Corrigendum: Prevalence of multidrug-resistant CTX-M extended spectrum beta-lactamase-producing Escherichia coli from different bovine faeces in China
- 1Lanzhou Institute of Husbandry and Pharmaceutical Sciences of Chinese Academy of Agricultural Science (CAAS), Lanzhou, China
- 2Key Laboratory of New Animal Drug Project of Gansu Province, Lanzhou, China
- 3Key Laboratory of Veterinary Pharmaceutical Development, Ministry of Agriculture, Lanzhou, China
- 4Shandong Institute of Animal Science and Veterinary Medicine, Jinan, China
- 5Gannan Tibetan Autonomous Prefecture Institute of Animal Husbandry Science, Gannan, China
CTX-M extended spectrum beta-lactamase-producing Escherichia coli cause severe health hazards in livestock breeding. To date, little is known about antibiotic resistance differences among bacterial isolates from yaks, cows, and beef cattle; therefore, the aims of this study were to analyse the prevalence of CTX-M-producing E. coli in yak, beef cattle, and dairy cattle feces from different provinces in China. A total of 790 fecal samples from yaks, beef cattle, and dairy cows were used. Among all the samples, 523 non duplicate E. coli isolates were identified, and 29.6% of samples harbored CTX-M producers. The results showed that these E. coli strains harbored 15 clusters of CTX-M genes: CTX-M-79, CTX-M-55, CTX-M-15, CTX-M-14, CTX-M-28, CTX-M-179, CTX-M-65, CTX-M-24, CTX-M-27, CTX-M-102, CTX-M-105, CTX-M-173, CTX-M-238, CTX-M-196, and CTX-M-10. The dominant resistance genes were CTX-M-15, CTX-M-14, and CTX-M-55. Moreover, the distribution of CTX-M genes was related to geographical region. Based on the above findings, we reasoned that bovines are potential reservoirs of antibiotic resistance, and this problem should be given adequate attention.
Introduction
Bacterial resistance has been reported worldwide and affects global public health. Among all kinds of bacterial resistance, the CTX-M extended spectrum beta-lactamase (ESBL) is the most dominant beta-lactam enzyme, and it is found mostly among the members of Enterobacteriaceae (1). In recent years, the CTX-M gene has been reported in different countries (2–5). The CTX-M β-lactamase family can be divided into five groups based on their amino acid identities (6): the CTX-M-1, CTX-M-2, CTX-M-8, CTX-M-9, and CTX-M-25 groups. Within each group, the differences in the sequence identities of the ESBLs are <10% (6, 7). The prevalence of ESBLs is variable in different geographical locations. In recent decades, the detection rate of ESBL Enterobacteriaceae has increased dramatically. Misuse of antibiotics in food animals may lead to the dissemination of resistance genes.
Escherichia coli is a normal commensal in animals, and the bovine manure is often used as a source of organic fertilizer for farmland. Organic manure treated in compost form is considered to have better biosecurity in organic farming than raw manure (8). Adequate temperatures are effective in reducing the number of harmful microorganisms that can survive (9). Sometimes inappropriate compost or raw manure discharged into the soil can lead to the drug-resistant bacteria entering the soil along with antibiotic residues (10, 11). Competition for nutrients from other microorganisms in the soil is a factor in the survival of E. coli (12). However, it has been documented that antibiotics found in feces are highly ecotoxic and can disrupt the balance of the ecosystem and inhibit biological treatment systems, thus perpetuating their presence in the environment as persistent and non-degradable pollution (13). Animals, humans and the environment are linked with each other in a shared ecology, and enough evidence is available regarding the existence of ESBL-producing bacteria in different ecological niches (14, 15). In this study, to explore the level of antibiotic-resistant E. coli, especially the production of CTX-M, and to provide useful information about the epidemiology of ESBLs, fecal samples from bovines (yaks, dairy cows, and beef cattle) from different areas were investigated.
Materials and Methods
Sampling
From 2018 to 2019, we collected 790 bovine fecal samples from Gansu, Qinghai, Sichuan, Shandong and Xinjiang in China (Figure 1). In the Gansu Province, we collected fecal samples from beef cattle (n = 180) and yaks (n = 90). In the Shandong Province, we collected fecal samples from beef cattle (n = 100) and dairy cattle (n = 80). In Xinjiang, we collected fecal samples from dairy cattle (n = 150). In Sichuan, we collected fecal samples from yaks (n = 100). In Qinghai, we collected fecal samples from yaks (n = 90). The fecal samples were collected after spontaneous defecation by sterile flocked swabs, stored in commercial universal transport medium (Yocon Biology Co., Beijing, China) and transported to the laboratory for analyses within 24 h of sampling.
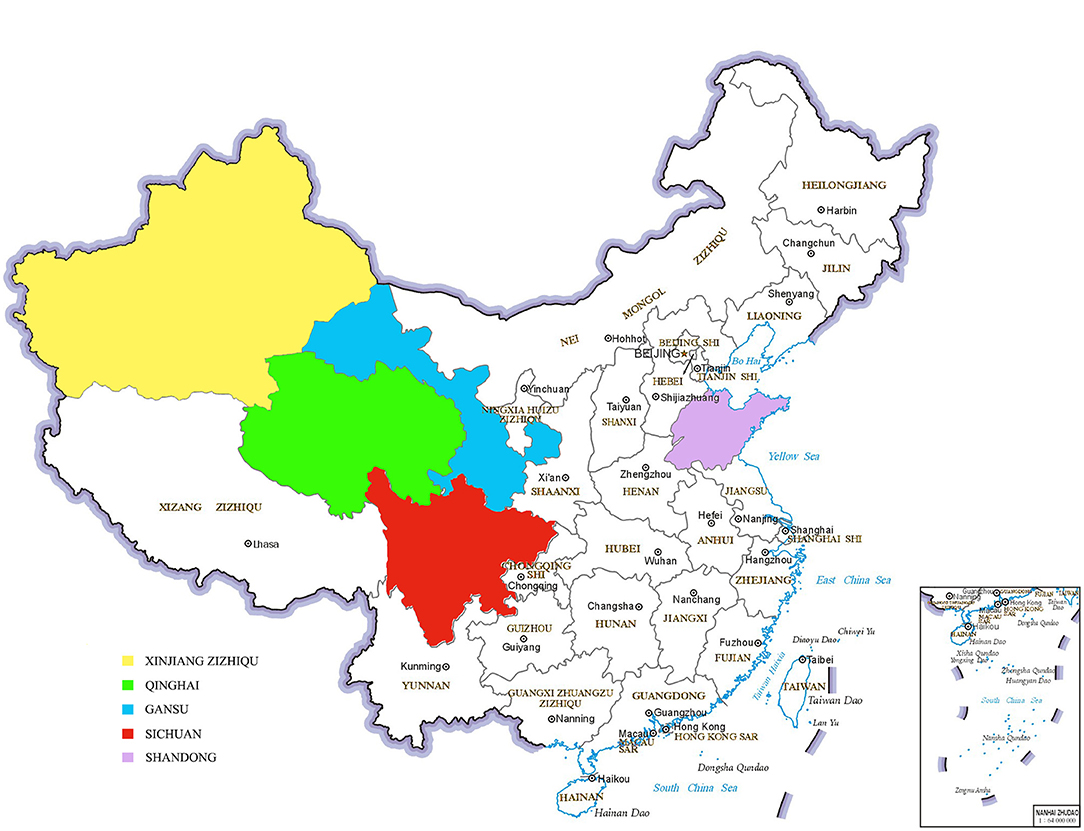
Figure 1. Map identification of sampling locations (Yellow is Xinjiang, green is Qinghai, blue is Gansu, red is Sichuan and purple is Shandong).
Bacterial Isolation and Identification
The isolation of E. coli was performed using selective chromogenic E. coli agar (Hopebio, Qingdao, China). In brief, samples were added to 2 ml of buffered peptone water and incubated at 35 ± 2°C for 16–18 h. Then, a loop of this broth was streaked onto chromogenic E. coli agar (Hopebio, Qingdao, China) and incubated at 35 ± 2°C for 18 h for E. coli selection. Presumptive E. coli colonies were identified by matrix-assisted laser desorption ionization–time of flight mass spectrometry (MALDI-TOF-MS) (Bruker Daltonics GmBH, Bremen, Germany). The data were processed by BioTyper 3.0 software. According to the manufacturer's recommendation, a score cut-off of ≥2.000 was considered E. coli. The identified E. coli isolates were cultured on commercial CHROMagar ESBL selective chromogenic medium (CHROMagar ESBL, France) and then the double-disc synergy test was used to detect the production of ESBL.
Antibiotic Susceptibility Testing
The antibiotic susceptibility of the E. coli isolates from bovines was determined using a Kirby–Bauer disk diffusion approach (K-B programme) according to the recommendations of the Clinical and Laboratory Standards Institute (CLSI) (16) and the European Committee on Antimicrobial Susceptibility Testing (17). The following antibiotics were used: ampicillin (AMP), aztreonam (ATM), imipenem (IPM), cefuroxime (CXM), cefepime (FEP), ceftazidime (CAZ), cefmetazole (CMZ), cefotaxime (CTX), gentamicin (GEN), kanamycin (KAN), chloramphenicol (CHL), tetracyclines (TCY), streptomycin (STR), ciprofloxacin (CIP), and colistin (COL). The colistin results were evaluated according to the European Committee on Antimicrobial Susceptibility Testing (18). The results for the other antibiotics were evaluated according to the CLSI guidelines. E. coli ATCC 25,922 was used as a quality control strain. According to the results of the K-B disk assays, 48 multidrug-resistant E. coli isolates were selected to test the minimum inhibitory concentrations (MICs) by microdilution methods.
Multilocus Sequence Typing
Conventional MLST was performed using seven housekeeping genes (adk, fumC, gyrB, icd, mdh, purA and recA), as described by Wirth et al. (19). The E. coli MLST database (https://pubmlst.org/bigsdb?db=pubmlst_escherichia_seqdef) was used to obtain the allele number and sequence type (ST).
Detection and Sequencing of ESBLs (CTX-M, TEM, SHV), Carbapenemases (OXA, KPC, IMP, VIM), and MCR Genes
All isolates were screened for blaCTX-M genotypes by PCR. The primers for the CTX-M-1 group, CTX-M-2 group, CTX-M-8 group, and CTX-M-9 group, as well as the PCR conditions were as previously described (20). The primers for the CTX-M-25 group were as previously reported (21). The primers for KPC (22), IMP (23), VIM (24), OXA (25), TEM (26), SHV (26), and MCR were as previously described. Positive PCR products were further purified using a TIANgel purification kit (Tiangen, Beijing, China) and sent for sequencing. Sequencing was performed by the Tianqi Company (Gansu, China). The sequences obtained from the tests were entered into the BLAST database for comparison (27).
Data Statistics and Analysis
The data involved in the study were kept in a special test logbook, which was then entered into Excel (Microsoft Corporation, Redmond, USA) for storage and processing. The results of the percentage comparisons were provided by the chi-square test in SPSS 26.0 (IBM, Armonk, USA).
Results
Bacterial Isolation and Identification
In total, 523 E. coli isolates were identified from 790 samples. The sampling area, species of animals and strain numbers of the E. coli isolates are reported in Table 1. Overall, 523 isolates were used in this study. Of these fifty-six strains of E. coli were obtained from yaks in Qinghai, 62 strains of E. coli were obtained from yaks in Sichuan, and 60 strains of E. coli were obtained from yaks in Gansu; 129 strains of E. coli were obtained from beef cattle in Gansu and 60 strains of E. coli were obtained from beef cattle in Shandong; and 108 strains of E. coli were obtained from dairy cows in Xinjiang and 48 strains of E. coli were obtained from dairy cows in Shandong.
Antibiotic Susceptibility Testing
In this study, 15 classes of antibiotics were used for sensitivity testing by K-B methods. The results (Figure 2) showed that the antimicrobial spectrum varied markedly between sampling areas; in addition, different animals in the same area had different antimicrobial profiles. In terms of animal species, antibiotic resistance rates in yaks were much lower than those in beef cattle and dairy cattle. Considering the sampling area, the highest antibiotic resistance was found in Shandong, followed by Gansu and Xinjiang, and the lowest resistance rates were found in Qinghai. Among the 15 antibiotics studied, the most frequently observed resistance phenotypes were ATM, CMZ, CAZ, CTX, AMP, and CIP.
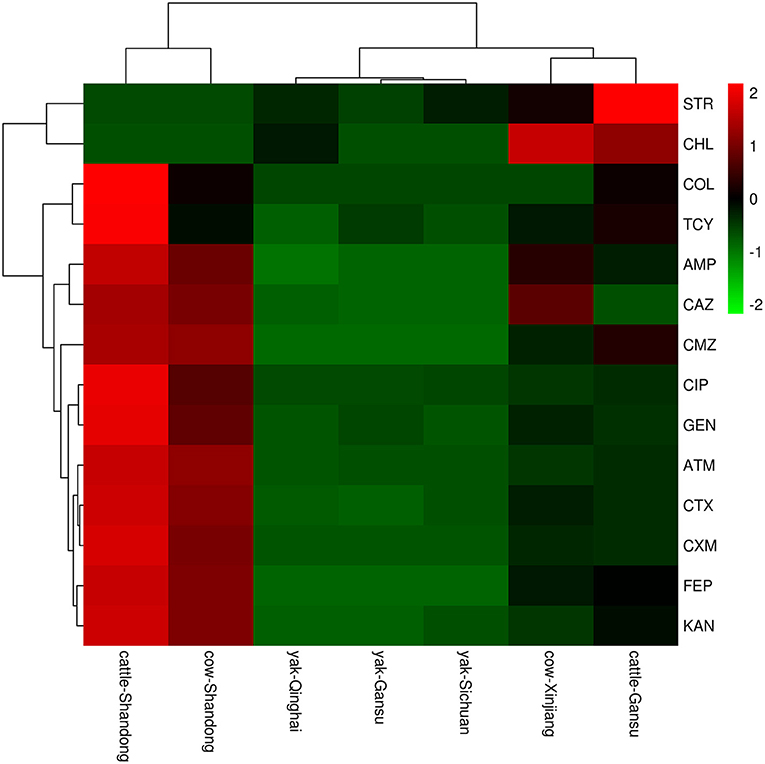
Figure 2. A heatmap of resistant phenotype percentage of E.coli isolation from different areas. The right side of the legend shows the color range of different R values. Species clustering trees are presented on the left side of the heat map.
More specifically, the E. coli isolates from the Shandong samples were more resistant to the antibiotics tested than those from other areas. In E. coli from beef, resistance was found to the tested antibiotics, from highest to lowest, as follows: ATM (100.0%), CTX (98.3%), CMZ (98.3%), AMP (93.3%), CAZ (93.3%), FEP (91.7%), CXM (88.3%), TCY (80.0%), CIP (68.3%), GEN (45.0%), KAN (36.7%), and COL (13.3%). No E. coli isolates exhibited resistance to CHL, STR or IPM. In E. coli isolates from dairy cows, the highest resistance was to ATM (100.0%), followed by CAZ (97.9%), CTX (95.8%), CMZ (91.7%), AMP (89.6%), CXM (89.6%), FEP (81.3%), CIP (43.8%), KAN (33.3%), GEN (33.3%), TCY (27.1%), and COL (4.2%). No E. coli isolates were resistant to CHL, STR, or IPM (Figure 3).
The distribution of drug resistance among E. coli isolates from Xinjiang cows is shown in Figure 4. The highest resistance was to AMP (87.0%), followed by CAZ (33.3%), CTX (13.2%), FEP (12.0%), CMZ (12.0%), TCY (10.9%), and CHL (9.3%). All isolates were sensitive to COL and IPM.
The distribution of antibiotic resistance among E. coli isolates from Gansu samples is shown in Figure 5. The antibiotic sensitivity of E. coli isolates from beef cattle and yaks was different. Among the beef cattle samples, of the 15 antibiotics tested, E. coli isolates presented resistance to 14 antibiotics, while E. coli isolates from yaks were only resistant to 7 antibiotics. Among the beef cattle samples, the highest resistance was found to AMP (76.0%), followed by STR (21.7%), while the lowest resistance was found to IPM (0%). Among yak samples, the highest resistance was to AMP (13.3%), followed by CTX (6.7%) and TCY (6.7%), while yak E. coli isolates were sensitive to 9 antibiotics (FEP, CMZ, COL, IPM, CXM, CIP, KAN, and CHL).
The distribution of antibiotic resistance among E. coli isolates from Qinghai yak is shown in Figure 6. Among the 15 antibiotics tested, E. coli isolates were resistant to six antibiotics: AMP (8.9%), CTX (8.9%), STR (5.4%), TCY (3.6%), CHL (3.6%), and CMZ (3.6%). E. coli isolates were sensitive to the rest of the antibiotics tested (FEP, CMZ, COL, IPM, ATM, CXM, CIP, GEN, and KAN).
The distribution of antibiotic resistance among E. coli isolates from the Sichuan yak is shown in Figure 7. Among the 15 antibiotics tested, E. coli isolates were resistant to 8 classes: AMP (12.9%), CTX (11.3%), STR (6.5%), TCY (6.5%), ATM (1.6%), CIP (1.6%), KAN (1.6%), and CAZ (1.6%). E. coli isolates were sensitive to the rest of the antibiotics tested.
E. coli isolates from yaks share the characteristics of high resistance to AMP, CTX, TCY, STR, and CAZ (Figure 8) and sensitivity to CXM, FEP, CMZ, COL, and IPM.
Moreover, multidrug-resistance phenotypes (resistance to >2 of the antibiotic classes tested) were observed. The results are shown in Figure 2. E. coli isolates from Shandong were the most represented. The most prevalent multidrug resistance phenotypes were AMP-FEP-CMZ (n = 4) and CTX-FEP-CMZ (n = 3), found in the beef cattle from Gansu; AMP-CAZ (n = 10), CTX-CAZ (n = 5), and AMP-CAZ-CTX-FEP-CMZ (n = 4), found in dairy cattle from Xinjiang; AMP-FEP-ATM-CAZ-CMZ-CTX-IPM (n = 7), MP-CXM-FEP-CAZ-CMZ-ATM-CTX-IPM (n = 6), and GEN-AMP-CXM-CIP-ATM-FEP-CMZ-CTX-KAN-IPM (n = 4), found in dairy cattle from Shandong; and AMP-CXM-CIP-FEP-CAZ-TCR-CMZ-CTX-ATM-IPM (n = 8), and AMP-CXM-FEP-CAZ-TCR-CMZ-CTX-ATM-IPM (n = 7), found in beef cattle.
The MIC results of 48 selected multidrug-resistant E. coli isolates are shown in Table 2. The results were similar to those of the K-B methods.
MLST
MLST analysis showed that 44 ST types were obtained from the 144 E. coli isolates, and they were further classified into 9 groups and 35 singletons. The ST types of the E. coli isolates were diverse; of them, the most predominant ST was ST95 (n = 9, 6.25%), followed by ST93 (n = 8, 5.6%), ST156 (n = 7, 4.9%), ST446 (n = 6, 4.2%), ST392 (n = 6, 4.2%), ST10 (n = 4, 2.8%), ST155 (n = 4, 2.8%), ST744 (n = 4, 2.8%), ST1637 (n = 4, 2.8%) and ST6345 (n = 4, 2.8%).
Group 2 was centered on ST10, which was the largest, containing 9 ST types (Figure 9A); group 4 was centered on ST95, containing 4 ST types (Figure 9B); group 7 was centered on ST446, containing 4 ST types (Figure 9C); and the rest of the groups had no center. Group 1 contained 3 ST types, group 3 contained 4 ST types, group 5 contained 2 ST types, group 6 contained 4 ST types, group 8 contained 4 ST types, and group 9 contained 2 ST types.
Compared with ST10, only one allele of ST744 and ST48 was different, and two alleles of ST4234, ST3489, ST373, and ST168 were different. Among the 35 singletons, there were more than 5 alleles that were not identical to each other.
PCR of Resistance Genes
We screened ESBL and carbapenemase genotypes by PCR in the E. coli isolates from yaks, beef cattle, and dairy cows. The results showed that the distribution of CTX-M genotypes was distinct in different bovines and in various sampling areas (Tables 3, 4). Considering the animal species, yaks had the fewest number of E. coli isolates harboring the CTX-M genotype (7.3%, 13/178); beef cattle had the largest number of E. coli isolates harboring the CTX-M genotype (50.8%, 96/189); and dairy cows had an intermediate number of E. coli isolates harboring the CTX-M genotype (30.8%, 48/156). Based on the geographical area, the highest prevalence of E. coli carrying the CTX-M gene was found among the Shandong samples (56.5%, 61/108), followed by the Gansu samples (31.7%, 60/189), the Xinjiang samples (22.2%, 24/108), the Sichuan samples (12.9%, 8/62), and finally, the lowest prevalence was among the Qinghai samples (3.6%, 2/56).
In yaks, the main genotype was CTX-M-14 (5.1%, 9/178). In beef cattle of Gansu, the most prevalent genotypes were CTX-M-55 (27.9%, 36/129), CTX-M-14 (24.0%, 31/129) and CTX-M-79 (21.7%, 28/129). In dairy cows from Xinjiang, the most common genotypes were CTX-M-14 (12.0%, 13/108), CTX-M-55 (11.1%, 12/108), and CTX-M-79 (10.2%, 11/108). In dairy cows and beef cattle from Shandong, the most prevalent genotypes were CTX-M-15 (37.0%, 40/108) and CTX-M-238 (20.4%, 22/108). CTX-M-79 was always present, accompanied by CTX-M-55.
In addition, many isolates carried more than one CTX-M gene. In E. coli isolates from Xinjiang, 4.6% (5/108) carried two CTX-M genes and 5.6% (6/108) carried three CTX-M genes. In E. coli isolates from beef cattle in Gansu, 19.4% (25/129) carried two CTX-M genes and 8.5% (11/129) carried three CTX-M genes. In E. coli isolates from Shandong, 1.9% (2/108) carried two CTX-M genes and no isolates carried three CTX-M genes.
This study showed that CTX-M genotypes were common in bovines, and the most prevalent were CTX-M-55, CTX-M-79, and CTX-M-15. Moreover, CTX-M-55 and CTX-M-15 emerged alone, while CTX-M-79 always emerged with CTX-M-55.
In E. coli isolates from Shandong, the detection of TEM was 45.4% (49/108), followed by isolates from Gansu beef at 39.5% (51/129), isolates from Xinjiang cows at 17.6% (19/108) and isolates from Sichuan at 3.2% (2/62). No IMP, VIM, OXA, SHV, KPC, or MCR were detected in any E. coli isolate.
Discussion
In this study, fecal samples were collected from yaks, beef cattle, and dairy cattle from the five provinces in China to investigate the prevalence of the CTX-M resistance gene. The results showed that the most predominant resistance gene types were CTX-M-15, CTX-M-14, CTX-M-55, CTX-M-79, and TEM-1. This study suggests that bovines may be carriers of the CTX-M genotype. It is necessary to examine the factors that contribute to its dissemination (28).
Understanding antibiotic use is essential for drawing conclusions about the epidemiological links between antibiotic use in farm animals, antibiotic resistance, animal health, and human health. In China, the majority of cattle farming is located in the northern regions; based on this, the farming models in the central and eastern regions consist mainly of modern intensive farming, as well as a very small proportion of individual farms. In the west, however, while the dairy industry is also concentrated in intensive farming, beef cattle and the endemic breed yak are often farmed in a diversified manner. While common beef cattle are generally jointly owned by large farms and individual households, yaks are generally owned entirely by herders.
According to a survey, mastitis, uterine infections, and enteritis are often treated with antibiotics in dairy farms in China. In beef cattle farming, respiratory disease is often the top cause of morbidity, with hoof disease being a close second. This is similar to other parts of the world (29, 30). We know from the beef farm veterinarians that large amounts of ceftiofur and florfenicol are being used to control respiratory outbreaks following calf transfers and weather changes (31, 32). Ceftiofur with non-steroidal anti-inflammatory drugs (NSAIDs) is also frequently used due to the high incidence of diseases such as laminitis. According to previous literature, the most commonly used antibiotic in US cattle farms is penicillin, which accounts for 32% of the total antibiotic use, much higher than other antibiotics (33, 34). First-generation cephalosporins, penicillin, and third-generation cephalosporins are the most commonly used in Canadian dairy farms (29). Regarding the Australian region, antibiotic use on New Zealand cattle farms includes mainly penicillin, macrolides and cephalosporins (35). The most common drugs used on cattle farms in the UK are β-lactams, with third-generation cephalosporins, such as ceftiofur sodium, being the most common regardless of route of administration, followed by penicillin/streptomycin combinations (36). In other parts of Europe, it has been reported that Austria has a similar use profile (37). In addition, a pan-European antimicrobial susceptibility monitoring programme, the VetPath study, has shown that E. coli isolated in Europe is generally highly resistant to cephalosporins (38). This is similar to the situation on large intensive farms in Shandong, Xinjiang and Gansu. Our results showed that the samples collected from Shandong had the most complicated drug-resistance phenotypes, and multidrug resistance was the most serious in this region. The yak samples had the least complicated resistance profiles. Among the antibiotics tested, it is worth noting that the detection rate for colistin resistance was low. This is because colistin was banned as a feed additive by the Chinese government in 2017 but can still be used therapeutically (39). It is also interesting to note that the detection rate for imipenem- and aztreonam-resistant E. coli was very high in Shandong, but these two types of drugs are not used in the farming process. We hypothesize that this is because resistance to atypical β-lactam antibiotics (β-lactam antibiotics other than penicillin and cephalosporins) can be mediated by the production of ESBLs and/or AmpCs (40, 41). It has been reported in the literature that ESBLs and AmpCs can hydrolyse carbapenem antibiotics at very low levels (42, 43). This resistance can be horizontally transmitted through environments such as sinks, feed and soil, and the various genetic and transcription factors by which resistance arises are easily activated in the environment (44, 45). The global epidemic of atypical β-lactam-resistant Enterobacteriaceae has posed a serious public health threat (44, 46, 47), and the results of this study are worth attention.
In contrast, the detection rate of drug-resistant bacteria in yaks was very low. Yaks live mainly in the western highlands of China and mainly graze in pastures (48). Unlike intensive farming environments, disease is less likely to break out on a large scale and unlike large farms with dedicated veterinarians, highland herders rely on their own experience for the treatment of sick yaks, apart from regular guidance from government veterinarians, and do not have records of drug use or standard prescriptions. This is largely in line with farming in low-income areas (49–51). These areas are more prone to antibiotic abuse compared to larger farms (52). However, because the incidence is low and the use of antibiotics is minimal, drug resistance genes are much less likely to spread.
A US study reported that 39 out of 42 stool samples contained E. coli carrying CTX-M (93%) (53). In another German prevalence survey on ESBL-producing E. coli, 96.5% of calves in the 10 large rural villages involved were ESBL-positive, 92.9% of which were E. coli (54). In contrast, a Nigerian study showed that the CTX-M gene was detected in only 12.8% (32/250) of cattle samples (55). In West and Central Africa, the prevalence of ESBL-E. coli in cattle is 0% (56). This explains the higher prevalence in Shandong, a middle- and high-income region, compared to the other regions in this study.
CTX-M-55, CTX-M-79, CTX-M-15, and TEM have been identified not only in animals but also in humans and the environment (57–59). Evidence has shown that antibiotic resistance genes and antibiotic-resistant bacteria can spread to humans via contaminated meat, house dust, manure, and waste water discharge (60–62). Meanwhile, direct evidence has shown the environmental transmission of antibiotic resistance genes and their bacterial hosts among livestock and humans (63).
At present, the daily excretion of the dairy cattle manure is as high as 200,000–300,000 t in China. In some places, untreated cattle manure is returned to the field, and resistant bacteria and genes are released into the environment following feces application. Antimicrobial resistance genes can be cotransferred among humans, animals, food, and the environment (64). Bovines are reservoirs and are the active spreaders of ESBL producers. It is necessary to survey and control antibiotic resistance on animal farms.
Our results will help to establish a baseline to guide the interventions in reducing antibiotic resistance in agriculture and on farms. The disadvantages of this study are the sampling area and the limited animal species. To obtain more accurate results, larger samples will be needed.
Data Availability Statement
The raw data supporting the conclusions of this article will be made available by the authors, without undue reservation.
Ethics Statement
The animal study was reviewed and approved by Research Ethics Committee of Lanzhou Institute of Husbandry and Pharmaceutical Science of CAAS. Written informed consent was obtained from the owners for the participation of their animals in this study.
Author Contributions
XW and ZD wrote the manuscript. NL and XW carried out the CTX-M gene detection and the antimicrobial susceptibility testing. WW was responsible for isolation of E. coli. LW, BL, XZ, FC, KZ, HC, and HS collected the samples. JZ provided the funding resource and was responsible for the paper quality. All authors contributed to the article and approved the submitted version.
Funding
This study was supported by the NSCF (grant number 31872520) and China Agriculture Research System of MOF and MARA (grant number CARS-37).
Conflict of Interest
The authors declare that the research was conducted in the absence of any commercial or financial relationships that could be construed as a potential conflict of interest.
Publisher's Note
All claims expressed in this article are solely those of the authors and do not necessarily represent those of their affiliated organizations, or those of the publisher, the editors and the reviewers. Any product that may be evaluated in this article, or claim that may be made by its manufacturer, is not guaranteed or endorsed by the publisher.
Acknowledgments
The authors acknowledge and thank the help of staff members in the cattle farm.
References
1. Hu Y-Y, Cai J-C, Zhou H-W, Chi D, Zhang X-F, Chen W-L, et al. Molecular typing of CTX-M-producing escherichia coli isolates from environmental water, swine feces, specimens from healthy humans, and human patients. Appl Environ Microbiol. (2013) 79:5988–96. doi: 10.1128/AEM.01740-13
2. Mulvey MR, Bryce E, Boyd D, Ofner-Agostini M, Christianson S, Simor AE, et al. Canadian hospital epidemiology committee, canadian nosocomial infection surveillance program, health canada. ambler class a extended-spectrum beta-lactamase-producing escherichia coli and klebsiella spp. in canadian hospitals. Antimicrob Agents Chemother. (2004) 48:1204–14. doi: 10.1128/AAC.48.4.1204-1214.2004
3. Dikoumba A-C, Onanga R, Boundenga L, Bignoumba M, Ngoungou E-B, Godreuil S. Prevalence and characterization of extended-spectrum beta-lactamase-producing enterobacteriaceae in major hospitals in Gabon. Microb Drug Resist. (2021) 1525–34. doi: 10.1089/mdr.2020.0497
4. Ewers C, de Jong A, Prenger-Berninghoff E, El Garch F, Leidner U, Tiwari SK, et al. Genomic diversity and virulence potential of ESBL- and AmpC-β-Lactamase-producing escherichia coli strains from healthy food animals across Europe. Front Microbiol. (2021) 12:626774. doi: 10.3389/fmicb.2021.626774
5. Le PQ, Awasthi SP, Hatanaka N, Hinenoya A, Hassan J, Ombarak RA, et al. Prevalence of mobile colistin resistance (mcr) genes in extended-spectrum β-lactamase-producing Escherichia coli isolated from retail raw foods in Nha Trang, Vietnam. Int J Food Microbiol. (2021) 346:109164. doi: 10.1016/j.ijfoodmicro.2021.109164
6. Bonnet R. Growing group of extended-spectrum beta-lactamases: the CTX-M enzymes. Antimicrob Agents Chemother. (2004) 48:1–14. doi: 10.1128/AAC.48.1.1-14.2004
7. Cantón R, Coque TM. The CTX-M beta-lactamase pandemic. Curr Opin Microbiol. (2006) 9:466–75. doi: 10.1016/j.mib.2006.08.011
8. Goyal S, Dhull SK, Kapoor KK. Chemical and biological changes during composting of different organic wastes and assessment of compost maturity. Bioresour Technol. (2005) 96:1584–91. doi: 10.1016/j.biortech.2004.12.012
9. Tran DTQ, Bradbury MI, Ogtrop FFV, Bozkurt H, Jones BJ, McCONCHIE R. Environmental drivers for persistence of escherichia coli and salmonella in manure-amended soils: a meta-analysis. J Food Prot. (2020) 83:1268–77. doi: 10.4315/0362-028X.JFP-19-460
10. Noble. Risks and benefits of soil amendment with composts in relation to plant pathogens. Australas Plant Path. (2011) 40:157–67. doi: 10.1007/s13313-010-0025-7
11. Huygens J, Daeseleire E, Mahillon J, Van Elst D, Decrop J, Meirlaen J, et al. Presence of antibiotic residues and antibiotic resistant bacteria in cattle manure intended for fertilization of agricultural fields: a one health perspective. Antibiotics. (2021) 10:410. doi: 10.3390/antibiotics10040410
12. Neher DA, Cutler AJ, Weicht TR, Sharma M, Millner PD. Composts of poultry litter or dairy manure differentially affect survival of enteric bacteria in fields with spinach. J Appl Microbiol. (2019) 126:1910–22. doi: 10.1111/jam.14268
13. Ata R, Yildiz Töre G. Characterization and removal of antibiotic residues by NFC-doped photocatalytic oxidation from domestic and industrial secondary treated wastewaters in meric-ergene basin and reuse assessment for irrigation. J Environ Manage. (2019) 233:673–80. doi: 10.1016/j.jenvman.2018.11.095
14. Pot M, Reynaud Y, Couvin D, Ducat C, Ferdinand S, Gravey F, et al. Wide distribution and specific resistance pattern to third-generation cephalosporins of enterobacter cloacae complex members in humans and in the environment in guadeloupe (French West Indies). Front Microbiol. (2021) 12:628058. doi: 10.3389/fmicb.2021.628058
15. Garcias B, Aguirre L, Seminati C, Reyes N, Allepuz A, Molina-Lopez RA, et al. Extended-spectrum β-Lactam Resistant Klebsiella pneumoniae and escherichia coli in wild European hedgehogs (Erinaceus europeus) living in populated areas. Animal. (2021) 11:2837. doi: 10.3390/ani11102837
16. CLSI. Performance Standards for Antimicrobial Susceptibility Testing. 31st Ed. Wayne: Clinical and Laboratory Standards Institute (2021).
17. EUCAST. EUCAST Guidelines for Detection of Resistance Mechanisms and Specific Resistances of Clinical and/or Epidemiological Importance. Version 1.0. Available online at: http://www.eucast.org/fileadmin/src/media/PDFs/EUCAST_files/Resistance_mechanisms/EUCAST_detection_of_resistance_mechanisms_v1.0_20131211.pdf (accessed December, 2012).
18. EUCAST. Clinical Breakpoints - Breakpoints and Guidance. Basel: The European Committee on Antimicrobial Susceptibility Testing (2021).
19. Wirth T, Falush D, Lan R, Colles F, Mensa P, Wieler LH, et al. Sex and virulence in Escherichia coli : an evolutionary perspective. Mol Microbiol. (2006) 60:1136–51. doi: 10.1111/j.1365-2958.2006.05172.x
20. Pitout JDD, Hossain A, Hanson ND. Phenotypic and molecular detection of CTX-M-beta-lactamases produced by Escherichia coli and Klebsiella spp. J Clin Microbiol. (2004) 42:5715–21. doi: 10.1128/JCM.42.12.5715-5721.2004
21. Navon-Venezia S, Chmelnitsky I, Leavitt A, Carmeli Y. Dissemination of the CTX-M-25 family beta-lactamases among Klebsiella pneumoniae, Escherichia coli and Enterobacter cloacae and identification of the novel enzyme CTX-M-41 in Proteus mirabilis in Israel. J Antimicrob Chemother. (2008) 62:289–95. doi: 10.1093/jac/dkn182
22. Smith Moland EHN, Herrera VL, Black JA, Lockhart TJ, Hossain A, Johnson JA, et al. Plasmid-mediated, carbapenem-hydrolysing beta-lactamase, KPC-2, in Klebsiella pneumoniae isolates. J Animicrob Chemother. (2003) 51:711–4. doi: 10.1093/jac/dkg124
23. Senda KAY, Ichiyama S, Nakashima K, Ito H, Ohsuka S, Shimokata K, et al. PCR detection of metallo-beta-lactamase gene (blaIMP) in gram-negative rods resistant to broad-spectrum beta-lactams. J Clin Microbiol. (1996) 34:2909–13. doi: 10.1128/jcm.34.12.2909-2913.1996
24. Tsakris A PS, Woodford N, Palepou MF, Babini GS, Douboyas J, Livermore DM. Outbreak of infections caused by Pseudomonas aeruginosa producing VIM-1 carbapenemase in Greece. J Clin Microbiol. (2000) 38:1290–2 doi: 10.1128/JCM.38.3.1290-1292.2000
25. Poirel L HC, Tolün V, Nordmann P. Emergence of oxacillinase-mediated resistance to imipenem in Klebsiella pneumoniae. Antimicrobial Agents Chemoth. (2004) 48:15–22. doi: 10.1128/AAC.48.1.15-22.2004
26. Yan JJ WS, Tsai SH, Wu JJ, Su IJ. Prevalence of SHV-12 among clinical isolates of Klebsiella pneumoniae producing extended-spectrum beta-lactamases and identification of a novel AmpC enzyme (CMY-8) in Southern Taiwan. Antimicrob Agents Chemother. (2000) 44:1438–42. doi: 10.1128/AAC.44.6.1438-1442.2000
27. Altschul SF, Madden TL, Schäffer AA, Zhang J, Zhang Z, Miller W, et al. Gapped BLAST and PSI-BLAST: a new generation of protein database search programs. Nucleic Acids Res. (1997) 25:3389–402.
28. Sun Y, Zeng Z, Chen S, Ma J, He L, Liu Y, et al. High prevalence of bla(CTX-M) extended-spectrum β-lactamase genes in Escherichia coli isolates from pets and emergence of CTX-M-64 in China. Clin Microbiol Infect. (2010) 16:1475–81. doi: 10.1111/j.1469-0691.2010.03127.x
29. Saini V, McClure JT, Léger D, Dufour S, Sheldon AG, Scholl DT, et al. Antimicrobial use on Canadian dairy farms. J Dairy Sci. (2012) 95:1209–21. doi: 10.3168/jds.2011-4527
30. Lhermie G, Sauvage P, Tauer LW, Chiu LV, Kanyiamattam K, Ferchiou A, et al. Economic effects of policy options restricting antimicrobial use for high risk cattle placed in U.S. feedlots. PLoS ONE. (2020) 15:e0239135. doi: 10.1371/journal.pone.0239135
31. Calderón-Amor J, Gallo C. Dairy calf welfare and factors associated with diarrhea and respiratory disease among chilean dairy farms. Animals. (2020) 10:1115. doi: 10.3390/ani10071115
32. Pratelli A, Cirone F, Capozza P, Trotta A, Corrente M, Balestrieri A, et al. Bovine respiratory disease in beef calves supported long transport stress: an epidemiological study and strategies for control and prevention. Res Vet Sci. (2021) 135:450–5. doi: 10.1016/j.rvsc.2020.11.002
33. Redding LE, Bender J, Baker L. Quantification of antibiotic use on dairy farms in pennsylvania. J Dairy Sci. (2019) 102:1494–507. doi: 10.3168/jds.2018-15224
34. de Campos JL, Kates A, Steinberger A, Sethi A, Suen G, Shutske J, et al. Quantification of antimicrobial usage in adult cows and preweaned calves on 40 large Wisconsin dairy farms using dose-based and mass-based metrics. J Dairy Sci. (2021) 104:4727–45. doi: 10.3168/jds.2020-19315
35. Bryan M, Hea SY. A survey of antimicrobial use in dairy cows from farms in four regions of New Zealand. N Z Vet J. (2017) 65:93–8. doi: 10.1080/00480169.2016.1256794
36. Rees GM, Barrett DC, Buller H, Mills HL, Reyher KK. Storage of prescription veterinary medicines on UK dairy farms: a cross-sectional study. Vet Rec. (2019) 184:153. doi: 10.1136/vr.105041
37. Firth CL, Käsbohrer A, Schleicher C, Fuchs K, Egger-Danner C, Mayerhofer M, et al. Antimicrobial consumption on Austrian dairy farms: an observational study of udder disease treatments based on veterinary medication records. PeerJ. (2017) 5:e4072. doi: 10.7717/peerj.4072
38. de Jong A, Garch FE, Simjee S, Moyaert H, Rose M, Youala M, et al. Monitoring of antimicrobial susceptibility of udder pathogens recovered from cases of clinical mastitis in dairy cows across Europe: VetPath results. Vet Microbiol. (2018) 213:73–81. doi: 10.1016/j.vetmic.2017.11.021
39. Wang Y, Xu C, Zhang R, Chen Y, Shen Y, Hu F, et al. Changes in colistin resistance and mcr-1 abundance in Escherichia coli of animal and human origins following the ban of colistin-positive additives in China: an epidemiological comparative study. Lancet Infect Dis. (2020) 20:1161–71. doi: 10.1016/S1473-3099(20)30149-3
40. Jacoby GA. AmpC beta-lactamases. Clin Microbiol Rev. (2009) 22:161–82, Table‘ of Contents. doi: 10.1128/CMR.00036-08
41. Goodman KE, Simner PJ, Tamma PD, Milstone AM. Infection control implications of heterogeneous resistance mechanisms in carbapenem-resistant Enterobacteriaceae (CRE). Exp Rev Anti Infect Ther. (2016) 14:95–108. doi: 10.1586/14787210.2016.1106940
42. Girlich D, Poirel L, Nordmann P. Do CTX-M beta-lactamases hydrolyse ertapenem? J Antimicrob Chemother. (2008) 62:1155–6. doi: 10.1093/jac/dkn317
43. Fröhlich C, Gama JA, Harms K, Hirvonen VHA, Lund BA, van der Kamp MW, et al. Cryptic β-Lactamase evolution is driven by low β-lactam concentrations. mSphere. (2021) 6:e00108–21. doi: 10.1128/mSphere.00108-21
44. Logan LK, Weinstein RA. The epidemiology of carbapenem-resistant enterobacteriaceae: the impact and evolution of a global menace. J Infect Dis. (2017) 215:S28–36. doi: 10.1093/infdis/jiw282
45. Mmatli M, Mbelle NM, Maningi NE, Osei Sekyere J. Emerging transcriptional and genomic mechanisms mediating carbapenem and polymyxin resistance in enterobacteriaceae: a systematic review of current reports. mSystems. (2020) 5:e00783–20. doi: 10.1128/mSystems.00783-20
46. Piddock LJ, Traynor EA, Wise R. A comparison of the mechanisms of decreased susceptibility of aztreonam-resistant and ceftazidime-resistant enterobacteriaceae. J Antimicrob Chemother. (1990) 26:749–62. doi: 10.1093/jac/26.6.749
47. Nakano R. [Carbapenem-resistant enterobacteriaceae (CRE): a menace to the public and the mechanisms of antimicrobial resistance]. Jpn J Antibiot. (2016) 69:81–9.
48. Wu H, Luo D, Li C, Zhang H, Shunxian A, Zhang Y, et al. Chicoric acid improves heart and blood responses to hypobaric hypoxia in tibetan yaks. Am J Chin Med. (2018) 46:339–55. doi: 10.1142/S0192415X18500179
49. Redding LE, Cubas-Delgado F, Sammel MD, Smith G, Galligan DT, Levy MZ, et al. The use of antibiotics on small dairy farms in rural Peru. Prev Vet Med. (2014) 113:88–95. doi: 10.1016/j.prevetmed.2013.10.012
50. Rabello RF, Bonelli RR, Penna BA, Albuquerque JP, Souza RM, Cerqueira AMF. Antimicrobial resistance in farm animals in brazil: an update overview. Animals. (2020) 10:552. doi: 10.3390/ani10040552
51. Sharma G, Mutua F, Deka RP, Shome R, Bandyopadhyay S, Shome BR, et al. A qualitative study on antibiotic use and animal health management in smallholder dairy farms of four regions of India. Infect Ecol Epidemiol. (2020) 10:1792033. doi: 10.1080/20008686.2020.1792033
52. Llanos-Soto SG, Vezeau N, Wemette M, Bulut E, Greiner Safi A, Moroni P, et al. Survey of perceptions and attitudes of an international group of veterinarians regarding antibiotic use and resistance on dairy cattle farms. Prev Vet Med. (2021) 188:105253. doi: 10.1016/j.prevetmed.2020.105253
53. Agga GE, Silva PJ, Martin RS. Detection of extended-spectrum beta-lactamase-producing and carbapenem-resistant bacteria from mink feces and feed in the United States. Foodborne Pathog Dis. (2021) 2021:1–10. doi: 10.1089/fpd.2020.2898
54. Waade J, Seibt U, Honscha W, Rachidi F, Starke A, Speck S, et al. Multidrug-resistant enterobacteria in newborn dairy calves in Germany. PLoS ONE. (2021) 16:e0248291. doi: 10.1371/journal.pone.0248291
55. Fashae K, Engelmann I, Monecke S, Braun SD, Ehricht R. Molecular characterisation of extended-spectrum ß-lactamase producing Escherichia coli in wild birds and cattle, Ibadan, Nigeria. BMC Vet Res. (2021) 17:33. doi: 10.1186/s12917-020-02734-4
56. Ouchar Mahamat O, Kempf M, Lounnas M, Tidjani A, Hide M, Benavides JA, et al. Epidemiology and prevalence of extended-spectrum β-lactamase- and carbapenemase-producing enterobacteriaceae in humans, animals and the environment in west and central Africa. Int J Antimicrob Agents. (2021) 57:106203. doi: 10.1016/j.ijantimicag.2020.106203
57. Liu W, Chen L, Li H, Duan H, Zhang Y, Liang X, et al. Novel CTX-M {beta}-lactamase genotype distribution and spread into multiple species of Enterobacteriaceae in Changsha, Southern China. J Antimicrob Chemother. (2009) 63:895–900. doi: 10.1093/jac/dkp068
58. Cunha MPV, Lincopan N, Cerdeira L, Esposito F, Dropa M, Franco LS, et al. Coexistence of CTX-M-2, CTX-M-55, CMY-2, FosA3, and QnrB19 in extraintestinal pathogenic escherichia coli from poultry in Brazil. Antimicrob Agents Chemother. (2017) 61:e02474–16. doi: 10.1128/AAC.02474-16
59. Birgy A, Madhi F, Hogan J, Doit C, Gaschignard J, Caseris M, et al. CTX-M-55-, MCR-1-, and FosA-producing multidrug-resistant escherichia coli infection in a child in France. Antimicrob Agents Chemother. (2018) 62:e00127–18. doi: 10.1128/AAC.00127-18
60. Kultima JR, Sunagawa S, Li J, Chen W, Chen H, Mende DR, et al. MOCAT: a metagenomics assembly and gene prediction toolkit. PLoS ONE. (2012) 7:e47656. doi: 10.1371/journal.pone.0047656
61. Li J, Jia H, Cai X, Zhong H, Feng Q, Sunagawa S, et al. An integrated catalog of reference genes in the human gut microbiome. Nat Biotechnol. (2014) 32:834–41. doi: 10.1038/nbt.2942
62. Yang X, Liu W, Liu Y, Wang J, Lv L, Chen X, et al. F33: A-: B-, IncHI2/ST3, and IncI1/ST71 plasmids drive the dissemination of fosA3 and bla CTX-M-55/-14/-65 in Escherichia coli from chickens in China. Front Microbiol. (2014) 5:688. doi: 10.3389/fmicb.2014.00688
63. Levy SB, FitzGerald GB, Macone AB. Spread of antibiotic-resistant plasmids from chicken to chicken and from chicken to man. Nature. (1976) 260:40–2. doi: 10.1038/260040a0
Keywords: blaCTX-M, E. coli, feces, yak, beef cattle, dairy cattle
Citation: Wei X, Wang W, Lu N, Wu L, Dong Z, Li B, Zhou X, Cheng F, Zhou K, Cheng H, Shi H and Zhang J (2022) Prevalence of Multidrug-Resistant CTX-M Extended Spectrum Beta-Lactamase-Producing Escherichia coli From Different Bovine Faeces in China. Front. Vet. Sci. 9:738904. doi: 10.3389/fvets.2022.738904
Received: 09 July 2021; Accepted: 19 May 2022;
Published: 01 August 2022.
Edited by:
Latiffah Hassan, Universiti Putra Malaysia, MalaysiaReviewed by:
Qazi Mohd Rizwanul Haq, Jamia Millia Islamia, IndiaHasan Badrul, Uppsala University, Sweden
Kun Li, Nanjing Agricultural University, China
Copyright © 2022 Wei, Wang, Lu, Wu, Dong, Li, Zhou, Cheng, Zhou, Cheng, Shi and Zhang. This is an open-access article distributed under the terms of the Creative Commons Attribution License (CC BY). The use, distribution or reproduction in other forums is permitted, provided the original author(s) and the copyright owner(s) are credited and that the original publication in this journal is cited, in accordance with accepted academic practice. No use, distribution or reproduction is permitted which does not comply with these terms.
*Correspondence: Jiyu Zhang, infzjy@sina.com
†These authors have contributed equally to this work and share first authorship