- 1Clinic for Swine and Small Ruminants, Forensic Medicine and Ambulatory Service, University of Veterinary Medicine Hannover, Foundation, Hanover, Germany
- 2Department of Biometry, Epidemiology and Information Processing, WHO Collaborating Centre for Research and Training Health at the Human-Animal-Environment Interface, University of Veterinary Medicine Hannover, Foundation, Hanover, Germany
- 3Food and Veterinary Institute Braunschweig/Hannover, Lower Saxony State Office for Consumer Protection and Food Safety (LAVES), Hanover, Germany
Introduction: Sheep are considered to be one of the main reservoirs for Coxiella burnetii, a gram-negative bacterium with high zoonotic potential. Infected sheep shed tremendous amounts of the pathogen through birth products which caused human Q fever epidemics in several countries. Information about the impact of an inactivated C. burnetii Phase I vaccine on humoral immune response, vaginal shedding, and lamb mortality in naturally pre-infected sheep is scarce.
Methods: Two identically managed and naturally C. burnetii-infected sheep flocks were examined for two lambing seasons (2019 and 2020). One flock (VAC) received a primary vaccination against Q fever before mating and the second flock served as control (CTR). In each flock, one cohort of 100 ewes was included in follow-up investigations. Serum samples at eight different sampling dates were analyzed by C. burnetii phase-specific ELISAs to differentiate between the IgG Phase I and II responses. Vaginal swabs were collected within three days after parturition and examined by a C. burnetii real-time PCR (IS1111). Lamb losses were recorded to calculate lamb mortality parameters.
Results: After primary vaccination, almost all animals from cohort VAC showed a high IgG Phase I response up until the end of the study period. In cohort CTR, the seropositivity rate varied from 35.1% to 66.3%, and the Phase I and Phase II pattern showed an undulating trend with higher IgG Phase II activity during both lambing seasons. The number of vaginal shedders was significantly reduced in cohort VAC compared to cohort CTR during the lambing season in 2019 (p < 0.0167). There was no significant difference of vaginal shedders in 2020. The total lamb losses were low in both cohorts during the two investigated lambing seasons (VAC 2019: 6.8%, 2020: 3.2%; CTR 2019: 1.4%, 2020: 2.7%).
Discussion: Neither the C. burnetii vaccine nor the C. burnetii infection seem to have an impact on lamb mortality. Taken together, the inactivated C. burnetii Phase I vaccine induced a strong IgG Phase I antibody response in naturally pre-infected sheep. It might also reduce vaginal shedding in the short term but seems to have little beneficial impact on lamb mortality.
Introduction
Coxiella burnetii is a gram-negative bacterium with high zoonotic potential, with ruminants being the main reservoir. Inhalation of contaminated aerosols and dust lead to infection with C. burnetii in humans and animals, respectively. Most human Q fever epidemics are associated with aborting or lambing sheep and goats (1, 2). Tremendous amounts of the pathogen are shed through birth products. Acute clinical symptoms in humans are shown by approximately half of the infected people mostly having severe flu-like symptoms (3). As a consequence of an acute infection, Q fever fatigue syndrome can occur in up to 20% of the patients and can last for several years (4). A small number of infected individuals develop chronic Q fever, which manifests as endocarditis or infections of aneurysms or vascular prostheses (5).
Abortion, stillbirth, and postpartum diseases such as retained placenta and (endo)metritis have been associated with C. burnetii infection in dairy cattle, but definitive evidence that C. burnetii is the causative agent is lacking (6, 7). In goat flocks, C. burnetii causes endemic abortion with losses of up to 90% or weak kids with low body weight (8, 9). Metritis can be present in goats after C. burnetii abortion (9), but this was not confirmed under experimental conditions (8). Experimentally infected sheep did not abort but gave birth to non-viable lambs (10). Under field conditions, C. burnetii shedding ewes gave birth to healthy lambs (11–14) and the abortion rate in infected sheep flocks might be <3% (15–18). Such a low number is tolerated in healthy sheep flocks and investigations are usually not initiated (19, 20). Co-infections with other abortifacient agents like Chlamydia (Chl.) abortus, Toxoplasma (T.) gondii, and border disease virus increases the abortion rates in a C. burnetii-infected sheep flock (21–25) and leads to a potential adverse overestimation of C. burnetii on the reproductive performance. Different ovine abortifacient agents such as Chl. abortus and Listeria monocytogenes, cause brownish vaginal exudate, placental retention, and endometritis (26, 27), but the impact of C. burnetii on the ewes' health post-partum is still unknown. Moreover, data about the effect of an inactivated C. burnetii Phase I vaccine on perinatal complications in sheep are missing.
C. burnetii undergoes a lipopolysaccharide (LPS) phase variation in which its virulent smooth LPS phase, Phase I (PhI), converts to an avirulent rough LPS phase, Phase II (PhII), upon a serial passage in non-immunocompetent subjects (28, 29). Both phase variations are used for diagnostic purposes to determine the clinical status of a C. burnetii infection. In general, an increase in IgG PhII antibodies without or with a low IgG PhI response is interpreted as a recently acquired infection (30, 31). The presence of IgG PhI alone or in combination with similar or lower IgG PhII antibody levels has been associated with antigen contact in the past (32, 33). Recently, the phase-specific serology was used to characterize the humoral immune response in infected C. burnetii sheep flocks after vaccination (33, 34). Due to the long-lasting IgG PhI response induced by the vaccine, booster vaccination for C. burnetii-infected and vaccinated sheep does not appear to be necessary, but further studies are needed to prove this concept (33).
In the mouse model, antibodies were unable to completely control C. burnetii infection but prevented the development of clinical disease at an early stage (35, 36). Moreover, the antibody response alone was unable to eliminate a C. burnetii infection (37), but B cells might play a crucial role in clearance of C. burnetii and in regulation of inflammatory response (38). The TH1-mediated cellular immunity was based on the release of proinflammatory cytokines such as interferon-γ (INF-γ). Impaired or absent INF-γ production by T cells resulted in an increased mortality rate of infected mice (39). Consequently, protective immunity is only achieved by humoral and cell-mediated immunity. The cell-mediated immunity eliminates the pathogen, and specific antibodies accelerate this process (40). To date, routine diagnostic assays for evaluating the cell-mediate response of C. burnetii are not available for large sample quantities in veterinary medicine.
Since 2010, a formalin-inactivated C. burnetii Nine Mile strain Phase I whole-cell vaccine (Coxevac®, CEVA Santé Animale, Libourne, France) is licensed for cattle and goats in several European countries (41). This vaccine is also commonly applied to sheep with 1 mL per vaccine dose, but information about efficiency is scarce (33). A decrease in vaginal shedding after vaccination was reported in several studies (16, 23, 33), but a significant difference was not achieved between non-vaccinated and immunized sheep (42, 43). Furthermore, vaginal shedding was also reduced in some flocks at the following lambing season without vaccination, indicating a self-limitation of Q fever in sheep flocks (15, 44, 45). Assumably, an increasing herd immunity contributes to the interruption of infection cycle, but this hypothesis needs further investigations (34, 42).
The present field study had two major objectives. Firstly, to evaluate the effect of an inactivated C. burnetii Phase I vaccine on phase-specific IgG response and vaginal pathogen shedding in a pre-infected flock. Secondly, to investigate the influence of an inactivated C. burnetii Phase I vaccine on perinatal complications in ewes and lambs. For both these aims, two identically managed naturally pre-infected C. burnetii sheep flocks were examined during two subsequent lambing seasons. One sheep flock was vaccinated against C. burnetii, and the second flock served as control.
Materials and methods
Flock description and Q fever history
The extensive sheep farm consisted of two sheep flocks (CTR and VAC) which were kept strictly separated because they were located in two different German federal states, namely Schleswig-Holstein and Mecklenburg-Western Pomerania. Each flock contained approximately 800 crossbred ewes (German Blackheaded Mutton x Coburg Fox). Both extensive flocks were managed identically by the same farmer. In addition, flock VAC was taken care of by shepherd A and flock CTR by shepherd B. Each year, the two flocks lambed consecutively on pasture in spring and ewes with lambs were transported to the same lambing barn and stayed there for less than five days. Afterwards, ewes and lambs were returned to the pastures, but animals from each flock were never mixed with the other flock.
In April 2017, flock CTR suffered several abortions and C. burnetii was diagnosed in one placenta from an aborted sheep (Cq 31; LSI VetMAXTMCoxiella burnetii, Thermo Fisher Scientific, Germany). Moreover, a proportion of 11.8% of examined ewes (n = 17) had antibodies against C. burnetii (Q Fever Antibody Test Kit, IDEXX, Liebefeld, Switzerland). At the same time, preputial swabs from 12 breeding sires were collected as a novel diagnostic tool to detect C. burnetii DNA (46), and five of these samples tested C. burnetii positive (Cq 37, 38, 38, 40, 42). In December 2017, blood samples were collected from 90 sheep for the annual flock health check. These specimens were also analyzed for C. burnetii antibodies by an ELISA (Q Fever Antibody Test Kit, IDEXX, Liebefeld, Switzerland), and a detection rate of 6.7% was obtained. In April 2018, flock CTR was revisited, and vaginal swabs and blood samples from 42 ewes were collected after lambing. None of the vaginal swabs tested C. burnetii DNA positive by real-time PCR (LSI VetMAXTMCoxiella burnetii, Thermo Fisher Scientific, Germany), but 16.7% of the animals showed a C. burnetii antibody response analyzed by the commercial ELISA (Q Fever Antibody Test Kit, IDEXX, Liebefeld, Switzerland).
In flock VAC, no reproductive problems occurred during the lambing season from March to April 2017. Nevertheless, samples were also collected from flock VAC after lambing in April 2017 to determine a possible Q fever infection. C. burnetii antibodies were detected in one female sheep (n = 19; Q Fever Antibody Test Kit, IDEXX, Liebefeld, Switzerland), and one preputial swab from a breeding ram (n = 11) tested C. burnetii positive by real-time PCR (Cq 35; LSI VetMAXTMCoxiella burnetii, Thermo Fisher Scientific, Germany). In December 2017, a low intra-flock seroprevalence of 2.3% was determined during a Q fever study by a commercial ELISA, and all preputial (n = 21) and pre-lambing vaginal swabs (n = 20) tested negative (46). However, 35 vaginal swabs (n = 44) tested C. burnetii positive (Cq 22–40; LSI VetMAXTMCoxiella burnetii, Thermo Fisher Scientific, Germany) taken from the lambing sheep in April 2018, and 15.9% of these animals had a positive C. burnetii antibody activity (Q Fever Antibody Test Kit, IDEXX, Liebefeld, Switzerland).
An overview of the Q fever history of both flocks from April 2017 to April 2018 is given in Table 1.
In July 2020, both shepherds (A and B) were tested for C. burnetii antibodies by a semi-quantitative Q fever immunofluorescence assay, IgG, (Focus Diagnostics, Cypress, CA, USA), as described elsewhere (47) per the treating physician's request. The following IgM and IgG titers were detected in shepherd A: IgM Ph I and Ph II negative, IgG Ph I and Ph II: 1:64; shepherd B: IgM Ph I 1:32, IgM Ph II <1:16, IgG Ph I 1:64, IgG Ph II: 1:4,096.
Vaccination
We decided to vaccinate all ewes (excluding gimmers) from flock VAC due to the detection of C. burnetii DNA during the lambing season in 2018 and to leave flock CTR unvaccinated as a control. This decision was supported by the assumption that the zoonotic risk from flock CTR was possibly lower due to the missing pathogen detection in sheep examined in April 2018. All ewes from flock VAC received 1 mL of an inactivated C. burnetii Phase I vaccine (Coxevac®, Ceva, Libourne, France, ChB: 1010FG1B) in August 2018. The volume of 1 mL contains 72 Q fever units (relative potency of Phase I antigen measured by ELISA in comparison with a reference item) and is approximately equivalent to 100 mg of inactivated corpuscular Phase I antigen of C. burnetii according to the manufacturer. A skin fold behind the shoulder was created to inject the vaccine subcutaneously with an automatic syringe (HSW ECO-MATIC, 1 mL, Henke Sass Wolf GmbH, Tuttlingen, Germany). Injection was performed with a new needle (Hypodermic-Needle, 20Gx1½”, WDT, Garbsen, Germany) for every 10th sheep. Three weeks later, the second dose was given, and thereby the primary vaccination was completed 4 weeks before tupping as recommended by the manufacturer. Flock VAC was only vaccinated in 2018, and no booster vaccination was administered in 2019.
Study cohorts
The number of ewes required from each flock to estimate the vaccine impact on vaginal shedding was calculated on the assumption of 2% and 20% expected vaginal shedders in the vaccinated and control flock, respectively, 90% power and 5% precision. Therefore, 100 healthy multiparous ewes aged between three and four years from each flock were included in the investigations. These animals were clinically examined and individually ear-tagged (Twintag, Kleißner, Tauberbischofsheim, Germany). Thereby, an individual follow-up of every study ewe was possible during the entire study period from August 2018 to July 2020. The amount of study ewes declined in both flocks due to animal losses in summer/winter 2019, and a reduced number of ewes were available during the lambing season in 2020.
Blood sample and vaginal swab collection
In August and September 2018, both study cohorts were blood sampled at the Vena jugularis (KABEVETTE®, KABE, Nümbrecht-Elsenroth, Germany). Samples were simultaneously collected at the vaccination date of study cohort VAC. Before the lambing seasons started, blood samples were taken from both cohorts in January/February 2019 and in January 2020. During the lambing seasons in 2019 and 2020, blood samples and vaginal swabs (Sarstedt, Nümbrecht, Germany) were taken within three days after the ewes had lambed or aborted. Approximately three months after the lambing season, the sheep were blood sampled again in June 2019/2020 (cohort VAC) and July 2019/2020 (cohort CTR). Blood samples were centrifuged within 6 h of sampling. The vaginal swabs and sera were stored at −18°C until laboratory examination. An overview of the sampling procedure is presented in Figure 1.
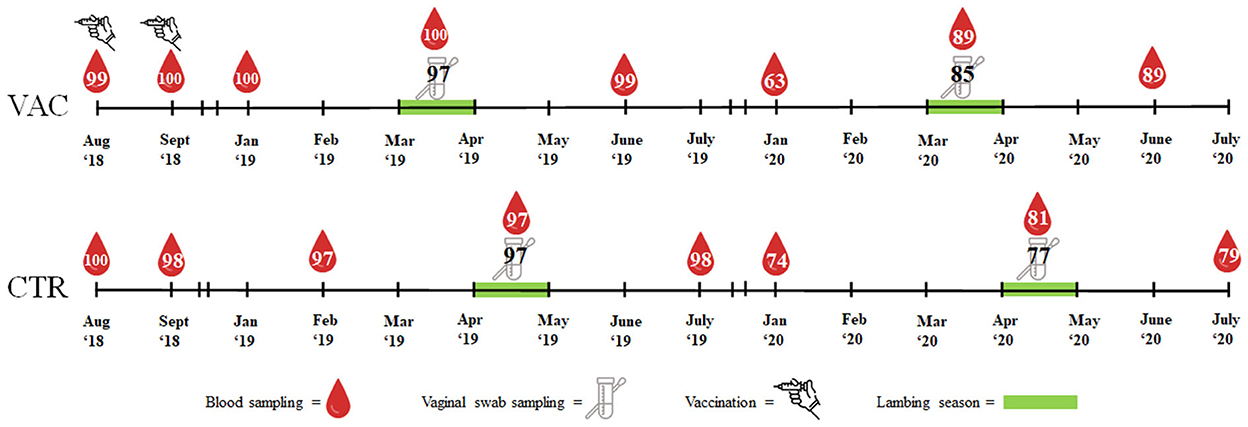
Figure 1. Overview of vaccination, sample types and number of samples in two sheep flocks. VAC, sheep flock vaccinated against Coxiella burnetii; CTR, control flock.
Laboratory examination of serum samples and vaginal swabs
Sheep sera were examined with two phase-specific ELISAs (EUROIMMUN, Lübeck, Germany). Each phase-specific ELISA detected either IgG PhI or IgG PhII antibodies separately. These ELISAs were applied in accordance with the manufacturer's instructions and have been recently described in detail (33). The test results were presented quantitatively in relative Units (RU) determined by a standard curve. The classification was as follows: <16 RU: negative, ≥16 RU till <22 RU: uncertain, and ≥22 RU: positive. This classification applies to both phase-specific ELISA tests.
DNA from the vaginal swabs was extracted with the NucleoSpin Tissue Kit (Macherey Nagel, Düren, Germany) in accordance with the manufacturer's instructions using the KingFisherTM Flex (ThermoFisher Scientific, Dreieich, Germany). C. burnetii-specific DNA-fragments were detected by amplifying IS1111 elements with a real-time PCR (LSI VetMAXTMCoxiella burnetii, Thermo Fisher Scientific, Germany). The real-time PCR was performed in accordance with the manufacturer, and cycle quantification (Cq) values of ≤ 45 were assessed as positive.
Reproductive performance
The number of lamb losses due to abortion, stillbirth, dystocia and postnatal death within three days were recorded to evaluate the effect of the C. burnetii vaccine on lamb mortality in naturally pre-infected sheep flocks. Live-born lambs were weighed with a baby scale (MTB 200, ADAM, Milton Keynes, UK), and the rectal body temperature of the lamb (mirolife VT 1831, Microlife AG, Widnau, Switzerland) was measured within 6 h after parturition. Simultaneously, a modified Apgar score (48) was applied to assess the vitality of the new born lambs (Table 2). The total scores were assessed as follows: 10 points = excellent condition, 6–9 points = moderately depressed, 0–5 points = severely depressed.
The percentage of abortion rate, stillbirth rate, lamb mortality, and total lamb losses were determined as previously described by Voigt and colleagues (49), with a slight modification of the total lamb losses including only lambs which had died within three days post-partum. Moreover, the rate of lambs which had died due to dystocia was reported.
The ewes were also weighed with a livestock scale (WA 200, Meier-Brakenberg, Extertal, Germany) within 6 h after parturition. Three days after lambing, the rectal body temperature (mirolife VT 1831, Microlife AG, Widnau, Switzerland) was taken, and the presence of vaginal discharge was evaluated to receive information about post-partum disorders in the ewes. The extensive husbandry conditions with guard dogs and predators [e.g., ravens (Corvus corax)] hampered the assessment of placental expulsion, because placentas were immediately eaten by these animals soon after the sheep's parturition. Therefore, this information was not included in the interpretation of the data due to the small number of observations.
Necropsy of lambs
During the lambing season in 2019 and 2020, necropsy was performed on aborted, stillborn, and dead lambs from both cohorts by using standardized protocols in accordance with the German Federal Research Institute for Animal Health (50). Samples from the lung, liver, and spleen were taken from each carcass, pooled, and investigated with real-time PCR for the presence of C. burnetii as described above for the vaginal swabs. In Germany, Chl. abortus is the main abortive pathogen in sheep, and co-infection with C. burnetii occurs regularly (23, 49). Therefore, organ pool samples were analyzed for the presence of Chl. abortus DNA by real-time PCR in accordance with published protocols (51, 52). Cycle quantification (Cq) values from the Chl. abortus PCR of <38 was assessed as positive.
Prior to the start of the lambing season in 2020, an increased number of ewes and yearlings aborted in flock CTR. Therefore, investigations were extended in this flock to identify the abortifacient agent. Abortion material from non-study ewes was included and examined as described above. Moreover, the organ pools were tested for a broader range of pathogens such as Brucella sp., Campylobacter fetus, Bluetongue Virus, Schmallenberg Virus, and Neospora caninum. In addition, brain tissue was tested for the presence of T. gondii. In the spring of 2020, Germany was seriously affected by the Covid-19 pandemic, and not all samples could be analyzed for the described pathogens. Details of the performed assays to detect the mentioned abortifacient agents are summarized in Supplementary Table S1.
Statistical analysis
First of all, data from the serological and molecular analyses as well as the animals' conditions were evaluated descriptively by calculating measures of location scales and dispersion measures.
The IgG PhI and IgG PhII values obtained by the ELISAs from all animals were examined by means of a fixed effects linear model. Herd and sampling time were included in the model and their interaction was added. Since the same animals were sampled at each time point, the sampling time was added in the model as a repeated measure of the ewes examined. Differences between IgG PhI and IgG PhII at each sampling time within each cohort were compared by the Wilcoxon Signed-Rank test. Moreover, the serological results from each seropositive animal at each sampling date were included in further analysis. In detail, the IgG PhII values were divided by the IgG PhI ELISA outcomes. This quotient was established to evaluate the development of phase-specific IgG response against C. burnetii during the entire study period of individual sheep. Quotients >1 indicated an increased IgG PhII response, whereas values of <1 demonstrated a higher IgG PhI activity. The McNemar test was used to check for significant differences of the quotient at two consecutive sampling times.
Several Fisher's exact tests were used to check whether there was a significant difference in the number of C. burnetii shedding animals in the two flocks and the two lambing seasons in 2019 and 2020.
Data on the condition of the ewes and lambs were examined for significant differences between the cohorts VAC and CTR. An independent two-sample t-test was used for continuous variables and a Fisher's exact test was applied for categorical variables.
For all calculations, we used the statistical software SAS (SAS Institute Inc., Cary, NC, USA).
Ethics approval statement
The procedures on sheep in both flocks were licensed by the federal state governments of Schleswig-Holstein (Az. V 242-39872/2018) and Mecklenburg-Western Pomerania (Az. 7221.3-2-017/18) and were conducted in accordance with German animal welfare legislation and the EU Directive 2010/63/EU for animal experiments. All animals were handled according to high ethical standards and national legislation. Written informed consent was obtained from the owner for the participation of his animals in this study.
Ethical review and approval was not required for the study on human participants in accordance with the local legislation and institutional requirements. Both shepherds provided their written informed consent to participate in this study.
Results
Humoral immune response
After vaccinating the cohort VAC, both IgG phase-specific antibodies increased sharply (Figure 2). The IgG PhII response declined continuously after September 2018. The IgG PhI values increased up until the lambing season in 2019 and remained on this high level until the end of the study. In total, median IgG PhI response was always above the median values of IgG PhII after vaccination (p < 0.05).
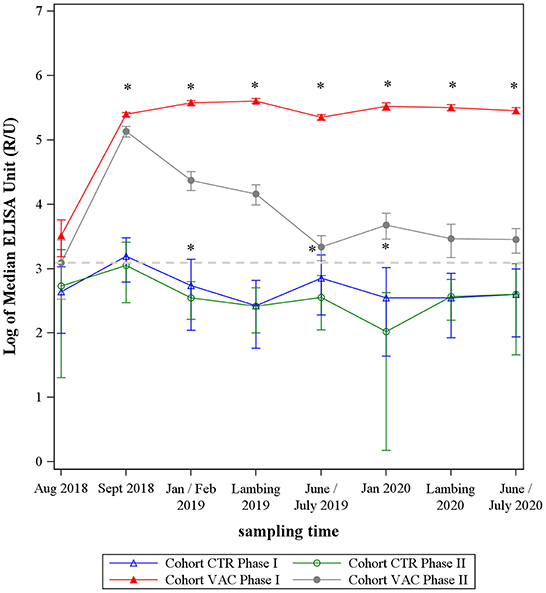
Figure 2. Median levels of Coxiella burnetii IgG Phase I and Phase II from two sheep cohorts. VAC: sheep vaccinated against Coxiella burnetii; CTR: control sheep; dashed line: cut-off value of both phase-specific ELISAs. *Significant difference between IgG Phase I and IgG Phase II median levels within each cohort (p< 0.05).
During the entire study period, the median values of cohort CTR remained at a low level and showed an undulating trend, remaining mainly under the ELISAs' cut-off value (Figure 2). The IgG PhI response was significantly higher than the IgG PhII level at three sampling dates (p < 0.05) (Figure 2).
On comparing both cohorts, the median levels of C. burnetii PhII did not differentiate between the study cohort VAC and CTR at the beginning (August 2018) of the study (p > 0.05), but cohort VAC had significantly higher IgG PhI antibodies than cohort CTR in August 2018 (Figure 2). After vaccination and up until the end of the study, the response of both phase-specific IgG's antibodies was significantly higher in cohort VAC compared to CTR (p < 0.05).
At the beginning of the study, 75.8% (n = 99) of ewes in cohort VAC tested seropositive by at least one of the phase-specific ELISAs (Figure 3). Of these seropositive animals, 40% showed an IgG PhII dominance, whereas 60% had a higher IgG PhI activity. After the first and second vaccinations, the number of sheep with an IgG PhI dominance increased significantly (p < 0.05) and remained at a high level (≥94.3%) until June 2020. The IgG PhII levels in two ewes were always higher than the IgG PhI activities during the entire study period (Figure 3). None of the ewes from cohort VAC tested completely negative in the period from August 2018 to June 2020.
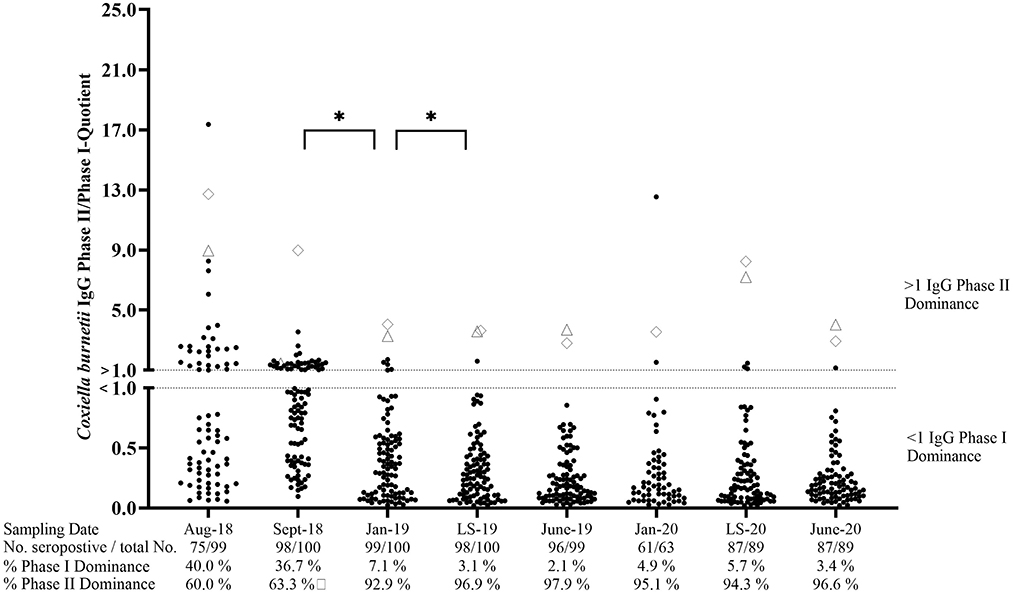
Figure 3. Coxiella burnetii IgG Phase II/Phase I quotients of seropositive sheep after vaccination. ♢ and Δ: Two sheep with an IgG Ph II dominance during the entire study period (one missing piece of data in January 2020 for Δ); LS, Lambing season. *Significant difference in phase-specific antibody dominance between two sampling dates (p< 0.05).
Cohort CTR contained 57% seropositive animals in August 2018 (Figure 4). During the following months, the percentage of seropositive ewes showed an undulating development and ranged from 35.1 to 66.3% of ELISA positive animals. The phase-specific investigation also revealed a dynamic pattern with an increasing number of IgG PhI positive sheep from September 2018 to February 2019 and from July 2019 to January 2020. In contrast, the number of animals with an IgG PhII dominance rose during both lambing seasons (LS), but only the surge from January 2020 up until the lambing season in 2020 was significant (p < 0.05). In general, the phase-specific response varied in individual animals, but 17 ewes tested completely negative during the entire study period. Moreover, two ewes always had an IgG Ph II dominance during the entire study period (Figure 4).
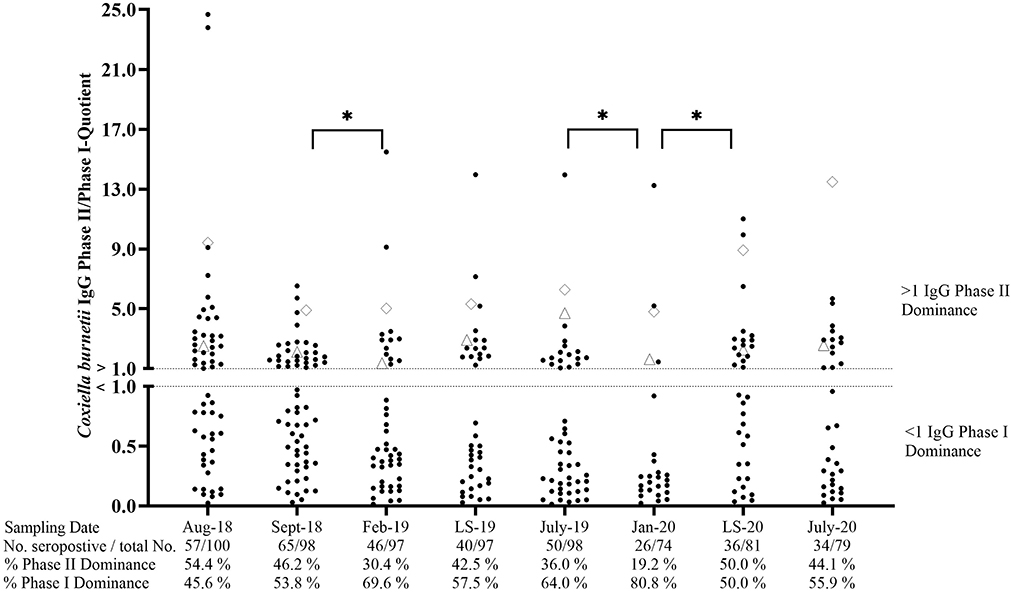
Figure 4. Coxiella burnetii IgG Phase II/Phase I quotients of naturally infected sheep. ♢ and Δ: two sheep with an IgG Phase II dominance during the entire study period. LS, Lambing season. *Significant difference in phase-specific antibody dominance between two sampling dates (p< 0.05).
Vaginal shedding
The number of vaginal shedders and the detected C. burnetii DNA amount on vaginal swabs were low in both cohorts during both lambing seasons. In 2019, significantly fewer sheep shed C. burnetii in cohort VAC than in cohort CTR (Table 3). In contrast, there was no significant difference in the number of shedders among VAC and CTR during the lambing season in 2020. None of the sheep excreted the pathogen during either of the lambing seasons. In cohort CTR, five of 12 vaginal shedders had no antibodies against C. burnetii at sampling date 2019, and the remaining seven showed an IgG response either to Phase I (n = 4), Phase II (n = 2), or tested positive with both ELISAs with a higher IgG PhII level (n = 1). During the lambing season in 2020, one of the vaginal shedders in the CTR group showed an IgG PhII response, whereas the second positive ewe tested negative with both phase-specific ELISAs. The four vaginal shedders (2019 and 2020) in cohort VAC tested positive with both phase-specific ELISAs post parturition, with an IgG PhI dominance during lambing.
Lamb mortality 2019
In 2019, 98 ewes in cohort CTR and 100 ewes in cohort VAC gave birth to 139 and 148 lambs, respectively. None of the study ewes aborted in 2019. More lamb losses occurred in the vaccinated cohort (n = 10) than in the control cohort (n = 2). The lamb mortality rate in cohort VAC included four lambs that died due to dystocia, four lambs that were stillborn, two lambs that died within 24 hours post-partum and had previously obtained an Apgar score of 7. Three lambs in cohort VAC (two with dystocia, one post-partum) were necropsied, and none of the organ pool samples tested positive for C. burnetii, but Chl. abortus (Cq 37) was detected in one lamb (with dystocia). Two lambs in cohort CTR died due to dystocia and were born dead, but further investigations were not performed.
Lamb mortality 2020
Flock CTR suffered from abortion in 2020 and also five ewes from the study cohort aborted. Among these five ewes, five lambs from three ewes were necropsied and four lambs from two ewes tested positive for Chl. abortus (Cq 22, 24, 30, 34). C. burnetii was not detected in the five aborted lambs. Two lambs died due to dystocia and were also necropsied and tested negative for Chl. abortus and C. burnetii. In total, 76 ewes lambed and 107 lambs were born alive, there being no lamb losses within three days post-partum.
Sixteen non-study sheep from flock CTR also aborted and were included in the investigations. Four aborted lambs from four ewes tested Chl. abortus positive, and one sheep aborted due to a T. gondii infection. C. burnetii DNA was not detected from abortion material of these sheep.
Abortion did not occur in cohort VAC during the lambing season in 2020, and this study population had only minor lamb losses (n = 4) from a total of 89 lambing ewes. Dystocia (n = 3) was the primary cause of lamb losses. Of these four lambs, one lamb was necropsied, but tested negative for C. burnetii and Chl. abortus DNA. Overall, 122 lambs were born alive in cohort VAC in 2020.
Details about the lamb mortality parameters are presented in Table 4. Moreover, information about the post-mortem results of both lambing seasons are summarized in Supplementary Table S2.
Conditions of ewes and lambs
The mean body weight of the ewes at parturition did not differ significantly among both cohorts in 2019. In contrast, ewes from cohort CTR were significantly heavier than their counterparts from cohort VAC in 2020 (Table 5). In 2019, the mean body temperature three days after lambing was significantly higher in ewes from cohort CTR than in those from cohort VAC, but there was no significant difference among both cohorts during the subsequent lambing period (Table 5). The number of ewes with vaginal discharge three days post-partum did not significantly differ among the two cohorts during both lambing seasons (Table 5).
The mean birth weight of live born lambs did not differ significantly among cohorts CTR and VAC during both lambing seasons. During both lambing periods, lambs from cohort CTR had a significantly lower body temperature post-partum than those from cohort VAC (Table 5).
Discussion
Immunization
Vaccination of naturally pre-infected sheep with an inactivated C. burnetii Phase I vaccine stimulated the IgG response significantly and IgG PhI levels remained high for two years, whereas IgG PhII response declined after primary vaccination. This is in line with short-term observations from a previous study, and the sharp rise in IgG PhI antibodies after vaccination proves the natural pre-infection of the sheep flock although the proportion of seropositive ewes was very low in April 2018 (33). The long-lasting IgG PhI response raises the question whether booster immunization of vaccinated pre-infected sheep is necessary. Treating mice with purified IgGs from Phase I vaccinated mice was able to inhibit the C. burnetii infection and demonstrated the essential contribution of antibodies to the vaccine-induced protection (36). The clearance and complete elimination of the pathogen in the late stage of infection is conducted by T cell-mediated immunity (35, 36). In the present study, we focused on the humoral immune response due to the lacking availability of assays to measure the cell-mediated immune response with a large sample size on a regular basis in veterinary medicine. Nevertheless, immunization with a C. burnetii Phase I vaccine stimulated simultaneously both the IgG response and the production of cytokine-producing CD4+ T cells in mice (53). The CD4+ T cells play an important role in signaling B cells and subsequently in triggering an antibody response (37). Therefore, we assume that the ongoing antigenic impact stimulated IgG PhI antibodies and also the cell-mediated response. Consequently, pre-infected sheep do not need a booster vaccination of C. burnetii Phase I vaccine after primary vaccination (33). This approach is supported by data recently published by Böttcher and colleagues (34) who controlled Q fever in an infected sheep flock merely due to primary vaccination of the replacement ewes. Future studies are necessary to evaluate the cell-mediated immune response, such as the release of INF-γ after application of a C. burnetii Phase I vaccine in sheep in order to confirm our assumptions.
Serology
Six seronegative non-vaccinated ewes shed the pathogen at parturition, indicating that phase-specific serology is unable to identify vaginal shedders. This is in accordance with results from phase-non-specific ELISAs (46, 54). Moreover, individual ewes from cohorts CTR and VAC showed a dominance of IgG PhII during the entire study period. The lack of seroconversion from high IgG PhII antibodies to an increase in IgG PhI was previously observed in sheep and cattle (33, 55). In cattle, IgG PhII positive animals became seronegative without developing IgG PhI antibodies, but it must be taken into account that the use of different phase-specific ELISA methods hampers a direct comparison (34, 55). In the present study, four sheep (two vaccinated and two non-vaccinated ewes) had high IgG PhII levels with a low IgG PhI response over a two-year period, and these animals did not shed the pathogen at parturition. In humans, high IgG PhII antibody levels also lasted for one year and probably longer after an acute Q fever infection (56, 57). Moreover, higher IgG PhII antibodies were also detected in humans suffering from a persistent C. burnetii infection, and pathogen antigen was detected in most cases in bone marrow samples (58). It is suggested that persisting C. burnetii antigens cause a continuous immune stimulation (59). Recently, low amounts of C. burnetii DNA were detected by qPCR (IS1111) in several tissue samples, such as cardiac valves, uterus, and bone marrow from naturally infected sheep (60). Detection of DNA indicates the presence of C. burnetii in these organs and might result in constant immune stimulation. However, confirmation of C. burnetii organisms in ovine organ samples with advanced diagnostic methods such as fluorescence in situ hybridization (61) is still pending and is necessary to support this hypothesis. It would be desirable to measure components of the cell-mediated immunity such as IL-2, IL-10, and IFN-γ, which were previously used to identify a chronic C. burnetii infection in mice and humans (62, 63), in order to gain deeper insights into the complexity of persistent C. burnetii infection in ruminants. Alongside the constant IgG PhII dominance of the previously described animals, a few ewes showed a similar phase pattern, but for a short period of time, especially in cohort CTR. We cannot rule out that this occurred due to reinfection with C. burnetii or serological cross-reactions with other pathogens such as Chlamydia spp., Bartonella spp., and Legionella spp. (64–66). The extensive management system of the sheep flocks allows potential exposure to different pathogens. Hence, evaluation of the disease status based on a single serum sample may lead to misinterpretation, and a second serum sample is needed to confirm the diagnosis as recommended in human medicine (56).
Interestingly, the IgG PhII response in cohort CTR showed an undulating trend with peaks during both lambing seasons. The rise in Phase II antibodies might be associated with high estradiol levels at the end of pregnancy due to the stimulating effect of this hormone on the production of specific antibodies (67). Moreover, it is suggested that estradiol can stimulate antibody production by B cells, probably by inhibiting T cell suppression of B cells (68). The impact of estradiol but also of progesterone on the immune response against C. burnetii has already been discussed in goats (30, 69). Therefore, the influence of sexual hormones on pathogen-induced IgG response in small ruminants needs further research.
Taken together, phase-specific serology is a helpful diagnostic tool to characterize the humoral response after naturally acquired C. burnetii or vaccination in small ruminants at herd level (31, 33, 34, 70). Nonetheless, it is not able to meet expectations of identifying single shedders and evaluating the disease status of individual sheep.
Shedding
The C. burnetii Phase I vaccine diminished the number of vaginal shedding significantly in the first lambing season, but there was no difference in the second year. Information on the efficiency of the inactivated C. burnetii Phase I vaccine in sheep is scarce (71). Astobiza and colleagues (21, 42, 43) conducted studies in C. burnetii pre-infected sheep flocks including control groups within the flocks. Leaving non-vaccinated animals in a flock can impact the vaccine efficiency, especially if the antigen is highly contagious, such as C. burnetii (42, 72). This might lead to a non-significant reduction in vaginal shedders and the bacterial load among vaccinated and non-vaccinated groups (21, 42). Moreover, self-reduction of C. burnetii shedding in sheep flocks has been reported (15, 43, 44), and infected sheep develop a natural immunity against the pathogen. However, gimmers might cause the C. burnetii infection to persist in a flock (15). In the present study, the female offspring were not included in the investigations, as we wanted to investigate the effect of the vaccine in multiparous sheep. Furthermore, repeated vaginal shedders were not observed in either sheep flocks, thus indicating that sheep do not develop a persistent C. burnetii infection as reported for goats (73).
Lamb mortality
Only in cohort CTR did ewes abort before the lambing season started in 2020. The abortion rate of 6.2% was higher than reported in sheep flocks from southern Germany and exceeded the critical benchmark of 3%, which is recommended for outbreak investigations (19, 49). Stillbirth and dystocia rates in both flocks were below the median stillbirth rate of 7.2% reported by Voigt and colleagues (49). However, they did not differentiate between stillborn and dystocia. The vaccinated study group had higher total lamb losses (number of losses at parturition and within 3 days post-partum) than the non-vaccinated counterpart. This is mainly associated with the two lambs, which died within 24 hours after lambing. Different farm management systems, sheep breeds, and study designs hamper the comparison of results from other countries. Nevertheless, the lamb losses in our study groups were slight compared to findings from the UK (total lamb losses of 7% and 10%) (74) and Norway (stillbirth rate: ~4%, neonatal mortality ~3%) (75).
During the entire study period, C. burnetii was neither diagnosed in aborted fetuses, stillborn lambs or lambs that had died due to dystocia. The main detected pathogen was Chl. abortus, which is the main cause of abortion in German sheep flocks (49). Co-infections of C. burnetii with other abortive pathogens like Chl. abortus and border disease virus were reported, or new infections, e.g., with T. gondii can occur during the subsequent lambing season (21, 23, 42). This may lead to possible overestimation of lamb losses due to C. burnetii infection in sheep. Therefore, it is essential to analyze abortion material for several pathogens. Moreover, the occurrence of placentitis including the presence of C. burnetii organisms in the placenta should be confirmed by microscopical examination in order to confirm a Coxiella-associated abortion.
Conditions of ewes and lambs
The body temperature from ewes in cohort CTR was significantly higher than in cohort VAC during the lambing period in 2019, but the mean values remained within the reference range for adult sheep (39–40.0°C) (76). Although the placental expulsion was not regularly observed because of the extensive management system, the presence of vaginal discharge three days post-partum indicates puerperal disorders such as retention of the fetal membranes (77). The presence of vaginal discharge did not differ significantly among both sheep flocks during the two lambing seasons.
During both lambing periods, lambs from cohort CTR had a significantly lower body temperature than the lambs from the vaccinated flock. A low body temperature at birth is correlated with a low birth weight and this reduces lamb survival (78). However, there was neither a significant difference in birth weight between lambs from both cohorts nor an increased number of postnatal lamb losses in cohort CTR. In general, the mean levels of the lambs' body temperature were within the physiological reference range (39.5–40.5°C) (76).
The authors are aware of the limitations of the investigations. The intra-flock prevalence of C. burnetii antibodies, and the amount of C. burnetii DNA were low in comparison to other studies (21, 42, 43). Under field conditions, it is challenging to apply a cohort study due to the self-limiting course of Q fever in sheep flocks, and the lack of appropriate diagnostic tools to diagnose C. burnetii before shedding. Nevertheless, the low amount of C. burnetii shedding was probably sufficient to induce a strong IgG PhII response in shepherd B, and emphasizes the high zoonotic risk for humans to acquire Q fever from infected sheep flocks. Finally, our study investigated the phase-specific IgG response, vaginal shedding, and lamb mortality on a high number of individual sheep over two lambing seasons. With this approach, we gained important insights into the impact of an inactivated C. burnetii Phase I vaccine, which plays a key role in disease prevention.
Conclusions
Taking our findings together, the inactivated C. burnetii Phase I vaccine reduces the number of vaginal shedders in a naturally infected sheep flock during the following lambing season and is an efficient control measure. Moreover, immunization stimulates the natural humoral immune response against C. burnetii for at least two years in sheep and a booster seems unnecessary. In the future, the cell-mediated immune response has to be evaluated as a primary component of C. burnetii defense. For this purpose, novel assays are urgently needed to measure the IFN-γ release on a large scale. Vaccination of infected sheep against C. burnetii had no impact on puerperal disorders in ewes and lamb mortality parameters. Therefore, sheep farmers do not have a direct economic benefit from vaccinating their animals against C. burnetii. Q fever concerns more public health than the health of sheep. Hence, it is desirable that the public health sector financially supports the vaccination of sheep, in particular of flocks, which are involved in public functions like dyke protection and landscape conservation. This holistic approach is in the spirit of the One Health concept (79). Furthermore, during abortion investigations, it is important to examine aborted fetuses and placentas for several agents, as different pathogens such as Chl. abortus or T. gondii can co-circulate in a flock and possibly distort the influence of C. burnetii on animal health.
Data availability statement
The original contributions presented in the study are included in the article/Supplementary material, further inquiries can be directed to the corresponding author/s.
Ethics statement
Ethical review and approval was not required for the study on human participants in accordance with the local legislation and institutional requirements. The patients/participants provided their written informed consent to participate in this study. The animal study was reviewed and approved by the federal state governments of Schleswig-Holstein (Az. V 242-39872/2018) and Mecklenburg-Western Pomerania (Az. 7221.3-2-017/18). Written informed consent was obtained from the owner for the participation of his animals in this study.
Author contributions
Conceptualization and methodology: BB, MR, and MG. Validation: BB, CS, TH, MR, and MG. Formal analysis, writing—original draft preparation, and visualization: BB and CS. Investigation: BB, CS, TH, SK, and MR. Resources, supervision, project administration, and funding acquisition: MR and MG. Data curation: BB, CS, and TH. Writing—review and editing: TH, SK, MR, and MG. All authors have read and agreed to the published version of the manuscript.
Funding
This work was funded by the Federal Ministry of Education and Research (BMBF) under project numbers 01Kl1726B and 01KI2008B as part of the Research Network Zoonotic Infectious Diseases. This Open Access publication was funded by the Deutsche Forschungsgemeinschaft (DFG, German Research Foundation) – 491094227 Open Access Publication Funding and the University of Veterinary Medicine Hannover, Foundation.
Acknowledgments
Special thanks go to Annika Wolf and Melanie Stöter for their excellent technical support. Furthermore, we wish to thank Frances Sherwood-Brock for her assistance with proofreading the manuscript to ensure correct English.
Conflict of interest
The authors declare that the research was conducted in the absence of any commercial or financial relationships that could be construed as a potential conflict of interest.
Publisher's note
All claims expressed in this article are solely those of the authors and do not necessarily represent those of their affiliated organizations, or those of the publisher, the editors and the reviewers. Any product that may be evaluated in this article, or claim that may be made by its manufacturer, is not guaranteed or endorsed by the publisher.
Supplementary material
The Supplementary Material for this article can be found online at: https://www.frontiersin.org/articles/10.3389/fvets.2022.1064763/full#supplementary-material
References
1. Bauer BU, Runge M, Campe A, Henning K, Mertens-Scholz K, Boden K, et al. Coxiella burnetii: a review focusing on infections in German sheep and goat flocks. Berl Munch Tierarztl Wochenschr. (2020) 133:184–200. doi: 10.2376/0005-9366-19030
2. Georgiev M, Afonso A, Neubauer H, Needham H, Thiéry R, Rodolakis A, et al. Q fever in humans and farm animals in four European countries, 1982 to 2010. Eur Surveill. (2013) 18:20407. doi: 10.2807/ese.18.08.20407-en
3. Anderson A, Bijlmer H, Fournier PE, Graves S, Hartzell J, Kersh GJ, et al. Diagnosis and management of Q fever—United States, 2013: recommendations from CDC and the Q fever working group. MMWR Recomm Rep. (2013) 62:1–30.
4. Morroy G, Keijmel SP, Delsing CE, Bleijenberg G, Langendam M, Timen A, et al. Fatigue following acute Q-fever: a systematic literature review. PLoS ONE. (2016) 11:e0155884. doi: 10.1371/journal.pone.0155884
5. Wegdam-Blans M, Kampschreur L, Delsing C, Bleeker-Rovers C, Sprong T, Van Kasteren M, et al. Chronic Q fever: review of the literature and a proposal of new diagnostic criteria. J Infect. (2012) 64:247–59. doi: 10.1016/j.jinf.2011.12.014
6. Agerholm JS. Coxiella burnetii associated reproductive disorders in domestic animals—a critical review. Acta Vet Scand. (2013) 55:1–11. doi: 10.1186/1751-0147-55-13
7. Garcia-Ispierto I, Tutusaus J, López-Gatius F. Does Coxiella burnetii affect reproduction in cattle? a clinical update. Reprod Domest Anim. (2014) 49:529–35. doi: 10.1111/rda.12333
8. Roest H, van Gelderen B, Dinkla A, Frangoulidis D, van Zijderveld F, Rebel J, et al. Q fever in pregnant goats: pathogenesis and excretion of Coxiella burnetii. PLoS ONE. (2012) 7:e48949. doi: 10.1371/journal.pone.0048949
9. van den Brom R, van Engelen E, Roest HIJ, van der Hoek W, Vellema P. Coxiella burnetii infections in sheep or goats: an opinionated review. Vet Microbiol. (2015) 181:119–29. doi: 10.1016/j.vetmic.2015.07.011
10. Martinov SP, Neikov P, Popov GV. Experimental Q fever in sheep. Eur J Epidemiol. (1989) 5:428–31. doi: 10.1007/bf00140134
11. Bellini C, Magouras I, Chapuis-Taillard C, Clerc O, Masserey E, Peduto G, et al. Q fever outbreak in the terraced vineyards of Lavaux, Switzerland. New Microbe New Infect. (2014) 2014:93–9. doi: 10.1002/nmi2.37
12. Fischer S, Göhring-Zwacka E, Kömpf D, Bauer C, Reick D, Pfaff G, et al. Labordiagnostische Untersuchung eines großen Q-Fieber-Ausbruchs im Landkreis Heidenheim/Baden-Württemberg. Gesundheitswesen. (2016) 78:V78. doi: 10.1055/s-0036-1578893
13. Gilsdorf A, Kroh C, Grimm S, Jensen E. Large Q Fever outbreak due to sheep farming near residential areas, Germany, 2005. Epidemiol Infect. (2008) 136:1084–7. doi: 10.1017/S0950268807009533
14. Porten K, Rissland J, Tigges A, Broll S, Hopp W, Lunemann M, et al. A super-spreading ewe infects hundreds with Q fever at a farmers' market in Germany. BMC Infect Dis. (2006) 6:147. doi: 10.1186/1471-2334-6-147
15. Álvarez-Alonso R, Zendoia II, Barandika JF, Jado I, Hurtado A, López CM, et al. Monitoring Coxiella burnetii infection in naturally infected dairy sheep flocks throughout four lambing seasons and investigation of viable bacteria. Front Vet Sci. (2020) 7:352. doi: 10.3389/fvets.2020.00352
16. Bauer B, Prüfer L, Walter M, Ganter I, Frangoulidis D, Runge M, et al. Comparison of Coxiella burnetii excretion between sheep and goats naturally infected with one cattle-associated genotype. Pathogens. (2020) 9:652. doi: 10.3390/pathogens9080652
17. Hilbert A, Schmoock G, Lenzko H, Moog U, Diller R, Fröhlich A, et al. Prevalence of Coxiella burnetii in clinically healthy German sheep flocks. BMC Res Notes. (2012) 5:152. doi: 10.1186/1756-0500-5-152
18. Joulié A, Laroucau K, Bailly X, Prigent M, Gasqui P, Lepetitcolin E, et al. Circulation of Coxiella burnetii in a naturally infected flock of dairy sheep: shedding dynamics, environmental contamination, and genotype diversity. Appl Environ Microbiol. (2015) 81:7253–60. doi: 10.1128/AEM.02180-15
19. Ganter M, Benesch C, Bürstel D, Ennen S, Kaulfuß KH, Mayer K, et al. Empfehlung für die Haltung von Schafen und Ziegen der Deutschen Gesellschaft für die Krankheiten der kleinen Wiederkäuer, Fachgruppe der DVG. Teil 1 Tierärztliche Praxis Großtiere. (2012) 40:314–25. doi: 10.1055/s-0038-1623133
21. Astobiza I, Barandika JF, Juste RA, Hurtado A, García-Pérez AL. Evaluation of the efficacy of oxytetracycline treatment followed by vaccination against Q fever in a highly infected sheep flock. Vet J. (2013) 196:81–5. doi: 10.1016/j.tvjl.2012.07.028
22. Berri M, Rekiki A, Boumedine KS, Rodolakis A. Simultaneous differential detection of Chlamydophila abortus, Chlamydophila pecorum and Coxiella burnetii from aborted ruminant's clinical samples using multiplex PCR. BMC Microbiol. (2009) 9:130. doi: 10.1186/1471-2180-9-130
23. Eibach R, Bothe F, Runge M, Ganter M. Long-term monitoring of a Coxiella burnetti-infected sheep flock after vaccination and antibiotic treatment under field conditions. Berl Munch Tierarztl Wochenschr. (2013) 126:3–9. doi: 10.2376/0005-9366-126-3
24. Hazlett MJ, McDowall R, DeLay J, Stalker M, McEwen B, van Dreumel T, et al. A prospective study of sheep and goat abortion using real-time polymerase chain reaction and cut point estimation shows Coxiella burnetii and Chlamydophila abortus infection concurrently with other major pathogens. J Vet Diagn Invest. (2013) 25:359–68. doi: 10.1177/1040638713484729
25. Santos S, Azenha D, Oliveira C, Almeida A. Coxiella burnetii and Chlamydia spp. coinfection in small ruminant abortion in Portugal Small Rumin Res. (2022) 207:106616. doi: 10.1016/j.smallrumres.2022.106616
26. Longbottom D, Coulter LJ. Animal chlamydioses and zoonotic implications. J Comp Pathol. (2003) 128:217–44. doi: 10.1053/jcpa.2002.0629
28. Hackstadt T, Peacock MG, Hitchcock PJ, Cole RL. Lipopolysaccharide variation in Coxiella burnetti: intrastrain heterogeneity in structure and antigenicity. Infect Immun. (1985) 48:359–65. doi: 10.1128/IAI.48.2.359-365
29. Stoker M, Fiset P. Phase variation of the Nine Mile and other strains of Rickettsia burneti. Can J Microbiol. (1956) 2:310–21. doi: 10.1139/m56-036
30. Roest H, Post J, van Gelderen B, van Zijderveld FG, Rebel JM, Q. fever in pregnant goats: humoral and cellular immune responses. Vet Res. (2013) 44:67. doi: 10.1186/1297-9716-44-67
31. Sting R, Molz K, Philipp W, Bothe F, Runge M, Ganter M. Quantitative real-time PCR and phase specific serology are mutually supportive in Q fever diagnostics in goats. Vet Microbiol. (2013) 167:600–8. doi: 10.1016/j.vetmic.2013.09.015
32. Hatchette T, Campbell N, Hudson R, Raoult D, Marrie TJ. Natural history of Q fever in goats. Vector Borne Zoonotic Dis. (2003) 3:11–5. doi: 10.1089/153036603765627415
33. Bauer BU, Knittler MR, Prüfer TL, Wolf A, Matthiesen S, Runge M, et al. Humoral immune response to Q fever vaccination of three sheep flocks naturally pre-infected with Coxiella burnetii. Vaccine. (2021) 39:1499–507. doi: 10.1016/j.vaccine.2021.01.062
34. Böttcher J, Bauer BU, Ambros C, Alex M, Domes U, Roth S, et al. Long-term control of Coxiellosis in sheep by annual primary vaccination of gimmers. Vaccine. (2022) 40:5197–206. doi: 10.1016/j.vaccine.2022.07.029
35. Zhang G, Russell-Lodrigue KE, Andoh M, Zhang Y, Hendrix LR, Samuel JE. Mechanisms of vaccine-induced protective immunity against Coxiella burnetii infection in BALB/c mice. J Immun. (2007) 179:8372–80. doi: 10.4049/jimmunol.179.12.8372
36. Zhang G, Peng Y, Schoenlaub L, Elliott A, Mitchell W, Zhang Y. Formalin-inactivated Coxiella burnetii phase I vaccine-induced protection depends on B cells to produce protective IgM and IgG. Infect Immun. (2013) 81:2112–22. doi: 10.1128/iai.00297-13
37. Read AJ, Erickson S, Harmsen AG. Role of CD4+ and CD8+ T cells in clearance of primary pulmonary infection with Coxiella burnetii. Infect Immun. (2010) 78:3019–26. doi: 10.1128/IAI.00101-10
38. Schoenlaub L, Elliott A, Freches D, Mitchell WJ, Zhang G, Roy CR. Role of B cells in host defense against primary Coxiella burnetii infection. Infect Immun. (2015) 83:4826–36. doi: 10.1128/IAI.01073-15
39. Andoh M, Zhang G, Russell-Lodrigue KE, Shive HR, Weeks BR, Samuel JE, et al. cells are essential for bacterial clearance, and gamma interferon, tumor necrosis factor alpha, and B cells are crucial for disease development in Coxiella burnetii infection in mice. Infect Immun. (2007) 75:3245–55. doi: 10.1128/iai.01767-06
40. Zhang G, Zhang Y, Samuel JE. Components of Protective immunity. In:Toman R, Heinzen RA, Samuel JE, Mege J-L, , editors. Coxiella burnetii: Recent Advances and New Perspectives in Research of the Q Fever Bacterium. Dordrecht: Springer Netherlands (2012). p. 91–104.
41. Achard D, Rodolakis A. Q fever vaccination in ruminants: a critical review. In:Caetano Simoes JC, Ferreira AS, Jorge de Silva G, , editors. The Principles and Practice of Q fever. New York: Nova Science Publishers Inc (2017). p. 367–89.
42. Astobiza I, Barandika JF, Ruiz-Fons F, Hurtado A, Povedano I, Juste RA, et al. Four-year evaluation of the effect of vaccination against Coxiella burnetii on reducing animal infection and environmental contamination in a naturally infected dairy sheep flock. Appl Environ Microbiol. (2011) 77:7405–7. doi: 10.1128/AEM.07181-11
43. Astobiza I, Barandika JF, Ruiz-Fons F, Hurtado A, Povedano I, Juste RA, et al. Coxiella burnetii shedding and environmental contamination at lambing in two highly naturally-infected dairy sheep flocks after vaccination. Res Vet Sci. (2011) 91:e58–63. doi: 10.1016/j.rvsc.2010.11.014
44. Berri M, Souriau A, Crosby M, Rodolakis A. Shedding of Coxiella burnetii in ewes in two pregnancies following an episode of Coxiella abortion in a sheep flock. Vet Micorbiol. (2002) 85:55–60. doi: 10.1016/s0378-113500480-1
45. Oliveira RD, Mousel MR, Pabilonia KL, Highland MA, Taylor JB, Knowles DP, et al. Domestic sheep show average Coxiella burnetii seropositivity generations after a sheep-associated human Q fever outbreak and lack detectable shedding by placental, vaginal, and fecal routes. PLoS ONE. (2017) 12:e0188054. doi: 10.1371/journal.pone.0188054
46. Wolf A, Prüfer TL, Schoneberg C, Campe A, Runge M, Ganter M, et al. Prevalence of Coxiella burnetii in German sheep flocks and evaluation of a novel approach to detect an infection via preputial swabs at herd-level. Epidemiol Infect. (2020) 148:1–7. doi: 10.1017/S0950268820000679
47. Dangel L, Koempf D, Fischer SF. Comparison of different commercially available enzyme-linked immunosorbent assays with immunofluorescence test for detection of phase II IgG and IgM antibodies to Coxiella burnetii. J Clin Microbiol. (2020) 58:e00951. doi: 10.1128/jcm.00951-19
48. Watterberg KL, Aucott S, Benitz WE, Cummings JJ, Eichenwald EC, Goldsmith J, et al. The apgar score. Pediatrics. (2015) 136:819–22. doi: 10.1542/peds.2015-2651
49. Voigt K, Frohnmayer S, Strobel H, Sauter-Louis C, Zerbe H. Time pattern and causes of lamb mortality on commercial sheep farms in Southern Germany operating conservation grazing and non-seasonal production systems—a field study. Berl Munch Tierarztl Wochenschr. (2018) 132:156–65. doi: 10.2376/0005-9366-18006
50. FLI. Amtliche Methodensammlung. (2021). Available online at: https://www.fli.de/de/publikationen/amtliche-methodensammlung/aktualisierungen-methodensammlung/ (accessed September 19, 2022).
51. OIE. Enzootic Abortion of Ewes (Ovine Chlamydiosis) (Infection with Chlamydia Abortus). (2018). Available online at: https://www.woah.org/fileadmin/Home/eng/Health_standards/tahm/3.08.05_ENZ_ABOR.pdf (accessed September 19, 2022).
52. Pantchev A, Sting R, Bauerfeind R, Tyczka J, Sachse K. New real-time PCR tests for species-specific detection of Chlamydophila psittaci and Chlamydophila abortus from tissue samples. Vet J. (2009) 181:145–50. doi: 10.1016/j.tvjl.2008.02.025
53. Chen C, van Schaik EJ, Gregory AE, Vigil A, Felgner PL, Hendrix LR, et al. Chemokine receptor 7 is essential for Coxiella burnetii whole-cell vaccine-induced cellular immunity but dispensable for vaccine-mediated protective immunity. J Infect Dis. (2019) 220:624–34. doi: 10.1093/infdis/jiz146
54. Joulié A, Rousset E, Gasqui P, Lepetitcolin E, Leblond A, Sidi-Boumedine K, et al. Coxiella burnetii circulation in a naturally infected flock of sheep: individual follow-up of antibodies in serum and milk. Appl Environ Microbiol. (2017) 83:e00222–17. doi: 10.1128/AEM.00222-17
55. Böttcher J, Vossen A, Janowetz B, Alex M, Gangl A, Randt A, et al. Insights into the dynamics of endemic Coxiella burnetii infection in cattle by application of phase-specific ELISAs in an infected dairy herd. Vet Microbiol. (2011) 151:291–300. doi: 10.1016/j.vetmic.2011.03.007
56. Wegdam-Blans MCA, Wielders CCH, Meekelenkamp J, Korbeeck JM, Herremans T, Tjhie HT, et al. Evaluation of commonly used serological tests for detection of Coxiella burnetii antibodies in well-defined acute and follow-up sera. Clin Vaccine Immunol. (2012) 19:1110–5. doi: 10.1128/CVI.05581-11
57. Wielders CCH, Kampschreur LM, Schneeberger PM, Jager MM, Hoepelman AIM, Leenders ACAP, et al. Early diagnosis and treatment of patients with symptomatic acute Q Fever do not prohibit IgG antibody responses to Coxiella burnetii. Clin Vaccine Immunol. (2012) 19:1661–6. doi: 10.1128/CVI.00322-12
58. Marmion B, Storm P, Ayres JG, Semendric L, Mathews L, Winslow W, et al. Long-term persistence of Coxiella burnetii after acute primary Q fever. QJM. (2005) 98:7–20. doi: 10.1093/qjmed/hci009
59. Marmion B, Sukocheva O, Storm P, Lockhart M, Turra M, Kok T, et al. Q fever: persistence of antigenic non-viable cell residues of Coxiella burnetii in the host—implications for post Q fever infection fatigue syndrome and other chronic sequelae. QJM. (2009) 102:673–84. doi: 10.1093/qjmed/hcp07
60. Jourdain E, Schelcher F, Lucas M-N, Poivre M, Lafon J, Barry S, et al. Post-mortem detection of Coxiella burnetii in eight ewes from a flock recently confronted with Q fever clustered human cases sampled 89–229 days postlambing. International intracellular bacteria meeting 2022, Lausanne, Switzerland (2022). p. 150
61. Buijs SB, Weehuizen JM, Jensen TK, Boye M, Hermans MHA, Nooijen PTGA, et al. Fluorescence in situ hybridization for detecting Coxiella burnetii in tissue samples from chronic Q fever patients. Clin Microbiol Infect. (2022). doi: 10.1016/j.cmi.2022.06.015
62. Meghari S, Bechah Y, Capo C, Lepidi H, Raoult D, Murray PJ, et al. Persistent Coxiella burnetii infection in mice overexpressing IL-10: an efficient model for chronic Q fever pathogenesis. PLoS Pathog. (2008) 4:e23. doi: 10.1371/journal.ppat.0040023
63. Schoffelen T, Sprong T, Bleeker-Rovers CP, Wegdam-Blans MCA, Ammerdorffer A, Pronk MJH, et al. A combination of interferon-gamma and interleukin-2 production by Coxiella burnetii-stimulated circulating cells discriminates between chronic Q fever and past Q fever. Clin Microbiol Infect. (2014) 20:642–50. doi: 10.1111/1469-0691.12423
64. La Scola B, Raoult D. Serological cross-reactions between Bartonella quintana, Bartonella henselae, and Coxiella burnetii. J Clin Microbiol. (1996) 34:2270–4. doi: 10.1128/JCM.34.9.2270-2274
65. Lukáčová M, Melničáková J, Kazár J. Cross-reactivity between Coxiella burnetii and chlamydiae. Folia Microbiol (Praha). (1999) 44:579–84. doi: 10.1007/BF02816263
66. Musso D, Raoult D. Serological cross-reactions between Coxiella burnetii and Legionella micdadei. Clin Diagn Lab Immunol. (1997) 4:208–12. doi: 10.1128/CDLI.4.2.208-212.1997
67. Ding J, Zhu BT. Unique effect of the pregnancy hormone estriol on antigen-induced production of specific antibodies in female BALB/c mice. Steroids. (2008) 73:289–98. doi: 10.1016/j.steroids.2007.10.012
68. Straub RH. The complex role of estrogens in inflammation. Endocr Rev. (2007) 28:521–74. doi: 10.1210/er.2007-0001
69. Bauer BU, Knittler MR, Herms TL, Frangoulidis D, Matthiesen S, Tappe D, et al. Multispecies Q fever outbreak in a mixed dairy goat and cattle farm based on a new bovine-associated genotype of Coxiella burnetii. Vet Sci. (2021) 8:252. doi: 10.3390/vetsci8110252
70. Bauer BU, Schoneberg C, Herms TL, Runge M, Ganter M. Surveillance of Coxiella burnetii shedding in three naturally infected dairy goat herds after vaccination, focusing on bulk tank milk and dust swabs. Vet Sci. (2022) 9:102. doi: 10.3390/vetsci9030102
71. O'Neill T, Sargeant J, Poljak Z, A. systematic review and meta-analysis of phase I inactivated vaccines to reduce shedding of Coxiella burnetii from sheep and goats from routes of public health importance. Zoonoses Public Health. (2014) 61:519–33. doi: 10.1111/zph.12086
72. Guatteo R, Seegers H, Joly A, Beaudeau F. Prevention of Coxiella burnetii shedding in infected dairy herds using a phase I C. burnetii inactivated vaccine. Vaccine. (2008) 26:4320–8. doi: 10.1016/j.vaccine.2008.06.023
73. Berri M, Rousset E, Champion J, Russo P, Rodolakis A. Goats may experience reproductive failures and shed Coxiella burnetii at two successive parturitions after a Q fever infection. Res Vet Sci. (2007) 83:47–52. doi: 10.1016/j.rvsc.2006.11.001
74. Binns SH, Cox IJ, Rizvi S, Green LE. Risk factors for lamb mortality on UK sheep farms. Prev Vet Med. (2002) 52:287–303. doi: 10.1016/S0167-587700255-0
75. Holmøy IH, Waage S. Time trends and epidemiological patterns of perinatal lamb mortality in Norway. Acta Vet Scand. (2015) 57:65. doi: 10.1186/s13028-015-0155-6
76. Nagy DW, Pugh DG. Handling and examining sheep and goats. In:Pugh DG, Baird AN, , editors. Sheep and Goat Medicine. Maryland Heights: Elsevier Saunders (2012). p. 1–17.
77. Fthenakis GC, Leontides LS, Amiridis GS, Saratsis P. Incidence risk and clinical features of retention of foetal membranes in ewes in 28 flocks in southern Greece. Prev Vet Med. (2000) 43:85–90. doi: 10.1016/s0167-587700088-4
78. Dwyer CM, Morgan CA. Maintenance of body temperature in the neonatal lamb: Effects of breed, birth weight, and litter size. J Anim Sci. (2006) 84:1093–101. doi: 10.2527/2006.8451093x
Keywords: abortion, Coxiella burnetii, IgG, lamb losses, Q fever, sheep, vaccination, zoonosis
Citation: Bauer BU, Schoneberg C, Herms TL, Kleinschmidt S, Runge M and Ganter M (2022) Impact of Coxiella burnetii vaccination on humoral immune response, vaginal shedding, and lamb mortality in naturally pre-infected sheep. Front. Vet. Sci. 9:1064763. doi: 10.3389/fvets.2022.1064763
Received: 08 October 2022; Accepted: 18 November 2022;
Published: 19 December 2022.
Edited by:
Jiabo Ding, Institute of Animal Sciences (CAAS), ChinaReviewed by:
Helen Roberts, Department for Environment, Food and Rural Affairs, United KingdomMadhur Sachan, Brigham and Women's Hospital and Harvard Medical School, United States
Copyright © 2022 Bauer, Schoneberg, Herms, Kleinschmidt, Runge and Ganter. This is an open-access article distributed under the terms of the Creative Commons Attribution License (CC BY). The use, distribution or reproduction in other forums is permitted, provided the original author(s) and the copyright owner(s) are credited and that the original publication in this journal is cited, in accordance with accepted academic practice. No use, distribution or reproduction is permitted which does not comply with these terms.
*Correspondence: Benjamin Ulrich Bauer, YmVuamFtaW4uYmF1ZXImI3gwMDA0MDt0aWhvLWhhbm5vdmVyLmRl