- 1College of Veterinary Medicine and Zoonoses Research Institute, Kyungpook National University, Daegu, South Korea
- 2Bacterial Disease Division, Animal and Plant Quarantine Agency, Gimcheon, South Korea
The third- or fourth-generation cephalosporins (3GC or 4 GC) are classified as “critically important antimicrobials for human medicine” by WHO, but resistance to these drugs is increasing rapidly in avian pathogenic E. coli (APEC). This study investigated the distribution and genetic characteristics of 3GC- or 4 GC-resistant APEC isolates from five major integrated broiler operations in Korea. The prevalence of 3GC- or 4GC-resistant APEC isolates in 1-week-old broilers was the highest in farms of operation C (53.3%); however, the highest prevalence of these isolates in 4-week-old broilers was the highest on the farms of operation A (60.0%), followed by operations E (50.0%) and C (35.7%). All 49 3GC- or 4GC-resistant APEC isolates had at least one β-lactamase-encoding gene. The most common β-lactamase-encoding genes was extended-spectrum β-lactamase gene, blaCTX−M−15, detected in 24 isolates (49.0%), followed by blaTEM−1 (32.7%). Sixteen isolates (32.7%) harbored class 1 integrons, and four isolates (8.2%) showed different gene cassette-arrangements. However, only 1 of 26 isolates harboring class 2 integrons carried a gene cassette. Furthermore, both CRISPR 1 and 2 arrays were detected in most isolates (36 isolates; 73.5%), followed by CRISPR 2 (18.4%) and CRISPR 1 (4.1%). Interestingly, CRISPR 2 was significantly more prevalent in multidrug resistant (MDR)-APEC isolates than in non-MDR APEC isolates, whereas CRISPR 3 and 4 were significantly more prevalent in non-MDR APEC isolates (each 11.1%; p < 0.05). None of the protospacers of CRISPR arrays were directly associated with antimicrobial resistance. Our findings indicate that the distribution and characteristics of 3GC or 4GC-resistant APEC isolates differed among the integrated broiler operations; moreover, improved management protocols are needed to control the horizontal transmission of 3GC or 4GC-resistant APEC isolates.
Introduction
Escherichia coli (E. coli) is one of the most common facultative gram-negative bacteria found in the gastrointestinal tract of humans and animals (1), but certain strains of E. coli are responsible for a wide range of infections (2). In particular, avian colibacillosis is an important infectious disease caused by avian pathogenic E. coli (APEC) (3). APEC is defined as an extra-intestinal pathogenic E. coli (ExPEC) that cause diverse local and systemic infections in poultry, including chicken, turkeys, ducks, and many other avian species (4). In many countries, it results in large economic losses, which are attributable to early mortality and reduced productivity caused by a complex of severe respiratory and systemic diseases in chickens (5). Moreover, previous studies indicate that APEC can be a source of genes for other ExPEC strains that impact human medicine, such as uropathogenic E. coli and newborn meningitis-causing E. coli (6). There are certain APEC subgroups that has to be considered as potential zoonotic agents, implying the importance of APEC as a source of future zoonosis (7).
In modern broiler production, diverse antimicrobials, including β-lactams, aminoglycosides, and fluoroquinolones, are the predominant alternatives for treating colibacillosis (3). However, the use of such antimicrobials has contributed to the emergence of antimicrobial resistance globally (8). In particular, the rates of resistance to third- or fourth-generation cephalosporins (3GCs or 4GCs, respectively) which are classified as “critically important antimicrobials for human medicine” by WHO (9), in E. coli are increasing rapidly (10, 11) because of the increased prevalence of plasmid-encoded enzymes, such as extended-spectrum β-lactamases (ESBLs) and plasmid-mediated AmpC (pAmpC) β-lactamases (12). E. coli isolates that produce ESBLs and/or pAmpC β-lactamases are reported to be resistant to extended-spectrum cephalosporins such as 3GCs and 4GCs, crucial antimicrobials for human and animal health (13).
The broiler chicken industry operates through several large integrated operations. In Korea, 94.8% of the broilers are produced by integrated operations, and the top five integrated operations account for 51.4% of the market share (14). Thus, intensively reared broilers are exposed to routine prophylactic and therapeutic antimicrobial treatments; moreover, previous studies have reported that antimicrobial resistance patterns in APEC vary according to the integrated broiler operations (15, 16). Therefore, this study investigated the distribution, characteristics, and results of the CRISPR array typing, a novel typing method, of 3GC- or 4GC-resistant APEC isolates from five major integrated broiler operations in Korea.
Materials and methods
Sampling and bacterial identification
Chickens that died at 1 or 4 weeks of age at 74 broiler farms of five integrated operations were transferred to the laboratory for the isolation of APEC. Isolation and identification of E. coli were performed following the standard microbiological protocols published by the Ministry of Food and Drug Safety (17). Briefly, liver and spleen swab samples were collected in 10 ml of tryptic soy broth (TSB; BD Biosciences, San Jose, CA, USA), and incubated at 37°C for 18–24 h. The enriched broth was streaked onto MacConkey agar (BD Biosciences) and incubated at 37°C for 18–24 h. Suspected colonies were confirmed using polymerase chain reaction (PCR) with specific primers as described previously (18). If APEC isolates from the same farm displayed the same antimicrobial susceptibility patterns, one isolate was selected randomly.
Antimicrobial susceptibility testing
Based on the Clinical and Laboratory Standards Institute (CLSI) guidelines (CLSI, 2021) (19), all isolates were investigated for antimicrobial resistance via the disk diffusion test with the following discs (BD Biosciences): ampicillin (AM, 10 μg), amoxicillin-clavulanate (20 μg), chloramphenicol (30 μg), ceftazidime (CAZ, 30 μg), cephalothin (CF, 30 μg), ciprofloxacin (5 μg), cefotaxime (CTX, 30 μg) cefuroxime (CXM, 30 μg), cefazoline (CZ, 30 μg), cefepime (FEP, 30 μg), cefoxitin (FOX, 30 μg), gentamicin (10 μg), imipenem (10 μg), nalidixic acid (30 μg), trimethoprim/sulfamethoxazole (1.25 μg), and tetracycline (30 μg). The minimum inhibitory concentrations (MICs) of CAZ, CF, CTX, CXM, CZ, ceftiofur (EFT), FEP and FOX at concentrations of 0.5–512 μg/mL were determined via standard agar dilution methods using Mueller-Hinton agar (BD Biosciences) according to the CLSI recommendations (2021) (19). Multidrug-resistance (MDR) was defined as acquired resistance to at least one agent from three or more antimicrobial classes (20).
Detection of β-lactamase-encoding genes
PCR amplification was performed to detect β-lactamase-encoding genes in 49 APEC isolates that exhibited resistance to 3GCs or 4 GCs. Primers for blaCTX−M, blaTEM, blaSHV, blaOXA, and pAmpC genes were used as described previously (21–23). The PCR products were purified using a QIAquick PCR purification kit (Qiagen, Hilden, Germany) and sequenced using an automatic sequencer (Cosmogenetech, Seoul, Korea). The sequences were then compared with those in the GenBank nucleotide database using the Basic Local Alignment Search Tool (BLAST) program available at the National Center for Biotechnology Information website (www.ncbi.nlm.nih.gov/BLAST).
Detection of integrons and gene cassettes
The presence of the integrase genes intl1 and intl2 in 49 3GC- or 4GC-resistant APEC isolates was also detected using PCR as described previously (24, 25). APEC isolates harboring the integrase genes were tested for gene cassettes (25). The PCR products of the gene cassettes were sequenced as described previously, and the obtained sequences were compared with those in the GenBank using the BLAST program available at the National Center for Biotechnology Information website (www.ncbi.nlm.nih.gov).
CRISPR locus sequence typing and spacer analysis
Four CRISPR loci in 49 3GC- or 4GC-resistant APEC isolates were screened using PCR as described previously (26). The PCR products were purified and sequenced according to the abovementioned procedure. The sequences were analyzed using CRISPRFinder (https://crispr.i2bc.paris-saclay.fr/Server/), as described by Grissa et al. (27), and only spacers were obtained for this study. The names and full sequences of all the spacers from this study are listed in Supplementary Table S1. The CRISPRTarget program (http://crispr.otago.ac.nz/CRISPRTarget/crispr_analysis.html) with a cut off value of 29 and nucleotide BLAST (https://blast.ncbi.nlm.nih.gov/Blast.cgi) were used to detect protospacers derived from phages or plasmids (28).
Statistical analysis
The Statistical Package for Social Science version 25 (IBM SPSS Statistics for Windows, Armonk, NY, USA) was used for statistical analysis, which included the independent samples t-test, Fisher's exact test, and Pearson's chi-square test with Bonferroni correction as described previously (10, 29). Differences were considered significant at p < 0.05.
Results
Prevalence of 3GC- or 4GC-resistant APEC isolates
The number of chicken farms confirmed with colibacillosis and the distribution of farms with 3GC- or 4GC-resistant APEC isolates among five integrated operations are presented in Table 1. The highest prevalence of farms confirmed with colibacillosis in 1-week-old broilers 100% and the lowest was 85.7%, without significant differences among operations. However, the highest prevalence in 4-week-old broilers was detected at operation C (93.3%), %), followed by operations D (85.7%), E (80.0%), B (75.0%), and A (33.3%), with significant differences among the operations (p < 0.05). Regarding the prevalence of 3GC- or 4GC-resistant APEC isolates, 1-week-old broilers showed the highest resistance on the farms of operation C (53.3%), but the prevalence in 4-week-old broilers was the highest on the farms of operation A (60.0%), followed by operations E (50.0%) and C (35.7%).
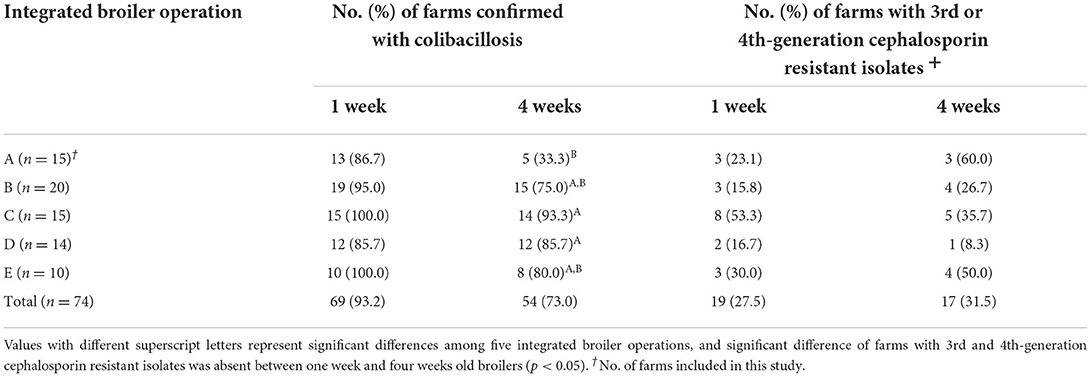
Table 1. Distribution of third- or fourth- generation cephalosporin-resistant avian pathogenic E. coli isolates from five integrated broiler operations.
Characteristics of 3GC- or 4GC-resistant APEC isolates
The genotypic and phenotypic characteristics of the 49 3GC- or 4GC-resistant APEC isolates are presented in Table 2. All 49 APEC isolates carried at least one β-lactamase-encoding gene. The most common β-lactamase-encoding gene was the ESBL gene, blaCTX−M−15, which was detected in 24 isolates (49.0%), followed by blaTEM−1 (32.7%), blaCTX−M−1 (14.3%), blaCTX−M−14 (14.3%), blaSHV−12 (6.1%), blaCTX−M−27 (4.1%), blaOXA−1 (4.1%), and blaTEM−135 (2.0%). Additionally, the pAmpC β-lactamase gene blaCMY−2, was detected in two isolates (4.1%).
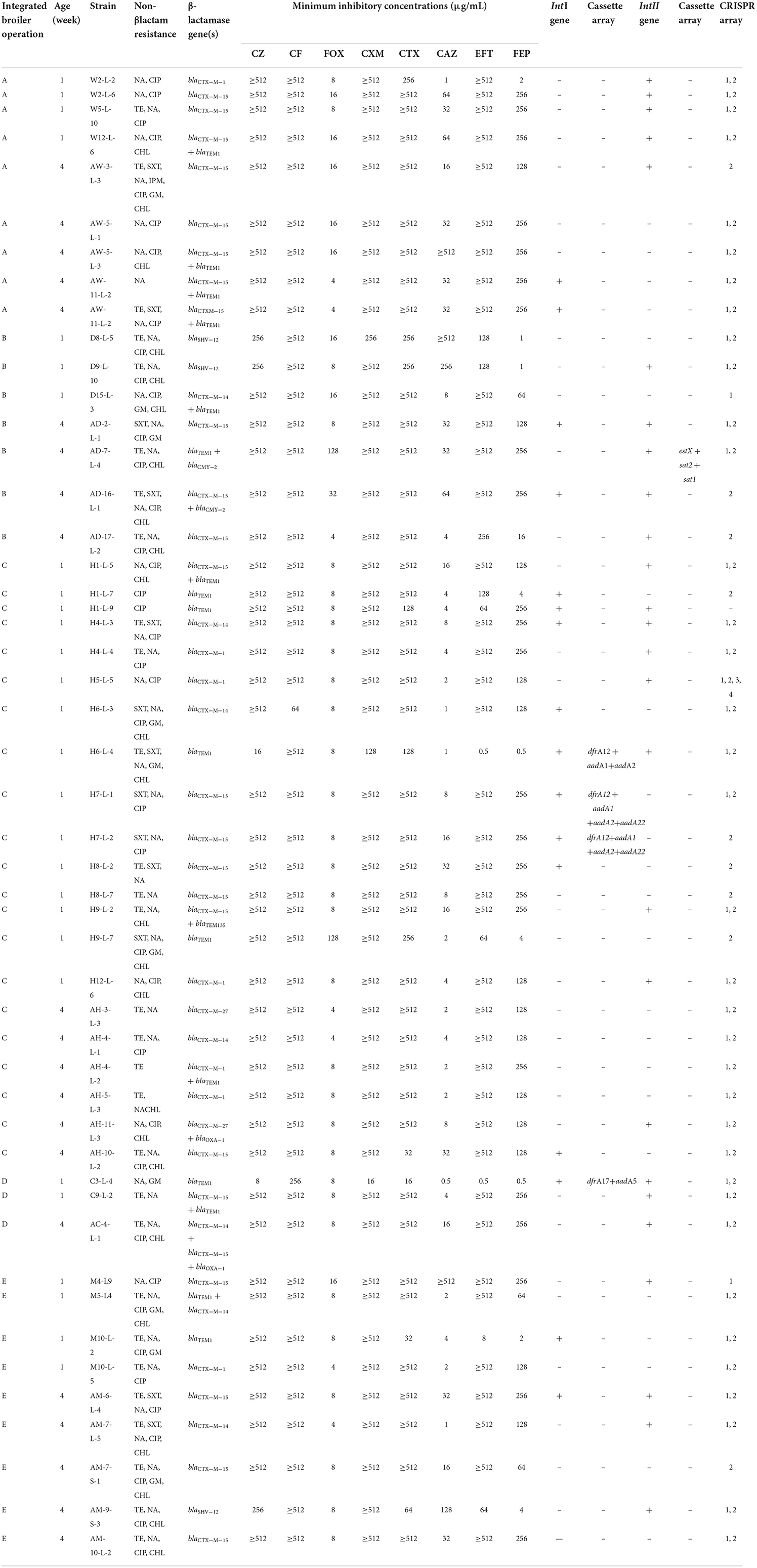
Table 2. Characteristics of the 49 third- or fourth- generation cephalosporin-resistant avian pathogenic E. coli from five integrated broiler operations.
Among the 49 3GC- or 4GC-resistant APEC isolates, 16 (32.7%) harbored class 1 integrons, and four (8.2%) contained different gene cassette arrangements, including aadA5+dfrA17 (n = 1), aadA1+aadA2+dfrA12 (n = 1), and aadA1+aadA2+aadA22+ dfrA12 (n = 2). However, 26 isolates (53.1%) harbored class 2 integrons, and only 1 isolate (2.0%) showed gene cassette arrangement estX+sat2+sat1.
Of the 49 isolates screened for four CRISPR loci, only one lacked all four loci. Moreover, both CRISPR 1 and 2 arrays were detected in most isolates (36 isolates; 73.5%), followed by CRISPR 2 (18.4%) and CRISPR 1 (4.1%). Interestingly, one isolate possessed four CRISPR loci simultaneously.
Distribution of MDR and non-MDR in each CRISPR array
The distribution of MDR and non-MDR isolates with respect to CRISPR locus among the 49 3GC- or 4GC-resistant APEC isolates is presented in Figure 1. Although the distribution of CRISPR 1 showed no significant differences between MDR (80.0%) and non-MDR (77.8%) APEC isolates, CRISPR 2 was significantly more prevalent in MDR APEC isolates (97.5%) than in non-MDR APEC isolates (77.8%, p < 0.05). Moreover, CRISPR 3 and 4 were absent in MDR APEC isolates and were significantly more prevalent in non-MDR APEC isolates (each 11.1%, p < 0.05).
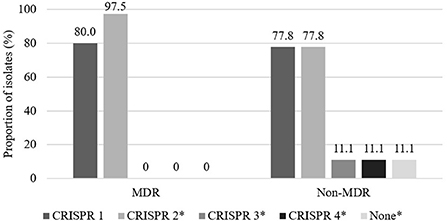
Figure 1. Distribution of CRISPR between multidrug resistant (MDR) and non-multidrug resistant (non-MDR) isolates in 49 third- or fourth-generation cephalosporin-resistant avian pathogenic E. coli. Multidrug resistance was defined as resistance to three or more antimicrobial classes. The asterisk indicates that prevalence between MDR and non-MDR isolates in each CRISPR array is significantly different (p < 0.05).
Protospacer matching with plasmid and phage sequences
The protospacers matching the plasmids and phages sequences, are presented in Table 3. The most prevalent protospacer was associated with gene regulation, comprising 35.9% protospacers of CRISPR 1; followed by 13.4% of hypothetical proteins from the protospacers of CRISPR 1; 10.3 and 6.5% of phage proteins from the protospacers of CRISPR 1 and 2, respectively; and 6.5% of lysozyme inhibitor proteins from the protospacers of CRISPR 2. In CRISPR 3, two protospacers were associated with a protein encoding the terminase large subunit. None of the protospacers of the CRISPR arrays weas directly associated with antimicrobial resistance.
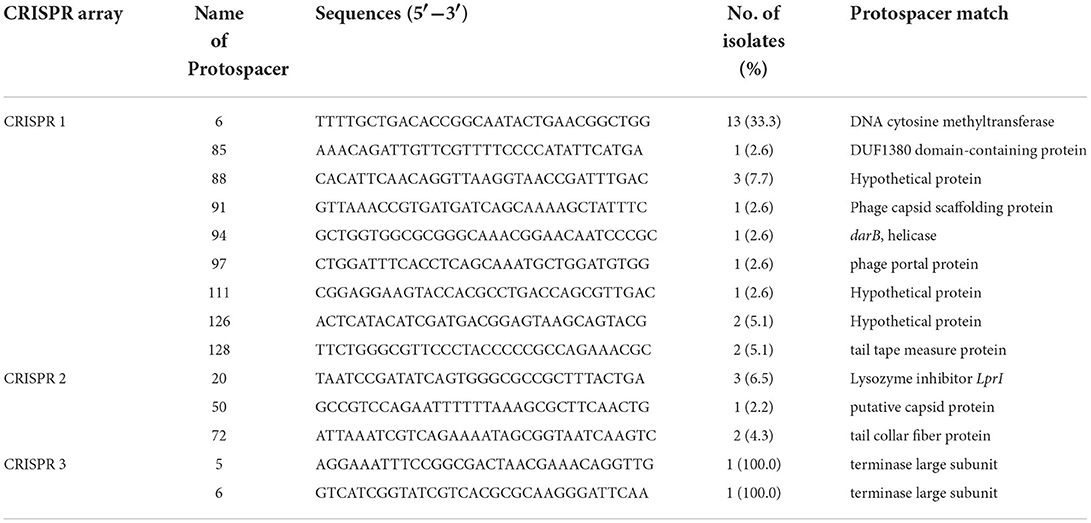
Table 3. Protospacer matching plasmids and phages, and spacer sequences in 49 third- or fourth- generation cephalosporin-resistant avian pathogenic E. coli isolated from five integrated broiler operations.
Discussion
In general, the broiler industry is a vertically integrated production, processing, and distribution system in Korea. This allows individual producers to combine different approaches, such as biosecurity and sanitation practices, housing technologies, and feeding schedules, to enhance production rates and food safety (30, 31). Despite the mass broiler operations in Korea, APEC is a major cause of large economic losses, and intensive antimicrobial treatment is applied by operation systems (3). Moreover, previous studies have reported the relationship between vertically integrated production systems and increased antimicrobial use, resulting in antimicrobial resistance (32, 33). Thus, APEC isolates from chicken in Korea grown by vertically integrated production have shown increased level of resistance during 1985–2005 (34).
The systemic form of colibacillosis usually results in syndromes including respiratory signs or lameness and mortality (35). However, the causative bacterium (E. coli) is present in the farm environment, and it is a normal component of the poultry intestinal microbiota (36). Although previous studies have attempted to identify virulence markers to differentiate the pathogenic strains, infection by highly virulent APEC is also considered a secondary disease caused by opportunistic bacteria (37, 38). Therefore, colibacillosis frequently occurs in young chickens on most farms because of impaired host defenses (39). Moreover, although Kemmett et al. (40) have reported that the prevalence of virulence factors in APEC populations declines with age, in this study, the significant differences in the rates of colibacillosis in 4-week-old chickens among the integrated operations were potentially induced by host factors such as immunosuppression or prior infection, as well as management techniques including the farm environment.
In 2019, 3GCs and 4 GCs were declared as critically important classes of antimicrobials by WHO because of their importance to human health (9). Moreover, 3GC-resistant isolates are often multi-drug resistant and increase its potential threat to human and animal medicine (11, 31, 41). In this study, the prevalence of farms with 3GC- or 4GC- resistant APEC isolates varies from 15.8 to 53.3% in 1-week-old broilers, and 8.3–60.0% in 4-week-old broilers. Although 3GCs and 4GCs are not approved for use in poultry in Korea, high rates of resistance to 3GCs or 4GCs have been continuously reported (42). Odoi et al. (43) reported that the emergence of 3GC- and 4GC-resistant isolates is independent of the use of 3GCs and 4 GCs in farms. Further, UK (44) revealed that the use of other β-lactam compounds, such as AM, induces selective resistance against 3GCs and 4GCs as well as gene expression of ESBLs and/or pAmpC β-lactamases. In Korea, AM is the most common antimicrobial agent used to treat or prevent necrotic enteritis in 2–4-week-old broilers (42). Thus, the high proportion of 3GC- or 4GC- resistant APEC isolates in 1- and 4-week-old broilers may be attributable to the transmission of β-lactam resistant strains persistently maintained in hatchery and farm environments following the use of AM.
Since the introduction of 3GCs in medicine, gram-negative bacteria, especially Enterobacteriaceae such as E. coli, have developed resistance through the production of plasmid-mediated β-lactamase enzymes, such as ESBL and pAmpC β-lactamase. Therefore, the molecular characteristics of ESBL- and pAmpC β-lactamase-producing 3GC- or 4GC-resistant APEC isolates were analyzed in this study. All 49 3GC- or 4GC-resistant APEC isolates possessed one or more β-lactamase genes. In this study, the most prevalent ESBL-encoding genes were CTX-M group I and CTX-M group IV, which is similar to the previous findings in poultry and humans worldwide (31, 45–47). CTX-M types are often associated with coresistance phenotypes- particularly resistant to fluoroquinolones and aminoglycosides (48). Therefore, the existance of CTX-M groups in food animals could reduce the effectiveness of antimicrobial treatment against bacterial infection, as well as prevent the selection of appropriate therapeutic agents.
Interestingly, three isolates contained blaSHV−12. Although blaSHV is one of the most prevalent genes conferring resistance to ESBL in E. coli (8, 49), E. coli containing blaSHV in Korea has not been reported in poultry industries, such as layer hatcheries (11), chicken meat industry (31), and layer operations (41). Thus, emergence of blaSHV−12 in broiler farms may influence the dissemination of the gene among bacterial populations via plasmid-mediated horizontal gene transfer. Therefore, monitoring is essential to track the transmission of blaSHV−12 between different reservoirs in the poultry industry.
Gundran et al. (49) reported that the coexistence of different β-lactamase genes is associated with increased resistance. In this study, 14 (28.6%) isolates contained more than one β-lactamase gene, and the most common combination was blaCTX−M−15+blaTEM−1 (12.2%), followed by blaCTX−M−14+blaTEM−1 (4.1%). Bajpai et al. (50) reported that blaTEM−1 itself is not an ESBL or pAmpC β-lactamase; however, it can acquire ESBL activity through mutations that alter sequences of the amino acids adjacent to the active site of β-lactamase. In this study, isolates containing multiple β-lactamase genes exhibited higher MICs than those containing only blaTEM1. These results are in accordance with the findings of previous studies that the possession of ESBL and pAmpC genes increases resistance to cephalosprins and induces MDR (51, 52).
Class 1 and 2 integrons are highly associated with antimicrobial resistance gene cassettes in bacterial isolates, especially in the isolates of the Enterobacteriaceae family (53–55). In this study, 16 and 26 of the 49 β-lactamase-producing E. coli isolates harbored class 1 and 2 integron genes, respectively. In particular, four isolates with class 1 integron contained gene cassettes comprising aadA and dfrA, which are related with aminoglycoside and trimethoprim resistance, respectively (56, 57). Among the isolates harboring class 2 integron, only one contained a gene cassette comprising estX + sat2 + sat1. Previous studies have reported that the presence of gene cassettes associated with antimicrobial resistance indicates increased resistance rates (58, 59) as well as increased potential for horizontal transfer (60).
E. coli comprises of four CRISPR loci, which are classified as Type I-E (CRISPR 1 and 2) or Type I-F (CRISPR 3 and 4), depending on the presence of the related gene cas (61). The relative prevalence of the CRISPR loci in this study was consistent with the findings of previous studies (62, 63). The prevalence of CRISPR 1 and 2 was high because these two loci have been preserved and stationary within E. coli over a long period, whereas the CRISPR 3 and 4 loci have recently emerged (26, 64). Interestingly, the prevalence of CRISPR 2 was significantly higher in MDR isolates, whereas CRISPR 3 and 4 were significantly more prevalent in non-MDR isolates. Further, Aydin et al. (65) and Kim and Lee (63) reported the overrepresentation of CRISPR I-F among susceptible isolates and the significantly higher prevalence of CRISPR 1 and 2 among MDR isolates. Although the CRISPR system is well known as an immune mechanism of prokaryotes (26, 66), CRISPR I-E system also plays several roles associated with functional abilities, such as gene regulation (67) and pathogenicity (68), by targeting bacterial chromosomes. Moreover, the CRISPR I-F system is considered to interfere with the acquisition of antimicrobial resistance plasmids, causing the isolate to be susceptible (65).
CRISPR arrays consist of diverse spacers, which are short sequences between each repeat, and even closely related isolates have different spacer compositions based on their previous encounters with different phages and plasmids (69). These spacers are derived from protospacers, which are short external sequences at specific locations that are inserted into the array during the process of infection. Thus, the protospacer identity can be crucial in revealing the roles of CRISPR (63). In this study, nine, three, and two protospacer matches were detected in CRISPR 1, 2, and 3, respectively, but none of the protospacers of CRISPR I-E and CRISPR I-F was directly associated with antimicrobial resistance. Our findings indicate that the distribution and characteristics of 3GC or 4GC-resistant APEC differed among the integrated broiler operations, and improved management protocols are required to control of the horizontal transmission of 3GC or 4GC-resistant APEC.
Data availability statement
The original contributions presented in the study are included in the article/Supplementary material, further inquiries can be directed to the corresponding author.
Ethics statement
Ethics approval regarding animal use was not necessary in this study because no live animal was used. The carcasses were collected from the farm and sent directly to the lab.
Author contributions
HK, S-KL, and YL conceived of the presented idea. HK developed the theory and performed the experiments. HK and S-KL verified the analysis. HK wrote the manuscript with supervision from S-KL and YL. All authors contributed to the article and approved the submitted version.
Funding
This work was supported by the Animal and Plant Quarantine Agency, Ministry of Agriculture, Food and Rural affairs, Republic of Korea (Grant Number Z-1543061-2021-23-02).
Conflict of interest
The authors declare that the research was conducted in the absence of any commercial or financial relationships that could be construed as a potential conflict of interest.
Publisher's note
All claims expressed in this article are solely those of the authors and do not necessarily represent those of their affiliated organizations, or those of the publisher, the editors and the reviewers. Any product that may be evaluated in this article, or claim that may be made by its manufacturer, is not guaranteed or endorsed by the publisher.
Supplementary material
The Supplementary Material for this article can be found online at: https://www.frontiersin.org/articles/10.3389/fvets.2022.1055320/full#supplementary-material
References
1. Kaper JB, Nataro JP, Mobley HLT. Pathogenic Escherichia coli. Nat Rev Microbiol. (2004) 2:123–40. doi: 10.1038/nrmicro818
2. Mellata M. Human and avian extraintestinal pathogenic Escherichia coli: infections, zoonotic risks, and antibiotic resistance trends. Foodborne Pathog Dis. (2013) 10:916–32. doi: 10.1089/fpd.2013.1533
3. Kim Y, bin, Yoon MY, Ha JS, Seo KW, Noh EB, Son SH, Lee YJ. Molecular characterization of avian pathogenic Escherichia coli from broiler chickens with colibacillosis. Poult Sci. (2020) 99:1088–95. doi: 10.1016/j.psj.2019.10.047
4. Kathayat D, Lokesh D, Ranjit S, Rajashekara G. Avian pathogenic Escherichia coli (Apec): an overview of virulence and pathogenesis factors, zoonotic potential, and control strategies. Pathogens. (2021) 10:467. doi: 10.3390/pathogens10040467
5. Alber A, Morris KM, Bryson KJ, Sutton KM, Monson MS, Chintoan-Uta C, et al. Avian pathogenic Escherichia coli (APEC) strain-dependent immunomodulation of respiratory granulocytes and mononuclear phagocytes in CSF1R-reporter transgenic chickens. Front Immunol. (2020) 10:3055. doi: 10.3389/fimmu.2019.03055
6. Ewers C, Li G, Wilking H, Kießling S, Alt K, Antáo EM, et al. Avian pathogenic, uropathogenic, and newborn meningitis-causing Escherichia coli: How closely related are they? Int J Med Microbiol. (2007) 297:163–76. doi: 10.1016/j.ijmm.2007.01.003
7. Jeong J, Lee JY, Kang MS, Lee HJ, Kang S, Lee OM, Kwon YK, Kim JH. Comparative characteristics and zoonotic potential of avian pathogenic Escherichia coli (Apec) isolates from chicken and duck in South Korea. Microorganisms. (2021) 9:946. doi: 10.3390/microorganisms9050946
8. Briñas L, Moreno MA, Teshager T, Sáenz Y, Porrero MC, Domínguez L, et al. Monitoring and characterization of extended-spectrum β-lactamases in Escherichia coli strains from healthy and sick animals in Spain in 2003. Antimicrob Agents Chemother. (2005) 49:1262–4. doi: 10.1128/AAC.49.3.1262-1264.2005
9. WHO. WHO list of Critically Important Antimicrobials for Human Medicine (WHO CIA list). (2019). Available online at: https://www.who.int/publications/i/item/9789241515528 (accessed July 21, 2022).
10. Kim H-Y. Statistical notes for clinical researchers: chi-squared test and Fisher's exact test. Restor Dent Endod. (2017) 42:152. doi: 10.5395/rde.2017.42.2.152
11. Seo KW, Shim JB, Kim Y bin, Son SH, Bi Noh E, Yoon S, Lim SK, Ju Lee Y. Impacts and characteristics of antimicrobial resistance of Escherichia coli isolates by administration of third-generation cephalosporins in layer hatcheries. Vet Microbiol. (2020) 243:643. doi: 10.1016/j.vetmic.2020.108643
12. Livermore DM. Current epidemiology and growing resistance of Gram-negative pathogens. Korean J Int Med. (2012) 27:128–42. doi: 10.3904/kjim.2012.27.2.128
13. Carattoli A. Animal reservoirs for extended spectrum b-lactamase producers. Clin Microb Inf. 14:117–23. doi: 10.1111/j.1469-0691.2007.01851.x
14. Korea Agricultural Marketing Information Service. Report of domestic distribution. Naju (2018), p. 251–277.
15. Meguenni N, Le Devendec L, Jouy E, le Corvec M, Bounar-Kechih S, Bakour R, Kempf I. First description of an extended-spectrum cephalosporin- and fluoroquinolone-resistant avian pathogenic Escherichia coli clone in Algeria. Avian Dis. (2015) 1:20–3. doi: 10.1637/10804-022414-Reg.1
16. Papouskova A, Cizek A, A. complex approach to a complex problem: The use of whole-genome sequencing in monitoring avian-pathogenic Escherichia coli – a review. Acta Veterinaria Brno. (2020) 89:273–82. doi: 10.2754/avb202089030273
17. Ministry of Food and Drug Safety (MFDS). Processing Standards and Ingredient Specifications for Livestock Products. Chongju, Korea (2018). Available online at: http://www.foodsafetykorea.go.kr/foodcode/01_02.jsp?idx=263 (accessed January 1, 2018).
18. Candrian U, Furrer B, Höfelein C, Meyer R, Jermini M, Lüthy J. Detection of Escherichia coli and identification of enterotoxigenic strains by primer-directed enzymatic amplification of specific DNA sequences. Int J Food Microbiol. (1991) 12:331–51. doi: 10.1016/0168-1605(91)90148-I
19. Clinical and Laboratory Standards Institute. Performance Standards for Antimicrobial Susceptibility Testing, 31st Edn. Ave Pittsburgh, PA (2021). p. 282.
20. Magiorakos AP, Srinivasan A, Carey RB, Carmeli Y, Falagas ME, Giske CG, et al. Multidrug-resistant, extensively drug-resistant and pandrug-resistant bacteria: an international expert proposal for interim standard definitions for acquired resistance. Clin Microbiol Inf. (2012) 18:268–81. doi: 10.1111/j.1469-0691.2011.03570.x
21. Pérez-Pérez FJ, Hanson ND. Detection of plasmid-mediated AmpC β-lactamase genes in clinical isolates by using multiplex PCR. J Clin Microbiol. (2002) 40:2153–62. doi: 10.1128/JCM.40.6.2153-2162.2002
22. Pitout JDD, Hossain A, Hanson ND. Phenotypic and molecular detection of CTX-M-β-lactamases produced by Escherichia coli and Klebsiella spp. J Clin Microbiol. (2004) 42:5715–21. doi: 10.1128/JCM.42.12.5715-5721.2004
23. Dallenne C, da Costa A, Decré D, Favier C, Arlet G. Development of a set of multiplex PCR assays for the detection of genes encoding important β-lactamases in Enterobacteriaceae. J Antimicrob Chemother. (2010) 65:490–5. doi: 10.1093/jac/dkp498
24. White PA, McIver CJ, Rawlinson WD. Integrons and gene cassettes in the Enterobacteriaceae. Antimicrob Agents Chemother. (2001) 45:2658–61. doi: 10.1128/AAC.45.9.2658-2661.2001
25. Shim JB, Seo KW, Kim Y, bin, Jeon HY, Lim SK, Lee YJ. Molecular characteristics of extended-spectrum and plasmid-mediated AmpC β-lactamase-producing Escherichia coli isolated from commercial layer in Korea. Poult Sci. (2019) 98:949–56. doi: 10.3382/ps/pey417
26. Touchon M, Charpentier S, Clermont O, Rocha EPC, Denamur E, Branger C, et al. Distribution within the Escherichia coli species is not suggestive of immunity-associated diversifying selection. J Bacteriol. (2011) 193:2460–7. doi: 10.1128/JB.01307-10
27. Grissa I, Vergnaud G, Pourcel C. The CRISPRdb database and tools to display CRISPRs and to generate dictionaries of spacers and repeats. BMC Bioinform. (2007) 8:172. doi: 10.1186/1471-2105-8-172
28. Biswas A, Gagnon JN, Brouns SJJ, Fineran PC, Brown CM. CRISPRTarget. RNA Biol. (2013) 10:817–27. doi: 10.4161/rna.24046
29. Yoon S, Lee YJ. Molecular characteristics of Escherichia coli from bulk tank milk in Korea. J Vet Sci. (2022) 23:84. doi: 10.4142/jvs.21084
30. Jeon HY, Seo KW, Kim YB, Kim DK, Kim SW, Lee YJ. Characteristics of third-generation cephalosporin-resistant Salmonella from retail chicken meat produced by integrated broiler operations Poult Sci. (2019) 98:1766–74. doi: 10.3382/ps/pey514
31. Seo KW, Kim Y, bin, Jeon HY, Lim SK, Lee YJ. Comparative genetic characterization of third-generation cephalosporin-resistant Escherichia coli from chicken meat produced by integrated broiler operations in South Korea. Poult Sci. (2018) 97:2871–9. doi: 10.3382/ps/pey127
32. Landers TF, Cohen B, Wittum TE, Larson EL. A review of antibiotic use in food animals: perspective, policy, and potential. Pub Health Rep. (2022) 1:4–22. doi: 10.1177/003335491212700103
33. Chuah LO, Shamila Syuhada AK, Mohamad Suhaimi I, Farah Hanim T, Rusul G. Genetic relatedness, antimicrobial resistance and biofilm formation of Salmonella isolated from naturally contaminated poultry and their processing environment in northern Malaysia. Food Res Int. (2018) 105:743–51. doi: 10.1016/j.foodres.2017.11.066
34. Kim TE, Jeong YW, Cho SH, Kim SJ, Kwon HJ. Chronological study of antibiotic resistances and their relevant genes in Korean avian pathogenic Escherichia coli isolates. J Clin Microbiol. (2007) 45:3309–15. doi: 10.1128/JCM.01922-06
35. Song HS, Bae YC, Kwon HM, Kwon YK, Joh SJ. Loop-mediated isothermal amplification assays for Enterococcussp, Escherichia coli and Staphylococcus aureus in chicken. FEMS Microbiol Lett. (2019) 366:fnz042. doi: 10.1093/femsle/fnz042
36. Kemmett K, Williams NJ, Chaloner G, Humphrey S, Wigley P, Humphrey T. The contribution of systemic Escherichia coli infection to the early mortalities of commercial broiler chickens. Avian Pathol. (2014) 43:37–42. doi: 10.1080/03079457.2013.866213
37. Collingwood C, Kemmett K, Williams N, Wigley P. Is the concept of avian pathogenic Escherichia coli as a single pathotype fundamentally flawed? Front Vet Sci. (2014) 1:5. doi: 10.3389/fvets.2014.00005
38. Mageiros L, Méric G, Bayliss SC, Pensar J, Pascoe B, Mourkas E, et al. Genome evolution and the emergence of pathogenicity in avian Escherichia coli. Nat Commun. (2021) 12:1–3. doi: 10.1038/s41467-021-20988-w
39. Monson MS, Lamont SJ. Genetic resistance to avian pathogenic Escherichia coli (APEC): current status and opportunities. Avian Pathol. (2021) 50:392–401. doi: 10.1080/03079457.2021.1879990
40. Kemmett K, Humphrey T, Rushton S, Close A, Wigley P, Williams NJ. A longitudinal study simultaneously exploring the carriage of apec virulence associated genes and the molecular Epidemiology of faecal and Systemic E. coli in commercial broiler chickens. PLoS ONE. (2013) 8:7749. doi: 10.1371/journal.pone.0067749
41. Seo KW, Shim JB, Lee YJ. Comparative genetic characterization of third-generation cephalosporin-resistant Escherichia coli isolated from a layer operation system in Korea. Poult Sci. (2019) 98:1472–9. doi: 10.3382/ps/pey513
42. Animal and Plant Quarantine Agency. Antimicrobial prescription guidelines for poultry. (2020). Available online at: www.qia.go.kr (accessed July 21, 2022).
43. Odoi JO, Takayanagi S, Yossapol M, Sugiyama M, Asai T. Third-Generation cephalosporin resistance in intrinsic colistin-resistant enterobacterales isolated from retail meat. Antibiotics. (2021) 10:1437. doi: 10.3390/antibiotics10121437
44. New Haw AVMD. UK Veterinary Antibiotic Resistance and Sales Surveillance Report. (2018). Available online at: https://assets.publishing.service.gov.uk/government/uploads/system/uploads/attachment_data/file/1072796/03.05.22_VARSS_Main_Report__Final_Accessible_version__3_.pdf (accessed July 21, 2022).
45. Ho PL, Chow KH, Lai EL, Lo WU, Yeung MK, Chan J, et al. Extensive dissemination of CTX-M-producing Escherichia coli with multidrug resistance to “critically important” antibiotics among food animals in Hong Kong, 2008-10. J Antimicrob Chemother. (2011) 66:765–8. doi: 10.1093/jac/dkq539
46. Hiroi M, Harada T, Kawamori F, Takahashi N, Kanda T, Sugiyama K, Masuda T, Yoshikawa Y, Ohashi N. A survey of-lactamase-producing Escherichia coli in farm animals and raw retail meat in Shizuoka prefecture, Japan. Jap J Inf Dis. (2011) 64:153–5. doi: 10.7883/yoken.64.153
47. Jo SJ, Woo GJ. Molecular characterization of plasmids encoding CTX-M β-Lactamases and their associated addiction systems circulating among Escherichia coli from retail chickens, chicken farms, and slaughterhouses in Korea. J Microbiol Biotechnol. (2015) 26:270–6. doi: 10.4014/jmb.1507.07048
48. Zeynudin A, Pritsch M, Schubert S, Messerer M, Liegl G, Hoelscher M, Belachew T, Wieser A. Prevalence and antibiotic susceptibility pattern of CTX-M type extended-spectrum β-lactamases among clinical isolates of gram-negative bacilli in Jimma, Ethiopia. BMC Infect Dis. (2018) 18:1–10. doi: 10.1186/s12879-018-3436-7
49. Gundran RS, Cardenio PA, Villanueva MA, Sison FB, Benigno CC, Kreausukon K, Pichpol D, Punyapornwithaya V. Prevalence and distribution of bla CTX-M, bla SHV, bla TEM genes in extended- spectrum β- Lactamase- producing E. coli isolates from broiler farms in the Philippines. BMC Vet Res. (2019) 15:1–8. doi: 10.1186/s12917-019-1975-9
50. Bajpai T, Pandey M, Varma M, Bhatambare GS. Prevalence of TEM, SHV, and CTX-M Beta-Lactamase genes in the urinary isolates of a tertiary care hospital. Avicenna J Med. (2017) 7:12–6. doi: 10.4103/2231-0770.197508
51. Moghaddam MJM, Mirbagheri AA, Salehi Z, Habibzade SM. Prevalence of class 1 integrons and extended spectrum beta lactamases among multi-drug resistant Escherichia coli isolates from north of Iran. Iran Biomed J. (2015) 19:233–9. doi: 10.7508/ibj.2015.04.007
52. Liu X, Liu H, Li Y, Hao C. High prevalence of β-lactamase and plasmid-mediated quinolone resistance genes in extended-spectrum cephalosporin-resistant Escherichia coli from dogs in Shaanxi, China. Front Microbiol. (2016) 7:1843. doi: 10.3389/fmicb.2016.01843
53. Ramírez MS, Piñeiro S, Centrón D, Kaufman S, Kovensky J, Vay C, et al. Novel insights about class 2 integrons from experimental and genomic epidemiology. Antimicrob Agents Chemother. (2010) 54:699–706. doi: 10.1128/AAC.01392-08
54. Chen T, Feng Y, Yuan JL Qi Y, Cao YX, Wu Y. Class 1 integrons contributes to antibiotic resistance among clinical isolates of Escherichia coli producing extended-spectrum beta-lactamases. Indian J Med Microbiol. (2013) 31:385–9. doi: 10.4103/0255-0857.118903
55. Sedighi M, Halajzadeh M, Ramazanzadeh R, Amirmozafari N, Heidary M, Pirouzi S. Molecular detection of β-lactamase and integron genes in clinical strains of Klebsiella pneumoniae by multiplex polymerase chain reaction. Rev Soc Bras Med Trop. (2017) 50:321–8. doi: 10.1590/0037-8682-0001-2017
56. Cameron A, Klima CL, Ha R, Gruninger RJ, Zaheer R, McAllister TA. A Novel aadA aminoglycoside resistance gene in bovine and porcine pathogens. mSphere. (2018) 3:e00568-17. doi: 10.1128/mSphere.00568-17
57. Ambrose SJ, Hall RM. DfrA trimethoprim resistance genes found in gram-negative bacteria: compilation and unambiguous numbering. J Antimicrob Chemother. (2021) 76:2748–56. doi: 10.1093/jac/dkab212
58. Gillings MR. Integrons: past, present, and future. Microbiol. and Mol. Biol. Rev. (2014) 78:257–77. doi: 10.1128/MMBR.00056-13
59. Gomig F, Galvão CW, de Freitas DL, Labas L, Etto RM, Esmerino LA, et al. Quinolone resistance and ornithine decarboxylation activity in lactose-negative Escherichia coli. Braz J Microbiol. (2015) 46:753–7. doi: 10.1590/S1517-838246320131291
60. Fonseca ÉL, Vicente AC. Integron functionality and genome innovation: an update on the subtle and smart strategy of integrase and gene cassette expression regulation. Microorganisms. (2022) 10:224. doi: 10.3390/microorganisms10020224
61. Xue C, Sashital DG. Mechanisms of type I-E and I-F CRISPR-Cas systems in Enterobacteriaceae. EcoSal Plus. (2019) 8:2. doi: 10.1128/ecosalplus.ESP-0008-2018
62. Medina-Aparicio L, Dávila S, Rebollar-Flores JE, Calva E, Hernández-Lucas I. The CRISPR-Cas system in Enterobacteriaceae. Pathog Dis. (2018) 76: fty002. doi: 10.1093/femspd/fty002
63. Kim K, Lee YJ. Relationship between CRISPR sequence type and antimicrobial resistance in avian pathogenic Escherichia coli. Vet Microbiol. (2022) 266: 109338. doi: 10.1016/j.vetmic.2022.109338
64. Savitskaya E, Lopatina A, Medvedeva S, Kapustin M, Shmakov S, Tikhonov A, et al. Dynamics of Escherichia coli type I-E CRISPR spacers over 42 000 years. Mol Ecol. (2017) 26:2019–26. doi: 10.1111/mec.13961
65. Aydin S, Personne Y, Newire E, Laverick R, Russell O, Roberts AP, et al. Presence of Type I-F CRISPR/Cas systems is associated with antimicrobial susceptibility in Escherichia coli. J Antimicrob Chemother. (2017) 72:2213–8. doi: 10.1093/jac/dkx137
66. Touchon M, Rocha EPC. The small, slow and specialized CRISPR and Anti-CRISPR of Escherichia and Salmonella. PLoS ONE. (2010) 5:e11126. doi: 10.1371/journal.pone.0011126
67. Bozic B, Repac J, Djordjevic M. Endogenous gene regulation as a predicted main function of Type I-E CRISPR/Cas system in E. coli. Molecules. (2019) 24:784. doi: 10.3390/molecules24040784
68. Kang H-J, Lee Y-J, Kang H-J, Lee Y-J. Citation: distribution of CRISPR in Escherichia coli isolated from bulk tank milk and its potential relationship with virulence. Animals. (2022) 12:503. doi: 10.3390/ani12040503
Keywords: avian pathogenic E. coli, third-or fourth-generation cephalosporin resistance, genetic characteristics, CRISPR typing method, multidrug resistance (MDR)
Citation: Kang HJ, Lim S-K and Lee YJ (2022) Genetic characterization of third- or fourth-generation cephalosporin-resistant avian pathogenic Escherichia coli isolated from broilers. Front. Vet. Sci. 9:1055320. doi: 10.3389/fvets.2022.1055320
Received: 27 September 2022; Accepted: 04 November 2022;
Published: 25 November 2022.
Edited by:
Faham Khamesipour, Shahid Beheshti University of Medical Sciences, IranReviewed by:
Amal Awad, Mansoura University, EgyptMashkoor Mohsin, University of Agriculture, Faisalabad, Pakistan
Raoudha Dziri, Tunis El Manar University, Tunisia
Copyright © 2022 Kang, Lim and Lee. This is an open-access article distributed under the terms of the Creative Commons Attribution License (CC BY). The use, distribution or reproduction in other forums is permitted, provided the original author(s) and the copyright owner(s) are credited and that the original publication in this journal is cited, in accordance with accepted academic practice. No use, distribution or reproduction is permitted which does not comply with these terms.
*Correspondence: Suk-Kyung Lim, aW1zazAwNDkmI3gwMDA0MDtrb3JlYS5rcg==; Young Ju Lee, eW91bmdqdSYjeDAwMDQwO2tudS5hYy5rcg==
†ORCID: Hyo Jung Kang orcid.org/0000-0003-0244-4724
Young Ju Lee orcid.org/0000-0001-6108-2845