- 1Food Security and Safety Niche Area, Faculty of Agriculture, Science and Technology, North-West University, Mmabatho, South Africa
- 2Department of Chemistry, Faculty of Natural and Agricultural Sciences, North-West University, Mmabatho, South Africa
- 3Indigenous Knowledge Systems (IKS) Centre, Faculty of Natural and Agricultural Sciences, North-West University, Mmabatho, South Africa
Bovine mastitis (BM) is the traditional infectious condition in reared cattle which may result in serious repercussions ranging from animal welfare to economic issues. Owing to the high costs associated with preventative practices and therapeutic measures, lower milk output, and early culling, bovine mastitis is accountable for most of the financial losses suffered in cattle farming. Streptococcus agalactiae, Staphylococcus aureus, Streptococcus dysgalactiae and coliform bacteria are the predominant pathogens for bovine mastitis. In addition, the occurrence of BM has been linked to lactation stage and poor management, in the latter case, the poor stabling conditions around udder hygiene. BM occurs throughout the world, with varying rates of Streptococcus agalactiae infection in different regions. Despite the modern techniques, such as the appropriate milking practices that are applied, lower levels of pathogen vulnerability may help to prevent the development of the disease, BM treatment is primarily reliant on antibiotics for both prophylactic and therapeutic purposes. Nevertheless, as a result of the proliferation of bacterial agents to withstand the antibiotic effects, these therapies have frequently proven ineffectual, resulting in persistent BM. Consequently, alternative medicines for the management of udder inflammation have been researched, notably natural compounds derived from plants. This review focuses on BM in terms of its risk factors, pathogenesis, management, the molecular identification of causative agents, as well as the application of ethno-veterinary medicine as an alternative therapy.
Introduction
Bovine mastitis (BM) is associated with the swelling of the udder which is caused by various factors, such as contagious pathogens, poor nutrition, and ineffectual management conditions on the farm (1, 2) (Figure 1). Its effects range from inferior animal welfare to mediocre industrial economic conditions, some of which encompass, without bounds, reduced milk yield, increased therapeutic costs, and premature culling (3–5). Moreover, different microorganisms, including bacteria, can cause mastitis. Pathogens that are most frequently encountered in a mastitic condition are Staphylococcus aureus, Streptococcus uberis, Streptococcus agalactiae, Streptococcus dysgalactiae, Escherichia coli, and other coliforms (6). Vanderhaeghen et al. (7) state that the clinical presentation of bovine mastitis assumes two distinct forms (clinical or subclinical) (8), which are distinguished by a rise in the pathological cell number. Clinical mastitis is marked by evident alterations in the quality and composition of the milk, including the level of coagulants, and is often followed by pervasive indicators and impairments, inter alia high temperatures, in the animal. On the other hand, BM caused by environmental pathogens is characterized by an increase in the somatic cell count (SCC), resulting in significant cost implications, especially on account of the increased cell count in milk samples (2, 8). According to Giesecke et al. (9), annual milk wastage owing to BM on farms in South Africa amounted to approximately ZAR 29.68 million. More recent data on the prevalence of BM in South Africa are unavailable, probably due to the fact that the condition is not commonly and regularly reported (10).
Antibiotics have long been considered the first line of defense against bacterial infections in dairy cattle, especially in the case of mastitis, where antibiotic residues occur in the milk and there is the risk that microbial resistance will spread to the environment (5, 11). Owing to the proliferation of multiple antibiotic-resistant (MAR) bacteria, which is a pressing public health concern for animal and human health, food security, and development, the use of antibiotics in animal production has been studied with great caution (12, 13). From the one-health perspective, mastitis-causing bacteria have broken through a number of hierarchical barriers, allowing for zoonotic transmission from bovines to people via milk and meat, thus putting public health at risk. Hence, there is a significant risk of microbial contaminants from the animal to the pastures and the equipment, to the effect that the consumption of raw milk may cause food-borne infections. Pasteurization of milk is thus essential to guarantee its quality and to extend its shelf-life (14).
In this age of the proliferation of microbial resistance to antimicrobials, it is imperative to source alternative medicines for the management and prevention of bovine mastitis. Hence, this review focuses on BM in terms of risk factors, pathogenesis, control and treatments, the molecular identification of causative agents, as well as the application of ethno-veterinary medicine (EVM) as an alternative therapy for BM.
Method for Selection of Literature
Keywords such as bovine mastitis, ethno-veterinary medicine, livestock and antimicrobial resistance were used to promote the literature search technique. In addition, phrases such as ethno-botany, alternative medicine, anti-inflammatory and antibacterial effect were employed to generate data for the biological activity and the phytochemical characteristics discussed in the review. These keywords were used alone and in combination to find relevant material from electronic data base such as Google Scholar, Pubmed, Science Direct, and Web of Science. To be considered for inclusion in the review, an article or study should have included and identified information about the usage of a specific medicinal plant in the ethno-medicinal context for treating BM within the research period (i.e., up until October 2021).
The following data were obtained for each study: the plant's scientific name, plant part, life form, disease treated, dose, preparation and administration. Articles reporting on the ethno-veterinary applications (Table 2) and the preparation and administration of medicinal plant products against antimicrobial resistant BM pathogens (Table 3) met the inclusion criteria. Review and research papers not involving the ethno-veterinary medicinal approach in combating antimicrobial resistance of mastitis pathogens were excluded. Finally, all papers that met the inclusion criteria were retrieved for the collection of adequate information. All scientific plant names were cross-referenced on the international databases, namely The World Flora Online (http://www.worldfloraonline.org/) and The Plant List (www.theplantlist.org).
Burden of Mastitis on Dairy Farms
Udder inflammation is often regarded as the ultimate threat to the dairy sector, resulting in both financial losses and harmful impacts on public health. Mastitis is also the foremost source of mortality in dairy cows. Mastitis-related financial losses are difficult to quantify; however, financial losses are attributed to the cost of medications, veterinary services, laboratory fees, and additional labor for the farmer. Losses also include those incurred on account of lost milk, culling/deaths and treatment costs (29). Amuta et al. (30) reported that the majority of farmers covered in their study in the North-West region of Nigeria considered clinical mastitis (CM)-related milk production losses to be significant (55%).
In Germany, milk wastage, ranging from 27 to 52%, (median rate; 37%) and rejected milk, ranging from 13 to 30%, (median value; 20%), were the most expensive charges associated with mastitis (29). Replacement expenditures, which were due to culling, and mastitis mortality, ranging from 3 to 28% with a median value of 22%, were the next biggest losses reported (median 22%). More so, Beyene and Tolosa (31) reported an average yearly drop of 59,719.08ETB, equivalent to USD 2,949.8, on account of mastitis, and a milk loss of 22.3% in crossbred cattle and of 2.24% in local zebu cattle in Ethiopia. The productive impact of subclinical mastitis in Colombian cattle ranches was estimated at about USD 800,000 per year, with milk production losses ranging from 1.3 to 13.5% per farm (32).
The losses in the value of milk and veterinary expenses in Central India on account of mastitis were reported to be about 49 and 37%, respectively (33). According to a study released by the University of Glasgow (https://www.fortunebusinessinsights.com/bovine-mastitis-market-103482), mastitis is predicted to cost the global dairy industry between USD 19.7 and 30 billion every year. Furthermore, another associated cost is a shortening of the lactation term of the animals. According to Khan and Khan (34), each infected animal's lactation period is reduced by about 57 days, while Seegers et al. (35) described reductions in mean milk output to be 375 kg for each lactation.
Risk Factors and Prevalence of Bovine Mastitis
The harmful factors associated with BM are classified as intrinsic and extrinsic and include, amongst others, species, age, parity, suckling period, antiquity of condition, state of habitat, and common handling conditions (36). These were all found to be remarkably related to BM. Conversely, milk-gland washing and drying and antiquity of condition had no remarkable outcome on the occurrence of BM (37). Nearly 30% of Saharan countries currently register instances of BM, leaving the rest of the continent in the dark (38). At the cow stage, the overall mastitis prevalence recorded on dairy farms in some parts of Africa is < 54%, with CM at < 14% and subclinical mastitis at 16 to 88%. Mastitis was found to be more than 50% prevalent in previous research studies conducted in various parts of the southern region of Saharan Africa, with subclinical mastitis ranging from 16 to 80% (39). Mastitis is also common in Asian countries, with a prevalence of over 50% (40). Kvapilik et al. (41) published the global prevalence of sub-CM as ranging from 15 to 75%. Also, with the increase in the counts of cattle farms, the frequency of BM is escalating tremendously on a yearly basis.
The Pathogenesis and Prevalence of Bovine Mastitis
Invading pathogens penetrate the irritated udder through the teat canal, which is located at the anterior margin of the cow mamilla (42, 43). In addition to physical stress and chemical irritants, bacterial pathogens grow and produce toxins that cause harm to the milk-producing tissue. The quantity of leukocytes in the milk increases as an effect of these factors. The initial line of defense is presented by the bovine mammary epithelial cells (bMECs). Thus, the bMECs play a significant role in that they provide broad and unique protection against resistant pathogenic microorganisms (42). More so, the udder may be contaminated by a number of microbial pathogens, which kill these cells and result in mastitis (44, 45).
A sphincter of smooth muscles covers the mamilla of the milk duct and works to ensure that it is closed from the outside (46). By preventing milk from escaping, it also prevents pathogens from entering the teat. Keratin generated from the stratified squamous epithelium lines the mamillary duct from the inside. The effectiveness of the keratin in the teat canal is to suppress the bacteria to a limited extent and to prevent their proliferation (47). Keratin is a waxy substance made up of phospholipids and fibrous proteins. Keratin fiber proteins are able to bind electrostatically to mastitis pathogens in the teat canal, thus altering the bacterial cell wall and making it more vulnerable to osmotic pressure. Invading pathogens are inhibited and killed when osmotic pressure is not maintained (48). During the milking process, germs near the teat's opening find a way into the teat canal, causing shock and damage to the keratin and mucous membranes surrounding the teat sinus (49). Also, after milking, the teat canal may remain partially open for 1 to 2 h, allowing infections to freely enter the teat canal (50).
The global prevalence rates of subclinical (SCM) and clinical mastitis (CM) are 42 and 15%, respectively (51). The SCM prevalence was found to be higher when compared to CM prevalence in the countries of the World. In most of the studies, CMT and its clinical manifestation were used for diagnosing SCM and CM, respectively. The pervasiveness rates for SCM and CM were found to be 45 and 18%, respectively in India. As stated by the College of Veterinary Medicine, Cornell University, clinical mastitis affects from 0 to 200 cows per 100 annually. Several studies from around the world have estimated the yearly occurrence of clinical mastitis as 25 to 30 cases per 100 cows (https://www.fortunebusinessinsights.com/bovine-mastitis-market-103482). In an Ethiopian study, the overall mastitis occurrence was reported to be 73.7% (283/384), of which 28.9% (82/283) was of clinical importance and 71.02% (201/283) was of subclinical significance (52). A Japanese research study stated the prevalent rates of CM and SCM to be 28 and 13%, respectively, while a closely-related margin of 23.6% was reported from an American study (53).
Diagnosis of Bovine Mastitis
When the udder of a cow is inflamed, there are several pointers or bio-signatures which are freed and/or influenced as a result of the changes in the milk. These indicators are set out as testing pointers for mastitis (54). Rossi et al. (55) have outlined several testing techniques, ranging from common examinations (somatic cell counts, the California mastitis test) (8) to molecular techniques (polymerase chain reactions) (56), and other sophisticated methods, such as loop-mediated isothermal amplification (LAMP) (57), genetic (58), proteome, as well as transcriptome (59, 60) analyses, nano-assembly and the micro-modeling of compact or mobile gadgets (61) which are used to detect these markers.
Conventional Screening Techniques for Bovine Mastitis
Regardless of its drawbacks, which include culturing time and costs (62), the microbiological investigation of milk is regarded as the gold standard for detecting bovine mastitis. However, during the inflammatory phase, leukocytes migrate in large numbers to the location site of the inflammation (63). Consequently, the cellularity of the milk can be used to assess the health of the mammary gland of the animal. Techniques including SCC, CMT and Somaticell® are the secondary ways for identifying BM (64).
Somatic Cell Count
Somatic cell count (SCC) is a technique that quantitatively detects the various categories of cells in milk (62). Using this method, figures between 100,000 and 272,000 cells mL−1 connote the presence of mastitis-causative agents (62). SCC can be carried out using an automated system (Somacount 300 automatic somatic cell counter) or manually by viewing stained microscope slides with appropriate reagents and then viewed using a microscope.
The California Mastitis Test
CMT is an elementary, low-cost, quick evaluation that can be conducted within the cattle ranch and is commonly used for identifying subclinical mastitis during milking ((64); 8). The California Mastitis Test is one of the most effective ways to identify mastitis (CMT). To perform the test, only a four-compartmentalized paddle and the CMT reagent are needed. Milk is appropriately collected in the CMT paddle and an equivalent amount of the indicator is added using a horizontal swirling motion for about 30 s. Results are then interpreted accordingly. A strong gel formation that tends to adhere to the paddle and with a leucocyte count of over five million per milliliter of milk sample would point to a strong positive result. A distinct gel formation with a leucocyte count of between 800,000 and 5,000,000,000 mL−1 of milk sample would also indicate a positive result. Distinct precipitate formation with a paddle movement but without gel formation and with a leucocyte count of 400,000 to 1,500,000 per milliliter of milk sample would show a weak positive result. On the other hand, a trace result, denoted by a slight precipitate, which would tend to disappear with paddle movement and which is associated with a leucocyte count of 150,000 to 500,000, would be representative of a mixture without a precipitate and would point to a negative test result (https://extension.missouri.edu/publications/g3653).
Somaticell®
This is a numerical assessment technique comparable to the Wisconsin Mastitis check that was created with the goal of producing outcomes matching those of the SCC, yet having an edge of portability (65). This technique is also advised for usage in bulk tank milk as a pilot test to determine whether it satisfies the current legal limits. A single-use graded plastic vial with a capped preset scale of SCC is used in this test (63).
Automatic Digital Diagnostic
These are newer testing techniques for the examination of mastitis in bovine. They are very easy, fast and field applicable methods (66). These techniques detect the physical, chemical, and biological changes of milk, or quantify the biomarkers in relation to mastitis. The methods include the DeLaval cell counter, the Afimilk mastitis detector, and the Draminski mastitis detector (67). The DeLaval cell counter is suitable for determining the somatic cell count.
Other newer techniques in diagnosing mastitis include the sensor-based system, infra-red thermography (IRT), and proteomic applications. Sensor-based detection systems are usually employed on large farms. A Portuguese study reported the use of magnetic nanoparticles for the actual identification of several staphylococci and specifically Staphylococcus aureus. The proteomic approach is helpful in diagnosing, preventing, and forecasting mastitis (68). It aids in the detection of the biomarkers of the intramammary infection (IMI). Hettinga et al. (69) reported the observation of some protein-related substances in relation to the host's reaction to intramammary infection.
Molecular Techniques for Bacterial Identification for Improved Treatment
Although culture-based approaches for diagnosing intramammary infection (IMI) remain the gold standard in many diagnostic centers, culture-independent methods for identifying bacterial infections in milk have grown more widespread in recent years (8).
When compared to culture-based approaches, molecular-based techniques have been proven to be more sensitive and more rapid since their findings may be ready in only a few hours (8). Bacterial taxonomy and phylogeny are based on conserved gene sequences, particularly the ones that denote ribosomal ribonucleic acids (rRNA). They are used as universal molecular chronometers since they contain sections of varying evolutionary degrees (some extremely conserved; others highly changeable and all-pervasive) (70).
Whole Genome Sequencing
This technique yields data on (a) pathogen naming, (b) the characterization of resistant genes (c) epidemiological typing, (d) the selection of peculiar types, regardless of primer development (to track genetic changes in organisms, their adaptations and modifications), and (e) the instant drafting of polymerase chain reaction (PCR) probes based on WGS figures (70). WGS has provided valuable information that has aided advances in present screening techniques, including the detection of the mecC gene (a homolog of the mecA gene that is responsible for methicillin resistance in MRSA) which have elicited the reworking of PCR tests to boost reactivity and circumvent fallacies (71). When prognostics drawn from WGS figures were compared to phenotypic drug vulnerability, WGS was found to be very insightful and specific, as well as to show significant similarities with phenotypic antibacterial susceptibility/resistance approaches (72).
Conventional Treatment Options for Bovine Mastitis
Prophylaxis
Bovine mastitis is characterized by the epidemiological triad; host, pathogen, and environment. The expression, “Better prevent than cure,” accurately describes the disease mastitis, as there are many changes, such as harm to the teat and teat canal and udder alveoli that cannot be cured. These are the effects of howl arising from the condition. Mastitis can be circumvented following good acceptable procedures (73, 74). Reduced new infections (NI) and pathogen transmission through improved management standards, the segregation of the animal, and mitigations in the exacerbation of subclinical to clinical mastitis through a regular supply of nutrients are all possible preventative approaches (75). The maintenance of udder health is a process that is always being improved upon and which has already progressed to a high level in that it allows for additional improvements to reduce antimicrobial usage, albeit in a slow and methodical manner (75). Researchers have been working on efficient vaccinations to prevent bovine mastitis for decades, but established vaccines, such as those for E. coli or S. aureus intramammary infections (IMI) provide only limited protection (76).
Therapeutic Measures
Dry-off Treatment
The dry phase linking two milking periods is critical for teat well-being in dairy cows. It offers an encouraging way to treat current teat contamination, but also provides for conditions where the udder would be likely to incubate a new intramammary infection (IMI). Infections caused by the dry phase often cause increased somatic cell counts (SCC) in early breastfeeding. Management of udder health during the dry season is critical for a smooth transition into the lactation period (75). Epithelial cells that have been damaged or have become senescent are biologically replaced (77). The regeneration and phagocytosis of pathogens cure a substantial amount of IMI without interference, and antimicrobial dry cow therapy is highly effective because the medication is not milked out, thereby allowing for a greater and more consistent concentration of antibiotics in the udder. Thus, most IMIs heal (78).
Use of Antibiotics
The goal of managing mastitis with antibiotics is to eradicate the pathogens from the cattle, or worse still, those from the udder. Antibiotics are often used to manage mastitis in lactating cows, as well as to prevent intramammary infections in non-lactating cows (4, 5). More or less 60–70% of the antimicrobials used in cattle are for the prevention and treatment of mastitis (79). Antibiotics, such as the beta-lactams (penicillin G) and macrolides (erythromycin), are generally accepted for the management of bovine mastitis (2, 80). Nevertheless, the non-selective use of antibacterials without the in-vitro sensitivity testing of causative organisms is one of the major reasons for failures in the treatment of mastitis (81). Bacterial isolation and antibiotic sensitivity tests are always required for effective antibiotic therapy. According to Holko et al. (82), over 62 percent of the examined mastitis bacterial pathogens were insusceptible to one or more antibacterial drugs. In the majority of instances, the identified microbes were insusceptible to cephalexin, neomycin, penicillin, and streptomycin. Resistance was discovered in 86% of S. uberis isolates and 79% of E. coli isolates.
Mode of Action of Antibiotics
The course of efficacy of chemotherapeutics can be classified on the basis of the functions that the agents influence which commonly include the inhibition of cell wall synthesis, the suppression of nucleic acid synthesis, the repression of ribosome function, cell membrane function, and folate metabolism inhibition (83) (Figure 2).
Development of Antimicrobial Resistance
The overuse of antibiotics in clinical practice has led to bacterial resistance to antimicrobial medicines (84). As it accounts for several losses, MR is a significant challenge in the management of microbial infections in the food and dairy sector and also in the treatment of human diseases. Antibiotic inactivation, target alteration, altered permeability, and the “bypassing” of metabolic pathways are some of the biochemical resistance mechanisms exploited by bacteria (84). The basic anatomy of a Gram-positive bacterium has a cytoplasmic membrane which is surrounded by a thick and stiff mesh, termed the cell wall. Conversely, Gram-negative bacteria have a thin cell wall that is bordered by a second lipid membrane, termed the outer sheath. In Gram-negative bacteria, the sheath is an extra protective layer that inhibits numerous chemicals from entering the organism (84). A growing number of antibiotic-resistant bacteria are jeopardizing the effectiveness of antimicrobials (83). Resistance can be discussed in two broad terms:
✓ Intrinsic resistance occurs when microorganisms lack natural antimicrobial target sites and the antibiotic has no effect on them.
✓ Acquired resistance occurs when a naturally susceptible bacterium develops a method to resist an antibiotic. Antibiotic resistance in bacteria can be acquired through mutations in regular genetic codes or the uptake of alien insusceptible genetic codes, or both. The presence of an enzyme that causes inactivity in the antibacterial drug, that reduces the uptake of the antimicrobial agent, and the proteinaceous transportation of the anti-infective agent, are all examples of acquired resistance mechanisms (85).
Genetics of Antimicrobial Resistance
Bacterial non-susceptibility is a complicated condition that begins with the existence of genes encoding resistance in plasmids or chromosomal genetic material. Considerably aided by the proliferation of flexible genetic features, such as plasmids, transposons, and integrons, there has been a rapid transmission of antibiotic resistance across the various human bacterial groups (86). This is considered to be a matter for serious veterinary concern. Antimicrobial resistance genes (ARGs) have been found to abound on transposable segments (Table 1). Hence, an exclusive occurrence at the genetic level can transmit multiple-drug support traits to a vulnerable receiver. The amplification of integrase genes (intI 1, intI 2 and intI 3) can be used to determine the presence of integrons (98). A couple of researchers have reported some ARGs and their location sites in some isolates of animal origin (Table 1).
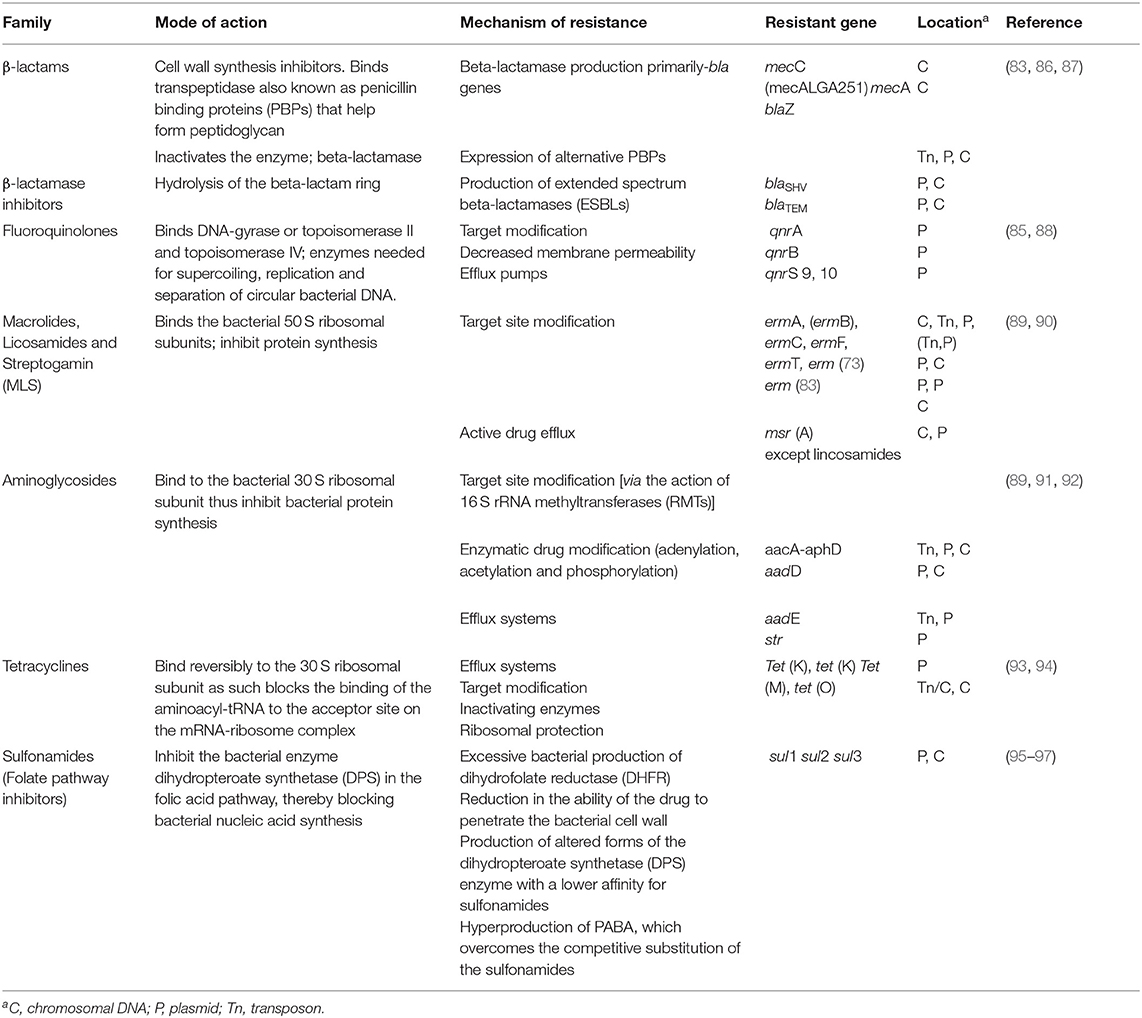
Table 1. Mode of action, mechanisms of resistance of antibiotics and some antimicrobial resistance genes (ARG) of animal origin.
Challenges of Treatment With Antibiotics
The shortcomings of this strategy include low cure rates, the increased occurrence of antibiotic-resistance; pressing public health concerns, reduced food safety, security, and their upshots (12, 13), as well as the appearance of flakes in cow products (5, 11, 99). Milk tainted with antibiotics can cause sensitive reactions, alterations in the microbial makeup of the gut, and the growth of antibiotic-resistant strains in the general public (100). Because antibiotics aren't allowed to reach them, bacteria that can live intracellularly within the udder and that cause sores to develop are laborious to treat. This is evident with S. aureus, where the cure rate, based on the generally accepted antibiotics (e.g., pirlimycin), is about 10–30% (76). Consequently, these pathogens have developed resistance to antibiotic treatment. Thus, the World Health Organization (WHO) has released guidelines for limiting antibiotic use in livestock farming (101, 102).
More so, from the one-health perspective, mastitis-causing bacteria have broken through a number of hierarchical barriers, allowing for zoonotic transmission from bovines to people via milk and meat, either locally or remotely, and as a result, disease control has been quite challenging (103).
Alternative Treatment to Conventional Antibiotics
Ethno-Veterinary Medicine
Ethno-veterinary medicine (EVM) is the application of humankind's expertise, abilities, practices, habits, and credence in managing and safekeeping the well-being of their animals (104). These hands-on skills are shared or transferred from age to age only through the oral medium (105). Both the scientific and consumer communities are increasingly turning to herbal plants as health promoters (106). In the interests of the foremost availability of these products and the cheap manufacturing costs around these items, studies using local plants must of necessity encompass multiple geographical locations. Kalayou et al. (28) investigated the antibacterial potency of several plant species against mastitis, with Croton aurea, Croton macrostachyus, Achyranthes aspera, Nicotiana tabacum and Vernonia species producing the most promising effects of all tested plants. Findings by Serunkuma et al. (107) established the efficacy of the acetone extract of the Acacia nilotica bark and the Tetradenia riparia flower against bacterial species cultured from mastitis samples from a farm. Across the board, the development of S. agalactiae, S. uberis, E. coli and S. aureus was suppressed by a methanol extract derived from Spathodea campanulata (108). Globally, the use of diverse plants from distinct geographical terrains to treat livestock infections remains common (Table 2). In-vitro assays into medicinal plants reveal their potency as antibacterial, anti-inflammatory or immune-modulatory agents (109).
Application of Ethno-Veterinary Medicine in the Treatment and Management of Mastitis
Ethno-veterinary medicine is a local animal healthcare system that incorporates traditional beliefs, knowledge, skills, methods, and practices. It includes the traditional treatment of veterinary illnesses, as well as the spiritual aspects of the treatment (24). Depending on the active substances to be extracted, the delivery route, and the medicinal purpose (prophylaxis or therapeutics), the method used to make ethno-veterinary medications differs. Infusions, decoctions, powders, drips, fumes, pastes, and ointments are made from medical plants, animal products, minerals, and other inorganic ingredients accessed by livestock owners and ranchers. These might be treated topically with drenches, or intra-nasally with smoke, vaccines, or suppositories, vapors, or massages (24).
Abbasi et al. (23) reported that paste made from the fruit juice of Citrus limon and sugar fed to animals and applied topically to the mammary glands for 10–15 days is used to treat mastitis in buffalo, cattle and goats. Also, the application of a paste of 200 g of fresh crushed roots of Withania somnifera to the udder of a cow and goat respectively for up to a week successfully treats the diseased condition. It is also noteworthy to state that the preparation and application of the smoke of the leaves and branches of Peganum harmala over a period of about 5 days successfully treats mastitis in cattle and horses. The anti-inflammatory effects of the topical application of the extract of fresh leaves of Rumex nepalensis to the affected part for about 5 days has also been reported. A poultice of young twigs of Calotropis procera applied to a swollen udder also relieves pain and inflammation (23). The treatment of BM using several of the EVM currently available is highlighted below (Table 3).
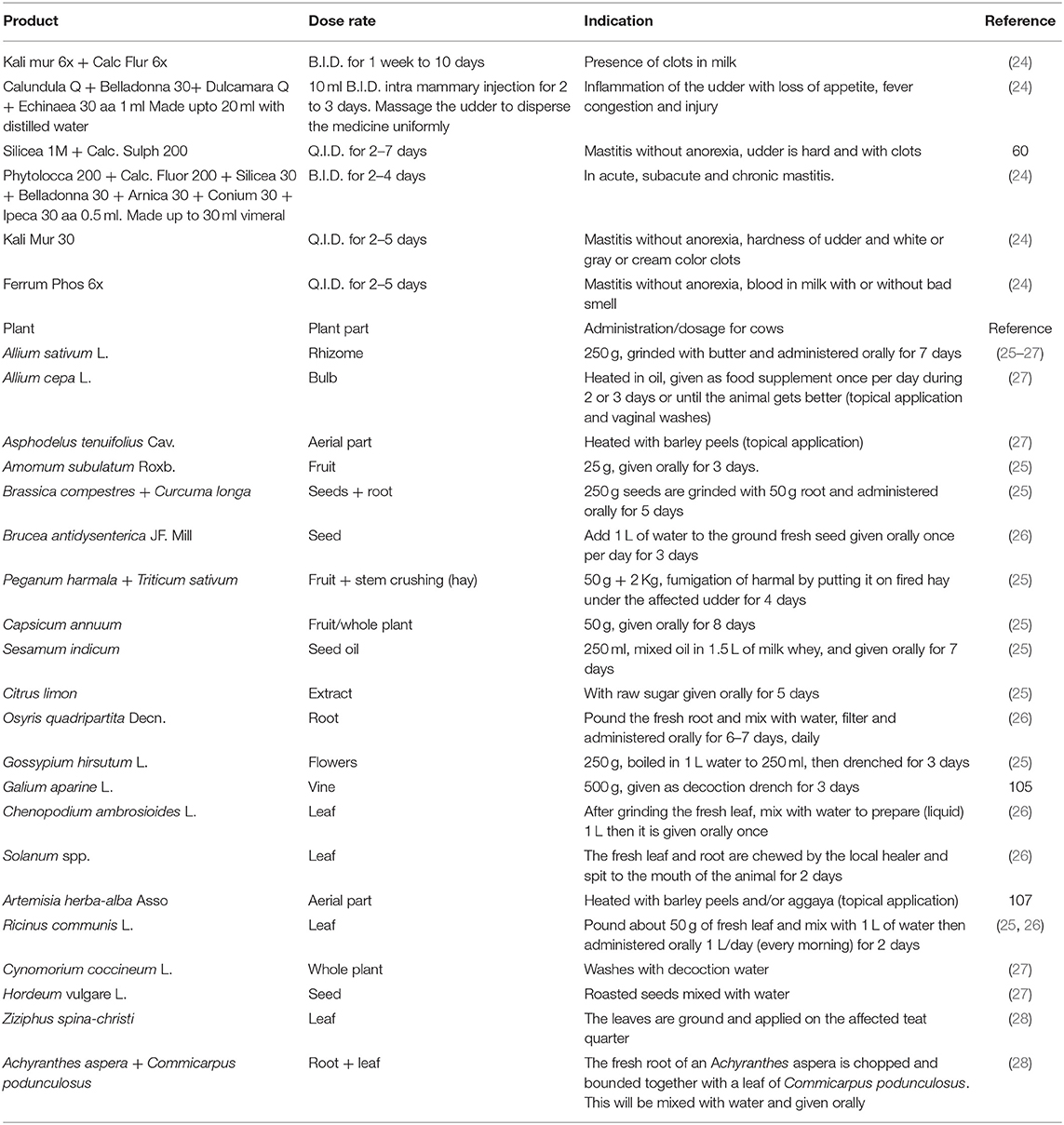
Table 3. Ethno-veterinary medicinal products, preparation and administration for the treatment and control of mastitis.
An herbal mixture of Aloe vera (L.) Burm. -F., Curcuma longa L. and calcium hydroxide, as documented by a traditional healer, was reported to be efficient against BM causing pathogens (110). Since the components of the mixture had a synergistic effect, their activity against the clinical mastitis pathogens was successful. The formulation was found to be antimicrobial and anti-inflammatory and to have wound cleansing and healing properties.
Natural Products (Plants): the Sleeping Giant of the Modern Pharmaceuticals
Globally, the plant domain renders a diversity of species utilized as treatments for various ailments (111). The World Health Organization (WHO) report states that traditional medicines, including those used for plant decoctions or functional compounds are used by a high percentage of the population of the world (112). An understanding of the plant parts used historically as treatments is the most essential requirement in the ethno-pharmacological approach (Figure 3). Chinese herbal medicine and Ayurveda are the prominent traditional medicines used in treating various diseases, but where no texts are available, the ethno-botanical survey is the only way in which to learn about the traditional uses of medicinal plants.
Natural products and/or organic by-product forms continue to play a pivotal role in the medication upshot process. As a result, biological diversity offers an endless supply of new chemical entities (NCEs) with their potential as therapeutic leads. These NCEs are derived productsgenerated by plants to shield them from herbivores and pathogens, or to attract pollinators (111).
The efficacy of secondary metabolites in plants is an indication of the strength of natural products as potent modern pharmaceuticals (113, 114). Researchers, including Smyth et al. (115) and Wu et al. (116), have issued reports on sample preparation and characterization. However, these reviews primarily focus on a chemosystematics-disposal method: the plant type chosen for testing is known to contain specific derivatives (amino acids, alkaloids and steroids); hence, the best extraction/purification/separation of these compounds is achieved by using the most appropriate extraction methodology and analytical technique (111, 117).
The antimicrobial peptides (AMPs) of plant origin are a source of biocontrol agents against bacteria, fungi, nematodes, insects, and pests (118). They are classified into different groups to include the type of charge, the disulfide bonds present, the cyclic structure, and the mechanism of action. The common types include cyclotide, defensins, hevein-like proteins, knotin-type proteins, lipid-transfer proteins, protease inhibitors, snakins, and thionins (119). Researchers have reported the isolation and identification of peptides, defensins, protease inhibitors, lectins, thionin-like peptides, and vicilin-like peptides from solanaceae (120, 121). Particularly peptides and peptide-rich extracts of plants have been found to promote antibacterial activity against various strains of bacteria (121). Although their mechanism of action remains unclear, their antibacterial activity could be attributed to changes in membrane permeability (119).
It is clear that peptide contact with the cell membrane induces changes in the structure and aggregation state of the peptides which are adapted by membrane lipids via changes in their lipid conformation and packing structure (122). Given that the outer membranes of Gram-negative bacteria or the cell walls of Gram-positive bacteria have negatively-charged surfaces, no fundamental difference was found in the way in which AMPs worked on them. Moreover, the cell wall of Gram-positive bacteria have pores (40 to 80 nm) through which multiple AMPs may readily pass to interact with the target location (123).
Characterization of Secondary Metabolites in Plants
Plants produce an extensive array of chemical molecules including those that are indirectly involved with development and growth and which are termed secondary metabolites (124). The polar difference of the fragment and the solvent utilized determine the extraction procedure for bioactive substances. More so, depending on the qualities of each biomolecule of interest, plant-derived particles from unrefined extracts can be additionally segregated, isolated, and refined using a mix of separation procedures and various approaches (125). Several of such techniques are presented below:
High Performance-Thin Layer Chromatography
HPTLC is a sophisticated form of TLC which yields superior separation efficiency results. For fingerprint analysis, TLC is the most frequent method. Capillary action pulls the energetic section into the immobilized part. The polarity of each component is used to distinguish samples. The HPTLC characterization is mostly carried out to investigate substances with small to medium polar differences. The approach is generally used in the process upshot, the observation of impurities in the herbal by-products, the identification of the pesticide, as well as its content, and in a standard check of herbal mixtures and wellness foods (126).
Unrefined extracts are used in conjunction with standard molecules, and software is available to assess the quantities of chemicals available in the test material (125).
High Performance Liquid Chromatography
Previously known as high pressure liquid chromatography, this is a more sophisticated method than HPTLC. A liquid sample is injected into a stream of solvent (mobile phase), and passes through a separation medium-filled column (stationary phase). As the sample components pass through the column, a process called differential migration separates them (127). The stationary phase usually involves a column filled with minute porous matter, with the liquid vigorous part being injected via the column. Currently, the most effective technology is a merger of LC/MS and HPLC (128).
Ultra-Performance Liquid Chromatography
This is a comparably recent approach in liquid chromatography that opens up new possibilities, particularly in terms of time and solvent consumption. This technique takes total advantage of chromatographic principles to stimulate separations using columns chocked with smaller particles and/or higher flow rates for faster processing with extraordinary resolution and sensitivity (129). The UPLC is based on the premise of using a stationary phase with particles smaller than two (2) μm in diameter (129).
Liquid Chromatography-Mass Spectroscopy
LC–MS is an analytical chemistry approach that merges the actual dissociation potential of liquid separation (or HPLC) with the aggregate scrutiny potentials of mass spectrometry (MS). The advantages of LC-MS are similar to those of GC-MS: excellent selectivity, as well as the capacity to work with complex compounds (125). The ability to multiplex LC-MS tests, allowing for the measurement of numerous medicines and metabolites in a single run, is a beneficial feature of these assays. When compared to GC-MS screening, the use of LC-tandem MS for toxicology screening is appealing since it has the ability to provide more confidence in the identification process and also to identify a wider spectrum of medicines, toxins, and their metabolites, and to simplify sample preparation (130).
Nuclear Magnetic Resonance
Nuclear Magnetic Resonance (NMR) is a nuclei (nuclear)-specific spectroscopy with numerous applications in the physical sciences and industry. The inherent angular momentum characteristics of atomic nuclei are investigated using nuclear magnetic resonance, which employs a massive magnet (magnetic). Similar to other spectroscopic techniques, NMR relies on ionizing emission elements (audio waves) to ease transference between degrees of atomic powers (131). NMR is mostly used to check for the structure of fragments. The combination of Mass Spectrometry, NMR and Liquid Chromatography (LC–NMR–MS) provides metabolic organizational information about innovative pharmaceuticals in production, as well as their regular uses (125). The speed and sensitivity of NMR detection have recently been improved, and have been proven to be effective in pharmacokinetics, drug metabolism and development and toxicity research (132).
Gas Chromatography-Mass Spectroscopy
GC-MS is frequently used to test the purity and stability of organic compounds, as well as to distinguish, both qualitatively and quantitatively, between the constituents of a mixture. The preparation of the sample, loading, and dissociation on a GC apparatus are the first steps in a GC-MS experiment. MS is the GC detector and it generates a chromatogram that indicates the amount of each chemical in relation to its retention duration (133). Its application is employed in a variety of spheres inclusive of environmental chemistry for studies on water, soil and the atmosphere, as well as in forensic science for drug detection (or metabolites) well as in forensic science for drug (134).
Infrared Spectroscopy
Infrared spectroscopy (IR spectroscopy or vibrational spectroscopy) studies the interplay between infrared radiation and different materials through absorption, emission, or reflection. It evaluates mixtures in various forms; solid, liquid, or gaseous. A sample's infrared spectrum is recorded by passing an infrared light beam through it. Absorption occurs when the IR frequency is similar to the frequency of vibration of (a) bond(s). The amount of energy absorbed at each frequency can be determined by examining the transmitted light (or wavelength). A monochromator can be used to scan the wavelength range and to make this measurement. Alternatively, a Fourier transform (FTIR) device can be used to measure the complete wavelength range, and then a dedicated process can be used to build a transmittance or absorbance spectrum. Other methods in molecular vibrational spectroscopy include Raman spectroscopy, high resolution electron energy loss spectroscopy (HREELS), and electron energy loss spectroscopy (EELS) (135).
Conclusion and Future Perspectives
Ethno-veterinary practices have been identified and evaluated as an upshot in lowering the use of antibiotics and other veterinary medicines in dairy farming. The application of people's intellects, skills, techniques, traditions and faith is the domain of ethno-veterinary medicine. Thus, these skills need to be properly documented as they are shared or transferred from age to age only through the oral medium. Given the increasing interest in herbal medicines, sustainability programmes should be put in place to avoid the threats to these plants and their possible extinction. Proper investigations involving in vivo studies into the aforementioned are critical to the success of the war being waged against antimicrobial resistance in the dairy industry. Hence, it is imperative that the ethno-pharmacology of medicinal plants should be exploited to optimize their valuable use as antibacterial, anti-inflammatory and immune-modulatory agents and antioxidants.
Author Contributions
CA: conceptualization and resources. DA, BO, TA, JF, KM, and CA: writing–review and editing. DA: writing–original draft preparation. CA, AA, and OF: supervision and editing. All authors contributed to the article and approved the submitted version.
Conflict of Interest
The authors declare that the research was conducted in the absence of any commercial or financial relationships that could be construed as a potential conflict of interest.
Publisher's Note
All claims expressed in this article are solely those of the authors and do not necessarily represent those of their affiliated organizations, or those of the publisher, the editors and the reviewers. Any product that may be evaluated in this article, or claim that may be made by its manufacturer, is not guaranteed or endorsed by the publisher.
Acknowledgments
The authors wish to appreciate the Department of Microbiology, School of Biological Sciences, North-West University, Mafikeng, South Africa. We are grateful to the North-West University and the Department of Microbiology, School of Biological Sciences for the financial support.
References
1. Dias RS, Eller MR, Duarte VS, Pereira ÂL, Silva CC, Mantovani HC, et al. Use of phages against antibiotic-resistant Staphylococcus aureus isolated from bovine mastitis. J Anim Sci. (2013) 91:3930–9. doi: 10.2527/jas.2012-5884
2. Angelopoulou A, Warda AK, Hill H, Ross RP. Nonantibiotic microbial solutions for bovine mastitis–live biotherapeutics, bacteriophage, phage lysins. Crit Rev Microbiol. (2019) 45:564–80. doi: 10.1080/1040841X.2019.1648381
3. Porter J, Anderson J, Carter L, Donjacour E, Paros M. In vitro evaluation of a novel bacteriophage cocktail as a preventative for bovine coliform mastitis. J Dairy Sci. (2016) 99:2053–62. doi: 10.3168/jds.2015-9748
4. Barbosa LN, de Almada AFB, Schmitz JA, Vechio MA, Bezerra K, dos Santos MC, et al. Bacteriophages' action against mastitis-causing bactéria. Res, Soc Dev. (2020) 9:e1849108541. doi: 10.33448/rsd-v9i10.8541
5. Zduńczyk S, Janowski T. Bacteriophages and associated endolysins in therapy and prevention of mastitis and metritis in cows: current knowledge. Anim Reprod Sci. (2020) 218:106504. doi: 10.1016/j.anireprosci.2020.106504
6. Sztachańska M, Barański W, Janowski T, Pogorzelska J, Zduńczyk S. Prevalence and etiological agents of subclinical mastitis at the end of lactation in nine dairy herds in North-East Poland. Pol J Vet Sci. (2016) 19:119–24. doi: 10.1515/pjvs-2016-0015
7. Vanderhaeghen W, Piepers S, Leroy F, Van Coillie E, Haesebrouck F, De Vliegher S. Identification, typing, ecology and epidemiology of coagulase negative staphylococci associated with ruminants. Vet J. (2015) 203:44–51. doi: 10.1016/j.tvjl.2014.11.001
8. Adkins PRF, Middleton JR. Methods for diagnosing mastitis. Vet Clin North Am Food Anim Pract. (2018) 34:479–91. doi: 10.1016/j.cvfa.2018.07.003
9. Giesecke WH, Van den Heever LW, Du Toit IJ. Bovine mastitis in Republic of South Africa. Bulletin de l'Office International des Epizooties. (1971) 76:621.
10. Mańombe E. Economic Value and Genetic Prediction of Clinical Mastitis in South African Holstein Cattle (MSc dissertation). Stellenbosch University, Cape Town, South Africa (2014).
11. Piepers S, de Vliegher S. Alternative approach to mastitis management–How to prevent and control mastitis without antibiotics? Braz J Vet Res Anim Sci. (2018) 55:e137149.e137125. doi: 10.11606/issn.1678-4456.bjvras.2018.137149
12. World Health Organization (WHO). Global action plan on antimicrobial resistance (2015). Available online at: http://www.who.int/drugresistance/globalaction_plan/en/ (accessed June 10, 2020).
13. Tang KL, Caffrey NP, Nóbrega DB, Cork SC, Ronksley PE, Barkema HW, et al. Restricting the use of antibiotics in food-producing animals and its associations with antibiotic resistance in food-producing animals and human beings: a systematic review and meta-analysis. Lancet Planet Health. (2017) 1:e316–27. doi: 10.1016/S2542-5196(17)30141-9
14. Abdullah SN, You KY, Hisham Khamis N, Chong CY. Modeling the dielectric properties of cow's raw milk under vat pasteurization. Prog Electromagn Res. (2019) 84:157–66. doi: 10.2528/PIERM19052202
15. Shoaib G, Shah GM, Shad N, Dogan Y, Siddique Z, Shah AH, et al. Traditional practices of the ethnoveterinary plants in the Kaghan Valley, Western Himalayas-Pakistan. Revista de Biología Tropical. (2021) 69:1–11. doi: 10.15517/rbt.v69i1.42021
16. Dinbiso TD, Tolosa TT, Begna FD. Ethnoveterinary practices of medicinal plants and non-plant remedies used in animal health management in Dawuro Zone, Southern Ethiopia. Geography. (2020) 2:1–31. doi: 10.21203/rs.3.rs-94568/v1
17. Ameen F, Reda SA, El-Shatoury SA, Riad EM, Enany ME, Alarfaj AA. Prevalence of antibiotic resistant mastitis pathogens in dairy cows in Egypt and potential biological control agents produced from plant endophytic actinobacteria. Saudi J Biol Sci. (2019) 26:1492–8. doi: 10.1016/j.sjbs.2019.09.008
18. Tilahun M, Etifu M, Shewage T. Plant diversity and ethnoveterinary practices of Ethiopia: a systematic review. Evid-based Complement Altern Med. (2019) 2019:1–9. doi: 10.1155/2019/5276824
19. Abidin SZU, Munem A, Khan R, Batiha GES, Amhad M, Zafar M, et al. Ethnoveterinary botanical survey of medicinal plants used in Pashto, Punjabi and Saraiki communities of Southwest Pakistan. Vet Med Sci. (2021) 7:2068–85. doi: 10.1002/vms3.582
20. Bhatt PR, Patel UD, Patel HB, Modi CM. Survey on ethnoveterinary practices around Junagadh, Gujarat, India. Indian J Pharm Sci. (2019) 81:161–7. doi: 10.4172/pharmaceutical-sciences.1000493
21. Özen R, Dogan G. Herbal medicine raw materials used as part of the veterinary medical folklore in the Elazig Province and its vicinity. Mersin Üniversitesi Tip Fakültesi Lokman Hekim Tip Tarihi ve Folklorik Tip Dergisi. (2017) 7:166–77.
22. Adeniran LA, Okpi S, Anjorin TS, Ajagbonna OP. Medicinal plants used in ethnoveterinary practices in the Federal Capital Territory, North-Central Nigeria. J Med Plant Res. (2020) 14:377–88. doi: 10.5897/JMPR2020.6975
23. Abbasi AM, Khan MA, Shah MH, Shah MM, Pervez A, Ahmad M. Ethnobotanical appraisal and cultural values of medicinally important wild edible vegetables of Lesser Himalayas-Pakistan. J Ethnobiol Ethnomed. (2013) 9:1–13. doi: 10.1186/1746-4269-9-66
24. Srivastava A. Application of Ethnoveterinary practices and Veterinary Homeopathy in treatment of mastitis in Dairy cattle (2021). Available online at: https://www.pashudhanpraharee.com/application-of-ethnoveterinary-practices-and-veterinary-homeopathy-veterinary-ayurveda-in-treatment-of-mastitis-in-dairy-cattle/ (accessed December 21, 2021).
25. Dilshad SR, Rehman NU, Ahmad N, Iqbal A. Documentation of ethnoveterinary practices for mastitis in dairy animals in Pakistan. Pak Vet J. (2010) 30:167–71.
26. Eshetu GR, Dejene TA, Telila LB, Bekele DF. Ethnoveterinary medicinal plants: preparation and application methods by traditional healers in selected districts of southern Ethiopia. Vet World. (2015) 8:674. doi: 10.14202/vetworld.2015.674-684
27. Volpato G, Saleh SML, Di Nardo A. Ethnoveterinary of Sahrawi pastoralists of Western Sahara: camel diseases and remedies. J Ethnobiol Ethnomedicine. (2015) 11:1–22. doi: 10.1186/s13002-015-0040-4
28. Kalayou S, Haileselassie M, Gebre-Egziabher G, Tiku'e T, Sahle S, Taddele H, et al. In–vitro antimicrobial activity screening of some ethnoveterinary medicinal plants traditionally used against mastitis, wound and gastrointestinal tract complication in Tigray Region, Ethiopia. Asian Pac J Trop Biomed. (2012) 2:516–22. doi: 10.1016/S2221-1691(12)60088-4
29. Doehring C, Sundrum A. The informative value of an overview on antibiotic consumption, treatment efficacy and cost of clinical mastitis at farm level. Prev Vet Med. (2019) 165:63–70. doi: 10.1016/j.prevetmed.2019.02.004
30. Amuta PO, Okolocha EC, Kudi CA, Gates MC. Assessing the perceptions and practices of peri-urban dairy farmers regarding bovine mastitis management in North-Western Nigeria. Prev Vet Med. (2021) 194:105442. doi: 10.1016/j.prevetmed.2021.105442
31. Beyene B, Tolosa T. Epidemiology and financial impact of bovine mastitis in an animal production and research center and smallholder dairy farms in Horo Guduru Wollega Zone, Western Ethiopia. J Dairy Vet Anim Res. (2017) 5:144–51. doi: 10.15406/jdvar.2017.05.00152
32. Romero J, Benavides E, Meza C. Assessing financial impacts of subclinical mastitis on colombian dairy farms. Front Vet Sci. (2018) 5:273. doi: 10.3389/fvets.2018.00273
33. Sinha MK, Thombare N, Mondal B. Subclinical mastitis in dairy animals: incidence, economics, and predisposing factors. Scient World J. (2014). doi: 10.1155/2014/523984
34. Khan M, Khan A. Basic facts of mastitis in dairy animals: A review. Pakistan Veter J. (2006) 26:204.
35. Seegers H, Fourichon C, Beaudeau F. Production effects related to mastitis and mastitis economics in dairy cattle herds. Veter Res. (2003) 34:475–91. doi: 10.1051/vetres:2003027
36. Tekle Y, Berihe T. Bovine mastitis: prevalence, risk factors and major pathogens in the Sidamo Zone SNNPRS, Ethiopia. Eur J Bio Med Sci Res. (2016) 4:27–43.
37. Abebe R, Hatiya H, Abera M, Megersa B, Asmare K. Bovine mastitis: prevalence, risk factors and isolation of Staphylococcus aureus in dairy herds at Hawassa milk shed, South Ethiopia. BMC Vet Res. (2016) 12:270. doi: 10.1186/s12917-016-0905-3
38. Motaung TE, Petrovski KR, Petzer I, Thekisoe O, Tsilo T. Importance of bovine mastitis in Africa: review. Anim Health Res Rev. (2017) 18:58–69. doi: 10.1017/S1466252317000032
39. Food and Agriculture Organization Food. Impact of mastitis in small scale dairy production systems. Animal Production and Health Working Paper No. 13. Rome (2014).
40. Sharma N, Rho JG, Hong YH, Kang TY, Lee HK, Yur TY, et al. Bovine mastitis: an Asian perspective. Asian J Anim Vet Adv. (2012) 7:454–76. doi: 10.3923/ajava.2012.454.476
41. Kvapilik J, Hanus O, Syrucek J, Vyletelova-Klimesova M, Roubal R. The economic importance of the losses of cow milk due to mastitis: a meta-analysis. Bul J Agric Sci. (2014) 20:1483–97.
42. Xiu L, Fu YB, Deng Y, Shi XJ, Bian ZY, Ruhan A, et al. Deep sequencing-based analysis of gene expression in bovine mammary epithelial cells after Staphylococcus aureus, Escherichia coli, and Klebsiella pneumoniae infection. Genet Mol Res. (2015) 14:16948–65. doi: 10.4238/2015.December.15.1
43. Cheng J, Zhang J, Han B, Barkema HW, Cobo ER, Kastelic JP, et al. Klebsiella pneumoniae isolated from bovine mastitis is cytopathogenic for bovine mammary epithelial cells. J Dairy Sci. (2020) 103:3493–504. doi: 10.3168/jds.2019-17458
44. Liu G, Liu Y, Ali T, Ferreri M, Gao J, Chen W, et al. Molecular and phenotypic characterization of aerococcus viridans associated with subclinical bovine mastitis. PLoS ONE. (2015) 10:e0125001. doi: 10.1371/journal.pone.0125001
45. Gao J, Li S, Zhang J, Zhou Y, Xu S, Barkema HW, et al. Prevalence of potential virulence genes in Klebsiella spp. isolated from cows with clinical mastitis on large Chinese dairy farms. Foodborne Pathog Dis. (2019) 16:856–63. doi: 10.1089/fpd.2019.2657
46. Hettinga K. Detection of mastitis pathogens by analysis of volatile bacterial metabolites. J Dairy Sci. (2008) 91:3834–9. doi: 10.3168/jds.2007-0941
47. Hota A, Jambagi K, Swain S. Bovine Mastitis: Pathogenesis and susceptibility. Agro Economist-An International Journal. (2020) 7:107–10.
48. Treece JM, Morse GE, Levy C. Lipid analysis of bovine teat canal keratin. J Dairy Sci. (1966) 49:1240. doi: 10.3168/jds.S0022-0302(66)88062-1
49. Capuco AV, Bright SA, Pankey JW, Wood DL, Miller RH, Bitman J. Increased susceptibility to intramammary infection following removal of teat canal keratin. J Dairy Sci. (1992) 75:2126. doi: 10.3168/jds.S0022-0302(92)77972-7
50. Jones GM. Understanding the basics of mastitis. Virginia Cooperative Extension. Publication No. 404-233 (2006). Virginia State University, USA. p: 1–7.
51. Krishnamoorthy K, Pazhamalai P, Mariappan VK, Manoharan S, Kesavan D, Kim SJ. Two-dimensional siloxene-graphene heterostructure-based high-performance supercapacitor for capturing regenerative braking energy in electric vehicles. Adv Func Mater. (2021) 31:2008422. doi: 10.1002/adfm.202008422
52. Fesseha H, Demlie MMT, Eshetu E. Effect of Lactobacillus species probiotics on growth performance of dual-purpose chicken. Veter Med: Res Rep. (2021) 12:75. doi: 10.2147/VMRR.S300881
53. Thompson-Crispi KA, Hine B, Quinton M, Miglior F, Mallard BA. Association of disease incidence and adaptive immune response in Holstein dairy cows. J Dairy Sci. (2012) 95:3888–93. doi: 10.3168/jds.2011-5201
54. Hussein HA, El-Razik K, Gomaa AM, Elbayoumy MK, Abdelrahman KA, Hosein HI. Milk amyloid A as a biomarker for diagnosis of subclinical mastitis in cattle. Veter World (2018) 11:34–41. doi: 10.14202/vetworld.2018.34-41
55. Rossi R, Amarante A, Correia L, Guerra S, Nobrega D, Latosinski G, et al. Diagnostic accuracy of Somaticell, California Mastitis Test, and microbiological examination of composite milk to detect Streptococcus agalactiae intramammary infections. J Dairy Sci. (2018) 101:10220–9. doi: 10.3168/jds.2018-14753
56. Mahmmod Y. The future of PCR technologies in diagnosis of bovine mastitis pathogens. Adv dairy Res. (2013) 2:e106. doi: 10.4172/2329-888X.1000e106
57. Bosward KL, House JK, Deveridge A, Mathews K, Sheehy PA. Development of a loop-mediated isothermal amplification assay for the detection of Streptococcus agalactiae in bovine milk. J Dairy Sci. (2016) 99:2142–50. doi: 10.3168/jds.2015-10073
58. Wu J, Li L, Sun Y, Huang S, Tang J, Yu P, Wang G. Altered molecular expression of the TLR4/NF-κB signaling pathway in mammary tissue of Chinese Holstein cattle with mastitis. PLoS ONE (2015) 10:e0118458. doi: 10.1371/journal.pone.0118458
59. Zhao Y, Liu H, Zhao X, Gao Y, Zhang M, Chen D. Prevalence and pathogens of subclinical mastitis in dairy goats in China. Trop Anim health Prod. (2015) 47:429–35. doi: 10.1007/s11250-014-0742-y
60. Kosciuczuk EM, Lisowski P, Jarczak J, Majewska A, Rzewuska M, Zwierzchowski L, et al. (2017). Transcriptome profiling of Staphylococci-infected cow mammary gland parenchyma. BMC Veter Res. (2017) 13:1–12. doi: 10.1186/s12917-017-1088-2
61. Ashraf A, Imran M. Diagnosis of bovine mastitis: from laboratory to farm. Trop Anim Health Prod. (2018) 50:1193–202. doi: 10.1007/s11250-018-1629-0
62. Souza FN, Cunha AF, Rosa DL, Brito MAV, Guimarães AS, Mendonça LC, et al. Somatic cell count and mastitis pathogen detection in composite and single or duplicate quarter milk samples. Pesquisa Veterinária Brasileira (2016) 36:811–8. doi: 10.1590/S0100-736X2016000900004
63. Ferronatto JA, Ferronatto TC, Schneider M, Pessoa LF, Blagitz MG, Heinemann MB, et al. Diagnosing mastitis in early lactation: use of Somaticell®, California mastitis test and somatic cell count. Ital J Anim Sci. (2018) 17:723–9. doi: 10.1080/1828051X.2018.1426394
64. Mira C, Libera A, Souza F, Lima S, Blagitz M. Milk cellularity in the diagnosis of intramammary infection in cattle. Revista de Ciências Agrárias/Amazonian J Agri Environ Sci. (2013) 56:7–11. doi: 10.4322/rca.2013.006
65. Rodrigues A, Cassoli L, Machado P, Ruegg P. Evaluation of an on-farm test to estimate somatic cell count. J Dairy Sci. (2009) 92:990–5. doi: 10.3168/jds.2008-1216
66. Kandeel S, Morin D, Calloway C, Constable P. Association of California mastitis test scores with intramammary infection status in lactating dairy cows admitted to a veterinary teaching hospital. J Veter Inter Med. (2018) 32:497–505. doi: 10.1111/jvim.14876
67. Godden S, Royster E, Timmerman J, Rapnicki P, Green H. Evaluation of an automated milk leukocyte differential test and the California Mastitis Test for detecting intramammary infection in early-and late-lactation quarters and cows. J Dairy Sci. (2017) 100:6527–44. doi: 10.3168/jds.2017-12548
68. Ryskaliyeva A, Henry C, Miranda G, Faye B, Konuspayeva G, Martin P. Combining different proteomic approaches to resolve complexity of the milk protein fraction of dromedary, Bactrian camels and hybrids, from different regions of Kazakhstan. PLoS ONE (2018) 13:e0197026. doi: 10.1371/journal.pone.0197026
69. Hettinga K, Van Valenberg H, De Vries S, Boeren S, Van Hooijdonk T, van Arendonk J, et al. (2011). The host defense proteome of human and bovine milk. PLoS ONE (2011) 6:e19433. doi: 10.1371/journal.pone.0019433
70. Váradi L, Luo JL, Hibbs DE, Perry JD, Anderson RJ, Orenga S, et al. Methods for the detection and identification of pathogenic bacteria: past, present, and future. Chem Soc Rev. (2017) 46:4818–32. doi: 10.1039/C6CS00693K
71. Paterson GK, Harrison EM, Holmes MA. The emergence of mecC methicillin-resistant Staphylococcus aureus. Trends Microbiol. (2014) 22:42–7. doi: 10.1016/j.tim.2013.11.003
72. Gordon NC, Price JR, Cole K, Everitt R, Morgan M, Finney J, et al. Prediction of Staphylococcus aureus antimicrobial resistance by whole-genome sequencing. J Clin Microbiol. (2014) 52:1182–91. doi: 10.1128/JCM.03117-13
73. Kumar A, Rahal A, Dwivedi SK, Gupta MK. Bacterial prevalence and antibiotic resistance profile from bovine mastitis in Mathura, India. Egypt J Dairy Sci. (2010) 38:31–4.
74. Deb R, Kumar A, Chakraborty S, Verma AK, Tiwari R, Dhama K, et al. Trends in diagnosis and control of bovine mastitis: a review. Pak J Biol Sci. (2013) 16:1653–61. doi: 10.3923/pjbs.2013.1653.1661
75. Krömker V, Leimbach S. Mastitis treatment—reduction in antibiotic usage in dairy cows. Reprod Domest Anim. (2017) 52:21–9. doi: 10.1111/rda.13032
76. Gomes F, Henriques M. Control of bovine mastitis: old and recent therapeutic approaches. Curr Microbiol. (2016) 72:377–82. doi: 10.1007/s00284-015-0958-8
77. Capuco AV, Akers RM, Smith JJ. Mammary growth in Holstein cows during the dry period: quantification of nucleic acids and histology. J Dairy Sci. (1997) 80:477–87. doi: 10.3168/jds.S0022-0302(97)75960-5
78. Kashif M, Rizwan M, Ali M, Ahmad T, Durrani AZ. Control of mastitis through dry cow therapy: a review. Veterinaria. (2016) 2:13–6.
79. Stevens M, Piepers S, De Vliegher S. Mastitis prevention and control practices and mastitis treatment strategies associated with the consumption of (critically important) antimicrobials on dairy herds in Flanders, Belgium. J Dairy Sci. (2016) 99:2896–903. doi: 10.3168/jds.2015-10496
80. Nader-Macías MEF, Bogni C, Sesma FJM, Espeche MC, Pellegrino M, Saavedra L, et al. Alternative approaches for the prevention of bovine mastitis. Probiotics, bioactive compounds and vaccines. Bioactives compounds. New York, NY, USA: Nova Science Publishers, Inc. (2011). p. 1–34.
81. Awandkar SP, Bhikane AU, Kulkarni MB. Antibiotic resistance trends in clinical bovine mastitis. Biolife. (2013) 1:139–43.
82. Holko I, Tančin V, Vršková M, TvaroŽková K. Prevalence and antimicrobial susceptibility of udder pathogens isolated from dairy cows in Slovakia. J Dairy Res. (2019) 86:436–9. doi: 10.1017/S0022029919000694
83. Dowling A, O'Dwyer J, Adley C. Antibiotics: mode of action and mechanisms of resistance. Antimicrobial research: Novel Bioknowledge and Educational Programs. (2017) 1:536–45.
84. Kapoor G, Saigal S, Elongavan A. Action and resistance mechanisms of antibiotics: a guide for clinicians. J Anaesthesiol Clin Pharmacol. (2017) 33:300–5. doi: 10.4103/joacp.JOACP_349_15
85. Harbottle H, Thakur S, Zhao S, White DG. Genetics of antimicrobial resistance. Anim Biotechnol. (2006) 17:111–24. doi: 10.1080/10495390600957092
86. Ibrahim RA, Cryer TL, Lafi SQ, Basha EA, Good L, Tarazi YH. Identification of Escherichia coli from broiler chickens in Jordan, their antimicrobial resistance, gene characterization and the associated risk factors. BMC Vet Res. (2019) 15:1–16. doi: 10.1186/s12917-019-1901-1
87. Tooke CL, Hinchliffe P, Bragginton EC, Colenso CK, Hirvonen V, Takebayashi Y, et al. β-Lactamases and β-lactamase inhibitors in the 21st Century. J Mol Biol. (2019) 431:3472–500. doi: 10.1016/j.jmb.2019.04.002
88. Correia S, Poeta P, Hébraud M, Capelo JL, Igrejas G. Mechanisms of quinolone action and resistance: where do we stand? J Med Microbiol. (2017) 66:551–9. doi: 10.1099/jmm.0.000475
89. Wendlandt S, Feßler AT, Monecke S, Ehricht R, Schwarz S, Kadlec K. The diversity of antimicrobial resistance genes among staphylococci of animal origin. Int J Med Microbiol. (2013) 303:338–49. doi: 10.1016/j.ijmm.2013.02.006
91. Islam S, Jalal S, Wretlind B. Expression of the MexXY efflux pump in amikacin-resistant isolates of Pseudomonas aeruginosa. Clin Microbiol Infect. (2004) 10:877–83. doi: 10.1111/j.1469-0691.2004.00991.x
92. Wachino JI, Arakawa Y. Exogenously acquired 16S rRNA methyltransferases found in aminoglycoside-resistant pathogenic gram-negative bacteria: an update. Drug Resist Updat. (2012) 15:133–48. doi: 10.1016/j.drup.2012.05.001
93. Nguyen F, Starosta AL, Arenz S, Sohmen D, Dönhöfer A, Wilson DN. Tetracycline antibiotics and resistance mechanisms. Biol Chem. (2014) 395:559–75. doi: 10.1515/hsz-2013-0292
94. Shin SW, Shin MK, Jung M, Belaynehe KM, Yoo HS. Prevalence of antimicrobial resistance and transfer of tetracycline resistance genes in Escherichia coli isolates from beef cattle. Appl Environ Microbiol. (2015) 81:5560–6. doi: 10.1128/AEM.01511-15
95. Sharma VK, Johnson N, Cizmas L, McDonald TJ, Kim H. A review of the influence of treatment strategies on antibiotic resistant bacteria and antibiotic resistance genes. Chemosphere. (2016) 150:702–14. doi: 10.1016/j.chemosphere.2015.12.084
96. Davis JL. Pharmacologic Principles. Equine Internal Medicine. (2018) 4:79–137. doi: 10.1016/B978-0-323-44329-6.00002-4
97. Jiang H, Cheng H, Liang Y, Yu S, Yu T, Fang J, et al. Diverse mobile genetic elements and conjugal transferability of sulfonamide resistance genes (sul1, sul2, and sul3) in Escherichia coli isolates from Penaeus vannamei and pork from large markets in Zhejiang, China. Front Microbiol. (2019) 10:1787. doi: 10.3389/fmicb.2019.01787
98. Awad A, Arafat N, Elhadidy M. Genetic elements associated with antimicrobial resistance among avian pathogenic Escherichia coli. Ann Clin Microbiol Antimicrob. (2016) 15:1–8. doi: 10.1186/s12941-016-0174-9
99. World Health Organisation (WHO). Antimicrobial resistance. Geneva (2018). Available online at: http://www.who.int/newsroom/fact-sheets/detail/antimicrobial-resistance
100. Kassa T, Wirtu G, Tegegne A. Survey of mastitis in dairy herds in the Ethiopian central highlands. SINET: Ethiopian J Sci. (1999) 22:291–301. doi: 10.4314/sinet.v22i2.18150
101. World Health Organization (WHO). The Medical Impact of Antimicrobial Use in Food Animals (1998). Available online at: https://apps.who.int/iris/bitstream/handle/10665/64439/WHO_EMC_ZOO_97.4.pdf (accessed January 10, 0.2020)
102. World Health Organization (WHO). WHO Guidelines on Use of Medically Important Antimicrobials in Food-producing Animals (2017). Available online at: https://apps.who.int/iris/bitstream/handle/10665/258970/9789241550130-eng.pdf (accessed January 10, 2020).
103. Maity S, Ambatipudi K. Mammary microbial dysbiosis leads to the zoonosis of bovine mastitis: a one-health perspective. FEMS Microbiology Ecology. (2021) 97:fiaa241. doi: 10.1093/femsec/fiaa241
104. McCorkle CM. Back to the future: Lessons from ethnoveterinary RDandE for studying and applying local knowledge. Agric Human Values. (1995) 12:52–80. doi: 10.1007/BF02217297
105. Maria VBM, Bevilaqua CML, Palha MDC, Braga RR, Schawanke K, Rodrigues ST, et al. Ethno veterinary knowledge of the inhabitants of Marajó Island, Eastern Amazonia. Brazil Acta Amazonica. (2011) 41:233–42. doi: 10.1590/S0044-59672011000200007
106. Hashemi SR, Davoodi H. Herbal plants as new immune-stimulator in poultry industry: a review. Asian J Anim Vet Adv. (2012) 7:105–16. doi: 10.3923/ajava.2012.105.116
107. Sserunkuma P, McGaw LJ, Nsahlai IV, Van Staden J. Selected southern African medicinal plants with low cytotoxicity and good activity against bovine mastitis pathogens. S Afr J Bot. (2017) 111:242–7. doi: 10.1016/j.sajb.2017.03.032
108. Das A, Guha C, Biswas U, Jana PS, Chatterjee A, Samanta I. Detection of emerging antibiotic resistance in bacteria isolated from subclinical mastitis in cattle in West Bengal. Vet World. (2017) 10:517–20. doi: 10.14202/vetworld.2017.517-520
109. Mushtaq S, Shah AM, Shah A, Lone SA, Hussain A, Hassan QP, et al. Bovine mastitis: an appraisal of its alternative herbal cure. Microb Pathog. (2018) 114:357–61. doi: 10.1016/j.micpath.2017.12.024
110. Balakrishnan NMN, Punniamurthy N, Mekala P, Ramakrishnan N, Kumar SK. Ethno-veterinary formulation for treatment of bovine mastitis. J Vet Sci. (2017) 18:377–82.
111. Brusotti G, Cesari I, Dentamaro A, Caccialanza G, Massolini G. Isolation and characterization of bioactive compounds from plant resources: the role of analysis in the ethnopharmacological approach. J Pharm Biomed Anal. (2014) 87:218–28. doi: 10.1016/j.jpba.2013.03.007
112. Ajose DJ, Okozi OM. Antibacterial activity of Ocimum gratissimum L. against some selected human bacterial pathogens. J Pharm Res Int. (2017) 20:1–8. doi: 10.9734/JPRI/2017/37897
113. de Andrade JP, Pigni NB, Torras-Claveria L, Berkov S, Codina C, Viladomat F, et al. Bioactive alkaloid extracts from Narcissus broussonetii: mass spectral studies. J Pharm Biomed Anal. (2012) 70:13–25. doi: 10.1016/j.jpba.2012.05.009
114. Jahn S, Seiwert B, Kretzing S, Abraham G, Regenthal R, Karst U. Metabolic studies of the Amaryllidaceous alkaloids galantamine and lycorine based on electrochemical simulation in addition to in vivo and in vitro models. Anal Chim Acta. (2012) 756:60–72. doi: 10.1016/j.aca.2012.10.042
115. Smyth WF, Smyth TPP, Ramachandran VN, Donnell FO, Brooks P. Dereplication of phytochemicals in plants by LC–ESI-MS and ESI-MSn, Trends Anal. Chem. (2012) 33:46–54. doi: 10.1016/j.trac.2011.09.015
116. Wu H, Guo J, Chen S, Liu X, Zhou Y, Zhang X, et al. Recent developments in qualitative and quantitative analysis of phytochemical constituents and their metabolites using liquid chromatography–mass spectrometry. J Pharm Biomed Anal. (2013) 72:267–91. doi: 10.1016/j.jpba.2012.09.004
117. Altemimi A, Lakhssassi N, Baharlouei A, Watson DG, Lightfoot DA. Phytochemicals: extraction, isolation, and identification of bioactive compounds from plant extracts. Plants. (2017) 6:42. doi: 10.3390/plants6040042
118. Nawrot R, Barylski J, Nowicki G, Broniarczyk J, Buchwald W, Gozdzicka-Józefiak A. Plant antimicrobial peptides. Folia Microbiol. (2014) 59:181–96. doi: 10.1007/s12223-013-0280-4
119. Campos ML, De Souza CM, De Oliveira KBS, Dias SC, Franco OL. The role of antimicrobial peptides in plant immunity. J Exp Bot. (2018) 69:4997–5011. doi: 10.1093/jxb/ery294
120. Dev SS, Venu AKHIL. Isolation and screening of antimicrobial peptides from Kanthari Mulaku (Capsicum frutescens). Int J Pharma Bio Sci. (2016) 7:174–9.
121. Afroz M, Akter S, Ahmed A, Rouf R, Shilpi JA, Tiralongo E, et al. Ethnobotany and antimicrobial peptides from plants of the solanaceae family: an update and future prospects. Front Pharmacol. (2020) 11:565. doi: 10.3389/fphar.2020.00565
122. Bechinger B, Gorr SU. Antimicrobial peptides: mechanisms of action and resistance. J Dent Res. (2017) 96:254–60. doi: 10.1177/0022034516679973
123. Malanovic N, Lohner K. Gram-positive bacterial cell envelopes: The impact on the activity of antimicrobial peptides. Biochim Biophys Acta. (2016) 1858:936–46. doi: 10.1016/j.bbamem.2015.11.004
124. Harborne JB. Classes and Functions of Secondary Products from Plants. In: Walton JN, Brown DE, editors. Chemicals from Plants—Perspectives on Plant Secondary Products. London, UK: Imperial College Press (1999). p. 1–25. doi: 10.1142/9789812817273_0001
125. Gupta N, Gudipati T, Prasad GBKS. Plant secondary metabolites of pharmacological significance in reference to diabetes mellitus: an update. Int J Curr Microbiol App Sci. (2018) 7:3409–48. doi: 10.20546/ijcmas.2018.705.398
127. Raja PMV, Barron AR. High Performance Liquid Chromatography. (2021). Available online at: https://chem.libretexts.org/@go/page/55862%20accessed%20July%2027 (accessed July 27, 2021).
128. Zhang Q, Ye M. Chemical analysis of the Chinese herbal medicine Gan-Cao (licorice). J Chromatogr A. (2009) 1216:1954–69. doi: 10.1016/j.chroma.2008.07.072
129. Reddy TS, Balammal G, Kumar A. Ultra performance liquid chromatography: an introduction and review. Int J Pharm Res Anal. (2012) 2:24–31.
130. Pitt JJ. Principles and applications of liquid chromatography-mass spectrometry in clinical biochemistry. Clin Biochem Rev. (2009) 30:19–34.
131. Kaseman D, Iye RSG. NMR: Introduction. Available online at: https://chem.libretexts.org/@go/page/1834 (accessed July 27, 2021).
132. Patil PS, Rajani S. An advancement of analytical techniques in herbal research. J Adv Sci Res. (2010) 1:8–14.
133. Lovestead TM, Urness KN. Gas Chromatography/Mass Spectrometry, Vol. 10. New York, NY: ASM Handbook (2019).
134. Kasthuri L, Bhagavannarayana G, Parthiban S, Ramasamy G, Muthu K, Meenakshisundaram S. Rare earth cerium doping effects in nonlinear optical materials: potassium hydrogen phthalate (KHP) and tris (thiourea) zinc (II) sulfate (ZTS). Cryst Eng Comm. (2010) 12:493–9. doi: 10.1039/B907513E
Keywords: mastitis, ethnobotany, antibiotics, milk output, infectious conditions
Citation: Ajose DJ, Oluwarinde BO, Abolarinwa TO, Fri J, Montso KP, Fayemi OE, Aremu AO and Ateba CN (2022) Combating Bovine Mastitis in the Dairy Sector in an Era of Antimicrobial Resistance: Ethno-veterinary Medicinal Option as a Viable Alternative Approach. Front. Vet. Sci. 9:800322. doi: 10.3389/fvets.2022.800322
Received: 22 October 2021; Accepted: 21 February 2022;
Published: 04 April 2022.
Edited by:
Nora Mestorino, National University of La Plata, ArgentinaReviewed by:
Mariana Florencia Lucas, Universidad del Salvador, ArgentinaTimothy Evans, University of Missouri, United States
Copyright © 2022 Ajose, Oluwarinde, Abolarinwa, Fri, Montso, Fayemi, Aremu and Ateba. This is an open-access article distributed under the terms of the Creative Commons Attribution License (CC BY). The use, distribution or reproduction in other forums is permitted, provided the original author(s) and the copyright owner(s) are credited and that the original publication in this journal is cited, in accordance with accepted academic practice. No use, distribution or reproduction is permitted which does not comply with these terms.
*Correspondence: Collins Njie Ateba, collins.ateba@nwu.ac.za