- 1Department of Clinical Sciences, Swedish University of Agricultural Sciences, Uppsala, Sweden
- 2Department of Medical Biochemistry and Microbiology, Uppsala University, Uppsala, Sweden
- 3Department of Biosciences, International Livestock Research Institute, Nairobi, Kenya
- 4Department of Disease Control, School of Veterinary Medicine, University of Zambia, Lusaka, Zambia
- 5SACIDS Foundation for One Health, Sokoine University of Agriculture, Morogoro, Tanzania
- 6Department of Veterinary Anatomy and Pathology, Sokoine University of Agriculture, Morogoro, Tanzania
- 7Department of Veterinary Services Ministry of Fisheries and Livestock, Central Veterinary Research Institute, Lusaka, Zambia
- 8Department of Biomedical Science and Veterinary Public Health, Swedish University of Agricultural Sciences, Uppsala, Sweden
- 9Department of Epidemiology and Disease Control, National Veterinary Institute, Uppsala, Sweden
Transboundary pathogens pose a threat to livelihood security in countries such as Zambia and Tanzania. This study aimed to investigate the seroprevalence of peste des petits ruminants virus (PPRV), foot and mouth disease virus (FMDV), sheep and goat pox virus (SGPV), Rift Valley fever virus (RVFV) and Brucella spp. in sheep and goats along the Tanzania-Zambia border. Another aim was to assess the association between certain predictor variables and seroprevalence, focusing on trade and proximity to an international border, to a town and to the Tanzania-Zambia highway. During September-October 2018, 486 serum samples from small ruminants in Zambia and 491 in Tanzania were collected and analyzed using enzyme-linked immunosorbent assays (ELISA). A questionnaire focused on management strategies was administered to each household. The animal-level seroprevalence in Zambia was 0.21% [95% confidence interval (CI) (0.01–1.14) for PPRV, 1.03% (95% CI 0.33–2.39) for FMDV, 0% (95% CI 0–0.76) for SGPV, 2.26% (95% CI 1.14–4.01) for RVFV and 1.65% (95% CI 0.71–3.22) for Brucella spp.]. In Tanzania, animal-level seroprevalence was 2.85% (95% CI 1.57–4.74) for PPRV, 16.9% (95% CI 13.7–20.5) for FMDV, 0.20% (95% CI 0.01–1.13) for SGPV, 3.26% (95% CI 1.87–5.24) for RVFV and 20.0% (95% CI 14.5–26.5) for Brucella spp. For PPRV (OR 6.83, 95% CI 1.37–34.0, p = 0.019) and FMDV (OR 5.68, 95% CI 1.58–20.3, p = 0.008), herds situated more than 30 km from an international border were more likely to be seropositive, while being located 10–30 km (OR 4.43, 95% CI 1.22–16.1 p = 0.024) from a border was identified as a risk factor for Brucella spp. For FMDV (OR 79.2, 95% CI 4.52–1388.9, p = 0.003), being situated within 30 km from a town was associated with seropositivity. Furthermore, contact with wild ruminants (OR 18.2, 95% CI 1.36–244), and the presence of sheep in the household (OR 5.20, 95% CI 1.00–26.9, p = 0.049), was associated with seropositivity for PPRV, and FMDV. No significant associations between trade or distance to the Tan-Zam highway and seroprevalence were found. We recommend that the impact of trade and proximity to borders, towns and roads should be further evaluated in larger studies, ideally incorporating aspects such as temporal trade fluctuations.
Introduction
Small ruminants (sheep and goats) are increasingly being recognized for their role in securing the livelihoods of farmers, traders and other stakeholders, especially in low- and lower-middle-income countries. Sheep and goats are important for income generation and storing wealth, and for food and nutritional security (1). However, these roles are severely constrained by transboundary animal diseases (TADs), which are contagious and capable of rapid cross-regional spread, irrespective of international borders. These diseases can have severe socio-economic consequences, apart from their direct and immediate impacts on trade, food and nutritional security, and animal welfare (2). Tanzania and Zambia are neighboring countries in south-east Africa, and despite their proximity, there are considerable differences in the spectra of transboundary animal pathogens reported in each of the countries. Border porosity, a high level of cross-border small ruminant movements and trade, as well as poor biosecurity pose a substantial risk of pathogen exchange across the border between the two countries (3–5).
There are several TADs that affect small ruminants, such as peste des petits ruminants (PPR), foot and mouth disease (FMD), sheep- and goat pox (SGP), Rift Valley fever (RVF) and brucellosis. Peste des petits ruminants is a highly contagious disease caused by PPR virus (PPRV), a member of the morbillivirus genus. Clinical signs indicative of PPRV include pyrexia, mucopurulent oculonasal discharges, cough, dyspnea, necrotic stomatitis and severe diarrhea (6). Morbidity and case fatality rates can be as high as 100 and 90%, respectively, in naïve populations (7). Due to the severe impacts of PPR, the OIE and FAO joined forces in 2015 with the goal of eradicating PPRV by 2030 (7). While PPRV is endemic in Tanzania (8), antibodies against PPRV in small ruminants without proven presence of the virus or clinical disease have been reported in Zambia (9). However, the risk of PPRV introduction into Zambia from Tanzania is considered high (3, 4). The fact that PPRV has also been detected in the neighboring Democratic Republic of the Congo (DRC) (10–13) and Angola (14), further increases the risk of PPRV introduction into Zambia.
Foot and mouth disease, caused by FMD virus (FMDV), is endemic in both Zambia and Tanzania (15–19). Despite a relatively low mortality rate, FMD has been identified as the principal animal disease of global concern due to its high morbidity and severe negative effects on animal production and trade (2). Since sheep and goats often only develop mild and transient clinical signs that are easily missed, they can act as reservoirs and contribute to the spread of the virus, as was seen in the FMD outbreak in the UK in 2001 (20). The fact that small ruminants are often excluded from vaccination campaigns for FMDV (21) further increases their ability to act as reservoirs. Sheep- and goatpox (here abbreviated as SGP) is another TAD caused by two closely related capripoxviruses, i.e., sheeppox virus (SPPV) and goatpox virus (GTPV), or sheep- and goatpox virus (here called SGPV). Both viruses are generally host-specific, although cross-transmission does occur (22). Sheep- and goatpox virus can cause high morbidity and mortality rates in small ruminants, especially in exotic breeds that are imported into an endemic area. The disease is characterized by pyrexia, generalized skin and internal pox lesions and lymphadenopathy (23). Sheep- and goatpox virus has not been discovered in Zambia, but has been found in both neighboring Tanzania (24) and DRC (11).
There are several TADs of sheep and goats that are zoonotic and capable of causing disease in humans as well as small ruminants. Rift Valley fever, caused by RVF virus (RVFV), is generally manifested by periodic epizootic outbreaks of abortions and neonatal mortalities in sheep, goats and cattle, as well as influenza-like and hemorrhagic disease in humans (25). While the disease is mainly transmitted between animals by mosquito bites, humans are typically infected following contact with blood and offal at slaughter or when consuming undercooked meat (26). Rift Valley fever virus is endemic in both Zambia and Tanzania, although no outbreaks have been reported in Zambia since 1989 (27) or in Tanzania since 2006–07 (28, 29).
Brucellosis is caused by members of the bacterial genus Brucella, and the disease is endemic in both Zambia and Tanzania. Small ruminants are generally infected by Brucella melitensis, B. abortus, B. suis and B. ovis (30), of which all but B. ovis are zoonotic (31). Humans risk infection when they come into contact with aborted fetuses or fetal membranes, e.g., when assisting an animal during parturition, and through consumption of unpasteurized dairy products and undercooked meat, or when slaughtering an animal (31). Clinical signs indicative of infection with Brucella spp. in sheep and goats include abortions, stillbirths and occasionally joint hygromas, orchitis and epididymitis (30), while common symptoms in humans are undulant fever, weakness, general malaise and joint pain (31).
Increased participation in animal trade has the potential to improve the livelihoods of poor smallholder farmers (32, 33), but it can also contribute to the transmission of diseases, both TADs and endemic diseases (34, 35). Livestock trade and animal movement tend to occur at higher frequencies close to international borders, which in turn can contribute to increased exposure to transboundary animal pathogens. Clustering of outbreaks of FMD in cattle in both Zambia and Tanzania have previously been shown close to international borders, including the Tanzania-Zambia border region. Furthermore, clustering has been observed close to the Tanzania-Zambia (or Tan-Zam) highway, a major transportation route connecting the two countries (15–19).
The aim of this study was to assess the seroprevalence of PPRV, FMDV, SGPV, RVFV, and Brucella spp. in sheep and goats in the Tanzania-Zambia border region. The study also aimed to investigate associations between certain predictor variables and seroprevalence, focusing on trade and proximity to the Tanzania-Zambia or Zambia-Malawi borders, to a larger town and to the Tan-Zam highway.
Materials and Methods
Study Area and Study Design
The study was designed to provide cross-sectional data on the seroprevalence of PPRV, FMDV, SGPV, RVFV and Brucella spp., and potential associations between selected predictor variables and seroprevalence, in sheep and goats in the border region between Zambia and Tanzania. Four districts, two in Zambia and two in Tanzania, where purposively selected for the study: Nakonde and Mbala districts in Zambia, and Tunduma and Momba districts in Tanzania. Mbala district was chosen due to its immediate proximity to the border, while Momba, Nakonde and Tunduma districts were chosen due to their border proximity and because the Tan-Zam highway runs through them (Figure 1). In Tanzania, there is a global and regional aligned national control program for PPR, and sero-surveys and vaccination campaigns have previously been conducted in both Tunduma and Momba districts. Unfortunately, these activities had to be scaled down in 2013 due to lack of funding (E. S. Swai, personal communication). Vaccinations for RVF were performed in Tunduma and Momba district until 2008, after which measures aiming to control the mosquito population were deemed more cost-efficient and therefore used instead (D. Mdetele, personal communication). Furthermore, both Zambia and Tanzania have control programs aiming to control the spread of FMD, although these efforts are mainly directed at cattle. There are no control programs for small ruminant diseases in Zambia.
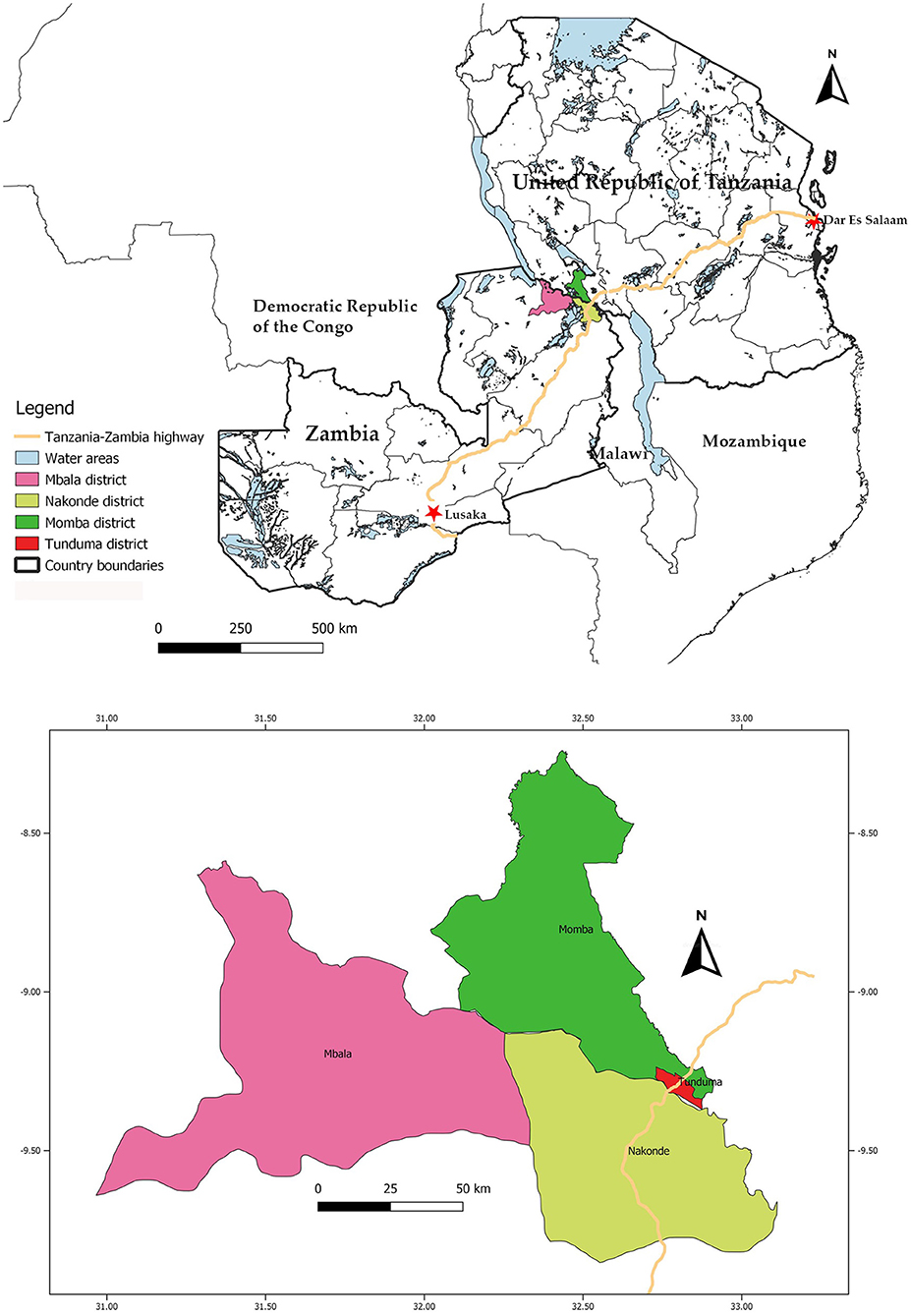
Figure 1. The location of the visited districts in the Tanzania-Zambia border region, and of the Tanzania-Zambia highway. Map created in QGIS version 3.4.4 software (https://qgis.org).
Village lists were obtained from local veterinary personnel, from which 40 villages on each side of the border were randomly selected using the randomize tool in excel. The lists were later edited with the assistance of local veterinary personnel, and villages that were inaccessible or did not contain sheep or goats were replaced with a neighboring village. Due to difficulties in finding sufficient numbers of small ruminants in some villages, 41 villages ended up being included in Tanzania and 42 in Zambia. On the Zambian side, 32 villages in Nakonde district and 10 in Mbala district were visited. More villages were visited in Nakonde district due to the high frequency of cross-border trade and the fact that the Tan-Zam highway transects the district. In Tanzania, 33 villages were randomly selected in Momba district, as Tunduma district only consisted of eight villages. In each village, four households were selected using snowball sampling methodology (36). To diversify which farmers were approached, conditions based on herd size were included in the snowball sampling strategy. For example, the farmers were asked to re-direct us to a small ruminant farmer who fulfilled one of the following criteria: (i) a household that kept fewer than five sheep and/or goats, (ii) a household that kept 5–15 sheep and/or goats, and (iii) a household that kept more than 15 sheep and/or goats. These groups were based on the average herd size of sheep and goats in Zambia (8.4 and 7.4, respectively) and Tanzania (5.2 and 6.8, respectively) (37, 38). The goal was to include at least one household in each group per village. If all three groups were included with the first three households, no conditions were given for the last household. If it was not possible to find representatives of all groups, another household was included irrespective of the group to which it belonged. Samples were obtained from three sheep or goats per household. The selection was non-random as it was generally the farmer who chose the animals to be sampled. Animals younger than 4 months of age were excluded to avoid interference from maternal antibodies. In the cases where it was not possible to obtain samples from three animals in a given household, more samples were collected either from subsequent households or from households in other villages.
The study was conducted in two different strata, specifically the Tanzanian and the Zambian sides of the border. The sample size per stratum was calculated assuming an infinite population, and using a 95% confidence interval (CI), 5% margin of error, 50% assumed true prevalence, and sensitivity and specificity values from the ID Vet ID Screen Rift Valley Fever Competition Multi-species competitive enzyme-linked immunosorbent assay (cELISA), in order to generate the largest required sample size. The sample size calculation was performed according to the instructions in Humphry, Cameron (39), using the “Sample size to estimate a true prevalence with an imperfect test” tool on the https://epitools.ausvet.com.au/ webpage (Ausvet, Australia). The necessary sample size was calculated to be 461, which was subsequently rounded up to 480 sheep or goats to allow for errors in sample procurement and analysis.
Sample and Data Collection
Data were collected between September and October 2018. Blood samples were obtained from the jugular vein using sterile needles and plain vacutainer tubes (BD Vacutainer, Plymouth, UK). The samples were then left standing in a cool box to coagulate and separate. At the end of each day, the serum was transferred to cryotubes and placed in a freezer at ~-20°C. The samples were later transported to an ultralow freezer for long-term storage at −80°C. For each sampled individual, information on age, sex, origin and signs of disease, both on the day of sample collection and within the last 12 months, was recorded. In addition, epidemiological data on herd characteristics and biosecurity, trade routines, management practices and herd disease history were collected in a questionnaire. The questionnaire was constructed using experience from interviews with farmers and veterinary personnel in Nakonde district and veterinary personnel in Tunduma district conducted by the first author in April-May 2018. The questionnaire was written in English and translated by an enumerator into the local languages, namely Swahili in Tanzania and Namwanga or Mambwe in Zambia. The enumerator asked the questions orally, clarified misunderstandings where necessary, and manually recorded the respondent's answers in English on the questionnaire sheet. The questionnaire took ~20–30 min to complete and contained a mix of open and closed questions.
Furthermore, the global positioning system (GPS) coordinates for each of the visited households were recorded. Distances to the Tanzania-Zambia and Zambia-Malawi borders, to the Tan-Zam highway and to a town were measured by entering the coordinates into ArcGIS online (Esri, California, USA), and using the distance measurement tool. Due to difficulties in obtaining data on road infrastructure in the study area, the closest linear distance was measured. When measuring border proximity, the linear distance to the closest border, either the Tanzania-Zambia or Zambia-Malawi border, was recorded. In most instances, the distance to the Tanzania-Zambia border was shorter, however eight households in Nakonde district in Zambia were closer to the Zambia-Malawi border and hence this distance was recorded. When measuring the distance to a town, Mbeya, Tunduma, Vwawa and Sumbawanga towns were included in Tanzania, and Nakonde and Mbala towns in Zambia.
Laboratory Analysis
Laboratory analysis was performed using commercially available enzyme-linked immunosorbent assays (ELISA) to detect antibodies against the selected pathogens. The following kits were used: ID Screen PPR competition ELISA (sensitivity 100%, specificity 100%; ID Vet, Grabels, France), ID Screen FMD NSP competition (sensitivity 100%, specificity 99.5%; ID Vet, Grabels, France), ID Screen Capripox Double Antigen Multi-species which detects antibodies to SPPV, GTPV and lumpy skin disease virus (LSDV) (sensitivity not known, specificity 99.7% in capripox-free regions; ID Vet, Grabels, France) and ID Screen Rift Valley fever Competition Multi-species (sensitivity 100%, specificity 100%; ID Vet, Grabels, France). For Brucella spp., two different cELISA were used: in Zambia Svanovir Brucella-Ab C-ELISA (sensitivity 100%, specificity 100%; Boehringer-Ingelheim Svanova Diagnostics, Uppsala, Sweden), and in Tanzania LTELISA Brucella cELISA kit, (sensitivity 98.9%, specificity 99.9%; LT Biotech Moksklininku 6A, Vilnius, Lithuania). Both ELISAs detect antibodies to B. abortus, B. melitensis and B. suis. The Brucella spp. analysis in Tanzania was conducted more than a year after the other analyses, and at that time a large number of samples could no longer be located. Hence, the number of analyzed samples for Brucella spp. in Tanzania was lower (n = 671) compared with the other pathogens.
Independent evaluations of the sensitivity and specificity have been performed on the PPRV and RVFV ELISAs, and the Brucella spp. ELISA used in Zambia. For the PPRV ELISA, sensitivity and specificity was estimated to be 94.5 and 99.4%, respectively (40). For RVFV, one study estimated sensitivity and specificity to both be 100% (41), and another to be 91–100% and 100%, respectively (42). For the Brucella spp. ELISA that was used in Zambia, sensitivity and specificity values of 99.4 and 98.9%, respectively have been estimated (43). All the kits were utilized, validated and interpreted according to the instructions provided by the manufacturer. The results in the PPR and RVF ELISAs were scored as positive, negative or doubtful. Doubtful samples were considered negative in the statistical analysis.
Statistical Analysis
True prevalence was calculated using the apparent prevalence and the sensitivity and specificity of the statistical test, in accordance with Rogan and Gladen (44) and using the “Estimated true prevalence and predictive values from survey testing” on https://epitools.ausvet.com.au (Ausvet, Australia). Furthermore, statistical analyses were performed using Stata IC 16/1 (StataCorp LLC, USA). Univariable and multivariable analyses were conducted on both animal- and herd-level data for the respective pathogens. A herd was considered seropositive for a pathogen if one or more of the sampled animals in the herd tested positive. As the sampling strategy was conducted in a population where all villages in the end were not eligible for practical reasons, and the number of farming households per village were not known, no corrections for sampling weights were done, and prevalence presented is unadjusted. The potential predictive variables included were age, sex, species, country, treatment frequency with acaricides, time of latest introduction of a new small ruminant to the herd, whether the household has bought sheep and/or goats from traders or at markets, time of latest selling event of a small ruminant, purchase of sheep or goats from other countries or residing in a community where this is done, selling sheep or goats to other countries or residing in a community where this is done, contact frequency with sheep and goats from other herds, contact frequency with cattle from other herds, contact with wild ruminants, herd size, proximity to the Tanzania-Zambia or Zambia-Malawi border, proximity to a town, and proximity to the Tan-Zam highway. Univariable analysis was conducted using the Chi2 test or Fischer's exact test where applicable. Multivariable analyses were performed in STATA using the meqrlogit command, a method which does not provide a pseudo R2 value. In the animal-level analysis, district, village and herd were included as random effects, and in the herd-level analysis, district and village were included as random effects. All predictive variables with a p-value of 0.25 or less in the univariable analysis were included in the multivariable analysis, unless multicollinearity was detected. Multicollinearity was tested in all the models using variance inflation factor (VIF), and a cut-off value of 10 was used. For example, the analysis identified collinearity between country and grazing strategy, therefore grazing strategy was removed from the analysis. The analyses were guided by directed acyclic graphs (Figure 2), where distance to the Tanzania-Zambia or Zambia-Malawi border was identified as a confounding variable. The frequencies of buying and selling new goats, as well as proximity to an international border, to a town and to the Tan-Zam highway were always included in the initial models, as these were of special interest for the scope of the study. Initially, the full model was run, and the variable with the highest conjoined p-value using the Wald Test was removed in a stepwise backward elimination procedure, which was continued until one significant variable remained. Confounding was controlled for in each step, and a variable was judged to be a confounder when it affected the coefficient of other models with >20%. However, no confounder was identified. Selection of the best fitting model was subsequently performed using the Akaike information criteria (AIC). Residual plots were also examined visually according to Dohoo and Martin (45). A p-value of <0.05 was considered statistically significant, but higher p-values are also presented here to show potential associations between a variable and seropositivity.
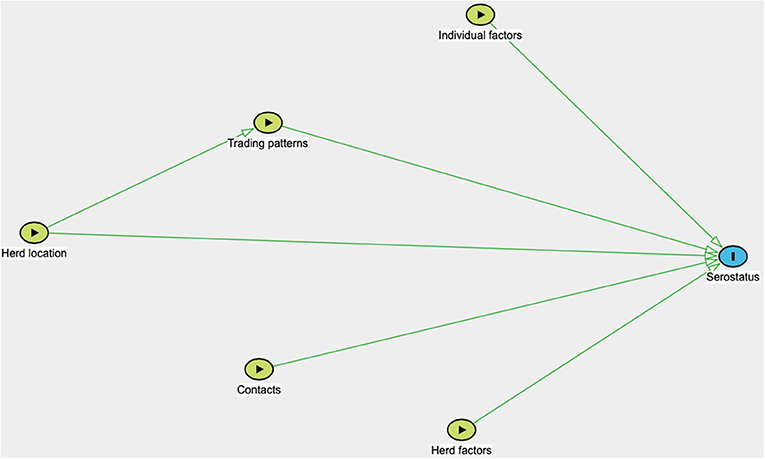
Figure 2. Directed acyclic graph illustrating variables potentially associated with pathogen seroprevalence. Herd location include the village-location, district-location and country-location of the sampled herd, as well as distance to the nearest international border, town and the Tan-Zam highway. Trading patterns include introduction of new animals, selling, buying from traders or at markets, and buying from or selling to other countries. Contact includes contact with wildlife and sheep, goats and cattle from other herds. Individual factors include age, sex, and species. Herd factors include herd size.
Ethical Considerations
The data collection was performed in collaboration with Tanzanian and Zambian district veterinary officers. Informed written consent was acquired prior to data collection. For farmers who were unable to sign the consent sheet, a thumbprint was obtained instead. The study received ethical approval from the International Livestock Research Institute's (ILRI) Institutional Research Ethics Committee (IREC) (ILRI-IREC2018-04).
Results
Descriptive Statistics
In total, 977 animals originating from 324 households were sampled in this study (Table 1). In Tanzania, 491 sheep and goats were sampled, which originated from 164 different households belonging to 41 villages. In Momba and Tunduma districts, 396 and 95 animals were sampled, respectively. In Zambia, 486 animals were sampled, originating from 160 different households in 42 villages. In Mbala and Nakonde districts, 119 and 367 animals were sampled, respectively. The majority of the sampled animals were goats (97%), female (79%) and between 1 and 3 years of age (56%). Almost all of them were local mixed breeds. Herd size ranged from 2 to 210 animals, although only eight herds contained 50 or more animals, and 24 herds 20 or more animals. The median herd size was 8. Around 95% of the sheep and goats grazed on communal grazing grounds for at least part of the year, and 88 and 62% respectively were estimated to be in contact with small ruminants and cattle from other herds at least on a monthly basis. Only 21% of the sampled sheep and goats displayed clinical signs of disease at the time of visit, and 44% were reported to have been sick in the previous 12 months. Commonly reported clinical signs included ocular and nasal discharge, coughing and diarrhea. Only seven households reported that they had vaccinated their small ruminants; all of these were located in Tanzania and had been vaccinated against contagious caprine pleuropneumonia (CCPP) during March to May 2018. Also, 88 and 82% of the farmers reported to never have treated their small ruminants with acaricides or anthelmintic drugs.
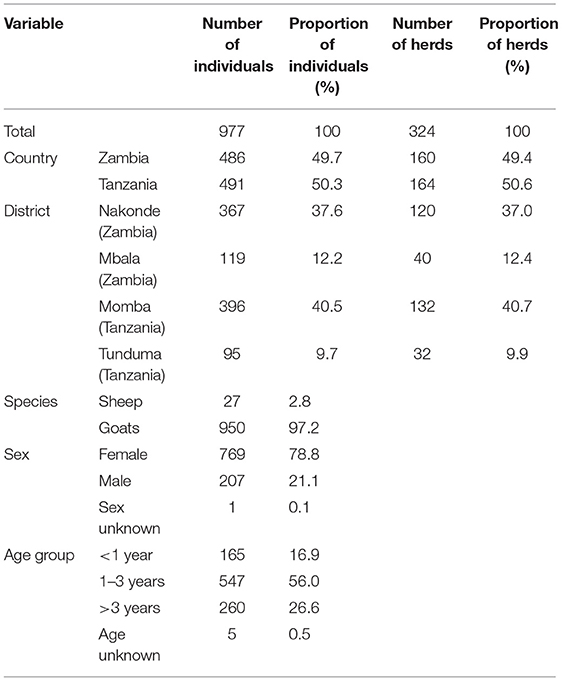
Table 1. Distribution of samples collected from sheep and goats in Zambia and Tanzania in September to October 2018.
The majority (78%) of farmers had not bought, bartered or in any other way acquired new sheep or goats in the previous 12 months, and 62% of these farmers reported only having acquired new animals once when they started keeping small ruminants. The most common source by far for acquiring new small ruminants was from other farmers in the village or nearby villages, but 7.9% reported having bought from traders or at markets at least once. Most of the farmers (46%) had sold sheep or goats within the previous 12 months, while 25% had never sold a sheep or a goat (Table 2).
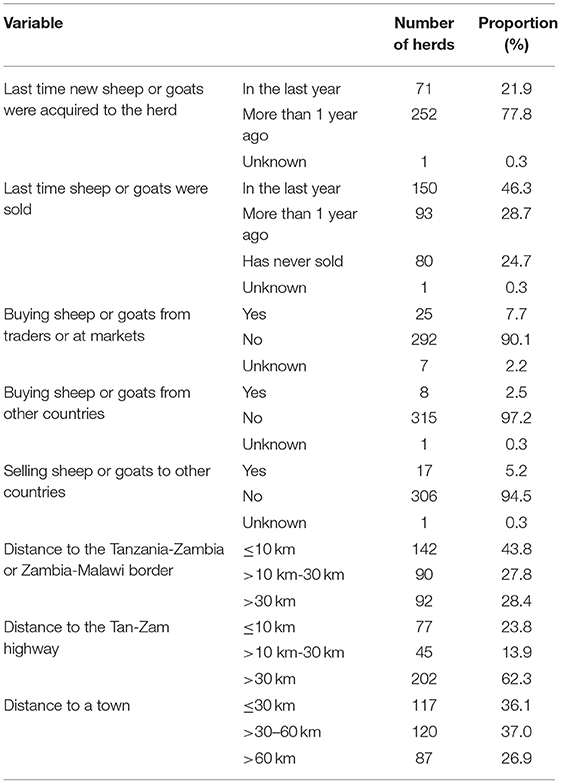
Table 2. Trade routines and distances to the Tanzania-Zambia or Zambia-Malawi border, to a town and to the Tan-Zam highway.
Seroprevalence
Only one goat tested positive for PPRV in Zambia, which equals to an apparent animal-level seroprevalence of 0.21% (95% 0.01–1.14), while 2.85% (95% CI 1.57–4.74) sheep and goats were seropositive in Tanzania. For FMDV, apparent animal-level seroprevalence in Zambia and Tanzania was 1.03% (95% CI 0.33–2.39) and 16.9% (95% CI 13.7–20.5), respectively, while for SGPV, only one goat in Tanzania tested positive, equaling to a seroprevalence of 0.20% (95% CI 0.01–1.13) in Tanzania and 0% (95% CI 0–0.76) in Zambia. For RVFV and Brucella spp., animal-level seroprevalence in Zambia was 2.26% (95% CI 1.14–4.01) and 1.65% (95% CI 0.71–3.22), respectively, while 3.26% (95% CI 1.87–5.24) and 20.0% (95% CI 14.5–26.5) sheep and goats were seropositive in Tanzania (Tables 3, 4).
Apparent herd-level seroprevalence for PPRV was 0.63% (95% CI 0.02–3.43) in Zambia and 7.30% (95% CI 3.84–12.4) in Tanzania. For FMDV and SGPV, herd-level seroprevalence in Zambia was 3.14% (95% CI 1.03–7.19) and 0% (95% CI 0–2.28) respectively, while 33.5% (95% CI 26.4–41.3) and 0.61% (95% CI 0.02–3.35) of the herds were seropositive in Tanzania. For RVFV and Brucella spp., herd-level seroprevalence was 5.62% (95% CI 2.60–10.4) and 5.00% (95% CI 2.18–9.61), respectively, in Zambia, while the corresponding numbers were 9.15% (95% CI 5.21–14.6) and 38.1% (95% CI 26.1–51.2), respectively, in Tanzania (Tables 5, 6).
For FMDV, true unadjusted animal- and herd-level seroprevalence in Zambia were 0.53% (95% CI 0.00–1.90) and 2.66% (95% CI 0.85–6.68), respectively, while the corresponding numbers in Tanzania were 16.5% (95% CI 13.4–20.1) and 33.2% (95% CI 26.4–40.8), respectively. True prevalence could not be calculated for SGPV since the sensitivity of the utilized ELISA is not known. For PPRV and RVFV, true prevalence was the same as the apparent prevalence, since the manufacturers of the ELISA tests that were utilized report 100% sensitivity and specificity. However, if the sensitivity and specificity values found in independent studies were used, true individual-level and herd-level seroprevalence for PPRV in Tanzania was estimated to 2.40% (95% CI 1.18–4.40) and 7.15% (95% CI 3.87–12.5). In Zambia, true animal- and herd-level seroprevalence of PPRV could not be accurately calculated due to the low apparent seroprevalence. For RVFV, true unadjusted animal- and herd-level seroprevalence was 2.49% (95% CI 1.39–4.40) and 6.18% (95% CI 3.28–11.4), respectively, in Zambia, and 3.58% (95% CI 2.21–5.74) and 10.1% (95% CI 6.18–16.0), respectively, in Tanzania. For Brucella spp. in Zambia, true unadjusted herd-level seroprevalence using the sensitivity and specificity values detected in an independent study was estimated to be 3.97% (95% CI 1.48–8.60), while true animal-level seroprevalence could not be calculated due to the low apparent seroprevalence. True seroprevalence for Brucella spp. in Tanzania was estimated to be 20.1% (95% CI 15.0–26.6) at animal-level and 38.5% (95% CI 27.4–51.0) at herd-level (Tables 7, 8).
Approximately 88.3% of the animals and 69.1% of the herds were seronegative to all the tested pathogens. Furthermore, 5.22% of the animals and 8.64% of the herds were seropositive for two or more of the pathogens, and one animal tested positive for three pathogens, i.e., PPRV, FMDV, and RVFV. Animals that were seropositive for FMDV were significantly more likely to be seropositive for PPRV (OR 7.15, p < 0.001), RVFV (OR 7.79, p < 0.001) and Brucella spp. (OR 3.63, p = 0.008). Otherwise, no associations of seropositivity between different pathogens were found.
Predictor Variable Analysis
Multivariable predictor variable analysis was performed for all the pathogens except SGPV, due to the low number of seropositive animals. For PPRV, the analysis was performed on the data from Tanzania since only one animal in Zambia tested positive. Predictor variables associated with herd-level seropositivity for PPRV are shown in Table 9, for FMDV in Table 10, for RVFV in Table 11, and for Brucella spp. in Table 12. Animal-level predictor variables are displayed in Supplementary Tables 1–4. Predictor variables with p-values <0.25 that were later included in the multivariable analysis, are found in Supplementary Tables 5–12. Unless otherwise specified, the results presented here were analyses made of herd-level data.
For PPRV, all seropositive animals were goats, females and originated from herds in daily contact with small ruminants and cattle from other herds. In the herd-level analysis, being situated more than 30 km from an international border was identified as a risk factor (OR 6.83, 95% CI 1.37–34.0, p = 0.019), and in fact no seropositive animals were found within a 10 km radius. Contact with wild ruminants (OR 18.2, 95% CI 1.36–244, p = 0.028) was identified as a risk factor, and in the animal-level analysis, being older than 3 years was associated with increased seropositivity (OR 82.0, 95% CI 2.87–2342, p = 0.010). No animals under 1 year of age tested positive for PPRV antibodies, which could indicate limited circulation of the virus in the 12 months leading up to the time of sampling in the surveyed areas.
In the herd-level analysis for FMDV, sheep and goats in Tanzania were significantly more likely to be seropositive than those in Zambia (OR 20.7, 95% CI 3.38–127, p = 0.001). Households that bought sheep and goats from traders or at markets were also more likely to be seropositive, although this association was not statistically significant (OR 2.47, 95% CI 0.95–6.43, p = 0.065). Furthermore, being situated within 30 km of a town (OR 79.2, 95% CI 4.52–1389, p = 0.003) and the Tanzania-Zambia/Zambia-Malawi border (OR 5.68, 95% CI 1.58–20.3, p = 0.008) was associated with a higher risk of seropositivity. The same was seen for households located more than 30 km of the Tan-Zam highway (OR 11.8, 95% CI 0.93–150.0, p = 0.057), although this was not statistically significant. Furthermore, an association between being seropositive and the presence of sheep in the herd, either solely or in addition to goats, was identified in the analysis (OR 5.20, 95% CI 1.00–26.9, p = 0.049). In the animal-level analysis, an association between seropositivity and a herd size of 21 or more animals was identified (OR 4.75, 95% CI 1.52–14.8, p = 0.007), and animals older than 3 years of age were significantly more likely to be seropositive (OR 5.03, 95% CI 2.46–10.3, p < 0.001). In fact, no animals under 1 year of age tested positive for antibodies to FMDV, indicating limited circulation of virus in the 12 months up to the time of sampling in the surveyed area.
For RVFV, a non-significant association was observed in the herd-level analysis between keeping sheep in the household and seropositivity (OR 3.11, 95% CI 0.78–12.4, p = 0.107). Furthermore, a non-significant association between the herd being situated more than 30 km from the Tanzania-Zambia or Zambia-Malawi borders and increased seroprevalence was identified (OR 2.54, 95% CI 0.86–7.53, p = 0.093). The same non-significant trends were seen in the animal-level analysis.
All animals seropositive for Brucella spp. in this study were goats. The herd-level analysis revealed that small ruminants in Tanzania were significantly more likely to be seropositive than those in Zambia (OR 17.7, 95% CI 6.19–50.4, p < 0.001). However, as two different ELISA kits were used, any comparisons should be made with caution. Interestingly, herds where no new animals had been introduced within the previous 12 months were seropositive to a higher extent, although this association was non-significant (OR 3.72, 95% CI 0.96–14.4, p = 0.057). As opposed to the other pathogens, the seroprevalence was higher within a 10 km distance (OR 4.05, 95% CI 0.94–17.4, p = 0.060), and significantly higher within a 10–30 km distance (OR 4.43, 95% CI 1.22–16.1, p = 0.024) from the Tanzania-Zambia or Zambia-Malawi international borders. In the animal-level analysis, a significant association was observed between seropositivity and being situated within 30 km of a town (OR 6.04, 95% CI 1.03–35.5, p = 0.047).
Clinical Signs
The study found associations between herd seropositivity for PPRV and mortalities in lambs and/or kids (p = 0.020) and in adult sheep and/or goats (p = 0.001) in the herd during year leading up to the study. Also, abortions were significantly less likely to have occurred during the previous 12 months in herds that were seropositive for Brucella spp. (p = 0.022).
Furthermore, an association between FMDV seropositivity and the presence of ocular and nasal discharge at the time of sampling (p = 0.005) was found. The study also detected multiple associations between seroprevalence and observations of clinical signs in the individual animal by the farmer during the 12 months leading up to the study (Table 13). Associations were found between PPRV and coughing (p = 0.019) and diarrhea (p = 0.001, FMDV and coughing (p = 0.001) and diarrhea (p = 0.001), and between RVFV and ocular and nasal discharge (p = 0.001).
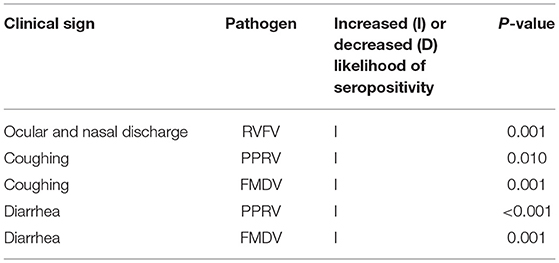
Table 13. Statistically significant associations between clinical signs observed in the individual animal during the year leading up to sample collection, and pathogen seropositivity.
Discussion
This study investigated animal- and herd-level seroprevalence of the transboundary pathogens PPRV, FMDV, SGPV, RVFV and Brucella spp. along the Tanzania-Zambia border. In addition, these results were used to investigate associations between different predictor variables and seroprevalence, focusing on, but not limited to, trade routines and proximity to a town, to the Tan-Zam highway and to the Tanzania-Zambia or Zambia-Malawi borders.
In this study, the detected animal- and herd-level seroprevalence of PPR in Tanzania was 2.85 and 7.30%, respectively, and seropositive animals were found in both Tunduma and Momba districts. The detected seroprevalence was lower than that reported in previous studies, where animal-level seroprevalence has ranged from 13 to 47% (24, 46–52). Potential reasons for this include differences in surveyed years, study design, and study area, as most previous studies were conducted in the northern and central regions of the country. All seropositive animals in this study were goats, although the difference in seroprevalence between species was not statistically significant. While some previous studies in Tanzania did not detect significant differences in PPR seroprevalence in sheep vs. goats (46, 52), others have found higher seroprevalence in goats (50), which can be explained by a higher susceptibility to PPRV infection compared to sheep (53). At the same time, goats are considered more likely to develop severe disease and die following PPRV infection (53), which may explain why e.g., a study in Pakistan detected a significantly higher seroprevalence in sheep (54). PPR vaccination campaigns have been performed previously in both Momba and Tunduma district previously, but these activities were scaled down in 2013 (E.S. Swai, personal communication), i.e., 5 years before the samples for this study were collected. Nine of the 14 seropositive animals in this study were reported to be 5 years or older by the farmer. As the vaccine generally gives rise to life-long immunity (55), it cannot be excluded that some of the seroconversions observed are due to vaccination and not natural infection. In Zambia, only one two-year old male goat tested positive for antibodies to PPRV. The positive animal had been born into the herd and had not been vaccinated for any disease, and none of the four other sampled animals from the same herd were seropositive. This result was in line with the fact that only antibodies without connection to clinical disease have previously been found in Zambia (9). The seropositive goat in this study tested positive on two separate ELISA runs, but the result needs to be verified using another methodology, preferably the virus neutralization test (VNT) or its equivalent.
The seroprevalence of FMDV was significantly higher in Tanzania than in Zambia. In Tanzania, animal-level and herd-level seroprevalence was 16.9 and 33.5%, respectively, and seropositive animals were found in both Tunduma and Momba districts and in 31 different villages. Twenty-one of these villages contained two or more seropositive small ruminants. The detected seroprevalence is lower compared to a previous study conducted in another part of Tanzania, where animal-level seroprevalence of FMDV in small ruminants was 39% (46). The animal-level and herd-level seroprevalence in Zambia was 1.03 and 3.14%, respectively, and seropositive animals were found in both Nakonde and Mbala districts. While previous studies on FMD in Zambia have focused on cattle, the result is similar to findings in a study in Zimbabwe (56), where FMDV seroprevalence in goats was 1.5%. As the area along the Tanzania-Zambia border has repeatedly been identified as a hotspot for FMDV outbreaks in cattle (15–18), the detected seroprevalence in this study was low, especially on the Zambian side of the border. As mortality in adult animals resulting from FMDV infection is low (57), a higher seroprevalence would be expected in a hotspot area, especially since contact rates between the sampled herds and cattle were reported to be high. However, possible explanations for the low seroprevalence are the fact that the aerosol production of FMDV in small ruminants is relatively small (58) and their population turnover rate is high compared with cattle (59). Hence, the present findings do not exclude the potential of small ruminants playing a role in the epidemiology of FMDV in the region.
SGPV has yet to be detected in Zambia, while its presence has been confirmed in Tanzania (24) and the DRC (11). In this study, only one animal tested positive for the presence of antibodies to SGPV, a 2-year old female goat in Momba district in Tanzania, corresponding to an animal- and herd-level seroprevalence of 0.20 and 0.61%, respectively. As SGPV is a highly contagious disease and none of the other two animals in the herd tested positive, the possibility of this test being a false positive should not be excluded.
The animal-level and herd-level seroprevalence of RVFV in this study was 2.26% and 5.62% in Zambia, and 3.26 and 9.15% in Tanzania. As no RVFV outbreak has been reported in Zambia since 1989 (27) or in Tanzania since 2007 (28, 29), these results may indicate inter-epidemic circulation of RVFV in the area, something that has previously been found in both Zambia (60, 61) and Tanzania (62, 63). This result is similar to findings in previous studies conducted during inter-epizootic periods in Tanzania, where animal-level seroprevalence in sheep and goats ranged from 5.4 to 11.7% (62–64). RVF vaccinations were performed in Tunduma and Momba district until 2008, i.e., 10 years prior to this study. As none of the RVFV seropositive animals in Tanzania in this study were older than 8 years, it is unlikely that they had seroconverted due to vaccinations rather than natural infection.
In this study, detected animal- and herd-level seroprevalence of Brucella spp. was 1.65 and 5.00%, respectively, in Zambia, and 20.0 and 38.1%, respectively, in Tanzania, and antibodies to the bacteria were detected in all the surveyed districts. In Zambia, the results are in line with a previous study (65), while it is lower than the seropositivity rate detected in sheep and goats at two informal small livestock markets in Zambia (66). The detected seroprevalence in Tanzania was higher than that previously reported (67–70), which at least in part is likely to be due to differences in study design and laboratory tests used. All seropositive animals in this study were goats, and in Tanzania, several previous studies have detected higher seroprevalence in this species compared to sheep (67, 70).
In this study, herds seropositive for FMDV and PPRV were significantly more likely to be situated more than 30 km from an international border compared to herds located at closer proximity. The same tendency was observed for RVFV although the association was non-significant. These findings stand in contrasts to results from previous spatio-temporal studies (15–18), where reported outbreaks of FMD in cattle in Zambia and Tanzania have been shown to be clustered close to several international borders, including the Tanzania-Zambia border. However, all the participating households in the present study were situated within a 90 km radius of a border. In a spatial analysis, an outbreak of a disease in any of these households would probably have been identified as having occurred in proximity to an international border. For Brucella spp., on the other hand, the seroprevalence was higher in herds located at closer proximity to a border. Unfortunately, not all samples in Tanzania could be analyzed for the presence of antibodies to Brucella spp., and among the herds that were situated more than 30 km from the border, only 43% were included. As disproportionately few herds in this group were included in the analysis, this result needs to be interpreted with caution. In addition to the importance of border proximity, clustering of FMD outbreaks in cattle along the Tan-Zam highway have been identified (15, 16). One probable reason is that cattle are often moved along this road to markets or slaughterhouses in other parts of Zambia and/or Tanzania. In this study, however, seroprevalence of FMD in sheep and goats was found to be higher in households situated 30 km or more from this major infrastructural route, although this association was non-significant. Compared with cattle, small ruminants are often sold locally (71), making less use of major transport routes such as the Tan-Zam highway, thereby possibly reducing (but not eliminating) its epidemiological significance. It should also be noted that most of the participating households were situated more than 30 km from the highway and therefore, this result should be interpreted with caution.
Furthermore, a significant association between proximity to a town and seropositivity for FMDV was identified based on herd-level data, and for Brucella spp. based on animal-level data. In the Tanzania-Zambia border region, ‘goat soup' (or supu ya mbuzi in Kiswahili) is considered a delicacy and is often sold at local restaurants. Therefore, many farmers opt to trek or transport their goats to a nearby town to sell them to a restaurant owner, which may explain the observed association with FMDV seropositivity. As many restaurants have limited access to refrigerators and freezers, the goats will be kept alive until their meat is needed, possibly encountering local animals in communal pastures, enabling them to exchange infectious pathogens. Furthermore, cities are more likely to contain livestock markets, and proximity to these markets has been associated with seropositivity for FMDV in cattle in Ethiopia in a previous study (72).
The introduction of new sheep or goats to the herd (through other means than within-herd births of lambs and kids) within the previous 12 months was identified as a non-significant protective factor for Brucella spp. in this study. This result contradicts previous studies, where frequent purchase of new animals has been identified as a risk factor for brucellosis (73, 74) and FMD (75). In the present study, acquiring new animals for the herd was rare, and those who did mostly got them from other farmers in the village or from nearby villages. The fact that most sheep and goats grazed on communal grazing grounds and were in frequent contact with sheep and goats from other herds, i.e., regularly coming into contact with the potential pool of source animals from which the farmer would be buying, could explain why this variable generally was not identified as a risk factor in this study. For Brucella spp., one potential explanation for the negative association between having introduced small ruminants to the herd within the past year and seropositivity is differences in economic status and access to capital. However, this needs to be investigated in future studies.
In addition, a non-significant association between seropositivity for FMDV and buying sheep and goats at markets or from traders was identified. At small livestock markets, animals of different species are often kept in proximity under stressful conditions, which facilitates pathogen exposure and spread (76). Livestock markets have previously been associated with the spread of infectious diseases (34, 77), and as traders often bring previously purchased animals with them when moving between villages, thereby allowing them to come into contact with local animals, traders risk contributing to the spread of disease (78).
Interestingly, annual contact with wild ruminants was identified as a risk factor for PPRV. In addition to sheep and goats, which are the main hosts of the virus, antibodies have been detected in various species of wild ruminants (79–81). In a previous study in Tanzania, a potential spillover of PPRV from domestic to wild ruminants has been observed (82), and the possibility of transmission in the opposite direction should not be ruled out. The importance of wildlife in the epidemiology of PPRV is currently being investigated due to its relevance in the planned eradication of the virus (7, 83). Furthermore, an association between keeping sheep, either solely or in addition to goats, and seropositivity for FMDV and RVFV were found, although for RVFV, this finding was not statistically significant. Previous studies in Ethiopia have identified that keeping cattle and small ruminants together is a risk factor associated with FMDV seroprevalence in cattle (72, 84). A plausible explanation for this observation could be the mild clinical picture in sheep and goats, which enables them to act as silent spreaders to cattle (57). However, some studies also indicate that sheep (85) and goats (86) are less efficient transmitters of FMDV compared to e.g., cattle, and there are indications that outbreaks in sheep often are self-cleared due to poor transmissibility (58). For RVFV, sheep are generally considered more likely than goats and cattle to develop severe disease (87), seroprevalence is generally higher in sheep (88), and there are indications that this species are more likely to be exposed to RVF during outbreaks (89). However, to the authors' knowledge, there is currently no data indicating that sheep are more infectious, which in turn could lead to a higher seroprevalence in other species in mixed herds. Furthermore, associations on animal-level data were found between seropositivity for FMDV, and ages above 3 years and herd sizes of 21 animals or more. These findings were expected, since older animals have had more time to be exposed to pathogens (72) and since the infectious pressure often is increased in herds that contain many animals (90).
For FMDV and Brucella spp., the seroprevalence was significantly higher in Tanzania than in Zambia, although for Brucella spp. this difference should be interpreted with caution as two different ELISA kits were used. For PPRV, the seroprevalence was significantly higher in Tanzania in univariable analysis, while only data from Tanzania were included in the multivariable analysis as only one animal was seropositive in Zambia. Given the absence of a natural demarcation between the two countries and the high levels of cross-border animal movement and trade, it was expected that the difference in seroprevalence would be less pronounced. One possible explanation for the higher seroprevalence in Tanzania of FMDV, Brucella spp. and PPRV is that at the time of sample procurement, trade in small ruminants was mainly directed from Zambia to Tanzania due to the high demand for goat meat on the Tanzanian side, coupled with comparatively low prices in Zambia. Furthermore, the animals that were sampled in Tanzania in this study were significantly older than the ones in Zambia (p < 0.001) and were in contact more often with sheep and goats (p < 0.001) and cattle (p < 0.001) from other herds. Herd sizes in Tanzania were also significantly larger than in Zambia (p = 0.020).
In addition, the study detected associations between various clinical signs reported by the farmers and seropositivity for certain pathogens. Most of these were expected, however one interesting finding was that abortions were significantly less likely to have occurred in herds that were seropositive for Brucella spp. While abortion in pregnant females is one of the most important sequelae to infection with Brucella spp., most sheep and goats will only abort once in their lifetime (30). It is possible that the seropositive females were infected, aborted and seroconverted more than a year before the samples were collected. Also, other aspects related to reproductive performance such as infertility and fetal loss in early pregnancy, which are easily overlooked by the farmer, were not included in this study.
While this study generated interesting results, it has some limitations. The lack of a registry of the populations of small ruminant farmers and of sheep and goats in both Zambia and Tanzania, and of disease prevalence in different regions, makes it challenging to design and execute a completely randomized study and to calculate sampling weights for adjustment of estimates. As the diseases included in this study are contagious, it can be expected for positive cases to be clustered in herds and villages. The sample size was however calculated based on a simple random sample, and hence did not take aspects such as clustering into account. In an attempt to compensate for this, the sample size calculation assumed a 50% true prevalence and used the sensitivity and specificity values from the ELISA with the lowest values, to yield a large necessary sample size. Also, to reduce potential clustering effects, we opted to collect the samples in a relatively large number of villages and households, a choice that was based on the combined knowledge and experience of the author group. Ideally, however, the study should have been designed as a multi-staged cluster sampling (91), with a listing of households with different numbers of animals. Also, differences in herd size were not considered when estimating seroprevalence, and while variations in size in general were small among the surveyed herds, with 50% having <8 animals, taking these differences into account should ideally have been done. The fact that the only three animals were sampled also in large herds, may have introduced a bias toward the null, and a lower prevalence is likely reported for these farms than may be true. Furthermore, to reduce the inherent biases associated with snowball sampling, such as a higher likelihood of e.g., respondents with wide social networks to be included in research, an attempt was made to stratify the included households based on their herd size. This stratification was based on the average herd sizes of sheep and goats in Zambia and Tanzania, but as there are large variations in herd sizes in both countries (38, 92), average herd size is probably a poor indicator of the number of animals kept by the average Zambian or Tanzanian farmer. In addition, the fact that it generally was the farmer who chose which animals were sampled is a potential source of bias. While this selection process appeared random, it is possible that some farmers chose his or her healthiest animals in order to present him- or herself as a good farmer, while others may have selected their sickest animals in a desire to find out why they are not doing well. Furthermore, although relatively uncommon, there are pastoralist households in the region where the study was conducted (93) and a few of them participated in the study. Comparing the seroprevalence between pastoralist herds and mixed crop-livestock herds would have added interesting information, but unfortunately this aspect was not included. Lastly, this study used measurements of linear distance to e.g., towns and borders, but a more representative measurement would be distance by roads. Unfortunately, accurate infrastructural information for the region, which in addition to major roads also includes smaller, more informal routes, was not included or in many instances was not available. As small ruminants are often sold in the village or in a nearby town, these smaller routes can play an important role in disease epidemiology.
Conclusions
This study indicates that FMDV, RVFV, and Brucella spp. are circulating in the Tanzania-Zambia border region, while SGPV did not appear to be widely present. The low seroprevalence of PPRV in Zambia implies that exposure to the virus is uncommon. However, its continued presence in Tanzania, together with the high degree of cross-border livestock movement, warrants continuous surveillance for PPRV in the area. The associations between seroprevalence and trade routines and proximity to a border, a town or the Tan-Zam highway varied in this study. It is recommended that these aspects should be investigated further in larger studies in future. If possible, seroepidemiological data should be combined with spatio-temporal analyses, ideally incorporating aspects such as the locations of small ruminant markets, informal livestock movement routes and temporal trade fluctuations. This has the potential to reduce the impact of inherent biases in spatio-temporal studies, e.g., the in general poor willingness among resource-constrained farmers to report disease and the lack of resources among veterinary personnel to confirm diagnoses (94, 95).
Data Availability Statement
The raw data supporting the conclusions of this article will be made available by the authors, without undue reservation.
Ethics Statement
The animal study was reviewed and approved by the International Livestock Research Institute's (ILRI) Institutional Research Ethics Committee (IREC) (ILRI-IREC2018-04). Written informed consent was obtained from the owners for the participation of their animals in this study.
Author Contributions
SLy, JL, MM, GM, KA, GD, MB, and JW: conceptualization. SLy, CM, SLi, LM, EO, and EW: investigation. SLy, SLi, LM, EO, and EW: data curation. SLy and JL: formal analysis. SLy, MM, GM, and JW: project administration. SLy: writing—original draft. JL, MM, GM, CM, KA, GD, SLi, LM, EO, EW, MB, and JW: writing—review and editing. JL, MM, GM, MB, and JW: supervision. MM and JW: funding acquisition. All authors contributed to the article and approved the submitted version.
Funding
This study was funded by the Swedish Research Council (Grant Nos. 2018-03956, 2016-05667, and 348-2014-4293).
Conflict of Interest
The authors declare that the research was conducted in the absence of any commercial or financial relationships that could be construed as a potential conflict of interest.
Publisher's Note
All claims expressed in this article are solely those of the authors and do not necessarily represent those of their affiliated organizations, or those of the publisher, the editors and the reviewers. Any product that may be evaluated in this article, or claim that may be made by its manufacturer, is not guaranteed or endorsed by the publisher.
Acknowledgments
Firstly, the authors are very grateful to the research participants for their important contributions to this study. We are also grateful for the invaluable help provided by the district veterinary personnel in Nakonde, Mbala, Tunduma, and Momba districts, and by the laboratory personnel at the University of Zambia (UNZA) and the Sokoine University of Agriculture (SUA). The field assistance of Mr. Edson Kinimi of SUA is highly appreciated. The LTELISA Brucella competitive ELISA kits were obtained through The World Academy of Sciences (TWAS) Grant Ref. No. 19-033 RG/BIO/AF/AC_I – FR3240310150.
Supplementary Material
The Supplementary Material for this article can be found online at: https://www.frontiersin.org/articles/10.3389/fvets.2022.809128/full#supplementary-material
References
1. Perry BD, Randolph TE, McDermott JJ, Scones KR, Thornton PK. Investing in Animal Health Research to Alleviate Poverty. Nairobi: ILRI (2002).
2. FAO OIE. The Global Framework for the Progressive Control of Transboundary Animal Diseases (GF-TADs) Paris: OIE, FAO (2004).
3. Chazya R, Muma JB, Mwacalimba KK, Karimuribo E, Mkandawire E, Simuunza M. A qualitative assessment of the risk of introducing peste des petits ruminants into northern zambia from Tanzania. Vet Med Int. (2014) 2014:202618. doi: 10.1155/2014/202618
4. Chazya R, Mkandawire E, Muma JB, Mwacalimba KK, Karimuribo E, Simuunza M. Peste des petits ruminants (PPR) introduction into Nothern Zambia from Tanzania via live goat consignment: a quantitative risk assessment study. Int J Sci Agricul. (2015) 2:1–23.
5. Karimuribo E, Chungu G, Kambarage DM. A qualitative assessment of the risk of introducing Contagious Caprine Pleuropneumonia into Zambia from south-western Tanzania. Tanzania Vet J. (2014) 29.
8. Torsson E, Kgotlele T, Berg M, Mtui-Malamsha N, Swai ES, Wensman JJ, et al. History and current status of peste des petits ruminants virus in Tanzania. Infect Ecol Epidemiol. (2016) 6:32701. doi: 10.3402/iee.v6.32701
9. OIE. Peste des petits ruminants, Zambia Final report 2016. Available online at: https://www.oie.int/wahis_2/public/wahid.php/Reviewreport/Review?reportid=19686 (accessed December 28, 2020).
10. Bwihangane BA, Misinzo G, Sviteck N, Bebora LC, George GC. Sero-Surveillance, Risk Factors and Molecular Diagnosis of Peste des Petits Ruminants Virus in South Kivu, Democratic Republic of Congo. Morogoro: Sokoine University of Agriculture (2015).
11. Birindwa BA, George GC, Ntagereka BP, Christoper O, Lilly BC. Mixed infection of peste-des-petits ruminants and Capripox in goats in South Kivu, Democratic Republic of Congo. J Adv Vet Anim Res. (2017) 4:348–55. doi: 10.5455/javar.2017.d233
12. Tshilenge GM, Walandila JS, Kikukama DB, Masumu J, Katshay Balowa L, Cattoli G, et al. Peste des petits ruminants viruses of lineages II and III identified in the Democratic Republic of the Congo. Vet Microbiol. (2019) 239:108493. doi: 10.1016/j.vetmic.2019.108493
13. FAO. Livestock Epidemic Causing Havoc in Democratic Republic of the Congo. FAO (2012). Available online at: rom: http://www.fao.org/food-chain-crisis/resources/news/detail/en/c/150852/ (accessed December 20, 2020).
14. OIE. Event Summary: Peste des Petits Ruminants, Angola. OIE (2013). Available online at: https://www.oie.int/wahis_2/public/wahid.php/Reviewreport/Review/viewsummary?fupser=&dothis=&reportid=12408 (accessed July 1, 2019).
15. Hamoonga R, Stevenson MA, Allepuz A, Carpenter TE, Sinkala Y. Risk factors for foot-and-mouth disease in Zambia, 1981-2012. Prev Vet Med. (2014) 114:64–71. doi: 10.1016/j.prevetmed.2014.01.014
16. Allepuz A, Stevenson M, Kivaria F, Berkvens D, Casal J, Picado A. Risk factors for foot-and-mouth disease in Tanzania, 2001-2006. Transbound Emerg Dis. (2015) 62:127–36. doi: 10.1111/tbed.12087
17. Sinkala Y, Simuunza M, Muma JB, Pfeiffer DU, Kasanga CJ, Mweene A. Foot and mouth disease in Zambia: spatial and temporal distributions of outbreaks, assessment of clusters and implications for control. Onderstepoort J Vet Res. (2014) 81:E1–6. doi: 10.4102/ojvr.v81i2.741
18. Picado A, Speybroeck N, Kivaria F, Mosha RM, Sumaye RD, Casal J, et al. Foot-and-mouth disease in Tanzania from 2001 to 2006. Transbound Emerg Dis. (2011) 58:44–52. doi: 10.1111/j.1865-1682.2010.01180.x
19. Perry BD, Hedger RS. History and epidemiology of foot-and mouth disease in Zambia: a review. Trop Anim Health Prod. (1984) 16:107–14. doi: 10.1007/BF02239854
20. Mansley LM, Dunlop PJ, Whiteside SM, Smith RG. Early dissemination of foot-and-mouth disease virus through sheep marketing in February 2001. Vet Rec. (2003) 153:43–50. doi: 10.1136/vr.153.2.43
21. Nyaguthii DM, Armson B, Kitala PM, Sanz-Bernardo B, Di Nardo A, Lyons NA. Knowledge and risk factors for foot-and-mouth disease among small-scale dairy farmers in an endemic setting. Vet Res. (2019) 50:33. doi: 10.1186/s13567-019-0652-0
22. Tuppurainen ESM, Venter EH, Shisler JL, Gari G, Mekonnen GA, Juleff N, et al. Review: capripoxvirus diseases: current status and opportunities for control. Transbound Emerg Dis. (2017) 64:729–45. doi: 10.1111/tbed.12444
23. Rao TV, Bandyopadhyay SK. A comprehensive review of goat pox and sheep pox and their diagnosis. Anim Health Res Rev. (2000) 1:127–36. doi: 10.1017/S1466252300000116
24. Kgotlele T, Chota A, Chubwa C, Nyasebwa O, Lyimo B, Torsson E, et al. Detection of peste des petits ruminants and concurrent secondary diseases in sheep and goats in Ngorongoro district, Tanzania. Comp Clin Path. (2019) 28:755–9. doi: 10.1007/s00580-018-2848-5
25. Javelle E, Lesueur A, Pommier de Santi V, de Laval F, Lefebvre T, Holweck G, et al. The challenging management of Rift Valley Fever in humans: literature review of the clinical disease and algorithm proposal. Ann Clin Microbiol Antimicrob. (2020) 19:4. doi: 10.1186/s12941-020-0346-5
26. Ikegami T, Makino S. The pathogenesis of Rift Valley fever. Viruses. (2011) 3:493–519. doi: 10.3390/v3050493
27. Dautu G, Sindato C, Mweene AS, Samui KL, Roy P, Noad R, et al. Rift Valley fever: real or perceived threat for Zambia. Onderstepoort J Vet Res. (2012) 79:a466. doi: 10.4102/ojvr.v79i2.466
28. Sindato C, Karimuribo ED, Pfeiffer DU, Mboera LE, Kivaria F, Dautu G, et al. Spatial and temporal pattern of Rift Valley fever outbreaks in Tanzania; 1930 to 2007. PLoS ONE. (2014) 9:e88897. doi: 10.1371/journal.pone.0088897
29. Ahmed A, Makame J, Robert F, Julius K, Mecky M. Sero-prevalence and spatial distribution of Rift Valley fever infection among agro-pastoral and pastoral communities during Interepidemic period in the Serengeti ecosystem, northern Tanzania. BMC Infect Dis. (2018) 18:276. doi: 10.1186/s12879-018-3183-9
30. Díaz Aparicio E. Epidemiology of brucellosis in domestic animals caused by Brucella melitensis, Brucella suis and Brucella abortus. Rev Sci Tech. (2013) 32:43–51, 3–60. doi: 10.20506/rst.32.1.2187
31. Seleem MN, Boyle SM, Sriranganathan N. Brucellosis: a re-emerging zoonosis. Vet Microbiol. (2010) 140:392–8. doi: 10.1016/j.vetmic.2009.06.021
32. Delgado C, Rosegant M, Steinfeld H, Ehui S, Courbois C. Livestock to 2020 The Next Food Revolution. Washington, DC: IFPRI (1999).
34. Fèvre EM, Coleman PG, Odiit M, Magona JW, Welburn SC, Woolhouse ME. The origins of a new Trypanosoma brucei rhodesiense sleeping sickness outbreak in eastern Uganda. Lancet. (2001) 358:625–8. doi: 10.1016/S0140-6736(01)05778-6
35. Kilpatrick AM, Chmura AA, Gibbons DW, Fleischer RC, Marra PP, Daszak P. Predicting the global spread of H5N1 avian influenza. Proc Natl Acad Sci USA. (2006) 103:19368–73. doi: 10.1073/pnas.0609227103
36. Kendall C, Kerr LR, Gondim RC, Werneck GL, Macena RH, Pontes MK, et al. An empirical comparison of respondent-driven sampling, time location sampling, and snowball sampling for behavioral surveillance in men who have sex with men, Fortaleza, Brazil. AIDS Behav. (2008) 12(4 Suppl.):S97–104. doi: 10.1007/s10461-008-9390-4
37. United Republic of Tanzania. 2016/17 Annual Agriculture Sample Survey Crop & Livestock Report. Dar Es Salaam, Tanzania (2017).
38. Ministry of Fisheries and Livestock. The 2017/2018 livestock and aquaculture census summary report. In: Office CS, editors. Livestock MoFa, Lusaka: Ministry of Fisheries and Livestock (2019).
39. Humphry RW, Cameron A, Gunn GJ. A practical approach to calculate sample size for herd prevalence surveys. Prev Vet Med. (2004) 65:173–88. doi: 10.1016/j.prevetmed.2004.07.003
40. Libeau G, Préhaud C, Lancelot R, Colas F, Guerre L, Bishop DH, et al. Development of a competitive ELISA for detecting antibodies to the peste des petits ruminants virus using a recombinant nucleoprotein. Res Vet Sci. (1995) 58:50–5. doi: 10.1016/0034-5288(95)90088-8
41. Comptet L, Pourquier P, Marié J-L, Davoust B. Preliminary validation of the ID screen rift valley fever multi-species ELISA. In: Poster Presented at the 2010 EAVLD Meeting. Lelystad: EAVLD (2010).
42. Kortekaas J, Kant J, Vloet R, Cêtre-Sossah C, Marianneau P, Lacote S, et al. European ring trial to evaluate ELISAs for the diagnosis of infection with Rift Valley fever virus. J Virol Methods. (2013) 187:177–81. doi: 10.1016/j.jviromet.2012.09.016
43. Biancifiori F, Garrido F, Nielsen K, Moscati L, Durán M, Gall D. Assessment of a monoclonal antibody-based competitive enzyme linked immunosorbent assay (cELISA) for diagnosis of brucellosis in infected and Rev. 1 vaccinated sheep and goats. New Microbiol. (2000) 23:399–406.
44. Rogan WJ, Gladen B. Estimating prevalence from the results of a screening test. Am J Epidemiol. (1978) 107:71–6. doi: 10.1093/oxfordjournals.aje.a112510
45. Dohoo I, Martin W, Stryhn H. Veterinary Epidemiologic Research. Charlottetown, PEI: AVC Inc. (2003).
46. Torsson E, Berg M, Misinzo G, Herbe I, Kgotlele T, Paarni M, et al. Seroprevalence and risk factors for peste des petits ruminants and selected differential diagnosis in sheep and goats in Tanzania. Infect Ecol Epidemiol. (2017) 7:1368336. doi: 10.1080/20008686.2017.1368336
47. Muse EA, Karimuribo ED, Gitao GC, Misinzo G, Mellau LS, Msoffe PL, et al. Epidemiological investigation into the introduction and factors for spread of Peste des Petits Ruminants, southern Tanzania. Onderstepoort J Vet Res. (2012) 79:457. doi: 10.4102/ojvr.v79i2.457
48. Mbyuzi AO, Komba EV, Kimera SI, Kambarage DM. Sero-prevalence and associated risk factors of peste des petits ruminants and contagious caprine pleuro-pneumonia in goats and sheep in the Southern Zone of Tanzania. Prev Vet Med. (2014) 116:138–44. doi: 10.1016/j.prevetmed.2014.06.013
49. Kivaria FM, Kwiatek O, Kapaga AM, Swai ES, Libeau G, Moshy W, et al. The incursion, persistence and spread of peste des petits ruminants in Tanzania: epidemiological patterns and predictions. Onderstepoort J Vet Res. (2013) 80:593. doi: 10.4102/ojvr.v80i1.593
50. Swai ES, Kapaga A, Kivaria F, Tinuga D, Joshua G, Sanka P. Prevalence and distribution of Peste des petits ruminants virus antibodies in various districts of Tanzania. Vet Res Commun. (2009) 33:927–36. doi: 10.1007/s11259-009-9311-7
51. Karimuribo EDL P.M., Mellau L.S.B., Swai E.S. Retrospective study on sero-epidemiology of peste des petits ruminants before its official confirmation in norhtern Tanzania in 2008. Roavs. (2011) 1:184–7.
52. Kgotlele T, Torsson E, Kasanga C, Wensman JJ, Misinzo G. Seroprevalence of Peste Des Petits Ruminants virus from samples collected in different regions of Tanzania in 2013 and 2015. J Vet Sci Technol. (2016) 7:394. doi: 10.4172/2157-7579.1000394
53. Kumar N, Maherchandani S, Kashyap SK, Singh SV, Sharma S, Chaubey KK, et al. Peste des petits ruminants virus infection of small ruminants: a comprehensive review. Viruses. (2014) 6:2287–327. doi: 10.3390/v6062287
54. Abubakar M, Jamal SM, Arshed MJ, Hussain M, Ali Q. Peste des petits ruminants virus (PPRV) infection; its association with species, seasonal variations and geography. Trop Anim Health Prod. (2009) 41:1197–202. doi: 10.1007/s11250-008-9300-9
55. Mariner JC, Gachanja J, Tindih SH, Toye P. A thermostable presentation of the live, attenuated peste des petits ruminants vaccine in use in Africa and Asia. Vaccine. (2017) 35:3773–9. doi: 10.1016/j.vaccine.2017.05.040
56. Bhebhe B, Vhoko K, Tsabalala P, Makaya PV, Mdlongwa E, Pfuneyi DM. Seroprevalence of foot-and-mouth disease in goats from Matabeleland South Province of Zimbabwe. Zimbabwe Vet J. (2016) 34:1–4.
57. Grubman MJ, Baxt B. Foot-and-mouth disease. Clin Microbiol Rev. (2004) 17:465–93. doi: 10.1128/CMR.17.2.465-493.2004
58. Kitching RP, Hughes GJ. Clinical variation in foot and mouth disease: sheep and goats. Rev Sci Techn. (2002) 21:505–12. doi: 10.20506/rst.21.3.1342
59. Otte M, Chilonda P. Cattle and Small Ruminant Production Systems in Sub-Saharan Africa - A Systematic Review. Rome: Food and Agriculture Organization of the United Nations (2002). doi: 10.5367/000000003101294451
60. Davies FG, Kilelu E, Linthicum KJ, Pegram RG. Patterns of Rift Valley fever activity in Zambia. Epidemiol Infect. (1992) 108:185–91. doi: 10.1017/S0950268800049633
61. Saasa N, Kajihara M, Dautu G, Mori-Kajihara A, Fukushi S, Sinkala Y, et al. Expression of a recombinant nucleocapsid protein of rift valley fever virus in vero cells as an immunofluorescence antigen and its use for serosurveillance in traditional cattle herds in Zambia. Vector Borne Zoonotic Dis. (2018) 18:273–7. doi: 10.1089/vbz.2017.2186
62. Sumaye RD, Geubbels E, Mbeyela E, Berkvens D. Inter-epidemic transmission of Rift Valley fever in livestock in the Kilombero River Valley, Tanzania: a cross-sectional survey. PLoS Negl Trop Dis. (2013) 7:e2356. doi: 10.1371/journal.pntd.0002356
63. Wensman JJ, Lindahl J, Wachtmeister N, Torsson E, Gwakisa P, Kasanga C, et al. A study of Rift Valley fever virus in Morogoro and Arusha regions of Tanzania - serology and farmers' perceptions. Infect Ecol Epidemiol. (2015) 5:30025. doi: 10.3402/iee.v5.30025
64. Kifaro EG, Nkangaga J, Joshua G, Sallu R, Yongolo M, Dautu G, et al. Epidemiological study of Rift Valley fever virus in Kigoma, Tanzania. Onderstepoort J Vet Res. (2014) 81:E1–5. doi: 10.4102/ojvr.v81i2.717
65. Muma JB, Samui KL, Siamudaala VM, Oloya J, Matop G, Omer MK, et al. Prevalence of antibodies to Brucella spp. and individual risk factors of infection in traditional cattle, goats and sheep reared in livestock-wildlife interface areas of Zambia. Trop Anim Health Prod. (2006) 38:195–206. doi: 10.1007/s11250-006-4320-9
66. Lysholm S, Fischer K, Lindahl JF, Munyeme M, Wensman JJ. Seropositivity rates of zoonotic pathogens in small ruminants and associated public health risks at informal urban markets in Zambia. Acta Trop. (2022) 225:106217. doi: 10.1016/j.actatropica.2021.106217
67. Mellau LSB, Kuya SL, Wambura PN. Seroprevalence of brucellosis in domestic ruminants in livestock-wildlife interface: a case study of Ngorongoro Conservation Area, Arusha, Tanzania. Tanzania Vet J. (2009) 26:44–50. doi: 10.4314/tvj.v26i1.49234
68. Shirima GM, Kunda JS. Prevalence of brucellosis in the human, livestock and wildlife interface areas of Serengeti National Park, Tanzania. Onderstepoort J Vet Res. (2016) 83:a1032. doi: 10.4102/ojvr.v83i1.1032
69. Assenga JA, Matemba LE, Muller SK, Malakalinga JJ, Kazwala RR. Epidemiology of Brucella infection in the human, livestock and wildlife interface in the Katavi-Rukwa ecosystem, Tanzania. BMC Vet Res. (2015) 11:189. doi: 10.1186/s12917-015-0504-8
70. Ntirandekura JB, Matemba LE, Kimera SI, Muma JB, Karimuribo ED. Brucellosis and its associated risk factors to humans and domestic ruminants in Kagera Ecosystem, Tanzania. Tanzania Vet J. (2021) 21:523–30. doi: 10.4314/ahs.v21i2.6
71. de Haan NC, Kimani T, Rushton J, Lubroth J. Why is small ruminant health important - peste des petits ruminants and its impact on poverty and economics? In: Munir M, editor. Peste des Petits Ruminants Virus. Berlin; Heidelberg: Springer Berling Heidelberg (2015). p. 195–226. doi: 10.1007/978-3-662-45165-6_12
72. Megersa B, Beyene B, Abunna F, Regassa A, Amenu K, Rufael T. Risk factors for foot and mouth disease seroprevalence in indigenous cattle in Southern Ethiopia: the effect of production system. Trop Anim Health Prod. (2009) 41:891–8. doi: 10.1007/s11250-008-9276-5
73. Asmare K, Sibhat B, Molla W, Ayelet G, Shiferaw J, Martin AD, et al. The status of bovine brucellosis in Ethiopia with special emphasis on exotic and cross bred cattle in dairy and breeding farms. Acta Trop. (2013) 126:186–92. doi: 10.1016/j.actatropica.2013.02.015
74. Nthiwa D, Alonso S, Odongo D, Kenya E, Bett B. zoonotic pathogen seroprevalence in cattle in a wildlife-livestock interface, Kenya. Ecohealth. (2019) 16:712–25. doi: 10.1007/s10393-019-01453-z
75. Osmani A, Robertson ID, Habib I. Seroprevalence and risk factors for foot-and-mouth disease in cattle in Baghlan Province, Afghanistan. Vet Med Sci. (2021) 7:1263–75. doi: 10.1002/vms3.477
76. Naguib MM, Li R, Ling J, Grace D, Nguyen-Viet H, Lindahl JF. Live and wet markets: food access versus the risk of disease emergence. Trends Microbiol. (2021) 29:573–81. doi: 10.1016/j.tim.2021.02.007
77. Mohamed EM, Elfadil AAM, El-Sanousi EM, Ibrahaem HH, Mohamed-Noor SE, Abdalla MA, et al. Seroprevalence and risk factors of caprine brucellosis in Khartoum state, Sudan. Vet World. (2018) 11:511–8. doi: 10.14202/vetworld.2018.511-518
78. Lysholm S, Johansson Wensman J, Munyeme M, Fischer K. Perceptions and practices among Zambian sheep and goat traders concerning small ruminant health and disease. PLoS ONE. (2020) 15:e0233611. doi: 10.1371/journal.pone.0233611
79. Banyard AC, Parida S, Batten C, Oura C, Kwiatek O, Libeau G. Global distribution of peste des petits ruminants virus and prospects for improved diagnosis and control. J Gen Virol. (2010) 91(Pt 12):2885–97. doi: 10.1099/vir.0.025841-0
80. Munir M. Role of wild small ruminants in the epidemiology of peste des petits ruminants. Transbound Emerg Dis. (2014) 61:411–24. doi: 10.1111/tbed.12052
81. Rahman AU, Wensman JJ, Abubakar M, Shabbir MZ, Rossiter P. Peste des petits ruminants in wild ungulates. Trop Anim Health Prod. (2018) 50:1815–9. doi: 10.1007/s11250-018-1623-6
82. Mahapatra M, Sayalel K, Muniraju M, Eblate E, Fyumagwa R, Shilinde L, et al. Spillover of peste des petits ruminants virus from domestic to wild ruminants in the serengeti ecosystem, Tanzania. Emerg Infect Dis. (2015) 21:2230–4. doi: 10.3201/eid2112.150223
83. Fine AE, Pruvot M, Benfield CT, Caron A, Cattoli G, Chardonnet P, et al. Eradication of Peste des Petits Ruminants virus and the wildlife-livestock interface. Front Vet Sci. (2020) 7:50. doi: 10.3389/fvets.2020.00050
84. Ahmed B, Megersa L, Mulatu G, Siraj M, Boneya G. Seroprevalence and associated risk factors of foot and mouth disease in cattle in West Shewa Zone, Ethiopia. Vet Med Int. (2020) 2020:6821809. doi: 10.1155/2020/6821809
85. Bravo de Rueda C, de Jong MC, Eblé PL, Dekker A. Estimation of the transmission of foot-and-mouth disease virus from infected sheep to cattle. Vet Res. (2014) 45:58. doi: 10.1186/1297-9716-45-58
86. Fukai K, Nishi T, Morioka K, Yamada M, Yoshida K, Yamakawa M. Horizontal transmission of foot-and-mouth disease virus O/JPN/2010 among different animal species by direct contact. Transbound Emerg Dis. (2020) 67:223–33. doi: 10.1111/tbed.13344
87. Bird BH, Ksiazek TG, Nichol ST, Maclachlan NJ. Rift Valley fever virus. J Am Vet Med Assoc. (2009) 234:883–93. doi: 10.2460/javma.234.7.883
88. Clark MHA, Warimwe GM, Di Nardo A, Lyons NA, Gubbins S. Systematic literature review of Rift Valley fever virus seroprevalence in livestock, wildlife and humans in Africa from 1968 to 2016. PLoS Negl Trop Dis. (2018) 12:e0006627. doi: 10.1371/journal.pntd.0006627
89. Bron GM, Strimbu K, Cecilia H, Lerch A, Moore SM, Tran Q, et al. Over 100 years of Rift Valley fever: a patchwork of data on pathogen spread and spillover. Pathogens. (2021) 10:708. doi: 10.3390/pathogens10060708
90. Boukary AR, Saegerman C, Abatih E, Fretin D, Alambédji Bada R, De Deken R, et al. Seroprevalence and potential risk factors for Brucella spp. infection in traditional cattle, sheep and goats reared in urban, periurban and rural areas of Niger. PLoS ONE. (2013) 8:e83175. doi: 10.1371/journal.pone.0083175
91. Thrusfield M. Surveys. Veterinary Epidemiology. 3rd ed. Oxford: Wiley-Blackwell (2005). p. 228–46.
92. Covarrubias K, Nsiima L, Zezza A. Livestock and livelihoods in rural Tanzania. A descriptive analysis of the 2009 National Panel Survey. Joint paper of the Wrold Bank, FAO, AU-IBAR, ILRI and the Tanzania Ministry of Livestock and Fisheries Development. The World Bank, FAO, AU-IBAR, Tanzania Ministry of Livestock and Fisheries Development (2012).
93. Odhiambo M. Review of the Literature on Pastoral Economics and Marketing: Kenya, Tanzania, Uganda and the Sudan. Nairobi: RECONCILE (2006).
94. George J, Häsler B, Komba E, Sindato C, Rweyemamu M, Mlangwa J. Towards an integrated animal health surveillance system in Tanzania: making better use of existing and potential data sources for early warning surveillance. BMC Vet Res. (2021) 17:109. doi: 10.1186/s12917-021-02789-x
Keywords: brucellosis, foot and mouth disease, peste des petits ruminants, Rift Valley fever, sheep and goat pox, seroprevalence, risk factors
Citation: Lysholm S, Lindahl JF, Munyeme M, Misinzo G, Mathew C, Alvåsen K, Dautu G, Linde S, Mitternacht L, Olovsson E, Wilén E, Berg M and Wensman JJ (2022) Crossing the Line: Seroprevalence and Risk Factors for Transboundary Animal Diseases Along the Tanzania-Zambia Border. Front. Vet. Sci. 9:809128. doi: 10.3389/fvets.2022.809128
Received: 04 November 2021; Accepted: 07 February 2022;
Published: 11 March 2022.
Edited by:
Kimberly VanderWaal, University of Minnesota Twin Cities, United StatesReviewed by:
Georgina Limon, The Pirbright Institute, United KingdomMurat Sevik, Necmettin Erbakan University, Turkey
Gemma L. Chaters, University of Glasgow, United Kingdom
Copyright © 2022 Lysholm, Lindahl, Munyeme, Misinzo, Mathew, Alvåsen, Dautu, Linde, Mitternacht, Olovsson, Wilén, Berg and Wensman. This is an open-access article distributed under the terms of the Creative Commons Attribution License (CC BY). The use, distribution or reproduction in other forums is permitted, provided the original author(s) and the copyright owner(s) are credited and that the original publication in this journal is cited, in accordance with accepted academic practice. No use, distribution or reproduction is permitted which does not comply with these terms.
*Correspondence: Sara Lysholm, c2FyYS5seXNob2xtJiN4MDAwNDA7c2x1LnNl
†These authors have contributed equally to this work