- 1Research Institute of Fish Culture and Hydrobiology, South Bohemian Research Center of Aquaculture and Biodiversity of Hydrocenoses, University of South Bohemia Ceske Budejovice, Ceské Budějovice, Czechia
- 2Instituto de Oceanografía y Cambio Global, IOCAG, Universidad de Las Palmas de Gran Canaria, Las Palmas de Gran Canaria, Spain
- 3Nishiura Station, South Ehime Fisheries Research Center, Ehime University, Matsuyama, Japan
Sturgeons are the most endangered species group and their wild populations continue to decrease. In this study, we apply intracytoplasmic sperm injection (ICSI), an assisted reproductive technology, for the first time in endangered and critically endangered sturgeons. Using various egg-sperm species combinations we performed different ICSI experiments with immobilized pre- or non-activated spermatozoa, single or many, fresh or cryopreserved. Then we evaluated the fertilization success as well as the paternity of the resultant embryos and larvae. Surprisingly, all experimental groups exhibited embryonic development. Normal-shaped feeding larvae produced in all egg-sperm species-combination groups after ICSI using single fresh-stripped non-activated spermatozoa, in one group after ICSI using single fresh-stripped pre-activated spermatozoa, and in one group after ICSI using multiple fresh-stripped spermatozoa. ICSI with single cryopreserved non-activated spermatozoa produced neurula stage embryos. Molecular analysis showed genome integration of both egg- and sperm-donor species in most of the ICSI transplants. Overall, ICSI technology could be used as an assisted reproduction technique for producing sturgeons to rescue valuable paternal genomes.
1. Introduction
Intracytoplasmic sperm injection (ICSI) is an assisted reproductive technology that aims to treat male factor infertility in both humans and animals as well as to make a significant contribution to basic science as a useful tool in the research of gamete biology (1–7). The technology involves the microinjection of a single sperm or a sperm head (nucleus) into the cytoplasm of a mature metaphase II egg, by passing the natural process of egg-sperm interaction (8). Although the cell membranes of the egg and sperm are not in contact, sperm delivered in this manner initiates Ca2+ responses in the egg that closely resemble those initiated by in vitro fertilization (9–12). Research in mice revealed that the ICSI technology produced viable fetuses that did not exhibit more spontaneous mutations than naturally conceived and gestated fetuses (13). The ICSI technology has been demonstrated in humans to support high rates of development to term (14, 15).
The use of ICSI technology provides the possibility to fertilize eggs with semen on reduced sperm concentration or motility (16). In this sense, ICSI could be an option to achieve in vitro-produced embryos in species like camelids in which the recovery of adequate quantity and quality of sperm for conventional in vitro fertilization is problematic (17). In addition, the ICSI technology offers fertilization using sexed semen (18), cryopreserved semen (19, 20), lyophilized semen (21, 22), or semen collected postmortem (23). The last provides the possibility to produce offspring from gametes of deceased animals (23). In this sense, it may be a useful reproductive technology for endangered species when no motile or reduced motility sperm is retrieved from cadavers (24). Furthermore, ICSI could contribute as a useful tool in conservation efforts of endangered species in which the males might develop a higher proportion of abnormal sperm during captivity. These are the cases for the clouded leopard and cheetah, where a high level of sperm abnormalities has been detected in their captive populations (25, 26). ICSI technology could have a useful application in aquaculture because it provides the possibility of hybridization between homogeneous and even heterogeneous fish species, but care should be taken to prevent genetic pollution to natural populations.
ICSI was first performed in starfish (27), followed by sea urchin (28), mammals such as human (14, 29), farm animals like sheep (5), horse (3), and pig (4), as well as amphibians like Xenopus (30, 31), with various successes. More recently, after interspecific ICSI using domestic cat eggs and wild felids such as cheetah or leopard fresh-stripped spermatozoa, developmental rates until blastocyst have been achieved (32). Success until blastocyst was also reported in an Italian endangered sheep breed named Pagliarola when lyophilised spermatozoa was used (22).
ICSI in fish has been performed in a few species like the crucian carp (33), zebrafish (34), Nile tilapia (19), medaka (7), and dojo loach (35). Hatching rates using fresh-stripped spermatozoa have been succeeded in medaka (13.4%) (7) and in Nile tilapia (8.5 %) (19), while production of fertile adults has been successful only in medaka (5 %) (36). To our knowledge, the ICSI technology has not yet been applied to large endangered fish species. Recent efforts have been made to apply assisted reproductive technologies in sturgeon, the most endangered group of animals (37). Application of the somatic cell nuclear transfer (SCNT) technology in sturgeons (38, 39) yielded a success rate of 0.8% in producing fin cell-donor-derived embryos (39).
Parmar et al. (8) proposed the ICSI technology as the last resort of species conservation when all other conventional methods of insemination fail. In the aim to put more arrows in the quiver for the conservation of endangered sturgeon species, the current study applies for the first time ICSI in sturgeons. We evaluate the ICSI technology using the endangered sterlet, Acipenser ruthenus, the critically endangered Siberian sturgeon, Acipenser baerii, the critically endangered beluga sturgeon, Huso huso, and the critically endangered Russian sturgeon, Acipenser gueldenstaedtii (37) in various egg-sperm species combinations. Using non-activated unfertilized eggs arrested in meiosis II stage, we performed different ICSI experiments. To start with, the ICSI of a single non-activated or pre-activated for 15 s fresh-stripped immobilized spermatozoa was used. The later experiment tested the spermatozoa efficiency to fertilize with induced acrosome reaction that showed to have a positive outcome in in vitro fertilization (40). Secondly, following the sturgeon reproductive biology, we applied ICSI of multiple non-activated fresh-stripped spermatozoa. Polyspermy happens naturally in the sturgeon populations (41), resulting in viable offspring after in vitro fertilization (42). Last but not least, after ICSI of single non-activated cryopreserved spermatozoa of 40 % motility, we tested the possibility to fertilize sturgeon eggs. After the different ICSI experiments, the success of fertilization as well as the paternity of the resulted embryos and larvae have been evaluated.
2. Methods
2.1. Ethics
The ICSI experiments took place at the aquaculture facility of the Genetic Fisheries Center and Laboratory of Germ Cells at the Faculty of Fisheries and Protection of Waters, University of South Bohemia in Ceské Budějovice, Vodnany, Czech Republic. All experimental procedures were performed in accordance with national (reference number: 2293/2015-MZE-17214) and institutional guidelines on animal experimentation and care and approved by the Animal Research committee of the University of South Bohemia in Ceské Budějovice.
2.2. Fish and gamete collection
During the ICSI experiments, a mature female Russian sturgeon (23–24-year-old), Siberian sturgeon (14–15-year-old), and beluga (22–23-year-old), as well as two mature female sterlets (4–5-year-old), were used as egg-donor species. Moreover, a mature male of sterlet (5–6-year-old), Siberian sturgeon (14–15-year-old), and beluga sturgeon (22–23-year-old) were used as sperm-donor species. All fish were kept at constant temperatures of 15°C at least 1 week before collection of gametes. In order to induce ovulation, the females were injected with an intramuscular injection of carp pituitary homogenized extract (CPE) at a dose of 5 mg/kg body weight (b.w.) in two steps: first with 0.5 mg/kg b.w. and second with 4.5 mg/kg b.w. 12 h after the first injection. Egg collection in sterlets was performed 22–24 h after the second injection. Beluga, Siberian, and Russian sturgeon females were stripped 28–30 h after the second injection.
In order to induce spermiation, the males were injected once (4 mg/kg b.w.) with CPE and spermatozoa was collected 24–36 h after hormonal injection by use of a catheter from the urogenital papilla, transferred to a separate cell culture container (250 mL), and stored at 4°C until sampling (1–3 h).
2.3. Gametes' preparation
Non-activated and unfertilized eggs from sterlet, Siberian sturgeon, Russian sturgeon, and beluga arrested in meiosis II stage were washed three times for 5 min with Phosphate Buffered Saline (PBS) to remove debris and somatic cells from the spawning (38). Then they were placed in a 6 cm2 petri dish filled with PBS at 15°C for ICSI.
To simulate reduced motility spermatozoa with acrosomal reaction retrieved from cadavers, we pre-activated the spermatozoa before ICSI. In an Eppendorf tube (1.5 ml) a drop of fresh stripped spermatozoa (1.5 μl) from sterlet or Siberian sturgeon was diluted (1/50) in filtrated water (15°C) for its activation for 15 s. The pre-activated spermatozoa were then transferred in PBS to return in inertia and held at 4°C until its use for the ICSI experiments.
Spermatozoa cryopreservation from beluga sturgeon was performed according to Glogowski et al. (43). Fresh sperm was diluted with cryomedium in a ratio of 1:1. The composition of cryomedium was 10 % ethanol, 23.4 mM sucrose, 0.25 mM KCl, and 30 mM Tris with pH adjusted to 8.0 by using HCl. The diluted sperm with cryomedium was pipetted into 0.5 ml pejets and kept 3 cm above liquid nitrogen surface for 10 min. Afterwards, the pejets were immersed and stored in liquid nitrogen until use. Beluga sturgeon's spermatozoa thawing was performed in a 36°C water bath for 15 s.
Spermatozoon motility was evaluated visually for the percentage of motile spermatozoa after activation and total duration of motility (in seconds). Motility parameters were measured immediately after initiation of spermatozoon activation until 100 % of spermatozoa were immotile. To induce the initiation of spermatozoon motility, a 49 μl drop of medium was placed on a glass slide and then a drop of 1 μl fresh spermatozoa was diluted using a microsampler. All experiments were performed in triplicate at room temperature (17–20°C), using light microscopy under 400 × magnification. To avoid subjective bias, all measurements were carried out by the same experimenter.
2.4. Egg-sperm species combinations, ICSI experiments, treatment, and culture of the embryos
In order to perform ICSI using single spermatozoa, fresh (non- or pre-activated) or cryopreserved, we placed a drop of non-diluted sperm (1.5 μl) close to the eggs. Both eggs and spermatozoa remained inactivated in a petri dish filled with PBS (15°C). To assure that the microinjection needle would aspirate only one spermatozoon at a time, the oil running through the microneedle was creating a small drop that was carefully removed before aspiration. This procedure was used to aspirate as little PBS as possible together with the single spermatozoon. ICSI using fresh single non- or pre- activated spermatozoa was performed between beluga eggs and Siberian sturgeon spermatozoa, between Siberian sturgeon eggs and sterlet spermatozoa, as well as between Russian sturgeon eggs and sterlet spermatozoa. ICSI using cryopreserved single spermatozoa was performed between sterlet eggs and beluga spermatozoa (~40 % motility, evaluation under microscope).
To perform the multiple ICSI, diluted spermatozoa in PBS (0.001 %, 15°C) was filled in a microneedle and was not floated with the eggs in a petri dish. Each egg was microinjected with a single manipulation in the animal pole (~ 0.23 μl/egg). This corresponds to ~ 3827 sterlet spermatozoa per Siberian or Russian sturgeon egg and ~ 3905 Siberian sturgeon spermatozoa/beluga egg. Multiple ICSI were performed between Siberian sturgeon eggs and sterlet spermatozoa, between Russian sturgeon eggs and sterlet spermatozoa, as well as between beluga eggs and Siberian sturgeon spermatozoa.
All ICSI experiments were performed under a stereomicroscope (Leica M165 FC.) using a hydraulic injector (Cell-Tram Oil; Eppendorf, Germany) connected to a micromanipulator (MO-152; Narishige, Japan) on the animal pole of the eggs where the multiple micropyles are located. Application of a shallow injection was used during the ICSI technology, as it has been demonstrated in sturgeon microinjection techniques to result in high developmental rates (39). All transplants were incubated in PBS (15°C) for 30–40 min, washed three times with PBS to remove the floating spermatozoa in the petri dish, and then activated with filtrated water (15°C).
At the same time, in vitro fertilization of the control groups with fresh-stripped sperm was performed: beluga eggs x Siberian sturgeon spermatozoa (n = 31, fertilization rate = 90.3%), Siberian sturgeon eggs x sterlet spermatozoa (n = 80, fertilization rate = 90%), and Russian sturgeon eggs x sterlet spermatozoa (n = 87, fertilization rate = 90.8%) (Table 1). Moreover, in vitro fertilization of the control group with cryopreserved spermatozoa was performed: sterlet eggs x beluga spermatozoa (n = 583, fertilization rate = 51.5%) (Table 2). To confirm that spermatozoa were not motile nor sticky in the surface of eggs and had no ability to fertilize the eggs in the injection medium (PBS), we made a negative control group. We placed beluga eggs (n = 184) with Siberian sturgeon spermatozoa in PBS for 30 min, then we washed the eggs three times with PBS to remove the spermatozoa from the petri dish, and placed the eggs in filtrated water. Using the IBM SPSS statistic 27 software, Fisher's exact test was used to determine if there was a significant association in developing rate between the control group and ICSI experiments for each species gamete combination and developmental stage (Supplementary Figure S1). The negative control group exhibited neither fertilization nor embryonic development (Table 1).
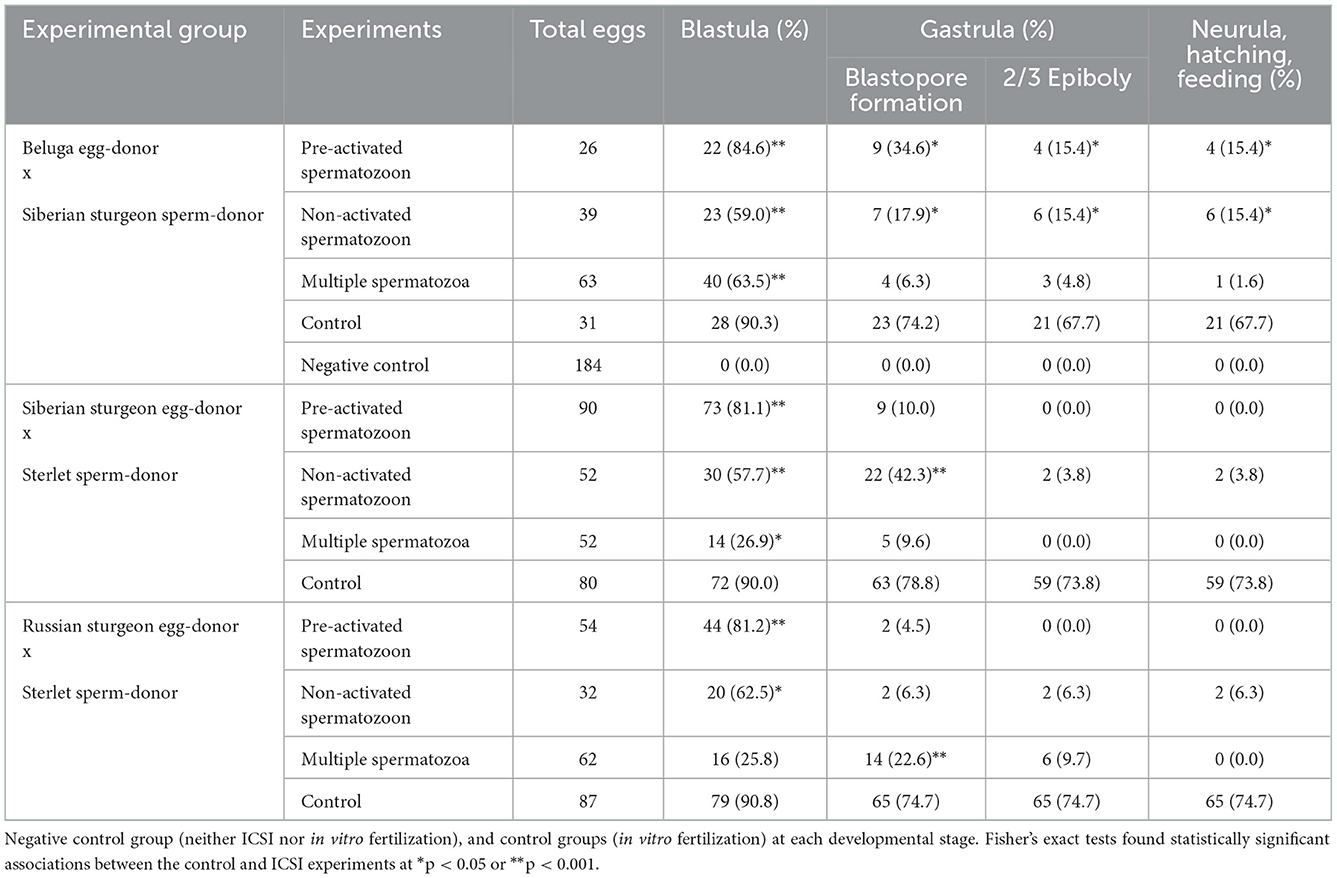
Table 1. Developing rates of transplants after the different ICSI experiments using fresh-stripped spermatozoa and non-activated eggs.
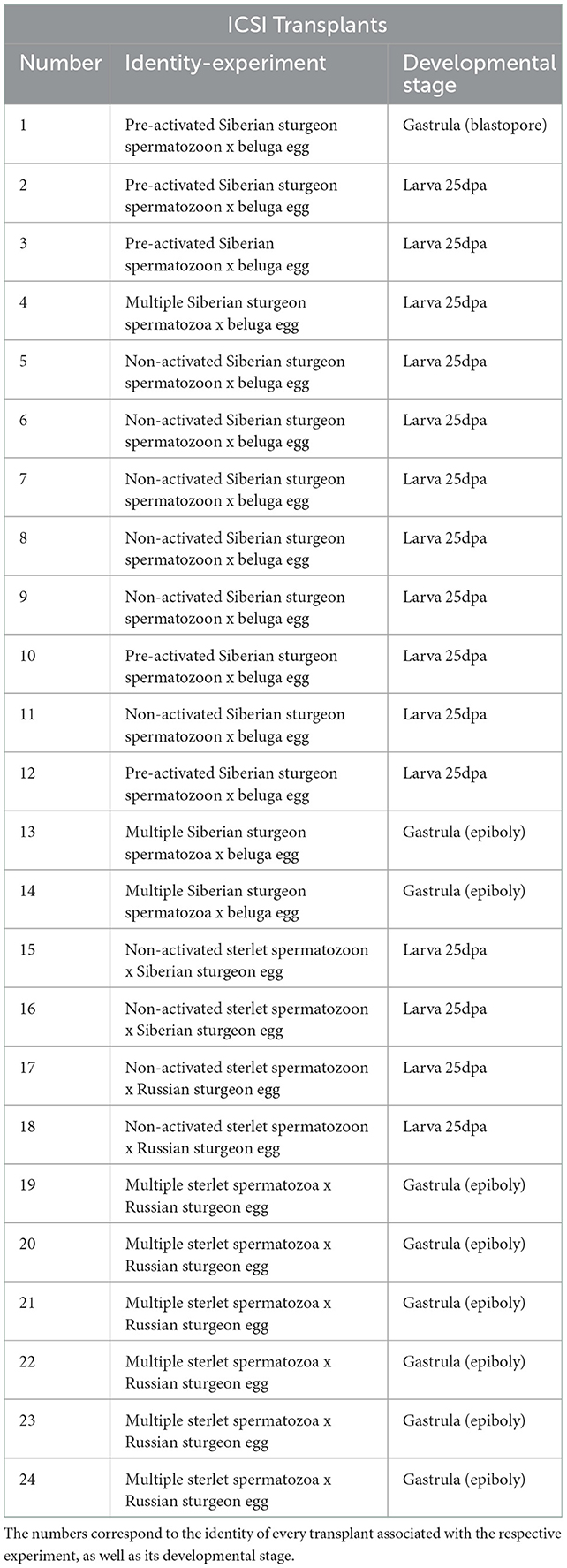
Table 2. Information of transplants after ICSI experiments with fresh-stripped spermatozoa used for the molecular analysis.
Immediately after activation of the ICSI transplants and control groups with filtrated water, all embryos were treated with 0.01% tannic acid (SIGMA-ALDRICH®) alternated with filtrated water for 10 min to remove the egg surface stickiness. Development was observed at the two-cell stage, corresponding to 3–4 h post activation (hpa). At 10 hpa, forceps were used to remove the outer layers of chorion for better observation of the development. Developing embryos were placed in 0.01% penicillin and 0.01% streptomycin in filtered water (15°C) for 3 days. The inner layer of the chorion was removed at 5–6 days post activation. First feeding started after yolk resorption, 25 dpa. The freshwater annelid worm tubifex sp. was given twice per day at 8:00 am and 16:00 pm. Tanks were cleaned twice per day 2 h after the meal. All embryos and larvae were held at the ambient photoperiod at a water temperature of 15°C.
2.5. Molecular genotyping
Genomic DNA was extracted from caudal fin tissue of five egg-donors (a beluga sturgeon, a Siberian sturgeon, a Russian sturgeon, and two sterlets), three sperm-donors (a Siberian sturgeon, a sterlet, and a beluga sturgeon), along with 37 ICSI transplants, and one in vitro produced control embryo using GenElute Mammalian Genomic DNA Miniprep Kit (SIGMA-ALDRICH®) according to the manufacturer's instructions. The presence of the beluga sturgeon's genome in the respective ICSI transplants was investigated using beluga specific primer pair 153_HHp + 153_uni (44) that amplifies 153 bp fragment of beluga nuclear DNA. The presence of sterlet's genome in the respective ICSI transplants was tested by sterlet specific primer pair 247_ARp + 247_uni (44), which amplifies 247 bp fragment from sterlet nuclear DNA. The presence of Siberian or Russian sturgeon's genome in the respective ICSI transplants was tested using specific primer pair 395_ABp + 395_uni (45), which amplifies 395 bp fragment from Siberian or Russian sturgeon's nuclear DNA. All reactions were performed according to (44, 45) in two independent replicates. Together with the species-specific primers from above, the genomic constitution of the ICSI transplants was estimated by parentage-like assignment using six microsatellite markers: AciG_35 (46), AfuG_135 (47), Aox_45 (48), Spl_101, Spl_163, and Spl_173 (49). Amplification and microsatellite fragment analysis were carried out according to the protocol described by Havelka et al. (50). Genotypes were scored in GENEIOUS 8.1.9, using Microsatellite Plugin 1.4.4.
3. Results
3.1. ICSI using fresh-stripped spermatozoa
ICSI of non-activated single spermatozoa from Siberian sturgeon into beluga unfertilized eggs reconstructed 39 transplants, from which 23 (59.0 %) exhibited initial cleavages and reached the blastula stage. Seven (17.9%) reached the gastrula stage from which 6 (15.4 %) fulfilled epiboly, neurulation, hatching, and feeding until 25 dpa, upon which they were sacrificed. Pre-activated and immobilized single spermatozoa from Siberian sturgeon were injected into beluga unfertilized eggs reconstructing 26 transplants, from which 22 (84.6 %) exhibited initial cleavages and reached the blastula stage. Nine transplants (34.6 %) formed the blastopore in the gastrula stage and 4 (15.4 %) fulfilled the epiboly, the neurula stage, hatched, and fed until 25 days post activation (dpa), upon which they were sacrificed. ICSI using multiple spermatozoa with a single manipulation from Siberian sturgeon into beluga eggs resulted in reconstruction of 63 transplants from which 40 (63.5 %) exhibited initial cleavage and reached the blastula stage. Four transplants (6.3 %) entered the gastrula stage, three (4.8 %) fulfilled epiboly, and one (1.6 %) entered the neurula stage, hatched, and fed until 25 dpa, upon which it was sacrificed (Table 1).
Sterlet pre-activated and immobilized single spermatozoa were injected into Siberian sturgeon unfertilized eggs reconstructing 90 transplants, from which 73 (81.1 %) exhibited initial cleavages and reached the blastula stage. Nine transplants (10.0 %) formed the blastopore at gastrula stage and stopped development. ICSI of non-activated single spermatozoa from sterlet into Siberian sturgeon unfertilized eggs reconstructed 52 transplants, from which 30 (57.7 %) exhibited initial cleavages and reached the blastula stage. Twenty-two (42.3 %) reached the gastrula stage from which 2 (3.8 %) fulfilled epiboly, entered the neurula stage, hatched, and fed until 25 dpa, upon which they were sacrificed. ICSI using multiple spermatozoa with a single manipulation from sterlet into Siberian sturgeon eggs resulted in reconstruction of 52 transplants from which 14 (26.9 %) exhibited initial cleavage and reached the blastula stage. Five (9.6 %) reached the gastrula stage at blastopore formation and stopped development (Table 1).
Sterlet pre-activated (15 s) and immobilized single spermatozoa were injected into Russian sturgeon unfertilized eggs reconstructing 54 transplants, from which 44 (81.2 %) exhibited initial cleavages and reached the blastula stage. Two transplants (4.5 %) formed the blastopore at gastrula stage and stopped development. ICSI of non-activated single spermatozoa from sterlet into Russian sturgeon unfertilized eggs reconstructed 32 transplants, from which 20 (62.5 %) exhibited initial cleavages and reached the blastula stage. Two (6.3 %) reached the gastrula stage, the neurula stage, hatched, and fed until 25 dpa, upon which they were sacrificed. ICSI using multiple spermatozoa with a single manipulation from sterlet into Russian sturgeon eggs resulted in reconstruction of 62 transplants from which 16 (25.8 %) exhibited initial cleavage and reached the blastula stage. Fourteen (22.6 %) reached the gastrula stage at blastopore formation and 6 (9.7 %) fulfilled epiboly and stopped development (Table 1).
3.2. Molecular analysis after ICSI using fresh-stripped spermatozoa
In total, 24 ICSI transplants were analyzed for the presence of DNA of both egg- and sperm-donor species (Table 2).
The contribution of Siberian sturgeon sperm-donor DNA in developing embryos was studied in 14 specimens (Figure 1). We combined two markers: 395_AB (45) and 153_HH (44). The marker 395_AB amplifies 395 bp band only if a sample contains Siberian's and/or Russian's sturgeon genomic DNA while the marker 153_HH amplifies 153 bp band only if a sample contains genomic DNA of beluga. Ten transplants coming from the group ICSI with pre-activated single spermatozoa (n = 5), from the group ICSI with non-activated single spermatozoa (n = 3), and from the group of ICSI with multiple spermatozoa (n = 2) showed presence of DNA from both parents, thus confirming successful incorporation of sperm-donor DNA in the transplants (71.4 %). One transplant from the group ICSI using non-activated single spermatozoa showed contribution of only sperm-donor's DNA without any contribution from the beluga egg-donor (7.1 %). Three transplants showed amplification of DNA only from females (21.4 %) from the group ICSI with multiple spermatozoa (n = 1) and from the group ICSI with non-activated spermatozoa (n = 2) (Figure 1).
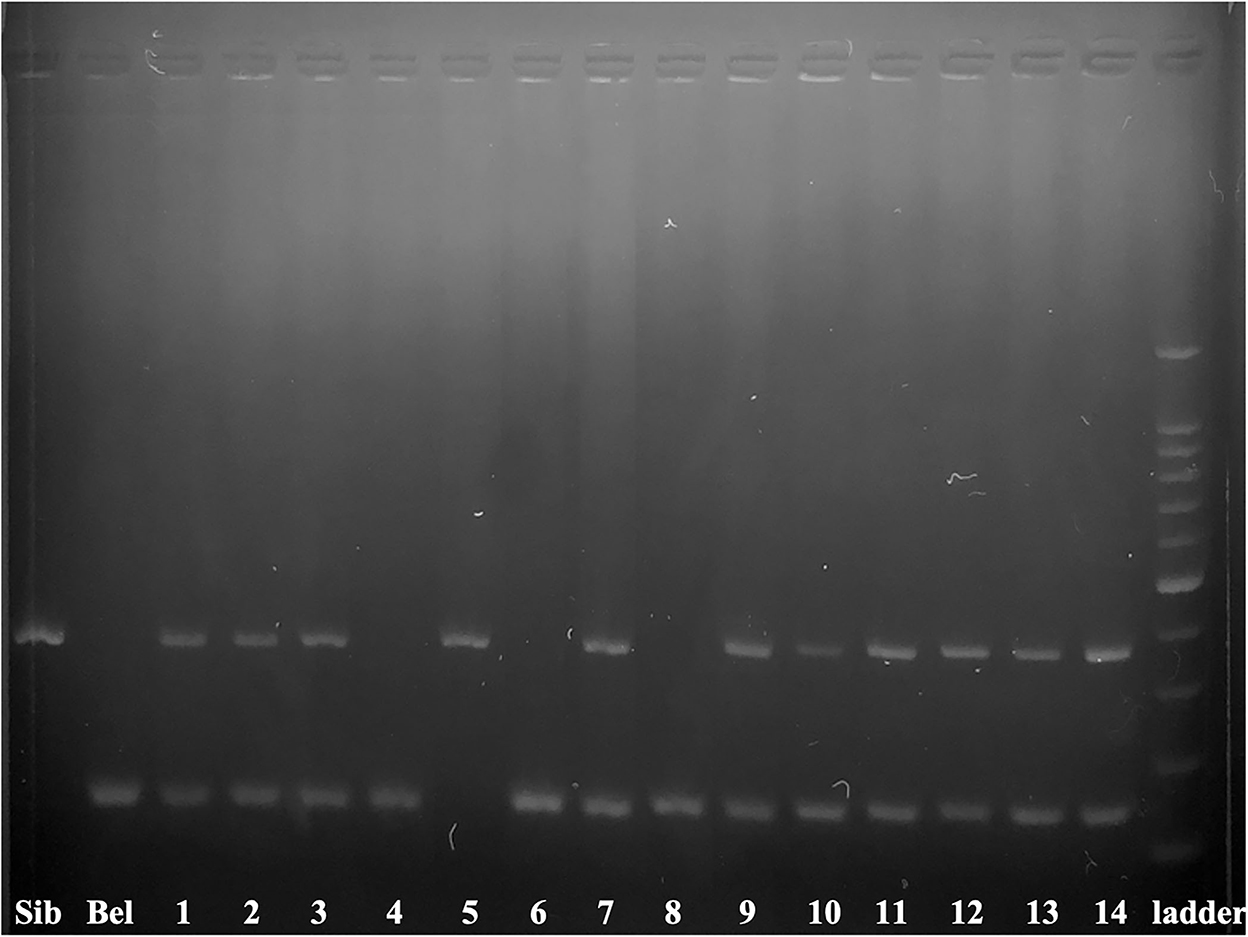
Figure 1. Electrophoretogram of 395_AB and 153_HH markers in 14 ICSI produced embryos. The marker 395_AB amplifies 395 bp band only when the sample contains Siberian and/or Russian sturgeon nuclear DNA while the marker 153_HH amplifies 153 bp band only when a sample contains nuclear DNA of beluga. Numbers correspond to the embryos and larvae of Table 2. Siberian sturgeon fin (Sib) and beluga fin (Bel) are used as positive controls 100–1500 bp ladder.
Efficiency of ICSI was studied in 10 transplants (Figure 2), by species specific markers. We combined two markers: 395_AB (45) and 247_AR (44). The marker 247_AR amplifies 247 bp band only if a sample contains genomic DNA of sterlet. Consequently, 9 specimens (90 %) showed presence of DNA from both parents, from the group ICSI using non-activated single sterlet spermatozoa into Siberian sturgeon's eggs (n = 2), the group ICSI using non-activated single sterlet spermatozoa into Russian sturgeon's eggs (n = 2), and the group ICSI using multiple sterlet spermatozoa into Russian sturgeon's eggs (n = 5). One specimen showed development of only egg-donor's DNA (10 %) in the group ICSI using multiple spermatozoa from sterlet into Russian sturgeon's eggs (Figure 2).
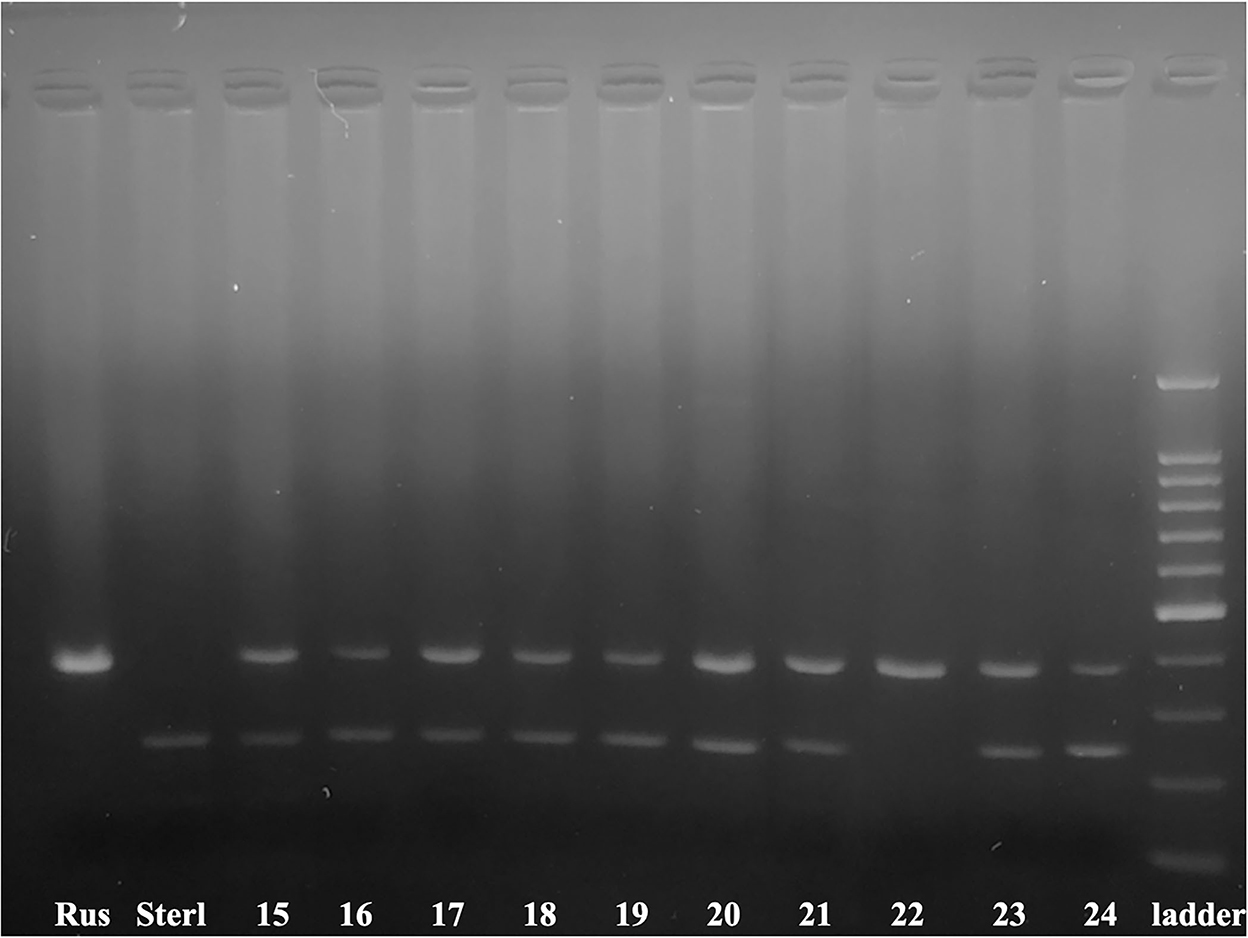
Figure 2. Electrophoretogram of 395_AB and 247_AR markers in 10 ICSI produced transplants. The marker 395_AB amplifies 395 bp band only when a sample contains Siberian and/or Russian sturgeon nuclear DNA and the marker 247_AR amplifies 247 bp band only when a sample contains nuclear DNA of sterlet. Numbers correspond to the embryos and larvae of Table 2. Russian sturgeon fin (Rus) and sterlet fin (Sterl) are positive controls 100–1500 bp ladder.
Microsatellite analysis of the ICSI transplants from Table 2 showed that 19 transplants (N°1–3, 7, 9–21, 23, and 24) contained both egg- and sperm-donor's DNA. One transplant (N°21) contained two haploid genomes from the sperm-donor according to the microsatellite markers AfuG_135 and Spl_163. Furthermore, one transplant (N°5) contained only sperm-donor's DNA, without any genomic contribution from the egg-donor (Table 3).
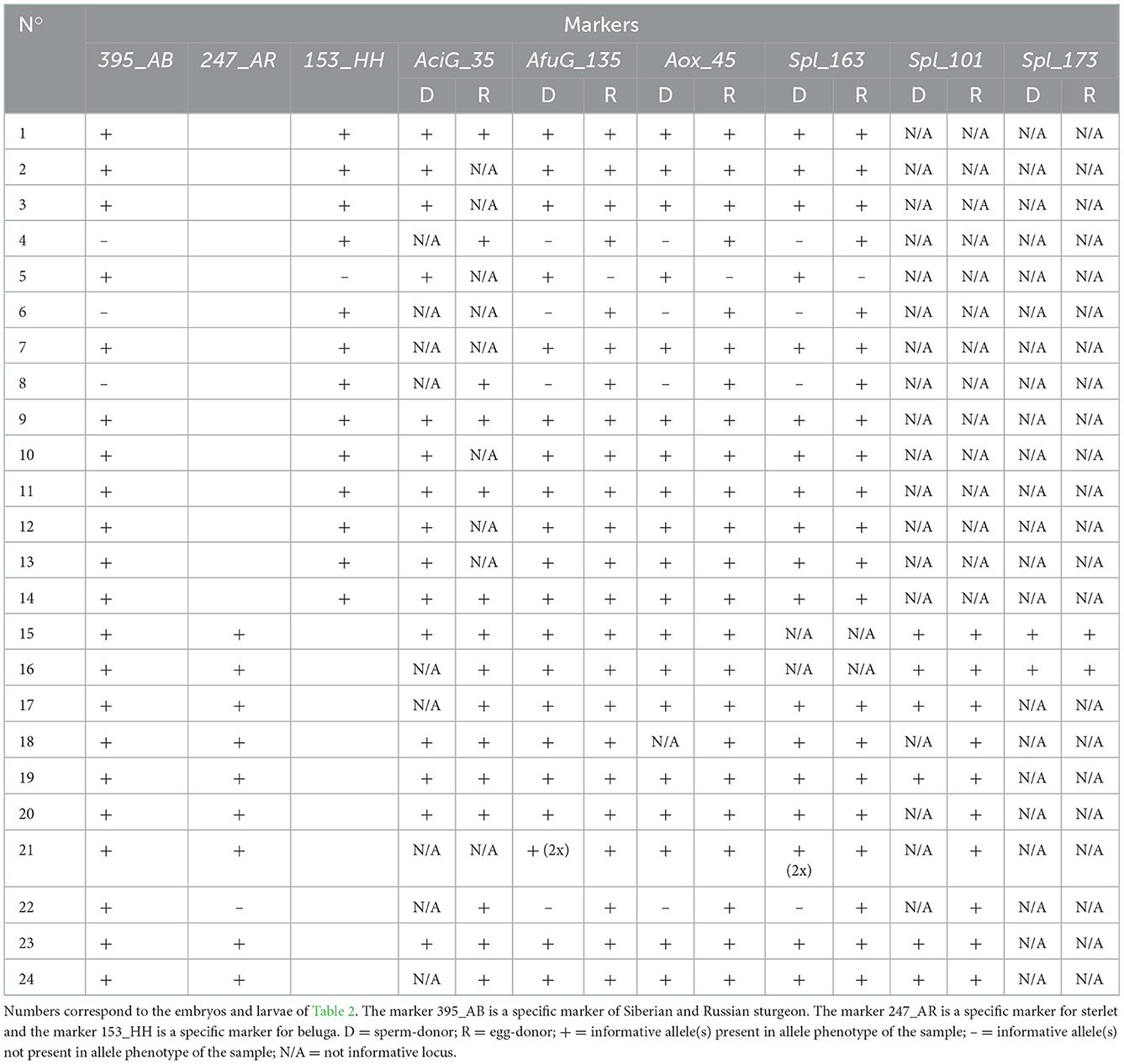
Table 3. Molecular analysis with microsatellite markers after different ICSI experiments using fresh-stripped spermatozoa.
3.3. ICSI using cryopreserved spermatozoa
Non-activated single cryopreserved spermatozoa from beluga sturgeon (~ 40 % motility) were injected into sterlet unfertilized and non-activated eggs. Ninety-seven transplants reconstructed, from which 22 (22.7 %) exhibited initial cleavages and reached the blastula stage. Thirteen transplants (9.3 %) formed the blastopore, 10 (6.2 %) fulfilled the epiboly, and 4 (4.1 %) reached the neurula stage (Table 4).

Table 4. Developing rates of transplants after ICSI using single non-activated cryopreserved beluga spermatozoa (~ 40 % motility) into non-activated sterlet eggs and control group at each developmental stage.
3.4. Molecular analysis after ICSI using cryopreserved spermatozoa
In total, 13 ICSI transplants were analyzed for the presence of DNA of both egg- and sperm-donor species (numbers 25–33 and 35–38) and one control embryo (number 34) that was produced after in vitro fertilization of beluga cryopreserved spermatozoa to sterlet eggs. The transplants were created after ICSI using single cryopreserved spermatozoa from beluga into sterlet eggs. Numbers 25–27 arrested at gastrula stage in blastopore formation, 28–33 arrested at gastrula stage at 2/3 epiboly, and 35–38 arrested at neurula stage. The control embryo (number 34) arrested at neurula stage (Table 5).
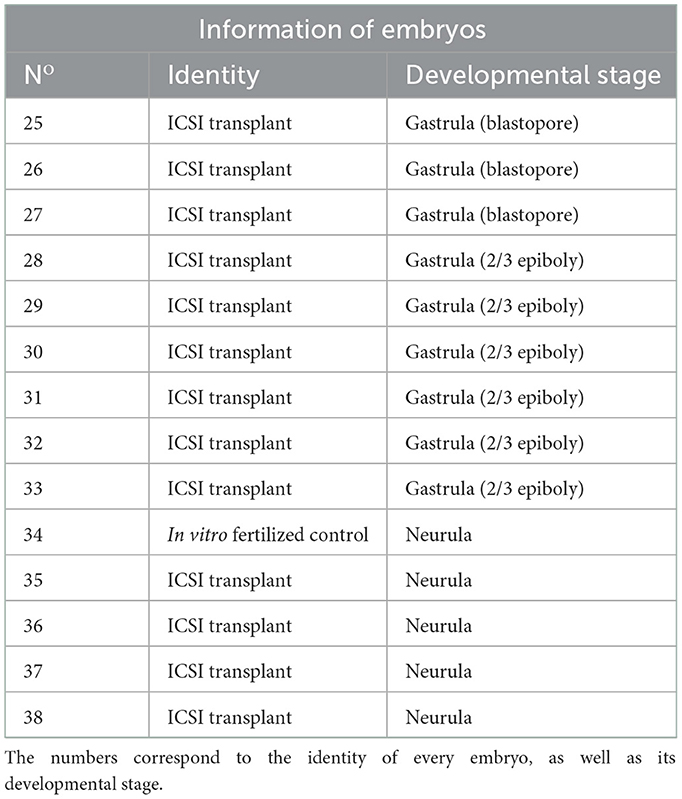
Table 5. Information of transplants after ICSI with single cryopreserved beluga spermatozoa into sterlet eggs and one in vitro produced control embryo used for the molecular analysis.
Molecular analysis of 14 embryos has been performed by species specific markers (Figure 3, Table 6). We combined two markers: 247_AR and 153_HH (44). Eight ICSI transplants (61.5 %) showed presence of DNA from both parents, while 5 (38.5 %) showed development of only sterlet egg-donor's DNA without contribution of beluga sperm-donor's DNA (Figure 3, Table 6).
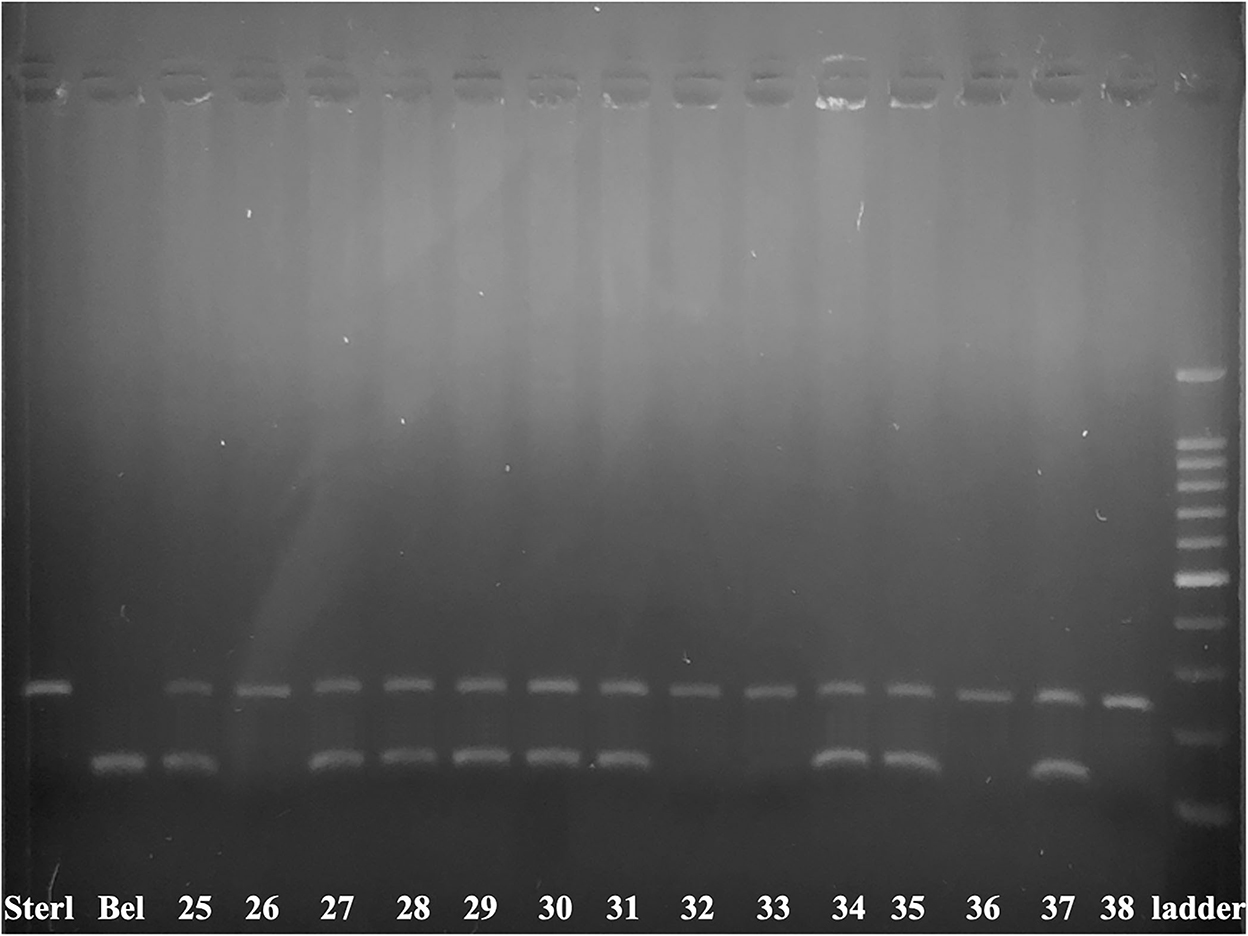
Figure 3. Electrophoretogram of 153_HH and 247_AR markers in 14 produced embryos. The marker 247_AR amplifies 247 bp band only when the sample contains nuclear DNA of sterlet, while the marker 153_HH amplifies the 153 bp band only when the sample contains nuclear DNA of beluga. Sterlet fin (Sterl) and beluga fin (Bel) are positive controls. The numbers 25–33, 35–38 are corresponding to transplants after ICSI of single non-activated cryopreserved beluga spermatozoa into sterlet's eggs. Number 34 is a control embryo produced after in vitro fertilization of cryopreserved beluga spermatozoa with sterlet eggs 100–1500 bp ladder.
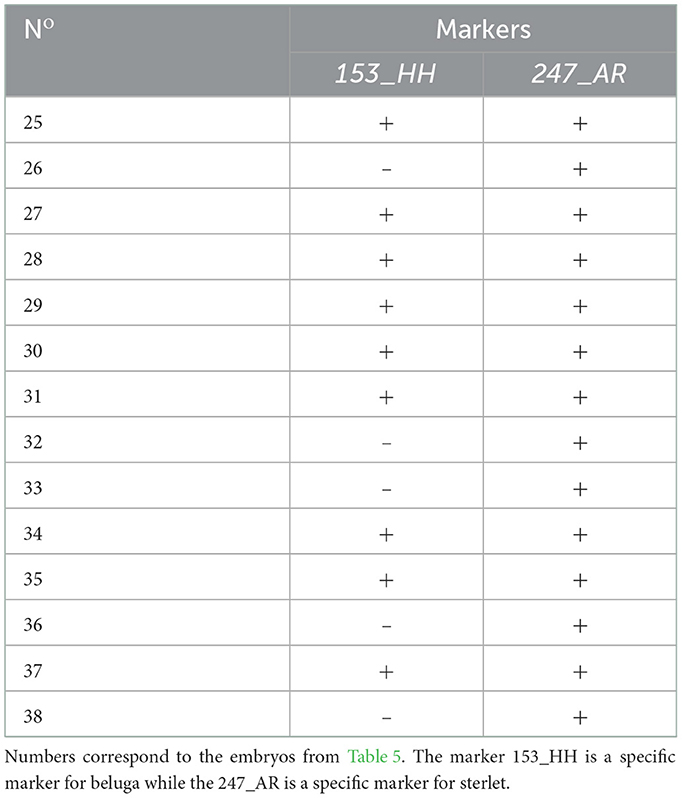
Table 6. Molecular analysis with species specific markers after ICSI using single cryopreserved spermatozoa from beluga sturgeon into sterlet eggs.
3.5. Phenotypic analysis of transplants after the ICSI technology
All resultant embryos and larvae followed a normal developmental pattern (38, 39, 51) without visible abnormalities in shape.
4. Discussion
As far as we know, this is the first study of ICSI technology in sturgeon, the most critically endangered fish in the world (37). In this study, different egg-sperm species combinations were used, ranging between endangered to critically endangered. We chose to perform ICSI using different species because sturgeon hybridize naturally, producing viable fertile offspring (52–55), and are used widely for aquaculture purposes (54).
ICSI technology does not require motile spermatozoa to produce healthy offspring as long as the sperm nucleus has intact genetic integrity (56, 57). Egg activation (7, 34, 35) or non-activation (19, 34) have been used in fish ICSI. After sturgeon ICSI, feeding larvae were produced in most experimental groups when fresh-stripped sperm injected (up to 15.4 %). Comparing our results with ICSI in teleost fish species which exhibit meroblastic embryonic development, various hatching rates are shown: 1.6 % in zebrafish (34), 8.5 % in Nile tilapia (19), and 13.4 % (7) and 6.7 % (36) in medaka. In amphibians which exhibit holoblastic embryonic development similar to sturgeon embryos (51), after ICSI of African clawed frog the success rate of production metamorphosed larvae was 0.7 % (58).
All the experimental groups with the fresh-stripped spermatozoa (ICSI of single pre-activated or non-activated spermatozoa and ICSI of multiple spermatozoa) and the cryopreserved spermatozoa group (ICSI of single non-activated spermatozoa) exhibited embryonic development. The critical stage proved to be the blastopore formation in gastrula stage, suspected as the stage in which the embryonic genes are expressing in sturgeon (59). The inability of the embryos to surpass that stage is similar to the results after sturgeon SCNT (38, 39). Comparing the embryonic developmental success after ICSI of single fresh-stripped spermatozoa to SCNT using single fin-cells (38), we observed an 8.6- to 12.6-fold higher success of development (blastula stage). Success in human ICSI compared to human SCNT has been attributed to fewer acquired mutations in spermatozoa compared to somatic cells (5- to 10-fold) from the same individual (60). When comparing the ICSI using multiple spermatozoa (~4000/egg) with the SCNT using multiple fin-cells (~300/egg) (39), we observed the same or half percentage of developing blastula embryos. This may be attributed to the nearly 13-fold higher cells transplanted in the case of ICSI using multiple spermatozoa compared to the SCNT using multiple fin-cells.
In all egg-sperm species-combination groups the experiment of ICSI using single fresh-stripped non-activated spermatozoa produced normal-shaped feeding larvae. In human ICSI, it is common practice to use pre-activated spermatozoa (61). In the present study, the experiment of ICSI using single fresh-stripped pre-activated spermatozoa produced normal-shaped feeding larvae only in one group. On the contrary, Psenicka et al. (40) supported that activation of acrosomal reaction in sturgeon spermatozoa (activated spermatozoa) makes them more successful in in vitro fertilization and transplants' hatching rate. After ICSI of multiple fresh-stripped spermatozoa, all three egg-sperm species-combination groups were able to form the blastopore in gastrula stage while only one group produced larvae. In that group, all experiments of ICSI were successful (production of feeding larvae), with single spermatozoa transplantation (15.4 % in both experiments; pre-activated and non-activated) being much more successful than the multiple spermatozoa transplantation (1.6 %). On the contrary, after sturgeon SCNT the use of multiple fin cells proved to be more promising with high rates of embryonic development (39). This probably happened because injection of ~4000 spermatozoa compared to ~300 fin cells creates significant pressure for the recipient egg. The sturgeon egg must have a saturation point in its ability to digest such a large number of spermatozoa permitting embryonic development.
Ciereszko et al. (62) suggested that cryopreserved spermatozoa will become a standard practice in future reproduction in sturgeon fisheries. Indeed, fertility preservation strategies using cryopreservation have enormous potential for helping sustain and protect rare and endangered species. Gametes' cryopreservation could help to maintain the species genetic heterozygosity, while minimizing movement of living animals (63). However, cryopreservation of sturgeon spermatozoa affects their ability to fertilize, due to a decrease of sperm motility function and induction of acrosomal damage (64, 65). In this study, single non-activated cryopreserved beluga spermatozoa fertilized sterlet eggs in vitro (control group) and after ICSI showed embryonic development (blastula stage) of 51.5 and 22.7 %, respectively. Some embryos from the ICSI group were able to reach the neurula stage (4.1 %) and stopped development. Studies of Nile tilapia ICSI using cryopreserved spermatozoa produced embryos until blastula stage (20 %) (19). The authors suggested that the spermatozoa selected for injections were damaged, compromising further development, something that probably happened in our study. Ice crystal formation due to cryopreservation can disrupt the sperm membrane, resulting in loss of cytoplasmic contents that may contribute to egg activation. Ice crystals can also damage the chromatin, resulting in fragmented DNA. Injection of eggs with spermatozoa containing fragmented chromatin has resulted in failure of spermatozoa decondensation, fertilization, and embryonic development in humans (66, 67).
After the different experiments of sturgeon ICSI we did not observe any developmental malformation in the resulting embryos and larvae. Sturgeon species are proving very good model species and could be used extensively for embryonic experimental studies. Fail of teleost ICSI to produce normal-shaped embryos and larvae could be attributed to the difficulty to inject through the micropyle of eggs. Precise control of the injection site of spermatozoon to the egg seems to be a prerequisite for successful medaka ICSI (7). In the case of zebrafish ICSI, stress concentration was observed in the contact area between the injection micropipette and the zebrafish egg, which causes egg breakage resulting in embryonic malformation and death (68).
Molecular analysis revealed that most of the ICSI transplants exhibit the genome of both egg- and sperm-donor species. This result confirms that sturgeon ICSI can be used as an assisted reproductive technology for producing offspring of selective genitors. Application of ICSI among sturgeons could potentially benefit the breeding facilities for consuming purposes. On the other hand, we observed ICSI transplants with only egg-donor or only sperm-donor DNA. We have already observed transplants after SCNT using a single or multiple fin-cells that expressed only egg-donor's DNA due to unusual disruption in early embryogenesis (38, 39). In the case of only sperm-donor-derived ICSI transplants we believe that the egg during the microinjections was exposed to a massive stress. Rouillon et al. (69) found that after goldfish SCNT the production of only sperm-donor-derived offspring happened due to an alteration of the meiotic furrow and the egg-donor DNA not contributing in the development. It is possible that the same happened in the present study. In the future it will be useful to perform ploidy analysis of the resulted embryos and larvae.
Our study clearly demonstrates that the ICSI technology is feasible for sturgeon's reproduction, but further studies are needed to improve efficiency. The development of ICSI technology in sturgeon could yield valuable applications. The sturgeon caviar industry is quite demanding and sex pre-selection of offspring through the use of sexed spermatozoa has great potential, because the production of only female sturgeons can result in higher production of caviar. Furthermore, producing predominantly female offspring is advantageous in accelerating the repopulation rate, especially in species that are notorious for slow reproduction (70), like in the case of sturgeon. The positive results shown here demonstrate that ICSI could be used as another genetic tool for improvement in aquaculture. ICSI would be best suited to reconstitution of desired lines from selected genitors and therefore using a small brood stock to produce large numbers of sturgeon with preferable characteristics.
Data availability statement
The original contributions presented in the study are included in the article/Supplementary material, further inquiries can be directed to the corresponding author.
Ethics statement
The animal study was reviewed and approved by Animal Research Committee of the University of South Bohemia in Ceské Budějovice (Reference Number: 2293/2015-MZE-17214).
Author contributions
EF designed, conducted the ICSI experiments, analyzed data, and wrote the manuscript. MH performed and analyzed the molecular analysis. MP managed the laboratory and provided necessary suggestions on the text together with TS. JL performed the statistical analysis and data visualization. MR and DG handled the sturgeon spawning. All authors contributed to the preparation of the manuscript and approved the final version.
Funding
The work was supported by the Ministry of Education, Youth, and Sports of the Czech Republic–project CENAKVA (LM2018099), project Biodiversity (CZ.02.1.01/0.0/0.0/16_025/0007370), Czech Science Foundation project 22-31141J and the Spanish Ministry of Science and Innovation–Beatriz Galindo individual grant (BEAGAL 18/00172) to JL.
Acknowledgments
We would like to thank Dr. Ievgen Lebeda for his scientific input in the molecular analysis.
Conflict of interest
The authors declare that the research was conducted in the absence of any commercial or financial relationships that could be construed as a potential conflict of interest.
Publisher's note
All claims expressed in this article are solely those of the authors and do not necessarily represent those of their affiliated organizations, or those of the publisher, the editors and the reviewers. Any product that may be evaluated in this article, or claim that may be made by its manufacturer, is not guaranteed or endorsed by the publisher.
Supplementary material
The Supplementary Material for this article can be found online at: https://www.frontiersin.org/articles/10.3389/fvets.2022.1054345/full#supplementary-material
References
1. Uehara T, Yanagimachi R. Microsurgical injection of spermatozoa into hamster eggs with subsequent transformation of sperm nuclei into male pronuclei. Biol Reprod. (1976) 15:467–70. doi: 10.1095/biolreprod15.4.467
2. Goto K, Kinoshita A, Takuma Y, Ogawa K. Fertilisation of bovine oocytes by the injection of immobilised, killed spermatozoa. Vet Rec. (1990) 127:517–20.
3. Cochran R, Meintjes M, Reggio B, Hylan D, Carter J, Pinto C, et al. Live foals produced from sperm-injected oocytes derived from pregnant mares. J Equine Vet Sci. (1997) 18:736–40. doi: 10.1016/S0737-0806(98)80504-2
4. Kolbe T, Holtz W. Birth of a piglet derived from an oocyte fertilized by intracytoplasmic sperm injection (ICSI). Anim Reprod Sci. (2000) 64:97–101. doi: 10.1016/S0378-4320(00)00204-9
5. Catt SL, Gomez MC, Maxwell WMC, Evans G, Catt JW. Birth of a male lamb derived from an in vitro matured oocyte fertilised by intracytoplasmic injection of a single presumptive male sperm. Vet Rec. (1996) 139:494–95. doi: 10.1136/vr.139.20.494
6. Deng M, Yang X. Full term development of rabbit oocytes fertilized by intracytoplasmic sperm injection. Mol Reprod Dev. (2001) 59:38–43. doi: 10.1002/mrd.1005
7. Otani S, Iwai T, Nakahata S, Sakai C, Yamashita M. Artificial Fertilization by Intracytoplasmic Sperm Injection in a Teleost Fish, the Medaka (Oryzias latipes). Biol Reprod. (2009) 80:175–83. doi: 10.1095/biolreprod.108.069880
8. Parmar MS, Pant C, Karuppanasamy K, Mili B, Upadhyay D, Kant V, et al. Intracytiplasmic Sperm Injection (ICSI) and its applications in veterinary sciences: an overview. Sci Int. (2013) 8:266–70. doi: 10.17311/sciintl.2013.266.270
9. Tesarik J, Sousa M. More than 90% fertilization rates after intracytoplasmic sperm injection and artificial induction of oocyte activation with calcium ionophore. Fertil Steril. (1995) 63:343–9. doi: 10.1016/S0015-0282(16)57366-X
10. Sato M, Ochi T, Nakase T, Hirota S, Kitamura Y, Nomura S, et al. Mechanical tension-stress induces expression of bone morphogenetic proteins BMP-2 and BMP-4, but not BMP-6, BMP-Z and GDF-5 mRNA, during distraction osteogenesis. J Bone Miner Res. (1999) 14:1084–10. doi: 10.1359/jbmr.1999.14.7.1084
11. Markoulaki S, Kurokawa M, Yoon SY, Matson S, Ducibella T, Fissore R, et al. Comparison of Ca2+ and CaMKII responses in IVF and ICSI in the mouse. Mol Hum Reprod. (2007) 13:265–72. doi: 10.1093/molehr/gal121
12. Swann K. The role of Ca2+ in oocyte activation during in vitro fertilization: Insights into potential therapies for rescuing failed fertilization. Biochim Biophys Acta Mol Cell Res. (2018) 1865:1830–7. doi: 10.1016/j.bbamcr.2018.05.003
13. Caperton L, Murphey P, Yamazaki Y, McMahan CA, Walter CA, Yanagimachi R, et al. Assisted reproductive technologies do not alter mutation frequency or spectrum. PNAS. (2007) 104:5085–90. doi: 10.1073/pnas.0611642104
14. Palermo G, Joris H, Devroey P, Van Steirteghem A. Pregnancies after intracytoplasmatic injection of single spermatozoon into an oocyte. Lancet. (1992) 340:17–8. doi: 10.1016/0140-6736(92)92425-F
15. Palermo GD, O'Neill CL, Chow S, Cheung S, Parrella A, Pereira N, et al. Intracytoplasmic sperm injection: state of the art in humans. Reproduction. (2017) 154:F93–F110. doi: 10.1530/REP-17-0374
16. Salamone DF, Canel NG, Rodríguez MB. Intracytoplasmic sperm injection in domestic and wild mammals. Reproduction. (2017) 154:F111–24. doi: 10.1530/REP-17-0357
17. Sansinena MJ, Taylor SA, Taylor PJ, Schmidt EE, Denniston RS, Godke RA, et al. In vitro production of llama (Lama glama) embryos by intracytoplasmic sperm injection: Effect of chemical activation treatments and culture conditions. Anim Reprod Sci. (2007) 99:342–53. doi: 10.1016/j.anireprosci.2006.05.020
18. O'Brien JK, Crichton EG, Evans KM, Schenk JL, Stojanov T, Evans G, et al. Sex ratio modification using sperm sorting and assisted reproductive technology—a population management strategy. In ‘The 2nd International Symposium of Assisted Reproductive Technologies for the Conservation and Genetic Management of Wildlife, Omaha, Nebraska. Omaha, NE: Henry Doorly Zoo (2002). p. 224–31.
19. Poleo GA, Godke RA, Tiersch TR. Intracytoplasmic sperm injection using cryopreserved, fixed, and freeze-dried sperm in eggs of Nile tilapia. Mar Biotechnol. (2005) 7:104–11. doi: 10.1007/s10126-004-0162-5
20. Ringleb J, Waurich R, Wibbelt G, Streich W, Jewgenow K. Prolonged storage of epididymal spermatozoa does not affect their capacity to fertilise in vitro-matured domestic cat (Felis catus) oocytes when using ICSI. Reprod Fert Dev. (2011) 23:818–25. doi: 10.1071/RD10192
21. Choi YH, Varner DD, Love CC, Hartman DL, Hinrichs K. Production of live foals via intracytoplasmic injection of lyophilized sperm and sperm extract in the horse. Reproduction. (2011) 142:529–38. doi: 10.1530/REP-11-0145
22. Anzalone DA, Palazzese L, Iuso D, Martino G, Loi P. Freeze-dried spermatozoa: an alternative biobanking option for endangered species. Anim Reprod Sci. (2018) 190:85–93. doi: 10.1016/j.anireprosci.2018.01.010
23. Fernández-González L, Hribal R, Stagegaard J, Zahmel J, Jewgenow K. Production of lion (Panthera leo) blastocysts after in vitro maturation of oocytes and intracytoplasmic sperm injection. Theriogenology. (2015) 83:995–9. doi: 10.1016/j.theriogenology.2014.11.037
24. Kishikawa H, Tateno H, Yanagimachi R. Fertility of mouse spermatozoa retrieved from cadavers and maintained at 4 degrees C. J Reprod Fertil. (1999) 116:217–22. doi: 10.1530/jrf.0.1160217
25. Wildt DE, Howard JG, Hall LL, Bush M. The reproductive physiology of the clouded leopard. I Electroejaculates contain high proportions of pleiomorphic spermatozoa throughout the year. Biol Reprod. (1986) 34:937–47. doi: 10.1095/biolreprod34.5.937
26. Roth TL, Howard JG, Donoghue AM, Swanson WF, Wildt DE. Function and culture requirements of snow leopard (Panthera unciaI) spermatozoa in vitro. J Reprod Fertil. (1994) 101:563–9. doi: 10.1530/jrf.0.1010563
27. Lillie FR. Studies of fertilization. VI The mechanism of fertilization in arbacia. J Exp Zool. (1914) 16:523–90. doi: 10.1002/jez.1400160404
28. Hiramoto Y. Microinjection of the live spermatozoa into sea urchin eggs. Exp Cell Res. (1962) 27:416–26. doi: 10.1016/0014-4827(62)90006-X
29. Tesarik J. Fertilization of oocytes by injecting spermatozoa, spermatids and spermatocytes. Rev Reprod. (1996) 1:149–52. doi: 10.1530/ror.0.0010149
30. Kroll KL, Amaya E. Transgenic Xenopus embryos form sperm nuclear transplantations reveal FGF signaling requirements during gastrulation. Development. (1996) 122:3173–83. doi: 10.1242/dev.122.10.3173
31. Sparrow DB, Latinkic B, Mohun TJ. A simplified method of generating transgenic Xenopus. Nucleic Acids Res. (2000) 28:E12–E12. doi: 10.1093/nar/28.4.e12
32. Moro LN, Sestelo AJ, Salamone DF. Evaluation of cheetah and leopard spermatozoa developmental capability after interspecific ICSI with domestic cat oocytes. Reprod Domest Anim. (2014) 49:693–700. doi: 10.1111/rda.12355
33. Yamashita M, Onozato H, Nakanishi T, Nagahama Y. Breakdown of the sperm nuclear envelope is a prerequisite for male pronucleus formation: direct evidence from the gynogenetic crucian carp Carassius auratus langsdorfii. Dev Biol. (1990) 137:155–60. doi: 10.1016/0012-1606(90)90016-C
34. Poleo GA, Denniston RS, Reggio BC, Godke RA, Tiersch TR. Fertilization of eggs of zebrafish, Danio rerio, by intracytoplasmic sperm injection. Biol Reprod. (2001) 65:961–6. doi: 10.1095/biolreprod65.3.961
35. Yasui GS, Saito T, Zhao Y, Fujimoto T, Yamaha E, Arai K, et al. Intra-ooplasmic injection of a multiple number of sperm to induce androgenesis and polyploidy in the dojo loach Misgurnus anguillicaudatus (Teleostei: Cobitidae). Zygote. (2018) 26:408–16. doi: 10.1017/S0967199418000448
36. Liu T, Liu L, Wei Q, Hong T. Sperm nuclear transfer and transgenic production in the fish medaka. Int J Biol Sci. (2011) 7:469–75. doi: 10.7150/ijbs.7.469
37. The I. U. C. N. IUCN Red List of Threatened Species. Version 2022-1, (2022). Available online at: http://www.iucnredlist.org (accessed September 05, 2022).
38. Fatira E, Havelka M, Labbé C, Depincé A, Iegorova V, Pšenička M, et al. Application of interspecific Somatic Cell Nuclear Transfer (iSCNT) in sturgeons and an unexpectedly produced gynogenetic sterlet with homozygous quadruple haploid. Sci Rep. (2018) 8:5997. doi: 10.1038/s41598-018-24376-1
39. Fatira E, Havelka M, Labbé C, Depincé A, Pšenička M, Saito T, et al. A newly developed cloning technique in sturgeons; an important step towards recovering endangered species. Sci Rep. (2019) 9:10453. doi: 10.1038/s41598-019-46892-4
40. Psenicka M, Kaspar V, Alavi SMH, Rodina M, Gela D, Li P, et al. Potential role of the acrosome of sturgeon spermatozoa in the fertilization process. J Appl Ichthyol. (2011) 27:678–82. doi: 10.1111/j.1439-0426.2010.01642.x
41. Ginsburg AS, Dettlaff TA. “The Russian Sturgeon Acipenser gueldenstaedtii. Part I. gametes and early development up to time of hatching” In: Animal Species for Developmental Studies. editors, Dettlaff TA, Vassetzky G. Boston, MA: Springer (1991) p. 15–66.
42. Iegorova V, Pšenička M, Lebeda I, Rodina M, Saito T. Polyspermy produces viable haploid/diploid mosaics in sturgeon. Biol Reprod. (2018) 99:695–706. doi: 10.1093/biolre/ioy092
43. Glogowski J, Kolman R, Szczepkowski M, Horvath A, Urbanyi B, Sieczynski P, et al. Fertilization rate of Siberian sturgeon (Acipenser baeri, Brandt) milt cryopreserved with methanol. Aquaculture. (2002) 211:367–73. doi: 10.1016/S0044-8486(02)00003-0
44. Havelka M, Fujimoto T, Hagihara S, Adachi S, Arai K. Nuclear DNA markers for identification of Beluga and Sterlet sturgeons and their interspecific Bester hybrid. Sci Rep. (2017) 7:1694. doi: 10.1038/s41598-017-01768-3
45. Havelka M, Boscari E, Sergeev A, Mugue N, Congiu L, Arai K, et al. A new marker, isolated by ddRAD sequencing, detects Siberian and Russian sturgeon in hybrids. Anim Genet. (2018) 50:115–6. doi: 10.1111/age.12733
46. Börk K, Drauch A, Israel JA, Pedroia J, May B. Development of new microsatellite primers for green and white sturgeon. Conserv Genet. (2008) 9:973–9. doi: 10.1007/s10592-007-9417-9
47. Welsh AB, Blumberg M, May B. Identification of microsatellite loci in lake sturgeon, Acipenser fulvescens, and their variability in green sturgeon, A. medirostris. Mol Ecol Notes. (2003) 3:47–55. doi: 10.1046/j.1471-8286.2003.00346.x
48. King TL, Lubinski BA, Spidle AP. Microsatellite, DNA variation in Atlantic sturgeon (Acipenser oxyrinchus oxyrinchus) and cross-species amplification in the Acipenseridae. Conserv Genet. (2001) 2:103–19. doi: 10.1023/A:1011895429669
49. McQuown EC, Sloss BL, Sheehan RJ. Microsatellite analysis of genetic variation in Sturgeon: new primer sequences for Scaphirhynchus and Acipenser. Trans Am Fish Soc. (2000) 129:1380 doi: 10.1577/1548-8659
50. Havelka M, Hulák M, Bailie DA, Prodöhl PA, Flajšhans M. Extensive genome duplications in sturgeons: new evidence from microsatellite data. J Appl Ichthyol. (2013) 29:704–8. doi: 10.1111/jai.12224
51. Dettlaff TA, Ginsburg AS, Schmalhausen OI. Sturgeon Fishes: Developmental Biology and Aquaculture. New York, US: Springer-Verlag (1993). p. 300.
52. Tsekov A, Ivanova P, Angelov M, Atanasova S, Bloesch J. Natural sturgeon hybrids along bulgarian black sea coast and in danube river. Acta Zool Bulg. (2008) 60:311–6.
53. Havelka M, Kašpar V, Hulák M, Flajšhans M. Sturgeon genetics and cytogenetics: a review related to ploidy levels and interspecific hybridization. Folia Zool. (2011) 60:93–103. doi: 10.25225/fozo.v60.i2.a3.2011
54. Shen L, Shi Y, Zou YC, Zhou XH, Wei QW. Sturgeon aquaculture in China: status, challenge and proposals based on nation-wide surveys of 2010–2012. J Appl Ichthyol. (2014) 30:1547–51. doi: 10.1111/jai.12618
55. Beridze T, Boscari E, Scheele F, Edisherashvili T, Anderson C, Congiu L, et al. Interspecific hybridization in natural sturgeon populations of the Eastern Black Sea: the consequence of drastic population decline? Conserv. Genet. (2022) 23:211–6. doi: 10.1007/s10592-021-01413-7
56. Nagy ZP, Liu J, Joris H, Verheyen G, Tournaye H, Camus M, et al. The result of intracytoplasmic sperm injection is not related to any of the three basic sperm parameters. Hum Reprod. (1995) 10:1123–9. doi: 10.1093/oxfordjournals.humrep.a136104
57. Yanagimachi R. Intracytoplasmic injection of spermatozoa and spermatogenetic cells: its biology and applications in human and animals. Reprod Biomed Online. (2005) 10:247–88. doi: 10.1016/S1472-6483(10)60947-9
58. Brun RB. Studies on fertilization in Xenopus laevis. Biol Reprod. (1974) 11:513–8. doi: 10.1095/biolreprod11.5.513
59. Pocherniaieva K, Güralp H, Saito T, Pšenička M, Tichopád T, Janko, et al. The timing and characterization of maternal to zygote transitionand mid-blastula transition in sterlet acipenser ruthenus and A.ruthenus x Acipenser gueldenstaedtii Hybrid. Turk J Fish Aquat Sci. (2019) 19:167–74. doi: 10.4194/1303-2712-v19_2_09
60. The Practice Committee of the American Society for Reproductive Medicine. Endometriosis and infertility. Fertil Steril. (2004) 81:1441–6. doi: 10.1016/j.fertnstert.2004.01.019
61. Palermo G, Nagy Z. Manual of Intracytoplasmic Sperm Injection in Human Assisted Reproduction: With Other Advanced Micromanipulation Techniques to Edit the Genetic and Cytoplasmic Content of the Oocyte. Cambridge: Cambridge University Press (2021).
62. Ciereszko A, Glogowski J, Dabrowski K, Tiersch TR, Mazik PM. Cryopreservation of Aquatic Species. Baton Rouge, LA: World Aquaculture Society (2000). p. 20–48.
63. Johnston LA, Lacy RC. Genome resource banking for species conservation: selection of sperm donors. Cryobiology. (1995) 32:68–77. doi: 10.1006/cryo.1995.1006
64. Billard R, Cosson J, Noveiri SB, Pourkazemi M. Cryopreservation and short- term storage of sturgeon sperm, a review. Aquaculture. (2004) 236:1–9. doi: 10.1016/j.aquaculture.2003.10.029
65. Psenicka M, Hadi Alavi SM, Rodina M, Cicova Z, Gela D, Cosson J, et al. Morphology, chemical contents and physiology of chondrostean fish sperm: a comparative study between Siberian sturgeon (Acipenser baerii) and sterlet (Acipenser ruthenus). J Appl Ichthyol. (2008) 24:371–7. doi: 10.1111/j.1439-0426.2008.01139.x
66. Sakkas D, Urner F, Bianchi PG, Wagner I, Jaquenoud N, Manicardi G, et al. Sperm chromatin anomalies can influence decondensation after intracytoplasmic sperm injection. Hum Reprod. (1996) 11:837–43. doi: 10.1093/oxfordjournals.humrep.a019263
67. Dumoulin JCM, Coonen E, Bras M, Bergers-Janssen JM, Ignoul-Vanvuchelen RCM, van Wissen LCP, et al. Embryo development and chromosomal anomalies after ICSI: effect of the injection procedure. Hum Reprod. (2001) 16:306–12. doi: 10.1093/humrep/16.2.306
68. Hajiyavand AM, Saadat M, Abena A, Sadak F, Sun X. Effect of injection speed on oocyte deformation in ICSI. Micromachines. (2019) 10:226. doi: 10.3390/mi10040226
69. Rouillon C, Depincé A, Chênais N, Le Bail PY, Labbé C. Somatic cell nuclear transfer in non-enucleated goldfish oocytes: understanding DNA fate during meiosis resumption and first cellular division. Sci Rep. (2019) 9:12462. doi: 10.1038/s41598-019-48096-2
Keywords: assisted reproduction, intracytoplasmic sperm injection, sturgeon, embryo, larva
Citation: Fatira E, Havelka M, Saito T, Landeira J, Rodina M, Gela D and Pšenička M (2022) Intracytoplasmic sperm injection in sturgeon species: A promising reproductive technology of selected genitors. Front. Vet. Sci. 9:1054345. doi: 10.3389/fvets.2022.1054345
Received: 27 September 2022; Accepted: 05 December 2022;
Published: 23 December 2022.
Edited by:
Sebastián Demyda-Peyrás, Universidad Nacional de La Plata, ArgentinaReviewed by:
Daniel Marcelo Lombardo, University of Buenos Aires, ArgentinaMohammad Reza Kalbassi, Tarbiat Modares University, Iran
Copyright © 2022 Fatira, Havelka, Saito, Landeira, Rodina, Gela and Pšenička. This is an open-access article distributed under the terms of the Creative Commons Attribution License (CC BY). The use, distribution or reproduction in other forums is permitted, provided the original author(s) and the copyright owner(s) are credited and that the original publication in this journal is cited, in accordance with accepted academic practice. No use, distribution or reproduction is permitted which does not comply with these terms.
*Correspondence: Effrosyni Fatira, ZWZmcm9zaW5pLmZhdGlyYUBnbWFpbC5jb20=