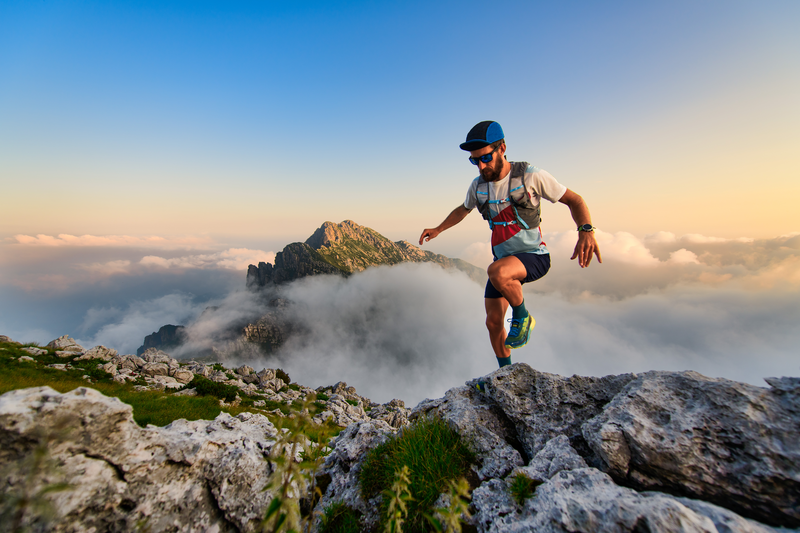
94% of researchers rate our articles as excellent or good
Learn more about the work of our research integrity team to safeguard the quality of each article we publish.
Find out more
ORIGINAL RESEARCH article
Front. Vet. Sci. , 23 February 2022
Sec. Veterinary Infectious Diseases
Volume 9 - 2022 | https://doi.org/10.3389/fvets.2022.799862
This article is part of the Research Topic Antimicrobial Use, Antimicrobial Resistance, and the Microbiome in Animals, volume II View all 13 articles
The occurrence of diarrhea in Tibetan piglets is highly notable, but the microorganisms responsible are yet unclear. Its high incidence results in serious economic losses for the Tibetan pig industry. Moreover, the dynamic balance of intestinal microflora plays a crucial role in maintaining host health, as it is a prime cause of diarrhea. Therefore, the present study was performed to analyze the characteristics of bacterial microbiota structure in healthy, diarrheal and treated weaned piglets in Tibet autonomous region for providing a theoretical basis to prevent and control diarrhea. The study was based on the V3–V4 region of the 16S rRNA gene and gut microbiota functions following the metagenome analysis of fresh fecal samples (n = 5) from different groups. The Shannon and Simpson indices differed substantially between diarrheal and treated groups (p < 0.05). According to our findings, the beta diversities, especially between healthy and diarrheal groups, were found different. Firmicutes, Bacteroidetes and Proteobacteria were the dominant phyla in three groups. Furthermore, the abundance of Fusobacteria in the diarrheal group was higher than the other groups. The dominant genera in the diarrheal group were Fusobacterium, Butyricimonas, Sutterella, Peptostreptococcus, and Pasteurella. Moreover, Lactobacillus, Megasphaera and Clavibacter were distinctly less abundant in this group. It is noteworthy that the specific decrease in the abundance of pathogenic bacteria after antibiotic treatment in piglets was noticed, while the level of Lactobacillus was evidently increased. In conclusion, fecal microbial composition and structure variations were discovered across the three groups. Also, the ecological balance of the intestinal microflora was disrupted in diarrheal piglets. It might be caused by a reduction in the relative number of beneficial bacteria and an increase in the abundance of pathogenic bacteria. In the context of advocating for non-resistant feeding, we suspect that the addition of probiotics to feed may prevent early-weaning diarrhea in piglets. Moreover, our findings might help for preventing diarrhea in weaned Tibetan piglets with a better understanding of microbial population dynamics.
The Tibetan pig is a valuable indigenous specie, as it is the only one that can survive in China's high altitude. Tibetan pigs are mainly found in semi-grassland and semi-farmland regions of Tibet (1). Under long-term harsh environmental conditions, Tibetan pigs have developed resistance against cold and diseases by developing different characteristics. These characteristics have made them indispensable for pig production in the plateau (2).
Early weaning is often used in intensive pig production, both at home and on farms (3). Meanwhile, weaning is an important turning point for piglet's growth to reduce the rate of vertical disease transmission and helps in the overall improvement of a pig farm. Conversely, earlier weaning caused psychological, environmental and nutritional stress in piglets, which induced diarrhea, dystrophia and slow growth (4) resulting in significant economic losses for the pig industry. Studies have argued that the diarrhea of weaned piglets is caused by infection with multiple pathogenic factors (bacteria, virus, etc.) and the intestinal dysfunction of piglets (5). Also, the imperfect immune system of the piglets, environmental changes, dietary changes, and improper feeding methods are conducive to the invasion of pathogenic strains, e.g., Escherichia coli (6).
The dynamic balance of intestinal microbiota plays an important role in the immune regulation of animals (7). Normal intestinal microbiota can stimulate the animal intestinal immune system by improving the intestinal self-recognition and immune ability of different bacteria. The intestine also serves as a barrier that can reduce the probability of host infection. Furthermore, weaning stress has disrupted the natural gut balance, reducing helpful microorganisms (8).
Due to the harsh cultural environment and intensive breeding strategies, Tibetan piglets frequently suffer from diarrhea after weaning. These factors are significantly decreasing the production performance and economics. The present study was performed to analyze the microbial diversity of different bacterial strains in healthy and diarrheal Tibetan piglets in Nyingchi, Tibet autonomous region, to investigate the etiology of diarrhea and develop a theoretical framework for it's prevention and treatment.
The experimental animals for this study were taken from five healthy sows (The sows were raised at Tibetan Pig Collaborative Research Center of Tibet Agriculture and Animal Husbandry University) maintained at similar conditions. The sows gave birth on the same day. Tibetan piglets and sows were bred together in a farrowing house (the temperature of the farrowing house was ~21°C, and the farrowing bed was strictly cleaned and disinfected). The feed was given to the piglets when they were 3 weeks old. At the age of 6 weeks, healthy Tibetan piglets were weaned and transferred to the nursery house (the temperature of the nursery house was 16°C).
Hermann-Bank (9) test criteria were used to determine healthy and diarrheal Tibetan piglets. The feces of healthy Tibetan piglets (piglets without any clinical symptoms) were granular or stripe-shaped for more than 2 days. While the feces of diarrheal piglets were thin and unformed for more than 2 days. The fecal samples of healthy and diarrheal piglets (the diarrheal early-weaned Tibetan piglets not birthed by the same sow) were simultaneously collected. All samples were transferred from the ranch to the laboratory using a vehicle-mounted refrigerator (−15°C). Then the samples were stored at −20°C for further evaluation. The marked diarrheal Tibetan piglets were treated with 1 mL of Gentamycin (4%) sulfate through intra muscular route (HuaXu Company, China, Product number: 17925752842). Five fecal samples from healthy piglets (group A; marked as A1, A2, A3, A4, and A5), five fecal samples from diarrheal piglets (group B; marked as B1, B2, B3, B4, and B5), and five fecal samples from post-treatment piglets (group C; marked as C1, C2, C3, C4, and C5) were selected.
The microbial DNA was extracted from 15 fecal samples of piglets using QIAamp Fast DNA Stool Mini Kit (QIAGEN, Hilden, Germany) as per the manufacturer's recommendations. The concentration and quality of DNA were detected with a nucleic acid detector (Nanodrop, Thermo Scientific NC2000, USA) and 1.2% agarose gel electrophoresis, respectively.
The standard bacteria V3–V4 hypervariable region gene PCR primers (forward primer: ACT CCT ACG GGA GGC AGC A; reverse primer: GGA CTA CHV GGG TWT CTA AT) were used. AxyPrep DNA Gel Extraction Kit (Axygen, CA, USA) and the 2% agarose gel electrophoresis were used for target fragment recovery and evaluation of PCR amplification product. Quant-iT PicoGreen dsDNA Assay Kit (Invitrogen, Waltham, Massachusetts, USA) was used to detect the recovered PCR products. Moreover, TruSeq Nano DNA Low Throughput Library Prep Kit (Illumina, CA, USA) was implied for sequence library construction. Amplified products' sequence ends were repaired by End Repair Mix2. PCR amplification was carried out to enrich the sequencing library template, and the library enrichment product was purified again via BECKMAN AMPure XP Beads. The library's final fragment-selection and purification were performed using 2% agarose gel electrophoresis.
The quality of libraries was examined on Agilent Bioanalyzer using Agilent High Sensitivity DNA Kit before sequencing procedure. The libraries with only one peak signal and no linker signal were considered for the process. Moreover, the libraries were quantified using Quant-iT PicoGreen dsDNA Assay Kit on Promega QuantiFluor fluorescence quantification system. The qualified library concentration was more than 2 nM. These qualified libraries were gradient diluted and mixed in proportion according to required sequencing. The MiSeq Reagent Kit V3 (600 cycles) was used to perform 2 × 300 bp paired-end sequencing on the MiSeq sequencing machine after the mixed libraries were denatured into single strands by sodium hydroxide.
Sequences analysis was established as operational taxonomic units (OTUs) via Uclust with over 97% similarity (10). The highest abundant sequence in each OUT was selected as the representative sequence (11). Then, OTUs were taxonomically classified and grouped by comparing with those in the Unite database (12). Micro microflora's richness and evenness index was calculated using the measurement indexes (Chao1, ACE, Shannon, and Simpson). Beta diversity based on the weighted UniFrac distance matrices were calculated with QIIME (Version 1.7.0), while the Cluster analysis was preceded by principal coordinate analysis (PCA) (13). The metastatic statistical algorithm was used to analyze the discrepancy in microbial communities between groups at the phylum and genus levels (14). The heat map was created via R software (v3.0.3), and all the data were evaluated statistically by one-way analysis of variance through SPSS 20.0 software (SPSS Inc., Chicago, Illinois 60606-6307, USA).
The current study subjected 15 fecal samples collected from Tibetan piglets to the high-throughput sequencing analysis. After optimizing the preliminary data, a total of 413,584, 427,913, and 408,109 high-quality valid sequences were obtained from the A, B and C groups, respectively. As shown in the dilution curve of species observation index, with the deepening test depth, its slope gradually decreased and reached the plateau stage. This finding indicated that the sequencing quantity of the samples was saturated, and the majority of bacteria were covered (Supplementary Figure 1). The sequences were established at the phylum, class, order, family, genus and species levels as OTUs via Uclust with over 97% similarity (Supplementary Figure 1). The three groups shared 1,315 bacteria species, as found by Venn map/diagram analysis (Figure 1). The diarrheal piglets showed 1,529 common bacteria species, which were not found in the healthy and antimicrobial-treated piglets. A total of 2,209 bacteria species were found to be common among the healthy piglets.
Figure 1. The fecal microflora of weaned piglets, analysed by a Venn diagram. (A) Healthy piglets; (B) Diarrheal piglets; (C) Treated piglets.
The alpha diversity of fecal microbiota was evaluated by using Chao1, ACE, Shannon and Simpson. The Simpson and Shannon index demonstrated that there was no striking difference in the micro microflora abundance between group A (0.887, 5.962) and B (0.834, 4.97) (p > 0.05) (Figure 2), whereas C (0.945, 6.582) group was significantly higher than that of the B group (p < 0.05) (Figure 2). The ACE and Chao1 indices showed that A group and C group had higher richness than B group, whereas no striking difference in the microflora richness was noticed among the three groups (p > 0.05) (Figure 2). Specifically, The Chao1 index amounted to 1,625.74, 1,427.63, and 1,899.73 in groups A, B, and C, while the ACE index reached 1,721.43, 1,504.51, and 1,972.87 in groups A, B, and C, respectively. However, significant differences were found in the microbial community structure by principal component analysis (PCA) in different groups, especially among healthy piglets, as compared with other two groups (Figure 3).
Figure 2. Diversity indices of the fecal microbiota in different Tibetan piglets. Chao1, ACE, Shannon, and Simpson indices were used to evaluate the alpha diversity of the fecal microbiota. The results were evaluated through one-way ANOVA. All of the data represent means ± SD. *p < 0.05.
Figure 3. Principal component analysis of the fecal microbiota. PCA map based on Euclidean distance. Each point indicates one sample. The distance of the two points indicates the difference in fecal microbiota. A: Healthy piglets; B: Diarrheal piglets; C: Treated piglets.
The bacterial community in the three groups were assessed at different taxonomical levels. Firmicutes (75.28 ± 12.70% in group A, 62.78 ± 15.75% in group B, 72.16 ± 12.65% in group C) and Proteobacteria (10.36 ± 8.48% in group A, 13.76 ± 18.62% in group B, 13.66 ± 15.87% in group C) were dominant in all samples at the phylum level (Figure 4A). Other phyla, including Bacteroidetes and Actinobacteria, presented a lower abundance (<8% of all samples) (Figure 4A). Interestingly, Fusobacteria in group B (13.02 ± 8.82%) was higher as compared to group A (0.08 ± 0.13%) and group C (2.58 ± 4.16%). Peptostreptococcaceae (21.92 ± 22.13%), Enterobacteriaceae (11.32 ± 18.75%), Streptococcaceae (12.32 ± 19.45%), Collinsella (3.86 ± 7.75%), Dorea (2.26 ± 2.09%) were predominant in the B group, whereas Psychrobacter (4.30 ± 9.61%) and Clostridium (2.04 ± 2.34%) in the C group at the genus level (Figures 4C,D). In addtion, Lactobacillus (47.10 ± 15.31% in group A, 2.00 ± 0.78% in group B, 12.22 ± 2.18% in group C) and Akkermansia (4.38 ± 9.68% in A group) were predominant bacteria genera. The relative abundance of genera Prevotella, Roseburia and Bacteroides were <2% in all samples (Figures 4C,D).
Figure 4. Relative abundance of gut bacterial taxa among different groups. (A) Phylum level; (B) Family level; (C) Genus level; (D) Taxonomic assignment at genus level. A1–A5: Healthy piglets; B1–B5: Diarrheal piglets; C1–C5: Treatment piglets.
The relative abundance of Fusobacteria in group B was significantly higher as compared to group A (p < 0.01) and group C (p < 0.05) at the phylum level (Figure 5A). The abundance of Elusimicrobia (p < 0.05) in the C group was significantly higher than group A and group B (Figure 5A). Furthermore, Fusobacterium, Butyricimonas, Sutterella, Peptostreptococcus, Pasteurella and Veillonella were the most abundant genus in group B, which were significantly higher than in other groups (p < 0.05) (Figure 5B). In contrast, Megasphaera and Clavibacter were less abundant in diarrheal piglets than healthy piglets (p < 0.05) (Figure 5B). Lactobacillus in the A group was significantly higher (p < 0.01) than in the B and C groups, whereas the abundance in group C was also significantly higher than that in group B (p < 0.01) (Figure 5B). Moreover, the relative abundance of Klebsiella, Bilophila, Roseburia, 1–68, Clostridium, Sutterella and Tissierella_Soehngenia in group C (p < 0.05) were significantly higher than in group A at the genus level (Figure 5B).
Figure 5. The metastatic composition of microbial diversity. (A) Microbial diversity at phylum level, (B) microbial diversity at genus level. A: Healthy piglets; B: Diarrheal piglets; C: Treated piglets.
We also performed Linear discriminant analysis effect size (LEfSe) tests to compare further intestinal microflora differences among the three groups (Figure 6). When comparing different Tibetan piglets, we found 4, 7 and 11 bacterial taxa that were abundant in healthy, diarrheal, and treated piglets. Furthermore, healthy piglets had the most enriched phylotypes from the phylum Lactobacillus, whereas diarrheal piglets had the most Sutterella, Fusobacterium, and Pasteurella phlylotype.
Figure 6. Linear discriminant analysis effect size (LEfSe) analysis of fecal microbiota composition in different groups of Tibetan piglets. (A) Histogram of the Linear Discriminant Analysis (LDA) scores computed for bacterial taxa differentially abundant among different groups. (B) A cladogram showing statistically and physiologically consistent distinctions among different groups. A: Healthy piglets; B: Diarrheal piglets; C: Treated piglets.
Piglet diarrhea is a common issue throughout the pig breeding process. The reasons for piglet diarrhea are quite a lot, such as weaning, nutritional, environmental and physiological stress on piglets (15). In addition, pathogenic bacteria, stress, management factors and excessive feed intake are also associated with piglet diarrhea (16). Moreover, intestinal epithelial mucosal barrier is the first line of defense that animals use to resist in such adverse conditions as it plays an important role in animals' normal intestinal functioning. Therefore, the changes in intestinal microbiota diversity would affect the intestinal function and cause diseases. This study evaluated the fecal microflora structure in healthy, diarrheal and treated piglets of Tibet autonomous region. The findings showed that diarrhea altered the bacterial microbiota structure of Tibetan pigs and impacted the variety of fecal microflora. There were a variety of bacteria in the feces of the Tibetan piglets. By Venn diagram analysis, 1,529 bacterial species were shared among the diarrheal piglets, which were not found in the healthy and treated piglets. Whereas, 2,209 bacterial species were found in the healthy group. PCA analysis showed a significant difference in bacterial community structure among the three groups, especially between healthy and diarrheal piglet groups based on Euclidean distance.
Generally, species are phylogenetically affiliated to phyla Firmicutes, Proteobacteria, and Bacteroidetes, which are abundant in Large White and Chinese Shanxi Black pigs (17). Our results indicated that Firmicutes, Bacteroidetes and Proteobacteria were the most dominant phyla in three groups of Tibetan piglets, which were consistent with previous observations in pig (18), bovine (19), sheep (20) and yak (21). Actinobacteria were mainly distributed in the stomach of herbivores, and they promote fiber decomposition and help in the digestive function of these animals (22). It is noteworthy that Actinobacteria was dominant phylum in Tibetan piglets, which was identified with predecessor's research in wild pigs (23). This phenomenon may be related to the herbivorous nature of Tibetan piglets. Some studies suggest that the abundance of Fusobacteria (24) and Fusobacteria phyla activate host inflammatory responses in order to protect against pathogens that promote tumor growth (25). Remarkably, the higher abundance of Fusobacteria in the fecal microbiota of diarrheal piglets may induce an immune response and increase the risk of pathogen infection of the host. Our results manifested that the Elusimicrobia level in the C group showed an upward trend as compared to the A and B groups, while it was known as an enigmatic bacterial phylum previously. The first representatives were termite gut-associated (26) isolated from the rumen (27) and the environment (28), that comprised of Planctomycetes, Verrucomicrobia, Chlamydia, Omnitrophica, Desantisbacteria (29), Kiritimatiellaeota (30) and Lentisphaerae (31). Cultivation and genome-based studies revealed that some species belonging to Elusimicrobia that are capable of glucose fermentation (32) with the ability to fix nitrogen (28).
Fusobacterium is being unveiled pathogen of gastrointestinal disorders. Previous research indicated that Fusobacterium plays a role in the pathogenesis of ulcerative colitis (33) and exert potentially carcinogenic (colorectal cancer) effects on the host (34). Butyricimonas bacteraemia has been described in patients with colon cancer (35) and patients with posttraumatic chronic bone and joint infections (36). It was isolated from a stool sample of a morbidly obese French patient living in Marseille, using the culturomics approach, which is critical to deciphering the links among gut microbiota and obesity (37). Recent reports link Sutterella with gastrointestinal diseases to induce substantial inflammation; rather, it can degrade IgA (38). Peptostreptococcus promotes colorectal carcinogenesis and modulates tumor immunity (39).
On the contrary, indole acrylic acid produced by commensal Peptostreptococcus species suppresses inflammation (40). Nevertheless, we observed that Peptostreptococcus was significantly higher in the diarrheal Tibetan piglets than in the other two groups. Its exact mechanism in Tibetan pigs needs to be further studied. Pasteurella are one of the important pathogens that infect a wide range of animals, including swine atrophic rhinitis (41), porcine respiratory disease complex (42), bovine hemorrhagic septicemia (43, 44), avian cholera (45–47) and rabbit respiratory disease (48, 49). A specific decrease in the abundance of Lactobacillus in diarrheal Tibetan piglets increased after antibiotic treatment in Tibetan piglets. Lactobacillus has been widely recognized for its role in gut microbiota, metabolism, immunity, and health maintenance (50–52).
Additionally, Lactobacillus is widely used in animal production because of its antibacterial activity and various biological characteristics (53). Megasphaera is a lactate-utilizing bacterium whose ruminal abundance is significantly elevated during fat milk depression (54), producing several short-chain fatty acids (SCFAs). These SCFAs serve as an energy source for host animals and play an important role in gut health (55). The genus Clavibacter harbors economically important plant pathogens, infecting crops such as potato and tomato (56, 57). Thus, our results conveyed important information that the relative abundances of pathogenic bacteria (such as Fusobacterium, Butyricimonas, Sutterella, Peptostreptococcus, Pasteurella) increased in the diarrheal Tibetan piglets, which disrupted the normal dynamic balance of the intestinal microbiota and led to a competitive decrease in the abundance of beneficial bacteria (Lactobacillus, Megasphaera). This phenomenon may also be the main cause of diarrhea in weaned Tibetan piglets. In addition, the intestinal microbial structure was changed by antibiotic treatment in weaned Tibetan piglets. Moreover, abundance of Lactobacillus was also increased significantly after antibiotic treatment.
Overall, there were significant difference in gut microbial composition and structure among the groups. Hence, the current study suggested that the decreased relative abundance of beneficial bacteria and increased relative abundance of pathogenic bacteria might cause diarrhea in Tibetan piglets. Therefore, this study provides a better insight into microbial population structure in order to prevent diarrhea in weaned Tibetan piglets.
The datasets presented in this study can be found in online repositories. The names of the repository/repositories and accession number(s) can be found at: https://www.ncbi.nlm.nih.gov/, PRJNA739650.
Ethical review and approval were not required for the animal study because the present used only fecal samples of Tibetan piglets. Fresh feces were collected by the Animal Care Staff (keepers) during their routine cleaning of the enclosure or directly from the soil without influencing the animals.
QK: conceptualization and writing original draft. WZ and MA: methodology. ZS, ZT, and YX: formal analysis and investigation. MK: review and editing. JL and SL: supervision, technical assistance, and funding. All authors participated in the writing of the manuscript, read, and approved the final manuscript.
This study was supported by National Natural Science Foundation of China (31760673) and Tibet Autonomous Region Department and College Joint Foundation Project (XZ202101ZR0020G).
The authors declare that the research was conducted in the absence of any commercial or financial relationships that could be construed as a potential conflict of interest.
All claims expressed in this article are solely those of the authors and do not necessarily represent those of their affiliated organizations, or those of the publisher, the editors and the reviewers. Any product that may be evaluated in this article, or claim that may be made by its manufacturer, is not guaranteed or endorsed by the publisher.
The Supplementary Material for this article can be found online at: https://www.frontiersin.org/articles/10.3389/fvets.2022.799862/full#supplementary-material
1. Li K, Lan YF, Luo HQ, Shahzad M, Zhang H, Wang L, et al. Prevalence of three oesophagostomum spp. from Tibetan Pigs an alyzed by Genetic Markers of nad1, cox3 and ITS1. Acta Parasitol. (2017) 62:90–6. doi: 10.1515/ap-2017-0010
2. Huang YW, Meng XJ. Novel strategies and approaches to develop the next generation of vaccines against porcine reproductive and respiratory syndrome virus (PRRSV). Virus Res. (2010) 154:141–9. doi: 10.1016/j.virusres.2010.07.020
3. Campbell JM, Crenshaw JD, Polo J. The biological stress of early weaned piglets. J Ani Sci Biotechnol. (2013) 4:19. doi: 10.1186/2049-1891-4-19
4. Nabuurs MJ. Etiologische en pathogenetische aspecten van diarree bij biggen na het spenen [Etiological and pathogenetic aspects of diarrhea in piglets following weaning]. Tijdschrift Voor Dierg. (1991) 116:1175–82.
5. de la Fé Rodríguez PY, Martin LO, Muñoz EC, Imberechts H, Butaye P, Goddeeris BM, et al. Several enteropathogens are circulating in suckling and newly weaned piglets suffering from diarrhea in the province of Villa Clara, Cuba. Trop Ani Health Prod. (2013) 45:435–40. doi: 10.1007/s11250-012-0236-8
6. Pohl CS, Medland JE, Mackey E, Edwards LL, Bagley KD, DeWilde MP, et al. Early weaning stress induces chronic functional diarrhea, intestinal barrier defects, and increased mast cell activity in a porcine model of early life adversity. Neurogastroenterol Motility. (2017) 29:10.1111/nmo.13118. doi: 10.1111/nmo.13118
7. Yurist-Doutsch S, Arrieta MC, Vogt SL, Finlay BB. Gastrointestinal microbiota-mediated control of enteric pathogens. Ann Rev Genet. (2014) 48:361–82. doi: 10.1146/annurev-genet-120213-092421
8. Xiang Q, Wu X, Pan Y, Wang L, Cui C, Guo Y, et al. Early-life intervention using fecal microbiota combined with probiotics promotes gut microbiota maturation, regulates immune system development, and alleviates weaning stress in piglets. Int J Mol Sci. (2020) 21:503. doi: 10.3390/ijms21020503
9. Hermann-Bank ML, Skovgaard K, Stockmarr A, Strube ML, Larsen N, Kongsted H, et al. Characterization of the bacterial gut microbiota of piglets suffering from new neonatal porcine diarrhea. BMC Vet Res. (2015) 11:139. doi: 10.1186/s12917-015-0419-4
10. Bokulich NA, Subramanian S, Faith JJ, Gevers D, Gordon JI, Knight R, et al. Quality-filtering vastly improves diversity estimates from Illumina amplicon sequencing. Nat Methods. (2013) 10:57–9. doi: 10.1038/nmeth.2276
11. Caporaso JG, Kuczynski J, Stombaugh J, Bittinger K, Bushman FD, Costello EK, et al. QIIME allows analysis of high-throughput community sequencing data. Nat Methods. (2010) 7:335–6. doi: 10.1038/nmeth.f.303
12. Kõljalg U, Nilsson RH, Abarenkov K, Tedersoo L, Taylor AF, Bahram M, et al. Towards a unified paradigm for sequence-based identification of fungi. Mol Ecol. (2013) 22:5271–7. doi: 10.1111/mec.12481
13. Ramette A. Multivariate analyses in microbial ecology. FEMS Microbiol Ecol. (2007) 62:142–60. doi: 10.1111/j.1574-6941.2007.00375.x
14. White JR, Nagarajan N, Pop M. Statistical methods for detecting differentially abundant features in clinical metagenomic samples. PLoS Computat Biol. (2009) 5:e1000352. doi: 10.1371/journal.pcbi.1000352
15. Hampson D. Postweaning Escherichia Coli Diarrhea in Pigs. Wallinford: CAB International (1994). p. 171–91.
16. Laine TM, Lyytikäinen T, Yliaho M, Anttila M. Risk factors for post-weaning diarrhea on piglet producing farms in Finland. Acta Vet Scand. (2008) 50:21. doi: 10.1186/1751-0147-50-21
17. Gao P, Liu Y, Le B, Qin B, Liu M, Zhao Y, et al. A comparison of dynamic distributions of intestinal microbiota between large white and Chinese Shanxi black pigs. Arch Microbiol. (2019) 201:357–67. doi: 10.1007/s00203-019-01620-4
18. Ding X, Lan W, Liu G, Ni H, Gu JD. Exploring possible associations of the intestine bacterial microbiome with the pre-weaned weight gaining performance of piglets in intensive pig production. Sci Rep. (2019) 9:15534. doi: 10.1038/s41598-019-52045-4
19. Jami E, Israel A, Kotser A, Mizrahi I. Exploring the bovine rumen bacterial community from birth to adulthood. ISME J. (2013) 7:1069–79. doi: 10.1038/ismej.2013.2
20. Omoniyi LA, Jewell KA, Isah OA, Neumann AP, Onwuka CF, Onagbesan OM, et al. An analysis of the ruminal bacterial microbiota in West African Dwarf sheep fed grass- and tree-based diets. J Appl Microbiol. (2014) 116:1094–105. doi: 10.1111/jam.12450
21. Xin J, Chai Z, Zhang C, Zhang Q, Zhu Y, Cao H, et al. Comparing the microbial community in four stomach of dairy cattle, yellow cattle and three yak herds in qinghai-tibetan plateau. Front Microbiol. (2019) 10:1547. doi: 10.3389/fmicb.2019.01547
22. Costa MC, Stämpfli HR, Allen-Vercoe E, Weese JS. Development of the faecal microbiota in foals. Equine Vet J. (2016) 48:681–8. doi: 10.1111/evj.12532
23. Yang G, Shi C, Zhang S, Liu Y, Li Z, Gao F, et al. Characterization of the bacterial microbiota composition and evolution at different intestinal tract in wild pigs (Sus scrofa ussuricus). PeerJ. (2020) 8:e9124. doi: 10.7717/peerj.9124
24. Liu S, Zhao L, Zhai Z, Zhao W, Ding J, Dai R, et al. Porcine epidemic diarrhea virus infection induced the unbalance of gut microbiota in piglets. Curr Microbiol. (2015) 71:643–9. doi: 10.1007/s00284-015-0895-6
25. Kelly D, Yang L, Pei Z. Gut microbiota, fusobacteria, colorectal cancer. Diseases. (2018) 6:109. doi: 10.3390/diseases6040109
26. Ohkuma M, Kudo T. Phylogenetic diversity of the intestinal bacterial community in the termite reticulitermes speratus. Appl Environ Microbiol. (1996) 62:461–8. doi: 10.1128/aem.62.2.461-468.1996
27. Ohkuma M, Sato T, Noda S, Ui S, Kudo T, Hongoh Y. The candidate phylum 'Termite Group 1' of bacteria: phylogenetic diversity, distribution, and endosymbiont members of various gut flagellated protists. FEMS Microbiol Ecol. (2007) 60:467–476. doi: 10.1111/j.1574-6941.2007.00311.x
28. Méheust R, Castelle CJ, Matheus Carnevali PB, Farag IF, He C, Chen LX, et al. Groundwater Elusimicrobia are metabolically diverse compared to gut microbiome Elusimicrobia and some have a novel nitrogenase paralog. ISME J. (2020) 14:2907–22. doi: 10.1038/s41396-020-0716-1
29. Probst AJ, Castelle CJ, Singh A, Brown CT, Anantharaman K, Sharon I, et al. Genomic resolution of a cold subsurface aquifer community provides metabolic insights for novel microbes adapted to high CO2 concentrations. Environ Microbiol. (2017) 19:459–74. doi: 10.1111/1462-2920.13362
30. Spring S, Bunk B, Spröer C, Schumann P, Rohde M, Tindall BJ, et al. Characterization of the first cultured representative of verrucomicrobia subdivision 5 indicates the proposal of a novel phylum. ISME J. (2016) 10:2801–16. doi: 10.1038/ismej.2016.84
31. Cho JC, Vergin KL, Morris RM, Giovannoni SJ. Lentisphaera araneosa gen. nov., sp. nov, a transparent exopolymer producing marine bacterium, and the description of a novel bacterial phylum, Lentisphaerae. Environ Microbiol. (2004) 6:611–21. doi: 10.1111/j.1462-2920.2004.00614.x
32. Wang G, Huang S, Cai S, Yu H, Wang Y, Zeng X, et al. Lactobacillus reuteri ameliorates intestinal inflammation and modulates gut microbiota and metabolic disorders in dextran sulfate sodium-induced colitis in mice. Nutrients. (2020) 12:2298. doi: 10.3390/nu12082298
33. Li DH, Li ZP, Yan Z., hang, Zhou GZ, Ren RR, et al. Fecal fusobacterium nucleatum harbored virulence gene fadA are associated with ulcerative colitis and clinical outcomes. Microbial Pathog. (2021) 157:104964. doi: 10.1016/j.micpath.2021.104964
34. Allen-Vercoe E, Jobin C. Fusobacterium and enterobacteriaceae: important players for CRC? Immunol Lett. (2014) 162:54–61. doi: 10.1016/j.imlet.2014.05.014
35. Ulger Toprak N, Bozan T, Birkan Y, Isbir S, Soyletir G. Butyricimonas virosa: the first clinical case of bacteraemia. N Microbes N Infect. (2015) 4:7–8. doi: 10.1016/j.nmni.2014.12.004
36. Ferry T, Laurent F, Ragois P, Chidiac C, Lyon BJI, Study Group. Post-traumatic chronic bone and joint infection caused by Butyricimonas spp, and treated with high doses of ertapenem administered subcutaneously in a 30-year-old obese man. BMJ Case Rep. (2015) 2015:bcr2015212359. doi: 10.1136/bcr-2015-212359
37. Togo AH, Diop A, Dubourg G, Nguyen TT, Andrieu C, Caputo A, et al. Butyricimonas phoceensis sp. nov., a new anaerobic species isolated from the human gut microbiota of a French morbidly obese patient. N Micro N Infect. (2016) 14:38–48. doi: 10.1016/j.nmni.2016.07.010
38. Kaakoush NO. Sutterella species, IgA-degrading bacteria in ulcerative colitis. Trends Microbiol. (2020) 28:519–22. doi: 10.1016/j.tim.2020.02.018
39. Long X, Wong CC, Tong L, Chu E, Ho Szeto C, Go M, et al. Peptostreptococcus anaerobius promotes colorectal carcinogenesis and modulates tumor immunity. Nat Microbiol. (2019) 4:2319–30. doi: 10.1038/s41564-019-0541-3
40. Wlodarska M, Luo C, Kolde R, d'Hennezel E, Annand JW, Heim CE, et al. Indoleacrylic acid produced by commensal peptostreptococcus species suppresses inflammation. Cell Host Microbe. (2017) 22:25–37.e6. doi: 10.1016/j.chom.2017.06.007
41. Horiguchi Y. Swine atrophic rhinitis caused by pasteurella multocida toxin and bordetella dermonecrotic toxin. Curr Top Microbiol Immunol. (2012) 361:113–29. doi: 10.1007/82_2012_206
42. Schmidt C, Cibulski SP, Andrade CP, Teixeira TF, Varela AP, Scheffer CM, et al. Swine influenza virus and association with the porcine respiratory disease complex in pig farms in southern Brazil. Zoonoses Public Health. (2016) 63:234–40. doi: 10.1111/zph.12223
43. Cuevas I, Carbonero A, Cano D, Pacheco IL, Marín JC, Borge C. First outbreak of bovine haemorrhagic septicaemia caused by Pasteurella multocida type B in Spain - short communication. Acta Vet Hung. (2020) 68:8–11. doi: 10.1556/004.2020.00014
44. Verma R, Jaiswal TN. Haemorrhagic septicaemia vaccines. Vaccine. (1998) 16:1184–92. doi: 10.1016/S0264-410X(98)80118-7
45. Pedersen K, Dietz HH, Jørgensen JC, Christensen TK, Bregnballe T, Andersen TH. Pasteurella multocida from outbreaks of avian cholera in wild and captive birds in Denmark. J Wildlife Dis. (2003) 39:808–16. doi: 10.7589/0090-3558-39.4.808
46. Petruzzi B, Dalloul RA, LeRoith T, Evans NP, Pierson FW, Inzana TJ. Biofilm formation and avian immune response following experimental acute and chronic avian cholera due to Pasteurella multocida. Vet Microbiol. (2018) 222:114–23. doi: 10.1016/j.vetmic.2018.07.005
47. Botzler RG. Epizootiology of avian cholera in wildfowl. J Wildlife Dis. (1991) 27:367–95. doi: 10.7589/0090-3558-27.3.367
48. Wang J, Sang L, Sun S, Chen Y, Chen D, Xie X. Characterization of Pasteurella multocida isolated from dead rabbits with respiratory disease in Fujian, China. BMC Vet Res. (2019) 15:438. doi: 10.1186/s12917-019-2191-3
49. Soriano-Vargas E, Vega-Sánchez V, Zamora-Espinosa JL, Acosta-Dibarrat J, Aguilar-Romero F, Negrete-Abascal E. Identification of pasteurella multocida capsular types isolated from rabbits and other domestic animals in mexico with respiratory diseases. Trop Ani Health Prod. (2012) 44:935–7. doi: 10.1007/s11250-011-9995-x
50. Li K, Zhang L, Zhang H, Lei Z, Luo H, Mehmood K, et al. Epidemiological investigation and risk factors of echinococcus granulosus in yaks (Bos grunniens), tibetan pigs and tibetans on qinghai tibetan plateau. Acta Trop. (2017) 173:147–52. doi: 10.1016/j.actatropica.2017.06.019
51. Wang K, Nan X, Tong J, Zhao G, Jiang L, Xiong B. Steam explosion pretreatment changes ruminal fermentation in vitro of corn stover by shifting archaeal and bacterial community structure. Front Microbiol. (2020) 11:2027. doi: 10.3389/fmicb.2020.02027
52. Dong H, Liu B, Li A, Iqbal M, Mehmood K, Jamil T, et al. Microbiome analysis reveals the attenuation effect of lactobacillus from yaks on diarrhea via modulation of gut microbiota. Front Cell Infect Microbiol. (2021) 10:610781. doi: 10.3389/fcimb.2020.610781
53. Wang Y, Li A, Jiang X, Zhang H, Mehmood K, Zhang L, et al. Probiotic potential of leuconostoc pseudomesenteroides and lactobacillus strains isolated from yaks. Front Microbiol. (2018) 9:2987. doi: 10.3389/fmicb.2018.02987
54. Weimer PJ, Da Silva Cabral L, Cacite F. Effects of ruminal dosing of holstein cows with megasphaera elsdenii on milk fat production, ruminal chemistry, and bacterial strain persistence. J Dairy Sci. (2015) 98:8078–92. doi: 10.3168/jds.2015-9721
55. Yoshikawa S, Araoka R, Kajihara Y, Ito T, Miyamoto H, Kodama H. Valerate production by Megasphaera elsdenii isolated from pig feces. J Biosci Bioeng. (2018) 125:519–24. doi: 10.1016/j.jbiosc.2017.12.016
56. Załuga J, Stragier P, Baeyen S, Haegeman A, Van Vaerenbergh J, Maes M, et al. Comparative genome analysis of pathogenic and non-pathogenic clavibacter strains reveals adaptations to their lifestyle. BMC Geno. (2014) 15:392. doi: 10.1186/1471-2164-15-392
Keywords: microbial diversity, bacteria, 16S rRNA, Tibetan piglets, diarrhea
Citation: Kong Q, Zhang W, An M, Kulyar MF-e-A, Shang Z, Tan Z, Xu Y, Li J and Liu S (2022) Characterization of Bacterial Microbiota Composition in Healthy and Diarrheal Early-Weaned Tibetan Piglets. Front. Vet. Sci. 9:799862. doi: 10.3389/fvets.2022.799862
Received: 22 October 2021; Accepted: 10 January 2022;
Published: 23 February 2022.
Edited by:
Moussa Sory Diarra, Agriculture and Agri-Food Canada (AAFC), CanadaReviewed by:
Kun Li, Nanjing Agricultural University, ChinaCopyright © 2022 Kong, Zhang, An, Kulyar, Shang, Tan, Xu, Li and Liu. This is an open-access article distributed under the terms of the Creative Commons Attribution License (CC BY). The use, distribution or reproduction in other forums is permitted, provided the original author(s) and the copyright owner(s) are credited and that the original publication in this journal is cited, in accordance with accepted academic practice. No use, distribution or reproduction is permitted which does not comply with these terms.
*Correspondence: Qinghui Kong, NzcwMzM3MDExQHFxLmNvbQ==; Suozhu Liu, MzQ2MjQ5NjEwQHFxLmNvbQ==; Jiakui Li, bGlqazIxMEBzaW5hLmNvbQ==
†These authors have contributed equally to this work
Disclaimer: All claims expressed in this article are solely those of the authors and do not necessarily represent those of their affiliated organizations, or those of the publisher, the editors and the reviewers. Any product that may be evaluated in this article or claim that may be made by its manufacturer is not guaranteed or endorsed by the publisher.
Research integrity at Frontiers
Learn more about the work of our research integrity team to safeguard the quality of each article we publish.