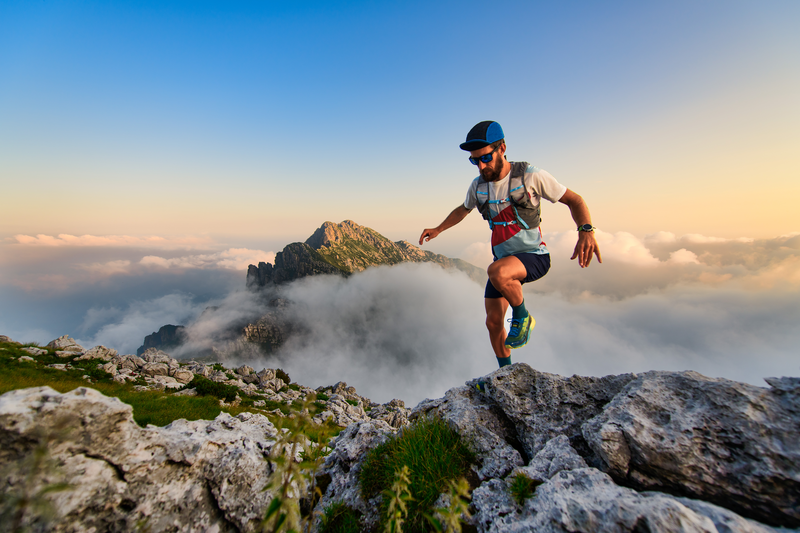
94% of researchers rate our articles as excellent or good
Learn more about the work of our research integrity team to safeguard the quality of each article we publish.
Find out more
ORIGINAL RESEARCH article
Front. Vet. Sci. , 12 December 2022
Sec. Animal Nutrition and Metabolism
Volume 9 - 2022 | https://doi.org/10.3389/fvets.2022.1076906
This article is part of the Research Topic Application of Natural Bioactive Compounds in Animal Nutrition View all 21 articles
The fermented feed has been used extensively as a growth promoter in agricultural animal production. However, the effects of fermented feed on swine gut microbiota are still largely unknown. The work presented here aimed to investigate the growth performance and gut microbiota of nursery pigs receiving the LPF diet (10% Lactobacillus plantarum and Pediococcus acidilactici co-fermented feed + basal diet) compared with pigs receiving the NC diet (basal diet). The data showed LPF diet numerically improved average daily gain and significantly increased fecal acetate, butyrate, and total short-chain fatty acid (SCFA) concentrations. Furthermore, gut microbiota structure and membership significantly changed in response to the addition of fermented feed in the diet. Gut microbiota results indicated that LPF treatment significantly enriched SCFA-producing bacteria such as Megasphaera, Roseburia, Faecalibacterium, Blautia, Selenomonas, Dialister, Acidaminococcus, Ruminococcus, and Bifidobacterium. Some of these bacteria also had anti-inflammatory and other beneficial functions. Overall, these findings suggested that Lactobacillus plantarum and Pediococcus acidilactici co-fermented feed benefited growth performance and established potential health impacts on the gut microbiota of nursery pigs.
Nowadays the beneficial effects of fermented feed on animal growth performance and health have received increasing attention. Fermentation is a metabolic process that produces biochemical changes in the primary food matrix through the action of microorganism enzymes (1). The importance of fermented feed is to improve the nutrient digestibility of raw material (e.g., corn and soybean meal) and increase the availability of vitamins and minerals by reducing the level of anti-nutritional factors (2). Apart from improved nutritional quality, fermented feed could benefit animal growth performance by boosting immune function and improving intestinal morphology (3, 4). Zhou et al. reported that fermented feed not only increased serum immunoglobulin levels but also improved lymphocyte proliferation and transformation (5). Another study revealed that supplementing piglet diets with fermented soybean meal significantly increased both villus height and villus: crypt ratio, which enlarged intestinal surface area and thereby improved nutrition uptake ability (6). Furthermore, Kiers et al. determined that fermented soybeans promoted feed intake and weight gain, as well as reduced diarrhea incidence of weaned piglets challenged with Escherichia coli (7).
Evidence is accumulating to demonstrate that incorporating fermented feed into swine diets has many beneficial effects on intestinal microbiota. Lactic acid-producing bacteria such as Lactobacillus plantarum and Pediococcus acidilactici are commonly used for fermentation, which generates a large amount of lactic acid and lowers the pH of the feed. Thus, the fermented feed can inhibit pathogenic bacterial growth, deliver probiotics, and prevent pathogenic bacteria from attaching to the intestinal walls (8). Studies have shown that fermented products reduced the proliferation of certain enteropathogens like E. coli and Salmonella in both swine and broiler (4, 8).
Although previous studies have remarkably expanded our knowledge regarding the impacts of fermented feed on gut microorganisms, they delivered information only on a limited set of microbial taxa. A gap in the understanding of how fermented feeds modulate the entire complex gut microbial ecosystem still exists. In this study, we tested Lactobacillus plantarum and Pediococcus acidilactici co-fermented feed and applied next-generation sequencing technology to achieve a depth insight into how the gut microbiota of nursery pigs evolves under the influence of fermented feed.
Animal management and care followed the Institute of Animal Husbandry and Veterinary Medicine of Hebei Province Animal Care and Use Committee guidelines (IAHVM20190910-1, Hebei, China).
This study was carried out at a commercial swine farm (Zhangjiakou, China). On the weaning day, a total of 32 piglets (body weight 14.65 ± 0.46 kg; 25 ± 1 d of age) were transferred to a nursery facility and were randomly assigned to the negative control (NC, n = 16) or fermented feed (LPF, n = 16) groups. The ambient temperature was set at 30°C upon pig arrival and was reduced 2°C per week until a 24°C setting was achieved. Each pen was fully slatted (1.8 × 2.0 m2) and was equipped with a nipple drinker and a feeder for ad libitum access to diets and water. Each pen housed eight pigs. After a 14-day adaption period, a 31-day feeding trial was conducted. All pigs were supplied with a common diet during the adaption period and then switched to the experimental diets at 39-day old. NC diet: basal diet; LPF diet: NC + 10% Lactobacillus plantarum and Pediococcus acidilactici co-fermented feed (M108, Dacheng (Wanda) Tianjin Co., Ltd, China). Diets meet or exceed the NRC (9) nutrient requirements and the Supplementary Tables S1, S2 detailed the basal diet and LPF diet formulations.
Individual pig body weight (BW) was measured at the beginning and the end of the study. Each dietary treatment data was used to calculate average daily gain (ADG).
Blood samples (n = 5) were collected into tubes via jugular vena cava from randomly selected pigs (69-day-old) at the end of the study and were assayed for total protein (TP), blood urea nitrogen (BUN), glutamate oxaloacetate transaminase (GOT), glutamate pyruvate transaminase (GPT), and superoxide dismutase (SOD). At the same time, ~1 g (n = 4) and 2 g (n = 10) rectal swab samples for individual animals were randomly collected for short-chain fatty acid analysis and gut microbiota analysis, respectively. Samples were temporally kept on dry ice and then stored in an ultra-low temperature freezer until further analysis.
Independent Samples T-Test program in IBM SPSS 22.0 statistical software was used for data statistical analysis and each animal served as the experimental unit. The probability value of p < 0.05 was considered significant.
Microbial genome DNA was extracted from the fecal samples using the DNeasy PowerLyzer PowerSoil Kit (Qiagen, Germantown, MD, USA), following the manufacturer's instructions. DNA concentration and purity were decided by NanoDrop One (Thermo Fisher Scientific, Madison, WI, USA) and then diluted to 20 ng/μL for downstream application.
The 16S rRNA gene hypervariable regions V3–V4 were used to identify bacteria and were amplified using primers 341F (5′-ACTCCTACGGGAGGCAGCA-3′) and 806R (5′-GGACTACHVGGGTWTCTAAT-3′). PCR reaction conditions are initial denaturation at 98°C for 2 min, followed by 30 cycles, including denaturation at 98°C for 15 s, annealing at 55°C for 30 s, and extension at 72°C for 30 s, with a final extension at 72°C for 5 min and 4°C hold. Agarose gel (1.2%) electrophoresis was applied to assess the success of PCR reactions. In addition, VAHTS DNA Clean Beads (Vazyme Biotech, Nanjing, Jiangsu, China) and Quant-iT PicoGreen dsDNA Assay Kit (Invitrogen, Carlsbad, CA, USA) were used to purify and quantify the amplicons, respectively. Purified PCR amplicons were then pooled together to generate a sequencing library. Agilent 2100 Bioanalyzer (Agilent, Santa Clara, CA, USA) and the Quant-iT PicoGreen dsDNA Assay Kit were applied to detect the quality and the concentration of the library, respectively. To detect potential bias introduced during PCR amplification and the MiSeq run, a mock community [ZymoBIOMICS™Microbial Community Standard (Zymo, Irvine, CA, USA)] was included in the sequencing library as a standard. Finally, the library was sequenced on the Illumina MiSeq sequencer with MiSeq Reagent Kit V3 (600 cycles) to generate paired-end reads.
The fastq files downloaded from the Illumina sequencer were analyzed using the QIIME2 (2019.4 release) microbiome bioinformatics platform (10). QIIME 2 plugin DADA2 processed sequencing data including quality control, denoising, merging, and removing chimera as well as singleton, and generated a feature table for the downstream analysis (11). Greengenes reference database (V13_8) trained Naive Bayes classifier was used to annotate sequences (12, 13).
Alpha diversity and beta diversity were estimated in QIIME2 at a sub-sampling depth of 62380 sequences for each sample. The analysis of similarity (ANOSIM) was performed to compare the dissimilarity between the treatments. In addition, the most differentially abundant bacteria between treatments were identified by LEfSe (Linear discriminant analysis Effect Size, LDA score > 2) at each taxonomic rank. Random forest R package with default setting was used to identify microbial signatures that best differentiate treatments at the feature level (14).
Experimental data such as BW was provided in Supplementary Table S3. The results indicated that the LPF diet numerically improved average daily gain (p = 0.392) and significantly improved the TP concentration (p = 0.002) as well as SOD (p = 0.017) compared with the NC diet (Figure 1). We also found that the LPF diet had no significant impacts on BUN, GPT, and GOT (Supplementary Table S4).
Figure 1. Effects of LPF diet on average daily gain, serum indexes, and fecal SCFAs of nursery pigs compared with NC diet. NC diet: basal diet, LPF diet: NC + 10% Lactobacillus plantarum and Pediococcus acidilactici co-fermented feed.
As shown in Figure 1, the LPF diet numerically improved the concentrations of propionate, isobutyrate, isovalerate, as well as valerate (p > 0.05) and significantly improved acetate (p = 0.020), butyrate (p = 0.017), and total SCFAs (p = 0.035) compared with NC diet (more detailed information are provided in Supplementary Table S5).
The gut microbiota alpha diversity was measured by the Shannon index, Observed_species, and Chao1, however, there were no significant differences between the treatments (Supplementary Figure S1). Beta diversity, including Bray-Curtis, Jaccard, Weighted Unifrac, and Unweighted Unifrac distances, was used to determine gut microbiota structural changes in response to co-fermented feed (Figure 2). The gut microbiota profiles of the NC and LPF groups were distinctly different. Principal coordinate analysis (PCoA) based on Bray-Curtis dissimilarity and Jaccard distance showed remarkable clusters for each experimental group. PCoA based on the Weighted and Unweighted Unifrac distances also revealed the distinct changes induced by co-fermented feed. The analysis of similarities (ANOSIM) confirmed the pattern that the swine gut microbiome was significantly different between NC and LPF treatments (Bray-Curtis: R = 0.38, P = 0.002; Jaccard: R = 0.43, P = 0.001; Weighted Unifrac: R = 0.28, P = 0.001; and Unweighted Unifrac: R = 0.54, P = 0.001).
Figure 2. LPF diet modulated gut microbiota beta diversity based on (A) Bray-Curtis, (B) Jaccard, (C) Weighted UniFrac, and (D) Unweighted UniFrac distances. The analysis of similarity (ANOSIM) was applied to estimate the dissimilarity between NC and LPF treatments. NC diet: basal diet, LPF diet: NC + 10% Lactobacillus plantarum and Pediococcus acidilactici co-fermented feed.
At the phylum level, the two treatment groups had a similar pattern that the dominant phyla Firmicutes and Bacteroidetes accounted for more than 95% of total sequences (Figure 3A). The top 15 bacteria at the family level are shown in Figure 3B, the most represented bacteria are Prevotellaceae, Clostridiaceae, and Ruminococcaceae in both groups. But the subdominant gut microbiota component varied at different treatments. For example, S24-7 and Streptococcaceae, the subdominant gut microbiota component in NC (10.7 and 7.4%, respectively) group, strikingly decreased in the LPF (3.8 and 3.1%, respectively) treatment. In addition, the relative abundance of Veillonellaceae was dramatically promoted by the LPF diet compared to that in the NC diet (7.5 vs. 1.9%). At the genus level (Figure 3C), Prevotella is the predominant bacteria in both groups (NC: 20.1% and LPF: 18.7%) among the top 15 genera. The relative abundance of Lactobacillus (8.6 vs. 3.8%), Roseburia (4.2 vs. 1.6%), Gemmiger (3.5 vs. 1.4%), Megasphaera (3.8 vs. 0.3%), and Faecalibacterium (2.7 vs. 1.3%) were largely enriched by the LPF diet compared to the NC diet. However, Streptococcus (7.2 vs. 2.9%) dramatically decreased in the LPF treatment compared to the NC group.
Figure 3. Gut microbiota compositions at (A) phylum, (B) family, and (C) genus levels for each treatment. NC diet: basal diet, LPF diet: NC + 10% Lactobacillus plantarum and Pediococcus acidilactici co-fermented feed.
A heatmap with cluster analysis showed the abundance of the top 50 bacterial taxa at the genus level, revealing visible compositional differences between piglets that received LPF feed and those fed the NC diet (Figure 4). Complete linkage hierarchical clustering based on Euclidean distance generated a separation of LPF and NC treatments, except for one sample from the LPF group joined the cluster containing samples from the NC group.
Figure 4. Heatmap of the relative abundance of top 50 genera. Clusters based on Euclidean distance using complete linkage clustering. NC diet: basal diet, LPF diet: NC + 10% Lactobacillus plantarum and Pediococcus acidilactici co-fermented feed.
We next performed LEfSe analysis to detect the most differentially abundant bacterial taxa between the NC and the LPF groups. A total of 44 represented bacterial taxa were identified (Figure 5A). Many biomarker genera like Megasphaera, Roseburia, Faecalibacterium, Blautia, Selenomonas, Dialister, Acidaminococcus, Ruminococcus, and Bifidobacterium were significantly more abundant in the LPF group compared with the NC group, whereas the relative abundance of Bacteroides was distinctly decreased by the LPF diet (Figure 5B).
Figure 5. (A) Linear discriminant analysis effect size (LefSe) of significantly different relative abundant bacterial taxa between groups. (B) Relative abundance of the important bacteria selected by LEfSe. NC diet: basal diet, LPF diet: NC + 10% Lactobacillus plantarum and Pediococcus acidilactici co-fermented feed.
For the other bacteria such as Gemmiger, Phascolarctobacterium, Peptococcus, Collinsella, Butyricicoccus, Oribacterium, Dorea, Bulleidia, Mitsuokella, Veillonellaceae, OPB56, Tremblayales, Actinobacteria, Coriobacteriia, Coriobacteriales, Coriobacteriaceae, Actinobacteria, Campylobacterales, Epsilonproteobacteria, Bifidobacteriales, and Bifidobacteriaceae were also higher in the LFP group, whereas the NC group had significantly more abundant Epulopiscium, Devosia, Anaerovorax, Parabacteroides, Bacteroidia, Ruminococcaceae, Lachnospiraceae, Mogibacteriaceae, Rhodobacterales, Hyphomicrobiaceae, Bacteroidaceae, RF16, and Rikenellaceae.
Microbial signatures that best differentiate the NC and LPF treatments were identified by random forest at the species level. The relative abundances of the top 500 bacterial features were included in the random forest model and the top 20 bacterial features that best predicted treatment are listed in Figure 6A. The relative abundances of these features for individual piglets are visualized on a heatmap (Figure 6B). Two members of Lactobacillus (Features #50 and #124) and Blautia (Features #224 and #440) were more abundant in the LPF group.
Figure 6. Gut microbiota signature of the pigs fed with LPF diet was determined by Random Forest. (A) Top 20 most predictive features that differentiate LPF-fed pigs from those fed with NC diet. (B) Heatmap shows the relative abundance of Features (log 10 transformed) selected by Random Forest. NC diet: basal diet, LPF diet: NC + 10% Lactobacillus plantarum and Pediococcus acidilactici co-fermented feed.
In the current study, the supplementation of 10% Lactobacillus plantarum and Pediococcus acidilactici co-fermented feed numerically increased the ADG, which is in accordance with previous reports that dietary fermented feed exhibited beneficial effects on swine production (15–18). However, several studies showed inconsistent results on the effects of fermented feed on growth performance. For example, Le et al. reported that Lactobacillus reuteri fermented wheat failed to improve the growth performance of weaned piglets (19). Liu et al. also observed that Bacillus subtilis fermented corn bran had no effects on ADFI and ADG of finishing pigs (20). The contradictory results could, at least in part, be explained by the different experimental animal ages and the different composition of fermented substrates, probiotic strains, as well as addition amount applied to the diet.
Weaning is the most stressful event in pig life, which could cause adverse impacts on gut health, such as villous atrophy, crypt hyperplasia, increased gut permeability, and intestinal inflammation (21). However, the SCFAs are considered beneficial to the gut and thus could help reduce weaning stress. A study conducted by Diao et al. demonstrated that SCFAs improved intestinal barrier function and reduced E. coli count in the ileal digesta in weaned piglets. Other studies confirmed that SCFAs help maintains gut barrier integrity (22, 23), which prohibits pathogens, toxins, or food proteins to pass into the blood. In addition, SCFAs have beneficial immune system effects in the intestinal mucosa (24, 25). Butyrate, a four-carbon short-chain fatty acid, is a primary energy source for intestinal epithelial cells and is anti-inflammatory (26). A study showed that butyrate improved the growth performance of weaning pigs fed diets containing 0.5% benzoic acid (27). Researchers also found that a mixture of SCFAs (propionic and formic) and capric acid significantly improved the growth performance of piglets (28). Overall, these findings suggested that SCFAs could reduce the detrimental effects of weaning stress and improve the growth performance of piglets. In the current study, the significantly increased acetate, butyrate, and total SCFAs may contribute to improved growth performance.
Consistent with previous studies (27, 29), our data showed that the Firmicutes and Bacteroidetes were the two dominant phyla and Prevotella was the most abundant genus in the gut microbiota of nursery piglets. The abundance of Prevotella might be linked with the diet style. Studies have shown that the relative abundance of Prevotella strikingly increased after the dietary transition from sow milk to corn/soybean meal-based diets (27). A human study also found that the relative abundance of Prevotella was associated with dietary habits and a high percentage of Prevotella could be a consequence of high fiber intake, improving metabolic energy absorption from consumed plant polysaccharides (30). These indicated that the gut microbiota coevolved with the diet.
LEfSe analysis was applied to identify the microbiota that was significantly changed by the LPF diet. Compared with the NC diet, LPF treatment significantly enriched SCFA-producing bacteria such as Megasphaera, Roseburia, Faecalibacterium, Blautia, Selenomonas, Dialister, Acidaminococcus, Ruminococcus, and Bifidobacterium (31–41). Some of those bacteria also have other beneficial functions, for example, Megasphaera could enhance large intestine functions and effectively prevent hyper-lactate accumulation-related diarrhea (42), Roseburia has benefits from immune modulation to inflammatory regulation (43), Blautia and Faecalibacterium had anti-inflammatory functions to help support gut health (44–46). In addition, this study indicated that the LPF diet selectively decreased the relative abundance of Bacteroides, an acetate producer (47). It may be that co-fermented feed additive prohibited the growth of Bacteroides directly or assisted the growth of antagonistic species against Bacteroides within the gastrointestinal tract. Taken together, our data indicated that the LPF diet improved the relative abundance of bacteria with potential probiotic properties.
In conclusion, this study demonstrated that the Lactobacillus plantarum and Pediococcus acidilactici co-fermented feed additive improved weaning pigs' growth performance, modulated gut microbiota diversity and composition, leading to enrichments of SCFA-producing bacteria.
The datasets presented in this study can be found in online repositories. The names of the repository/repositories and accession number(s) can be found at: https://www.ncbi.nlm.nih.gov/, https://www.ncbi.nlm.nih.gov/bioproject/PRJNA891080.
The animal study was reviewed and approved by Institute of Animal Husbandry and Veterinary Medicine of Hebei Province Animal Care and Use Committee.
YY, XWe, and QZ conceived and designed this experiment. YY, GY, XM, XWa, GL, ZZ, and SZ collected rectal samples and growth performance data. XWe and YY analyzed the data. XWe, YY, and QZ drafted and revised the manuscript with input from GY, XM, ZZ, SZ, GL, and XWa. All authors read and approved the final manuscript.
This work was supported by Hebei Swine Innovation Team Efficient Feeding Management Positions of Modern Agro-industry Technology Research System (HBCT2018110202), Application and Popularization of Soybean Protein Reduction Replacement Technology for Swine Feed (Hebei Agricultural Science22038), and Basic Research Funds of Hebei Academy of Agriculture and Forestry Sciences (2021110101).
The authors declare that the research was conducted in the absence of any commercial or financial relationships that could be construed as a potential conflict of interest.
All claims expressed in this article are solely those of the authors and do not necessarily represent those of their affiliated organizations, or those of the publisher, the editors and the reviewers. Any product that may be evaluated in this article, or claim that may be made by its manufacturer, is not guaranteed or endorsed by the publisher.
The Supplementary Material for this article can be found online at: https://www.frontiersin.org/articles/10.3389/fvets.2022.1076906/full#supplementary-material
Supplementary Figure S1. Alpha diversity for different dietary supplements was measured by (A) Shannon index, (B) Observed species, and (C) Chao1.
Supplementary Table S1. Composition and nutrient levels of basal diets (air-dry basis).
Supplementary Table S2. Composition of Lactobacillus plantarum and Pediococcus acidilactici co-fermented feed.
Supplementary Table S3. Effects of fermented feed additive on growth performances of nursery pigs.
Supplementary Table S4. Effects of fermented feed additive on serum chemistry of nursery pigs.
Supplementary Table S5. Effects of fermented feed additive on short chain fatty acid concentration in fecal samples.
1. Karovičová Z, Kohajdova J. Fermentation of cereals for specific purpose. J Food Nutr Res. (2007) 46:51–7.
2. Nkhata SG, Ayua E, Kamau EH, Shingiro JB. Fermentation and germination improve nutritional value of cereals and legumes through activation of endogenous enzymes. Food Sci Nutr. (2018) 6:2446–58. doi: 10.1002/fsn3.846
3. Missotten JA, Michiels J, Ovyn A, De Smet S, Dierick NA. Fermented liquid feed for pigs. Arch Anim Nutr. (2010) 64:437–66. doi: 10.1080/1745039X.2010.512725
4. Sugiharto S, Ranjitkar S. Recent advances in fermented feeds towards improved broiler chicken performance, gastrointestinal tract microecology and immune responses: a review. Anim Nutr. (2019) 5:1–10. doi: 10.1016/j.aninu.2018.11.001
5. Zhou H, Wang C, Ye J, Chen H, Tao R. effects of dietary supplementation of fermented ginkgo biloba L. residues on growth performance, nutrient digestibility, serum biochemical parameters and immune function in weaned piglets. Anim Sci J. (2015) 86:790–9. doi: 10.1111/asj.12361
6. Wang N, Chen Q, Le G, Shi Y, Sun J. Effect of lactic acid fermented soyabean meal on the growth performance, intestinal microflora and morphology of weaned piglets. J Anim Feed Sci. (2007) 16:75. doi: 10.22358/jafs/66728/2007
7. Kiers JL, Meijer JC, Nout MJ, Rombouts FM, Nabuurs MJ, van der Meulen J. Effect of fermented soya beans on diarrhoea and feed efficiency in weaned piglets. J Appl Microbiol. (2003) 95:545–52. doi: 10.1046/j.1365-2672.2003.02011.x
8. Missotten JA, Michiels J, Degroote J, De Smet S. Fermented liquid feed for pigs: an ancient technique for the future. J Anim Sci Biotechnol. (2015) 6:4. doi: 10.1186/2049-1891-6-4
10. Bolyen E, Rideout JR, Dillon MR, Bokulich NA, Abnet CC, Al-Ghalith GA, et al. Reproducible, interactive, scalable and extensible microbiome data science using qiime 2. Nat Biotechnol. (2019) 37:852–7. doi: 10.1038/s41587-019-0209-9
11. Callahan BJ, McMurdie PJ, Rosen MJ, Han AW, Johnson AJ, Holmes SP. Dada2: high-resolution sample inference from illumina amplicon data. Nat Methods. (2016) 13:581–3. doi: 10.1038/nmeth.3869
12. Bokulich NA, Kaehler BD, Rideout JR, Dillon M, Bolyen E, Knight R, et al. Optimizing taxonomic classification of marker-gene amplicon sequences with qiime 2's Q2-feature-classifier plugin. Microbiome. (2018) 6:90. doi: 10.1186/s40168-018-0470-z
13. McDonald D, Price MN, Goodrich J, Nawrocki EP, DeSantis TZ, Probst A, et al. An improved greengenes taxonomy with explicit ranks for ecological and evolutionary analyses of bacteria and archaea. ISME J. (2012) 6:610–8. doi: 10.1038/ismej.2011.139
14. Qi Y. Random Forest for Bioinformatics. Ensemble Machine Learning. New York, NY: Springer (2012). p. 307–23.
15. Feng J, Liu X, Xu Z, Lu Y, Liu Y. The effect of Aspergillus oryzae fermented soybean meal on growth performance, digestibility of dietary components and activities of intestinal enzymes in weaned piglets. Anim Feed Sci Technol. (2007) 134:295–303. doi: 10.1016/j.anifeedsci.2006.10.004
16. Canibe N, Miettinen H, Jensen B. Effect of adding Lactobacillus plantarum or a formic acid containing-product to fermented liquid feed on gastrointestinal ecology and growth performance of piglets. Livest Sci. (2008) 114:251–62. doi: 10.1016/j.livsci.2007.05.002
17. Mukherjee R, Chakraborty R, Dutta A. Role of fermentation in improving nutritional quality of soybean meal - a review. Asian Aust J Anim Sci. (2016) 29:1523–9. doi: 10.5713/ajas.15.0627
18. Wang C, Lin C, Su W, Zhang Y, Wang F, Wang Y, et al. Effects of supplementing sow diets with fermented corn and soybean meal mixed feed during lactation on the performance of sows and progeny. J Anim Sci. (2018) 96:206–14. doi: 10.1093/jas/skx019
19. Le MH, Galle S, Yang Y, Landero JL, Beltranena E, Ganzle MG, et al. Effects of feeding fermented wheat with lactobacillus reuteri on gut morphology, intestinal fermentation, nutrient digestibility, and growth performance in weaned pigs. J Anim Sci. (2016) 94:4677–87. doi: 10.2527/jas.2016-0693
20. Liu P, Zhao J, Guo P, Lu W, Geng Z, Levesque CL, et al. Dietary corn bran fermented by Bacillus subtilis Ma139 decreased gut cellulolytic bacteria and microbiota diversity in finishing pigs. Front Cell Infect Microbiol. (2017) 7:526. doi: 10.3389/fcimb.2017.00526
21. Wei X, Tsai T, Howe S, Zhao J. Weaning induced gut dysfunction and nutritional interventions in nursery pigs: a partial review. Animals. (2021) 11. doi: 10.3390/ani11051279
22. Peng L, Li ZR, Green RS, Holzman IR, Lin J. butyrate enhances the intestinal barrier by facilitating tight junction assembly via activation of amp-activated protein kinase in Caco-2 cell monolayers. J Nutr. (2009) 139:1619–25. doi: 10.3945/jn.109.104638
23. Brestoff JR, Artis D. Commensal bacteria at the interface of host metabolism and the immune system. Nat Immunol. (2013) 14:676–84. doi: 10.1038/ni.2640
24. Furusawa Y, Obata Y, Fukuda S, Endo TA, Nakato G, Takahashi D, et al. Commensal microbe-derived butyrate induces the differentiation of colonic regulatory T cells. Nature. (2013) 504:446–50. doi: 10.1038/nature12721
25. Arpaia N, Campbell C, Fan X, Dikiy S, van der Veeken J, deRoos P, et al. Metabolites produced by commensal bacteria promote peripheral regulatory T-cell generation. Nature. (2013) 504:451–5. doi: 10.1038/nature12726
26. van der Beek CM, Dejong CHC, Troost FJ, Masclee AAM, Lenaerts K. Role of short-chain fatty acids in colonic inflammation, carcinogenesis, and mucosal protection and healing. Nutr Rev. (2017) 75:286–305. doi: 10.1093/nutrit/nuw067
27. Wei X, Bottoms KA, Stein HH, Blavi L, Bradley CL, Bergstrom J, et al. Dietary organic acids modulate gut microbiota and improve growth performance of nursery pigs. Microorganisms. (2021) 9:110. doi: 10.3390/microorganisms9010110
28. Hanczakowska E, Szewczyk A, Swiatkiewicz M, Okoń K. Short- and medium-chain fatty acids as a feed supplement for weaning and nursery pigs. Pol J Vet Sci. (2013) 16:647–54. doi: 10.2478/pjvs-2013-0092
29. Wei X, Tsai T, Knapp J, Bottoms K, Deng F, Story R, et al. Zno modulates swine gut microbiota and improves growth performance of nursery pigs when combined with peptide cocktail. Microorganisms. (2020) 8:146. doi: 10.3390/microorganisms8020146
30. De Filippo C, Cavalieri D, Di Paola M, Ramazzotti M, Poullet JB, Massart S, et al. Impact of diet in shaping gut microbiota revealed by a comparative study in children from europe and rural Africa. Proc Natl Acad Sci USA. (2010) 107:14691–6. doi: 10.1073/pnas.1005963107
31. Yoshikawa S, Araoka R, Kajihara Y, Ito T, Miyamoto H, Kodama H. Valerate production by Megasphaera elsdenii isolated from pig feces. J Biosci Bioeng. (2018) 125:519–24. doi: 10.1016/j.jbiosc.2017.12.016
32. Tamanai-Shacoori Z, Smida I, Bousarghin L, Loreal O, Meuric V, Fong SB, et al. Roseburia Spp: a marker of health? Fut Microbiol. (2017) 12:157–70. doi: 10.2217/fmb-2016-0130
33. Machiels K, Joossens M, Sabino J, De Preter V, Arijs I, Eeckhaut V, et al. A Decrease of the butyrate-producing species Roseburia hominis and Faecalibacterium prausnitzii defines dysbiosis in patients with ulcerative colitis. Gut. (2014) 63:1275–83. doi: 10.1136/gutjnl-2013-304833
34. Ferreira-Halder CV, Faria AVS, Andrade SS. Action and function of Faecalibacterium prausnitzii in health and disease. Best Pract Res Clin Gastroenterol. (2017) 31:643–8. doi: 10.1016/j.bpg.2017.09.011
35. Paynter MJ, Elsden SR. Mechanism of propionate formation by Selenomonas ruminantium, a rumen micro-organism. J Gen Microbiol. (1970) 61:1–7. doi: 10.1099/00221287-61-1-1
36. Taylor H, McDonald J, Serrano Contreras J, Li J, Marchesi J, Hart A. Dop08 deep remission in paediatric Crohn's disease is associated with increased abundance of dialister species and increased valerate. J Crohns Colitis. (2020) 14(Suppl_1):S045–6. doi: 10.1093/ecco-jcc/jjz203.047
37. Buckel W, Barker HA. Two pathways of glutamate fermentation by anaerobic bacteria. J Bacteriol. (1974) 117:1248–60. doi: 10.1128/jb.117.3.1248-1260.1974
38. Oh JK, Vasquez R, Kim SH, Hwang IC, Song JH, Park JH, et al. Multispecies probiotics alter fecal short-chain fatty acids and lactate levels in weaned pigs by modulating gut microbiota. J Anim Sci Technol. (2021) 63:1142–58. doi: 10.5187/jast.2021.e94
39. Ze X, Duncan SH, Louis P, Flint HJ. Ruminococcus bromii is a keystone species for the degradation of resistant starch in the human colon. ISME J. (2012) 6:1535–43. doi: 10.1038/ismej.2012.4
40. Sun M, Wu W, Liu Z, Cong Y. Microbiota metabolite short chain fatty acids, Gpcr, and inflammatory bowel diseases. J Gastroenterol. (2017) 52:1–8. doi: 10.1007/s00535-016-1242-9
41. Fukuda S, Toh H, Taylor TD, Ohno H, Hattori M. Acetate-producing bifidobacteria protect the host from enteropathogenic infection via carbohydrate transporters. Gut Microbes. (2012) 3:449–54. doi: 10.4161/gmic.21214
42. Ushida K, Kishimoto A, Piao SJ, Itoh M, Shiga A, Nakanishi N, et al. An epidemiological survey on pigs showing symptoms of infectious enteric diseases and dyspepsia in Japan. Anim Sci J. (2009) 80:556–61. doi: 10.1111/j.1740-0929.2009.00671.x
43. Nie K, Ma K, Luo W, Shen Z, Yang Z, Xiao M, et al. Roseburia intestinalis: a beneficial gut organism from the discoveries in genus and species. Front Cell Infect Microbiol. (2021) 11:757718. doi: 10.3389/fcimb.2021.757718
44. Sokol H, Pigneur B, Watterlot L, Lakhdari O, Bermúdez-Humarán LG, Gratadoux JJ, et al. Faecalibacterium prausnitzii is an anti-inflammatory commensal bacterium identified by gut microbiota analysis of crohn disease patients. Proc Natl Acad Sci USA. (2008) 105:16731–6. doi: 10.1073/pnas.0804812105
45. Quévrain E, Maubert MA, Michon C, Chain F, Marquant R, Tailhades J, et al. Identification of an anti-inflammatory protein from Faecalibacterium prausnitzii, a commensal bacterium deficient in crohn's disease. Gut. (2016) 65:415–25. doi: 10.1136/gutjnl-2014-307649
46. Hosomi K, Saito M, Park J, Murakami H, Shibata N, Ando M, et al. Oral administration of Blautia wexlerae ameliorates obesity and type 2 diabetes via metabolic remodeling of the gut microbiota. Nat Commun. (2022) 13:4477. doi: 10.1038/s41467-022-32015-7
Keywords: fermented feed, Lactobacillus plantarum, Pediococcus acidilactici, nursery pig, growth performance, gut microbiota
Citation: Yang Y, Yan G, Meng X, Wang X, Zhao Z, Zhou S, Li G, Zhang Q and Wei X (2022) Effects of Lactobacillus plantarum and Pediococcus acidilactici co-fermented feed on growth performance and gut microbiota of nursery pigs. Front. Vet. Sci. 9:1076906. doi: 10.3389/fvets.2022.1076906
Received: 22 October 2022; Accepted: 22 November 2022;
Published: 12 December 2022.
Edited by:
Wen-Chao Liu, Guangdong Ocean University, ChinaReviewed by:
Na Li, Beijing University of Chinese Medicine, ChinaCopyright © 2022 Yang, Yan, Meng, Wang, Zhao, Zhou, Li, Zhang and Wei. This is an open-access article distributed under the terms of the Creative Commons Attribution License (CC BY). The use, distribution or reproduction in other forums is permitted, provided the original author(s) and the copyright owner(s) are credited and that the original publication in this journal is cited, in accordance with accepted academic practice. No use, distribution or reproduction is permitted which does not comply with these terms.
*Correspondence: Xiaoyuan Wei, eHcwMTBAdWFyay5lZHU=; Qiuliang Zhang, eG1zenFsQDE2My5jb20=
†These authors have contributed equally to this work
Disclaimer: All claims expressed in this article are solely those of the authors and do not necessarily represent those of their affiliated organizations, or those of the publisher, the editors and the reviewers. Any product that may be evaluated in this article or claim that may be made by its manufacturer is not guaranteed or endorsed by the publisher.
Research integrity at Frontiers
Learn more about the work of our research integrity team to safeguard the quality of each article we publish.