- 1Institute of Sciences of Food Production (ISPA), National Research Council (CNR), Bari, Italy
- 2Department of Veterinary Medicine, University of Bari “Aldo Moro”, Bari, Italy
- 3Institute of Sciences of Food Production (ISPA), National Research Council (CNR), Lecce, Italy
The present study investigated the effects of different grape pomace storage techniques on the effectiveness as feed on in vitro ruminant digestion efficiency. Grape pomace from an autochthonous red grape variety (cv Nero di Troia) was used as fresh (GP) or ensiled, both without additives (SIL) and with the addition of a bacterial strain, Lactiplantibacillus plantarum 5BG (SIL+). All the different storage treatments were subject to chemical and microbiological evaluation, as well as in vitro digestibility, and gas production. Microbiological data revealed the good quality of grape pomace and silages due to the lactic acid bacteria populations and low presence, or absence, of undesirable microorganisms. The addition of L. plantarum 5BG influenced the chemical characteristics of the silage (SIL+). Ensiling technique deeply changed the polyphenolic composition, reducing anthocyanins, flavonols, and flavanols (condensed tannins precursors), particularly when L. plantarum 5BG was added. Antioxidant capacity was reduced by ensiling, in correlation with the polyphenolic content decrease. The oxygen radical absorbance capacity (ORAC) value of SIL+ was the lowest (P < 0.01) and its total phenol content was lower than SIL (P < 0.01). No statistical differences were observed between GP, SIL, and SIL+ on the antioxidant capacity by TEAC assay (P > 0.05). Ensiling did not affect the grape pomace nutrient profile, except for the reduction in NFC content. Apparent in vitro digestibility showed how ensiling increased dry matter (DM), organic matter (OM), neutral detergent fiber (NDF), crude protein (CP), ether extract (EE), and non-fiber carbohydrates (NFC) disappearance (P < 0.01), particularly with the L. plantarum 5BG inoculation. Moreover, SIL+ showed the lowest propionic acid (P < 0.05) and the highest methane (P < 0.01), butyric acid (P < 0.01), and nitrogen (P < 0.05) in vitro production. Ensiling GP resulted in a better in vitro digestibility, particularly if L. plantarum 5BG strain is added, probably due to the reduction of flavanols and their lower microbial activity inhibition.
Introduction
The use of agricultural by-products as animal dietary supplement is the result of multiple efforts in reducing the carbon footprint of the livestock industry (1, 2). By-products are generally inexpensive and their production can be considered free of greenhouse gas emissions as they are allocated to the primary product (3). This leads to an increased interest in exploiting plant products and by-products as feed additives to solve both animal nutrition problems and livestock production impact. In fact, plant by-products are often rich in readily fermentable carbohydrates and fats, useful for animal digestion processes, but also in plant secondary compounds that have been reported to suppress CH4 concentration, reduce rumen protozoa counts, and modulate rumen fermentation patterns (4).
Grape pomace (GP) is the by-product of a winemaking process and consists of pressed grapes (skin and seeds with residual pulp) and stalk residues. Considering that the winemaking process does not allow a complete extraction of polyphenolic compounds, GP represents an abundant and inexpensive polyphenols source. In particular, GP is rich of flavonoids, the largest group of polyphenols predominantly found in skins, seeds, and stems (5). Polyphenols have been intensively studied for their anti-inflammatory and anti-microbial properties and for their health-associated effects against chronic diseases, such as cancer, neurodegeneration, and cardiovascular pathologies (6). The health benefits of GP polyphenols, especially flavonoids, have received great interest of researchers, and the food and nutraceutical industries (7). Grape pomace also might be considered an important feed ingredient in ruminants' diet, being rich in bioactive polyphenols and soluble fiber, particularly when climatic conditions limit the availability of other feeds (8, 9).
In contrast to humans, in farm animals the potential health-promoting effects of bioactive polyphenols have been considered only recently (5, 9, 10). The use of GP is suggested as an alternative ingredient in animal feeding, effective in enhancing the oxidative stability of the meat (inhibiting the meat lipid peroxidation), in reducing the addition of synthetic antioxidant as vitamin E, and in modulating the intestinal microbiota (increasing the presence of specific beneficial bacteria strains) (5). Moreover, the addition of GP to dairy cows' feed was found to modulate milk's fatty acid composition and methane emissions (11–13). So, when shortage of feeds occurs, the addition of GP could represent an alternative feed resource in ruminants (14).
Although GP can be used fresh as a feeding ingredient, the seasonality in its production combined to not suitable storage techniques can lead to nutrient losses and spoilage processes (8). Ensiling is an appropriate method to preserve this product along the time, allowing farmers to use it continuatively in the year. Moreover, the use of suitable bacterial inoculants could improve the quality and aerobic stability of silages (15–17). Most of the inoculants used belong to lactic acid bacteria (LAB) (15–20). During the ensiling process, LAB converts water-soluble carbohydrates (WSC) into organic acids (e.g., lactic acid and acetic acid) which rapidly reduce the silage pH. The acidic and anaerobic conditions help to inhibit the proliferation of undesirable microorganisms (e.g., Clostridia) and preserve nutrient components (19). Furthermore, LAB is known to degrade phenolic compounds (21), but few studies have focused on the effects of LAB inoculants on changes of phenolic compounds in silages (19) and on silage digestibility. Considering the results of previous publications, it could be hypothesized that ensiling GP could improve its nutritive characteristics as animal feeding and that the addition of starter strains could be useful both to improve its digestibility as well as its impact on rumen gas production. Therefore, the aim of this research was to evaluate the effects of ensiling with and without addition of Lactiplantibacillus plantarum 5BG (previously named Lactobacillus plantarum) (22) on the chemical composition, in particular polyphenolic compounds, microbiological, and nutritional quality of GP silages.
Materials and Methods
The protocol for animal research was approved by the Ethics Committee for animal testing–CESA (process number 2-X/17) of the Department of Veterinary Medicine of the University of Bari “Aldo Moro”, Bari, Italy.
Preparation of Silage Grape Pomace
Fresh grape pomace samples (Vitis vinifera L., cv Nero di Troia) from red cultivars were collected in the Puglia Region (South Italy), immediately after the crush of grape juice and fermentation processes for red wine production had been completed. The whole sampled grape pomace was randomly subdivided in three experimental treatments: (A) fresh grape pomace (GP); (B) silage (SIL); and (C) silage inoculated with L. plantarum 5BG (SIL+).
For silage, grape pomace was randomly assigned to SIL or SIL+, and 8 silos for each experimental group were prepared. Fresh GP was subdivided in 8 parts of similar volume of each silo. Silos were a cylindric plastic container (35 L vol; about 30 cm diameter ×50 cm high). Each silo was filled with manually pressed pomace. In the SIL+ group, inoculation of GP was performed with the strain L. plantarum 5BG (23) belonging to the Culture Collection of the Institute of Sciences of Food Production, National Research Council (ITEM 17403, http://server.ispa.cnr.it/ITEM/Collection/). A freeze-dried powder of L. plantarum 5BG was inoculated in GP at a final concentration of ~6 log cfu/g of GP. All the silos had a gas release valve in the lid and were stored upright at ambient temperature (18–25°C). The silage density was calculated as the ratio between the ensiled GP and silo volume (kg GP m3) and it was on average value of 658.54 ± 16.20 kg GP m3. The silos were opened after 1 month from the starting of the experiment (T0).
Fresh (GP) and ensiled grape pomace (SIL and SIL+) were subjected to the evaluation of pH, chemical and microbiological characteristics, in vitro digestibility, and gas production.
All samples (GP, SIL, SIL+) were stored lyophilized (Labconco Corp., Kansas City, MO) at −20°C until polyphenolic analysis. Afterward samples (GP, SIL, and SIL+) were ground using a CT 193 Cyclotec mill fitted with 1-mm screen (FOSS, Hilleroed, Denmark), and analyzed for phenols and antioxidant capacity at least in triplicate.
Chemical Analyses
Before and after in vitro digestion, all GP, SIL, and SIL+ samples were analyzed in triplicate, considering each silo as the experimental unit. Dry matter (DM) was determined using standard procedures (24) (method 930.15). Ash was determined by standard procedures (24) (method 942.05) using a muffle furnace at 550°C for 16 h. Fat was determined using the Soxhlet extraction procedure (24) (Method 991.36), crude protein (CP) was determined by Kjeldahl N ×6.25 procedures (24) (Method 968.06). Neutral detergent fiber (NDF) and acid detergent fiber (ADF) were determined with the ANKOM fiber analyzer according to Van Soest et al. (25) and was corrected for residual acid-insoluble ash. Sodium sulfite was added to the solution for NDF determination. Non-fiber carbohydrates (NFC) were calculated by subtracting CP, ether extract (EE), and NDF from the organic matter (OM) and the metabolizable energy (ME) values were calculated using the equation:
as suggested by Robinson et al. (26), where CP, EE, and OM residual are as g/kg of DM. The water soluble carbohydrate (WSC) content was determined as described by McDonald et al. (27).
Microbiological Analyses
Microbiological analyses were carried out on SIL and SIL+ at different times (0, 24, 48, 144 h) after silos opening, as well as on GP. Twenty grams of samples were homogenized with 180 mL of sterile buffered peptone water (BPW, Biolife) in a Stomacher (Seward, London, United Kingdom) for 2 min. The resulting suspensions were serially diluted in the same diluent and plated in duplicate on the following agar media for the detection and enumeration of microorganisms: Plate Count Agar (PCA, Difco, Franklin Lakes, NJ) supplemented with 100 mg/L of cycloheximide (EMD Millipore Corp., Billerica, MA) incubated at 30°C for 24 h to determine the total aerobic mesophilic bacterial counts (AMB); de Man Rogosa Sharpe (MRS) agar (Oxoid Ltd, Basingstoke, UK) supplemented with 100 mg/l of cycloheximide incubated at 30°C for 48 h for the determination of LAB; Sabouraud Dextrose Agar (SDA, Oxoid) supplemented with 200 mg/L chloramphenicol (Sigma, Milan, Italy) for the enumeration of yeasts and molds, incubated at 25°C for 72 h; violet red bile glucose agar (VRBGA, Difco) for total Enterobacteriaceae, incubated aerobically at 37°C for 24 h; sulfite polymyxin sulfadiazine (SPS) agar (Biolife, Milan, Italy) for detecting Clostridium perfringens, incubated anaerobically at 37°C for 24 h; the chromogenic and selective Listeria agar (ALOA, Biolife) for the enumeration of Listeria monocytogenes after 48 h of incubation at 37°C. Moreover, an aliquot of each microbial suspension was heat-treated for 20 min at 90°C, plated on a plate count agar (PCA, Difco) and incubated for 24 h at 30°C for spore-forming bacteria (SFB) counts. LAB, AMB, yeasts, molds, and Enterobacteriaceae counts were used as indicators of the overall microbiological quality of samples, while SFB, C. perfringens, and Ls. monocytogenes were also considered as potential pathogens and indicators of the product microbiological safety (28). Each sample was tested in triplicate.
Preparation of Polyphenolic Extracts From Grape Pomace
Polyphenols were extracted in triplicate from 500 mg (DW, dry weight) GP, SIL, and SIL+ after silos opening (T0), macerated with 50 mL extraction solvent (70% acetone + 0.01% TFA), at 4°C, over-night. After centrifugation of the slurry (10 min at 2,000 × g) the supernatant was collected, further 10 mL of extraction solvent were added to the pellet, and the extraction was repeated on a rotary shaker for 1 h. Pooled supernatants were evaporated in vacuo at 32°C using a model R-205 Büchi rotavapor (Büchi Labortechnik AG, Switzerland) and re-suspended in acidified water (0.01% trifluoracetic acid) (TFA) at known volume. Extracts were filtered on 0.45 μm CA syringe filter (Filtres Fioroni, France), portioned and stored at −20°C until analysis. The extraction experiments were performed twice, with each triplicated extraction considered for HPLC injection.
Identification and Quantification of Polyphenolic Compounds
The HPLC separation, identification, and quantification of polyphenols in GP, SIL, and SIL+ extracts were performed using the same chromatographic method and column as already reported (29). The polyphenolic compounds were identified by comparing their peak retention times and UV-visible spectra with those of commercial standards, where available. Spiking experiments using sample solutions and standards as well as comparisons to any relevant published grape pomace analytical characterization were used to verify peak identities (30).
The identified phenolic compounds were quantified by the external standard method using a six-points calibration curve of oenin (1.25–250 mg/L), gallic acid (1–100 mg/L), syringic acid (0.5–100 mg/L), catechin (1–100 mg/L), and rutin (0.5–100 mg/L). When reference standard compounds were not available, the quantification was done using the calibration curve of the most structurally related substance, including a molecular correction factor (31).
Phenols and Hydrophilic Antioxidant Capacity
The polyphenolic extracts were assessed for total phenol content and reducing capacity by the Folin-Ciocalteu (F-C) assay, as well as their antioxidant capacity using the ABTS assay (Trolox-equivalent antioxidant capacity - TEAC) and the oxygen radical absorbance capacity (ORAC) assay (29). A rapid microplate methodology, using a microplate reader (Infinite M-200, Tecan Group Ltd, Männedorf, Switzerland) and 96-well plates (Costar, 96-well clear round bottom plate, Corning) was used.
Two independent plates (at least) were performed for each sample, which was tested in triplicate for each dilution (four dilutions of each extract).
In vitro Rumen Digestion
All the operations of rumen fluid sampling were performed at the slaughterhouse, suddenly after animal slaughtering. Rumen fluid was obtained by a total of 24 animals (Limousine steers, aged 12 months, reared in the same farm). All animals were fed ad libitum with the same feed ratio (86.7% of dry matter, 15.9% of crude protein, 9.2% of crude fiber, 25.6% of NDF, 10.1% of ADF, and 2.6% of ADL). Samples of ruminal contents (filtered through eight layers of gauze cloth) were collected in thermos flasks (previously filled with distilled water at 39°C to avoid thermal shock to the rumen fluid), insufflating CO2 into the headspace to ensure the environment remained anaerobic and taken within 30 min to the laboratory. After transport, the top layer of ruminal contents was discarded, and the remaining portion was mixed and blended under a CO2 headspace for 1 min to remove any additional particles and/or attached organisms. The combined fluid and contents were strained through 6 layers of cheesecloth to form the inoculum for the in vitro fermentation (32).
In vitro fermentation was conducted for 48 h using the Daisy II incubator system (ANKOM Tech., Fairport, NY), as described by Maggiolino et al. (32). The unit consisted of four incubation vessels with a capacity of 2 L for each. Each vessel contained 1.6 L of buffer solution, 400 mL of rumen liquor, and 25 nylon filter bags (ANKOM F57, ANKOM Tech., Fairport, NY). The buffer solution consisted of 1.33 L buffer A (KH2PO4, 10.0 g/L; MgSO4 H2O, 0.5 g/L; NaCl, 0.5 g/L; CaCl2 H2O, 0.1 g/L; and urea, 0.5 g/L) and 266 mL of buffer B (Na2CO3, 15.0 g/L and Na2S7H2O, 1.0 g/L), mixed in each digestion vessel and the pH was adjusted to 6.8, as reported by the method of Marten and Barnes (33). Each digestion trial for each sample was performed in duplicate. Bags were rinsed in acetone and allowed to air dry before drying at 100°C for 24 h, after recording dry bag weight. They were used and filled with a total of 500 mg each GP, SIL, and SIL+ (all samples were stored at −20°C before analysis). All samples had been previously ground until the particle size reached 2 mm screen using a hammer mill (Pullerisette 19, Fritsch GmbH, Laborgeratebau, Germany). Twenty-five bags were put in each incubation vessel. After digestion, all bags were weighed again. All analysis for nutrient parameters was performed after digestion and percentage of disappearance for each parameter was calculated as: (PB-PA)/PB, where PB is the quantity (g/kg) of parameter in the samples before the digestion and PA is the quantity (g/kg) of the parameter after digestion. Results were expressed as percentage.
In vitro Gas Production and Analysis
For gas and volatile fatty acid analysis, an automated pressure transducer system (Ankom Technology, Macedon, NY) was used as described by Maggiolino et al. (32). It was equipped with 8 different 250 mL bottles. The same buffer solutions for the in vitro digestion section were used. Each vessel received 133.3 mL of Buffer A and 26.7 mL of Buffer B. Then, 40 mL of rumen fluid was added. The GP, SIL, and SIL+ (500 mg) were pre-weighed into each vessel. The head space of each vessel was insufflated with CO2 for 2 min to ensure anaerobic conditions.
Vessels were put in an oscillating water bath (39°C with an oscillating frequency of 45/min), to reproduce movements similar to those found in the rumen, and digestion was simulated for a 48-h fermentation period. After this period, vessels were removed from the water bath and placed into an ice bath while gas samples were drawn into evacuated test tubes, as described by Trotta et al. (34). Gas samples were analyzed for methane production with gas chromatography (Agilent Technologies, Santa Clara, CA) by using the total gas volume at standard temperature and pressure (35). Flasks were opened, pH measured, and 1 mL aliquot of the fermentation medium was combined in a 1.5 mL centrifuge tube with 0.1 mL of 500 g/L metaphosphoric acid and 0.1 mL of 85 mM of 2-ethyl butyrate. Samples were centrifuged at 39,000 × g, at 23°C for 15 min. Afterward, they were processed for volatile fatty acid (VFA) concentrations (35) using a gas chromatograph with FID (Agilent Technologies, Inc., Santa Clara, CA) equipped with a 2 m ×3 mm packed column (45.60 Carboxen 1000, Supelco, Inc., Bellefonte, PA). For determination of ammonia nitrogen, 2 mL fluid and 2 mL trichloro-acetic acid solution (10%, w/v) were mixed to deproteinize the samples and then centrifuged for 5 min at 1,500 × g. The supernatant (2 mL) was processed in order to measure the ammonia nitrogen concentration according to a spectrophotometric method (36).
Statistical Analysis
One-way analysis of variance (ANOVA) was performed to differentiate among the treatments (GP, SIL, and SIL+), the phenolic content, the in vitro digestion data, and gas emission results. The ANOVA was performed using the general linear model (GLM) by SAS software (37), according to the following model:
where yij represents all the previous cited patterns as dependent variables; μ is the overall mean; αi is the silo random effect; G was the effect of the jth group (GP, SIL, and SIL+) (j = 1, …3) and εijk was the error term.
The microbial data after ensiling were subject to a multifactorial ANOVA according to the following model:
where yij represents all the microbial patterns as dependent variables; μ is the overall mean; αi is the silo random effect; G was the effect of the jth group (SIL and SIL+) (j = 1, 2); T represents the effect of the kth time after desilation (k = 1, …4); G × T represents the effect of the binary interaction of the jth group and kth time (1,…,8) and εijk was the error term. When not significant, the binary interaction was dropped from the model. A Tukey test was applied to evaluate the differences according to time.
The significance was set at P < 0.05, and the results were expressed as means and standard error of the means.
Results
Chemical Composition and Microbiological Analyses
The chemical composition of GP, SIL, and SIL+ is shown in Table 1. There are no differences after ensiling with or without L. plantarum 5BG in DM, CP, EE, NDF, ADF, and ash (P > 0.05), although ME showed the highest values in SIL+ (P < 0.01) and lower in SIL compared to GP (P < 0.05). NFC content was lower in SIL+ than GP and SIL (P < 0.01). Moreover, ensiling (both SIL and SIL+) showed lower pH compared to the GP (P < 0.001) and WSC disappeared (P < 0.001).
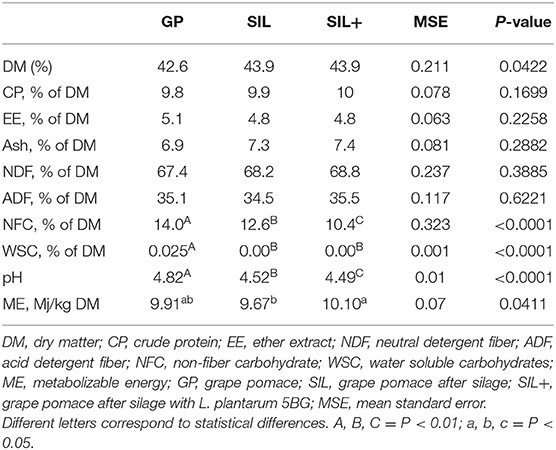
Table 1. Impact of ensiling with or without L. plantarum 5BG on chemical composition and pH of grape pomace.
The results of GP microbiological analyses, before ensiling, are shown in Figure 1. Total AMB and LAB loads were 6.22 ± 0.07 log cfu/g and 5.96 ± 0.13 log cfu/g, respectively. Yeast load was 7.39 ± 0.08 log cfu/g, while the cell densities of SFB, Enterobacteriaceae, and C. perfringens were 2.94, 2.26, and 0.8 log cfu/g, respectively.
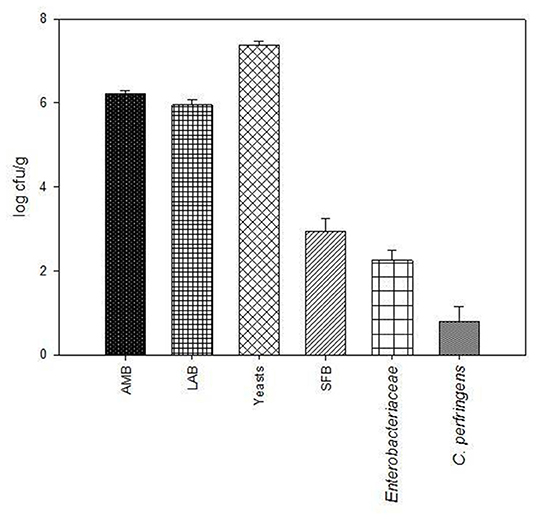
Figure 1. Microbiological characteristics of grape pomace (GP). The microbial loads (log cfu/g) of total aerobic mesophilic bacteria (AMB), lactic acid bacteria (LAB), yeasts, spore-forming bacteria (SFB), Enterobacteriaceae, and C. perfringens are reported as means and standard deviation (error bars).
In SIL (Figure 2) the AMB counts did not vary significantly over time, while in SIL+ the values were significantly lower at the opening of the silage (0 h) (5 ± 0.04 log cfu/g) than at 144 h (P < 0.01). However, after 6 d (144 h) both silages presented equal values (6.3 log cfu/g). SIL+ showed LAB population ranging from 5.60 ± 0.15 log cfu/g (0 h) to 6.17 ± 0.15 log cfu/g (144 h) (P > 0.05), and both silages did not show significant differences at the same times.
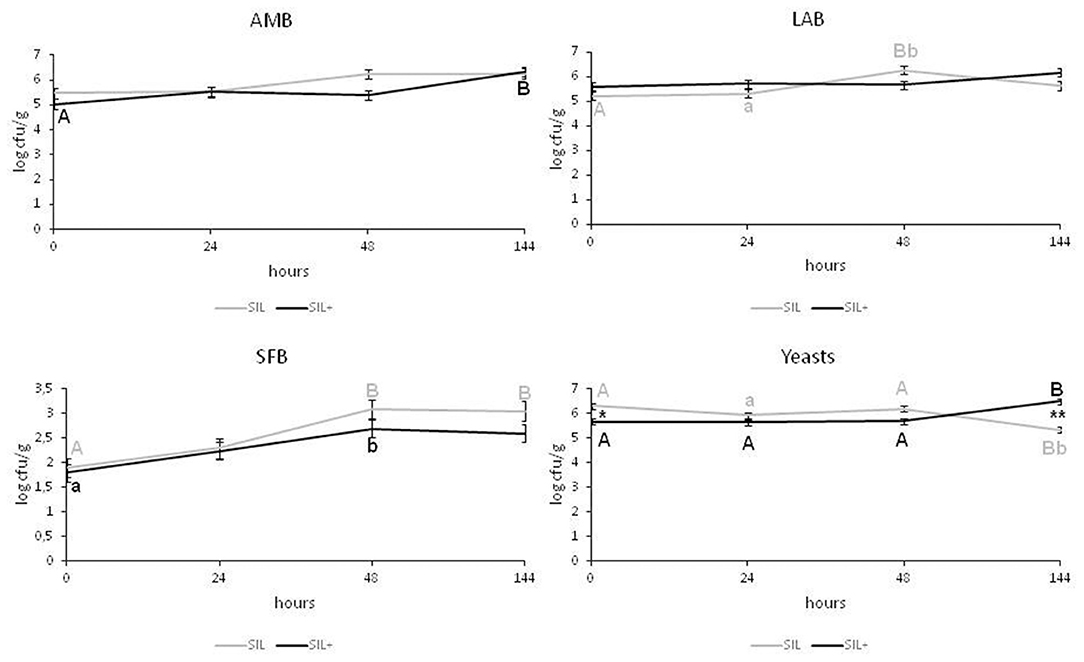
Figure 2. Microbiological characteristics of the silage with (SIL+) or without (SIL) L. plantarum 5BG inoculation. The microbial loads (log cfu/g) of total aerobic mesophilic bacteria (AMB), lactic acid bacteria (LAB), and spore-forming bacteria (SFB) and yeasts are reported. Values of each time point are the means of three replicates ± standard error (error bars). Different letters of the same color show statistical differences among time (A, B = P < 0.01; a, b = P < 0.05); *, ** show statistical differences between groups at the same time (*P < 0.05; **P < 0.01).
At the opening of the silages and in all experimental times, SFB showed no differences between SIL and SIL+ (P > 0.05); however, they increased in SIL (P <0.01) and SIL+ (P < 0.05) after 48 h and then remained constant in both experimental trials.
After opening, the SIL yeast population was higher than SIL+ (5.66 ± 0.1 log cfu/g) (P < 0.05), but after 6 d yeasts increased in SIL+ reaching values (6.5 ± 0.19 log cfu/g) higher than SIL (P < 0.01).
Moreover, enterobacteria and C. perfringens were present in GP, but both were absent in silage; while molds and Ls. monocytogenes were absent in both GP and silages.
Identification and Quantification of Phenolic Compounds and Antioxidant Activity
The chromatographic profiles of GP extract at λ = 520, 280, and 350 nm are shown in Figure 3 and the identified compounds are listed in Table 2. The analytical profile of SIL and SIL+ extracts was comparable to that one of GP, only quantitative differences occurred.
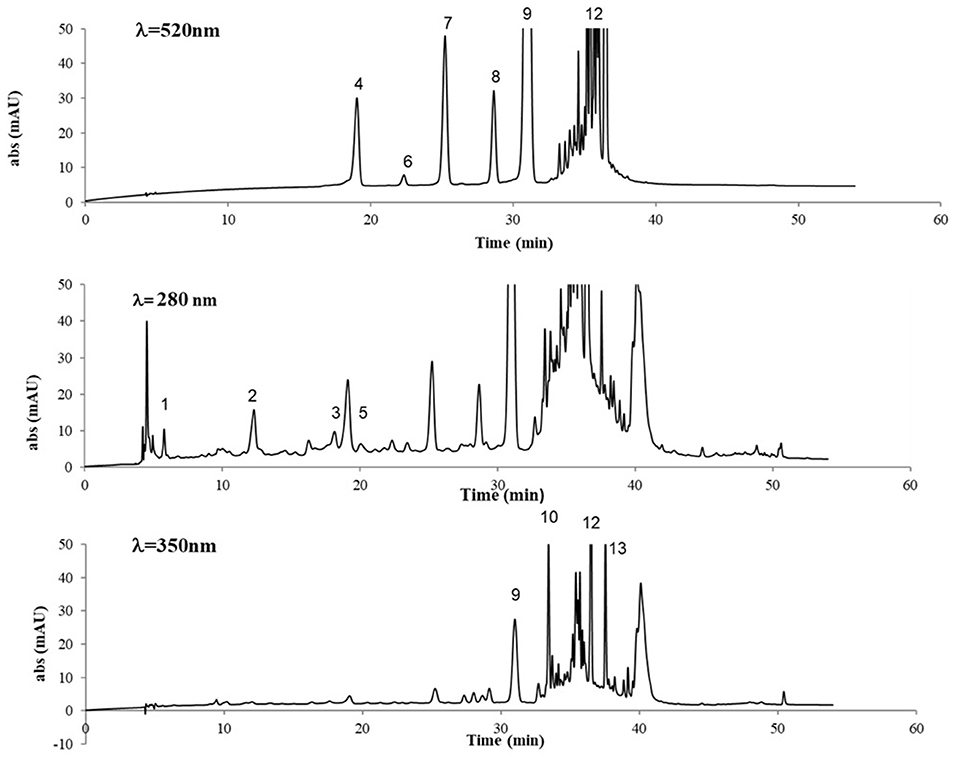
Figure 3. HPLC separation of phenolic compounds in GP extract (V. vinifera L., cv Nero di Troia), at λ = 520, 280, and 350 nm. For peak assignment, see Table 2.
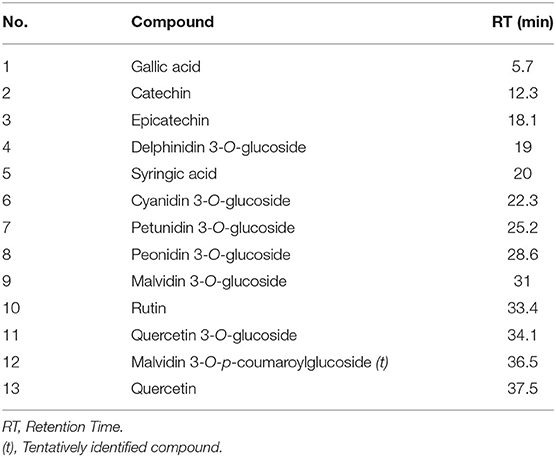
Table 2. Peaks assignment of phenolic compounds extracted from grape pomace (V. vinifera L., cv Nero di Troia).
The targeted HPLC analysis of GP extracts at λ = 520 nm revealed the presence of anthocyanins, with the typical five peaks of the monoglycosilated anthocyanins present in grape, according to the order delphinidin 3-O-glucoside, cyanidin 3-O-glucoside, petunidin 3-O-glucoside, peonidin 3-O-glucoside, and the predominant malvidin 3-O-glucoside, which were identified by comparison with reference compounds. Additionally, some peaks were detected with retention time higher than that of malvidin 3-O-glucoside, representing acylated anthocyanins, which were not assigned to specific structures, exclusively based on the UV-Vis spectra, except for the peak at RT= 36.5 being tentatively identified as malvidin 3-O-p-coumaroylglucoside (Figure 3).
Phenolic profile content of GP, SIL, and SIL+ is reported in Table 3. GP showed a total anthocyanins content higher than SIL (P < 0.05) and SIL+ (P < 0.01). SIL showed monoglycosilated anthocyanins, rutin, and epicatechin content lower than GP (P < 0.01) and the highest content of syringic acid (P < 0.01). SIL+ showed the lowest content of gallic acid, monoglycosilated anthocyanins, rutin, catechin, and epicatechin (P < 0.01) and the highest content of acylated anthocyanins (P < 0.01) and quercetin (P < 0.05). Moreover, SIL+ showed a catechin and epicatechin contents lower than SIL (P < 0.05) and GP (P < 0.01).
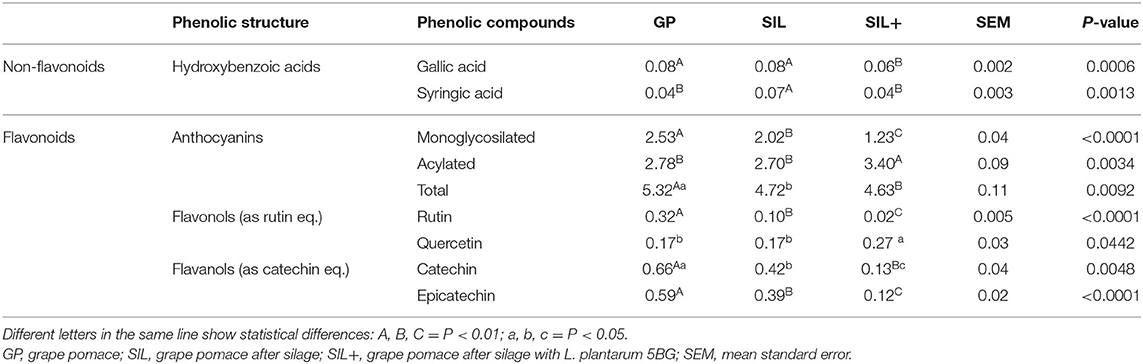
Table 3. Phenolic profile content (mg/g dry weight) in grape pomace (GP), after silage with (SIL+) or without (SIL) L. plantarum 5BG inoculation, quantified by HPLC analysis (n = 3).
Table 4 reports the total phenol content (TPC) and the antioxidant activity of GP, SIL, and SIL+. The TPC in SIL+ was lower than SIL (P < 0.01). The lowest ORAC activity was measured in SIL+ (P < 0.01), although no statistical differences were observed between GP, SIL, and SIL+ about TEAC activity (P > 0.05).
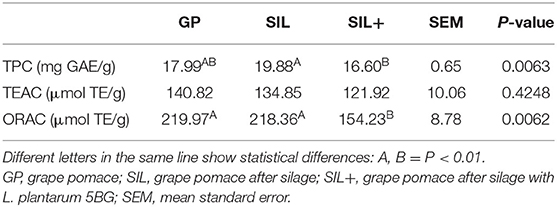
Table 4. Total phenol content (TPC) and antioxidant activity (by TEAC and ORAC assays) in grape pomace (GP), and after silage with (SIL+) or without (SIL) L. plantarum 5BG (per g dry weight).
In vitro Digestion and Gas Production
Table 5 reports the results of apparent percentage of disappearance after in vitro digestion. SIL+ showed the highest DM, OM, NDF, CP, EE, and NFC disappearance (P < 0.01). Moreover, SIL showed higher DM, OM, NDF, CP, EE, and NFC percentage of disappearance compared to GP (P < 0.01).
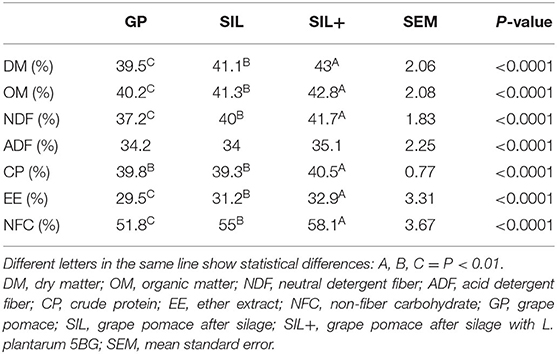
Table 5. Percentage of disappearance after in vitro digestion in grape pomace (GP), after silage with (SIL+) or without (SIL) L. plantarum 5BG.
In vitro gas production results are reported in Table 6. SIL+ showed the lowest propionic (P < 0.05) (P < 0.01) in vitro production and the highest butyric, methane (P < 0.01) and nitrogen (P < 0.05) in vitro production, although the total gas production and the total VFA production did not show differences among treatments (P > 0.05).
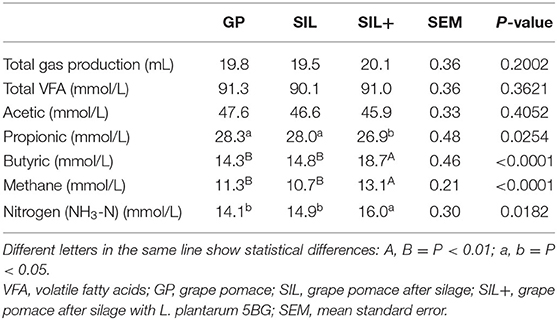
Table 6. In vitro gas production in grape pomace (GP), after silage with (SIL+) or without (SIL) L. plantarum 5BG.
Discussion
Chemical Composition Characterization and Ensiling Effects
Feed digestibility is an important factor in the nutritional efficiency assessment of a ration. Among the three experimental treatments (GP, SIL, and SIL+), DM, OM, CP, EE, ash, NDF, ADF, and NFC values are often not consistent with those reported in the literature. For example, Spanghero et al. (38) observed slightly higher content of DM in Italian red and white grape pomace, but lower in the Californian red one. On the other hand, in both grape pomaces investigated, they observed similar values of OM and CP, higher values of EE and ADF and lower values of NDF. Massaro Junior et al. (39), studying South African red grape pomace, observed lower DM content, similar OM and NDF content, and higher EE, CP, and ADF content. Nevertheless, the high variability in the GP chemical composition depends on different factors such as the type of grape, grape maturity, wine production methods, components proportions (seeds and pulp), harvest methods (8, 38, 40–42). For these reasons it is difficult to compare values and results among different studies. Some authors stated that grape pomace DM should be >250 g/kg to obtain a good quality silage; some others defined an interval between 280 and 400 g/kg of DM to avoid high nutrient losses (39). The GP used in our experiment for ensiling showed a suitable DM content and the nutrient losses were almost null. Similarly, some authors observed no change in DM, CP, and ash after anaerobic storage (14). It can be supposed that this lack of differences can be due to the short storage time (43), and that ensiling both with and without L. plantarum 5BG addition had no great effect on grape pomace nutritional composition. Results showed that pH decreased with ensiling, and it decreased even more when L. plantarum 5BG was added. There are different approaches to the suitability of pH values for silage: some authors defined pH value 4.2 as the upper threshold for a positive assessment of a silage, while other researchers stated that the final pH is not important; what matters is the decreasing rate, as this parameter is more important in inhibiting a secondary fermentation occurrence (39, 44). The decrease of pH values during the ensiling process can be related to fermentation activities involving NFC consumption (39) but also WSC (14).
The results of the present work are consistent with this knowledge. LAB fermentation activities lead to the organic acid production with consequent pH reduction (19). Probably, the strain addition improved the fermentation processes inducing a lower concentration of NFC and WSC, and lower pH values. This result could be due to an efficient homofermentative lactic acid production promoted by the LAB strain (19).
The ensiling process reduced the grape pomace ME, but the L. plantarum 5BG addition seemed to enhance it. Similarly, Alipour and Rouzbehan (14) reported that ensiling reduced the grape pomace ME, probably due to a reduction of some nutrient availability and the incapacity of this storage process to reduce all the tannins and their negative effects on digestibility. Probably, the L. plantarum 5BG was able to increase grape pomace ME throughout a greater reduction of phenolic substances and consequently higher digestibility.
Microbiological Quality
Plant-associated microbiota is composed of bacteria, including LAB, Bacillus sp., Clostridium sp., and coliform bacteria, as well as yeasts and molds. In general, a LAB number is considered a fundamental factor in predicting the suitability for the silage fermentation and in evaluating the need to apply the bacterial inoculants at ensiling. In fact, when the LAB number exceeds 5 log cfu/g, the silage can be well preserved. On the contrary, the low LAB number and the high number of harmful microorganisms could indicate the need to control the fermentation of the silage by LAB inoculation (18). The proliferation of undesirable microorganisms can cause direct DM and nutrients losses, but also indirect issues, due to reduced palatability and the risk of negative effects on the animal performance and health (16). In this current work, the epiphytic microbiota in GP was evaluated, and decided to proceed with the inoculation with L. plantarum 5BG, although high LAB counts were observed (ca. 5.9 log cfu/g). During ensiling, the role of LAB is to decrease pH by converting carbohydrates into organic acids which help to preserve the silage. Moreover, LAB inhibits the growth of undesirable microorganisms by competing for nutrients and synthesizing antimicrobial and antifungal agents (45). Different LAB species synthesize some metabolites, such as bacteriocins, hydrogen peroxide, and organic acids that inhibit the growth of pathogenic and spoilage bacteria, yeast, and molds (46). L. plantarum 5BG, used in our study as a grape pomace inoculant, was able to produce antimicrobial compounds and organic acids responsible for antifungal properties (47).
The results of the current work showed that the LAB number present in both silages (SIL and SIL+) can guarantee the quality of the silage. In fact, Enterobacteriaceae and C. perfringens were absent in the silages and SFB were in low number. After ensiling, both LAB and yeast populations were still high in number probably due to the carbon source quantities in GP that were suitable in supporting microbial growth. As observed in this study (Table 1), after ensiling, NCF decreased significantly probably due to their use by microorganisms. Furthermore, after ensiling, the tannin precursors decreased; therefore, a reduction of their inhibitory effect on the bacterial population could be hypothesized (21).
Yeast counts in GP were higher than the reported values in other silage crops (18, 48) and similar to those found in grape marc at the beginning of storage (49), while, after opening, yeasts were lower in SIL+ than in untreated and acidified marc studied by Maragkoudakis et al. (49). Probably, the yeast number decreased as results of pH reduction and antifungal activity of L. plantarum 5BG (47). During air exposure, yeast population remained substantially stable, however, as it is known, yeasts can cause aerobic deterioration and reduce the nutritional value of the silage (18). Therefore, further research is needed to improve yeast control.
HPLC Analysis: Anthocyanins and Other Phenolic Compounds
While the silages, with or without the L. plantarum 5BG addition, did not show effects on the anthocyanins chromatographic profile (Figure 3), it revealed evidence of change in the anthocyanins concentration. In fact, ensiling decreased total anthocyanins content by 12%, whereas monoglycosylated anthocyanins content decreased much more (by 20%). Ensiling with L. plantarum 5BG addition led to a more significant reduction of monoglycosilated anthocyanins content than GP (reduction of 52%); comparing the two silage techniques, a 40% reduction occurred with LAB strain inoculation than without any supplement. The acylated anthocyanins content increased only in SIL+, reaching values of almost 20 and 25% higher than GP and SIL, respectively.
All these results point to the conclusion that the silage processes (with or without LAB strain inoculation) lead to an overall decreasing trend in the total anthocyanins content. On the other hand, acylated anthocyanins increased, exclusively with the addition of L. plantarum 5BG. Thus, it can be hypothesized that, during silage with inoculated LAB strain, monoglycosilated anthocyanins undergo acylation by acyltransferases of bacterial origin (50).
The total amount of anthocyanins found in GP (cv Nero di Troia) was within the range reported in other research (51–53), or to grape skin of the same cultivar Nero di Troia, limited to monoglycosilated anthocyanins (54).
The represented phenolic acids in the samples were the hydroxybenzoic acids, i.e., gallic and syringic acids. Gallic acid content did not change during silage (SIL) but decreased in SIL+. Syringic acid content was higher in SIL. As grape pomace is a highly oxidized product, syringic acid is supposed to be formed through oxidative degradation of malvidin 3-O-glucoside (55). The value we found in GP was consistent with previous reports for different grape cultivar pomace (56). With silage, a degradative oxidation of (monoglycosilated) anthocyanins occurred (with a 20% decrease), producing syringic acid from the degradation of malvidin 3-O-glucoside, possibly also from microbiological intervention. When GP was silaged with the addition of L. plantarum 5BG, monoglycosilated anthocyanins were further degraded (more than 50%) compared to GP sample, as oxidation of phenolic compounds can be supported by laccase from LAB origin (57). Conversely, syringic acid content did not change; the intervention of the strain 5BG could lead to a different metabolic fate of syringic acid.
Among flavonols, in GP rutin was predominant, followed by quercetin. In SIL samples, rutin content dropped (72% decrease), as well as in SIL+ samples (94% decrease). Moreover, the reduction in SIL+ was remarkable (78% reduction), even when compared to SIL. On the contrary, quercetin tended to increase in SIL+, being concomitant with the rutin decrease. This result was expected as quercetin is the aglycone which is released after rutin hydrolysis.
Catechin and epicatechin amounts occurred in GP samples after anthocyanins. The total flavanol content (catechin and epicatechin) was in the same range as reported in grape by-products from different red grape varieties (52, 58). The catechin was predominant over epicatechin, as usually reported, except in a few grape cultivars (59, 60).
Flavanols (catechin and epicatechin) content in SIL and SIL+ decreased by ~36% and ~80% of the value in GP, respectively. The reduction of flavanol content could be a positive aspect, as catechin and epicatechin are the precursor compounds of condensed tannins, which, as described in the published literature, tend to influence the digestibility of the feeding ration (61, 62). When GP has been used for feeding purposes (13), the reported content of epicatechin was 0.11 mg g−1, comparable to the content found in our experiments after ensiling with L. plantarum 5BG addition.
It has been reported already that the anaerobic storage of a plant matrix leads to a decrease of polyphenolic content (43). In ensiled grape pomace in particular, Alipour and Rouzbehan (14) found a reduction of total phenols and tannins. Moreover, it has been shown that storage increased the polymerization of existing tannins into higher oligomers (63). Thus, it can be hypothesized that polymerization occurs at the expenses of tannins precursors (flavanols). L. plantarum could be more efficient to promote polymerization than the existing microorganisms in the silage.
Hydrophilic Antioxidants: Phenols and Antioxidant Capacity
The phenols content in GP (cv Nero di Troia) was in the same range as in past publications, both in different Italian red grape cultivars (53), and international cultivars (64), also used for feeding cows (13, 53).
With ensiling, the phenol content was statistically lower in SIL+. The same trend was shown for the antioxidant capacity: silage, with or without L. plantarum 5BG addition, reduced the total phenol content and, consequently, the antioxidant capacity of the samples.
The antioxidant capacity in GP, assessed by TEAC assay, was similar to that reported by González-Paramás et al. (58), but lower than Rockenbach et al. (65). The ORAC value in our experiments revealed the same trend reported by Yilmaz and Toledo (64) in international grape varieties, even if they used β-phycoerithrin as fluorescent marker instead of fluorescein.
Apparent Percentage of Disappearance After in vitro Digestion and Gas Production
Rumen digestibility represents an important factor to define feeds nutrients productive efficiency and animals' environmental impact. Therefore, the evaluation of the inclusion of innovative food on rumen digestibility plays a key role for in field application (66). Ensiling did not affect most of the chemical constituents, both with and without L. plantarum 5BG inclusion, but a more significant effect on in vitro digestibility was found. Ensiling increased the DM, OM, NDF, CP, EE, and NFC percentage of disappearance, and these results were even improved by L. plantarum 5BG inoculation. Past publications reported that ensiling GP released secondary compounds as tannins (38) and that they may negatively affect digestibility, particularly because they can bind crude proteins, minerals, and carbohydrates (41, 67). The current study showed that ensiling reduced the GP flavanols (tannins precursors) content and that the L. plantarum 5BG addition reduced even more their concentration. Similarly, other researchers reported that anaerobic storage like ensiling can be used to reduce tannins content of grape pomace (43, 68). It is known that L. plantarum is a LAB species able to degrade phenolic compounds, but the metabolic pathways of their degradation in LAB have not been completely described (21). Furthermore, many factors can influence the results of a LAB addition, such as the characteristics of raw materials, epiphytic microbiota, and the silage management. A recent work (20) investigated the biotransformation of phenolic compounds in sainfoin silage inoculated with L. plantarum and observed the reduction of flavanols (catechin and epicatechin). The flavanols content reduction can explain digestibility differences found in this study among SIL, SIL+, and GP (61, 62). The antioxidant activity of these secondary compounds (tannins, anthocyanins, etc.) can be exerted also on the rumen microbial activity reducing and/or inhibiting some of them and, consequently, reducing the digestive capability. It is well known that Lactobacillus addition, apart from accelerating lactic acid production and inhibiting detrimental microorganisms' growth, may also further control microorganism population conferring antimicrobial properties to silage and outcompeting them for free sugars (69, 70). On the other hand, it is inferred that Lactobacillus addition improves digestibility likely throughout the reduction of all phenolic compounds that may inhibit microbial activity (71, 72). Total gas production values for GP were similar to those reported by other authors (38), and they increased in SIL+. Differently from what was reported by some authors (14), the reduction in flavanols content in SIL+ could be the main cause of the increase in gas production during digestion. The total volatile fatty acids production did not change, but there was an increase in butyric acid and a slightly decrease in propionic acid in SIL+ compared to both GP and SIL.
The substrate for the production of butyric acid is dietary fiber, which undergoes different metabolic pathways as a result of bacterial fermentation, starting from glucose and, consequently, carbohydrates availability (73). Ensiling reduced the NFC content, likely because the latter represent the first suitable substrate for the growth of the microbiota, as also confirmed by the L. plantarum 5BG addition. Moreover, NFC digestibility increased with ensiling probably due to the lower total phenol content and flavanols (condensed tannins precursors), resulting in a higher and faster butyric acid production and thus a lower and slower propionic acid production.
Ammonia nitrogen represents one of the major sources for microbial growth and microbial protein synthesis in the rumen (32, 74) and its production rate is strictly linked to the dietary rumen protein degradation (75). The increased ammonia nitrogen values observed in L. plantarum-added silage probably could be due to the increased protein digestibility, considering that high antioxidant component inclusion in feed tends to reduce the ammonia nitrogen concentration (75). This result shows how ensiling processes can give more nitrogen availability and digestibility with a consequent higher nutrient availability for microbial populations. In fact, some authors reported that ammonia concentration, a key metabolite for nitrogen production, decreased due to a lower protein degradation in the feed and a lower microbial protein synthesis (32, 75).
The CH4 production tended to be higher in the SIL+ compared to the other treatments. It seems that ensiling with L. plantarum 5BG increased its production. Methane production depends not only on the available fermentable substrate amount but also to the proportion of VFA produced during the fermentation (76). Also condensed tannins content has been reported to correlate negatively with CH4 production (77). Tannins have both the ability to reduce protein and carbohydrate availability for rumen microorganism fermentation, complexing them (61), and the capacity to inhibit bacteria, protozoas, and archeans, activity (62). Furthermore, tannins may inhibit the growth or activity of rumen methanogen and protozoa through bactericidal or bacteriostatic activities (78), most likely through binding proteins and enzymes of the microbial cells (79). Although the lower content of NFC, the lower phenols content in the SIL+ probably had a synergic effect combining more substrate availability for fermentation and lower phenols inhibition of fermentation.
Conclusion
The results of this study suggested GP as an alternative ingredient in animal feeding. The nutrient content of GP did not show important changes with ensiling, except for the reduction of NFC content, probably because of its role in fermentation kinetics. In addition, the level of condensed tannins precursors (flavanols) was reduced by the ensiling process. This was more effective if the L. plantarum 5BG was added to the silage. Moreover, total phenol content and antioxidant activity were both reduced by strain 5BG addition. These combined observations are consistent with the in vitro digestibility of the ensiled grape pomace, particularly when L. plantarum 5BG was added. In fact, all parameters investigated show increased percentage of disappearance, confirming a better in vitro digestibility of GP if ensiled with L. plantarum 5BG. Furthermore, ensiling with L. plantarum 5BG addition was able to affect in vitro rumen fermentation patterns and methane in vitro production. Although total volatile fatty acids production did not show differences, butyric acid and methane production slightly increased. In conclusion, ensiled grape pomace can represent an important source of digestible nutrient for ruminants and SIL+ may be an alternative feed. However, additional research demonstrating the in vivo utilization, or at least further advanced in vitro techniques are needed.
Data Availability Statement
The raw data supporting the conclusions of this article will be made available by the authors, without undue reservation.
Ethics Statement
The animal study was reviewed and approved by Ethics Committee for Animal Testing–CESA (process number 2-X/17) of the Department of Veterinary Medicine of the University of Bari.
Author Contributions
PDB: investigation, formal analysis, writing-original draft, and writing-review and editing. AM: conceptualization, investigation, data curation, formal analysis, writing-original draft, and writing-review and editing. CA: investigation. PDP: conceptualization, investigation, funding acquisition, resources, project administration, and writing-review and editing. FB: investigation, visualization, supervision, writing-original draft, and writing-review and editing. All authors contributed to the article and approved the submitted version.
Funding
This research was funded in part by the CNR-DiSBA project NutrAge (FOE-2019, DSB.AD004.271).
Conflict of Interest
The authors declare that the research was conducted in the absence of any commercial or financial relationships that could be construed as a potential conflict of interest.
Publisher's Note
All claims expressed in this article are solely those of the authors and do not necessarily represent those of their affiliated organizations, or those of the publisher, the editors and the reviewers. Any product that may be evaluated in this article, or claim that may be made by its manufacturer, is not guaranteed or endorsed by the publisher.
Acknowledgments
The authors would like to thank Stella Lisa Lonigro, Leone D'Amico, Giovanna Calzaretti, and Francesco Giannico for their technical assistance. We are grateful to the winery Crifo (Ruvo di Puglia, BA, Italy) for providing grape pomace. The authors are also grateful to Ludovico Varallo for the English revision of the manuscript. The authors thank the Regional Project Rete di Laboratori per l'Innovazione degli Alimenti Funzionali (LAIFF) (PO Puglia FESR 2007-2013 Asse I, Linea 1.2 - PO Puglia FSE 2007-2013 Asse IV) which allowed the spectrofluorimeter Infinite M-200 (Tecan Group Ltd., Männedorf, Switzerland) purchasing.
References
1. Salami SA, Valenti B, O'grady MN, Kerry JP, Mattioli S, Licitra G, et al. Influence of dietary cardoon meal on growth performance and selected meat quality parameters of lambs, and the antioxidant potential of cardoon extract in ovine muscle homogenates. Meat Sci. (2019) 153:126–34. doi: 10.1016/j.meatsci.2019.03.017
2. Moate PJ, Jacobs JL, Hixson JL, Deighton MH, Hannah MC, Morris GL, et al. Effects of feeding either red or white grape marc on milk production and methane emissions from early-lactation dairy cows. Animals. (2020) 10:976. doi: 10.3390/ani10060976
3. Williams SRO, Fisher PD, Berrisford T, Moate PJ, Reynard K. Reducing methane on-farm by feeding diets high in fat may not always reduce life cycle greenhouse gas emissions. Int J Life Cycle Assess. (2014) 19:69–78. doi: 10.1007/s11367-013-0619-8
4. Cieslak A, Szumacher-Strabel M, Stochmal A, Oleszek W. Plant components with specific activities against rumen methanogens. Animal. (2013) 7:253–65. doi: 10.1017/S1751731113000852
5. Brenes A, Viveros A, Chamorro S, Arija I. Use of polyphenol-rich grape by-products in monogastric nutrition. A review. Anim Feed Sci Technol. (2016) 211:1–17. doi: 10.1016/j.anifeedsci.2015.09.016
6. Ferri M, Bin S, Vallini V, Fava F, Michelini E, Roda A, et al. Recovery of polyphenols from red grape pomace and assessment of their antioxidant and anti-cholesterol activities. N Biotechnol. (2016) 33:338–44. doi: 10.1016/j.nbt.2015.12.004
7. Yu J, Ahmedna M. Functional components of grape pomace: their composition, biological properties and potential applications. (2013) 48:221–37. doi: 10.1111/j.1365-2621.2012.03197.x
8. Basalan M, Gungor T, Owens FN, Yalcinkaya I. Nutrient content and in vitro digestibility of Turkish grape pomaces. Anim Feed Sci Technol. (2011) 169:194–8. doi: 10.1016/j.anifeedsci.2011.07.005
9. Winkler A, Weber F, Ringseis R, Eder K, Dusel G. Determination of polyphenol and crude nutrient content and nutrient digestibility of dried and ensiled white and red grape pomace cultivars. Arch Anim Nutr. (2015) 69:187–200. doi: 10.1080/1745039X.2015.1039751
10. Santos GT, Lima LS, Schogor ALB, Romero JV, De Marchi FE, Grande PA, et al. Citrus pulp as a dietary source of antioxidants for lactating holstein cows fed highly polyunsaturated fatty acid diets. Asian-australas J Anim Sci. (2014) 27:1104–13. doi: 10.5713/ajas.2013.13836
11. Moate PJ, Williams SRO, Torok VA, Hannah MC, Ribaux BE, Tavendale MH, et al. Grape marc reduces methane emissions when fed to dairy cows. J Dairy Sci. (2014) 97:5073–87. doi: 10.3168/jds.2013-7588
12. Manso T, Gallardo B, Salvá A, Guerra-Rivas C, Mantecón AR, Lavín P, et al. Influence of dietary grape pomace combined with linseed oil on fatty acid profile and milk composition. J Dairy Sci. (2016) 99:1111–20. doi: 10.3168/jds.2015-9981
13. Ianni A, Di Maio G, Pittia P, Grotta L, Perpetuini G, Tofalo R, et al. Chemical-;nutritional quality and oxidative stability of milk and dairy products obtained from Friesian cows fed with a dietary supplementation of dried grape pomace. J Sci Food Agric. (2019) 99:3635–43. doi: 10.1002/jsfa.9584
14. Alipour D, Rouzbehan Y. Effects of ensiling grape pomace and addition of polyethylene glycol on in vitro gas production and microbial biomass yield. Anim Feed Sci Technol. (2007) 137:138–49. doi: 10.1016/j.anifeedsci.2006.09.020
15. Tabacco E, Piano S, Revello-Chion A, Borreani G. Effect of Lactobacillus buchneri LN4637 and Lactobacillus buchneri LN40177 on the aerobic stability, fermentation products, and microbial populations of corn silage under farm conditions. J Dairy Sci. (2011) 94:5589–98. doi: 10.3168/jds.2011-4286
16. Borreani G, Tabacco E, Schmidt RJ, Holmes BJ, Muck RE. Silage review: factors affecting dry matter and quality losses in silages. J Dairy Sci. (2018) 101:3952–79. doi: 10.3168/jds.2017-13837
17. Muck RE, Nadeau EMG, Mcallister TA, Contreras-Govea FE, Santos MC, Kung L. Silage review: Recent advances and future uses of silage additives. J Dairy Sci. (2018) 101:3980–4000. doi: 10.3168/jds.2017-13839
18. Ni K, Wang F, Zhu B, Yang J, Zhou G, Pan Y, et al. Effects of lactic acid bacteria and molasses additives on the microbial community and fermentation quality of soybean silage. Bioresour Technol. (2017) 238:706–15. doi: 10.1016/j.biortech.2017.04.055
19. Ren H, Feng Y, Pei J, Li J, Wang Z, Fu S, et al. Effects of Lactobacillus plantarum additive and temperature on the ensiling quality and microbial community dynamics of cauliflower leaf silages. Bioresour Technol. (2020) 307:123238. doi: 10.1016/j.biortech.2020.123238
20. Xu D, Ding Z, Wang M, Bai J, Ke W, Zhang Y, et al. Characterization of the microbial community, metabolome and biotransformation of phenolic compounds of sainfoin (Onobrychis viciifolia) silage ensiled with or without inoculation of Lactobacillus plantarum. Bioresour Technol. (2020) 316:123910. doi: 10.1016/j.biortech.2020.123910
21. Rodríguez H, Curiel JA, Landete JM, De Las Rivas B, De Felipe FL, Gómez-Cordovés C, et al. Food phenolics and lactic acid bacteria. Int J Food Microbiol. (2009) 132:79–90. doi: 10.1016/j.ijfoodmicro.2009.03.025
22. Zheng J, Wittouck S, Salvetti E, Franz CM, Harris H, Mattarelli P, et al. A taxonomic note on the genus Lactobacillus: Description of 23 novel genera, emended description of the genus Lactobacillus Beijerinck 1901, and union of Lactobacillaceae and Leuconostocaceae. Int J Syst Evol Microbiol. (2020) 70:2782–858. doi: 10.1099/ijsem.0.004107
23. Lavermicocca P, Angiolillo L, Lonigro SL, Valerio F, Bevilacqua A, Perricone M, et al. Lactobacillus plantarum 5BG survives during refrigerated storage bio-preserving packaged spanish-style table olives (cv. Bella di Cerignola). Front Microbiol. (2018) 9:889. doi: 10.3389/fmicb.2018.00889
25. Van Soest PJ, Robertson JB, Lewis BA. Methods for dietary fiber, neutral detergent fiber, and nonstarch polysaccharides in relation to animal nutrition. J Dairy Sci. (1991) 74:3583–97. doi: 10.3168/jds.S0022-0302(91)78551-2
26. Robinson PH, Givens DI, Getachew G. Evaluation of NRC, UC Davis and ADAS approaches to estimate the metabolizable energy values of feeds at maintenance energy intake from equations utilizing chemical assays and in vitro determinations. Anim Feed Sci Technol. (2004) 114:75–90. doi: 10.1016/j.anifeedsci.2003.12.002
27. Mcdonald P, Henderson A, Heron S. The Biochemistry of Silage. UK: Chalcombe Publications (1991).
28. Ávila CLS, Carvalho BF. Silage fermentation—updates focusing on the performance of micro-organisms. J Appl Microbiol. (2020) 128:966–84. doi: 10.1111/jam.14450
29. Gerardi C, Albano C, Calabriso N, Carluccio MA, Durante M, Mita G, et al. Techno-functional properties of tomato puree fortified with anthocyanin pigments. Food Chem. (2018) 240:1184–92. doi: 10.1016/j.foodchem.2017.08.057
30. Aleixandre-Tudo J, Du Toit W. The role of UV-Visible spectroscopy for phenolic compounds quantification in winemaking. In: Frontiers and New Trends in the Science of Fermented Food and Beverages. London, UK: IntechOpen (2019). Available online at: https://doi.org/10.5772/intechopen.79550
31. Chandra A, Rana J, Li Y. Separation, identification, quantification, and method validation of anthocyanins in botanical supplement raw materials by HPLC and HPLC–MS. J Agric Food Chem. (2001) 49:3515–21. doi: 10.1021/jf010389p
32. Maggiolino A, Lorenzo JM, Quiñones J, Latorre MA, Blando F, Centoducati G, et al. Effects of dietary supplementation with Pinus taeda hydrolyzed lignin on in vivo performances, in vitro nutrient apparent digestibility, and gas emission in beef steers. Anim Feed Sci Technol. (2019) 255:114217. doi: 10.1016/j.anifeedsci.2019.114217
33. Marten GC, Barnes RF. Prediction of energy digestibility of forages with rumen fermentation and fungal enzyme systems. In: Pigden CBW, Graham M, editors. Standardization of Analytical Methodology for Feeds Workshop. Ottawa, Canada: International Development Research Centre (1980).
34. Trotta RJ, Klotz JL, Harmon DL. Effects of source and level of dietary energy supplementation on in vitro digestibility and methane production from tall fescue-based diets. Anim Feed Sci Technol. (2018) 242:41–7. doi: 10.1016/j.anifeedsci.2018.05.010
35. Xu M, Rinker M, Mcleod KR, Harmon DL. Yucca schidigera extract decreases in vitro methane production in a variety of forages and diets. Anim Feed Sci Technol. (2010) 159:18–26. doi: 10.1016/j.anifeedsci.2010.05.005
36. Naziroglu M, Guler T, Yuce A. Effect of vitamin e on ruminal fermentation in vitro. J Vet Med A Physiol Pathol Clin Med. (2002) 49:251–5. doi: 10.1046/j.1439-0442.2002.00418.x
38. Spanghero M, Salem AZM, Robinson PH. Chemical composition, including secondary metabolites, and rumen fermentability of seeds and pulp of Californian (USA) and Italian grape pomaces. Anim Feed Sci Technol. (2009) 152:243–55. doi: 10.1016/j.anifeedsci.2009.04.015
39. Massaro Junior FL, Bumbieris Junior VH, Zanin E, Da Silva LDDF, Galbeiro S, Pereira ES, et al. Effect of storage time and use of additives on the quality of grape pomace silages. J Food Process Preserv. (2020) 44:e14373. doi: 10.1111/jfpp.14373
40. Laufenberg G, Kunz B, Nystroem M. Transformation of vegetable waste into value added products:: (A) the upgrading concept; (B) practical implementations. Bioresour Technol. (2003) 87:167–98. doi: 10.1016/S0960-8524(02)00167-0
41. Baumgärtel T, Kluth H, Epperlein K, Rodehutscord M. A note on digestibility and energy value for sheep of different grape pomace. Small Ruminant Research. (2007) 67:302–6. doi: 10.1016/j.smallrumres.2005.11.002
42. Ruberto G, Renda A, Amico V, Tringali C. Volatile components of grape pomaces from different cultivars of Sicilian Vitis vinifera L. Bioresour Technol. (2008) 99:260–8. doi: 10.1016/j.biortech.2006.12.025
43. Ben Salem H, Saghrouni L, Nefzaoui A. Attempts to deactivate tannins in fodder shrubs with physical and chemical treatments. Anim Feed Sci Technol. (2005) 122:109–21. doi: 10.1016/j.anifeedsci.2005.04.009
44. Jobim CC, Nussio LG, Reis RA, Schmidt P. Avanços metodológicos na avaliação da qualidade da forragem conservada. J Revista Brasileira de Zootecnia. (2007) 36:101–19. doi: 10.1590/S1516-35982007001000013
45. Zheng M, Niu D, Zuo S, Mao P, Meng L, Xu C. The effect of cultivar, wilting and storage period on fermentation and the clostridial community of alfalfa silage. Ital J Anim Sci. (2018) 17:336–46. doi: 10.1080/1828051X.2017.1364984
46. Schnürer J, Magnusson J. Antifungal lactic acid bacteria as biopreservatives. Trends Food Sci Technol. (2005) 16:70–8. doi: 10.1016/j.tifs.2004.02.014
47. Valerio F, Di Biase M, Lattanzio VMT, Lavermicocca P. Improvement of the antifungal activity of lactic acid bacteria by addition to the growth medium of phenylpyruvic acid, a precursor of phenyllactic acid. Int J Food Microbiol. (2016) 222:1–7. doi: 10.1016/j.ijfoodmicro.2016.01.011
48. Dolci P, Tabacco E, Cocolin L, Borreani G. Microbial dynamics during aerobic exposure of corn silage stored under oxygen barrier or polyethylene films. Appl Environ Microbiol. (2011) 77:7499–507. doi: 10.1128/AEM.05050-11
49. Maragkoudakis PA, Nardi T, Bovo B, D'andrea M, Howell KS, Giacomini A, et al. Biodiversity, dynamics and ecology of bacterial community during grape marc storage for the production of grappa. Int J Food Microbiol. (2013) 162:143–51. doi: 10.1016/j.ijfoodmicro.2013.01.005
50. He F, Liang N-N, Mu L, Pan Q-H, Wang J, Reeves MJ, et al. Anthocyanins and their variation in red wines i. monomeric anthocyanins and their color expression. Molecules. (2012) 17:1571–601. doi: 10.3390/molecules17021571
51. Kammerer D, Claus A, Carle R, Schieber A. Polyphenol Screening of pomace from red and white grape varieties (Vitis vinifera L.) by HPLC-DAD-MS/MS. J Agric Food Chem. (2004) 52:4360–7. doi: 10.1021/jf049613b
52. Rockenbach II, Gonzaga LV, Rizelio VM, Gonçalves AEDSS, Genovese MI, Fett R. Phenolic compounds and antioxidant activity of seed and skin extracts of red grape (Vitis vinifera and Vitis labrusca) pomace from Brazilian winemaking. Food Res Int. (2011) 44:897–901. doi: 10.1016/j.foodres.2011.01.049
53. Sri Harsha PSC, Gardana C, Simonetti P, Spigno G, Lavelli V. Characterization of phenolics, in vitro reducing capacity and anti-glycation activity of red grape skins recovered from winemaking by-products. Bioresour Technol. (2013) 140:263–8. doi: 10.1016/j.biortech.2013.04.092
54. Nicoletti I, Bello C, De Rossi A, Corradini D. Identification and quantification of phenolic compounds in grapes by HPLC-PDA-ESI-MS on a semimicro separation scale. J Agric Food Chem. (2008) 56:8801–8. doi: 10.1021/jf801411m
55. Lopes P, Richard T, Saucier C, Teissedre P-L, Monti J-P, Glories Y. Anthocyanone A: A quinone methide derivative resulting from malvidin 3-O-glucoside degradation. J Agric Food Chem. (2007) 55:2698–704. doi: 10.1021/jf062875o
56. Melo PS, Massarioli AP, Denny C, Dos Santos LF, Franchin M, Pereira GE, et al. Winery by-products: Extraction optimization, phenolic composition and cytotoxic evaluation to act as a new source of scavenging of reactive oxygen species. Food Chem. (2015) 181:160–9. doi: 10.1016/j.foodchem.2015.02.087
57. Claus H, Mojsov K. Enzymes for wine fermentation: current and perspective applications. Fermentation. (2018) 4:52. doi: 10.3390/fermentation4030052
58. González-Paramás AM, Esteban-Ruano S, Santos-Buelga C, De Pascual-Teresa S, Rivas-Gonzalo JC. Flavanol content and antioxidant activity in winery byproducts. J Agric Food Chem. (2004) 52:234–8. doi: 10.1021/jf0348727
59. Rodríguez Montealegre R, Romero Peces R, Chacón Vozmediano JL, Martínez Gascueña J, García Romero E. Phenolic compounds in skins and seeds of ten grape Vitis vinifera varieties grown in a warm climate. J Food Compos Anal. (2006) 19:687–93. doi: 10.1016/j.jfca.2005.05.003
60. Cheng VJ, Bekhit AE-DA, Mcconnell M, Mros S, Zhao J. Effect of extraction solvent, waste fraction and grape variety on the antimicrobial and antioxidant activities of extracts from wine residue from cool climate. Food Chem. (2012) 134:474–82. doi: 10.1016/j.foodchem.2012.02.103
61. Jayanegara A, Wina E, Soliva CR, Marquardt S, Kreuzer M, Leiber F. Dependence of forage quality and methanogenic potential of tropical plants on their phenolic fractions as determined by principal component analysis. Anim Feed Sci Technol. (2011) 163:231–43. doi: 10.1016/j.anifeedsci.2010.11.009
62. Min BR, Solaiman S, Shange R, Eun J-S. Gastrointestinal bacterial and methanogenic archaea diversity dynamics associated with condensed tannin-containing pine bark diet in goats using 16S rDNA amplicon pyrosequencing. Int J Microbiol. (2014) 2014:141909. doi: 10.1155/2014/141909
63. Makkar HPS, Singh B. Effect of storage and urea addition on detannification and in sacco dry matter digestibility of mature oak (Quercus incana) leaves. Anim Feed Sci Technol. (1993) 41:247–59. doi: 10.1016/0377-8401(93)90017-E
64. Yilmaz Y, Toledo RT. Oxygen radical absorbance capacities of grape/wine industry byproducts and effect of solvent type on extraction of grape seed polyphenols. J Food Compos Anal. (2006) 19:41–8. doi: 10.1016/j.jfca.2004.10.009
65. Rockenbach II, Rodrigues E, Gonzaga LV, Caliari V, Genovese MI, Gonçalves AEDSS, et al. Phenolic compounds content and antioxidant activity in pomace from selected red grapes (Vitis vinifera L. and Vitis labrusca L) widely produced in Brazil. Food Chem. (2011) 127:174–9. doi: 10.1016/j.foodchem.2010.12.137
66. Olivo PM, Santos GTD, Ítavo LCV, Silva Junior RCD, Leal ES, Prado RMD. Assessing the nutritional value of agroindustrial co-products and feed through chemical composition, in vitro digestibility, and gas production technique %J Acta Scientiarum. Anim Sci. (2017) 39:289–95. doi: 10.4025/actascianimsci.v39i3.34024
67. Mcsweeney CS, Palmer B, Mcneill DM, Krause DO. Microbial interactions with tannins: nutritional consequences for ruminants. Anim Feed Sci Technol. (2001) 91:83–93. doi: 10.1016/S0377-8401(01)00232-2
68. Makkar HPS. Effects and fate of tannins in ruminant animals, adaptation to tannins, and strategies to overcome detrimental effects of feeding tannin-rich feeds. Small Ruminant Res. (2003) 49:241–56. doi: 10.1016/S0921-4488(03)00142-1
69. Holzer M, Mayrhuber E, Danner H, Braun R. The role of Lactobacillus buchneri in forage preservation. Trends Biotechnol. (2003) 21:282–7. doi: 10.1016/S0167-7799(03)00106-9
70. Gollop N, Zakin V, Weinberg ZG. Antibacterial activity of lactic acid bacteria included in inoculants for silage and in silages treated with these inoculants. J Appl Microbiol. (2005) 98:662–6. doi: 10.1111/j.1365-2672.2004.02504.x
71. Oliveira DA, Salvador AA, Smânia A, Smânia EFA, Maraschin M, Ferreira SRS. Antimicrobial activity and composition profile of grape (Vitis vinifera) pomace extracts obtained by supercritical fluids. J Biotechnol. (2013) 164:423–32. doi: 10.1016/j.jbiotec.2012.09.014
72. Bueno ICS, Brandi RA, Fagundes GM, Benetel G, Muir JP. The role of condensed tannins in the in vitro rumen fermentation kinetics in ruminant species: feeding type involved? Animals. (2020) 10:635. doi: 10.3390/ani10040635
73. Liang J, Fang W, Wang Q, Zubair M, Zhang G, Ma W, et al. Metagenomic analysis of community, enzymes and metabolic pathways during corn straw fermentation with rumen microorganisms for volatile fatty acid production. Bioresour Technol. (2021) 342:126004. doi: 10.1016/j.biortech.2021.126004
74. Alexander G, Singh B, Sahoo A, Bhat TK. In vitro screening of plant extracts to enhance the efficiency of utilization of energy and nitrogen in ruminant diets. Anim Feed Sci Technol. (2008) 145:229–44. doi: 10.1016/j.anifeedsci.2007.05.036
75. Liu H, Li K, Zhao J, Deng W. Effects of polyphenolic extract from Eucommia ulmoides Oliver leaf on growth performance, digestibility, rumen fermentation and antioxidant status of fattening lambs. Anim Sci J. (2018) 89:888–94. doi: 10.1111/asj.12998
76. Davies ZS, Mason D, Brooks AE, Griffith GW, Merry RJ, Theodorou MK. An automated system for measuring gas production from forages inoculated with rumen fluid and its use in determining the effect of enzymes on grass silage. Anim Feed Sci Technol. (2000) 83:205–21. doi: 10.1016/S0377-8401(99)00138-8
77. Patra AK. Meta-analyses of effects of phytochemicals on digestibility and rumen fermentation characteristics associated with methanogenesis. J Sci Food Agric. (2010) 90:2700–8. doi: 10.1002/jsfa.4143
78. Anantasook N, Wanapat M, Cherdthong A, Gunun P. Effect of plants containing secondary compounds with palm oil on feed intake, digestibility, microbial protein synthesis and microbial population in dairy cows. Asian Australas J Anim Sci. (2013) 26:820–6. doi: 10.5713/ajas.2012.12689
79. Supapong C, Cherdthong A, Seankamsorn A, Khonkhaeng B, Wanapat M, Gunun N, et al. Effect of Delonix regia seed meal supplementation in Thai native beef cattle on feed intake, rumen fermentation characteristics and methane production. Anim Feed Sci Technol. (2017) 232:40–8. doi: 10.1016/j.anifeedsci.2017.08.004
Keywords: grape pomace, silage, polyphenol, antioxidant capacity, lactic acid bacteria, in vitro digestion
Citation: De Bellis P, Maggiolino A, Albano C, De Palo P and Blando F (2022) Ensiling Grape Pomace With and Without Addition of a Lactiplantibacillus plantarum Strain: Effect on Polyphenols and Microbiological Characteristics, in vitro Nutrient Apparent Digestibility, and Gas Emission. Front. Vet. Sci. 9:808293. doi: 10.3389/fvets.2022.808293
Received: 03 November 2021; Accepted: 07 January 2022;
Published: 24 February 2022.
Edited by:
Anil Kumar Puniya, National Dairy Research Institute (ICAR), IndiaReviewed by:
Federica Mannelli, University of Florence, ItalyAnusorn Cherdthong, Khon Kaen University, Thailand
Copyright © 2022 De Bellis, Maggiolino, Albano, De Palo and Blando. This is an open-access article distributed under the terms of the Creative Commons Attribution License (CC BY). The use, distribution or reproduction in other forums is permitted, provided the original author(s) and the copyright owner(s) are credited and that the original publication in this journal is cited, in accordance with accepted academic practice. No use, distribution or reproduction is permitted which does not comply with these terms.
*Correspondence: Aristide Maggiolino, YXJpc3RpZGUubWFnZ2lvbGlubyYjeDAwMDQwO3VuaWJhLml0