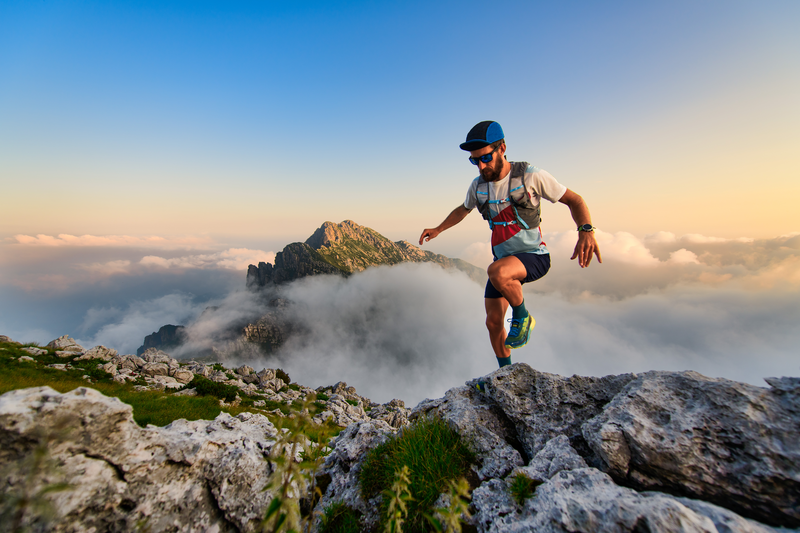
94% of researchers rate our articles as excellent or good
Learn more about the work of our research integrity team to safeguard the quality of each article we publish.
Find out more
REVIEW article
Front. Vet. Sci. , 06 January 2023
Sec. Veterinary clinical, anatomical, and comparative pathology
Volume 9 - 2022 | https://doi.org/10.3389/fvets.2022.1046636
Melanomas in humans and dogs are highly malignant and resistant to therapy. Since the first development of immunotherapies, interest in how the immune system interacts within the tumor microenvironment and plays a role in tumor development, progression, or remission has increased. Of major importance are tumor-infiltrating lymphocytes (TILs) where distribution and cell frequencies correlate with survival and therapeutic outcomes. Additionally, efforts have been made to identify subsets of TILs populations that can contribute to a tumor-promoting or tumor-inhibiting environment, such as the case with T regulatory cells versus CD8 T cells. Furthermore, cancerous cells have the capacity to express certain inhibitory checkpoint molecules, including CTLA-4, PD-L1, PD-L2, that can suppress the immune system, a property associated with poor prognosis, a high rate of recurrence, and metastasis. Comparative oncology brings insights to comprehend the mechanisms of tumorigenesis and immunotolerance in humans and dogs, contributing to the development of new therapeutic agents that can modulate the immune response against the tumor. Therapies that target signaling pathways such as mTOR and MEK/ERK that are upregulated in cancer, or immunotherapies with different approaches such as CAR-T cells engineered for specific tumor-associated antigens, DNA vaccines using human tyrosinase or CGSP-4 antigen, anti-PD-1 or -PD-L1 monoclonal antibodies that intercept their binding inhibiting the suppression of the T cells, and lymphokine-activated killer cells are already in development for treating canine tumors. This review provides concise and recent information about diagnosis, comparative mechanisms of tumor development and progression, and the current status of immunotherapies directed toward canine melanoma.
Melanoma is the one of the most aggressive and metastatic types of cancer in humans and dogs (1–4). In humans, melanoma accounts for 80% of skin cancers (1, 2). Early detection is a major factor in observed outcomes: for patients with an early diagnosis, the 5-year survival rate is 90%, while this rate decreases dramatically to 10% in patients diagnosed with advanced melanoma (5), where the median overall survival is less than a year (6). Additionally, melanoma is highly resistant to conventional therapies, and new cases are on the increase every year. Melanoma diagnoses have increased annually worldwide by 2.6% and more than 3% in countries like the US, UK, Sweden, and Norway (7, 8).
Chemotherapy after surgical excision was the standard treatment for many years, but overall survival remained low especially for the latest stages of melanoma (9). In the last decade, the understanding of the mechanisms and pathways leading to melanoma and the discovery of effective immunotherapies have shown great promise as more effective treatments for this neoplasm (3, 4, 10, 11). Mutations in the RAS and RAF family of oncogenes have been detected in a significant percentage (15 and 50%, respectively) of human melanoma patients (1, 12), leading to the development of BRAF and MEK inhibitors as potential therapeutic agents (5). Likewise, great progress has been made in the understanding of how the immune system communicates with tumor cells in melanoma and conversely how cancerous cells can modulate the immune response of the patient, establishing the rationale for the development of therapeutic monoclonal antibodies against immune checkpoint molecules (6). Currently, two classes of checkpoint molecules inhibitors have been approved by the FDA, monoclonal antibodies against Programed Cell Death Protein-1 (PD-1), PD-Ligand 1 (PD-L1), and against Cytotoxic T Lymphocyte Antigen-4 (CTLA-4) (13), and more are under development, including anti-PD-L2 monoclonal antibodies (14).
Similarly, canine melanoma is a very aggressive neoplasm, accounting for 3–8% of all neoplastic diseases in dogs and a median overall survival of 8–12 months after diagnosis (15, 16). The most common location for canine melanoma is the oral cavity, accounting for up to 35.8% of all malignant tumors at that site (3), with occurrence at lower frequencies in the skin, eye, and digits (15). In contrast to the human disease, virtually no driver mutations have been identified for canine melanomas (17–19), although investigators are continuing to probe the MAPK signaling pathway since key proteins in this cascade are up-regulated in melanoma (20). As yet, no effective treatments for canine melanoma have been developed that parallel the recent advances made in treating the human disease.
Considering that melanoma is one the most aggressive cancers in both humans and dogs, it is crucial to devise new treatments, especially for advanced cases where available options are of limited efficacy (21, 22). After three decades using conventional modalities, the discovery of immunotherapies is transforming how this disease can be managed (22). In this review, we will address important pathways in the pathogenesis of melanoma, along with the fundamentals of diagnosis, new immunotherapies that have been developed, and their efficacy for the treatment of malignant melanoma in humans. We endeavor to highlight the promise, as well as the pitfalls, of translating mechanistic insights and thus therapeutic opportunities between humans and dogs.
Melanomas arise from melanocytes, which have an embryologic origin in melanoblasts that are derived from the neuroectoderm of the neural crest (23). Melanoblasts migrate from the neural crest to the integumentary system and localize within the epidermis and hair follicles where they differentiate into melanocytes or remain as melanocyte stem cells (23, 24). Melanin production by melanocytes occurs in cytoplasmic organelles called melanosomes, via a reaction catalyzed by tyrosinase through a chain of conversions that transform tyrosine to DOPA and on to melanin. The resulting granules of melanin are then transferred from melanocytes to keratinocytes by a membrane vesicle-mediated process (25, 26). This is the usual process for pigmentation of dermal keratinocytes by melanocytes. An alternative pathway provides for production of melanocytes from a bipotential precursor cell (Schwann/melanoblast) that migrates along nerve sheaths (24, 27). This process is regulated in part by microphthalmia-associated transcription factor (MITF) and the receptor tyrosine kinase KIT and its ligand (15, 27, 28).
The diagnosis of melanomas can be challenging, as they can resemble other types of tumors such as carcinomas, sarcomas, lymphomas, or tumors of an osteogenic origin (29). In addition, even though most melanomas have very characteristic melanin granules in their cytoplasm, they can also be amelanotic (Figures 1A, B). In these instances, the recognition of morphologic features described by Smedley et al. and the use of immunohistochemical (IHC) markers are very useful tools (30, 31). In addition to their intracytoplasmic melanin granules, melanomas can feature varied intratumor cell morphology, the presence of neoplastic cells at the epidermal-dermal or mucosal-submucosal junction, finely stippled to vesiculated nuclei, and often a single central and prominent nucleolus (31). Several histological morphologies have been described for melanoma: epithelioid (Figure 1C) with polygonal melanocytes arranged in cords or nests is the most frequently diagnosed; next, fusiform (Figure 1D) with spindloid cells arranged in streaming bundles; mixed with a combination of both polygonal and spindloid morphologies; small-round with cells arranged in cords; and, in cases of more undifferentiated tumors, melanomas can exhibit a neuron-like appearance (15, 31–34). In less frequency, cellular, balloon cell, signet ring, and clear cell morphologies have been reported (31).
Figure 1. Common canine melanoma morphologies. The panel shows the more classical melanoma morphologies. (A) Dog oral melanoma, 20X. Amelanotic, cords of polygonal cells with no apparent intracytoplasmic melanin granules. Neoplastic cells have a large nucleus with a prominent nucleolus. (B) Dog oral melanoma 20X. Amelanotic melanoma immunolabeled for Melan-A detected in the cytoplasm. (C) Dog oral melanoma 20X. Epithelioid melanoma with polygonal cells arranged in cords and nests, with scattered intracytoplasmic melanin granules. (D) Dog cutaneous melanoma 20X. Fusiform melanoma, elongated to spindloid cells arranged in streaming bundles, with occasional intracytoplasmic melanin granules (inset 4.a-d, 40X).
As melanoma can resemble multiple tumor types, IHC has become a valuable diagnostic tool. Whereas, SOX10, S100, HMB-45 and Melan A are normally used as markers of human melanoma, (35, 36) common markers used to identify canine melanomas are Melan-A, PNL2, and tyrosinase related protein 1 and 2 (TRP-1 and TRP-2) (29, 30). Melan-A (Figure 1B) is a protein marker specific for melanocyte differentiation, and its immunolabeling is highly sensitive and specific (29, 37). PNL2 is a monoclonal antibody that recognizes an as-yet unidentified cytoplasmic melanocytic antigen and is described as exhibiting staining specificity similar to that of anti-Melan-A (29). Tyrosinase, the third most-common marker, is a cytoplasmic protein Involved in melanocyte differentiation and melanin biosynthesis (29). It has been reported that immunolabeling for these markers is reduced in tumors with spindloid or undifferentiated morphology (29). These three markers combined can reach a sensitivity of 100% (29, 30). Other markers such as S100 have been suggested in the past for humans and dogs, with good results in canine melanoma cell lines (38), but its specificity is considered low by other investigators (29, 30), similar to results for vimentin (37). HMB-45 (Human Melanoma Black) has also been reported in canine melanoma with high variability among the few reports available, and its use is not recommended in canine neoplasms (31).
Another antigen, more recently recognized, is chondroitin sulfate proteoglycan-4 (CSPG4), also known as high molecular weight-melanoma associated antigen (HMW-MAA), an early cell-surface progression marker associated with proliferation, migration, and invasion. This maker, first described in human melanoma but lately reported in canine melanoma, has promising potential therapeutic use as the basis for a DNA vaccine (39–42), and has been reported to be expressed more frequently in amelanotic melanomas (40).
Although originating most frequently at oral, cutaneous, digital, subungual, or ocular sites (Figure 2), melanomas can also occur in the gastrointestinal tract, nervous system, and muco-cutaneous junctions (33). Traditionally, oral melanoma has been most closely associated with malignancy, whereas the cutaneous counterpart typically has a relatively benign course of disease, and digit or subungual with increased rate of recurrence. Ocular melanoma has been described to be locally aggressive, but with limited metastatic potential (15, 18, 31, 33, 34, 43, 44). However, it must be emphasized that location alone should not be used to determine prognosis (44).
Figure 2. The panel shows the different locations of canine melanoma. (A) Oral melanoma (10X). Small nests of neoplastic melanocytes infiltrate the mucosal epithelium, and large sheets of neoplastic cells obscure the propria mucosa. Brown intracytoplasmic granules consistent with melanin are throughout the neoplasm. (B) Cutaneous melanoma (10X), haired skin. Large nests of neoplastic melanocytes infiltrate the dermis. Numerous melanomacrophages infiltrate the periphery of the neoplasm. (C) Ocular melanoma (montage of scan at 10X). A large, multilobulated neoplasm extends from and occupies the back of the eye. (D) Digital melanoma (10X). Sheets of neoplastic melanocytes obscure the dermis and infiltrate the bone.
The main features used for prognostic determination were nuclear atypia, growth fraction, and mitotic index, as reported by Smedley et al. and supported by the most recent melanoma consensus, revised in 2020 by the Veterinary Cancer Society/American College Veterinary Pathology Oncology-Pathology Working Group (44). Nuclear atypia was found to be highly correlated with outcome, especially for pigmented melanomas with epithelioid morphology. The authors suggest a threshold of >30% of cells with atypical nuclei from a total of 200 cells observed as indictive of a poor prognosis for oral and lip neoplasms and >20% of cells with atypical nuclei for cutaneous neoplasms (31).
Growth fraction (or Ki67 index) is measured by using IHC for the Ki67 protein (Ki67p). Detection of Ki67p is indicative of mitotic activity of intrinsic cell populations, as it is only present in actively proliferating cells (i.e., in G1-M phases of the cell cycle but not G0) and is commonly used as a marker for aggressiveness of the neoplasms (45). For oral melanomas, Ki67p is determined by calculating the average number of positively labeled melanocytes over a total of five areas of 1 mm2 optical grid at 400x magnification. In cutaneous melanomas, the Ki67 index is determined by estimating the percentage of positive labeled melanocytes per 500 counted cells. Ki67p is significantly different for benign and malignant melanocytic neoplasms, with increased numbers of immunolabeled cells found in oral melanomas and associated with a poor prognosis and reduced survival (31),
Regarding the mitotic index, briefly, it is well-established that oral and lip melanocytic neoplasms with >4 mitoses per 2.37 mm2 observed microscopic field have an increased risk of death within 1 year of diagnosis, and, for cutaneous and digital melanocytic neoplasms, >3 mitoses in 2.37 mm2 are statistically correlated with low 2-year survival rate (31). In addition, the VCS/ACVP consensus group has suggested addition of tumor thickness to the prognosis indicators for cutaneous melanomas (44). A tumor thickness of >0.95 cm is associated with poor prognosis and increased risk of recurrence and developing metastasis (46). The authors also reported that tumor thickness is greater in melanoma than melanocytoma.
Melanoma is considered one of the cancers with the highest degree of mutations (47, 48), and four major subtypes based on the genetic mutation burden have been described in humans. The first three groups encompass activating driver mutations in B-Raf proto-oncogene serine/threonine kinase (BRAF), the GDP/GTP binding protein RAS (RAS), and the tumor suppressor Neurofibromatosis Factor 1 (NF1), respectively, while the fourth group (“Triple Wild Type”) lacks any of these three alterations, although it may have a low frequency of other mutations such as those observed in KIT (12). The detection of these of mutations in patients informs the therapeutic plan and gives insight into their prognosis. Some patients with mutated BRAF could benefit from targeted therapies such as BRAF and MEK inhibitors, and, while these inhibitors might not be effective for some other patients, they could still benefit from other approaches including immunotherapies (22, 49).
The BRAF mutation is a somatic missense point mutation that results in a single substitution of glutamic acid for valine at amino acid 600 (V600E) in human BRAF, conferring a constitutively active state to the BRAF protein kinase. This translates into a continuous state of survival of the cell, increasing proliferation and promotion of metastasis (50). The BRAF mutation has been described in 40–50% of all human melanoma cases (34, 47, 49) and is more commonly seen in younger patients (12). Additionally, in patient with nevi that have the BRAF mutation, a second hit could induce malignancy by an inactivation mutation in the phosphatase and tensin protein (PTEN), since this protein has a role as a negative regulator of cell growth and survival signaling pathways (47, 51).
Mutation of RAS is the second most common genetic alteration in human melanoma, present in 15–25% of all cases (47, 49). This mutation alters the RAS protein and maintains protein activation, which continuously stimulates the mitogen/extracellular signal-regulated kinase (MEK/ERK) pathway and in some cases can also lead to the activation of the Phosphatidylinositol-3-kinase (PI3K) signaling pathway, driving cell survival and proliferation (49). Mutation in Neurofibromatosis Factor 1 gene (NF1) is the third most common mutation in melanoma and induces loss of the regulatory effect of NF1 on the RAS protein (48, 49). Consequently, RAS is continuously activated, and the cell enters into a proliferative state. This mutation is more frequently detected in older patients and is associated with better response to checkpoint molecule blockade therapies (12) as described below.
The fourth group is the Triple Wild Type, lacking BRAF, RAS and NF1 mutations. Even though melanomas in this group do not have any of the three mutations described above, they can have other mutations like GNAQ (G signaling protein) and c-KIT (CD117, a tyrosine kinase receptor for stem cell growth factor) in a lower frequency (< 7%); these proteins are associated with intracellular signaling and can result in uncontrolled cell proliferation (12, 52). Triple Wild Type melanomas are mostly from non-cutaneous origin, such as mucosal melanoma and, while less common, are very aggressive and associated with a poor prognosis (34, 48, 53). Other mutations found in human mucosal melanomas include activating mutations in SF3B1, loss of CDKN2A, PTEN, and SPRED1 (54). PTEN mutation has been reported in canine mucosal melanoma, although its role in disease severity or progression has not been established (55).
Activation of the signaling pathway mTOR (mammalian target of rapamycin), a serine/threonine kinase, has been strongly associated with the development of different neoplasms, including melanoma (34, 56–58). This complex is activated by the PI3K/AKT pathway and regulates cell growth. Its overexpression can lead to aberrant proliferation and increased migration, conferring on the neoplastic cells more invasive capabilities (59–62). There are two main components in this complex: mTORC1, which regulates numerous processes of protein synthesis that promote cell growth and can also inhibit certain catabolic process such autophagy contributing to the malignancy of the neoplasm (60, 63, 64); and mTORC2, associated with control of cellular structure, regulation of the cytoskeleton, and cell survival (60, 61, 63).
Another factor that has been reported commonly in cancer in multiple species is the variability of Somatically-acquired Copy Number Alterations (SCNAs), which consist of deletion or amplification of DNA fragments encoding regulators of cell proliferation (65). Recent studies report similar increases in SCNAs in human and canine mucosal melanoma (55, 66). In canine mucosal melanoma, increased aberrations by gain of chromosome 13 and 17, and loss of chromosome 2 and 22 were reported using comparative genomic hybridization in situ, and copy number assessments using fluorescence in situ hybridization revealed gain of c-MYC and loss of CDKN2A, whereas in humans there was gain of 1q, 6p, 8q, 7, and loss of 6q and 10 (66, 67).
The comparative oncology approach of identifying a mutation burden in canine melanoma similar to that in human disease has met with only limited success, as canine melanomas do not appear to have any of the mutations described above in high frequency. Although the BRAF mutation was found in 3 out of 54 canine melanomas studied (68), it is far less common than reported in the human disease, where it occurs in 40–50% of cases (17–19, 69). Additionally, NRAS and NF-1 mutations in canine melanoma have also been reported, but at a very low frequency (70). Although no predominant mutation has been described for canine melanoma to date, the search continues for a driver mutation that could be involved in the development of melanoma in dogs (20), while other investigators have proposed the use of the dog as an animal model to study Triple Wild Type and mucosal melanoma in humans (18, 32, 34). This parallel approach could bring new perspectives regarding risk factors and experimental paradigms from which both species will benefit. For instance, activation of the mTOR signaling pathway has been also explored in canine melanoma as well as in the human disease, and studies in canine melanoma cell lines have demonstrated activation of this pathway along with the inhibitory efficacy of Rapamycin and other drugs (71–73). Clearly there is much to be learned about the genetic drivers of melanoma in dogs.
Melanoma is considered an immunogenic tumor, and in most of the cases the tumors have a high degree of lymphocytic infiltration. However, most melanomas continue to grow, suggesting that tumor infiltrating immune cells fail to control and modulate tumor invasion (16, 74, 75). Furthermore, the tumor cells may suppress tumor infiltrating lymphocytes (TILs) and thereby escape immune surveillance. Nevertheless, the presence of TILs is associated with better responses to targeted and immune-directed therapies (76–78). Additionally, it has been demonstrated that the presence of TILs typically correlates with a favorable prognosis in melanoma (79–84) and other human cancers (85–88).
In view of this association, a system to characterize TILs infiltration in human melanoma was established by Clark (83) and has been adapted by other investigators for application to canine melanoma and other neoplasias. Briefly, the TIL distribution is classified as absent, brisk, or non-brisk. The “absent” category is described as no lymphocytes directly opposed to the tumor cells; the “non-brisk” category as isolated, focal and/or segmental infiltration in the tumor; and the “brisk” category as TILs segmentally infiltrating either at the entire base of the tumor or diffusely infiltrating within the tumor (83). Focal TILs that are in areas of fibrosis or remain in perivascular spaces but do not penetrate the tumor are also considered under the absent sub-classification (81). Other studies propose a combined system that considers the distribution and density of these TILs (89). These authors also reported that dense TIL infiltration with a brisk distribution was strongly associated with better prognosis in contrast to the non-brisk and absent distribution (83).
TILs comprise a heterogenous population of different lymphocytes bearing the co-receptors for the T cell receptor CD4 or CD8, including CD4 T regulatory cells (Tregs), CD4 T helper cells, CD4 memory cells, and CD8 effector cells (90). A majority of studies that aim to classify the subset of TILs in the tumor microenvironment use IHC markers for surface proteins, such as CD20 and CD79a for B cells, CD3 for T cells, CD4 and CD8 for T cells, and FOXP3 for regulatory T cells (91, 92).
Human melanoma patients with increased TIL abundance in the primary tumor have improved survival times compared to those with low TIL infiltration (79), better progression-free survival (84), and longer disease-free survival (89), while low TIL infiltration is associated with tumor recurrence and distant metastasis (87, 89). However, other investigators suggest that TIL infiltration alone is not predictive of outcome for non-small cell lung cancer and suggest that the subset of these TILs is more important (86), as also described in patients with metastatic colorectal cancers (87). These authors emphasize the importance of characterizing TILs and report that high numbers of CD8 T cells expressing cytolytic enzymes and CD4 T cells lacking inhibitory receptors were associated with a good prognosis when compared with TILs expressing PD-1 (86). Interestingly, the association between high Ki67 expression levels and high TILs levels has been described in triple-negative breast cancer, suggesting that the antitumor immune response is increased in aggressive tumors (93). Based on the findings described above, profiling of TILs in the tumor microenvironment provides a useful framework for understanding the contribution of different subsets of lymphocytes to formation of a tumor-promoting or tumor-inhibiting environment, associated with CD8 T cells or T regulatory cells, respectively.
Several studies in canine tumors have reported a relationship similar to that described above in human melanoma, associating density of TILs positive for the T cell co-receptor CD3 with favorable outcome in histiocytic sarcoma following resection (94). Another study in canine gliomas determined the nature of the infiltration, differentiating between CD3 T cells and those positive for the Forkhead box protein P3 (FOXP3), a regulator of Treg cell gene expression, finding similar results with generally low numbers of Treg cells, although higher FOXP3 densities were found in high grade tumors (95). Higher levels of Treg cells in peripheral blood and tumor-draining lymph nodes have been reported in dogs with melanomas when compared to healthy controls (96), and has been linked to an increased risk of death (97, 98). As explained in another study, subsets of TILs have an important role on tumor progression and outcomes, as CD8 T cells are key determinants of antitumor immunity, and CD4 T cells can either support this immune response or suppress it, as the case for Treg cells (99). These authors evaluated TILs in canine oral melanoma, taking into consideration both TIL density and distribution using the classification proposed by Clark et al. (described above), reporting that higher CD4 and CD8 TIL levels were found in less aggressive stages and also among primary tumors when compared to recurrent tumors. Additionally, patients with a brisk and non-brisk CD8 T cell infiltration had higher overall survival rates when compared to those with absent infiltration, and no impact on survival was appreciated from variable T reg and CD4 T cell infiltration (99).
Thus, the relationship between TILs and cancerous cells in the tumor microenvironment is an important consideration for the development of therapies targeting the regulatory molecules of the immune system. Tumor infiltration is not limited to tumor growth suppression, since it can also be associated with tumor growth and immunosuppression (100). Importantly, it has been reported that TILs are associated with better responses to targeted and immunotherapies in humans (76–78), especially for melanoma (101).
In patients with cancer, lymphocytes can experience chronic exhaustion and express PD-1 and CTLA-4 co-inhibitory receptors. At the same time, cancerous cells can overexpress PD-1 ligand (PD-L1) and CTLA-4 and thus inhibit an antitumor response by the immune system. PD-L1, PD-L2 and CTLA-4 are highly expressed across different cancer types (102, 103). Cancer cells can also recruit immune-suppressor cells like T regulatory lymphocytes, myeloid derived suppressor cells (MDSC), and type 2 macrophages, releasing immunosuppressive cytokines like IL-10, thereby allowing for tumor growth and proliferation, as has been described in several studies (100, 104–106).
Interestingly, expression of PD-1's alternative ligand PD-L2 has been detected in several human tumors, and some studies have reported an overexpression of PD-L2 in absence of PD-L1 (14). Other studies have found PD-L2 to be highly correlated with PD-1 and PD-L1 expression (103, 105) or with cancer infiltration and metastasis (105, 107). The degree to which tumor-expressed PD-L2 can substitute for PD-L1 as an immunosuppressing agent is not well-established, but an association between resistance to anti-PD-L1 immunotherapies and PD-L2 overexpression suggests an important role for PD-L2 in immune escape (108).
After decades of conventional treatment for advanced melanoma with limited success, surgical excision, chemotherapy and radiation are now being complemented with immunotherapies (109). After the discovery of the roles of checkpoint molecules such as PD-1, its ligand PD-L1, and CTLA-4, efforts have been redirected toward developing antibodies to inhibit their activity and release the patient's own immune system to fight cancer (22, 109).
In humans, patients with BRAF mutations are candidates for targeted therapies such as BRAF and MEK inhibitors. These therapies have been shown to inhibit tumor growth by decreasing the kinase activity in the MEK/ERK signaling pathway (49, 110, 111). Clinical trials have demonstrated that combined therapies are more effective than MEK or BRAF inhibition alone, with higher response rate and longer progression free survival (49). Vemurafenid and Dabrafenid or Trametinib, BRAF-mutant inhibitors approved by the FDA for the treatment of BRAF-V600-mutated-melanoma, have reported remission in 90% of patients (22). However, the clinical benefit is limited by the development of therapy resistance due to mRNA splice variants of BRAF and re-activation of alternate signaling pathways (22, 112, 113). There are several clinical trials using MEK and BRAF inhibitors in combination with immunotherapies that show improving response rates and duration of results, especially for patients unresponsive to BRAF/MEK inhibitor therapy; response rates up to 70% are reported (111, 112, 114).
Another strategy studied for the treatment of melanoma is the activation of anti-cancer immunity, by either stimulating dendritic cells or amplifying T cell activation. For several years, attempts were made using vaccines containing melanoma antigens without success due to the immunostatic effect of the tumor microenvironment or the poor immunogenicity of the antigens, inducing either high toxicity or insufficient responses (22, 109). Similar results were seen with agonistic antibodies for dendritic cells and cytotoxic lymphocytes (OX40 and CX3CL, respectively) (109). However, studies measuring the expression of OX40 in TILs have detected an upregulation of OX40 on T regulatory lymphocytes (115), and anti-OX40 antibodies have been proposed as adjuvants alongside immunotherapeutics (116).
Nowadays, immunotherapy research in multiple species is focused on the development of checkpoint molecule inhibitors and their efficacy in different types of cancers, and dramatic advances have been made in treatment of human disease. A variety of antibodies inhibiting the suppressive effect that cancer cells have over TILs have been developed during the last decades. Immunotherapies that have shown good promise are mononuclear antibodies against CTLA-4, PD-1 and PD-L1 (22, 117–120). Antibodies against checkpoint molecules approved by the FDA in the treatment of metastatic melanoma are Ipilimumab (anti CTLA-4), Nivolumab and Pembrolizumab (anti PD-1) (22). In addition, an anti PD-L1 antibody (Atezolizumab) has been approved for urothelial carcinoma and non-small cell lung cancer (NSCLC) (121). These drugs have an advantage over therapies that target tumors driven by BRAF, thus making them available for patients with other types of mutations or lacking the most frequent mutations. An advantage of the newly-developed Atezolizumab is that, in addition to binding free PD-L1, it also displaces the ligand from PD-1 and appears to be more effective than other anti-PD-L1 antibody drugs currently in clinical trials (121, 122). Additionally, immunotherapies targeting PD-1 and/or PD-L1 have shown fewer side effects than those targeting CTLA-4 (123). A meta-analysis of 19,217 human patients on immune checkpoint inhibitors showed toxicity-related death rates < 0.4% for anti-PD-1 and anti-PD-L1, 1.08% for anti CTLA-4 and 1.23% for combined therapies (124).
Exploration of the utility of immunotherapies in canine cancer therapy is in its very early days, with much of the published work having been conducted in vitro. For example, combined treatment with trametinib and sapanisertib in order to inhibit both MEK and mTOR pathways (Figure 3A) has been reported to induce apoptosis and reduce cell survival in canine melanoma cell lines (72), and one study reported that inhibition of the mTOR pathway reduced invasion and angiogenesis in hemangiosarcoma cell lines (125). However, combining these drugs with immunotherapies or targeting both pathways resulted in toxicities in studies done in cell lines and xenografts (72, 73).
Figure 3. Summary of small molecule- and antibody-based approaches to melanoma treatment. (A) Targeted therapies that aim to suppress central mechanisms of cellular proliferation and metastasis by inhibition of the mTOR (Sapanisertib), and MERK/ERK (Vemurafenid, Trametinib) pathways. The mTOR pathway activates gene expression that leads to cytoskeletal rearrangement, migration, and inhibition of autophagy. The RAS/BRAF/MEK/ERK pathway activation leads to increased protein synthesis, and promotes cell proliferation and survival. (B) Immunotherapies described to date in canine tumors. (a) Immunogens encoded by DNA vaccines, including tyrosinase (Oncept) or CSPG4, are first recognized by dendritic cells (DC) which later present antigen fragments to T cells at the lymph node; these T cell are able to target the antigen at the tumor microenvironment; (b) CAR-T cells engineered for specific tumor-associated antigens (TAAs) and cloned in vitro before being reincorporated into the patient; (c) Monoclonal antibodies recognizing PD-L1 blocking its binding with PD-1 receptor; and (d) Lymphokine-activated killer cells activated in vitro with IL-2. Created with BioRender.com.
Availability of immunotherapeutic agents is limited in the treatment of canine cancers. The less comprehensive characterization and understanding of the immune response to tumors in this species is a major obstacle, due in part to limited reagent availability (11). However, recent studies have explored vaccines, adoptive cell transfer, and anti PD-1 and PD-L1 therapy (21, 126, 127). Currently, Oncept, a xenogenic human tyrosinase DNA vaccine, is the sole vaccine approved by the USDA for the treatment of canine melanoma (Figure 3B) (21, 128, 129). Although one study reported improvements in survival times (130), it has been established that the vaccine alone is not enough to maintain anti-tumor efficacy, since the tumor microenvironment can suppress the immune response, and, once lymphocytes have been activated, they can express immunosuppressive checkpoint molecules (11, 21, 128, 129). Since the discovery of CSPG4 antigen in canine melanomas, efforts have been made to develop a DNA vaccine targeting this tumor associated antigen (Figure 3B), seeking to induce a safe immune response with longer overall disease-free survival when used as an adjuvant with other therapies and surgery (39–42, 131).
Additionally, adoptive cell transfer has been explored for the treatment in canine cancers, using lymphokine-activated killer (LAK) cells and CAR-T cells. These therapies have been evaluated for canine osteosarcoma (in vitro), B cell lymphoma, and melanoma (in vitro and in vivo), (11, 100, 128, 132) resulting in high toxicity for osteosarcoma (100) but modest antitumor activity for B cell lymphoma and melanoma (132, 133). Although LAK therapy demonstrated an immune-enhancing effect, it is not sufficient as monotherapy and thus should be evaluated as an adjuvant for other immunotherapies (128, 132). Similarly, CAR-T cells present several disadvantages, such the high cost and time required to engineer the cells, the risk of mutations in the receptor, antigen selection for the engineering of the receptor, and the fact that CAR-T cells are transient and not sustainable over long periods of time (100, 129).
Increased levels of CTLA-4 have also been reported in canine melanocytic tumors and associated with an immune-suppressed tumor microenvironment leading to immune escape and worsened prognosis (98). Great efforts have been made toward the development of a canine anti-CTLA4 monoclonal antibody, with promising results in mouse models (134). Another group has developed a chimeric heavy-chain antibody targeting cells expressing CTLA-4, such as Tregs and CD4 helper T cells, and reported an induction of the cytokine IFN-γ, whose pleiotropic actions include anti-tumor activity (135).
Lastly, one group has made great progress in the detection of overexpression of PD-1 from TILs and PD-L1 from malignant tumors in several canine cancers, such melanoma, osteosarcoma, hemangiosarcoma, and others, in the interest of establishing a rationale for application of anti-PD therapeutics in dogs (136, 137). Anti PD-1 chimeric antibody has been developed by a research group which reported reduction of tumor burden, objective partial response in 26% of the treated dogs with a Stage IV oral malignant melanoma, and a trend of increase of survival times, with maintained complete regression for more than 1 year in 2 cases (127, 138). In a pilot clinical study, these investigators demonstrated that anti-PD-L1 therapy can induce an objective antitumor response for oral melanoma (126). Furthermore, the expression of these checkpoint molecules has also been reported by our laboratory as increased in melanoma when compared with its benign counterpart melanocytoma and is associated with the density of CD3+ TILs (139). Recently, a group tested the cross-reactivity of different FDA-approved human immune checkpoint inhibitors (ICI) in canine tissue, showing two anti PD-L1 (Atezolizumab and Avelumab) with cross-reactivity, and Atezolizumab with the most robust T-cell cytokine production in vitro (140). A schematic representation of the immunotherapies in canine cancers is shown in Figure 3B. These studies establish the basis for future research on the development of checkpoint molecule inhibitors as a treatment for melanoma and other cancers in dogs, and it is expected that in the future, these therapies could be an effective and affordable option for the veterinary practice.
Comparative oncology between humans and dogs will set the foundations for a better understanding of common factors that are associated with the development of melanoma in humans. Additionally, it will provide insight into which factors are associated with the development of melanoma in dogs, and by what mechanism. As dogs spontaneously develop melanoma, are exposed to the same environmental hazards as humans, and have similar physiology to humans, they are excellent candidates for future development of immunotherapies. The evident lack of effective therapies for melanoma in dogs increases the urgency of the search for an effective treatment. Human benefit from immunotherapies has been documented in numerous studies, and it would be remarkable to continue exploring this option for treating dog cancers.
Lastly, study of the frequencies of occurrence, the spatial and temporal distribution, and functional categorization of TILs has great therapeutic potential. As new insights are gathered from recent studies, TILs will provide a representation of patients' immune system and the tumor microenvironment, offering a promising tool for determining therapeutic approaches.
VS conducted the literature search and drafted and edited the manuscript. SK provided guidance on clinical manifestations of canine melanoma. TL provided guidance on diagnostics. WH provided guidance on molecular mechanisms. SK, TL, and WH contributed to editing of the manuscript. All authors contributed to the article and approved the submitted version.
Funding for this work was provided by the Research and Graduate Studies Office of the Virginia-Maryland College of Veterinary Medicine.
The authors acknowledge with gratitude Kristobal Gudenschwager for his guidance with the images.
The authors declare that the research was conducted in the absence of any commercial or financial relationships that could be construed as a potential conflict of interest.
All claims expressed in this article are solely those of the authors and do not necessarily represent those of their affiliated organizations, or those of the publisher, the editors and the reviewers. Any product that may be evaluated in this article, or claim that may be made by its manufacturer, is not guaranteed or endorsed by the publisher.
1. Gorantla VC, Kirkwood JM. State of melanoma: an historic overview of a field in transition. Hematol Oncol Clin North Am. (2014) 28:415–35. doi: 10.1016/j.hoc.2014.02.010
2. Howlader N, Noone AM, Krapcho M, Miller D, Brest A, Yu M, et al. SEER Cancer Statistics Review, 1975-2018. Bethesda, MD: National Cancer Institute. Available online at: https://seer.cancer.gov/csr/1975_2018/
3. Kim WS, Vinayak A, Powers B. Comparative review of malignant melanoma and histologically well-differentiated melanocytic neoplasm in the oral cavity of dogs. Vet Sci. (2021) 8:261. doi: 10.3390/vetsci8110261
4. Von Rueden SK, Fan TM. Cancer-immunity cycle and therapeutic interventions- opportunities for including pet dogs with cancer. Front Oncol. (2021) 11:3420. doi: 10.3389/fonc.2021.773420
5. Hepner A, Salgues A, Anjos CAD, Sahade M, Camargo VP, Garicochea B, et al. Treatment of advanced melanoma—A changing landscape. Rev Assoc Med Bras. (1992) (2017) 63:814–23. doi: 10.1590/1806-9282.63.09.814
6. Mashima E, Inoue A, Sakuragi Y, Yamaguchi T, Sasaki N, Hara Y, et al. Nivolumab in the treatment of malignant melanoma: review of the literature. Onco Targets Ther. (2015) 8:2045–51. doi: 10.2147/OTT.S62102
7. Apalla Z, Lallas A, Sotiriou E, Lazaridou E, Ioannides D. Epidemiological trends in skin cancer. Dermatol Pract Concept. (2017) 7:1–6. doi: 10.5826/dpc.0702a01
8. Mattia G, Puglisi R, Ascione B, Malorni W, Care A, Matarrese P. Cell death-based treatments of melanoma:conventional treatments and new therapeutic strategies. Cell Death Dis. (2018) 9:112. doi: 10.1038/s41419-017-0059-7
9. Rajkumar S, Watson IR. Molecular characterisation of cutaneous melanoma: creating a framework for targeted and immune therapies. Br J Cancer. (2016) 115:145–55. doi: 10.1038/bjc.2016.195
10. Klingemann H. Immunotherapy for dogs: still running behind humans. Front Immunol. (2021) 12:665784. doi: 10.3389/fimmu.2021.665784
11. Klingemann H. Immunotherapy for dogs: running behind humans. Front Immunol. (2018) 9:133. doi: 10.3389/fimmu.2018.00133
12. Cancer Genome Atlas N. Genomic classification of cutaneous melanoma. Cell. (2015) 161:1681–96. doi: 10.1016/j.cell.2015.05.044
13. Alsaab HO, Sau S, Alzhrani R, Tatiparti K, Bhise K, Kashaw SK, et al. PD-1 and PD-L1 checkpoint signaling inhibition for cancer immunotherapy: mechanism, combinations, and clinical outcome. Front Pharmacol. (2017) 8:561. doi: 10.3389/fphar.2017.00561
14. Yearley JH, Gibson C, Yu N, Moon C, Murphy E, Juco J, et al. PD-L2 expression in human tumors: relevance to anti-PD-1 therapy in cancer. Clin Cancer Res. (2017) 23:3158–67. doi: 10.1158/1078-0432.CCR-16-1761
15. Nishiya AT, Massoco CO, Felizzola CR, Perlmann E, Batschinski K, Tedardi MV, et al. Comparative aspects of canine melanoma. Vet Sci. (2016) 3:7. doi: 10.3390/vetsci3010007
16. Tarone L, Giacobino D, Camerino M, Ferrone S, Buracco P, Cavallo F, et al. Canine melanoma immunology and immunotherapy: relevance of translational research. Front Vet Sci. (2022) 9:803093. doi: 10.3389/fvets.2022.803093
17. Fowles JS, Denton CL, Gustafson DL. Comparative analysis of MAPK and PI3K/AKT pathway activation and inhibition in human and canine melanoma. Vet Comp Oncol. (2015) 13:288–304. doi: 10.1111/vco.12044
18. Gillard M, Cadieu E, De Brito C, Abadie J, Vergier B, Devauchelle P, et al. Naturally occurring melanomas in dogs as models for non-UV pathways of human melanomas. Pigment Cell Melanoma Res. (2014) 27:90–102. doi: 10.1111/pcmr.12170
19. Shelly S, Chien MB, Yip B, Kent MS, Theon AP, McCallan JL, et al. Exon 15 BRAF mutations are uncommon in canine oral malignant melanomas. Mamm Genome. (2005) 16:211–7. doi: 10.1007/s00335-004-2441-x
20. Lee BH, Neela PH, Kent MS, Zehnder AM. IQGAP1 is an oncogenic target in canine melanoma. PLoS ONE. (2017) 12:e0176370. doi: 10.1371/journal.pone.0176370
21. Bergman PJ. Veterinary oncology immunotherapies. Vet Clin North Am Small Anim Pract. (2018) 48:257–77. doi: 10.1016/j.cvsm.2017.10.004
22. Domingues B, Lopes JM, Soares P, Populo H. Melanoma treatment in review. Immunotargets Ther. (2018) 7:35–49. doi: 10.2147/ITT.S134842
23. Petit V, Larue L. Any route for melanoblasts to colonize the skin! Exp Dermatol. (2016) 25:669–73. doi: 10.1111/exd.13061
24. Sommer L. Generation of melanocytes from neural crest cells. Pigment Cell Melanoma Res. (2011) 24:411–21. doi: 10.1111/j.1755-148X.2011.00834.x
25. D'Mello SAN, Finlay GJ, Baguley BC, Askarian-Amiri ME. Signaling pathways in melanogenesis. Int J Mol Sci. (2016) 17:1144. doi: 10.3390/ijms17071144
26. Correia MS, Moreiras H, Pereira FJC, Neto MV, Festas TC, Tarafder AK, et al. Melanin transferred to keratinocytes resides in nondegradative endocytic compartments. J Invest Dermatol. (2018) 138:637–46. doi: 10.1016/j.jid.2017.09.042
27. Mort RL, Jackson IJ, Patton EE. The melanocyte lineage in development and disease. Development. (2015) 142:620–32. doi: 10.1242/dev.123729
28. Smith SH, Goldschmidt MH, McManus PM, A. Comparative review of melanocytic neoplasms. Vet Pathol. (2002) 39:651–78. doi: 10.1354/vp.39-6-651
29. Ramos-Vara JA, Miller MA. Immunohistochemical identification of canine melanocytic neoplasms with antibodies to melanocytic antigen PNL2 and tyrosinase: comparison with melan A. Vet Pathol. (2010) 48:443–50. doi: 10.1177/0300985810382095
30. Smedley RC, Lamoureux J, Sledge DG, Kiupel M. Immunohistochemical diagnosis of canine oral amelanotic melanocytic neoplasms. Vet Pathol. (2011) 48:32–40. doi: 10.1177/0300985810387447
31. Smedley RC, Spangler WL, Esplin DG, Kitchell BE, Bergman PJ, Ho HY, et al. Prognostic markers for canine melanocytic neoplasms: a comparative review of the literature and goals for future investigation. Vet Pathol. (2011) 48:54–72. doi: 10.1177/0300985810390717
32. Simpson RM, Bastian BC, Michael HT, Webster JD, Prasad ML, Conway CM, et al. Sporadic naturally occurring melanoma in dogs as a preclinical model for human melanoma. Pigment Cell Melanoma Res. (2014) 27:37–47. doi: 10.1111/pcmr.12185
33. Prouteau A, André C. Canine melanomas as models for human melanomas: clinical, histological, and genetic comparison. Genes. (2019) 10:501. doi: 10.3390/genes10070501
34. Hernandez B, Adissu HA, Wei BR, Michael HT, Merlino G, Simpson RM. Naturally occurring canine melanoma as a predictive comparative oncology model for human mucosal and other triple wild-type melanomas. Int J Mol Sci. (2018) 19:394. doi: 10.3390/ijms19020394
35. Saleem A, Narala S, Raghavan SS. Immunohistochemistry in melanocytic lesions: updates with a practical review for pathologists. Semin Diagn Pathol. (2022) 39:239–47. doi: 10.1053/j.semdp.2021.12.003
36. Ohsie SJ, Sarantopoulos GP, Cochran AJ, Binder SW. Immunohistochemical characteristics of melanoma. J Cutan Pathol. (2008) 35:433–44. doi: 10.1111/j.1600-0560.2007.00891.x
37. Ramos-Vara JA, Beissenherz ME, Miller MA, Johnson GC, Pace LW, Fard A, et al. Retrospective study of 338 canine oral melanomas with clinical, histologic, and immunohistochemical review of 129 cases. Vet Pathol. (2000) 37:597–608. doi: 10.1354/vp.37-6-597
38. Koenig A, Wojcieszyn J, Weeks BR, Modiano JF. Expression of S100a, vimentin, NSE, and melan A/MART-1 in seven canine melanoma cells lines and twenty-nine retrospective cases of canine melanoma. Vet Pathol. (2001) 38:427–35. doi: 10.1354/vp.38-4-427
39. Riccardo F, Iussich S, Maniscalco L, Lorda Mayayo S, La Rosa G, Arigoni M, et al. CSPG4-specific immunity and survival prolongation in dogs with oral malignant melanoma immunized with human CSPG4 DNA. Clin Cancer Res. (2014) 20:3753. doi: 10.1158/1078-0432.CCR-13-3042
40. Mayayo SL, Prestigio S, Maniscalco L, Rosa G, Arico A, Maria R, et al. Chondroitin sulfate proteoglycan-4: a biomarker and a potential immunotherapeutic target for canine malignant melanoma. Vet J. (2011) 190:e26–30. doi: 10.1016/j.tvjl.2011.02.020
41. Rolih V, Barutello G, Iussich S, De Maria R, Quaglino E, Buracco P, et al. CSPG4: a prototype oncoantigen for translational immunotherapy studies. J Transl Med. (2017) 15:151. doi: 10.1186/s12967-017-1250-4
42. Piras LA, Riccardo F, Iussich S, Maniscalco L, Gattino F, Martano M, et al. Prolongation of survival of dogs with oral malignant melanoma treated by en bloc surgical resection and adjuvant CSPG4-antigen electrovaccination. Vet Comp Oncol. (2017) 15:996–1013. doi: 10.1111/vco.12239
43. Lacroux C, Raymond-Letron I, Bourgès-Abella N, Deviers A, Serra F, Degorce-Rubiales F, et al. Study of canine cutaneous melanocytic tumours: evaluation of histological and immunohistochemical prognostic criteria in 65 cases. Rev Med Vet. (2012) 163: 393–401.
44. Smedley RC, Bongiovanni L, Bacmeister C, Clifford CA, Christensen N, Dreyfus JM, et al. Diagnosis and histopathologic prognostication of canine melanocytic neoplasms: a consensus of the oncology-pathology working group. Vet Comparat Oncol. (2022) 3:12827. doi: 10.1111/vco.12827
45. Li LT, Jiang G, Chen Q, Zheng JN. Ki67 is a promising molecular target in the diagnosis of cancer (Review). Mol Med Rep. (2015) 11:1566–72. doi: 10.3892/mmr.2014.2914
46. Silvestri S, Porcellato I, Mechelli L, Menchetti L, Rapastella S, Brachelente C. Tumor thickness and modified clark level in canine cutaneous melanocytic tumors. Vet Pathol. (2019) 56:180–8. doi: 10.1177/0300985818798094
47. Davis EJ, Johnson DB, Sosman JA, Chandra S. Melanoma: what do all the mutations mean? Cancer. (2018) 124:3490–9. doi: 10.1002/cncr.31345
48. Vanni I, Tanda ET, Dalmasso B, Pastorino L, Andreotti V, Bruno W, et al. Non-BRAF mutant melanoma: molecular features and therapeutical implications. Front Mol Biosci. (2020) 7:172. doi: 10.3389/fmolb.2020.00172
49. Mackiewicz J, Mackiewicz A. BRAF and MEK inhibitors in the era of immunotherapy in melanoma patients. Contemp Oncol. (2018) 22:68–72. doi: 10.5114/wo.2018.73890
50. Davies H, Bignell GR, Cox C, Stephens P, Edkins S, Clegg S, et al. Mutations of the BRAF gene in human cancer. Nature. (2002) 417:949–54. doi: 10.1038/nature00766
51. Chen C-Y, Chen J, He L, Stiles BL. PTEN: Tumor suppressor and metabolic regulator. Front Endocrinol. (2018) 9:338. doi: 10.3389/fendo.2018.00338
52. Abbaspour Babaei M, Kamalidehghan B, Saleem M, Huri HZ, Ahmadipour F. Receptor tyrosine kinase (c-Kit) inhibitors: a potential therapeutic target in cancer cells. Drug Des Devel Ther. (2016) 10:2443–59. doi: 10.2147/DDDT.S89114
53. Moreira RN, Santos CR, Lima NL, Verli FD, Marinho SA. Oral and cutaneous melanoma: similarities and differences. J Clin Med Res. (2010) 2:155–8. doi: 10.4021/jocmr416w
54. Ma Y, Xia R, Ma X, Judson-Torres RL, Zeng H. Mucosal melanoma: pathological evolution, pathway dependency, and targeted therapy. Front Oncol. (2021) 11:702287. doi: 10.3389/fonc.2021.702287
55. Di Palma S, McConnell A, Verganti S, Starkey M. Review on canine oral melanoma: an undervalued authentic genetic model of human oral melanoma? Vet Pathol. (2021) 58:881–9. doi: 10.1177/0300985821996658
56. Chamcheu JC, Roy T, Uddin MB, Banang-Mbeumi S, Chamcheu R-CN, Walker AL, et al. Role and therapeutic targeting of the PI3K/Akt/mTOR signaling pathway in skin cancer: a review of current status and future trends on natural and synthetic agents therapy. Cells. (2019) 8:803. doi: 10.3390/cells8080803
57. Karbowniczek M, Spittle CS, Morrison T, Wu H, Henske EP. mTOR Is Activated in the majority of malignant melanomas. J Investig Dermatol. (2008) 128:980–7. doi: 10.1038/sj.jid.5701074
58. Strickland LR, Pal HC, Elmets CA, Afaq F. Targeting drivers of melanoma with synthetic small molecules and phytochemicals. Cancer Lett. (2015) 359:20–35. doi: 10.1016/j.canlet.2015.01.016
59. Ciołczyk-Wierzbicka D, Gil D, Zarzycka M, Laidler P. mTOR inhibitor everolimus reduces invasiveness of melanoma cells. Hum Cell. (2020) 33:88–97. doi: 10.1007/s13577-019-00270-4
60. Palušová V, Renzová T, Verlande A, Vaclová T, Medková M, Cetlová L, et al. Dual Targeting of BRAF and mTOR signaling in melanoma cells with pyridinyl imidazole compounds. Cancers. (2020) 12:1516. doi: 10.3390/cancers12061516
61. Zou Z, Tao T, Li H, Zhu X. mTOR signaling pathway and mTOR inhibitors in cancer: progress and challenges. Cell Biosci. (2020) 10:31. doi: 10.1186/s13578-020-00396-1
62. Schmid P, Hegde PS, Zou W, Kowanetz M, Mariathasan S, Molinero L, et al. Association of PD-L2 expression in human tumors with atezolizumab activity. Am J Clin Oncol. (2016) 34(15_suppl):11506. doi: 10.1200/JCO.2016.34.15_suppl.11506
63. Foster KG, Fingar DC. Mammalian target of rapamycin (mTOR): conducting the cellular signaling symphony. J Biol Chem. (2010) 285:14071–7. doi: 10.1074/jbc.R109.094003
64. Gremke N, Polo P, Dort A, Schneikert J, Elmshäuser S, Brehm C, et al. mTOR-mediated cancer drug resistance suppresses autophagy and generates a druggable metabolic vulnerability. Nat Commun. (2020) 11:4684. doi: 10.1038/s41467-020-18504-7
65. Esteves L, Caramelo F, Ribeiro IP, Carreira IM, de Melo JB. Probability distribution of copy number alterations along the genome: an algorithm to distinguish different tumour profiles. Sci Rep. (2020) 10:14868. doi: 10.1038/s41598-020-71859-1
66. Wong K, van der Weyden L, Schott CR, Foote A, Constantino-Casas F, Smith S, et al. Cross-species genomic landscape comparison of human mucosal melanoma with canine oral and equine melanoma. Nat Commun. (2019) 10:353. doi: 10.1038/s41467-018-08081-1
67. Poorman K, Borst L, Moroff S, Roy S, Labelle P, Motsinger-Reif A, et al. Comparative cytogenetic characterization of primary canine melanocytic lesions using array CGH and fluorescence in situ hybridization. Chromosome Res Int J Mol Supramol Evolut Aspects Chrom Biol. (2015) 23:171–86. doi: 10.1007/s10577-014-9444-6
68. Mochizuki H, Kennedy K, Shapiro SG, Breen M, BRAF. Mutations in canine cancers. PLoS One. (2015) 10:e0129534. doi: 10.1371/journal.pone.0129534
69. Barutello G, Rolih V, Arigoni M, Tarone L, Conti L, Quaglino E, et al. Strengths and weaknesses of pre-clinical models for human melanoma treatment: dawn of dogs' revolution for immunotherapy. Int J Mol Sci. (2018) 19:799. doi: 10.3390/ijms19030799
70. Mayr B, Schaffner G, Reifinger M, Zwetkoff S, Prodinger B. N-ras mutations in canine malignant melanomas. Vet J. (2003) 165:169–71. doi: 10.1016/S1090-0233(02)00245-9
71. Ferreira M, Reis Filho N, Pascoli A, Arosti B, Pazzini J, Huppes R, et al. The importance of the PI3K/AKT/mTOR signaling pathway in canine neoplasms: literature review. Arch med vet. (2016) 48:139–43. doi: 10.4067/S0301-732X2016000200002
72. Wei BR, Hoover SB, Peer CJ, Dwyer JE, Adissu HA, Shankarappa P, et al. Efficacy, tolerability and pharmacokinetics of combined targeted MEK and dual mTORC1/2 inhibition in a preclinical model of mucosal melanoma. Mol Cancer Ther (. (2020). doi: 10.1158/1535-7163.MCT-19-0858
73. Wei BR, Michael HT, Halsey CH, Peer CJ, Adhikari A, Dwyer JE, et al. Synergistic targeted inhibition of MEK and dual PI3K/mTOR diminishes viability and inhibits tumor growth of canine melanoma underscoring its utility as a preclinical model for human mucosal melanoma. Pigment Cell Melanoma Res. (2016) 29:643–55. doi: 10.1111/pcmr.12512
74. Obeid JM, Erdag G, Smolkin ME, Deacon DH, Patterson JW, Chen L, et al. PD-L1, PD-L2 and PD-1 expression in metastatic melanoma: Correlation with tumor-infiltrating immune cells and clinical outcome. Oncoimmunology. (2016) 5:e1235107. doi: 10.1080/2162402X.2016.1235107
75. Gata VA, Lisencu CI, Vlad CI, Piciu D, Irimie A, Achimas-Cadariu P. Tumor infiltrating lymphocytes as a prognostic factor in malignant melanoma. Review of the literature. J BUON. (2017) 22:592–8.
76. Yan Y, Wongchenko MJ, Robert C, Larkin J, Ascierto PA, Dreno B, et al. Genomic features of exceptional response in vemurafenib +/- cobimetinib-treated patients with BRAFV600-mutated metastatic melanoma. Clin Cancer Res. (2019). doi: 10.1158/1078-0432.CCR-18-0720
77. Hendricks WPD, Zismann V, Sivaprakasam K, Legendre C, Poorman K, Tembe W, et al. Somatic inactivating PTPRJ mutations and dysregulated pathways identified in canine malignant melanoma by integrated comparative genomic analysis. PLoS Genet. (2017) 3:196337. doi: 10.1101/196337
78. Wang K, Xu J, Zhang T, Xue D. Tumor-infiltrating lymphocytes in breast cancer predict the response to chemotherapy and survival outcome: a meta-analysis. Oncotarget. (2016) 7:44288–98. doi: 10.18632/oncotarget.9988
79. Schatton T, Scolyer RA, Thompson JF, Mihm MC Jr. Tumor-infiltrating lymphocytes and their significance in melanoma prognosis. Methods Mol Biol. (2014) 1102:287–324. doi: 10.1007/978-1-62703-727-3_16
80. Mihm MC Jr, Clemente CG, Cascinelli N. Tumor infiltrating lymphocytes in lymph node melanoma metastases: a histopathologic prognostic indicator and an expression of local immune response. Laboratory investigation. J Tech Methods Pathol. (1996) 74:43–7.
81. Clemente CG, Mihm MC Jr, Bufalino R, Zurrida S, Collini P, Cascinelli N. Prognostic value of tumor infiltrating lymphocytes in the vertical growth phase of primary cutaneous melanoma. Cancer. (1996) 77:1303–10. doi: 10.1002/(SICI)1097-0142(19960401)77:7<1303::AID-CNCR12>3.0.CO;2-5
82. Rao UN, Lee SJ, Luo W, Mihm MC Jr, Kirkwood JM. Presence of tumor-infiltrating lymphocytes and a dominant nodule within primary melanoma are prognostic factors for relapse-free survival of patients with thick (t4) primary melanoma: pathologic analysis of the e1690 and e1694 intergroup trials. Am J Clin Pathol. (2010) 133:646–53. doi: 10.1309/AJCPTXMEFOVYWDA6
83. Clark WH Jr, Elder DE, Guerry Dt, Braitman LE, Trock BJ, Schultz D, et al. Model predicting survival in stage I melanoma based on tumor progression. J Natl Cancer Inst. (1989) 81:1893–904. doi: 10.1093/jnci/81.24.1893
84. Zablocka T, Nikolajeva A, Kreismane M, Pjanova D, Isajevs S. Addressing the importance of melanoma tumor-infiltrating lymphocytes in disease progression and clinicopathological characteristics. Mol Clinoncol. (2021) 15:255. doi: 10.3892/mco.2021.2417
85. Tang Y, Zhang AXJ, Chen G, Wu Y, Gu W. Prognostic and therapeutic TILs of cervical cancer-Current advances and future perspectives. Mol Therapy Oncol. (2021) 22:410–30. doi: 10.1016/j.omto.2021.07.006
86. Federico L, McGrail DJ, Bentebibel SE, Haymaker C, Ravelli A, Forget MA, et al. Distinct tumor-infiltrating lymphocyte landscapes are associated with clinical outcomes in localized non-small cell lung cancer. J Eur Soc Med Oncol. (2021) 3:21. doi: 10.1016/j.annonc.2021.09.021
87. Wang E, Shibutani M, Nagahara H, Fukuoka T, Iseki Y, Okazaki Y, et al. Prognostic value of the density of tumor-infiltrating lymphocytes in colorectal cancer liver metastases. Oncol Lett. (2021) 22:837. doi: 10.3892/ol.2021.13098
88. Hong J, Rui W, Fei X, Chen X, Shen K. Association of tumor-infiltrating lymphocytes before and after neoadjuvant chemotherapy with pathological complete response and prognosis in patients with breast cancer. Cancer Med. (2021) 54:4302. doi: 10.1002/cam4.4302
89. Park CK, Kim SK. Clinicopathological significance of intratumoral and peritumoral lymphocytes and lymphocyte score based on the histologic subtypes of cutaneous melanoma. Oncotarget. (2017) 8:14759–69. doi: 10.18632/oncotarget.14736
90. Linette GP, Carreno BM. Tumor-infiltrating lymphocytes in the checkpoint inhibitor era. Curr Hematol Malig Rep. (2019) 14:286–91. doi: 10.1007/s11899-019-00523-x
91. Maibach F, Sadozai H, Seyed Jafari SM, Hunger RE, Schenk M. Tumor-infiltrating lymphocytes and their prognostic value in cutaneous melanoma. Front Immunol. (2020) 11:2105. doi: 10.3389/fimmu.2020.02105
92. Wouters MCA, Nelson BH. Prognostic Significance of Tumor-Infiltrating B Cells and Plasma Cells in Human Cancer. Clin Cancer Res. (2018) 24:6125–35. doi: 10.1158/1078-0432.CCR-18-1481
93. Honda C, Kurozumi S, Katayama A, Hanna-Khalil B, Masuda K, Nakazawa Y, et al. Prognostic value of tumor-infiltrating lymphocytes in estrogen receptor-positive and human epidermal growth factor receptor 2-negative breast cancer. Mol Clin Oncol. (2021) 15:252. doi: 10.3892/mco.2021.2414
94. Lenz JA, Assenmacher CA, Costa V, Louka K, Rau S, Keuler NS, et al. Increased tumor-infiltrating lymphocyte density is associated with favorable outcomes in a comparative study of canine histiocytic sarcoma. Cancer Immunol Immunother. (2021) 3:3033. doi: 10.1007/s00262-021-03033-z
95. Krane GA, O'Dea CA, Malarkey DE, Miller AD, Miller CR, Tokarz DA, et al. Immunohistochemical evaluation of immune cell infiltration in canine gliomas. Vet Pathol. (2021) 58:952–63. doi: 10.1177/03009858211023946
96. Tominaga M, Horiuchi Y, Ichikawa M, Yamashita M, Okano K, Jikumaru Y, et al. Flow cytometric analysis of peripheral blood and tumor-infiltrating regulatory T cells in dogs with oral malignant melanoma. J Vet Diag Invest. (2010) 22:438–41. doi: 10.1177/104063871002200317
97. Porcellato I, Brachelente C, De Paolis L, Menchetti L, Silvestri S, Sforna M, et al. FoxP3 and IDO in canine melanocytic tumors. Vet Pathol. (2018) 56:189–99. doi: 10.1177/0300985818808530
98. Porcellato I, Brachelente C, Cappelli K, Menchetti L, Silvestri S, Sforna M, et al. FoxP3, CTLA-4, and IDO in canine melanocytic tumors. Vet Pathol. (2021) 58:42–52. doi: 10.1177/0300985820960131
99. Yasumaru CC, Xavier JG, Strefezzi RF, Salles-Gomes COM. Intratumoral T-lymphocyte subsets in canine oral melanoma and their association with clinical and histopathological parameters. Vet Pathol. (2021) 58:491–502. doi: 10.1177/0300985821999321
100. Bujak JK, Pingwara R, Nelson MH, Majchrzak K. Adoptive cell transfer: new perspective treatment in veterinary oncology. Acta Vet Scand. (2018) 60:60. doi: 10.1186/s13028-018-0414-4
101. van Houdt IS, Sluijter BJ, Moesbergen LM, Vos WM, de Gruijl TD, Molenkamp BG, et al. Favorable outcome in clinically stage II melanoma patients is associated with the presence of activated tumor infiltrating T-lymphocytes and preserved MHC class I antigen expression. Int J Cancer. (2008) 123:609–15. doi: 10.1002/ijc.23543
102. Orth MF, Buecklein VL, Kampmann E, Subklewe M, Noessner E, Cidre-Aranaz F, et al. Expression patterns of PD-L1 and PD-1 provide rationales for immune checkpoint inhibition in soft tissue sarcomas. Cancer Immunol Immunother. (2019) 3:569418. doi: 10.1101/569418
103. Zhang Q, Salzler R, Dore A, Yang J, Ma D, Olson WC, et al. Multiplex immuno-liquid chromatography-mass spectrometry-parallel reaction monitoring (LC-MS-PRM) quantitation of CD8A, CD4, LAG3, PD1, PD-L1, and PD-L2 in frozen human tissues. J Proteome Res. (2018) 17:3932–40. doi: 10.1021/acs.jproteome.8b00605
104. Redman JM, Madan RA. Spotlight on atezolizumab and its potential as an oncology agent. Expert Rev Anticancer Ther. (2018) 18:719–22. doi: 10.1080/14737140.2018.1483241
105. Basu A, Yearley JH, Annamalai L, Pryzbycin C, Rini B. Association of PD-L1, PD-L2, and immune response markers in matched renal clear cell carcinoma primary and metastatic tissue specimens. Am J Clin Pathol. (2018) 2:141. doi: 10.1093/ajcp/aqy141
106. Lechner A, Schlosser H, Rothschild SI, Thelen M, Reuter S, Zentis P, et al. Characterization of tumor-associated T-lymphocyte subsets and immune checkpoint molecules in head and neck squamous cell carcinoma. Oncotarget. (2017) 8:44418–33. doi: 10.18632/oncotarget.17901
107. Guo PD, Sun ZW, Lai HJ, Yang J, Wu PP, Guo YD, et al. Clinicopathological analysis of PD-L2 expression in colorectal cancer. Onco Targets Ther. (2018) 11:7635–42. doi: 10.2147/OTT.S177329
108. Umezu D, Okada N, Sakoda Y, Adachi K, Ojima T, Yamaue H, et al. Inhibitory functions of PD-L1 and PD-L2 in the regulation of anti-tumor immunity in murine tumor microenvironment. Cancer Immunol Immunother. (2018) 3:2263. doi: 10.1007/s00262-018-2263-4
109. Chen DS, Mellman I. Oncology meets immunology: the cancer-immunity cycle. Immunity. (2013) 39:1–10. doi: 10.1016/j.immuni.2013.07.012
110. Cantwell-Dorris ER, O'Leary JJ, Sheils OM. BRAFV600E: implications for carcinogenesis and molecular therapy. Mol Cancer Ther. (2011) 10:385–94. doi: 10.1158/1535-7163.MCT-10-0799
111. Eroglu Z, Ribas A. Combination therapy with BRAF and MEK inhibitors for melanoma: latest evidence and place in therapy. Ther Adv Med Oncol. (2015) 8:48–56. doi: 10.1177/1758834015616934
112. Kozar I, Margue C, Rothengatter S, Haan C, Kreis S. Many ways to resistance: how melanoma cells evade targeted therapies. Biochimica et biophysica acta Rev Cancer. (2019) 1871:313–22. doi: 10.1016/j.bbcan.2019.02.002
113. Goff SL, Rosenberg SA, BRAF. inhibition: Bridge or boost to T cell therapy? Clin Cancer Res. (2019) 5:286. doi: 10.1158/1078-0432.CCR-19-0286
114. Verver D, van der Veldt A, van Akkooi A, Verhoef C, Grunhagen DJ, Louwman WJ. Treatment of melanoma of unknown primary in the era of immunotherapy and targeted therapy: a Dutch population-based study. Int J Cancer (. (2019). doi: 10.1002/ijc.32229
115. Aspeslagh S, Postel-Vinay S, Rusakiewicz S, Soria J-C, Zitvogel L, Marabelle A. Rationale for anti-OX40 cancer immunotherapy. Eur J Cancer. (2016) 52:50–66. doi: 10.1016/j.ejca.2015.08.021
116. Redmond WL, Linch SN, Kasiewicz MJ. Combined targeting of costimulatory (OX40) and coinhibitory (CTLA-4) pathways elicits potent effector T cells capable of driving robust antitumor immunity. Cancer Immunol Res. (2014) 2:142–53. doi: 10.1158/2326-6066.CIR-13-0031-T
117. Ohaegbulam KC, Assal A, Lazar-Molnar E, Yao Y, Zang X. Human cancer immunotherapy with antibodies to the PD-1 and PD-L1 pathway. Trends Mol Med. (2015) 21:24–33. doi: 10.1016/j.molmed.2014.10.009
118. Zheng P, Zhou Z. Human cancer immunotherapy with PD-1/PD-L1 blockade. Biomark Cancer. (2015) 7:15–8. doi: 10.4137/BIC.S29325
119. Ok CY, Young KH. Targeting the programmed death-1 pathway in lymphoid neoplasms. Cancer Treat Rev. (2017) 54:99–109. doi: 10.1016/j.ctrv.2017.01.009
120. Xu-Monette ZY, Zhang M, Li J, Young KH. PD-1/PD-L1 blockade: have we found the key to unleash the antitumor immune response? Front Immunol. (2017) 8:1597. doi: 10.3389/fimmu.2017.01597
121. Zhang F, Qi X, Wang X, Wei D, Wu J, Feng L, et al. Structural basis of the therapeutic anti-PD-L1 antibody atezolizumab. Oncotarget. (2017) 8:90215–24. doi: 10.18632/oncotarget.21652
122. Lee HT, Lee JY, Lim H, Lee SH, Moon YJ, Pyo HJ, et al. Molecular mechanism of PD-1/PD-L1 blockade via anti-PD-L1 antibodies atezolizumab and durvalumab. Sci Rep. (2017) 7:5532. doi: 10.1038/s41598-017-06002-8
123. Robert C, Schachter J, Long GV, Arance A, Grob JJ, Mortier L, et al. Pembrolizumab vs. ipilimumab in advanced melanoma. N Engl J Med. (2015) 372:2521–32. doi: 10.1056/NEJMoa1503093
124. Wang DY, Salem JE, Cohen JV, Chandra S, Menzer C, Ye F, et al. Fatal toxic effects associated with immune checkpoint inhibitors: a systematic review and meta-analysis. JAMA oncology. (2018) 4:1721–8. doi: 10.1001/jamaoncol.2018.3923
125. Pyuen AA, Meuten T, Rose BJ, Thamm DH. In vitro effects of PI3K/mTOR inhibition in canine hemangiosarcoma. PLoS One. (2018) 13:e0200634. doi: 10.1371/journal.pone.0200634
126. Maekawa N, Konnai S, Takagi S, Kagawa Y, Okagawa T, Nishimori A, et al. A canine chimeric monoclonal antibody targeting PD-L1 and its clinical efficacy in canine oral malignant melanoma or undifferentiated sarcoma. Sci Rep. (2017) 7:8951. doi: 10.1038/s41598-017-09444-2
127. Igase M, Nemoto Y, Itamoto K, Tani K, Nakaichi M, Sakurai M, et al. A pilot clinical study of the therapeutic antibody against canine PD-1 for advanced spontaneous cancers in dogs. Sci Rep. (2020) 10:18311. doi: 10.1038/s41598-020-75533-4
128. Regan D, Guth A, Coy J, Dow S. Cancer immunotherapy in veterinary medicine: current options and new developments. Vet J. (2016) 207:20–8. doi: 10.1016/j.tvjl.2015.10.008
129. Atherton MJ, Morris JS, McDermott MR, Lichty BD. Cancer immunology and canine malignant melanoma: a comparative review. Vet Immunol Immunopathol. (2016) 169:15–26. doi: 10.1016/j.vetimm.2015.11.003
130. Verganti S, Berlato D, Blackwood L, Amores-Fuster I, Polton GA, Elders R, et al. Use of Oncept melanoma vaccine in 69 canine oral malignant melanomas in the UK. J Small Anim Pract. (2017) 58:10–6. doi: 10.1111/jsap.12613
131. Camerino M, Giacobino D, Manassero L, Iussich S, Riccardo F, Cavallo F, et al. Prognostic impact of bone invasion in canine oral malignant melanoma treated by surgery and anti-CSPG4 vaccination: a retrospective study on 68 cases (2010–2020). Vet Comp Oncol (. (2021). doi: 10.1111/vco.12761
132. Mie K, Shimada T, Akiyoshi H, Hayashi A, Ohashi F. Change in peripheral blood lymphocyte count in dogs following adoptive immunotherapy using lymphokine-activated T killer cells combined with palliative tumor resection. Vet Immunol Immunopathol. (2016) 177:58–63. doi: 10.1016/j.vetimm.2016.06.007
133. Panjwani MK, Smith JB, Schutsky K, Gnanandarajah J, O'Connor CM, Powell DJ Jr, et al. Feasibility and safety of RNA-transfected CD20-specific chimeric antigen receptor T Cells in dogs with spontaneous B cell lymphoma. Mol Ther. (2016) 24:1602–14. doi: 10.1038/mt.2016.146
134. Mason NJ, Chester N, Xiong A, Rotolo A, Wu Y, Yoshimoto S, et al. Development of a fully canine anti-canine CTLA4 monoclonal antibody for comparative translational research in dogs with spontaneous tumors. MAbs. (2021) 13:2004638. doi: 10.1080/19420862.2021.2004638
135. Marable J, Ruiz D, Jaiswal AK, Bhattacharya R, Pantazes R, Agarwal P, et al. Nanobody-based CTLA4 inhibitors for immune checkpoint blockade therapy of canine cancer patients. Sci Rep. (2021) 11:20763. doi: 10.1038/s41598-021-00325-3
136. Maekawa N, Konnai S, Okagawa T, Nishimori A, Ikebuchi R, Izumi Y, et al. Immunohistochemical analysis of PD-L1 expression in canine malignant cancers and PD-1 expression on lymphocytes in canine oral melanoma. PLoS ONE. (2016) 11:e0157176. doi: 10.1371/journal.pone.0157176
137. Maekawa N, Konnai S, Ikebuchi R, Okagawa T, Adachi M, Takagi S, et al. Expression of PD-L1 on canine tumor cells and enhancement of IFN-gamma production from tumor-infiltrating cells by PD-L1 blockade. PLoS ONE. (2014) 9:e98415. doi: 10.1371/journal.pone.0098415
138. Igase M, Inanaga S, Tani K, Nakaichi M, Sakai Y, et al. Long-term survival of dogs with stage 4 oral malignant melanoma treated with anti-canine PD-1 therapeutic antibody: A follow-up case report. Vet Comp Oncol. (2022) 20:901–5. doi: 10.1111/vco.12829
139. Stevenson VB, Perry SN, Todd M, Huckle WR, LeRoith T. PD-1, PD-L1, and PD-L2 gene expression and tumor infiltrating lymphocytes in canine melanoma. Vet Pathol. (2021) 58:692–8. doi: 10.1177/03009858211011939
Keywords: PD-1, PD-L1, PD-L2, dog melanoma, tumor infiltrating lymphocytes, immunotherapy
Citation: Stevenson VB, Klahn S, LeRoith T and Huckle WR (2023) Canine melanoma: A review of diagnostics and comparative mechanisms of disease and immunotolerance in the era of the immunotherapies. Front. Vet. Sci. 9:1046636. doi: 10.3389/fvets.2022.1046636
Received: 16 September 2022; Accepted: 12 December 2022;
Published: 06 January 2023.
Edited by:
Fernando Costa Ferreira, University of Lisbon, PortugalReviewed by:
Maria Dos Anjos Pires, University of Trás-os-Montes and Alto Douro, PortugalCopyright © 2023 Stevenson, Klahn, LeRoith and Huckle. This is an open-access article distributed under the terms of the Creative Commons Attribution License (CC BY). The use, distribution or reproduction in other forums is permitted, provided the original author(s) and the copyright owner(s) are credited and that the original publication in this journal is cited, in accordance with accepted academic practice. No use, distribution or reproduction is permitted which does not comply with these terms.
*Correspondence: William R. Huckle, d3JodWNrbGVAdnQuZWR1
Disclaimer: All claims expressed in this article are solely those of the authors and do not necessarily represent those of their affiliated organizations, or those of the publisher, the editors and the reviewers. Any product that may be evaluated in this article or claim that may be made by its manufacturer is not guaranteed or endorsed by the publisher.
Research integrity at Frontiers
Learn more about the work of our research integrity team to safeguard the quality of each article we publish.