected lesions. The proteins encoded by two of these mRNAs, ATP binding cassette subfamily A member 13 (ABCA13) and Matrix Metallopeptidase 8 (MMP8) were significantly overexpressed in whole blood of animals with focal histological lesions, the most frequent pathological form in the subclinical stages of the disease. In the current study, the potential of sensitive early diagnostic tools of commercial ELISAs, based on the detection of these two biomarkers, was evaluated in serum samples of 704 Holstein Friesian cows (566 infected animals and 138 control animals from PTB-free farms). For this evaluation, infected animals were classified into three groups, according to the type of histological lesions present in their gut tissues: focal (n = 447), multifocal (n = 59), and diffuse (n = 60). The ELISA based on the detection of ABCA13 was successfully validated showing good discriminatory power between animals with focal lesions and control animals (sensitivity 82.99% and specificity 80.43%). Conversely, the MMP8-based ELISA showed a poor discriminatory power between the different histological groups and non-infected controls. The ABCA13-based ELISA showed a higher diagnostic value (0.822) than the IDEXX ELISA (0.517), the fecal bacterial isolation (0.523) and the real-time PCR (0.531) for the detection of animals with focal lesions. Overall, our results indicate that this ABCA13 ELISA greatly improves the identification of subclinically infected animals with focal lesions that are undetectable using current diagnostic methods.
Bovine paratuberculosis (PTB) or Johne's disease (JD) is a chronic contagious and debilitating enteritis of domestic and wild ruminants caused by Mycobacterium avium subspecies paratuberculosis (Map). PTB produces important economic losses in dairy herds worldwide due to increase in mortality, decrease in milk production, weight loss, premature culling and reduced slaughter value (1–4). PTB has also been related to reduced fertility rates (5, 6) and increased susceptibility to other diseases, particularly mammary infections (7). The importance of this disease would be even greater when considering its zoonotic potential and the risk of transmission of viable Map through pasteurized milk and milk products (8–10). The association of Map with human diseases like Crohn's disease (CD), type I diabetes (T1D), multiple sclerosis (MS) or rheumatoid arthritis (RA) has been documented (11–16).
The main clinical signs of the disease are progressive weight loss, diarrhea, and decreased milk yield (17). Map typically enters a herd through the purchase of subclinically infected cattle shedding the bacteria with feces that contaminate the environment, although it can also enter through contaminated feces adhering to vehicles, equipment, and visitors. Access to contaminated pasture or water sources and contact with other ruminants may also be involved in the spread of the disease (18–20).
Different pathological forms associated with Map infection can be established: focal, multifocal and diffuse (21). Briefly, focal lesions consist of small scattered and well-demarcated granulomas composed by macrophages and few Langhans giant cells, mainly located in the jejunal and ileal lymph nodes and not affecting the intestinal lamina propria. Multifocal lesions consist of numerous well-demarcated granulomas in the intestinal lymphoid tissues and in the intestinal lamina propria. Diffuse lesions are characterized by extensive severe and diffuse granulomatous enteritis and lymphadenitis, that markedly alter the normal histological structure. According to the inflammatory cell type present in the infiltrate and the number of acid-fast bacilli (AFB), diffuse lesions were further subdivided into diffuse lymphoplasmocytic paucibacillary, diffuse intermediate and diffuse histocytic multibacillary lesions (22). Three epidemiopathogenic forms of the infection (apparently free, latent and patent) have been described according to the immunopathological classification, epidemiological aspects and diagnostic results (23). The latent forms show no clinical signs and are characterized by a low bacterial load, a predominant cell-mediated immune response and the presence of mild histological lesions in the intestine and associated lymph nodes. The patent forms are characterized by a variable degree of signs associated with advanced multifocal and diffuse lesions. Apparently free PTB animals are those negative by all diagnostic tests not showing any visible microscopic lesions.
Although vaccination has been shown to be an efficient tool (24, 25) and is widely practiced in some settings (26), most PTB control programs in cattle are based on testing and culling test-positive cows combined with good management practices (27). However, the efficiency of the control programs based on the “test and cull” policy is strongly conditioned by the diagnostic methods used to detect Map infection, the performance of which varies depending on the stage of the Map infection. Current diagnostic tests have low sensitivities to detect subclinical infections. Consequently, novel diagnostic tools with high sensitivity and specificity would improve the diagnostic efficacy necessary to control the disease. The potential of emerging -omic approaches to complement and enhance the diagnosis of Map infection in cattle has been previously reviewed (28) and point out the potential of host biomarkers as tools to develop novel diagnostic methods for PTB (29–34). In a previous study, whole RNA-sequencing (RNA-Seq) identified host genes differentially expressed in peripheral blood samples collected from animals with focal or diffuse lesions in gut tissues versus (vs.) control animals without any detected lesion (35). Genes encoding for bovine proteins MMP8 (Matrix metallopeptidase 8) and ABCA13 (ATP binding cassette subfamily A member 13) showed significantly higher expression in animals with focal lesions (log2 fold change 1.89 and 3.74, respectively), while genes encoding for FAM84A (family with sequence similarity 84 member A), SPARC (secreted protein acidic and cysteine rich) and desmin (DES) were up-regulated in animals showing diffuse lesions when compared to control animals (log2 fold change 2.15, 2.21 and 3.75, respectively). Subsequently, the diagnostic performance of commercial ELISAs based on the detection of those five blood candidate biomarkers to detect animals with focal, multifocal and diffuse lesions was studied (n = 155) and compared to that of conventional PTB diagnostic methods (IDEXX ELISA and feces and tissues real-time polymerase chain reaction (PCR) and bacteriological culture) (36). The results indicated that the biomarker-based ELISAs consistently showed higher sensitivity values than the conventional immunologic and microbiologic diagnostic methods. The ABCA13-based ELISA had the best diagnostic performance for global detection of animals with any type of lesions (69.41% sensitivity vs. 28.41%, 11.36%, and 25% of the IDEXX ELISA, fecal culture and fecal PCR, respectively) improving overall detection of animals with PTB-specific histological lesions. Most importantly, the ABCA13-based ELISA showed the most accurate diagnostic performance for detection of animals with focal lesions, the most frequent pathological form in the subclinical stages of the disease, with a 79.25% sensitivity vs. the 14.55%, 5.45%, and 9.09% sensitivities of the IDEXX ELISA, fecal culture and fecal PCR, respectively. The MMP8-based ELISA showed the highest diagnostic accuracy for global detection of animals with multifocal and diffuse lesions with a sensitivity of 96.97% vs. the 54.44%, 51.51%, and 21.21% of the IDEXX ELISA, fecal culture and fecal PCR, respectively.
ATP-binding cassette proteins are a superfamily of transporter proteins that play important physiological roles in living organisms and are vital for normal brain function (37–39). Through coupling the energy of ATP hydrolysis, they translocate solutes such as lipids, ions, peptides and xenobiotics through biological membranes (38, 40), but they also intervene in a wide variety of other processes such us signal transduction, protein secretion, drug and antibiotic resistance, antigen presentation and bacterial pathogenesis and sporulation (41). Within this superfamily of proteins, there is the subfamiliy ABCA, functionally characterized by shuttling lipid molecules across cell membranes (42). Disruption to ABC transporter activity results in lipid accumulation and an elevated level of inflammatory cytokines in lung tissue (43). One of the most polymorphic genes of this subfamily is ABCA13, with 165 alterations described (44). ABCA13 is expressed in several human tissues and is involved in several pathologic and physiologic processes in the human body (45). Thus, it has been associated with neurological disorders such as major depressive disorder (46), Lewy body dementia (47), schizophrenia and bipolar disorder (48), and autism spectrum disorder (49, 50). Conversely, ABCA13 has also been linked to cancer, sometimes associated with a poor prognosis (51–55) and on other occasions with a longer patient survival (56, 57). ABCA13 is also considered a useful marker for predicting lymph node metastasis in resected gastric cancer patients in the early stage (54). Among its related pathways are CDK-mediated phosphorylation and removal of Cdc6 and Innate Immune System. Gene Ontology (GO) annotations related to this gene include ATPase activity and cholesterol transporter activity. In cattle, the ABCA13 gene has been related to osteogenesis imperfecta, the origin of this disease being attributed to the additive effects of this gene with QRFPR and IFIM5 (58).
Matrix metalloproteinases (MMPs) are metal-dependent enzymes that degrade components of the extracellular matrix and other components such as receptors, growth factors, cytokines and chemokines (59–61) and that also play an important role as effective regulators of cell proliferation and differentiation, tissue homeostasis and immune response (62) via signal transduction in immune cell signaling (63). Some MMPs are related to cancer progression, metastasis and invasion (64, 65) through the induction of changes during the epithelial-mesenchymal transition in the tumor microenvironment (66). The MMPs family includes collagenases, gelatinases, stromelysins, membrane type MMPs (MT-MMPs), matrilysins and various other MMPs (67), whose activity is tightly regulated by a family of endogenous inhibitors termed as tissue inhibitors of metalloproteinases (TIMPs) (68). Twenty-eight forms of MMPs have been identified in vertebrates and 24 in humans (69). Among them, metalloproteinase 8 (MMP8) or neutrophil metalloproteinase, an enzyme released by neutrophils and by other cells of the non-polymorphonuclear lineage such as gingival fibroblasts, bone and plasma cells (70) and that breaks down type I, II, III, V, VII, VIII and X collagen, gelatin, aggrecan, elastin, fibronectin, laminin and nidogen (71) which plays an important role in cell proliferation, migration, and angiogenesis through the development of capillary-like network structures (72). MMP8 is involved in tumor survival and morbidity since its expression has been associated with pro- and anti-inflammatory effects in different types of tumor microenvironments (73). Thus, sometimes it is related to a worse outcome of certain cancers (72, 74–76) although some authors defend its protective role (77). There is evidence suggesting that Map can induce the expression of MMPs, which are the main proteases in the pathogenesis of inflammatory bowel disease (78). TIMSPs have been suggested as potential biomarkers for tuberculosis (TB). TIMPs-1,−2 and−3 facilitate remodeling and repair of tissue following destruction by MMPs. For instance, the concentration of MMP8 has been demonstrated to decrease rapidly during TB treatment (79). MMP9 and TIMP1 are known to be up-regulated in TB infection and have been proposed as biomarkers for diagnosis of TB (80).
Two candidate biomarkers, ABCA13 and MMP8, were selected for further validation, based on the results obtained in the RNA-SEQ analysis (35) and in the pre-validation study (36). The aim of the present study was to carry out a robust validation of the diagnostic potential of ELISAs based on detection of ABCA13 and MMP8, using reference serum samples from well characterized animals, classified according to the different pathological forms associated with Map infection [focal (n = 447), multifocal (n = 59), and diffuse (n = 60)], and control animals from PTB-free farms (n = 138).
A total of 704 Holstein-Friesian cows, with an average age of 5.2 years ranging from 0.5 to 13.4, were included in this study. The positive reference group consisted of 566 cows from 8 different regions of Spain (Basque Country, Catalonia, Navarra, Aragón, Cantabria, Castilla y León, La Rioja and Asturias) commercially slaughtered in five abattoirs located in the Basque Country and Asturias (23, 36, 81). The negative reference group consisted of 138 live cows from 4 PTB-free farms of Asturias.
Samples of blood and feces were collected from all the animals while tissue samples (ileocecal valve, caudal ileum, and caudal jejunal and ileal lymph nodes) were only taken from the slaughtered animals, in situ after evisceration either in the Asturian abattoirs or in the necropsy room at NEIKER. Tissue samples were split for molecular, microbiological, and histopathological analysis.
Additionally, in order to assess the analytical specificity of the ABCA13-based ELISA in relationship with other mycobacterial infections, two sets of samples from animals infected with Mycobacterium bovis, the causative etiological agent of TB, were used. One bovine set consisted of 30 samples from experimentally infected cows, 10 sera collected at 8- and 12-weeks post-infection and 20 plasma samples taken at 16 weeks post-infection. The other set consisted of 27 sera from cows naturally infected both with a positive result in the comparative intradermal tuberculin test and a negative result in the IDEXX ELISA for the detection of Map-specific antibodies.
Tissue samples from positive reference animals were processed as described in (36) and classified according to the type and extension of the histological lesions present in their intestinal tissues and associated lymph nodes into three groups according to González et al. (21) (see Supplementary Figure 1): focal (n = 447, 78.98%), multifocal (n = 59, 10.42%), and diffuse (n = 60, 10.60%). The Map infection status of these 566 positive reference animals was further characterized (Table 1) by serology (IDEXX Map Ab test –IDEXX, Montpellier, France), bacteriological isolation and real-time PCR of tissues and feces (in some cases), following procedures previously described (23, 36). The percentage of positive animals by one or more diagnostic techniques increased in parallel with the severity of the lesions. Thus, the percentage of positive animals for at least one of the techniques in the focal, multifocal and diffuse groups was 31.99%, 88.14%, and 100%, respectively. More specifically, in the group of animals with focal lesions, 3.36 % were positive by serum IDEXX ELISA and 15.51, and 16.85% were positive by fecal culture and real-time PCR, respectively. In the group of animals with multifocal lesions, 30.51 % were positive by serum IDEXX ELISA and 50.85% and 59.32% were positive by fecal culture and real-time PCR, respectively. In the diffuse group, 86.67% of animals were positive by serum ELISA and 85 and 90% were positive by fecal culture and real-time PCR, respectively. In the same way, the percentage of animals positive by all diagnostic techniques increased as we moved from the focal to the diffuse group, being 0.89%, 8.0%, and 13.64% for the focal, multifocal and diffuse group, respectively. The PTB-free status of the negative reference animals from farms with no prior history of clinical disease was verified yearly by repeatedly negative IDEXX serum ELISA results during a period of 3 to 5 years depending on the specific farm (2016–2021). In one of the farms, the PTB-free status (n = 61) was also confirmed by fecal real-time PCR and bacterial isolation.
Table 1. Mycobacterium avium subspecies paratuberculosis (Map)-infectious status of the 566 infected animals according to the reference histopathological classification (21).
The ABCA13 and MMP8 serum concentration was measured using commercially available Enzyme-Linked Immunosorbent Assays (ELISAs) according to the manufacturer's instructions (MyBioSource, San Diego, CA. USA). Competitive Bovine ATP-binding cassette subfamily A member 13 ELISA kit (detection range 1–5,000 pg/mL) and Bovine Matrix Metalloproteinase 8 (detection range 3.12–100 ng/mL) were used for specific detection of ABCA13 and MMP8 bovine biomarkers, respectively. According to the manufacturer, a standard curve was used to determine the concentration of these biomarkers in the serum samples. A 4-parameter MMF equation provided the best fitting between the average Define optical density (OD) of each standard dilution and its concentration. Standards and blanks were tested in duplicate but a single well was used with test samples. The mean value of the blank control was subtracted from the raw OD values before result interpretation. The concentration of ABCA13 and MMP8 in each sample was interpolated from the standard curve.
Data obtained from ELISA quantification was analyzed using the pROC and Optimal Cutpoints packages of R Program Statistical environment version 3.6.0 (http://www.Rproject.org/), with confidence intervals (CI) stated at 95% for the final results. The area under the curve (AUC) and optimal cut-off value for each group was determined individually by Receiver operator characteristic (ROC) curve analysis. The optimal cut-off values for sensitivity and specificity were based on maximum Youden Index (J = Se + Sp-1). The discriminatory power of this ELISA-based ABCA13 to discern between the different histopathological groups and the control group was considered as follows: AUC values ≥0.9 were considered to have excellent discriminatory power; 0.8 ≤ AUC <0.9 good discriminatory power; 0.7 ≤ AUC <0.8 fair discriminatory power; and AUC <0.7 poor discriminatory (78, 82).
The model developed by Juste and Casal (83) was fed with the sensitivity and specificity of the different testing methods used in this study in order to compare their performance for PTB control in defined epidemiological circumstances.
The diagnostic performance of the ABCA13-based ELISAs was investigated by ROC analysis of the biomarker concentrations using a total of 704 sera and plasma samples, including 447 from focal animals, 59 from multifocal, 60 from diffuse, and 138 sera from control animals (PTB-free farms). ROC analysis of the MMP8-based ELISA was performed using 698 samples, 442 were from animals with focal lesions, 58 with multifocal, 60 with diffuse, and 138 from negative controls (6 sera were only analyzed by the ABCA13-based ELISA as we did not have enough volume to perform both ELISAs). The individual concentrations of ABCA13 and MMP8 in the serum of each cow are shown in Figure 1 and the mean values of the ABCA13 and MMP8 biomarkers concentrations for each group are shown in Table 2. The AUCs values, optimal cut-off points, specificities and sensitivities of each ELISA were estimated by ROC analysis for each histopathological form individually as well as for all histopathological forms globally (Table 3). ROC curves corresponding to the ABCA13 and MMP8-based ELISAs for the different histopathological groups compared to the PTB-free control group are shown in Figures 2, 3, respectively.
Figure 1. ABCA13 and MMP8 expression levels in the 704 animals included in the study. (A) ABCA13 expression levels in sera of Holstein Friesian cattle showing different types of histological lesions consistent with paratuberculosis (PTB) in their intestinal tissues [focal (n = 447), multifocal (n = 59), and diffuse (n = 60)] and control animals from PTB-free farms (n = 138). (B) MMP8 expression levels in serum of Holstein Friesian cattle showing different types of histological lesions consistent with PTB in their intestinal tissues [focal (n = 442), multifocal (n = 58), and diffuse (n = 60)] and control animals from PTB-free farms (n = 138). Biomarkers were quantified by specific ELISAs supplied by MyBioSource, San Diego, CA, USA; ABCA13, bovine ATP binding cassette subfamily A member 13; MMP8, bovine matrix metallopeptidase 8. The data are represented as scatter plots with each dot representing a single animal. The mean of each histopathological group is represented by a gross black point and the standard deviation by a vertical line. The asterisks indicate whether differences between each histopathological group and the control are or not significant (***p < 0.001).
Table 3. Diagnostic performance of the ABCA13 and MMP8-based ELISAs for the detection of animals with different types of PTB histological lesions.
Figure 2. Receiver Operator Characteristic Curves (ROC curves) of the ABCA13 biomarker-based ELISA in Holstein Friesian cows with focal (n = 447), multifocal (n = 59), diffuse histological lesions (n = 60) and any type of paratuberculosis (PTB) lesions (n = 566) vs. control animals from PTB-free farms (n = 138); All, includes all animals with focal, multifocal and diffuse lesions; ABCA13, bovine ATP binding cassette subfamily A member 13.
Figure 3. Receiver Operator Characteristic Curves (ROC curves) of the MMP8 biomarker-based ELISA in Holstein Friesian cows with focal (n = 442), multifocal (n = 58), diffuse lesions (n = 60) and any type of lesions (n = 560) vs. control animals from PTB-free farms (n = 138); All, includes all animals with focal, multifocal and diffuse lesions; MMP8, bovine matrix metallopeptidase 8.
The ELISA based on the detection of the ABCA13 biomarker showed a good discriminatory power (0.8 < AUC <0.9) between animals with focal lesions and control animals with an AUC value of 0.869 [(0.836–0.903, 95% CI), P < 0.001], a sensitivity of the 82.99% and a specificity of 80.43%. However, its discriminatory power between animals with multifocal or diffuse lesions and the control animals was poor (AUC <0.7). Nevertheless, when it comes to differentiating between animals with any type of lesion and control animals the ABCA13-based ELISA showed a good discriminatory power with an AUC of 0.827 (0.792–0.862, 95% CI), p < 0.001), a sensitivity of the 77.56% and a specificity of the 80.43%. Global detection improves as a consequence of the good performance of the ELISA for detection of animals with focal lesions, which are more numerous in the analysis of the group including all the lesion types.
The MMP8-based ELISA showed a poor discriminatory power between the different histopathological groups and the control animals. The AUC values were <0.7 in all cases (Table 3) with the sensitivities ranging from 48.64% to 89.66%, the specificities from 26.09% to 57.97%, and the diagnostic values from 0.530 to 0.6.
Given the good discriminatory power of the ABCA13-based ELISA its diagnostic performance was compared with that of other conventional PTB diagnostic methods (IDEXX ELISA for serum detection of Map-specific antibodies, PCR and bacteriological culture of feces and tissues) and the Ziehl-Neelsen staining (see Table 4). The MMP8-based ELISA was not compared with the conventional methods due to its low diagnostic performance. The ABCA13-based ELISA showed a higher diagnostic value (semi-sum of sensitivity and specificity) (0.822) than the IDEXX ELISA (0.517), the fecal bacteriological culture (0.523), and real-time PCR (0.531) for the detection of animals with focal lesions. In the same way, this biomarker-based ELISA had the best diagnostic value for detection of animals with multifocal lesions (0.707) and for the global detection of animals with any type of lesions (0.790) when compared with the above-mentioned techniques. However, when it comes to the detection of animals with diffuse lesions the method that presented the best diagnostic value was the conventional IDEXX ELISA (0.933). The combined use of the ABCA13-based ELISA and the IDEXX ELISA would increase sensitivity from 77.66% to 83.03% as the IDEXX ELISA detected 31 animals (3 focal, 4 multifocal, and 24 diffuse) that were not detected by the ABCA13 ELISA. Regarding specificity, the IDEXX ELISA performance was better. The ABCA13 ELISA detected 27 control animals as positive (80.43% specificity) while the IDEXX ELISA detected the 138 negative control animals as negative (100% specificity).
Table 4. Comparison of the diagnostic performance of the ABCA13-based ELISA with that of other conventional paratuberculosis (PTB) diagnostic methods.
In addition, the performance of each diagnostic test was compared in a 20 year dynamics simulation study of low level PTB clinical incidence on a cattle farm of 200 heads (Table 4) with the Juste & Casal model (83). It can be observed that the use of the optimal cut-off value of the ABCA13 ELISA (3.67 ng/mL) with the higher diagnostic value would allow an advance in infection eradication to 6 years from program start (>70% reduction) with just an 54% decrease in the benefit/cost ratio (0.45 vs. 0.98) compared to the antibody ELISA. Using a more conservative cut-off value of 7 ng/mL (obtained as the mean value of negative control group + 2 standard deviations (84), characterized by a sensitivity of 42.40% and a higher specificity of 97.1%), it would be possible to eradicate the infection in 14 years (>30% reduction) with an 8% lower benefit/cost ratio (0.90).
The cross-reactivity of the ABCA-13-based ELISA was assessed using sera and plasma samples from cows experimentally (n = 30) infected with M. bovis and confirmed natural cases detected in the national bovine TB eradication program (n = 27). No cross-reactivity with sera and plasma samples of experimentally infected animals was detected (30 out of 30 samples were negative), however, the ABCA13-based ELISA showed some degree of crossed-reactivity with sera from natural cases (7 samples out of 27 cross-reacted with ABCA13, 25.92%).
The goals of PTB control programs vary from eradication in areas of low prevalence, to control in areas with high prevalence, and increased surveillance in areas with no prior history of disease (85). Early detection of Map subclinical infections is critical for PTB control; however, current diagnostic tools have low sensitivities for the detection of subclinically infected animals. Host biomarkers may provide improved diagnostic tools for PTB increasing the effectiveness of control programs. In the present study, we performed a robust validation of ELISAs based on the detection of two candidate biomarkers using a large collection of serum samples (n = 704) from positive and negative reference animals. Evaluation of these two biomarkers showed the potential of one of them, the ABCA13, for detection of most histopathological forms of bovine PTB while the other, the MMP8, was ruled out due to its low sensitivity. Animals, studied at the end of their productive life, were initially classified according to the different pathological forms associated with Map infection (uninfected, focal, multifocal and diffuse lesions), and thus avoided the high rate of false negative results yielded by the most used reference immunological and microbiological methods. The unexpected shortcoming of the pathological characterization was the low number of uninfected negative animals found among these animals. This led to a search for a set of reference negative sera characterized by other, more readily implemented, methods. This was done by reviewing records of PTB serological testing from Asturian farms voluntarily engaged in an animal health program. Finally, a reasonable number of farms with between 3 and 5 years of whole herd negative follow-up was gathered as the largest number of negative samples available. These criteria were not as rigorous as histopathological examination, but by way of repeated whole herd negative results, and absence of clinical cases, provided the closest approximation that allows an estimation of specificity in natural conditions.
The MMP8-based ELISA had a poor discriminatory power (AUC values <0.7) between the different histopathological groups and the control animals (AUCs ranging from 0.515 to 0.564) making it a poor candidate to its use as a PTB diagnostic biomarker. The percentage of positivity detected by the MMP8-based ELISA and the serum levels of this protein varied only slightly between the different histopathological groups (Figure 1 and Table 2), and no significant differences in the serum levels were detected between the different histological groups and the control. These results were in disagreement with those found in the RNA-Seq analysis (35) and the pre-validation study (36) underlying the fact that correlations between RNA expression and protein levels are not always strong (86) and the importance of the current study, based on the analysis of a large number of samples, to verify the potential of putative novel biomarkers.
The ABCA13 ELISA had a good discriminatory power (0.8 ≤ AUC <0.9, p < 0.001) between animals with focal lesions, animals with any type of lesion, and the control animals from PTB-free farms. Furthermore, the ABCA13-based ELISA had higher sensitivities and diagnostic values than the IDEXX ELISA and the fecal and tissue bacteriological isolation and real-time PCR for the detection of animals with focal, multifocal lesions and those with any type of lesion. More specifically, the ELISA based on the detection of ABCA13 had the best diagnostic performance for the detection of animals with focal lesions and for global detection of animals with any type of lesions, being capable of detecting 83.99 and 77.59% of these animals, respectively. However, the IDEXX ELISA, fecal PCR and fecal culture only detected 3.36%, 6.15%, and 4.65% animals with focal lesions and 15.02, 31.63, and 9.18% of the animals with any type of lesion, respectively. The ABCA13-based ELISA specificity values ranged between 80.43 and 82.61%, however, the IDEXX ELISA, the fecal PCR and fecal bacteriological culture had better specificity (100%). These relatively low values of specificity as well as the high rate of positives in the natural TB cases indicate that the high sensitivity of this ELISA might be counterbalanced by some degree of unspecific reactivity due to causes other than PTB. However, it cannot be ruled out that this lower specificity performance could be due to the existence of infected animals in the PTB-free farms that have not been recognized due to the low sensitivity of the reference criteria (IDEXX ELISA) (87) used for the determination of the PTB-infectious status of the negative control animals. It should be pointed out that these sensitivity and specificity values correspond to the optimal cut-off value estimated by ROC analysis. However, the quantitative nature of the protocol reported here becomes a strength since it could be adapted to each specific epidemiological and economic circumstance. For instance, simply by raising the cut-off to 7.0 ng/mL (the upper 99.5% ABCA13 concentration of the negative control group), specificity can become 97.10% still keeping a 42.40% sensitivity that is more than twice that of the antibody IDEXX ELISA (Table 4). The simulation study showed that the ABCA13 ELISA increase in sensitivity could greatly improve the chances of reaching PTB eradication in a reasonable period of time. Even though the benefit/cost would still be negative and therefore, less attractive than vaccination (83), this method would provide a new alternative to farmers or animal health managers to quickly bring under control any outbreak of PTB.
The study of the cross-reactivity of the ABCA13-based ELISA with sera from experimentally and naturally infected M. bovis cows showed that young experimentally infected animals with a negative background do not cross-react. However, 25.92% of the naturally infected animals did. This cross-reactivity migh be due to the uncontrolled history of these animals where exposure to other mycobacteria cannot be ruled out. Moreover, it is also possible that the animals included in this group, which are negative by IDEXX ELISA, are not detected as positive for PTB because of the low sensitivity of the IDEXX ELISA to detect animals with focal lesions. Further studies will be performed to asses potential cross-reactions with other micobacteria or pathogens.
The percentage of positive animals in the ABCA13-based ELISA varied between the different pathological forms. The percentage of animals with a positive ABCA13 result was higher in animals with focal lesions, followed by animals with multifocal and diffuse lesions (83.99%, 61.02% and 53.33%, respectively). Likewise, the individual mean expression levels of ABCA13 in the focal, multifocal and diffuse groups was significantly higher than in the control group (see Figure 1, Table 2). This expression pattern across pathological forms was similar to that observed for intelectin 2 (ITLN2) in the ileocecal valve (ICV) in a recently published study (81). These findings validated our initial study where RNA-seq results showed that the ABCA13 and ITLN2 genes were overexpressed in peripheral blood (log2 fold change = 3.7) and ICV (log2 fold change 10.6) of animals with focal lesions compared to control animals without lesions (35). In fact, the precursor genes of ABCA13 and ITLN2, were the most overexpressed genes in peripheral blood and ICV of animals with focal lesions, respectively.
As we have previously mentioned, the aim of this study was to carry out a broad-scale validation of the ABCA13-based ELISA (n = 704) to confirm the potential of ABCA13 as a diagnostic biomarker. Animals with focal lesions very often go unnoticed in infected farms due to the low sensitivity of conventional methods to detect this group of animals (Table 4). To this goal, ABCA13-based ELISA could be a useful diagnostic tool for the detection of animals with focal lesions, thus complementing conventional methods that have a better diagnostic performance on the diffuse lesions group (Table 4).
The ELISA based on the ABCA13 biomarker has proven to be useful for global diagnosis of animals with lesions associated with PTB, although its diagnostic performance is not good in the case of animals with diffuse lesions. Thus, it could be considered a new tool in the diagnosis of Map infections, either individually, or in combination with other conventional diagnostic methods that detect animals with diffuse lesions accurately as is the case of the IDEXX ELISA. With respect to this, the IDEXX ELISA is very sensitive to detect animals with diffuse lesions, further supporting the idea of using the ABCA13-based ELISA and the IDEXX ELISA in combination, which would increase sensitivity from 77.66% to 83.03% as the IDEXX ELISA detected 31 animals (3 focal, 4 multifocal, and 24 diffuse) that were not detected by the ABCA13 ELISA. Future studies will be focus on validating the assay in younger cohorts as detection of positive animals or selection of negative ones will be more useful at early stages in their life.
Similar studies using biomarkers, but evaluated in a smaller number of animals, have been described by other authors. Espinosa et al. (88) assessed the serum levels of haptoglobin and serum amyloid A, two inflammatory acute-phase proteins detected during the course of PTB infection, in 190 PTB naturally infected animals classified according to the different pathological forms associated with infection (59 uninfected animals without lesions, 73 with focal lesions, 19 with multifocal, 11 with diffuse paucibacillary, and 28 with diffuse multibacillary lesions). Their results reflected a significant increase in the levels of these proteins in the infected animals compared to the control group, more specifically, in those with types of lesions characterized by a low bacterial load and with predominance of a cell-mediated immune response. The authors concluded that these molecules could show potential as putative biomarkers of PTB infection, especially for identification of subclinical animals showing pathological forms related to latency or resistance to the development of advance lesions. Park et al. (89) identified alpha-2-macroglobulin (A2M) as a new promising biomarker improving the diagnostic sensitivity of bovine PTB. They showed that serum A2M levels were significantly higher in Map-exposed (n = 20), subclinical shedder (n = 27), subclinical non-shedder (n = 50) and clinical shedder (n = 18) groups that in the healthy control group (n = 11, from a PTB free farm, negative by fecal PCR and IDEXX and ID Vet ELISA). A2M ELISA showed superior diagnostic performance (90.4 sensitivity and 100% specificity) than other biomarkers and two commercial ELISAs for detection of anti-Map antibodies although the number of healthy control animals included in the study to estimate the specificity is quite small due to difficulty finding a PTB-free farm.
In summary, we could conclude that the ELISA based on the detection of the ABCA13 biomarker has potential for use as a new diagnostic tool for the detection of subclinical animals, which would allow the establishment of an adequate management protocol on farms that, together with hygienic-sanitary measures, could help to reduce the incidence of PTB and, ultimately, reduce the prevalence of this costly and widespread disease. As PTB has a long incubation period before disease becomes evident, early diagnosis of subclinical animals is important to control the spread of the disease.Conversely, we could rule out the use of the ELISA, based on the detection of the MMP8 protein as a diagnostic tool for the detection of Map infection.
The datasets presented in this study can be found in online repositories. The names of the repository/repositories and accession number(s) can be found at: NCBI Gene Expression Omnibus (GEO) database under the accession number GSE137395.
The animal study was reviewed and approved the SERIDA Animal Ethics Committee and authorized by the Regional Consejería de Agroganadería y Recursos Autóctonos del Principado de Asturias –Spain- (authorization codes PROAE 29/2015 and PROAE 66/2019). All the procedures were carried out in accordance with Directive 2012/63/EU of the European Parliament and the Spanish RD53/2013. Peripheral blood and fecal samples were collected by trained personnel and in accordance with good veterinary practice. Written informed consent was obtained from the owners for the participation of their animals in this study.
RC and MA-H: conceptualization and funding acquisition. CB-V and NI: data curation. RAJ, TI, and RC: formal analysis. CB-V, RAJ, JMG, AB, MC, and RC: investigation. CB-V, NI, JA, PV, MC, and TI: methodology. PV, AB, and MQ: resources. RAJ and TI: validation. CB-V and RC: writing—original draft. CB-V, MA-H, NI, PV, RAJ, JMG, AB, MC, JA, MAQ, TI, and RC: writing—review and editing. All authors contributed to the article and approved the submitted version.
This study was part of the I+D+i project (RTI2018-094192-R-C22) and was funded by the Spanish MCIN/AEI/10.13039/501100011033/ Ministry of Science, Innovation and the European Regional Development Funds (FEDER Una manera de hacer Europa) and by the Gobierno del Principado de Asturias, Regional funds PCTI 2021–2023 (GRUPIN: IDI2021-000102) co-funded by FEDER. We acknowledge the National Institute for Agricultural Research (INIA) for the scholarships of CB-V (Ayuda CPD2016-0142 financiada por MCIN/AEI/ 10.13039/501100011033 y FSE El FSE invierte en tu future) and MC.
The authors declare that the research was conducted in the absence of any commercial or financial relationships that could be construed as a potential conflict of interest.
All claims expressed in this article are solely those of the authors and do not necessarily represent those of their affiliated organizations, or those of the publisher, the editors and the reviewers. Any product that may be evaluated in this article, or claim that may be made by its manufacturer, is not guaranteed or endorsed by the publisher.
We would like to acknowledge ASTEGA Veterinary Services for their collaboration in the sampling work and the daily work of SERIDA's farm operators in the care and maintenance of animals. We gratefully acknowledge Kevin P. Dalton for proofreading the manuscript.
The Supplementary Material for this article can be found online at: https://www.frontiersin.org/articles/10.3389/fvets.2022.816135/full#supplementary-material
Supplementary Figure 1. Histological characterization of bovine tissue samples. Animals were classified according to the type of PTB- associated histological lesions present in their gut tissues and associated lymph nodes after staining with hematoxylin-eosin (A–F) and Ziehl-Neelsen (G). (A) Animal with no lesions; (B) Focal lesion in ileocecal lymph node. Small and focal granulomas (arrows) consisting of macrophages and lymphocytes are observed in the Peyer's patch; (C) Multifocal lesion in the ileocecal valve. Small granulomas (arrows) consisting of macrophages and lymphocytes are present in the intestinal mucosa as well as Langhans multinucleated giant cells (star) isolated or associated with these granulomas; (D) Paucibacillary diffuse lesion in distal jejunum. Diffuse granulomatous enteritis with lymphocyte-predominant inflammatory infiltrate. A granuloma in the intestinal mucosa (arrow) consisting of a small number of macrophages and multinucleated giant cells can be observed. An isolated giant cell is also observed (star); (E) Intermediated diffuse lesion in distal jejunum. The infiltrate consists of lymphocytes, plasma cells and a moderate number of macrophages. Granulomas are observed (arrows); (F) Multibacillary diffuse lesion in ileocecal valve. The infiltrated consists of macrophages within a large number of acid-alcohol resistant bacilli to Ziehl-Neelsen staining (G). (A–F) Bar = 100 microns and (G) Bar = 50 microns.
1. McGregor H, Abbott KA, Whittington RJ. Efects of Mycobacterium avium subsp. paratuberculosis infection on serum biochemistry, body weight and wool growth in Merino sheep: a longitudinal study. Small Rumin Res. (2015) 125:146–53. doi: 10.1016/j.smallrumres.2015.02.004
2. Bush RD, Windsor PA, Toribio JALML. Losses of adult sheep due to ovine Johne's disease in 12 infected flocks over a 3-year period. Aust Vet J. (2006) 84:246–53. doi: 10.1111/j.1751-0813.2006.00001.x
3. Benedictus G, Dijkhuizen AA, Stelwagen J. Economic losses due to paratuberculosis in dairy cattle. Vet Rec. (1987) 121:142–6. doi: 10.1136/vr.121.7.142
4. Kudahl AB, Nielsen SS, Sørensen JT. Relationship between antibodies against Mycobacterium avium subsp. paratuberculosis in milk and shape of lactation curves. Pre Vet Med. (2004) 62:119–34. doi: 10.1016/j.prevetmed.2003.11.008
5. Ozsvari L, Harnos A, Lang Z, Monostori A, Strain S, Fodor I. The impact of paratuberculosis on milk production, fertility, and culling in large commercial hungarian dairy herds. Front Vet Sci. (2020) 7:565324. doi: 10.3389/fvets.2020.565324
6. Smith RL, Strawderman RL, Schukken YH, Wells SJ, Pradhan AK, Espejo LA, et al. Effect of Johne's disease status on reproduction and culling in dairy cattle. J Dairy Sci. (2010) 93:3513–24. doi: 10.3168/jds.2009-2742
7. Tiwari A, VanLeeuwen JA, Dohoo IR, Stryhn H, Keefe GP, Haddad JP. Effects of seropositivity for bovine leukemia virus, bovine viral diarrhoea virus, against Mycobacterium avium subspecies paratuberculosis, and Neospora caninum on culling in dairy cattle in four Canadian provinces. Vet Microbiol. (2005) 109:147–58. doi: 10.1016/j.vetmic.2005.05.011
8. Millar D, Ford J, Sanderson J, Withey S, Tizard M, Doran T, et al. IS900 PCR to detect Mycobacterium paratuberculosis in retail supplies of whole pasteurized cows' milk in England and Wales. Appl Environ Microbiol. (1996) 62:3446–52. doi: 10.1128/AEM.62.9.3446-3452
9. Grant IR, Ball HJ, Neill SD, Rowe MT. Inactivation of Mycobacterium paratuberculosis in cows' milk at pasteurization temperatures. Appl Environ Microbiol. (1996) 62:631–6. doi: 10.1128/AEM.62.2.631-636
10. Monif GRG. Translation of hypothesis to therapy in Crohn's disease. Inflamm Bowel Dis. (2016) 22:E8–9. doi: 10.1097/MIB.0000000000000692
11. Feller M, Huwiler K, Stephan R, Altpeter E, Shang A, Furrer H, et al. against Mycobacterium avium subspecies paratuberculosis and Crohn's disease: a systematic review and meta-analysis. Lancet Infect Dis. (2007) 7:607–13. doi: 10.1016/S1473-3099(07)70211-6
12. Kuenstner JT, Naser S, Chamberlin W, Borody T, Graham DY, McNees A, et al. The consensus from the against Mycobacterium avium ssp. paratuberculosis (MAP) Conference 2017. Front Public Health. (2017) 5:208. doi: 10.3389/fpubh.2017.00208
13. Niegowska M, Rapini N, Piccini S, Mameli G, Caggiu E, Manca Bitti ML, et al. Type 1 diabetes at-risk children highly recognize against Mycobacterium avium subspecies paratuberculosis epitopes homologous to human Znt8 and proinsulin. Sci Rep. (2016) 6:22266. doi: 10.1038/srep22266
14. Yokoyama K, Cossu D, Hoshino Y, Tomizawa Y, Momotani E, Hattori N. Anti-mycobacterial antibodies in paired cerebrospinal fluid and serum samples from Japanese patients with multiple sclerosis or neuromyelitis optica spectrum disorder. J Clin Med. (2018) 7:522. doi: 10.3390/jcm7120522
15. Mameli G, Cocco E, Frau J, Marrosu MG, Sechi LA. Epstein-Barr virus and against Mycobacterium avium subsp. paratuberculosis peptides are recognized in sera and cerebrospinal fluid of MS patients. Sci Rep. (2016) 6:22401. doi: 10.1038/srep22401
16. Bo M, Erre GL, Niegowska M, Piras M, Taras L, Longu MG, et al. Interferon regulatory factor 5 is a potential target of autoimmune response triggered by Epstein-Barr virus and against Mycobacterium avium subsp. paratuberculosis in rheumatoid arthritis: investigating a mechanism of molecular mimicry. Clin Exp Rheumatol. (2018) 36:376–81.
17. Sweeney RW. Pathogenesis of paratuberculosis. Vet Clin North Am Food Anim Pract. (2011) 27:537–46. doi: 10.1016/j.cvfa.2011.07.001
18. McAloon CG, Doherty ML, Whyte P, More SJ, O'Grady L, Citer L, et al. Relative importance of herd-level risk factors for probability of infection with paratuberculosis in Irish dairy herds. J Dairy Sci. (2017) 100:9245–57. doi: 10.3168/jds.2017-12985
19. Puerto-Parada M, Arango-Sabogal JC, Paré J, Doré E, Côté G, Wellemans V, et al. Risk factors associated with against Mycobacterium avium subsp. paratuberculosis herd status in Québec dairy herds. Prev Vet Med. (2018) 152:74–80. doi: 10.1016/j.prevetmed.2018.02.010
20. Correa-Valencia NM, Ramírez NF, Arango-Sabogal JC, Fecteau G, Fernández-Silva JA. Prevalence of against Mycobacterium avium subsp. paratuberculosis infection in dairy herds in Northern Antioquia (Colombia) and associated risk factors using environmental sampling. Prev Vet Med. (2019) 170:104739. doi: 10.1016/j.prevetmed.2019.104739
21. González J, Geijo MV, García-Pariente C, Verna A, Corpa JM, Reyes LE, et al. Histopathological classification of lesions associated with natural paratuberculosis infection in cattle. J Comp Pathol. (2005) 133:184–96. doi: 10.1016/j.jcpa.2005.04.007
22. Balseiro A, Pérez V, Juste RA. Chronic regional intestinal inflammatory disease: a trans-species slow infection? Comp Immunol Microbiol Infect Dis. (2019) 62:88–100. doi: 10.1016/j.cimid.2018.12.001
23. Vázquez P, Ruiz-Larrañaga O, Garrido JM, Iriondo M, Manzano C, Agirre M, et al. Genetic association analysis of paratuberculosis forms in Holstein-friesian cattle. Vet Med Int. (2014) 2014:321327. doi: 10.1155/2014/321327
24. Bastida F, Juste RA. Paratuberculosis control: a review with a focus on vaccination. J Immune Based Ther Vaccines. (2011) 9:8. doi: 10.1186/1476-8518-9-8
25. Cho J, Tauer LW, Schukken YH, Gomez MI, Smith RL, Lu Z, et al. Economic analysis of Mycobacterium avium subspecies paratuberculosis vaccines in dairy herds. J Dairy Sci. (2012) 95:1855–72. doi: 10.3168/jds.2011-4787
26. Juste RA, Garrido JM, Elguezabal N, Sevilla IA. Paratuberculosis vaccines and vaccination. In: Behr MA, Stevenson K, Kapur V, editors. Paratuberculosis Organism, Disease, Control. 2nd ed. Wallingford: CABI International (2020). p. 365–79.
27. Kudahl AB, Nielsen SS, Ostergaard S. Strategies for time of culling in control of paratuberculosis in dairy herds. J Dairy Sci. (2011) 94:3824–34. doi: 10.3168/jds.2010-3933
28. Britton LE, Cassidy JP. O'Donovan J, Gordon SV, Markey B. Potential application of emerging diagnostic techniques to the diagnosis of bovine Johne's disease (paratuberculosis). Vet J. (2015) 209:32–9. doi: 10.1016/j.tvjl.2015.10.033
29. Seth M, Lamont EA, Janagama HK, Widdel A, Vulchanova L, Stabel JR, et al. Biomarker discovery in subclinical mycobacterial infections of cattle. PLoS ONE. (2009) 4:e5478. doi: 10.1371/journal.pone.0005478
30. You Q, Verschoor CP, Pant SD, Macri J, Kirby GM, Karrow NA. Proteomic analysis of plasma from Holstein cows testing positive for against Mycobacterium avium subsp. paratuberculosis (MAP). Vet Immunol Immunopathol. (2012) 148:243–51. doi: 10.1016/j.vetimm.2012.05.002
31. Cha SB, Yoo A, Park HT, Sung KY, Shin MK, Yoo HS. Analysis of transcriptional profiles to discover biomarker candidates in against Mycobacterium avium subsp. paratuberculosis -infected macrophages, RAW 2647. J Microbiol Biotechnol. (2013) 23:1167–75. doi: 10.4014/jmb.1302.02021
32. Malvisi M, Palazzo F, Morandi N, Lazzari B, Williams JL, Pagnacco G, et al. Responses of bovine innate immunity to against Mycobacterium avium subsp. paratuberculosis infection revealed by changes in gene expression and levels of microRNA. PLoS ONE. (2016) 11:e0164461. doi: 10.1371/journal.pone.0164461
33. Palacios A, Sampedro L, Sevilla IA, Molina E, Gil D, Azkargorta M, et al. Mycobacterium tuberculosis extracelular vesicle-associated lipoprotein LpqH as a potential biomarker to distinguish paratuberculosis infection or vaccination from tuberculosis infection. BMC Vet Res. (2019) 15:188. doi: 10.1186/s12917-019-1941-6
34. Alonso-Hearn M, Salgado M, de Silva K. Editorial: advances in the diagnosis and control of Johne's disease. Front Vet Sci. (2021) 8:771891. doi: 10.3389/fvets.2021.771891
35. Alonso-Hearn M, Canive M, Blanco-Vázquez C, Torremocha R, Balseiro A, Amado J, et al. RNA-Seq analysis of ileocecal valve and peripheral blood from Holstein cattle infected with Mycobacterium avium subsp, paratuberculosis revealed dysregulation of the CXCL8/IL8 signaling pathway. Sci Rep. (2019) 9:14845. doi: 10.1038/s41598-019-51328-0
36. Blanco Vázquez C, Alonso-Hearn M, Juste RA, Canive C, Iglesias T, Iglesias N, et al. Detection of latent forms of against Mycobacterium avium subsp. paratuberculosis infection using host Biomarker-based ELISA greatly improves paratuberculosis diagnostic sensitivity PLoS ONE. (2020) 15:e0236336. doi: 10.1371/journal.pone.0236336
37. Ueda K, ABC. proteins protect the human body and maintain optimal health. Biosci Biotechnol Biochem. (2011) 75:401–9. doi: 10.1271/bbb.100816
38. Schneider E, Hunke S. ATP-binding-cassette (ABC) transport systems: functional and structural aspects of the ATP-hydrolyzing subunits/domains. FEMS Microbiol Rev. (1998) 22:1–20. doi: 10.1111/j.1574-6976.1998.tb00358.x
39. Barbet R, Peiffer I, Hutchins JRA, Hatzfeld A, Garrido E, Hatzfeld JA. Expression of the 49 human ATP binding cassette (ABC) genes in pluripotent embryonic stem cells and in early and late-stage multipotent mesenchymal stem cells: possible role of ABC plasma membrane transporters in maintaining human stem cell pluripotency. Cell Cycle. (2012) 11:1611–20. doi: 10.4161/cc.20023
40. Davidson AL, Dassa E, Orelle C, Chen J. Structure, function, and evolution of bacterial ATP-binding cassette systems. Microbiol Mol Biol Rev. (2008) 72:317–64. doi: 10.1128/MMBR.00031-07
41. Higgins CF, ABC. transporters: from microorganisms to man. Annu Rev Cell Biol. (1992) 8:67–113. doi: 10.1146/annurev.cb.08.110192.000435
42. Albrecht C, Viturro E. The ABCA subfamily–gene and protein structures, functions and associated hereditary diseases. Pflugers Arch. (2007) 453:581–9. doi: 10.1007/s00424-006-0047-8
43. Chai AB, Ammit AJ, Gelissen IC. Examining the role of ABC lipid transporters in pulmonary lipid homeostasis and inflammation. Respir Res. (2017) 18:41. doi: 10.1186/s12931-017-0526-9
44. Hlaváč V, Václavíková R, Brynychová V, KoŽevnikovová R, Kopečková K, Vrána D, et al. Role of genetic variation in ABC transporters in breast cancer prognosis and therapy response. Int J Mol Sci. (2020) 21:9556. doi: 10.3390/ijms21249556
45. Dongsheng H, Zhuo Z, Jiamin L, Hailan M, Lijuan H, Fan C, et al. Proteomic analysis of the peri-infarct area after human umbilical cord mesenchymal stem cell transplantation in experimental stroke. Aging Dis. (2016) 7:623–34. doi: 10.14336/AD.2016.0121
46. Chen J, Waheed Khan RA, Wang M, He K, Wang Q, Li Z, et al. Association between the variability of the ABCA13 gene and the risk of major depressive disorder and schizophrenia in the Han Chinese population. World J Biol Psychiatry. (2017) 18:550–6. doi: 10.1080/15622975.2016.1245442
47. Rajkumar AP, Bidkhori G, Shoaie S, Clarke E, Morrin H, Hye A, et al. Postmortem cortical transcriptomics of Lewy body dementia reveal mitochondrial dysfunction and lack of neuroinfammation. Am J Geriatr Psychiatry. (2020) 28:75–86. doi: 10.1016/j.jagp.2019.06.007
48. Knight HM, Pickard BS, Maclean A, Malloy MP, Soares DC, McRae AF, et al. A cytogenetic abnormality and rare coding variants identify ABCA13 as a candidate gene in schizophrenia, bipolar disorder, and depression. Am J Hum Genet. (2009) 85:833–46. doi: 10.1016/j.ajhg.2009.11.003
49. Iritani S, Torii Y, Habuchi C, Sekiguchi H, Fujishiro H, Yoshida M, et al. The neuropathological investigation of the brain in a monkey model of autism spectrum disorder with ABCA13 deletion. Int J Dev Neurosci. (2018) 71:130–9. doi: 10.1016/j.ijdevneu.2018.09.002
50. Yoshida K, Go Y, Kushima I, Toyoda A, Fujiyama A, Imai H, et al. Single-neuron and genetic correlates of autistic behavior in macaque. Sci Adv. (2016) 2:e1600558. doi: 10.1126/sciadv.1600558
51. Frydrych LM, Ulintzf P, Bankhead A, Sifuentes C, Greenson J, Maguire L, et al. Rectal cancer sub-clones respond differentially to neoadjuvant therapy. Neoplasia. (2019) 21:1051–62. doi: 10.1016/j.neo.2019.08.004
52. Nymoen DA, Holth A, Hetland Falkenthal TE, Tropé CG, Davidson B. CIAPIN1 and ABCA13 are markers of poor survival in metastatic ovarian serous carcinoma. Mol Cancer. (2015) 14:44. doi: 10.1186/s12943-015-0317-1
53. Hlaváč V, Brynychová V, Václavíková R, Ehrlichová M, Vrána D, Pecha V, et al. The expression profile of ATP-binding cassette transporter genes in breast carcinoma. Pharmacogenomics. (2013) 14:515–29. doi: 10.2217/pgs.13.26
54. Araújo TM, Seabra AD, Lima EM, Assumpção PP, Montenegro RC, Demachki S, et al. Recurrent amplification of RTEL1 and ABCA13 and its synergistic effect associated with clinicopathological data of gastric adenocarcinoma. Mol Cytogenet. (2016) J9:52. doi: 10.1186/s13039-016-0260-x
55. Han J, Han N, Xu Z, Zhang C, Liu J, Ruan M. Expression profle of circular RNA and construction of circular RNA-Micro RNA network in salivary adenoid cystic carcinoma. Cancer Cell Int. (2021) 21:28. doi: 10.1186/s12935-020-01681-2
56. Dréan A, Rosenberg S, Lejeune FX, Goli L, Nadaradjane AA, Guehennec J, et al. ATP binding cassette (ABC) transporters: expression and clinical value in glioblastoma. J Neuro-Oncol. (2018) 138:479–86. doi: 10.1007/s11060-018-2819-3
57. Hlavata I, Mohelnikova-Duchonova B, Vaclavikova R, Liska V, Pitule P, Novak P, et al. The role of ABC transporters in progression and clinical outcome of colorectal cancer. Mutagenesis. (2012) 27:187–96. doi: 10.1093/mutage/ger075
58. Zhang X, Hirschfeld M, Beck J, Kupke A, Köhler K, Schütz E, et al. Osteogenesis imperfecta in a male holstein calf associated with a possible oligogenic origin. Vet Q. (2020) 40:58–67. doi: 10.1080/01652176.2020.1721611
59. Lauhio A, Färkkilä E, Pietiläinen KH, Åström P, Winkelmann A, Tervahartiala T, et al. Association of MMP-8 with obesity, smoking and insulin resistance. Eur J Clin Invest. (2016) 46:757–65. doi: 10.1111/eci.12649
60. Kunt AT, Isbir S, Görmus U. Polymorphisms of MMP9 and TIMP2 in patients with varicose veins. In Vivo. (2015) 29:461–5.
61. Shiomi T, Lemaître V, D'Armiento J, Okada Y. Matrix metalloproteinases, a disintegrin and metalloproteinases, and a disintegrin and metalloproteinases with thrombospondin motifs in non-neoplastic diseases. Pathol Int. (2010) 60:477–96. doi: 10.1111/j.1440-1827.2010.02547.x
62. Löffek S, Schilling O, Franzke C. Biological role of matrix metalloproteinases: a critical balance. Eur Respir J. (2011) 38:191–208. doi: 10.1183/09031936.00146510
63. Long Z. Computational analysis of the metal selectivity of matrix metalloproteinase 8. PLoS One. (2020) 15:e0243321. doi: 10.1371/journal.pone.0243321
64. Chambers AF, Matrisian LM. Changing views of the role of matrix metalloproteinases in metastasis. J Natl Cancer Inst. (1997) 89:1260–70. doi: 10.1093/jnci/89.17.1260
65. Sternlicht MD, Werb Z. How matrix metalloproteinases regulate cell behavior. Annu Rev Cell Dev Biol. (2001):463–516. doi: 10.1146/annurev.cellbio.17.1.463
66. Smith BN, Bhowmick NA. Role of EMT in metastasis and therapy resistance. J Clin Med. (2016) 5:17. doi: 10.3390/jcm5020017
67. Bajbouj K, Ramakrishnan RK, Hamid Q. Role of Matrix Metalloproteinases in Angiogenesis and Its Implications in Asthma. J Immunol Res. (2021) 2021:6645072. doi: 10.1155/2021/6645072
68. Johnson JL. Metalloproteinases in aterosclerosis. Eur J Pharmacol. (2017) 816:9 −106. doi: 10.1016/j.ejphar.2017.09.007
69. Kathamuthu GR, Kumar NP, Moideen K, Nair D, Banurekha VV, Sridhar R, et al. Matrix metalloproteinases and tissue inhibitors of metalloproteinases are potential biomarkers of pulmonary and extra-pulmonary tuberculosis. Front Immunol. (2020) 11:419. doi: 10.3389/fimmu.2020.00419
70. Hanemaaijer R, Sorsa T, Konttinen YT, Ding Y, Sutinen M, Visser H, et al. Matrix metalloproteinase-8 Is expressed in rheumatoid synovial fibroblasts and endothelial cells. regulation by tumor necrosis factor-alpha and doxycycline. J Biol Chem. (1997) 272:31504–9. doi: 10.1074/jbc.272.50.31504
71. Maccoll E, Khalil RA. Matrix metalloproteinases as regulators of vein structure and function: implications in chronic venous disease. J Pharmacol Exp Ther. (2015) 355:410–28. doi: 10.1124/jpet.115.227330
72. Qin G, Luo M, Chen J, Dang Y, Chen G, Li L, et al. Reciprocal activation between MMP-8 and TGF-β1 stimulates EMT and malignant progression of hepatocellular carcinoma. Cancer Lett. (2016):85–95. doi: 10.1016/j.canlet.2016.02.001
73. Juurikka K, Butler GS, Salo T, Nyberg P, Astrom P. The role of MMP8 in cancer: a systematic review. Int J Mol Sci. (2019) 2018:4506. doi: 10.3390/ijms20184506
74. Sirniö P, Tuomisto A, Tervahartiala T, Sorsa T, Klintrup K, Karhu T, et al. High-serum MMP-8 levels are associated with decreased survival and systemic inflammation in colorectal cancer. Br J Cancer. (2018):213–19. doi: 10.1038/s41416-018-0136-4
75. Kushlinskii NE, Gershtein ES, Alferov AA, Bezhanova SD, Mushtenko VV, Pushkar DY, et al. Prognostic role of matrix metalloproteinases 2, 7, 8, 9, and their type 1 tissue inhibitor in blood serum of patients with kidney cancer. Bull Exp Med. (2020) 168:673–6. doi: 10.1007/s10517-020-04778-w
76. Kelppe J, Thorén H, Haglund C, Sorsa T, Hagström J. MMP-7,−8,−9, E-cadherin, and beta-catenin expression in 34 amelobastoma cases. Clin Exp Dent Res. (2021) 7:63–9. doi: 10.1002/cre2.331
77. Korpi JT, Kervinen V, Mäklin H, Väänänen A, Lahtinen M, Läärä E, et al. Collagenase-2 (matrix metalloproteinase-8) plays a protective role in tongue cancer. Br J Cancer. (2008) 98:766–75. doi: 10.1038/sj.bjc.6604239
78. Muller MP, Tomlinson G, Marrie TJ, Tang P, McGeer A, Low DE, et al. Can routine laboratory tests discriminate between severe acute respiratory syndrome and other causes of community-acquired pneumonia?. Clin Infect Dis. (2005) 40:1079–10. doi: 10.1086/428577
79. DeLong ER, DeLong DM, Clarke-Pearson DL. Comparing the areas under two or more correlated receiver operating characteristic curves: a non-parametric approach. Biometrics. (1988) 44:837–45.
80. Garry FB. Control of paratuberculosis in dairy herds. Vet Clin North Am Food Anim Pract. (2011) 27:599–607. doi: 10.1016/j.cvfa.2011.07.006
81. Blanco Vázquez C, Balseiro A, Alonso-Hearn M, Juste RA, Iglesias N, Canive M, et al. Bovine intelectin 2 expression as a biomarker of paratuberculosis disease progression. Animals. (2021) 11:1370. doi: 10.3390/ani11051370
82. Park HE, Park HT, Jung YH, Yoo HS. Establishment a real-time reverse transcription PCR based on host biomarkers for the detection of the subclinical cases of against Mycobacterium avium subsp. Paratuberculosis PLoS ONE. (2017) 12:e0178336. doi: 10.1371/journal.pone.0178336
83. Juste RA, Casal J. An economic and epidemiologic simulation of different control strategies for ovine paratuberculosis. Prev Vet Med. (1993) 15:101–15. doi: 10.1016/0167-5877(93)90106-4
84. Li L, Wagner B, Freer H, Schilling M, Bannantine JP, Campo JJ, et al. Early detection of against Mycobacterium avium subsp. paratuberculosis infection in cattle with multiplex-bead based immunoassays. PLoS ONE. (2017) 12:e0189783. doi: 10.1371/journal.pone.0189783
85. Barkema HW, Orsel K, Nielsen SS, Koets AP, Rutten VPMG, Bannantine JP, et al. Knowledge gaps that hamper prevention and control of against Mycobacterium avium subspecies paratuberculosis infection. Transbound Emerg Dis. (2018) 1:125–48. doi: 10.1111/tbed.12723
86. Vogel C, Marcotte EM. Insights into the regulation of protein abundance from proteomic and transcriptomic analyses. Nat Rev Genet. (2012) 13:227–32. doi: 10.1038/nrg3185
87. Whitlock RH, Wells SJ, Sweeney RW, Van Tiem J, ELISA. and fecal culture for paratuberculosis (Johne 's disease): sensitivity and specificity of each method. Vet Microbiol. (2000) 77:387–98. doi: 10.1016/s0378-1135(00)00324-2
88. Espinosa J, de la Morena R, Benavides J, García-Pariente C, Fernández M, Tesouro M, et al. Assessment of acute-phase protein response associated with the different pathological forms of bovine paratuberculosis. Animals. (2020) 10:1925. doi: 10.3390/ani10101925
Keywords: paratuberculosis, subclinical infection, focal lesions, diagnosis, biomarkers, ATP binding cassette subfamily A member 13
Citation: Blanco-Vázquez C, Alonso-Hearn M, Iglesias N, Vázquez P, Juste RA, Garrido JM, Balseiro A, Canive M, Amado J, Queipo MA, Iglesias T and Casais R (2022) Use of ATP-Binding Cassette Subfamily A Member 13 (ABCA13) for Sensitive Detection of Focal Pathological Forms of Subclinical Bovine Paratuberculosis. Front. Vet. Sci. 9:816135. doi: 10.3389/fvets.2022.816135
Received: 16 November 2021; Accepted: 24 January 2022;
Published: 10 March 2022.
Edited by:
Michael Kogut, Agricultural Research Service (USDA), United StatesReviewed by:
Douwe Bakker, Independent Researcher, Lelystad, NetherlandsCopyright © 2022 Blanco-Vázquez, Alonso-Hearn, Iglesias, Vázquez, Juste, Garrido, Balseiro, Canive, Amado, Queipo, Iglesias and Casais. This is an open-access article distributed under the terms of the Creative Commons Attribution License (CC BY). The use, distribution or reproduction in other forums is permitted, provided the original author(s) and the copyright owner(s) are credited and that the original publication in this journal is cited, in accordance with accepted academic practice. No use, distribution or reproduction is permitted which does not comply with these terms.
*Correspondence: Rosa Casais, cm9zYWNnQHNlcmlkYS5vcmc=
Disclaimer: All claims expressed in this article are solely those of the authors and do not necessarily represent those of their affiliated organizations, or those of the publisher, the editors and the reviewers. Any product that may be evaluated in this article or claim that may be made by its manufacturer is not guaranteed or endorsed by the publisher.
Research integrity at Frontiers
Learn more about the work of our research integrity team to safeguard the quality of each article we publish.