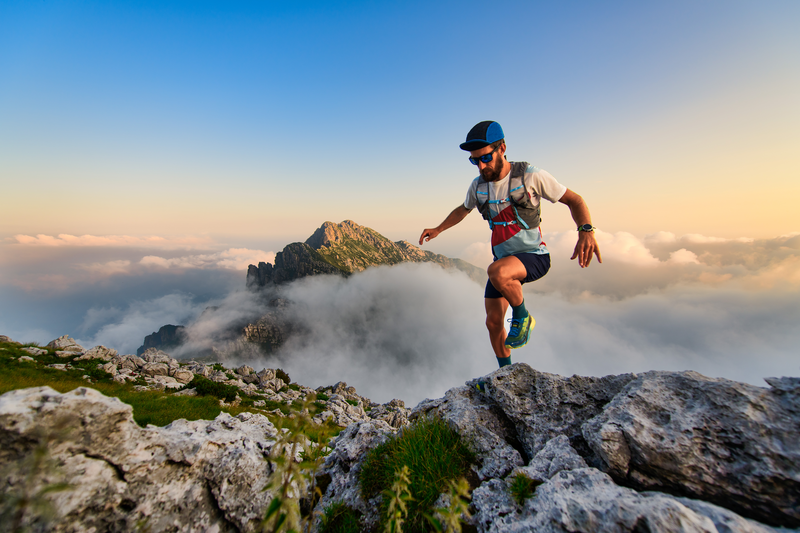
95% of researchers rate our articles as excellent or good
Learn more about the work of our research integrity team to safeguard the quality of each article we publish.
Find out more
REVIEW article
Front. Vet. Sci. , 20 December 2022
Sec. Comparative and Clinical Medicine
Volume 9 - 2022 | https://doi.org/10.3389/fvets.2022.1072730
This article is part of the Research Topic Rising Stars in Comparative and Clinical Medicine: 2022 View all 29 articles
Echinococcosis is a neglected, WHO-listed cyclozoonotic parasitic disease that is caused by a number of species belonging to the genus Echinococcus. This disease is widespread across the globe, resulting in heavy economic losses for farmers and cystic disease in aberrant human hosts. This review paper briefly discussed taxonomy, a brief history, the magnitude of economic losses, host spectrum and life cycle, risk factors, and clinical manifestations. Furthermore, the copro- and sero-ELISA-based prevalence of echinococcosis on different continents was summarized. Finally, the authors analyzed the frequency and use of molecular epidemiology in the taxonomy of Echinococcus species based on molecular markers. This review will serve as a quick reference to Echinococcus.
Infectious diseases, including parasitic infestations, are important health problems in both animals and humans (1–4), which cause economic losses and severe illness (5–10). Parasites are capable of causing acute, chronic, and debilitating types of diseases, leading to production losses in animals (11–15), and many pieces of research have shown the positive role of alternative/complementary medicine in treating parasitic diseases. Echinococcosis is a neglected silent cyclozoonotic parasitic disease caused by the metacestode stages of the genus Echinococcus belonging to the family Taeniidae, affecting a wide spectrum of animal species, including livestock and wildlife, and it also has zoonotic implications (16–19). The genus Echinococcus contains at least nine valid species with different strains and genotypes, namely E. granulosus, E. multilocularis, E. vogeli, E. oligarthra, E. canadensis, E. equinus, E. felidis, E. shiquicus, and E. ortleppi, which are important ones (20, 21).
Echinococcosis is included in the World Health Organization's (WHO) list of neglected tropical diseases. This disease is widespread in its distribution and persists in a variety of environmental conditions in temperate, circumpolar, tropical, and sub-tropical regions (Figure 1). The parasite survives well in arid climatic conditions and subpolar oceanic environmental conditions. Eurasia, Australia, Africa, and South America have a very high disease prevalence, and 50 million people are infected with the disease worldwide (22, 23). Cystic echinococcosis (CE) encompasses a wide geographical area from the eastern parts of Asia to northern America and from the upper northern hemisphere to the southern countries of the African continent (24, 25).
Variable-sized cysts comprise the metacestode stages of E. granulosus, which are filled with a transparent liquid. The presence of this clear liquid led to the coining of the term “hydatids.” A number of scientists and physicians, including Hippocrates, Galen, Aretaeus, Wolckerus, and Bonet, described the different features of hydatids in their timelines. Some researchers described them as an accumulation of serum and mucus in between the laminar cell layers. Francesco Redi first provided evidence of hydatids' metacestode nature and reported that cysticerci could move like animals (26). Jacob Hartman, a professor of medicine at the University of Königsberg/Germany, confirmed the animal-like nature of cysticerci, describing it as a small, spherical structure with a metacestode bladder. Edward Tyson, a professor at Oxford, also found the motility of the hydatids of Cysticercus tenuicollis to be like that of living creatures. Simon Pallas in the Netherlands described hydatids as a distinct group of bladder worms with small bodies on the inner walls of hydatids. Later, Ephraim Goeze discovered tapeworm scoleces in these small bodies called brood capsules. In 1801, Karl Rudolphi introduced the word Echinococcus to the world of science (27).
In the mid-nineteenth century, two different forms of echinococcosis were identified: cystic echinococcosis (CE) and alveolar echinococcosis (AE). There remained a heated debate over the aetiological agents responsible for the causation of CE and AE. Two schools of thought emerged, one with “unicasts,” believing that both forms occur due to the same species of Echinococcus, and the other with “dualists,” claiming that two different species are responsible for causing two different forms of the disease. However, the understanding of the dualist school of thought was supported by Professor Adolf Possely. Clear experimental proof supporting the concepts of a dualist school of thought was provided in 1928 by Posselt in his work “Der Alveolarechinokokkus und seine Chirurgie” (alveolar echinococcus and its surgery). He provided plausible arguments that two different species cause CE and AE, as their morphology, anatomy, and clinical signs clearly differ from each other (28).
Echinococcosis is a neglected tropical disease of public health concern and has serious economic implications. Out of nine known species of Echinococcus, E. granulosus and E. multilocularis pose significant threats to human and animal health in addition to substantial economic losses (29). Treatment costs, production losses, and mortality in infected animals and aberrant human hosts are economic and social calamities caused by this infection. The cosmopolitan distribution of these ailments has led to losses of USD 3 billion annually (30). It has been reported that echinococcosis has led to annual economic losses of USD 212.35 million in India, USD 232.3 million in Iran, and USD 7.708 million in Turkey (31). Followed by Fasciola hepatica, CE is the most important cause of condemnation of livestock viscera. It has been estimated that in Chile, CE leads to economic losses amounting to USD 14.35 million per year (32). Echinococcosis causes substantial economic losses in Pakistan. Losses due to the disease have been estimated at USD 276.20 per 100 infected goats and sheep and USD 165.72 per 100 infected large ruminants and camels. There are losses in terms of quantity and quality of milk, wool, and meat, retarded growth, decreased fertility, and carcass condemnation (33).
Echinococcus species enjoy two different host species: the intermediate host and the definitive host. The definitive hosts of this cestode are the carnivores, especially the dogs that carry this parasite in their small intestines. Both wild and domesticated ruminants, camels, and humans serve as intermediate hosts of different Echinococcus species (23, 34, 35). The life cycles of different species of Echinococcus are depicted in Figure 2. All of the species enjoy a heteroxenic life cycle. The size of adult worms varies from 2 to 11 mm, with 2–7 proglottid segments. Scolex has two rows of rostellar hooks. Each proglottid has a single genital opening, and the mature segment is called the penultimate segment. After fertilization, eggs are fully developed in the uterus and released into the environment along with dog feces. Contaminated water or vegetation ingested by the intermediate host leads to the release of oncospheres from embryonated eggs that penetrate the intestinal wall and spread to various body tissues through circulation. Cyst formation primarily occurs in the lungs and liver, and such infected tissue, when eaten by the canids, releases protoscoleces. They develop into mature worms, completing the life cycle (36–39).
The dynamics of the completion of the life cycle of the Echinococcus species are well supported by the presence of dogs. Home slaughtering practices and feeding the dogs with hydatid cysts facilitate the completion of the parasite's life cycle. Younger children have a great affection for pets, including dogs. Children of such an age are likely at greater risk of suffering from echinococcosis. Regions with widespread grassland are best suited for grazing sheep, goats, and cattle. The pastoral dogs and the nomadic lifestyle play a role in the completion of the synanthropic life cycle of E. granulosus (40). Lack of alertness about the life cycle, poor hygiene, open slaughtering practices, close involvement of ruminants and dogs, and improper disposal of condemned carcasses/offal support the spread of echinococcosis (33). In South Asian countries like Pakistan and India, the slaughtering of food animals is carried out either in an open environment or in slaughterhouses that are easy for dogs to access. Such access by the dogs and ingestion of the offal leads to the completion of the Echinococcus life cycle (18). A general overview of risk factors for E. granulosus infection in dogs and humans is described in Figures 3, 4.
The burden of human echinococcosis can be expressed in disability-adjusted life years (DALYs), which are used to express the burden of human cases of CE and AE. The annual global burden of AE is approximately 18,200 cases, which leads to almost 666,000 DALYs (41). With regard to CE, the annual global burden amounts to 188,000 new cases every year and leads to 184,000 DALYs (42).
Echinococcosis can appear in four different forms: cystic echinococcosis (CE), alveolar echinococcosis (AE), polycystic echinococcosis (PE), and unicystic echinococcosis (UE), caused by E. granulosus, E. multilocularis, E. vogeli, and E. oligarthra, respectively. All of the species share the same common definitive hosts, which are the canids, except E. oligarthra, which has members of the family Felidae as the definitive host (43). Definitive hosts harbor adult worms in the small intestine and release the embryonated eggs of the parasite in the environment with feces. Echinococcus granulosus and E. multilocularis enjoy a wide range of intermediate hosts, including bovines, which harbor the larval stages of the parasite in their visceral organs after ingestion of the embryonated eggs (44, 45). Humans and monkeys serve as aberrant hosts for all species, and diseases may progress from asymptomatic to severe clinical diseases, which can eventually cause death (27, 46). The disease pattern of echinococcosis is usually asymptomatic in livestock, and the diagnosis is generally made on necropsy findings in the abattoir; however, it has serious implications regarding the condemnation of the carcasses. In human CE, clinical signs include asthenia, weight loss, epigastric pain, hepatomegaly, and cholestatic jaundice (47). It may become a fatal disease when the cysts rupture and their fluid contents and protoscoleces are drained into the peritoneal cavity, leading to anaphylactic shock (48).
Sequencing of mitochondrial cytochrome-c oxidase and NADH-dehydrogenase genes has revealed at least 10 genotypes (G1-G10) of CE (49). Echinococcus granulosus sensu lato species complex is a major veterinary and medical concern causing cystic echinococcosis (CE) in the target species across the globe. Genotyping results have indicated that E. granulosus sensu stricto (G1 common sheep strain, G2 Tasmania sheep strain, and G3 buffalo strain), E. equinus (G4 horse strain), E. ortleppi (G5 cattle strain), and E. canadensis (G6 camel strain, G7 pig strain, G8 cervid strain, G9 human strain, and G10 Fennoscandian cervid strain) are the members of this species complex (50–53). Most of the cysts are formed in the liver (70%) and the lungs (20%), while up to 10% are found in other body tissues like the brain, kidneys, marrow cavities of the bones, and ocular orbits. The chances of the formation of cysts in the central nervous system are only 1–2% of the total E. granulosus infections (54, 55). Apart from economic concerns, hydatidosis is a major public health issue as the hydatid cysts grow as unilocular fluid-filled bladders in the internal organs like the lungs and liver of humans (56).
There are a number of diagnostic techniques for echinococcosis, including ultrasonography, serological tests, e.g., enzyme-linked immunosorbent assay, immunoelectrophoresis, immunoblotting, indirect fluorescence antibody test (IFAT), and latex agglutination test (LAT), (57) and molecular methods, e.g., loop-mediated isothermal amplification (LAMP)-based techniques, and polymerase chain reaction (PCR). Each of these has its own advantages and limitations (58, 59).
For the diagnosis of Echinococcus infection, post-mortem analysis of the small intestine and arecoline purgation are reliable tools. Intestinal scraping, fecal sedimentation, and fecal centrifugal flotation methods have high sensitivity and are good indicators of worm burden in the host intestine. The morphology of the eggs of E. granulosus, E. multilocularis, and Taenia species resembles each other, suggesting that methods are not very sensitive and specific (60). The coproantigenic ELISA has also been another tool, as it can detect the E. granulosus antigen both in patent and prepatent stages. The sensitivity of this ELISA is higher for animals with a high infection burden. Another diagnostic tool to access the infection is PCR, which has high specificity and sensitivity (61). Cerebral hydatidosis is a rare form of echinococcosis and accounts for only 2% of total cases. The incidence of this form is higher in children because of frequent contact with pet dogs. However, adults can also get the infection. It is important to distinguish neurohydatidosis from other intracranial cysts when making a diagnosis. In this peculiar form, serological testing is usually not useful as far as diagnostic tools are concerned. The only reliable diagnostic technique is MRI to assess the size of the hydatid cyst and devise a plan to excise it (62) surgically.
Various techniques have been developed over the past 30 years to identify Echinococcus variants. However, several molecular techniques have been used in the past, such as PCR-RAPD (random amplification of polymorphic DNA), PCR-RFLP (restriction fragment length polymorphism), a Southern Blot approach, DNA fingerprinting, and dideoxy fingerprinting. PCR is the preferred method for parasite identification, molecular epidemiology research, and confirmation purposes (63).
Nested PCR and multiplex PCR are frequently used for the differential detection of different Echinococcus species. PCRs that are followed by sequencing help detect variations within species and genotypes (64). While sequencing is a time-consuming and skill-intensive method, it is also the most accurate method for identifying the species of Echinococcus and finding genetic variation.
Recently, approaches like loop-mediated isothermal amplification (LAMP) that are low-cost and simple to use have been created and tested (65). Due to the fact that DNA may be amplified using a straightforward water bath without the use of complicated instruments, LAMP is an ideal technology to utilize in low-resource settings where alveolar and cystic echinococcosis are common. However, because of its high sensitivity, this system can be tricked into giving false positives.
Contemporary methods using single-locus or multilocus microsatellite analysis have been developed to explore the genetic diversity, the population structure of parasites, and the geographic relatedness of Echinococcus species (66, 67).
For the identification of parasitic diseases, real-time PCR (qPCR) has a number of advantages over traditional PCR, including improved sensitivity and specificity, a shorter reaction time, and quantitative estimation of the amount of DNA in the sample (68, 69).
Researchers have long been interested in finding ways to break the life cycle of the Echinococcus species in order to decrease the spread of illness in both animals and people. Using anthelmintics and creating awareness among the masses about slaughter hygiene is one of the most important strategies to reduce the prevalence of echinococcosis. Limited treatment regimens are available for curing patients suffering from echinococcosis, and the focus is on giving benzimidazoles that have only parastiostatic activity. Oral administration over a long time has also led to the development of resistance to varying degrees in the metacestode stages of Echinococcus (47). Amphotericin-B, nitazoxanide, and isoflavones have also been reported to possess anti-Echinococcus activity, but the efficacy of these chemotherapeutic agents is not much higher (70). Imatinib, an anticancer agent, has been found highly effective in killing protoscoleces and metacestodes in vitro at a concentration of 25 μM. This drug was evaluated after keenly investigating the signaling pathways of Echinococcus (71). Pharmacotherapy poses different threats because of drug residues in milk and meat, which can lead to health issues for the end consumer. In addition, low dosage or frequent administration of anthelmintics leads to parasite drug resistance (36). Despite these limitations and drawbacks, vaccination remains a suitable and long-term solution against Echinococcus infection. Vaccine development is a crucial phase, and attention must be paid to developing multifunctional vaccines considering the circulating serotypes and genotypes of E. granulosus (72). Identifying the strain is key to implementing control strategies to curtail disease transmission. Echinococcus spp. continue to be a global problem despite our extensive knowledge of the organism, its control techniques, vaccine research, and clinical case management (73).
Eradication of this notorious infection has been a challenge for both developed and developing countries (74). However, the community's involvement in controlling the incidence of the disease is of high value. Zhang et al. proposed a number of strategies to decrease the number of future cases. Promotion and awareness seminars about the epidemiology of the disease, inspection of animal offal at the slaughterhouses, proper disposal, and condemnation of carcasses with cysts, monthly deworming of dogs with praziquantel, and ultrasonography of suspected patients and their treatment are important to highlight here (75). Killing stray dogs to prevent the shedding of embryonated eggs in the environment is another prevention strategy that was adopted in the past. A complete ban on home-slaughtering practices and rodent control programs must be institutionalized, as E. multilocularis targets rodents as its intermediate host (76). One alternative is to hire a Hydatid Disease Control Officer (HDCO), whose responsibilities would include registering dogs, dosing them monthly with dewormers, and educating the public about echinococcosis control programs (75).
Echinococcus granulosus infection is endemic in Southeast European countries, e.g., Bulgaria and Romania, and E. multilocularis infection is hyperendemic in France, Germany, Turkey, and Switzerland (77). G1 (the sheep strain) and G4 (the horse strain) are endemic in the United Kingdom and can be found in sheep, dogs, and horse/foxhounds, respectively (78, 79). Liver lesions due to E. multilocularis were revealed upon necropsy of nutria (Myocastor coypus) in a French wildlife park. There was evidence of contamination of the interior and exterior of the park, with fox feces contacting E. multilocularis. Later, the intestines of five other foxes shot in the park were found to harbor E. multilocularis. A coprological analysis of other definitive hosts like wildcats, bears, and wolves showed a 5.3% prevalence (80). An epidemiological study in Turkey between 2006 and 2010 revealed that CE is endemic in Turkey.
Of the 166 buffaloes inspected after slaughter, the organs of 10.24% of the buffaloes had CE. Females and older animals had a prevalence rate of 21.66 and 37.93% compared to males and younger animals, with prevalence rates of 3.77 and 4.38%, respectively. A statistically significant difference between sex and age was observed. There were hydatid cysts in both the liver and the lungs; however, the lungs were the predominant site of encystations, with 47.06% of all cyst formation detected, compared to the liver, which had 29.41% of all cyst cases. G1, G2, and G3 genotypes were found after performing mitochondrial cox1 sequencing analyses. Another study was conducted in Ankara, Turkey, to compare fecal sedimentation technique and fecal centrifugal flotation to harvest taeniid eggs from the feces, identify E. granulosus eggs through PCR, and determine the prevalence of E. granulosus infection in the study area. Of 100 fecal samples, 27 had eggs. Echinococcus granulosus-specific PCR was carried out on the positive samples, and 14 samples were positive, resulting in a prevalence of 51.85% (50). The sedimentation technique was found to be significantly better than the flotation method, as the earlier one detected eggs in 27 samples while the latter one detected only 10 samples as positive for the eggs.
An epidemiological study was conducted in 16 counties in Romania to determine the prevalence of echinococcosis in sheep and cattle in the country. Out of 643 sheep and 1,878 cattle examined for hydatidosis, 421 sheep and 754 cattle tested positive for the cysts, giving an overall prevalence of 65.6 and 40.1%, respectively. Germinal layers were collected, and DNA was extracted to obtain an idea of the genetic diversity in the isolates. 12S ribosomal DNA and cytochrome c oxidase were used as genetic markers. The genetic diversity analysis results showed a dominance of the G1 genotype in the E. granulosus sensu stricto complex (81). A similar type of genetic diversity analysis was performed in Serbia to find which genotypes prevail in intermediate hosts (sheep and cattle) of the E. granulosus sensu stricto complex. The sequence analysis of the cytochrome c oxidase subunit 1 (cox1) mitochondrial gene from germinal layers of hydatid cysts showed that G2 and G3 are prevalent in the country (82).
In Canada, only E. canadensis and E. multilocularis have been found to be prevalent, which cause CE and AE, respectively. Both of these parasitic species were confirmed to be circulating between wild canids like wolves and foxes, which serve as definitive hosts, deer, moose, and small mammals like rodents, which serve as intermediate hosts (23, 83, 84). The USA is not endemic to echinococcosis. This infection is hard to manage and treat because of the parasite's multiorgan cyst formation potential and the high recurrence rate (85). CE has been reported in many countries in South America, including Brazil, Argentina, Chile, Peru, and Uruguay. Studies in Chile confirmed that CE is prevalent in both livestock and animals (86, 87).
No detailed data are available with regard to the prevalence of echinococcosis in Afghanistan (88). A solitary study conducted in 1988 showed that 73% of stray dogs in Kabul harbored E. granulosus (89). However, CE clinical disease has been reported in Afghan immigrants and US soldiers who returned to the USA after performing duties in Afghanistan (90). Eighty percent of the Afghans work in agriculture or with animals, and many also have their own pets, like dogs. The population of stray dogs was found to be 10 times greater than that of domestic dogs. Poor law enforcement, political instability, and limited control strategies implementation are the factors for disseminated cases of CE and AE (75).
Echinococcosis is still underdiagnosed in Pakistan. Because of their limited scope, the few published reports on the disease's current state in the country cannot be considered indicative of the problem nationally. A total of 106 cases were reported to Agha Khan Hospital, Karachi, from 1995 to 2006, and 21 of them were Afghan refugees (91). The high prevalence of echinococcosis in Afghanistan and extensive free-border movement from Afghanistan into Pakistan highlight the need for conducting an extensive epidemiological study in Pakistan to assess the current status of the disease in Pakistan (92). Almost a three-decade-old study is available in the literature about the prevalence and serological investigation of echinococcosis in Islamabad, Pakistan. According to that study, hydatidosis was observed in 58.9, 38.90, and 33.06% of the slaughtered camels, cattle, and buffalo at a local slaughterhouse. Most of the cysts observed were infertile, while the specificity, sensitivity, and efficiency of indirect hemagglutination (IHA) and enzyme-linked immunosorbent assay (ELISA) were low (93).
Almost 466 million people live in Central Asian countries and are at risk of suffering from echinococcosis. Occupational exposure to farmers, herdsmen, and farmers is very high. The semi-nomadic lifestyle of the inhabitants of these areas and the raising of sheep and cattle together with dogs make the environment conducive for the Echinococcus species to complete their life cycle (75).
Echinococcosis is highly endemic in China, covering more than 21 provinces that comprise 87% of the total geographical area of the country. The copro-antigen ELISA, copro-DNA PCR, and necropsy of the dogs strongly suggested a high prevalence of both CE and AE in the dogs in China. As far as prevalence in cattle and sheep is concerned, CE in sheep and cattle was 50% and 44%, respectively (94).
After the fall of the Soviet Union, the number of human echinococcosis cases in Mongolia started to rise due to declining health facilities and poor dog deworming programs (95). Human CE is widely distributed in 12 provinces of Mongolia, with 10 of them adjoining Russia and China (91, 96). There is no echinococcosis control program institutionalized in Mongolia (96).
The dissolution of the Soviet Union led to the re-emergence of both human CE and AE in Kazakhstan, which are still endemic in the country due to several factors (97). Of them, nomadic lifestyles and the adoption of obsolete breeding practices shut down large farms; the establishment of small farms; the abandonment of dog deworming practices, and improper disposal of animal carcasses are important factors leading to the endemicity of echinococcosis (98).
Kyrgyzstan, Tajikistan, and Uzbekistan are endemic to human CE and AE (41, 99). The prevalence of CE in sheep and cattle in all three countries has been found to be alarming. Stray dogs and wild canines, including foxes, were found to be infected with E. granulosus (100). The control measures in these three countries are almost identical to those in Kazakhstan because they share similar geographical patterns, socioeconomic backgrounds, and literacy levels.
Iran is a camel-rich country with more than 1.5 million camels. CE is endemic in humans in Iran (101). Low-level endemicity was observed in northern and western Iran's sheep, goat, cattle, and camel populations (102, 103). Fecal samples from dogs and jackals were also found to be positive for containing E. granulosus DNA. Effective control measures were implemented in Kerman from 1991 to 1994, involving killing stray dogs and deworming pets and sheepdogs. These efforts were found to be highly effective as they decreased the incidence of the disease. An epidemiological study was conducted in five different regions of the country. Four hundred and thirty-eight dromedaries were examined, of which 135 were positive, resulting in a prevalence of up to 30.82%. The highest number of cysts were found in the lungs. The age group older than 15 years had the highest prevalence (31).
An epidemiological study was conducted to assess the prevalence of Echinococcus granulosus in client-owned dogs in the Sidi Kacem province of Morocco. The reason for choosing this province was that its climatic diversity, geographic conditions, and social system make it a model representative of the country. Dogs from both rural and urban areas were included in the study. Arecoline hydrobromide was administered orally to the dogs as a purgative. Of the 273 dogs included in the study, the feces of 224 dogs had E. granulosus worms, resulting in an overall prevalence of 82.1%. Compared to urban-owned dogs (18.8%), dogs in rural areas had a high prevalence (38%). A comparison was made between the prevalence of echinococcosis in dogs that had access to the slaughterhouse and those that did not. Results showed that dogs with access to slaughterhouses had a higher prevalence (62.7%) as compared to dogs (29.1%) that did not have such access (104).
Apart from infecting dogs as definitive hosts and omnivores and herbivores as intermediate hosts, Echinococcus also infects wild animals. The first confirmed case of echinococcosis caused by E. felidis was reported in a lion in South Africa about 80 years ago. Molecular characterization was performed on the eggs and archived feces (105). Hyena (Crocuta crocuta) also serves as a definitive host for E. felidis (106). Echinococcus fields have also been found to cause echinococcosis in hippos. Six hippopotami were investigated for cysts, and three were found to have hepatic cysts. The morphology of rostellar hooks helped identify E. felidis, and confirmation was performed through nuclear and mitochondrial DNA sequencing (107).
Echinococcus multilocularis enjoys a sylvatic life cycle. The first confirmed report appeared in 2011, when a red fox was found to be the definitive host for E. multilocularis. This study aimed to identify rodent species that serve as intermediate hosts of E. multilocularis. Liver samples from 1,566 rodents were collected and examined for E. multilocularis-specific lesions. Samples were subjected to PCR and sequencing to identify the pathogen. Tissues were examined histologically for E. granulosus-specific lesions. Microtus agrestis (1/187), Arvicola amphibious (8/439), Myodes glareolus (0/655), and Apodemus spp. (0/285) had a lesion size of more than 6 mm, which is specific to E. granulosus (108).
The magnitude of Echinococcus in north African countries is widespread because of the high population of stray dogs, which consume the carcasses of infected ruminants and camels and thus take up the cysts from such condemned carcasses (109). The majority of cases of hydatidosis in Tanzania are due to the Echinococcus granulosus G1 genotype. This study investigated the genetic relationship between the localization of E. granulosa G1 hydatid cysts in the liver and lungs of humans, sheep, and cattle. Host species and localization differentiation were two factors responsible for genetic differentiation, as determined by single-strand conformation polymorphism and allozyme variation (110).
Eight hundred and thirty-two fecal samples from six conservation areas in Kenya were collected to determine the prevalence of E. granulosus in wild mammals, including jackals, hyenas, leopards, lions, and wild dogs. A total of 120 samples were positive, containing taeniid eggs, showing an overall prevalence of 14.4%. A total of 1160 eggs were collected and subjected to restriction fragment length polymorphisms—polymerase chain reaction (RFLP-PCR) of the gene nad1, and sequencing was performed later. Of these samples, 26 were of E. felidis, and 12 were of E. granulosus sensu stricto complex (106).
The field of molecular epidemiology (ME) has altered our understanding of the spread of infectious diseases. This area of epidemiology provides the tools that can characterize the etiological agents of infectious illnesses. Furthermore, molecular epidemiology is useful for surveillance tasks, particularly when investigating emerging diseases. Molecular epidemiology is becoming increasingly important in the context of disease emergence and conservation for both human and domestic animal health, as well as for infectious agents in wildlife (111).
The parasite cestode Echinococcus is the cause of echinococcosis, which has two types—cystic and alveolar—and affects people worldwide. When investigating the epidemiology of infectious diseases, it is crucial to comprehend their etiology. It is important to understand how different host species are involved in the transmission of echinococcosis because this has been a problem in many endemic regions. This is even more important when various Echinococcus species, “strains,” or genotypes are present. Molecular epidemiology has been extremely helpful in understanding the wide genetic and phenotypic variability exhibited between the species present within the Echinococcus genus (112, 113).
Formally identifying a species requires effective communication, which is essential when the targeted species have public health significance and necessitate coordinated control measures. Regarding Echinococcus, there have long been taxonomic and nomenclatural ambiguities, especially at the species level. This occurred due to the lack of significant morphological features and the frequent overriding of the importance of host occurrence by taxonomic considerations (111). Echinococcus transmission ecology in areas with a variety of affected host species has been a topic of study for some time. Fortunately, there is widespread agreement that ten species of Echinococcus should be recognized based on morphological, genetic, and ecological factors. Molecular approaches usually confirm original taxonomic assumptions and, more importantly, the validity of certain morphological traits. They have also been instrumental in resolving taxonomic issues.
Echinococcus granulosus is the species most frequently responsible for cystic echinococcosis in humans and has the broadest spectrum of intermediate hosts (112, 113). In some regions, like Australia, it also impacts wildlife, although cycles involving cattle keep it in balance most of the time. In areas with a range of intermediate hosts that can be sensitive to other species besides E. granulosus, E. granulosus is also common. Because they allow for species identification from metacestode stages, molecular tools are essential in these situations. For instance, in some areas of Europe and the Middle East, livestock may contract E. granulosus, E. ortleppi, and E. intermedius, which also pose a risk to humans (112–114). It is necessary to ascertain whether distinct species cohabit in such situations to avoid impeding control strategies and focused public wellbeing measures. In many gulf countries, E. granulosus and E. intermedius are both kept sympatrically in cycles, with dogs acting as the final hosts and camelids and ovines serving as the intermediate hosts (114). Either species may occasionally infect people in mixed infestations (115). Camels are the main intermediate host for E. intermedius and, to a lesser extent, E. granulosus in a number of Middle Eastern regions. E. granulosus was the only species found in both animals and people, according to a ME investigation in Iran's Mazandaran Province. Additionally, E. granulosus was only found in sheep and camels, according to a ME study in Riyadh, Saudi Arabia (116). Cattle are thought to have a minor role in the spread of E. granulosus. However, recent investigations in Sudan and Ethiopia revealed that cattle serve as the most significant intermediate hosts for E. granulosus, E. ortleppi, and E. intermedius (117, 118).
Previous studies used host preference and phenotypic variations to discriminate between the genotypes G1, G2, and G3 of the E. granulosus species (111). Despite the need to maintain genotypic recognition in light of the phenotypic features that have epidemiological significance, current molecular characterization at a number of loci has not provided any evidence that G2 and G3 require species delimitation (119, 120).
Dogs serve as the final hosts for E. ortleppi and E. equinus, whereas cattle and horses serve as the intermediate and final hosts (111). Both of these species demonstrate high host selectivity for both types of hosts. However, only E. ortleppi can infect humans. Dogs' high host specificity likely explains why both species are sporadic and do not have a wide geographic distribution since they rarely have access to the metacestode stages in horses and cattle, especially since public health care has progressed in the endemic regions. However, according to recent ME studies, both species are still spreading over the African and South American continents, some regions of Europe, and Turkey (121, 122). Interestingly, the first ME investigation on echinococcosis carried out in Bhutan (123) discovered that both E. ortleppi and E. granulosus were locally transmitted.
It has been known for a long time that E. granulosus is distinct from other Echinococcus species and is maintained in cycles that include domestic pigs and cervids as intermediate hosts (111). According to previous ME findings (124), the four distinct Echinococcus genotypes (G6, 7, 8, and 10) are sustained in these life cycles. These studies added to the morphological descriptions of the adult parasites that originated in cervids and pigs. Nomenclatural issues have made it difficult to fully understand these forms' transmission cycles, despite molecular techniques' value in demonstrating the genetic distinctiveness of these forms.
There are two subspecies of the species E. canadensis: G8 and G10 (111). Initially, it was proposed to include the genotypes G6 and G7 in the species E. canadensis (125). Unfortunately, this did not reflect their ecological or geographical distribution. (124). According to molecular techniques (124, 126), the four genotypes now clearly represent two species. Sequences of G6 and G7 wildlife isolates are notably different from those of G8 and G10, and those of the latter were remarkably diverse from one another (122). Phylogenetic analysis revealed that the G6/G7 and G8/G10 groups should be considered as two discrete species, E. intermedia and E. canadensis (126). This was previously advocated (124) because the name E. intermedius had already been proposed for the species infecting pigs and camels.
In North America and Scandinavia, both genotypes of E. canadensis primarily infect wolves and other cervids, with occasional human infections (114). On the other hand, the majority of E. intermedius infections are found in the Middle East, Africa, and Europe, typically in regions where E. granulosus is also common. E. intermedius infections are also sustained in domestic cycles and are communicable to humans (114). The known geographic range of E. intermedius will likely expand as more ME surveillance is done. For instance, according to current data from various West African countries, the genotype G6 of E. intermedius is the most prevalent species due to the widespread use of camels (127) and is, therefore, the CE strain that poses the greatest risk to the local population's health. According to the authors of research on the illness in wild canids in Quebec and Maine (128), coyotes are more likely than wolves to contaminate urban green spaces and peri-urban habitats, according to the authors of research on the illness in wild canids in Quebec and Maine (128). This is true even though both wolves and coyotes have been shown to be hosts for E. canadensis. Overview about prevalent genotypes of Echinococcus species and molecular markers investigated in different countries and different markers used to investigate prevalence of Echinococcus species and corresponding number of studies covered in this review are mentioned in Tables 1, 2, respectively.
Table 1. Overview of prevalent genotypes of Echinococcus species and molecular markers that were investigated in different countries.
Table 2. Different markers were used to investigate the prevalence of Echinococcus species and a corresponding number of studies covered in this review.
The taxonomy of Echinococcus has mostly been elucidated due to molecular science. As a result, a useful and educational lexicon for use in epidemiological studies has been created. We must now have the resources available to carry out epidemiological studies. These are useful in clarifying life cycles and transmission patterns in endemic areas, as previously discussed. This will be especially important in regions with several transmission cycles and the potential for mixed infections. Molecular techniques will increasingly inform and direct public health initiatives regarding clinical treatment. Those diagnosed with echinococcosis whose Echinococcus species of infection is unclear or who may have infections from many Echinococcus species are most likely to benefit. The discovery of genotypic variation within a species and its relationship with virulence foreshadows the creation of markers for clinical use.
Conceptualization: MA and AA. Data curation and writing—original draft preparation: MA. Reviewing and editing: AA. Both authors contributed to the article and approved the submitted version.
The researchers are thankful to the Deanship of Scientific Research, Qassim University, Saudi Arabia for funding the publication of this review.
The authors declare that the research was conducted in the absence of any commercial or financial relationships that could be construed as a potential conflict of interest.
All claims expressed in this article are solely those of the authors and do not necessarily represent those of their affiliated organizations, or those of the publisher, the editors and the reviewers. Any product that may be evaluated in this article, or claim that may be made by its manufacturer, is not guaranteed or endorsed by the publisher.
1. Ismael SMM, Salem SA, Elshahidy MS. Isolation and molecular characterization of circulating foot and mouth disease virus in Egypt during 2018-2020. Int J Vet Sci. (2021) 10:162–71. doi: 10.47278/journal.ijvs/2021.046
2. Nasr EA, Fawzy RE, Marian GS, Abbas AM, Khalifa E. Using of gamma interferon γIFN and multiplex PCR (m-PCR) for detection of bovine tuberculosis in dairy herds in Egypt. Int J Vet Sci. (2021) 10:229–33. doi: 10.47278/journal.ijvs/2021.035
3. Osman SA, Tharwat M, Saeed EMA. An outbreak of epidemiological, clinical and treatment outcomes of ovine listeriosis in Qassim region, Saudi Arabia. Int J Vet Sci. (2021) 10:312–6. doi: 10.47278/journal.ijvs/2021.060
4. Ali S, Ijaz M, Ahmed A, Aziz MU, Naveed M, Javed MU, et al. Prevalence and associated risk factors of bovine babesiosis in Lahore, Pakistan. Agrobiol Rec. 2. (2020) 17–23. doi: 10.47278/journal.abr/2020.007
5. Zaman MA, Mehreen U, Qamar W, Qamar MF, Kashif M, Shahid Z, et al. Brief account of bovine theileriosis prevalence in some South Asian countries. Agrobiol Rec. (2020) 2:38–48. doi: 10.47278/journal.abr/2020.010
6. Sharif M, Tunio SA, Bano S. Synergistic effects of Zinc oxide nanoparticles and conventional antibiotics against methicillin resistant Staphylococcus aureus. Adv Life Sci. (2021) 8:167–71.
7. Reshetnikova TI, Zenkin AS, Krylova TG. Experimental use of the triazavirin antiviral medication in conditions of group administration at the pig-breeding unit. Adv Life Sci. (2021) 8:381–6.
8. Özcan U, Sezener MG, Sayilkan BU, Ergüden VE, Küllük E, Yaman SA, et al. New aspect in neonatal calf diarrhea: presence of Escherichia coli CS31A at unexpected ratio. Kafkas Univ Vet Fak Derg. (2021)27:133–4.
9. Özdemir O, Ortatatli M, Terzi F, Hatipoglu FH, Çiftçi MK, Ateş MB, et al. The usability of cytological and immunocytological methods for rapid diagnosis of encephalitic listeriosis in ruminants. Kafkas Univ Vet Fak Derg. (2021) 27:225–33.
10. Aksel EG, Akçay A, Arslan K, Sohel MH, Güngör G, Akyüz B, et al. The effects of MBL1 gene polymorphism on subclinical mastitis in Holstein cows. Kafkas Univ Vet Fak Derg. (2021) 27:389–95.
11. Strbac F, Bosco A, Amadesi A, Rinaldi L, Stojanović D, Simin N, et al. Ovicidal potential of five different essential oils to control gastrointestinal nematodes of sheep. Pak Vet J. (2021) 41:353–8.
12. Selim A, Alahadeb AA. Prevalence and molecular characterization of Ehrlichia canis in Egyptian dogs. Pak Vet J. (2021) 41:117–21. doi: 10.29261/pakvetj/2020.061
13. Jalil PJ, Shnawa BH, Hammad SM. Silver nanoparticles: Green synthesis, characterization, blood compatibility and protoscolicidal efficacy against Echinococcus granulosus. Pak Vet J. (2021) 41:393–9. doi: 10.29261/pakvetj/2021.039
14. Wajiha, Qureshi NA. Invitro anticoccidial, antioxidant activities and biochemical screening of methanolic and aqueous leaves extracts of selected plants. Pak Vet J. (2021) 41:57–63. doi: 10.29261/pakvetj/2020.071
15. Ali KM, Hassan EA, Abuowarda MM, Mahmoud MA, Torad FA. Bilateral panophthalmia as a late sequel of leishmaniasis in dogs. Pak Vet J. (2021) 41:13–18. doi: 10.29261/pakvetj/2021.006
16. Rialch A, Raina OK, Tigga MN, Anandanarayanan A, Ganaie ZA, Aftab A, et al. Evaluation of Echinococcus granulosus recombinant EgAgB8/1, EgAgB8/2 and EPC1 antigens in the diagnosis of cystic echinococcosis in buffaloes. Vet Parasitol. (2018) 252:29–34. doi: 10.1016/j.vetpar.2018.01.020
17. Ma J, Wang H, Lin G, Zhao F, Li C, Zhang T, et al. Surveillance of Echinococcus isolates from Qinghai, China. Vet Parasitol. (2015) 207:44–8. doi: 10.1016/j.vetpar.2014.11.012
18. Sadjjadi SM, Mikaeili F, Karamian M, Maraghi S, Sadjjadi FS, Shariat-Torbaghan S, et al. that the Echinococcus granulosus G6 genotype has an affinity for the brain in humans. Int J Parasitol. (2013) 43:875–7. doi: 10.1016/j.ijpara.2013.06.008
19. Singh BB, Sharma JK, Ghatak S, Sharma R, Bal MS, Tuli A, et al. Molecular epidemiology of echinococcosis from food producing animals in north India. Vet Parasitol. (2012) 186:503–6. doi: 10.1016/j.vetpar.2011.11.064
20. Nakao M, Lavikainen A, Yanagida T, Ito A. Phylogenetic systematics of the genus Echinococcus (Cestoda: Taeniidae). Int J Parasitol. (2013) 43:1017–29. doi: 10.1016/j.ijpara.2013.06.002
21. Lymbery AJ, Thompson RCA. Species of Echinococcus: pattern and process. Parasitol Today. (1996) 12:486–91. doi: 10.1016/S0169-4758(96)10071-5
22. Hammad SJ, Cavallero S, Milardi GL, Gabrielli S, D?Amelio S, Al-Nasiri FS. Molecular genotyping of Echinococcus granulosus in the North of Iraq. Vet Parasitol. (2018) 249:82–7. doi: 10.1016/j.vetpar.2017.11.010
23. Eckert J, Deplazes P, Eckert J, Deplazes P. Aspects of echinococcosis, a zoonosis of increasing concern biological, epidemiological and clinical aspects of Echinococcosis. Zoonos Increas Conc. (2004) 17:107–35. doi: 10.1128/CMR.17.1.107-135.2004
24. Schneider R, Gollackner B, Schindl M, Tucek G, Auer H. Echinococcus canadensis G7 (pig strain): an underestimated cause of cystic echinococcosis in Austria. Am J Trop Med Hyg. (2010) 82:871–4. doi: 10.4269/ajtmh.2010.09-0639
25. Sadjjadi SM. Present situation of echinococcosis in the middle east and Arabic North Africa. Parasitol Int. (2006) 55:197–202. doi: 10.1016/j.parint.2005.11.030
27. Eckert J, Thompson RCA. Historical aspects of echinococcosis. Adv Parasitol. (2017) 95:1–54. doi: 10.1016/bs.apar.2016.07.003
28. Posselt A. Der Alveolarechinokokkus und seine Chirurgie. In: Hosemann, G, Schwarz, E, Lehmann, JC, Posselt, A, editors. Die Echinokokken-Krankheit F Enke. Stuttgart (1928) pp. 305–418.
29. Torgerson PR, Macpherson CNL. The socioeconomic burden of parasitic zoonoses: global trends. Vet Parasitol. (2011) 182:79–95. doi: 10.1016/j.vetpar.2011.07.017
31. Ehsan M, Akhter N, Bhutto B, Arijo A, Ali A, Gadahi J. Prevalence and genotypic characterization of bovine Echinococcus granulosus isolates by using cytochrome oxidase 1 (Co1) gene in Hyderabad, Pakistan. Vet Parasitol. (2017) 239:80–5. doi: 10.1016/j.vetpar.2017.04.006
32. Rojas C, Ebi D, Gauci CG, Scheerlinck JP, Wassermann M, Jenkins DJ, et al. Microdiversity of Echinococcus granulosus sensu stricto in Australia. Parasitology. (2016) 143.1026–33. doi: 10.1017/S0031182016000445
33. Latif AA, Tanveer A, Maqbool A, Siddiqi N, Kyaw-Tanner M, Traub RJ, et al. Morphological and molecular characterisation of Echinococcus granulosus in livestock and humans in Punjab, Pakistan. Vet Parasitol. (2010) 170:44–9. doi: 10.1016/j.vetpar.2010.02.003
34. Elham M, Hassan B, Ghasem NA, Gholamreza R, Parviz S. Epidemiological study of hydatidosis in the dromedaries (Camelus dromedarius) of different regions of Iran. Asian Pac J Trop Biomed. (2014) 4:148–51. doi: 10.12980/APJTB.4.2014C725
35. Jenkins DJ, Romig T, Thompson RCA. Emergence/re-emergence of Echinococcus spp. Glob Update. (2005) 35:1205–19. doi: 10.1016/j.ijpara.2005.07.014
36. Pourseif MM, Moghaddam G, Saeedi N, Barzegari A, Dehghani J, Omidi Y, et al. Current status and future prospective of vaccine development against Echinococcus granulosus. Biologicals. (2017) 51:1–11. doi: 10.1016/j.biologicals.2017.10.003
37. Conceição MAP, Cravo I, Costa IMH, Ferreira R, Costa RPR, Castro A, et al. Echinococcus granulosus ss in dog—a report in center-northern Portugal. Vet Parasitol Reg Stud Rep. (2017) 9:84–7. doi: 10.1016/j.vprsr.2017.05.002
38. Brehm, K. The role of evolutionarily conserved signalling systems in Echinococcus multilocularis development and host–parasite interaction. Med Microbiol Immunol. (2010) 199:247–59. doi: 10.1007/s00430-010-0154-1
39. Thompson RCA, Donald P. Towards a taxonomic revision of the genus Echinococcus. Treands Parasitol. (2002) 18:452–7. doi: 10.1016/S1471-4922(02)02358-9
40. Nakao M, Li T, Han X, Ma X, Xiao N, Qiu J, et al. Genetic polymorphisms of Echinococcus tapeworms in China as determined by mitochondrial and nuclear DNA sequences. Int J Parasitol. (2010) 40:379–85. doi: 10.1016/j.ijpara.2009.09.006
41. Torgerson PR, Keller K, Magnotta M, Ragland N. The global burden of alveolar echinococcosis. PLoS Negl Trop Dis. (2010) 4:e722. doi: 10.1371/journal.pntd.0000722
42. Torgerson PR, Devleesschauwer B, Praet N, Speybroeck N, Willingham AL, Kasuga F, et al. World health organization estimates of the global and regional disease burden of 11 foodborne parasitic diseases, 2010: a data synthesis. PLoS Med. (2015) 12:e1001920. doi: 10.1371/journal.pmed.1001920
43. Scioscia NP, Beldomenico PM, Petrigh RS, Pierangeli N, Denegri GM. Epidemiological studies on Echinococcus in Pampas fox (Lycalopex gymnocercus) and European hare (Lepus europaeus) in Buenos Aires province, Argentina. Parasitol. (2013) 112:3607–13. doi: 10.1007/s00436-013-3548-3
44. Santa MA, Pastran SA, Klein C, Duignan P, Ruckstuhl K, Romig T, et al. Detecting co-infections of Echinococcus multilocularis and Echinococcus canadensis in coyotes and red foxes in Alberta, Canada using real-time PCR. Int J Parasitol Parasit Wildlife. (2018) 7:111–5. doi: 10.1016/j.ijppaw.2018.03.001
45. Pleydell DRJ, Yang YR, Danson FM, Raoul F, Craig PS, Donald P, et al. Landscape composition and spatial prediction of alveolar echinococcosis in Southern Ningxia, China. PLoS Negl Trop Dis. (2008) 2:e287. doi: 10.1371/journal.pntd.0000287
46. Li T, Ito A, Pengcuo R, Sako Y, Chen X, Qiu D, et al. Post-treatment follow-up study of abdominal cystic echinococcosis in tibetan communities of Northwest Sichuan Province, China. PLoS Negl Trop Dis. (2012) 5:1–8. doi: 10.1371/journal.pntd.0001364
47. Brunetti E, Kern P, Angèle D, Panel W. Acta Tropica Expert consensus for the diagnosis and treatment of cystic and alveolar echinococcosis in humans. Acta Tropica. (2010) 114:1–16. doi: 10.1016/j.actatropica.2009.11.001
48. Yang D, Zhang T, Zeng Z, Zhao W, Zhang W, Liu A, et al. The first report of human-derived G10 genotype of Echinococcus canadensis in China and possible sources and routes of transmission. Parasitol Int. (2015) 64:330–3. doi: 10.1016/j.parint.2015.05.001
49. Bowles J, Blair D, McManus DP. Genetic variants within the genus Echinococcus identified by mitochondrial DNA sequencing. Mol. Biochem Parasitol. (1992) 54:165–73. doi: 10.1016/0166-6851(92)90109-W
50. Beyhan YE, Umur S. Molecular characterization and prevalence of cystic echinococcosis in slaughtered water buffaloes in Turkey. Vet Parasitol. (2011) 181:174–9. doi: 10.1016/j.vetpar.2011.04.038
51. Snabel V, Altintas N, D'Amelio S, Nakao M, Romig T, Yolasigmaz A, et al. Cystic Echinococcosis in Turkey: genetic variability and first record of the pig strain (G7) in the country. Parasitol. (2009) 105:145–54. doi: 10.1007/s00436-009-1376-2
52. Thompson RCA. Experimental Parasitology: The taxonomy, phylogeny and transmission of Echinococcus. (2008) 119:439–46. doi: 10.1016/j.exppara.2008.04.016
53. Nakao M, Manus DPMC, Schantz PM, Craig PS, Ito A. A molecular phylogeny of the genus Echinococcus inferred from complete mitochondrial genomes. Parasitology. (2007) 134:713–22. doi: 10.1017/S0031182006001934
54. Nemati A, Kamgarpour A, Rashid M, Nazari SS. Case report giant cerebral hydatid cyst in a child-a case report and review of literature. BJMP. (2010) 3:338–41.
55. McManus DP, Zhang L, Castrodale LJ, Le TH, Pearson M, Blair D, et al. Molecular genetic characterization of an unusually severe case of hydatid disease in Alaska caused by the cervid strain of Echinococcus granulosus. Am. J Trop Med Hyg. (2002) 67:296–8. doi: 10.4269/ajtmh.2002.67.296
56. Zhang W, Mcmanus DP. Recent advances in the immunologyand diagnosis of echinococcosis. FEMS Immunol Med Microbiol. (2006) 47:24–41. doi: 10.1111/j.1574-695X.2006.00060.x
57. Manzano-román R, Sánchez-ovejero C, Hernández-gonzález A, Casulli A, Siles-lucas M. Serological diagnosis and follow-up of human cystic echinococcosis: a new hope for the future? BioMed Res Int. (2015) 2015:e428205. doi: 10.1155/2015/428205
58. Tamarozzi F, Nicoletti GJ, Neumayr A. Acceptance of standardized ultrasound classification, use of albendazole and long-term follow-up in clinical management of cystic echinococcosis: a systematic review. Curr Opin Infect Dis. (2014) 27:425–31. doi: 10.1097/QCO.0000000000000093
59. Deplazes P, Hegglin D, Gloor S, Romig T. Wilderness in the city: the urbanization of Echinococcus multilocularis. Trends Parasitol. (2004) 20:77–84. doi: 10.1016/j.pt.2003.11.011
60. Barnes TS, Deplazes P, Gottstein B, Jenkins DJ, Mathis A, Siles-lucas M, et al. Acta tropica challenges for diagnosis and control of cystic hydatid disease. Acta Tropica. (2012) 123:1–7. doi: 10.1016/j.actatropica.2012.02.066
61. Bhandari S, Naval CL, Gupta V, Jain S, Yadav S. Intracranial hydatid cyst—a rare manifestation of Echinococcus infestation. Indian J Med Spec. (2015) 6:170–2. doi: 10.1016/j.injms.2015.07.006
62. Hemphill AB, Stadelmann R, Rufener M, Spiliotis G, Boubaker J, Müller N. Treatment of Echinococcosis: albendazole and mebendazole–what else? Parasite. (2014) 21:70. doi: 10.1051/parasite/2014073
63. McManus DP. Molecular genetic variation in Echinococcus and Taenia: an update. Southeast Asian J Trop Med Public Health. (1997) 28:110–6.
64. Nakao M, Sako Y, Yokoyama N, Fukunaga M, Ito A. Mitochondrial genetic code in cestodes. Mol Biochem Parasitol. (2000) 111:415e.424 doi: 10.1016/S0166-6851(00)00334-0
65. Wassermann M, Mackenstedt U, Romig T. A loop-mediated isothermal amplification (LAMP) method for the identification of species within the Echinococcus granulosus complex. Vet Parasitol. (2014) 200:97–103. doi: 10.1016/j.vetpar.2013.12.012
66. Bartholomei-Santos ML, Heinzelmann LS, Oliveira RP, Chemale G, Gutierrez AM, Kamenetzky L, et al. Isolation and characterization of microsatellites from the tapeworm Echinococcus granulosus. Parasitology. (2003) 126:599–605.
67. Bart JM, Knapp J, Gottstein B, El-Garch F, Giraudoux P, Glowatzki ML, et al. EmsB, a tandem repeated multi-loci microsatellite, new tool to investigate the genetic diversity of Echinococcus multilocularis. Infect Genet E6. (2006) 390–400. doi: 10.1016/j.meegid.2006.01.006
68. Dinkel A, Kern S, Brinker A, Oehme R, Vaniscotte A, Giraudoux P, et al. A real-time multiplex-nested PCR system for coprological diagnosis of Echinococcus multilocularis and host species. Parasitol Res. (2011) 109:493–8. doi: 10.1007/s00436-011-2272-0
69. Knapp J, Millon L, Mouzon L, Umhang G, Raoul F, Ali ZS, et al. Real time PCR to detect the environmental faecal contamination by Echinococcus multilocularis from red fox stools. Vet Parasitol. (2014) 201:40–7. doi: 10.1016/j.vetpar.2013.12.023
70. Hemer S, Brehm K In vitro efficacy of the anticancer drug imatinib on Echinococcus multilocularis larvae. Int J Antim Agents. (2012) 40:458–62. doi: 10.1016/j.ijantimicag.2012.07.007
71. Vercruysse J, Knox DP, Schetters TPM. Veterinary parasitic vaccines : pitfalls and future directions. Treands Parasitol. (2004) 20:488–92 doi: 10.1016/j.pt.2004.07.009
72. Thompson RCA, Jenkins DJ. Echinococcus as a model system: biology and epidemiology. Int J Parasitol. (2014) 44:865–77. doi: 10.1016/j.ijpara.2014.07.005
73. Larrieu E. Critical analysis of cystic echinococcosis control programs and praziquantel use in South America. Rev Panam Salud Publica. (2010) 31:81–7. doi: 10.1590/S1020-49892012000100012
74. Zhang W, Zhang Z, Wu W, Shi B, Li J, Zhou X, et al. Epidemiology and control of echinococcosis in central Asia, with particular reference to the People's Republic of China. Acta Tropica. (2015) 141:235–43. doi: 10.1016/j.actatropica.2014.03.014
75. Craig PS. Control of cystic echinococcosis/hydatidosis. Adv Parasitol. (2002) 61:443–508. doi: 10.1016/S0065-308X(05)61011-1
76. Eckert J, Deplazes P. Biological, epidemiological, and clinical aspects of echinococcosis, a zoonosis of increasing concern. Clin Microbiol Rev. (2004) 17:107e.
77. Boufana B, Lett WS, Lahmar S, Buishi I, Bodell AJ, Varcasia A, et al. equinus and Echinococcus granulosus sensu stricto from the United Kingdom: Genetic diversity and haplotypic variation. Int J Parasitol. (2015) 45:161–6. doi: 10.1016/j.ijpara.2014.10.005
78. Mcmanus DP, Thompson RCA. Comment on the Status of Echinococcus granulosus in the UK. Parasitol Today. (1989) 5:365–7. doi: 10.1016/0169-4758(89)90114-2
79. Umhang G, Lahoreau J, Hormaz V, Boucher JM, Guenon A, Montange D, et al. Surveillance and management of Echinococcus multilocularis in a wildlife park. Parasitol Int. (2016) 65:245–50. doi: 10.1016/j.parint.2016.01.008
80. Mitrea IL, Ionita M, Costin II, Predoi G, Avram E, Rinaldi L, et al. and genetic characterization of Echinococcus granulosus in naturally infected adult sheep and cattle in Romania. Vet Parasitol. (2014) 206:159–66. doi: 10.1016/j.vetpar.2014.10.028
81. Debeljak Z, Boufana B, Interisano M, Vidanovic D, Kulisic Z. First insights into the genetic diversity of Echinococcus granulosus sensu stricto (s.s.) in Serbia. Vet Parasitol. (2016) 223:57–62. doi: 10.1016/j.vetpar.2016.04.007
82. Romig T, Ebi D, Wassermann, M. Taxonomy and molecular epidemiology of Echinococcus granulosus sensu lato. Vet Parasitol. (2015) 213:76–84. doi: 10.1016/j.vetpar.2015.07.035
83. Liccioli S, Duignan PJ, Lejeune M, Deunk J, Majid S. Parasitology international a new intermediate host for Echinococcus multilocularis : the southern red-backed vole (Myodes gapperi) in urban landscape in Calgary, Canada. Parasitol Int. (2013) 62:355–7. doi: 10.1016/j.parint.2013.03.007
84. Zhang KJ, Schaldenbrand M. Multiorgan Echinococcus infection: treatment of an immigrant in the United States. IDCases. (2015) 9:38–41. doi: 10.1016/j.idcr.2017.05.011
85. Espinoza S, Salas AM, Vargas A, Freire V, Diaz E, Sánchez G, et al. Detection of the G3 genotype of Echinococcus granulosus from hydatid cysts of Chilean cattle using COX1and ND1mitochondrial markers. Parasitol Res. (2014) 113:139–147. doi: 10.1007/s00436-013-3636-4
86. Manterola C, Benavente F, Melo A, Vial M. Description of Echinococcus granulosus genotypes in human hydatidosis in a region of southern Chile. Parasitol Int. (2008) 57:342–6. doi: 10.1016/j.parint.2008.02.005
87. Carter C, Bonatti H, Hranjec T, Barroso LF, Donowitz G, Sawyer RG, et al. Epigastric cystic echinococcus involving stomach, liver, diaphragm, and spleen in an immigrant from Afghanistan. Surg Infect. (2009) 10:453–6. doi: 10.1089/sur.2008.051
88. Le Riche PD, Soe AK, Alemzada Q. Parasites of dogs in Kabul, Afghanistan. Br Vet J. (1988) 144:370–3. doi: 10.1016/0007-1935(88)90067-X
89. Kronmann KC, Shields WW, Sheer TA, Crum-Cianflone NF. Echinococcus in a U.S. Marine after deployment to Afghanistan. Am J Gastroenterol. (2008) 103:2151e.2153. doi: 10.1111/j.1572-0241.2008.01982_13.x
90. Mumtaz K, Kamani L, Chawla T, Hamid S. Hepatic cystic echinococcosis : clinical characteristics and outcomes in Pakistan. Trop Doct. (2015) 39:215–7. doi: 10.1258/td.2009.080463
91. Wang NJ, Wang D, Hu X, Zhong Z, Jiang A, Yang S, et al. Genetic variability of Echinococcus granulosus. based on the mitochondrial 16S ribosomal RNA gene. Mitochondr DNA. (2015) 26:396–401. doi: 10.3109/19401736.2013.840590
92. Khan MQ, Afzal M, Ali S. Prevalence and serology of hydatidosis in large ruminants of Pakistan. Vet Parasitol. (1990) 37:163–8. doi: 10.1016/0304-4017(90)90071-I
93. Vaniscotte A, Raoul F, Poulle ML, Romig T. Role of dog behaviour and environmental fecal contamination in transmission of Echinococcus multilocularis in Tibetan communities. Parasitology. (2011) 138:1316–29. doi: 10.1017/S0031182011000874
94. Ito A, Agvaandaram G, Chuluunbaatar B. Short report : histopathological. Serological, and molecular confirmation of indigenous alveolar echinococcosis cases in Mongolia. Am J Trop Med Hyg. (2010) 82:266–9. doi: 10.4269/ajtmh.2010.09-0520
95. Jabbar A, Narankhajid M, Nolan MJ, Jex AR, Campbell BE, Gasser RB. A fi rst insight into the genotypes of Echinococcus granulosus from humans in Mongolia. Mol Cell Probes. (2011) 25:49–54. doi: 10.1016/j.mcp.2010.11.001
96. Torgerson PR. The emergence of echinococcosis in central Asia. Int J Parasitol. (2013) 140:1667–73. doi: 10.1017/S0031182013000516
97. Shaikenov BS, Torgerson PR, Usenbaye AE, Baitursyno KK, Rysmukhambeto AT, Abdybeko AM, et al. The changing epidemiology of echinococcosis in Kazakhstan due to transformation of farming practices. Acta Trop. (2003) 85:287–93. doi: 10.1016/S0001-706X(02)00236-X
98. Usubalieva J, Minbaeva G, Ziadinov I, Deplazes P. Human alveolar echinococcosis in Kyrgyzstan. Emerg Infect Dis. (2013) 19:1095–8. doi: 10.3201/eid1907.121405
99. Ziadinov I, Deplazes P, Mathis A, Mutunova B. Veterinary parasitology frequency distribution of Echinococcus multilocularis and other helminths of foxes in Kyrgyzstan. Vet Parasitol. (2010) 171:286–92. doi: 10.1016/j.vetpar.2010.04.006
100. Ahmadi NA. retrospective analysis of human cystic echinococcosis in Hamedan province, an endemic region of Iran. Ann Trop Med Parasitol. (2008) 102:603–9. doi: 10.1179/136485908X337517
101. Mansoorlakooraj H, Saadati D, Javadi R, Heydari S, Torki E, Gholami H, et al. Veterinary parasitology short communication a survey on hydatidosis in livestock in Northern Iran based on data collected from slaughterhouses from 2004 to 2008. Vet Parasitol. (2011) 182:364–7. doi: 10.1016/j.vetpar.2011.05.013
102. Pour AA, Hosseini SH. The prevalence and fertility of hydatid cysts in buffaloes from Iran. J Helminthol. (2012) 86:373–7. doi: 10.1017/S0022149X11000514
103. Beiromvand M, Akhlaghi L, Hossein S, Massom F, Mobedi I, Reza A, et al. of Echinococcus multilocularis in Carnivores in Razavi Khorasan Province, Iran using mitochondrial DNA. PLoS xxx. (2011) 5: xxx. doi: 10.1371/journal.pntd.0001379
104. Dakkak A, El Berbri I, Petavy AF, Boué F, Bouslikhane M, Fassi Fihri O, et al. Echinococcus granulosus infection in dogs in Sidi Kacem Province (North-West Morocco). Acta Trop. (2017) 165:26–32. doi: 10.1016/j.actatropica.2016.07.007
105. Huttner M, Siefert L, Mackenstedt U, Romig TA. Survey of Echinococcus species in wild carnivores and livestock in East Africa. Int J Parasitol. (2009) 39:1269e.1276. doi: 10.1016/j.ijpara.2009.02.015
106. Kagendo D, Magambo J, Agola EL, Njenga SM, Zeyhle E, Mulinge E, et al. A survey for Echinococcus spp of carnivores in six wildlife conservation areas in Kenya. Parasitol Int. (2014) 63:604–11. doi: 10.1016/j.parint.2014.04.001
107. Halajian A, Luus-Powell WJ, Roux F, Nakao M, Sasaki M, Lavikainen A. Echinococcus felidis in hippopotamus, South Africa. Vet Parasitol. (2017) 243:24–8. doi: 10.1016/j.vetpar.2017.06.001
108. Miller AL, Olsson GE, Walburg MR, Sollenberg S, Skarin M, Ley C, et al. First identification of Echinococcus multilocularis in rodent intermediate hosts in Sweden. Int J Parasitol Paras Wildlife. (2016) 5:56–63. doi: 10.1016/j.ijppaw.2016.03.001
109. Chaâbane-banaoues R, Oudni-m M, Cabaret J, Selim M, Mezhoud H, Babba H. Infection of dogs with Echinococcus granulosus : causes and consequences in an hyperendemic area. Parasit Vector. (2015) 17:1–9. doi: 10.1186/s13071-015-0832-3
110. Oudni-M'rad M, Cabaret J, M'rad S, Chaâbane-Banaoues R, Mekki M, Zmantar S, et al. Genetic relationship between the Echinococcus granulosus sensu stricto cysts located in lung and liver of hosts. Infect Genet Evol. (2016) 44:356–60. doi: 10.1016/j.meegid.2016.07.024
111. Thompson RA. Parasite zoonoses and wildlife: One health, spillover and human activity. Int J Parasitol. (2013) 43:1079–88. doi: 10.1016/j.ijpara.2013.06.007
112. Thompson RA. Biology and Systematics of Echinococcus. Adv Parasitol. (2017) 95:65–109. doi: 10.1016/bs.apar.2016.07.001
113. Romig T, Deplazes P, Jenkins D, Giraudoux P, Massolo A, Craig P, et al. Life cycle patterns of Echinococcus species. Adv Parasitol. (2017) 95:213–314. doi: 10.1016/bs.apar.2016.11.002
114. Deplazes P, Rinaldi L, Rojas CA, Torgerson P, Harandi M, Romig T, et al. Global distribution of alveolar and cystic echinococcosis. Adv Parasitol. (2017) 95:315–493. doi: 10.1016/bs.apar.2016.11.001
115. Lashkarizadeh MR, Hooshmand N, Nasibi S, Mohammadi MA, Shamsaddini S, Kamyabi H, et al. Genetic profile of hydatid cysts in patients with multi-organ involvement: mixed infections by different strains. Vector Borne Zoonotic Dis. (2019) 19:724–30. doi: 10.1089/vbz.2018.2427
116. Metwally DM, Qassim LE, Al-Turaiki IM, Almeer RS, El-Khadragy MF. Gene-based molecular analysis of COX1 in Echinococcus granulosus cysts isolated from naturally infected livestock in Riyadh, Saudi Arabia. PLoS ONE. (2018) 13:e0195016. doi: 10.1371/journal.pone.0195016
117. Ahmed ME, Salim B, Grobusch M, Aradaib IE. First molecular characterization of Echinococcus granulosus (sensu stricto) genotype 1 among cattle in Sudan. BMC Vet Res. (2018) 14:36. doi: 10.1186/s12917-018-1348-9
118. Terefe Y, Addy RNF, Alemu S, Mackenstedt U, Romig T, Wassermann M, et al. Genetic characterization of Echinococcus species in eastern Ethiopia. Vet Parasitol Reg Stud Rep. (2019) 17:100302. doi: 10.1016/j.vprsr.2019.100302
119. Kinkar L, Laurimäe T, Sharbatkhori M, Mirhendi H, Kia EB, Ponce-Gordo F, et al. New mitogenome and nuclear evidence on the phylogeny and taxonomy of the highly zoonotic tapeworm Echinococcus granulosus sensu stricto. Infect Genet Evol. (2017) 52:52–8. doi: 10.1016/j.meegid.2017.04.023
120. Kinkar L, Laurimäe T, Acosta-Jamett G, Andresiuk V, Balkaya I, Casulli A, et al. Distinguishing Echinococcus granulosus sensu stricto genotypes G1 and G3 with confidence: a practical guide. Infect Genet Evol. (2018) 64:178–84. doi: 10.1016/j.meegid.2018.06.026
121. Kesik HK, Kilinc SG, Simsek S, Gul A. Occurrence of liver hydatid cysts in a donkey and molecular characterization of Echinococcus equinus. J Parasitol. (2019) 105:442–5. doi: 10.1645/19-3
122. Addy RNF, Wassermann M, Banda F, Mbaya H, Aschenborn J, Aschenborn O, et al. Genetic polymorphism and population structure of Echinococcus ortleppi. Parasitology. (2016) 144:450–8. doi: 10.1017/S0031182016001840
123. Thapa NK, Armua-Fernandez MT, Kinzang D, Gurung RB, Wangdi P, Deplazes P, et al. Detection of Echinococcus granulosus and Echinococcus ortleppi in Bhutan. Parasitol Int. (2017) 66:139–41. doi: 10.1016/j.parint.2016.12.010
124. Lymbery AJ, Jenkins EJ, Schurer JM, Thompson RCA. Echinococcus canadensis, E. borealis, and E intermedius What's in a name? Trends Parasitol. (2014) 31:23–9. doi: 10.1016/j.pt.2014.11.003
125. Nakao M, McManus DP, Schantz PM, Craig PS, Ito AA. Molecular phylogeny of the genus Echinococcus inferred from complete mitochondrial genomes. Parasitology. (2006) 134:713–22.
126. Laurimäe T, Kinkar L, Moks E, Romig T, Omer RA, Casulli A, et al. Molecular phylogeny based on six nuclear genes suggests that Echinococcus granulosus sensu lato genotypes G6/G7 and G8/G10 can be regarded as two distinct species. Parasitology. (2018) 145:1929–37. doi: 10.1017/S0031182018000719
127. Ohiolei JA, Xia C-Y, Li L, Liu J-Z, Tang W-Q, Wu Y-T, et al. Genetic variation of Echinococcus spp. in yaks and sheep in the tibet autonomous region of China based on mitochondrial DNA. Parasit Vectors. (2019) 12:608–10. doi: 10.1186/s13071-019-3857-1
128. Schurer JM, Émilie B, Bryant A, Revell S, Chavis G, Lichtenwalner A, et al. Echinococcus in wild canids in Québec (Canada) and Maine (USA). PLoS Negl Trop Dis. (2018) 12:e0006712. doi: 10.1371/journal.pntd.0006712
129. Breyer I, Georgieva D, Kurdova R. B. Gottstein, Echinococcus granulosus strain typing in Bulgaria: the G1 genotype is predominant in intermediate and definitive wild hosts. Parasitol Res. (2004) 93:127–30. doi: 10.1007/s00436-004-1116-6
130. Bart JM, Abdukader M, Zhang YL, Lin RY, Wang YH, Nakao M, et al. Genotyping of human cystic echinococcosis in Xinjiang. PR China Parasitol. (2006) 133:571–9. doi: 10.1017/S0031182006000734
131. Busi M, Šnábel V, Varcasia A, Garippa G, Perrone V, De Liberato C, et al. Genetic variation within and between G1 and G3 genotypes of Echinococcus granulosus in Italy revealed by multilocus DNA sequencing. Vet. Parasitol. (2007) 50:75–83. doi: 10.1016/j.vetpar.2007.09.003
132. Casulli A, Manfredi MT, La Rosa G, Di Cerbo AR, Genchi C, Pozio E, et al. Echinococcus ortleppi and E. granulosus G1, G2 and G3 genotypes in Italian bovines. Vet Parasitol. (2008) 155:168–72. doi: 10.1016/j.vetpar.2008.04.004
133. Vural G, Baca AU, Gauci CG, Bagci O, Gicik Y, Lightowlers MW, et al. Variability in the Echinococcus granulosus cytochrome C oxidase 1 mitochondrial gene sequence from livestock in Turkey and a re-appraisal of the G1–3 genotype cluster. Vet. Parasitol. (2008) 154:347–50. doi: 10.1016/j.vetpar.2008.03.020
134. Moro PL, Nakao M, Ito A, Schantz PM, Cavero C, Cabrera L, et al. Molecular identification of Echinococcus isolates from Peru. Parasitol Int. (2009) 58:184–6. doi: 10.1016/j.parint.2009.01.005
135. Pour AA, Hosseini SH, Shayan P. Comparative genotyping of Echinococcus granulosus infecting buffalo in Iran using cox1 gene. Parasitol Res. (2011) 108:1229–34. doi: 10.1007/s00436-010-2170-x
136. Mario L, Takano K, Brochado JF, Costa CV, Soares AG, Yamano K, et al. Infection of humans and animals with Echinococcus granulosus (G1 and G3 strains) and E. ortleppi in Southern Brazil. Vet Parasitol. (2011) 177:97–103. doi: 10.1016/j.vetpar.2010.11.018
137. Hajialilo E, Harandi MF, Sharbatkhori M, Mirhendi H, Rostami S. Genetic characterization of Echinococcus granulosus in camels, cattle and sheep from the southeast of Iran indicates the presence of the G3 genotype. J. Helminthol. (2012) 86:263–70. doi: 10.1017/S0022149X11000320
138. Guo ZH, Kubo M, Kudo M, Nibe K, Horii Y, Nonaka N, et al. Growth and genotypes of Echinococcus granulosus found in cattle imported from Australia and fattened in Japan. Parasitol Int. (2011) 60:498–502. doi: 10.1016/j.parint.2011.09.002
139. Nejad MR, Taghipour N, Nochi Z, Mojarad EN, Mohebbi SR, Harandi MF, et al. Molecular identification of animal isolates of Echinococcus granulosus from Iran using four mitochondrial genes. J Helminthol. (2012) 86:485–92. doi: 10.1017/S0022149X1100071X
140. Balbinotti H, Santos GB, Badaraco J, Arend AC, Graichen DÂS, Haag KL, et al. Echinococcus ortleppi (G5) and Echinococcus granulosus sensu stricto (G1) loads in cattle from Southern Brazil. Vet Parasitol. (2012) 188:255–60. doi: 10.1016/j.vetpar.2012.04.007
141. Pezeshki A, Akhlaghi L, Sharbatkhori M, Razmjou E, Oormazdi H, Mohebali M, et al. Genotyping of Echinococcus granulosus from domestic animals and humans from Ardabil Province, northwest Iran. J Helminthol. (2013) 87:387–91. doi: 10.1017/S0022149X1200051X
142. Khademvatan S, Yousefi E, Rafiei A, Rahdar M, Saki J. Molecular characterization of livestock and human isolates of Echinococcus granulosus from south-west Iran. J Helminthol. (2013) 87:240–4. doi: 10.1017/S0022149X12000296
143. Adwan G, Adwan K, Bdir S, Abuseir S. Molecular characterization of Echinococcus granulosus isolated from sheep in Palestine. Exp Parasitol. (2013) 134:195–9. doi: 10.1016/j.exppara.2013.03.024
144. Liu Q, Cao L, Zhang Y, Xu D, Shang L, Wang X, et al. Genotypes of Echinococcus granulosus in animals from Yushu, Northeastern China. Vector Borne Zoonotic Dis. (2013) 13:134–7. doi: 10.1089/vbz.2012.1050
145. Sharma M, Sehgal R, Fomda BA, Malhotra A, Malla N. Molecular characterization of Echinococcus granulosus cysts in north Indian patients: identification of G1, G3, G5 and G6 genotypes. PLoS Negl Trop Dis. (2013) 7:e2262. doi: 10.1371/journal.pntd.0002262
146. Konyaev SV, Yanagida T, Nakao M, Ingovatova GM, Shoykhet YN, Bondarev AY, et al. Genetic diversity of Echinococcus spp in Russia. Parasitology. (2013) 140:1637–47. doi: 10.1017/S0031182013001340
147. Pestechian N, Safa AH, Tajedini M, Rostami-Nejad M, Mousavi M, Yousofi H, et al. Genetic diversity of Echinococcus granulosus in center of Iran. Korean J Parasitol. (2014) 52:413. doi: 10.3347/kjp.2014.52.4.413
148. Wang J, Wang N, Hu D, Zhong X, Wang S, Gu X, et al. Genetic diversity of Echinococcus granulosus in Southwest China determined by the mitochondrial NADH dehydrogenase subunit 2 gene. Sci World J. (2014) 2:867839. doi: 10.1155/2014/867839
149. Zhang T, Yang D, Zeng Z, Zhao W, Liu A, Piao D, et al. Genetic characterization of human-derived hydatid cysts of Echinococcus granulosus sensu lato in Heilongjiang Province and the first report of G7 genotype of E. canadensis in humans in China. PloS ONE. (2014) 9:e109059. doi: 10.1371/journal.pone.0109059
150. Zhong X, Wang N, Hu D, Wang J, Liu T, Gu X, et al. Sequence analysis of cytb gene in Echinococcus granulosus from Western China. Korean J Parasitol. (2014) 52:205. doi: 10.3347/kjp.2014.52.2.205
151. Hu D, Song X, Wang N, Zhong X, Wang J, Liu T, et al. Molecular identification of Echinococcus granulosus on the Tibetan Plateau using mitochondrial DNA markers. Genet Mol Res. (2015) 14:13915–23. doi: 10.4238/2015.October.29.12
152. Amer S, Helal IB, Kamau E, Feng Y, Xiao L. Molecular characterization of Echinococcus granulosus sensu lato from farm animals in Egypt. PLoS ONE. (2015) 10:e0118509. doi: 10.1371/journal.pone.0118509
153. Farhadi M, Fazaeli A, Haniloo A. Genetic characterization of livestock and human hydatid cyst isolates from northwest Iran, using the mitochondrial cox1 gene sequence. Parasitol Res. (2015) 114:4363–70. doi: 10.1007/s00436-015-4673-y
154. Moghaddas E, Borji H, Naghibi A, Shayan P, Razmi GR. Molecular genotyping of Echinococcus granulosus from dromedaries (Camelus dromedarius) in eastern Iran. J Helminthol. (2015) 89:100–4. doi: 10.1017/S0022149X13000631
155. Chaligiannis I, Maillard S, Boubaker GM, Saratsis A, Gottstein B, Sotiraki S, et al. Echinococcus granulosus infection dynamics in livestock of Greece. Acta Trop. (2015) 150:64–70. doi: 10.1016/j.actatropica.2015.06.021
156. Babazadeh M, Sharifiyazdi H, Moazeni M, Gorjipour S, Heidari M. Molecular characterization of a new microvariant of the G3 genotype for Echinococcus granulosus in water buffalo in Iran. Vet Res Forum. (2015) 6:83–7.
157. Sharbatkhori M, Tanzifi A, Rostami S, Rostami M, Harandi MF. Echinococcus granulosus sensu lato genotypes in domestic livestock and humans in Golestan province, Iran. Rev Inst Med Trop. (2016) 58:38. doi: 10.1590/S1678-9946201658038
158. Spotin A, Mahami-Oskouei M, Harandi MF, Baratchian M, Bordbar A, Ahmadpour E, et al. Genetic variability of Echinococcus granulosus complex in various geographical populations of Iran inferred by mitochondrial DNA sequences. Acta Trop. (2017) 165:10–16. doi: 10.1016/j.actatropica.2016.03.002
159. Faruk MO, Siddiki AMA, Karim MF, Masuduzzaman M, Chowdhury S, Islam MS, et al. Molecular characterization of Echinococcus granulosus through amplification of 12S rRNA gene and cox1 gene fragments from cattle in Chittagong, Bangladesh. World Acad Sci Eng Technol Int Sci Int J Biol Biomol Agric Food Biotechnol Eng. (2017) 11:301–5.
160. Ebrahimipour M, Sadjjadi SM, Darani HY, Najjari M. Molecular studies on cystic echinococcosis of camel (Camelus dromedarius) and report of Echinococcus ortleppi in Iran. Iran J Parasitol. (2017) 12:323.
161. Jafari R, Sanei B, Baradaran A, Spotin A, Bagherpour B, Darani HY, et al. Genetic characterization of Echinococcus granulosus strains isolated from humans based on nad1 and cox1 gene analysis in Isfahan, central Iran. J Helminthol. (2018) 92:696–702. doi: 10.1017/S0022149X17000967
162. Oguz B, Ozdal N, Kilinc OO, Deger MS. Preliminary studies on the prevalence and genotyping of Echinococcus granulosus infection in stray dogs in Van Province, Turkey. J Vet Res. (2018) 62:497. doi: 10.2478/jvetres-2018-0061
163. Corrêa F, Stoore C, Horlacher P, Jiménez M, Hidalgo C, Alvarez Rojas CA, et al. First description of Echinococcus ortleppi and cystic echinococcosis infection status in Chile. PLoS ONE. (2018) 13:e0197620. doi: 10.1371/journal.pone.0197620
164. Gorgani-Firouzjaee T, Kalantrai N, Ghaffari S, Alipour J, Siadati S. Genotype characterization of livestock and human cystic echinococcosis in Mazandaran province, Iran. J Helminthol. (2019) 93:255–9. doi: 10.1017/S0022149X1800010X
165. Yan B, Liu X, Wu J, Zhao S, Yuan W, Wang B, et al. Genetic diversity of Echinococcus granulosus genotype G1 in Xinjiang, Northwest of China. Korean J. Parasitol. (2018) 56:391. doi: 10.3347/kjp.2018.56.4.391
166. Abedi B, Maghsood AH, Khansarinejad B, Fallah M, Matini M, Gholami S, et al. Genotyping of Echinococcus granulosus isolates from livestock based on mitochondrial cox1 gene, in the Markazi province, Iran. J Parasit Dis. (2019) 43:592–6. doi: 10.1007/s12639-019-01132-4
167. Ohiolei JA, Yan HB, Li L, Magaji AA, Luka J, Zhu GQ, et al. Cystic echinococcosis in Nigeria: first insight into the genotypes of Echinococcus granulosus in animals. Parasit Vectors. (2019) 12:1–10. doi: 10.1186/s13071-019-3644-z
168. Mohaghegh MA, Yousofi-Darani H, Jafarian AH, Mirbadie SR, Fasihi-Harandi M, Ghavimi R, et al. Isolated human and livestock Echinococcus granulosus genotypes using real-time PCR of cox1 gene in Northeast Iran. Acta Parasitol. (2019) 64:679–5. doi: 10.2478/s11686-019-00117-w
169. Matini M, Fallah M, Maghsood AH, Saidijam M, Harandi MF. Echinococcus granulosus sensu stricto in livestock and human in Hamadan, Western Iran. Iran J Parasitol. (2019) 14:288. doi: 10.18502/ijpa.v14i2.1141
170. Guo B, Zhang Z, Zheng X, Guo Y, Guo G, Zhao L, et al. Prevalence and molecular characterization of Echinococcus granulosus sensu stricto in Northern Xinjiang. China Korean J Parasitol. (2019) 57:153. doi: 10.3347/kjp.2019.57.1.153
171. Laurimäe T, Kinkar L, Varcasia A, Dessì G, Sgroi G, D'Alessio N, et al. First detection of zoonotic tapeworm Echinococcus granulosus sensu lato genotype G7 in continental Italy. Parasitol Res. (2019) 118:2193–201. doi: 10.1007/s00436-019-06346-2
172. Kim HJ, Yong TS, Shin MH, Lee KJ, Park GM, Suvonkulov U, et al. Phylogenetic characteristics of Echinococcus granulosus sensu lato in Uzbekistan. Korean J Parasitol. (2020) 58:205. doi: 10.3347/kjp.2020.58.2.205
173. Alvi MA, Ohiolei JA, Saqib M, Li L, Tayyab MH, Alvi AA, et al. Echinococcus granulosus (sensu stricto) (G1, G3.) and E. ortleppi (G5) in Pakistan: phylogeny, genetic diversity and population structural analysis based on mitochondrial DNA. Parasit. Vectors. (2020) 13:1–10. doi: 10.1186/s13071-020-04199-8
174. Shahbazi AE, Saidijam M, Maghsood AH, Matini M, Haghi MM, Fallah M, et al. Genotyping of fresh and Parafinized human hydatid cysts using nad1 and cox1 genes in Hamadan Province. West Iran Iran J Parasitol. (2020) 15:259. doi: 10.18502/ijpa.v15i2.3309
175. Muqaddas H, Mehmood N, Arshad M. Genetic variability and diversity of Echinococcus granulosus sensu lato in human isolates of Pakistan based on cox1 mt-DNA sequences (366bp). Acta Trop. (2020) 207:105470. doi: 10.1016/j.actatropica.2020.105470
176. Kesik HK, Celik F, Simsek S, Kilinc SG, Ahmed H, Gul A. Molecular characterization and haplotype analyses of lung hydatid cyst isolates of cattle and first report of Echinococcus canadensis. (G6/G7) in cattle isolates in Turkey. Acta Parasitol. (2020) 2021:1–10. doi: 10.1007/s11686-021-00432-1
177. Shahabi S, Sarkari B, Barazesh A. Echinococcus granulosus sensu stricto G1 is the predominant genotype in human and livestock isolates from Turkey and Iran, based on mitochondrial nad5 gene differentiation. Parasit Vectors. (2021) 14:1–6. doi: 10.1186/s13071-021-04869-1
178. Babaei Z, Taherkhani R, Barazesh A, Taherzadeh M, Khorami S, Fouladvand M, et al. Genotyping and phylogenetic analysis of hydatid cysts isolated from livestock in Bushehr province. Iran J Parasit Dis. (2021) 45:197–203. doi: 10.1007/s12639-020-01293-7
179. Miambo RD, Afonso SMS, Noormahomed EV, Malatji MP. Mukaratirwa S. Prevalence and molecular characterization of cystic hydatidosis in livestock slaughtered in southern Mozambique. J Parasit Dis. (2021) 46:186–95. doi: 10.1007/s12639-021-01434-6
180. Nematdoost K, Ashrafi K, Majidi-Shad B, Kia EB, Zeinali A, Sharifdini M. Genetic characterization of Echinococcus granulosus sensu lato in livestock and human isolates from North of Iran indicates the presence of E. ortleppi in Cattle. Acta Parasitol. (2021) 66:446–54. doi: 10.1007/s11686-020-00293-0
Keywords: Echinococcus, prevalence, epidemiology, prevention, global scenario
Citation: Alvi MA and Alsayeqh AF (2022) Food-borne zoonotic echinococcosis: A review with special focus on epidemiology. Front. Vet. Sci. 9:1072730. doi: 10.3389/fvets.2022.1072730
Received: 17 October 2022; Accepted: 16 November 2022;
Published: 20 December 2022.
Edited by:
Masudur Rahman, Sylhet Agricultural University, BangladeshReviewed by:
Filip Štrbac, University of Belgrade, SerbiaCopyright © 2022 Alvi and Alsayeqh. This is an open-access article distributed under the terms of the Creative Commons Attribution License (CC BY). The use, distribution or reproduction in other forums is permitted, provided the original author(s) and the copyright owner(s) are credited and that the original publication in this journal is cited, in accordance with accepted academic practice. No use, distribution or reproduction is permitted which does not comply with these terms.
*Correspondence: Abdullah F. Alsayeqh, YS5hbHNheWVxaEBxdS5lZHUuc2E=
Disclaimer: All claims expressed in this article are solely those of the authors and do not necessarily represent those of their affiliated organizations, or those of the publisher, the editors and the reviewers. Any product that may be evaluated in this article or claim that may be made by its manufacturer is not guaranteed or endorsed by the publisher.
Research integrity at Frontiers
Learn more about the work of our research integrity team to safeguard the quality of each article we publish.