- Center for Infectious Animal Diseases, Faculty of Tropical AgriSciences, Czech University of Life Sciences Prague, Prague, Czechia
Coxiella burnetii is a zoonotic bacterium with an obligatory intracellular lifestyle and has a worldwide distribution. Coxiella burnetii is the causative agent of Q fever in humans and coxiellosis in animals. Since its discovery in 1935, it has been shown to infect a wide range of animal species including mammals, birds, reptiles, and arthropods. Coxiella burnetii infection is of public and veterinary health and economic concern due to its potential for rapid spread and highly infectious nature. Livestock are the primary source of C. burnetii infection in most Q fever outbreaks which occurs mainly through inhalation of contaminated particles. Aside from livestock, many cases of Q fever linked to exposure to wildlife. Changes in the dynamics of human-wildlife interactions may lead to an increased potential risk of interspecies transmission and contribute to the emergence/re-emergence of Q fever. Although C. burnetii transmission is mainly airborne, ticks may act as vectors and play an important role in the natural cycle of transmission of coxiellosis among wild vertebrates and livestock. In this review, we aim to compile available information on vectors, domestic, and wild hosts of C. burnetii, and to highlight their potential role as bacterial reservoirs in the transmission of C. burnetii.
Introduction
Coxiella burnetii, a member of the Coxiellaceae family and the aetiologic agent of Q fever disease in humans and the epizootic disease coxiellosis in animals, is an obligate intracellular gram-negative bacterium. Coxiella burnetii infection occurs in a wide variety of animals such as mammals, birds, reptiles, and arthropods (1). Due to its widespread availability, environmental stability, and low infective dose, C. burnetii is reported as an emerging pathogen and classified as a potential bioterror agent (2).
Coxiella burnetii has a wide and diverse host range. The pathogen primarily affects sheep, goats, and cattle which are considered the primary reservoirs of the pathogen and the primary source of human outbreaks (3, 4). Coxiella burnetii has a worldwide geographical distribution, apart from Antarctica and New Zealand (5, 6). People get infected through inhalation of bacteria contaminated aerosols expelled by infected animal feces, urine, milk, and birth products, while alternative routes of the infection such as sexual, oral, or congenital are uncommon (Figure 1) (7, 8). As the infective dose through inhalation is <10 bacterial cells, exposure to infected animals and their products poses a significant risk for acquiring the pathogen, particularly for farmers and veterinarians (9–11).
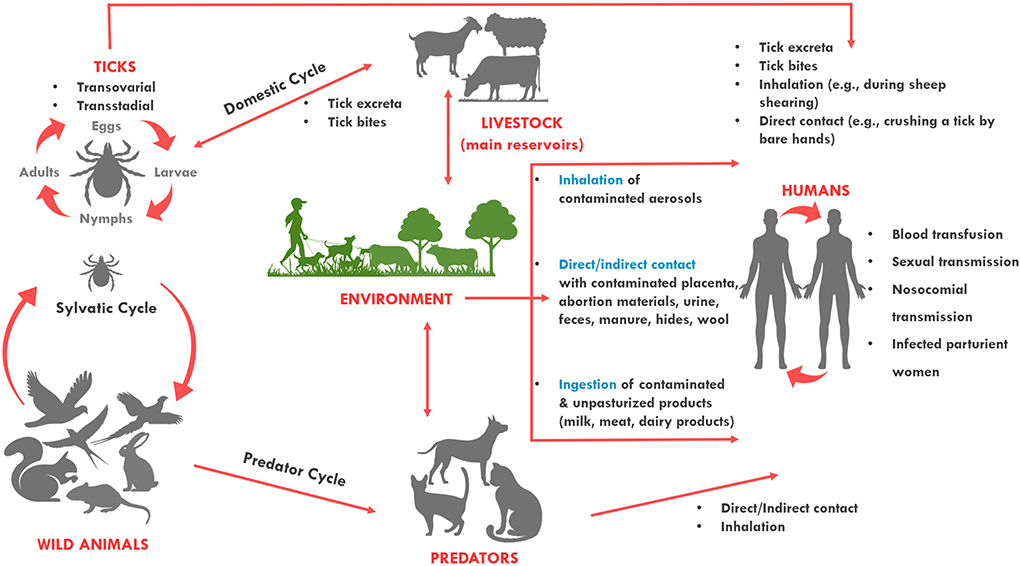
Figure 1. Transmission routes of Coxiella burnetii. The bacterium is most often transmitted to humans during parturition of animals. It can be transmitted to humans when they directly come into contact with or inhale contaminated dust from infected animals. It can also be transmitted from a tick bite or by ingesting contaminated/unpasteurized milk and dairy products. Among humans, the bacterium can be transmitted through blood transfusion, sexual, nosocomial and vertical transmission. Sheep, cattle, and goats are the most common reservoirs of the organism for human infection, but infected cats and less commonly dogs can also transmit the infection to humans. Ticks also harbor the organism and are thought to maintain transmission to wildlife species. Dogs and cats, and humans can also become infected when they contact (or ingest) wildlife species.
Apart from domestic ruminants, a diverse range of other domestic animals (e.g., dog, cat, rabbit, pig, horse, camel, buffalo, rodent, birds) have been reported to be infected with C. burnetii. Additionally, these animals have been also documented to spread the pathogen to humans without manifesting clinical signs of coxiellosis (1). Furthermore, more than a 100 different wild mammal species, which can act as reservoirs for both humans and domestic livestock, have been shown to harbor C. burnetii (12).
In this review, we provide compiled available information on vectors, domestic, and wild hosts of C. burnetii, and highlight their potential role as bacterial reservoirs in the transmission of C. burnetii. This review provides a short synopsis of much broader topics that have been covered in depth by recent publications (5, 12–17) for those who are interested in more details on C. burnetii in animals.
Coxiella burnetii in animals
Coxiella burnetii in ticks
Q fever was first recognized in 1,935 among abattoir workers in Australia by pathologist Derrick (18). Two years later, the pathogenic agent was first isolated from Dermacentor andersonii (Nine Mile isolate) (19) and Haemaphysalis humerosa (20) in the US. Since then, ticks have been discussed as possible vectors for transmission of the bacterium (21).
The role of ticks in Q fever epidemiology is being disputed due to the rare detection of C. burnetii in ticks. Also, difficulties in distinguishing C. burnetii from Coxiella-like endosymbionts (CLEs) of ticks, which are non-infectious for the vertebrate hosts but necessary for tick fitness, is problematic (13, 22). CLEs have a strong genetic similarity to C. burnetii therefore, routine PCR detection usually cross-react and its results may lead to misinterpretation of real prevalence of C. burnetii in ticks (23, 24). Moreover, in contrast to majority of vector-borne diseases, ticks are not essential as vector in transmission of C. burnetii (25). However, several studies have identified ticks as a potential risk for coxiellosis in livestock and other domestic animals (26–30). On the other hand, ticks may play a significant role as reservoirs of C. burnetii in wild animals (31).
Coxiella burnetii was isolated from over 40 species of hard ticks and 14 soft tick species collected from vegetation, and domestic and wild animals (25, 32). Ticks can get infected with C. burnetii either through a blood meal from an infected animal at all stages of their development or transovarially. However, under experimental conditions, not all tick species were capable of being infected or being able to transmit the pathogen to experimental animals or to their offspring (25). Seven species of hard and soft ticks, including D. andersoni, Ha. humerosa, Hyalomma aegyptium, Hy. asiaticum, Ixodes holocyclus, Ornithodoros hermsi, and Or. moubata have yet to be demonstrated in experiments to be competent vectors of C. burnetii (13). In ticks, C. burnetii has been detected in several tick tissues, including midgut, hemolymph, Malpighian tubules, salivary glands, and ovaries (33). Ticks have also been shown to excrete considerable amount of infectious feces (up to 1010 organisms per gram of feces) (34). This finding emphasizes the potential risk of tick-borne infection posed by tick excreta, through inhalation (e.g., among shearers), direct contact (e.g., using bare hands to crush a tick), or tick bites (13, 31, 35).
Nevertheless, from the perspective of public health, the epidemiological importance of C. burnetii tick-borne transmission is lower compared to airborne transmission (13). However, inhaling tick excreta can be a significant source of infection. Additionally, ticks may play a meaningful role in the natural cycle of transmission of coxiellosis among wild-living animals and livestock (31). By crossing these species barriers, C. burnetii may increase its diversity of virulence and resistance factors (13).
Furthermore, several studies showed variable C. burnetii prevalence in ticks collected from vegetation. Although the prevalence of C. burnetii in ticks sampled from animals (6.6%) is higher than in ticks collected from vegetation (2.8%) (36), the C. burnetii prevalence in ticks collected from vegetation varies geographically. For instance, C. burnetii could not detected in 1891 I. ricinus collected in the Netherlands (37), or 887 I. ricinus collected in Sweden (38). However, C. burnetii was detected in I. ricinus, D. reticulatus, D. marginatus, Ha.concinna, and Ha. inermis in Slovakia (39), in I. ricinus, D. marginatus, and Ha.concinna in Hungary (40), in I. ricinus in Germany (41), in Ha. punctata in Spain (42), and in I. ricinus in Austria (43). These results suggest that ticks may play an important role at least in the forest cycle of C. burnetii (31).
Coxiella burnetii in livestock and domestic animals
As already mentioned, livestock is the most frequent reservoir of C. burnetii and frequent contacts with domestic ruminants are one of the most important risk factors for C. burnetii infection in humans. Therefore, the detection and control of infected herds is a critical issue in control of Q fever from the public health perspective (25). Massive excretion of C. burnetii into the environment occurs during parturition in infected females, with shedding a very large number of bacteria in birth products and in urine, feces, and milk. Shedding of C. burnetii may persist over several months in various body tissues and fluids such as vaginal mucus, feces, urine, and milk (44). According to experimental studies, C. burnetii shedding through vaginal discharge is more frequently observed in sheep, while milk is the most common route of pathogen shedding in cattle and goats (45–47).
In most Q fever outbreaks, infected ruminants are typically the primary source of infection (3, 4) and the number of cases is correlated to the local livestock population (48). Transmission of C. burnetii between ruminant hosts mainly occurs via the airborne route. However, other factors such as transboundary animal trade and transportation of animal source foods enhance movement of the pathogen across large distances (16, 49). Aside from livestock, pet animals, especially dogs and cats kept in a close contact with their owners, are known to be important reservoirs of C. burnetii during urban Q fever outbreaks. Many cases of human Q fever have been reported from infected dogs and cats (15, 50–57). Most of these reported cases of Q fever in humans were associated with exposure to parturient pets. Although the main origins of infection in pets remain poorly understood, it has been speculated that dogs and cats may be infected by tick bite, consumption of placenta or milk from infected livestock, consumption of raw meat, inhalation of aerosolized bacteria in the environment, and preying of contaminated animal species (16, 17).
Coxiella burnetii infection has been reported in other domestic mammals—though less frequently than livestock —, including horses, rabbits, pigs, camels, water buffalo, rats, and mice (11, 30, 35, 58–72). So far, serological evidence of C. burnetii infection in many horses have been reported (66, 73–75). However, the epidemiological importance of horses as C. burnetii reservoirs has not been adequately studied.
The role of European rabbits as a reservoir for C. burnetii was evaluated and rabbits were identified as a major source of coxiellosis in livestock and Q fever in humans in Europe, and it is possible that they have a similar role in Australia (68, 76).
The role of pigs in epidemiology of C. burnetii is relatively obscure. Their susceptibility to C. burnetii infection has been confirmed based on previous serological study (77), but there is a lack of evidence that pigs serve as reservoirs of C. burnetii. Until today, no C. burnetii transmission from pigs to humans has been documented. The study on evaluation of the prevalence and genotypes of C. burnetii in pigs from South Korea revealed low seropositivity among pigs (59).
Domestic water buffalo (Bubalus bubalis) is a globally important livestock species due to its high-quality milk, meat and leather. Many studies revealed that buffalo may have a significant impact on the epidemiology of Q fever and emphasizes the necessity for C. burnetii surveillance and control measures in buffalo (60, 65–67).
In camels, C. burnetii is among the most widespread zoonotic pathogens (61). Camel populations worldwide are estimated to be ~30 million, with dromedary camels constituting 95% of the population (78). Dromedary camels (Camelus dromedarius) play an important role as a high-quality protein source for people in semi-dry and arid zones of Africa (5). The most recent C. burnetii serological studies conducted in herds and farms in Africa, Arabian Peninsula, and Asia reported a very high prevalence of Q fever antibodies in the sera of camels (5, 79, 80).
The pathogenesis of Q fever in domestic animals is not fully understood. Coxiella burnetii infections in animals are frequently asymptomatic. In the acute phase, C. burnetii can be detected in the blood, lungs, spleen, and liver (17). When persistent shedding of bacterium occurs in feces and urine, the infection often becomes chronic. However, unlike humans, animals do not usually develop chronic endocarditis (17). Nonetheless, recent studies reported C. burnetii detection in inflamed cardiac valves in slaughtered cattle (81) as well as chronic endometritis in dairy cattle, resulting chronic subfertility (82). Chronic C. burnetii infection mostly affects female uterus and mammary glands. Therefore, the most significant clinical cases of C. burnetii infection is limited to pregnant animals and are commonly associated with abortions, stillbirths, the birth of small or weak offspring, and mastitis (17, 25).
In laboratory animals, C. burnetii inoculation of guinea pigs and mice leads to pneumonia, hepatitis and splenomegaly (83, 84). In addition, the importance of C. burnetii strain in determining the severity of pathological changes was reported (17). Splenomegaly in guinea pigs and mice is considered to be an indication of C. burnetii strain pathogenicity. Furthermore, the method of inoculation appears to impact pathogenesis. In mice, intranasal inoculation causes pneumonia, whereas intraperitoneal inoculation causes hepatosplenomegaly (84).
Little is known about the pathogenesis of C. burnetii infections in companion animals. In pet animals, especially dogs and cats, reproductive anomalies, including dystocia, stillbirths and perinatal mortality have been recorded. However, many studies reported short-lived bacterial shedding and indicated that bacterial shedding by companion animals is rare given the lack of C. burnetii DNA in samples from companion animals (85).
Coxiella burnetii in wildlife
Coxiella burnetii occurs in many free-living and captive wildlife species worldwide that have been suspected to play a role in the epidemiology of Q fever (12, 86–92). González-Barrio and Ruiz-Fons discussed in details about how it is exceedingly difficult to identify C. burnetii infection in wildlife, particularly farmed wild species and free-roaming wildlife (12, 93, 94), and highlighted the importance of daily survey to easily detect reproductive disorders associated to C. burnetii in captive animals of zoological gardens (12).
Natural infections of C. burnetii have been reported in a large variety of wild species (Table 1). Starting with birds, C. burnetii infection has been documented in avian wildlife in addition to farm and pet birds (14, 98)—e.g., barn swallow (Hirundo rustica), eclectus parrot (Eclectus roratus), hen (Gallus gallus domesticus), turkey (Meleagris spp.), magpie (Pica spp.), pheasant (Phasianus colchicus), wood-pigeon (Columba palumbus), turtle dove (Streptopelia turtur), pigeon (Columba livia), house sparrow (Passer domesticus), Italian sparrow (Passer italiae), rook (Corvus frugileus), hooded crow (Corvus cornix), carrion crow (Corvus corone), raven (Corvus corax), Eurasian griffon vulture (Gyps fulvus), black kite (Milvus migrans), redstart (Phoenicurus phoenicurus), white wagtail (Motacilla alba), western yellow wagtail (Motacilla flava), common quail (Coturnix coturnix), Japanese quail (Coturnix japonica), black-headed gull (Chroicocephalus ridibundus), common gull (Larus canus), white-winged tern (Chlidonias leucopterus), common tern (Sterna hirundo), common starling (Sturnus vulgaris), wild ducks (Anas spp.), common blackbird (Turdus merula), fieldfare (Turdus pilaris), thrush nightingale (Luscinia luscinia), willow warbler (Phylloscopus trochilus), great white pelican (Pelecanus onocrotalus), Eurasian reed warbler (Acrocephalus scirpaceus), and wood sandpiper (Tringa graleola). Ebani and Mancianti reviewed C. burnetii infections in birds from 1952, when they were first documented, to the present, and supports the potential role of avian populations in the epidemiology of C. burnetii (14). However, it is yet unclear how C. burnetii spreads among avian wildlife and what factors affect the transmission of the pathogen, as information about prevalence rates in different geographic locations is scarce (14).
The pathogenesis of C. burnetii infection in birds is not well-defined. Previous studies observed Coxiella persistence in birds with (polyorganous lesions resulting in mortality) and without clinical signs (101, 106, 154).
In reptiles, two earlier studies have identified C. burnetii in India, where two tortoises, snakes and skinks were seropositive for C. burnetii (108, 155). A recent study examined turtles in Illinois and Wisconsin, USA, for C. burnetii using qPCR, and 5 out of 605 turtles yielded positive results for C. burnetii (107). Furthermore, C. burnetii has been detected in various reptilian ticks, including tortoise tick H. aegyptium from Romania (156), Amblyomma exornatum from Guinea Bissau, A. nuttalli from Ghana, and A. variegatum in Africa (157).
In mammals, C. burnetii infection has been detected in a broad range of species. In cervids, C. burnetii has been reported in black-tailed deer (Odocoileus hemionus colombianus), California mule deer (O. h. californicus), Rocky Mountain mule deer (O. h. hemionus), and white-tailed deer (Odocoileus virginianus) in Canada and US (123, 135, 136). For European cervids, the infection has been documented in fallow deer (Dama dama), red deer (Cervus elaphus), and roe deer (Capreolus capreolus) (94, 95, 114, 115, 121, 124–133). The serological evidence of the infection was also reported in Sika deer (Cervus arbor) in Japan (134). Furthermore, the presence of C. burnetii has been detected in wild boars, hares, and many rodent species; including Eurasian wild boars (Sus scrofa) (95, 115, 137), European hares (Lepus europaeus) (95, 116), European rabbit (Oryctolagus cuniculus) (68, 70, 76), Japanese hare (Lepus brachyurus) (134), cursor grass mouse (Akodon cursor) (138, 158), bank vole (Myodes glareolus) (88), delta pygmy rice rat (Oligoryzomys nigripes) (138), the Atlantic forest hocicudo (Oxymycterus dasytrichus) (138), field vole (Microtus agrestis) (88), reed vole (Microtus fortis) (139), red vole (Myodes rutilus) (139), black rat (Rattus rattus) (140), brown rat (Rattus norvegicus) (139–141), house mouse (Mus musculus) (42, 138), long-tailed field mouse (Apodemus sylvaticus) (42, 88, 142), large Japanese field mouse (Apodemus speciosus) (139), striped field mouse (Apodemus agrarius) (139), American red squirrel (Tamiasciurus hudsonicus) (143), Carolina flying squirrel (Glaucomys sabrinus) (143), and Siberian chipmunk (Tamias sibiricus) (139). Rodents are considered to be significant reservoirs of infection in the domestic cycle of C. burnetii (159), and several rodent species have been found to be a source of livestock coxiellosis (140, 160).
Additionally, C. burnetii has been implicated in reproductive losses in captive exotic ungulates, including waterbuck (Kobus ellipsiprymnus) (119), sable antelope (Hippotragus niger) (119), and many gazelles, such as arbor gazelle (Gazella arbor neglecta), dama gazelle (Nanger dama mhorr) and Cuvier's gazelle (Gazella cuvieri) (112, 113). Reproductive disorders caused by C. burnetii in endangered species such as exotic ungulates that are bred in captivity for conservation programs may be of critical threat and pose a risk for the programs (113). Other bovids reported to be exposed to/infected by C. burnetii are Alpine ibex (Capra ibex) (110), Bighorn sheep (Ovis arborsis) (111), Alpine chamois (Rupicapra rupicapra) (109), Spanish ibex (Capra pyrenaica) (121), mouflon (Ovis orientalis) (114–117), muskox (Ovibos moschatus) (118), saiga antelope (Saiga tatarica) (120), and yak (Bos mutus) (122).
Apart from terrestrial mammals, C. burnetii has also been detected in marine wildlife—e.g., sea otter (Enhydra lutris) (150), northern fur seal (Callorhinus ursinus) (151, 152), steller sea lion (Eumetopias jubatus) (151, 153, 161), and harbor seal (Phoca vitulina richardsi) (153).
Other mammals that have been shown to harbor C. burnetii are coyote (Canis latrans) (144), red fox (Vulpes vulpes) (88, 116, 145), jaguar (Panthera onca) (148), wild cat (Felis silvestris) (121), common genet (Genetta genetta) (145), western grey kangaroo (Macropus fuliginosus) (149), North African hedgehog (Atelerix algrus) (147), and Amur hedgehog (Erinaceus amurensis) (146).
Coxiellosis causes similar clinical outcomes and pathologies in wild animals as it does in domestic animals (12). Placentitis is one of the most common lesions identified in wild animals with coxiellosis which have been observed in dama gazelle (113), Steller sea lion (161), and Pacific harbor seal (162).
Disease control
Preventive veterinary practices are critical in the control of coxiellosis. Two methods are available to control coxiellosis in animals: vaccination and antibiotic treatment.
Vaccination is one of the most effective management strategies to reduce abortion rates and spread of the bacterium. WOHA recommends only the administration of vaccines containing or prepared from phase I C. burnetii as it has been scientifically showed that the full-length phase I lipopolysaccharide is the protective antigen of C. burnetii (163). Vaccines prevent successive transmission to healthy individuals and humans, and reduce but do not eliminate shedding of the bacterium (164–166). Two vaccines against C. burnetii are currently commercially available for veterinary use in many regions of the world. The first one is an inactivated bivalent vaccine developed from Chlamydia abortus and phase II C. burnetii (Chlamyvax®, Mérial, Lyon, France), indicated for use in sheep and goats. The second one is an inactivated non-adjuvanted phase I C. burnetii antigen Nine Mile strain vaccine (Coxevac®, CEVA Santé Animale, Libourne, France) recommended for use in goats and cattle (167).
Antibiotic treatment is another available option to control coxiellosis in animals. Pregnant animals might have a decrease in abortion rates and C. burnetii shedding by receiving antibiotic treatment with oxytetracycline (20 mg/kg) during the last trimester of pregnancy (168). However, antibiotic treatment of animals is not recommended since the effect of the treatment is not sufficiently demonstrated and proportionate use of antibiotics is required to avoid microbial resistance (8).
Conclusion
Q fever is a significant zoonotic disease worldwide that affects both public and veterinary health, as well as has a detrimental socioeconomic impact on livestock industry. In view of the threats related to this disease, a thorough understanding of transmission routes and potential sources of infection is crucial. Coxiella burnetii has been detected in various hosts, including humans, domestic and wild animals, pets, birds, and arthropods. Ticks are considered as vectors of C. burnetii and may pose a risk for infection of animals and humans. However, further field studies should be implemented to assess the role of the ticks as vectors for C. burnetii under natural conditions. Understanding their role can help us to develop and/or improve vector control strategies that would lead to decrease of C. burnetii risk. It is also noteworthy that our understanding about C. burnetii infection patterns in ticks and role of ticks as possible C. burnetii vectors are limited since CLEs are likely to have been mistakenly identified as C. burnetii in many studies. The development of the diagnostic tests to distinguish between C. burnetii and CLEs to improve our understanding of Q fever epidemiology should also be a key area of future research.
Another point of contention is the role of wildlife—livestock—human interactions which should be further investigated. It is important to develop effective preventive and control strategies using on evidence-based “One Health” approach. In the context of inadequate biosafety controls implemented in the wildlife—livestock—human interface, the possibility of a high rate of transmission of the zoonotic pathogens, including C. burnetii, at these interfaces cannot be precluded.
Further studies are also required to better understand the pathogenicity of C. burnetii for its arthropod and wild hosts. Research about possible routes of transmission of C. burnetii between different host should not be omitted as well. Finally, we should focus also on research evaluating the pathogenicity of CLEs for humans and other mammals or at least their ability to infect them.
Author contributions
SSC and JČ: conceptualization and writing—review and editing. SSC: writing—original draft preparation and visualization. JČ: supervision. All authors contributed to the article and approved the submitted version.
Conflict of interest
The authors declare that the research was conducted in the absence of any commercial or financial relationships that could be construed as a potential conflict of interest.
Publisher's note
All claims expressed in this article are solely those of the authors and do not necessarily represent those of their affiliated organizations, or those of the publisher, the editors and the reviewers. Any product that may be evaluated in this article, or claim that may be made by its manufacturer, is not guaranteed or endorsed by the publisher.
References
2. Rathish B, Pillay R, Wilson A, Pillay VV. Comprehensive review of bioterrorism. In: StatPearls. Treasure Island, FL: StatPearls Publishing (2022).
3. Pouquet M, Bareille N, Guatteo R, Moret L, Beaudeau F. Coxiella burnetii infection in humans: to what extent do cattle in infected areas free from small ruminants play a role? Epidemiol Infect. (2020) 148:e232. doi: 10.1017/S0950268820001880
4. Pexara A, Solomakos N, Govaris A. Q fever and seroprevalence of Coxiella burnetii in domestic ruminants. Vet Ital. (2018) 54:265–79. doi: 10.12834/VetIt.1113.6046.3
5. Devaux CA, Osman IO, Million M, Raoult D. Coxiella burnetii in dromedary camels (Camelus dromedarius): a possible threat for humans and livestock in North Africa and the near and middle east? Front Vet Sci. (2020) 7:558481. doi: 10.3389/fvets.2020.558481
6. Hilbink F, Penrose M, Kovacova E, Kazar J. Q. fever is absent from New Zealand. Int J Epidemiol. (1993) 22:945–9. doi: 10.1093/ije/22.5.945
7. Angelakis E, Raoult D. Q. fever. Vet Microbiol. (2010) 140:297–309. doi: 10.1016/j.vetmic.2009.07.016
9. Vanderburg S, Rubach MP, Halliday JEB, Cleaveland S, Reddy EA, Crump JA. Epidemiology of Coxiella burnetii infection in Africa: a onehealth systematic review. PLoS Negl Trop Dis. (2014) 8:e2787. doi: 10.1371/journal.pntd.0002787
10. Raoult D, Marrie T, Mege J. Natural history and pathophysiology of Q fever. Lancet Infect Dis. (2005) 5:219–26. doi: 10.1016/S1473-3099(05)70052-9
11. Sánchez J, Souriau A, Buendía AJ, Arricau-Bouvery N, Martínez CM, Salinas J, et al. Experimental Coxiella burnetii infection in pregnant goats: a histopathological and immunohistochemical study. J Comp Pathol. (2006) 135:108–15. doi: 10.1016/j.jcpa.2006.06.003
12. González-Barrio D, Ruiz-Fons F. Coxiella burnetii in wild mammals: a systematic review. Transbound Emerg Dis. (2019) 66:662–71. doi: 10.1111/tbed.13085
13. Duron O, Sidi Boumedine K, Rousset E, Moutailler S, Jourdain E. The importance of ticks in Q fever transmission: what has (and has not) been demonstrated? Trends Parasitol. (2015) 31:536–52. doi: 10.1016/j.pt.2015.06.014
14. Ebani VV, Mancianti F. Potential role of birds in the epidemiology of Coxiella burnetii, Coxiella-like agents and Hepatozoon spp. Pathogens. (2022) 11:298. doi: 10.3390/pathogens11030298
15. Abdel-Moein KA, Zaher HM. Parturient cat as a potential reservoir for Coxiella burnetii: a hidden threat to pet owners. Vector Borne Zoonotic Dis. (2021) 21:264–8. doi: 10.1089/vbz.2020.2714
16. Shapiro A, Bosward K, Mathews K, Vincent G, Stenos J, Tadepalli M, Norris J. Molecular detection of Coxiella burnetii in raw meat intended for pet consumption. Zoonoses Public Health. (2020) 67:443– 452. doi: 10.1111/zph.12707
18. Derrick EH. “Q” fever, a new fever entity: clinical features, diagnosis and laboratory investigation. Med J Aust. (1937) 2:281–99. doi: 10.5694/j.1326-5377.1937.tb43743.x
19. Davis GE, Cox HR, Parker RR, Dyer RE. A filter-passing infectious agent isolated from ticks. Public Health Rep. (1938) 53:2259. doi: 10.2307/4582746
20. Smith D, Derrick E. Studies in the epidemiology of Q fever. Aust J Exp Biol Med Sci. (1940) 18:1–8. doi: 10.1038/icb.1940.1
21. Shipman M, Lubick K, Fouchard D, Gurram R, Grieco P, Jutila M, et al. Proteomic and systems biology analysis of the monocyte response to Coxiella burnetii infection. PLoS ONE. (2013) 8:e69558. doi: 10.1371/journal.pone.0069558
22. Hussain S, Perveen N, Hussain A, Song B, Aziz MU, Zeb J, et al. The symbiotic continuum within ticks: opportunities for disease control. Front Microbiol. (2022) 13:854803. doi: 10.3389/fmicb.2022.854803
23. Jourdain E, Duron O, Barry S, González-Acuña D, Sidi-Boumedine K. Molecular methods routinely used to detect Coxiella burnetii in ticks cross-react with Coxiella-like bacteria. Infect Ecol Epidemiol. (2015) 5:29230. doi: 10.3402/iee.v5.29230
24. Duron O, Noël V, McCoy KD, Bonazzi M, Sidi-Boumedine K, Morel O, et al. The recent evolution of a maternally-inherited endosymbiont of ticks led to the emergence of the Q fever pathogen, Coxiella burnetii. PLoS Pathog. (2015) 11:e1004892. doi: 10.1371/journal.ppat.1004892
25. Eldin C, Mélenotte C, Mediannikov O, Ghigo E, Million M, Edouard S, et al. From Q fever to Coxiella burnetii infection: a paradigm change. Clin Microbiol Rev. (2017) 30:115–90. doi: 10.1128/CMR.00045-16
26. van Engelen E, Schotten N, Schimmer B, Hautvast JLA, van Schaik G, van Duijnhoven Y. Prevalence and risk factors for Coxiella burnetii (Q fever) in dutch dairy cattle herds based on bulk tank milk testing. Prev Vet Med. (2014) 117:103–9. doi: 10.1016/j.prevetmed.2014.08.016
27. Cantas H, Muwonge A, Sareyyupoglu B, Yardimci H, Skjerve E. Q fever abortions in ruminants and associated on-farm risk factors in northern Cyprus. BMC Vet Res. (2011) 7:13. doi: 10.1186/1746-6148-7-13
28. Asadi J, Khalili M, Kafi M, Ansari-Lari M, Hosseini SM. Risk factors of Q fever in sheep and goat flocks with history of abortion. Comp Clin Path. (2014) 23:625–30. doi: 10.1007/s00580-012-1661-9
29. Benaissa MH, Ansel S, Mohamed-Cherif A, Benfodil K, Khelef D, Youngs CR, et al. Seroprevalence and risk factors for Coxiella burnetii, the causative agent of Q fever in the dromedary camel (Camelus dromedarius) population in Algeria. Onderstepoort J Vet Res. (2017) 84:e1–7. doi: 10.4102/ojvr.v84i1.1461
30. DráŽovská M, Prokeš M, Vojtek B, MojŽišová J, Ondrejková A, Korytár L. First serological record of Coxiella burnetii infection in the equine population of Slovakia. Biologia. (2022) 77:1645–9. doi: 10.1007/s11756-021-00898-4
31. Kazar J. Coxiella burnetii infection. Ann N Y Acad Sci. (2005) 1063:105–14. doi: 10.1196/annals.1355.018
32. Koka H, Sang R, Kutima HL, Musila L. Coxiella burnetii detected in tick samples from pastoral communities in Kenya. Biomed Res Int. (2018) 2018:8158102. doi: 10.1155/2018/8158102
34. Philip CB. Observations on experimental Q fever. J Parasitol. (1948) 34:457–64. doi: 10.2307/3273312
35. Abdullah H, Hussein HA, El-Razik KA, Barakat AM, Soliman YA. Q fever: a neglected disease of camels in Giza and Cairo Provinces, Egypt. Vet World. (2019) 12:1945–50. doi: 10.14202/vetworld.2019.1945-1950
36. Körner S, Makert GR, Ulbert S, Pfeffer M, Mertens-Scholz K. The prevalence of Coxiella burnetii in hard ticks in Europe and their role in Q fever transmission revisited-a systematic review. Front Vet Sci. (2021) 8:655715. doi: 10.3389/fvets.2021.655715
37. Sprong H, Tijsse-Klasen E, Langelaar M, de Bruin A, Fonville M, Gassner F, et al. Prevalence of Coxiella burnetii in ticks after a large outbreak of Q fever. Zoonoses Public Health. (2012) 59:69–75. doi: 10.1111/j.1863-2378.2011.01421.x
38. Wallménius K, Pettersson JH-O, Jaenson TGT, Nilsson K. Prevalence of Rickettsia spp, Anaplasma phagocytophilum, and Coxiella burnetii in adult Ixodes ricinus ticks from 29 study areas in central and southern Sweden. Ticks Tick Borne Dis. (2012) 3:100–6. doi: 10.1016/j.ttbdis.2011.11.003
39. Reháček J, Úrvölgyi J, Kocianová E, Sekeyová Z, Vavrekova M, Kováčová E. Extensive examination of different tick species for infestation with Coxiella burnetii in Slovakia. Eur J Epidemiol. (1991) 7:299–303. doi: 10.1007/BF00145682
40. Špitalská E, Kocianová E. Detection of Coxiella burnetii in ticks collected in Slovakia and Hungary. Eur J Epidemiol. (2002) 18:263–6. doi: 10.1023/A:1023330222657
41. Hildebrandt A, Straube E, Neubauer H, Schmoock G. Coxiella burnetii and coinfections in Ixodes ricinus ticks in central Germany. Vector Borne Zoonotic Dis. (2011) 11:1205–7. doi: 10.1089/vbz.2010.0180
42. Barandika JF, Hurtado A, García-Sanmartín J, Juste RA, Anda P, García-Pérez AL. Prevalence of tick- borne zoonotic bacteria in questing adult ticks from Northern Spain. Vector Borne Zoonotic Dis. (2008) 8:829–36. doi: 10.1089/vbz.2008.0023
43. Reháček J, Kaaserer B, Úrvölgyi J, Lukáčová M, Kováčová E, Kocianová E. Isolation of Coxiella burnetii and of an unknown rickettsial organism from Ixodes ricinus ticks collected in Austria. Eur J Epidemiol. (1994) 10:719–23. doi: 10.1007/BF01719288
44. Rodolakis A. Q Fever in dairy animals. Ann N Y Acad Sci. (2009) 1166:90–3. doi: 10.1111/j.1749-6632.2009.04511.x
45. Rodolakis A, Berri M, Héchard C, Caudron C, Souriau A, Bodier CC, et al. Comparison of Coxiella burnetii shedding in milk of dairy bovine, caprine, and ovine herds. J Dairy Sci. (2007) 90:5352–60. doi: 10.3168/jds.2006-815
46. Plummer PJ, McClure JT, Menzies P, Morley PS, van den Brom R, van Metre DC. Management of Coxiella burnetii infection in livestock populations and the associated zoonotic risk: a consensus statement. J Vet Intern Med. (2018) 32:1481–94. doi: 10.1111/jvim.15229
47. Szymańska-Czerwińska M, Jodełko A, Zareba-Marchewka K, Niemczuk K. Shedding and genetic diversity of Coxiella burnetii in polish dairy cattle. PLoS ONE. (2019) 14:e0210244. doi: 10.1371/journal.pone.0210244
48. Brom RV den, Engelen E, van Roest HIJ, Hoek W van der, Vellema P. Coxiella burnetii infections in sheep or goats: an opinionated review. Vet Microbiol. (2015) 181:119–29. doi: 10.1016/j.vetmic.2015.07.011
49. Tilburg JJHC, Roest HJIJ, Nabuurs-Franssen MH, Horrevorts AM, Klaassen CHW. Genotyping reveals the presence of a predominant genotype of Coxiella burnetii in consumer milk products. J Clin Microbiol. (2012) 50:2156–8. doi: 10.1128/JCM.06831-11
50. Archer BN, Hallahan C, Stanley P, Seward K, Lesjak M, Hope K, et al. Atypical outbreak of Q fever affecting low-risk residents of a remote rural town in New South Wales. Commun Dis Intell Q Rep. (2017) 41:E125–33.
51. Buhariwalla F, Cann B, Marrie TJ. A dog-related outbreak of Q fever. Clin Infect Dis. (1996) 23:753–5. doi: 10.1093/clinids/23.4.753
52. Pinsky RL, Fishbein DB, Greene CR, Gensheimer KF. An outbreak of cat-associated Q fever in the United States. J Infect Dis. (1991) 164:202–4. doi: 10.1093/infdis/164.1.202
53. Hornok S, Dénes B, Meli ML, Tánczos B, Fekete L, Gyuranecz M, et al. Non-pet dogs as sentinels and potential synanthropic reservoirs of tick-borne and zoonotic bacteria. Vet Microbiol. (2013) 167:700–3. doi: 10.1016/j.vetmic.2013.08.011
54. Laughlin T, Waag D, Williams J, Marrie T. Q fever: from deer to dog to man. Lancet. (1991) 337:676–7. doi: 10.1016/0140-6736(91)92494-M
55. Marrie TJ, Langille D, Papukna V, Yates L. Truckin' pneumonia—an outbreak of Q fever in a truck repair plant probably due to aerosols from clothing contaminated by contact with newborn kittens. Epidemiol Infect. (1989) 102:119–27. doi: 10.1017/S0950268800029757
56. Marrie TJ, Durant H, Williams JC, Mintz E, Waag DM. Exposure to parturient cats: a risk factor for acquisition of Q fever in maritime Canada. J Infect Dis. (1988) 158:101–8. doi: 10.1093/infdis/158.1.101
57. Norris JM, Bosward KL, Heller J. Q fever: pets, vets and validating tests. Microbiol Aust. (2013) 34:186. doi: 10.1071/MA13064
58. Browne AS, Fèvre EM, Kinnaird M, Muloi DM, Wang CA, Larsen PS, et al. Serosurvey of Coxiella burnetii (Q fever) in dromedary camels (Camelus dromedarius) in Laikipia County, Kenya. Zoonoses Public Health. (2017) 64:543–9. doi: 10.1111/zph.12337
59. Seo M-G, Ouh I-O, Lee S-H, Kwak D. Detection and genotyping of Coxiella burnetii in pigs, South Korea, 2014–2015. Emerg Infect Dis. (2016) 22:2192–5. doi: 10.3201/eid2212.161236
60. Klemmer J, Njeru J, Emam A, El-Sayed A, Moawad AA, Henning K, et al. Q fever in Egypt: epidemiological survey of Coxiella burnetii specific antibodies in cattle, buffaloes, sheep, goats and camels. PLoS ONE. (2018) 13:e0192188. doi: 10.1371/journal.pone.0192188
61. Hussein MF, Alshaikh MA, Al-Jumaah RS, GarelNabi A, Al-Khalifa I, Mohammed OB. The Arabian camel (Camelus dromedarius) as a major reservoir of Q fever in Saudi Arabia. Comp Clin Path. (2015) 24:887–92. doi: 10.1007/s00580-014-2002-y
62. Bellabidi M, Benaissa MH, Bissati-Bouafia S, Harrat Z, Brahmi K, Kernif T. Coxiella burnetii in camels (Camelus dromedarius) from Algeria: Seroprevalence, molecular characterization, and ticks (Acari: Ixodidae) vectors. Acta Trop. (2020) 206:105443. doi: 10.1016/j.actatropica.2020.105443
63. Fu M, He P, OuYang X, Yu Y, Wen B, Zhou D, et al. Novel genotypes of Coxiella burnetii circulating in rats in Yunnan Province, China. BMC Vet Res. (2022) 18:204. doi: 10.1186/s12917-022-03310-8
64. Webster JP, Lloyd G, Macdonald DW, Q. fever (Coxiella burnetii) reservoir in wild brown rat (Rattus norvegicus) populations in the UK. Parasitology. (1995) 110:31–5. doi: 10.1017/S0031182000081014
65. Keshavamurthy R, Singh BB, Kalambhe DG, Aulakh RS, Dhand NK. Prevalence of Coxiella burnetii in cattle and buffalo populations in Punjab, India. Prev Vet Med. (2019) 166:16–20. doi: 10.1016/j.prevetmed.2019.03.003
66. Khademi P, Ownagh A, Mardani K, Khalili M. Prevalence of Coxiella burnetii in milk collected from buffalo (water buffalo) and cattle dairy farms in Northwest of Iran. Comp Immunol Microbiol Infect Dis. (2019) 67:101368. doi: 10.1016/j.cimid.2019.101368
67. Kidsin K, Panjai D, Boonmar S. The first report of seroprevalence of Q fever in water buffaloes (Bubalus bubalis) in Phatthalung, Thailand. Vet World. (2021) 14:2574–8. doi: 10.14202/vetworld.2021.2574-2578
68. Caraguel C, Bassett S, González-Barrio D, Elsworth P, Chaber A-L. Comparison of three serological tests for the detection of Coxiella burnetii specific antibodies in European wild rabbits. BMC Vet Res. (2020) 16:315. doi: 10.1186/s12917-020-02526-w
69. Bolaños-Rivero M, Carranza-Rodríguez C, Rodríguez NF, Gutiérrez C, Pérez-Arellano J-L. Detection of Coxiella burnetii DNA in peridomestic and wild animals and ticks in an endemic region (Canary Islands, Spain). Vector Borne Zoonotic Dis. (2017) 17:630–4. doi: 10.1089/vbz.2017.2120
70. Sánchez M, Valcárcel F, González J, González MG, Martín-Hernández R, Tercero JM, et al. Seasonality of Coxiella burnetii among wild rabbits (Oryctolagus cuniculus) and the Hyalomma lusitanicum (Acari: Ixodidae) in a meso-mediterranean ecosystem. Pathogens. (2021) 11:36. doi: 10.3390/pathogens11010036
71. Leon A, Richard E, Fortier C, Laugier C, Fortier G, Pronost S. Molecular detection of Coxiella burnetii and Neospora caninum in equine aborted foetuses and neonates. Prev Vet Med. (2012) 104:179–83. doi: 10.1016/j.prevetmed.2011.11.001
72. Akter R, Legione A, Sansom FM, El-Hage CM, Hartley CA, Gilkerson JR, et al. Detection of Coxiella burnetii and equine herpesvirus 1, but not Leptospira spp. or Toxoplasma gondii, in cases of equine abortion in Australia - a 25 year retrospective study. PLoS ONE. (2020) 15:e0233100. doi: 10.1371/journal.pone.0233100
73. Lubova VA, Leonova GN, Shutikova AL, Bondarenko EI. Indication Q fever pathogen in the south of Far east. Klin Lab Diagn. (2020) 65:724–8. doi: 10.18821/0869-2084-2020-65-11-724-728
74. Desjardins I, Joulié A, Pradier S, Lecollinet S, Beck C, Vial L, et al. Seroprevalence of horses to Coxiella burnetii in an Q fever endemic area. Vet Microbiol. (2018) 215:49–56. doi: 10.1016/j.vetmic.2017.11.012
75. Li J, Li Y, Moumouni PFA, Lee S-H, Galon EM, Tumwebaze MA, et al. First description of Coxiella burnetii and Rickettsia spp infection and molecular detection of piroplasma co-infecting horses in Xinjiang Uygur Autonomous Region, China. Parasitol Int. (2020) 76:102028. doi: 10.1016/j.parint.2019.102028
76. González-Barrio D, Maio E, Vieira-Pinto M, Ruiz-Fons F. European rabbits as reservoir for Coxiella burnetii. Emerg Infect Dis. (2015) 21:1055–8. doi: 10.3201/eid2106.141537
77. Marmion BP, Stoker MGP. Epidemiology of Q fever in great britain. BMJ. (1958) 2:809–16. doi: 10.1136/bmj.2.5100.809
78. Gossner C, Danielson N, Gervelmeyer A, Berthe F, Faye B, Kaasik Aaslav K, et al. Human–dromedary camel interactions and the risk of acquiring zoonotic middle east respiratory syndrome coronavirus infection. Zoonoses Public Health. (2016) 63:1–9. doi: 10.1111/zph.12171
79. Zhu S, Zimmerman D, Deem SL. A review of zoonotic pathogens of dromedary camels. Ecohealth. (2019) 16:356–77. doi: 10.1007/s10393-019-01413-7
80. Selmi R, Mamlouk A, ben Yahia H, Abdelaali H, ben Said M, Sellami K, et al. Coxiella burnetii in Tunisian dromedary camels (Camelus dromedarius): Seroprevalence, associated risk factors and seasonal dynamics. Acta Trop. (2018) 188:234–9. doi: 10.1016/j.actatropica.2018.09.008
81. Agerholm JS, Jensen TK, Agger JF, Engelsma MY, Roest HIJ. Presence of Coxiella burnetii DNA in inflamed bovine cardiac valves. BMC Vet Res. (2017) 13:69. doi: 10.1186/s12917-017-0988-5
82. de Biase D, Costagliola A, del Piero F, di Palo R, Coronati D, Galiero G, et al. Coxiella burnetii in infertile dairy cattle with chronic endometritis. Vet Pathol. (2018) 55:539–42. doi: 10.1177/0300985818760376
83. Russell-Lodrigue KE, Zhang GQ, McMurray DN, Samuel JE. Clinical and pathologic changes in a guinea pig aerosol challenge model of acute Q fever. Infect Immun. (2006) 74:6085–91. doi: 10.1128/IAI.00763-06
84. Russell-Lodrigue KE, Andoh M, Poels MWJ, Shive HR, Weeks BR, Zhang GQ, et al. Coxiella burnetii isolates cause genogroup-specific virulence in mouse and guinea pig models of acute Q fever. Infect Immun. (2009) 77:5640–50. doi: 10.1128/IAI.00851-09
85. Ma GC, Norris JM, Mathews KO, Chandra S, Šlapeta J, Bosward KL, et al. New insights on the epidemiology of Coxiella burnetii in pet dogs and cats from New South Wales, Australia. Acta Trop. (2020) 205:105416. doi: 10.1016/j.actatropica.2020.105416
86. López-Olvera JR, Vidal D, Vicente J, Pérez M, Luján L, Gortázar C. Serological survey of selected infectious diseases in mouflon (Ovis aries musimon) from south-central Spain. Eur J Wildl Res. (2009) 55:75–9. doi: 10.1007/s10344-008-0215-6
87. Kazimírová M, Hamšíková Z, Špitalská E, Minichová L, Mahríková L, Caban R, et al. Diverse tick-borne microorganisms identified in free-living ungulates in Slovakia. Parasit Vectors. (2018) 11:495. doi: 10.1186/s13071-018-3068-1
88. Meredith AL, Cleaveland SC, Denwood MJ, Brown JK, Shaw DJ. Coxiella burnetii (Q-Fever) seroprevalence in prey and predators in the united kingdom: evaluation of infection in wild rodents, foxes and domestic cats using a modified ELISA. Transbound Emerg Dis. (2015) 62:639–49. doi: 10.1111/tbed.12211
89. González J, González MG, Valcárcel F, Sánchez M, Martín-Hernández R, Tercero JM, et al. Prevalence of Coxiella burnetii (Legionellales: Coxiellaceae) infection among wildlife species and the tick Hyalomma lusitanicum (Acari: Ixodidae) in a meso-mediterranean ecosystem. J Med Entomol. (2019) 57:551–6. doi: 10.1093/jme/tjz169
90. Bielawska-Drózd A, Cieslik P, Zakowska D, Głowacka P, Wlizło-Skowronek B, Zieba P, et al. Detection of Coxiella burnetii and Francisella tularensis in tissues of wild-living animals and in ticks of North-west Poland. Pol J Microbiol. (2018) 67:529–34. doi: 10.21307/pjm-2018-059
91. Davoust B, Marié J-L, Pommier de Santi V, Berenger J-M, Edouard S, Raoult D. Three-toed sloth as putative reservoir of Coxiella burnetii, Cayenne, French Guiana. Emerg Infect Dis. (2014) 20:1760–1. doi: 10.3201/eid2010.140694
92. Stevenson S, Gowardman J, Tozer S, Woods M. Life-threatening Q fever infection following exposure to kangaroos and wallabies. BMJ Case Rep. (2015) 2015:bcr2015210808. doi: 10.1136/bcr-2015-210808
93. Arricau-Bouvery N, Rodolakis A. Is Q fever an emerging or re-emerging zoonosis? Vet Res. (2005) 36:327–49. doi: 10.1051/vetres:2005010
94. Ruiz-Fons F, Rodríguez Ó, Torina A, Naranjo V, Gortázar C, de la Fuente J. Prevalence of Coxiella burnetti infection in wild and farmed ungulates. Vet Microbiol. (2008) 126:282–6. doi: 10.1016/j.vetmic.2007.06.020
95. Astobiza I, Barral M, Ruiz-Fons F, Barandika JF, Gerrikagoitia X, Hurtado A, et al. Molecular investigation of the occurrence of Coxiella burnetii in wildlife and ticks in an endemic area. Vet Microbiol. (2011) 147:190–4. doi: 10.1016/j.vetmic.2010.05.046
96. Tokarevich NK, Panferova YuA, Freylikhman OA, Blinova OV, Medvedev SG, Mironov SV, et al. Coxiella burnetii in ticks and wild birds. Ticks Tick Borne Dis. (2019) 10:377–85. doi: 10.1016/j.ttbdis.2018.11.020
97. Ebani VV, Nardoni S, Giani M, Rocchigiani G, Archin T, Altomonte I, et al. Molecular survey on the occurrence of avian haemosporidia, Coxiella burnetii and Francisella tularensis in waterfowl from central Italy. Int J Parasitol Parasites Wildl. (2019) 10:87–92. doi: 10.1016/j.ijppaw.2019.07.008
98. To H, Sakai R, Shirota K, Kano C, Abe S, Sugimoto T, et al. Coxiellosis in domestic and wild birds from Japan. J Wildl Dis. (1998) 34:310–6. doi: 10.7589/0090-3558-34.2.310
99. Martinov S. Contemporary state of the problem Q fever in Bulgaria. Biotechnol Biotechnol Equip. (2007) 21:353–61. doi: 10.1080/13102818.2007.10817473
100. Raska K, Syucek L. Q fever in domestic and wild birds. Bull World Health Organ. (1956) 15:329–37.
101. Ebani VV, Bertelloni F, Mani P. Molecular survey on zoonotic tick-borne bacteria and chlamydiae in feral pigeons (Columba livia domestica). Asian Pac J Trop Med. (2016) 9:324–7. doi: 10.1016/j.apjtm.2016.03.005
102. Stein A, Raoult D. Pigeon pneumonia in provence: a bird-borne Q fever outbreak. Clin Infect Dis. (1999) 29:617–20. doi: 10.1086/598643
103. Babudieri B, Moscovici C. Experimental and natural infection of birds by Coxiella burnetii. Nature. (1952) 169:195–6. doi: 10.1038/169195a0
104. Basova NN, Chernikova TM, Suchkov YG, Rudnev MM. Q fever and psittacosis in wild birds. Vopr Virusol. (1960) 5:586–91.
105. Hubálek Z. Pathogenic microorganisms associated with gulls and terns (Laridae). J Vertebr Biol. (2021) 70:21009.1-98. doi: 10.25225/jvb.21009
106. Vapniarsky N, Barr BC, Murphy B. Systemic coxiella -like infection with myocarditis and hepatitis in an eclectus parrot (Eclectus roratus). Vet Pathol. (2012) 49:717–22. doi: 10.1177/0300985811409251
107. Sander WE, King R, Graser W, Kapfer JM, Engel AI, Adamovicz L, et al. Coxiella burnetii in 3 species of turtles in the upper midwest, United States. Emerg Infect Dis. (2021) 27:3199–202. doi: 10.3201/eid2712.211278
108. Yadav MP, Sethi MS. Poikilotherms as reservoirs of Q-fever (Coxiella burnetii) in Uttar Pradesh. J Wildl Dis. (1979) 15:15–7. doi: 10.7589/0090-3558-15.1.15
109. Pioz M, Loison A, Gauthier D, Gibert P, Jullien J-M, Artois M, et al. Diseases and reproductive success in a wild mammal: example in the alpine chamois. Oecologia. (2008) 155:691–704. doi: 10.1007/s00442-007-0942-5
110. Marreros N, Hüssy D, Albini S, Frey CF, Abril C, Vogt H-R, et al. Epizootiologic investigations of selected abortive agents in free-ranging alpine ibex (Capra ibex ibex) in Switzerland. J Wildl Dis. (2011) 47:530–43. doi: 10.7589/0090-3558-47.3.530
111. DeForge JR, Cone LA. The serologic prevalence of Q fever (Coxiella burnetii) complement-fixing antibodies in the Peninsular bighorn sheep of Southern California. Am J Trop Med Hyg. (2006) 75:315–7. doi: 10.4269/ajtmh.2006.75.315
112. García E, Espeso G, Fernández R, Gómez-Martín Á, Rodríguez-Linde JM, de la Fe C. Coxiella burnetii detected in three species of endangered North African gazelles that recently aborted. Theriogenology. (2017) 88:131–3. doi: 10.1016/j.theriogenology.2016.09.019
113. Lloyd C, Stidworthy MF, Wernery U. Coxiella burnetii abortion in captive dama gazelle (Gazella dama) in the United Arab Emirates. J Zoo Wildl Med. (2010) 41:83–9. doi: 10.1638/2009-0005.1
114. Fernández-Aguilar X, Cabezón Ó, Colom-Cadena A, Lavín S, López-Olvera JR. Serological survey of Coxiella burnetii at the wildlife–livestock interface in the Eastern Pyrenees, Spain. Acta Vet Scand. (2015) 58:26. doi: 10.1186/s13028-016-0209-4
115. Hubálek Z, Juticová Z, Svobodová Š, Halouzka J. A serologic survey for some bacterial and viral zoonoses in game animals in the Czech Republic. J Wildl Dis. (1993) 29:604–7. doi: 10.7589/0090-3558-29.4.604
116. Psaroulaki A, Chochlakis D, Angelakis E, Ioannou I, Tselentis Y. Coxiella burnetii in wildlife and ticks in an endemic area. Trans R Soc Trop Med Hyg. (2014) 108:625–31. doi: 10.1093/trstmh/tru134
117. Ioannou I, Sandalakis V, Kassinis N, Chochlakis D, Papadopoulos B, Loukaides F, et al. Tick-borne bacteria in mouflons and their ectoparasites in Cyprus. J Wildl Dis. (2011) 47:300–6. doi: 10.7589/0090-3558-47.2.300
118. Afema JA, Beckmen KB, Arthur SM, Huntington KB, Mazet JAK. Disease complexity in a declining alaskan muksox (Ovibos moschatus) population. J Wildl Dis. (2017) 53:311–29. doi: 10.7589/2016-02-035
119. Clemente L, Fernandes TL, Barahona MJ, Bernardino R, Botelho A. Confirmation by PCR of Coxiella burnetii infection in animals at a zoo in Lisbon, Portugal. Vet Rec. (2008) 163:221. doi: 10.1136/vr.163.7.221
120. Orynbayev MB, Beauvais W, Sansyzbay AR, Rystaeva RA, Sultankulova KT, Kerimbaev AA, et al. Seroprevalence of infectious diseases in saiga antelope (Saiga tatarica tatarica) in Kazakhstan 2012–2014. Prev Vet Med. (2016) 127:100–4. doi: 10.1016/j.prevetmed.2016.03.016
121. Candela MG, Caballol A, Atance PM. Wide exposure to Coxiella burnetii in ruminant and feline species living in a natural environment: zoonoses in a human–livestock–wildlife interface. Epidemiol Infect. (2017) 145:478–81. doi: 10.1017/S0950268816002454
122. Yin MY, Tan QD, Qin SY, Hu LY, Liu GH, Zhou DH, et al. First serologic survey of Q fever in free- range yaks in China. Iran J Vet Res. (2015) 16:210–2.
123. Chomel BB, Carniciu ML, Kasten RW, Castelli PM, Work TM, Jessup DA. Antibody prevalence of eight ruminant infectious diseases in California mule and black-tailed deer (Odocoileus hemionus). J Wildl Dis. (1994) 30:51–9. doi: 10.7589/0090-3558-30.1.51
124. Giovannini A, Cancellotti FM, Turilli C, Randi E. Serological investigation for some bacterial and viral pathogens in fallow deer (Cervus dama) and wild boar (Sus scrofa) of the San Rossore preserve, Tuscany, Italy. J Wildl Dis. (1988) 24:127–32. doi: 10.7589/0090-3558-24.1.127
125. Smetanova K, Schwarzova K, Kocianova E. Detection of Anaplasma phagocytophilum, Coxiella burnetii, Rickettsia spp, and Borrelia burgdorferi s l. in ticks, and wild-living animals in Western and Middle Slovakia. Ann N Y Acad Sci. (2006) 1078:312–5. doi: 10.1196/annals.1374.058
126. González-Barrio D, Fernández-de-Mera IG, Ortiz JA, Queirós J, Ruiz-Fons F. Long-term dynamics of Coxiella burnetii in farmed red deer (Cervus elaphus). Front Vet Sci. (2015) 2:74. doi: 10.3389/fvets.2015.00074
127. Baradel JM, Barrat J, Blancou J, Boutin JM, Chastel C, Dannacher G, et al. Bilan d'une enquête sérologique effectuée sur différents mammifères sauvages de France. Revue Scientifique et Technique de l'OIE. (1988) 7:861–83. doi: 10.20506/rst.7.4.375
128. Ebani VV, Rocchigiani G, Bertelloni F, Nardoni S, Leoni A, Nicoloso S, et al. Molecular survey on the presence of zoonotic arthropod-borne pathogens in wild red deer (Cervus elaphus). Comp Immunol Microbiol Infect Dis. (2016) 47:77–80. doi: 10.1016/j.cimid.2016.06.003
129. Kreizinger Z, Szeredi L, Bacsadi Á, Nemes C, Sugár L, Varga T, et al. Occurrence of Coxiella burnetii and Chlamydiales species in abortions of domestic ruminants and in wild ruminants in Hungary, Central Europe. J Vet Diagn Invest. (2015) 27:206–10. doi: 10.1177/1040638714563566
130. Tavernier P, Sys SU, de Clercq K, de Leeuw I, Caij AB, de Baere M, et al. Serologic screening for 13 infectious agents in roe deer (Capreolus capreolus) in Flanders. Infect Ecol Epidemiol. (2015) 5:29862. doi: 10.3402/iee.v5.29862
131. Candela MG, Serrano E, Sevila J, León L, Caro MR, Verheyden H. Pathogens of zoonotic and biological importance in roe deer (Capreolus capreolus): Seroprevalence in an agro-system population in France. Res Vet Sci. (2014) 96:254–9. doi: 10.1016/j.rvsc.2014.02.003
132. Blancou J. Serologic testing of wild roe deer (Capreolus capreolus) from the trois fontaines forest region of Eastern France. J Wildl Dis. (1983) 19:271–3. doi: 10.7589/0090-3558-19.3.271
133. Rijks JM, Roest HIJ, van Tulden PW, Kik MJL, Gröne A. Coxiella burnetii infection in roe deer during Q fever epidemic, the Netherlands. Emerg Infect Dis. (2011) 17:2369–71. doi: 10.3201/eid1712.110580
134. Ejercito CLA, Cai L, Htwe KK, Taki M, Inoshima Y, Kondo T, et al. Serological evidence of Coxiella burnetii infection in wild animals in Japan. J Wildl Dis. (1993) 29:481–4. doi: 10.7589/0090-3558-29.3.481
135. Yates L, Embil J, Marrie TJ. Seroepidemiology of Coxiella burnetii among wildlife in nova scotia. Am J Trop Med Hyg. (1993) 49:613–5. doi: 10.4269/ajtmh.1993.49.613
136. Kirchgessner MS, Dubovi EJ, Whipps CM. Seroepidemiology of Coxiella burnetii in wild white-tailed deer (Odocoileus virginianus) in New York, United States. Vector Borne Zoonotic Dis. (2012) 12:942–7. doi: 10.1089/vbz.2011.0952
137. Jado I, Carranza-Rodríguez C, Barandika JF, Toledo Á, García-Amil C, Serrano B, et al. Molecular method for the characterization of Coxiella burnetii from clinical and environmental samples: variability of genotypes in Spain. BMC Microbiol. (2012) 12:91. doi: 10.1186/1471-2180-12-91
138. Rozental T, Ferreira MS, Guterres A, Mares-Guia MA, Teixeira BR, Gonçalves J, et al. Zoonotic pathogens in Atlantic Forest wild rodents in Brazil: Bartonella and Coxiella infections. Acta Trop. (2017) 168:64–73. doi: 10.1016/j.actatropica.2017.01.003
139. Liu L, Manxia H, Ming L, Chao J, Yingqun F, Yu Y, et al. Coxiella burnetii in rodents on Heixiazi island at the Sino-Russian border. Am J Trop Med Hyg. (2013) 88:770–3. doi: 10.4269/ajtmh.12-0580
140. Reusken C, van der Plaats R, Opsteegh M, de Bruin A, Swart A. Coxiella burnetii (Q fever) in Rattus norvegicus and Rattus rattus at livestock farms and urban locations in the Netherlands; could Rattus spp. represent reservoirs for (re)introduction? Prev Vet Med. (2011) 101:124–30. doi: 10.1016/j.prevetmed.2011.05.003
141. Runge M, von Keyserlingk M, Braune S, Becker D, Plenge-Bönig A, Freise JF, et al. Distribution of rodenticide resistance and zoonotic pathogens in Norway rats in Lower Saxony and Hamburg, Germany. Pest Manag Sci. (2013) 69:403–8. doi: 10.1002/ps.3369
142. Pascucci I, di Domenico M, Dall'Acqua F, Sozio G, Cammà C. Detection of lyme disease and Q fever agents in wild rodents in central Italy. Vector Borne Zoonotic Dis. (2015) 15:404–11. doi: 10.1089/vbz.2015.1807
143. Thompson M, Mykytczuk N, Gooderham K, Schulte-Hostedde A. Prevalence of the bacterium Coxiella burnetii in wild rodents from a Canadian natural environment park. Zoonoses Public Health. (2012) 59:553–60. doi: 10.1111/j.1863-2378.2012.01493.x
144. McQuiston JH, Childs JE. Q fever in humans and animals in the United States. Vector Borne Zoonotic Dis. (2002) 2:179–91. doi: 10.1089/15303660260613747
145. Millán J, Proboste T, Fernández de Mera IG, Chirife AD, de la Fuente J, Altet L. Molecular detection of vector-borne pathogens in wild and domestic carnivores and their ticks at the human–wildlife interface. Ticks Tick Borne Dis. (2016) 7:284–90. doi: 10.1016/j.ttbdis.2015.11.003
146. Gong X-Q, Xiao X, Liu J-W, Han H-J, Qin X-R, Lei S-C, et al. Occurrence and genotyping of Coxiella burnetii in Hedgehogs in China. Vector Borne Zoonotic Dis. (2020) 20:580–5. doi: 10.1089/vbz.2019.2589
147. Balti G, Galon C, Derghal M, Souguir H, Guerbouj S, Rhim A, et al. Atelerix algirus, the North African Hedgehog: suitable wild host for infected ticks and fleas and reservoir of vector-borne pathogens in Tunisia. Pathogens. (2021) 10:953. doi: 10.3390/pathogens10080953
148. Widmer CE, Azevedo FCC, Almeida AP, Ferreira F, Labruna MB. Tick-borne bacteria in free-living jaguars (Panthera onca) in Pantanal, Brazil. Vector Borne Zoonotic Dis. (2011) 11:1001–5. doi: 10.1089/vbz.2011.0619
149. Potter AS, Banazis MJ, Yang R, Reid SA, Fenwick SG. Prevalence of Coxiella burnetii in Western Grey Kangaroos (Macropus fuliginosus) in Western Australia. J Wildl Dis. (2011) 47:821–8. doi: 10.7589/0090-3558-47.4.821
150. Duncan C, Gill V, Worman K, Burek-Huntington K, Pabilonia K, Johnson S, et al. Coxiella burnetii exposure in northern sea otters Enhydra lutris kenyoni. Dis Aquat Organ. (2015) 114:83–7. doi: 10.3354/dao02857
151. Minor C, Kersh GJ, Gelatt T, Kondas A, Pabilonia KL, Weller CB, et al. Coxiella burnetii in Northern fur seals and steller sea lions of alaska. J Wildl Dis. (2013) 49:441–6. doi: 10.7589/2012-09-226
152. Duncan C, Kersh GJ, Spraker T, Patyk KA, Fitzpatrick KA, Massung RF, et al. Coxiella burnetii in Northern Fur Seal (Callorhinus ursinus) Placentas from St. Paul Island, Alask. Vector Borne Zoonotic Dis. (2012) 12:192–5. doi: 10.1089/vbz.2011.0715
153. Kersh GJ, Lambourn DM, Raverty SA, Fitzpatrick KA, Self JS, Akmajian AM, et al. Coxiella burnetii infection of marine mammals in the Pacific Northwest, 1997-2010. J Wildl Dis. (2012) 48:201–6. doi: 10.7589/0090-3558-48.1.201
154. Shivaprasad HL, Cadenas MB, Diab SS, Nordhausen R, Bradway D, Crespo R, et al. Coxiella-like infection in psittacines and a toucan. Avian Dis. (2008) 52:426–32. doi: 10.1637/8192-120707-Reg
155. Stephen S, Rao KN. Coxiellosis in reptiles of South Kanara district, Karnataka. Indian J Med Res. (1979) 70:937–41.
156. Paştiu AI, Matei IA, Mihalca AD, D'Amico G, Dumitrache MO, Kalmár Z, et al. Zoonotic pathogens associated with Hyalomma aegyptium in endangered tortoises: evidence for host-switching behaviour in ticks? Parasit Vectors. (2012) 5:301. doi: 10.1186/1756-3305-5-301
157. Mendoza-Roldan JA, Mendoza-Roldan MA, Otranto D. Reptile vector-borne diseases of zoonotic concern. Int J Parasitol Parasites Wildl. (2021) 15:132–42. doi: 10.1016/j.ijppaw.2021.04.007
158. Tokarz R, Markotić A, Margaletić J, Lipkin WI, Habuš J, Jain K, et al. Molecular survey of zoonotic agents in rodents and other small mammals in Croatia. Am J Trop Med Hyg. (2016) 94:466–73. doi: 10.4269/ajtmh.15-0517
159. Aitken ID. Clinical aspects and prevention of Q fever in animals. Eur J Epidemiol. (1989) 5:420–4. doi: 10.1007/BF00140132
160. Foronda P, Plata-Luis J, del Castillo-Figueruelo B, Fernández-Álvarez Á, Martín-Alonso A, et al. Serological survey of antibodies to Toxoplasma gondii and Coxiella burnetii in rodents in north-western African islands (Canary Islands and Cape Verde). Onderstepoort J Vet Res. (2015) 82:e1–4. doi: 10.4102/ojvr.v82i1.899
161. Kersh GJ, Lambourn DM, Self JS, Akmajian AM, Stanton JB, Baszler T, et al. Coxiella burnetii infection of a steller sea lion (Eumetopias jubatus) found in Washington state. J Clin Microbiol. (2010) 48:3428–31. doi: 10.1128/JCM.00758-10
162. Lapointe J-M, Gulland FM, Haines DM, Barr BC, Duignan PJ. Placentitis due to Coxiella burnetii in a pacific harbor seal (Phoca vitulina richardsi). J Vet Diagn Invest. (1999) 11:541–3. doi: 10.1177/104063879901100612
163. OIE. Manual of Diagnostic Tests and Vaccines for Terrestrial Animals. Chapter 3.1.17. Q Fever. Paris: OIE (2018). Available online at: https://www.woah.org/fileadmin/Home/eng/Health_standards/tahm/3.01.17_Q_FEVER.pdf
164. Guatteo R, Seegers H, Taurel A-F, Joly A, Beaudeau F. Prevalence of Coxiella burnetii infection in domestic ruminants: a critical review. Vet Microbiol. (2011) 149:1–16. doi: 10.1016/j.vetmic.2010.10.007
165. Arricau-Bouvery N, Souriau A, Bodier C, Dufour P, Rousset E, Rodolakis A. Effect of vaccination with phase I and phase II Coxiella burnetii vaccines in pregnant goats. Vaccine. (2005) 23:4392–402. doi: 10.1016/j.vaccine.2005.04.010
166. Hogerwerf L, van den Brom R, Roest HIJ, Bouma A, Vellema P, Pieterse M, et al. Reduction of Coxiella burnetii prevalence by vaccination of goats and sheep, the Netherlands. Emerg Infect Dis. (2011) 17:379–86. doi: 10.3201/eid1703.101157
167. Long CM. Q fever vaccine development: current strategies and future considerations. Pathogens. (2021) 10:1223. doi: 10.3390/pathogens10101223
Keywords: Coxiella burnetii, Q fever, coxiellosis, ticks, livestock, wildlife
Citation: Celina SS and Cerný J (2022) Coxiella burnetii in ticks, livestock, pets and wildlife: A mini-review. Front. Vet. Sci. 9:1068129. doi: 10.3389/fvets.2022.1068129
Received: 12 October 2022; Accepted: 31 October 2022;
Published: 11 November 2022.
Edited by:
Valentina Virginia Ebani, University of Pisa, ItalyReviewed by:
Ana Cláudia Coelho, University of Trás-os-Montes and Alto Douro, PortugalCopyright © 2022 Celina and Cerný. This is an open-access article distributed under the terms of the Creative Commons Attribution License (CC BY). The use, distribution or reproduction in other forums is permitted, provided the original author(s) and the copyright owner(s) are credited and that the original publication in this journal is cited, in accordance with accepted academic practice. No use, distribution or reproduction is permitted which does not comply with these terms.
*Correspondence: Seyma S. Celina, Y2VsaW5hc2V5bWEmI3gwMDA0MDtnbWFpbC5jb20=