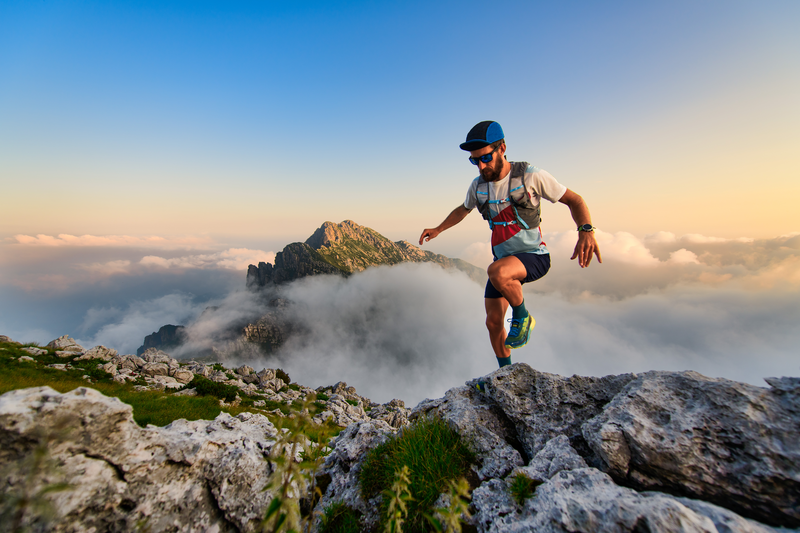
95% of researchers rate our articles as excellent or good
Learn more about the work of our research integrity team to safeguard the quality of each article we publish.
Find out more
ORIGINAL RESEARCH article
Front. Vet. Sci. , 17 January 2023
Sec. Animal Reproduction - Theriogenology
Volume 9 - 2022 | https://doi.org/10.3389/fvets.2022.1043379
The present study looks for components in seminal plasma (SP) and/or serum that are closely related to in vivo fertility of buffalo bulls. Fourteen healthy mature buffalo bulls were classified according to their in vivo fertility into fertile (n = 10) and subfertile (n = 4) groups. Semen and serum samples were collected from all animals for 12 replicates. The collected ejaculates were examined for sperm characteristics before being centrifuged to collect SP for hormonal (FSH, LH, testosterone, and IGF-1), biochemical [total antioxidant capacity (TAC), catalase (CAT), glutathione peroxidase (GPx), nitric oxide (NO), malondialdehyde (MDA), fructose, total protein, albumin, triglycerides, cholesterol, and high-density lipoprotein (HDL)] and proteomic (SDS-PAGE) analyses. Likewise, serum levels of FSH, LH, testosterone, IGF-1, glucose, total protein, albumin, triglycerides, cholesterol, and HDL were determined. All sperm characteristics and the majority of sperm kinematics were (P < 0.01) different between fertile and subfertile groups. Seminal and serum levels of FSH, LH, testosterone, and IGF-1 were higher (P < 0.01) in the fertile group, but only seminal fructose, total protein, albumin, triglycerides, cholesterol, and HDL were higher (P < 0.01) in the fertile group. Moreover, the fertile group had greater TAC, CAT, GPx, and NO, but the subfertile group had greater MDA. Protein bands of 14, 15, 26, 30, and 55 kDa were larger and denser in the SP of the fertile group but were smaller and faint to absent in that of the subfertile group. Also, the protein fractions of detected protein bands demonstrated a substantial influence of fertility on those of 16, 26, 30, and 55 kDa. In conclusion, sperm characteristics and kinematics with serum, and/or seminal hormonal and biochemical components, should be evaluated for reliable prediction of buffalo bull fertility. Furthermore, protein bands of 26, 30, and 55 kDa may represent fertility-associated proteins in buffalo bull SP.
Seminal plasma (SP) is an extremely complicated biological fluid containing electrolytes, hormones, proteins, enzymes, carbohydrates, and lipids that have an important impact on sperm cell function and its cryosurvival (1). Thus, SP is a reliable indicator of male fertility. Increased reactive oxygen species (ROS) in semen samples adversely affect sperm quality and fertility. Moreover, the high content of polyunsaturated fatty acids in buffalo bull sperm as compared to bull sperm renders them more vulnerable to oxidative stress (2, 3).
In humans, seminal fructose (4) is the main source of energy production alongside glucose in the majority of mammalian species. Glucose and fructose were found to have beneficial effects on mammalian gametes in terms of metabolizable energy and fertility potential (5). Additionally, fructose was higher in highly fertile bulls, suggesting that it could be used as a seminal biomarker for bull fertility (6). Both serum and seminal testosterone levels were positively related to sperm quality and fertility in rams (7, 8), and it promotes sperm production in bulls (9). Both LH and FSH regulate testosterone synthesis in Leydig cells and are also responsible for male fertility (9). Reproductive biologists believe that metabolic hormones play a role in spermatogenesis/steroidogenesis, and seminal concentrations of IGF-1 are positively related to stallion fertility (10). Also, the post-thaw sperm motility and viability were greatly improved by adding IGF-1 (11) to the freezing extender in buffalo bulls.
Both albumin and globulin constitute the major fraction of SP proteins, whereas non-protein nitrogen, amino acids, and peptides represent limited quantities. Proteins of SP have amphoteric properties and thus, low protein content in SP reduced its buffering capacity, and ultimately decreased sperm quality and fertility (12). Seminal plasma lipids play an important role in sperm membrane structure and function. Furthermore, there is evidence that semen volume, sperm motility, and concentration are influenced by lipid species in SP (13). SP proteins are species-specific and some of them are closely related to male fertility (14, 15). Previous research has focused on identifying and isolating specific seminal proteins that may affect buffalo sperm capacitation and/or fertilization (14, 16, 17). Previous proteomic studies on SP proteins, to the best of our knowledge, did not combine hormonal and biochemical components with proteomic analyses. As a result, the present study looks for hormonal, biochemical, and proteomic components in SP and/or serum collected from buffalo bulls of different in vivo fertility which could be used as markers of fertility.
Except otherwise specified, the chemicals used were of high purity and procured from Merck KGaA (Darmstadt, Germany).
A total of 14 healthy and mature Egyptian buffalo bulls (Bubalus bubalis, 4–5 years old) with good body condition scores were classified depending upon their conception rate (CR) obtained following insemination of 330 estrus pluriparous buffalo cows (4–8 years old) during the last two previous breeding seasons using their frozen-thawed straws into fertile (n = 10, CR ≥ 55%) and subfertile (n = 4, CR ≤ 35%) groups in line with Kumar et al. (18). All buffalo bulls were kept at Mahallet-Mousa Research Farm located in Mahallet-Mousa, Kafrelsheikh (latitude 31° 06′ N and longitude 30° 56′ E), Egypt. Animals were maintained in open yards, fed a concentrated diet combination and roughages according to the National Research Council (19) standards, with free access to clean water and mineral blocks. All animal experiments were conducted according to the ARRIVE guidelines (https://arriveguidelines.org) and approved by the Committee for Ethics in Research, Faculty of Veterinary Medicine, Kafrelsheikh University, Egypt.
From September to November, semen samples were collected twice a week at 07:00–08:00 a.m. using an artificial vagina (with an inner sleeve temperature of 40°C) for 6 weeks (12 ejaculates/animal). Immediately after collection, the collected ejaculates were visually examined for color, consistency, and hygienic quality, and also ejaculate volume was noticed. From each ejaculate, an aliquot (200 μL) was used to determine the following sperm characteristics and kinematics:
Sperm kinematics were determined using a computer-aided sperm motion analyzer (CASA; Hamilton Thorne, Inc., Beverly, MA, USA) system. The sperm motility was calculated with speed standards set as fast; >80 μm/s, medium; >60 μm/s, slow; >20 μm/s, and static. From each ejaculate, an aliquot was diluted (1:10) with prewarmed Tris buffer before being (5 μL) loaded into a prewarmed (37°C) Makler counting chamber to determine total motility (%), progressive motility (%), average path velocity (VAP, μm/s), straight linear velocity (VSL, μm/s), curvilinear velocity (VCL, μm/s), straightness (STR, %), linearity (LIN, %), and wobble coefficient (WOB, %) according to Kumar et al. (3). For each evaluation, eight microscopic fields were randomly selected and analyzed by the CASA system. An eosin-nigrosin-stained semen smear was examined to estimate the proportion of viable spermatozoa (20). At least 200 spermatozoa were examined under an oil immersion lens (1,000×) where sperm cells with unstained heads expressed percent sperm viability.
To assess the functional integrity of the sperm plasma membrane, sperm cells were subjected to a hypo-osmotic swelling test [HOST; (21)]. In brief, a prewarmed hypo-osmotic solution (1,000 μL) of 150 mOsm/kg osmolarity (fructose = 1.351 g, sodium citrate = 0.735 g dissolved in 100 mL Milli-Q water) was mixed with 100 μL semen. This sperm suspension was incubated at 37°C for at least 30 min. After incubation, an aliquot (2 μL) of this suspension was spotted onto a prewarmed clean glass slide, covered by a prewarmed coverslip (18 × 18 mm), and visualized under 400× magnification. At least 200 spermatozoa were carefully examined for the proportion of spermatozoa showing curling or swelling of their tails (% HOST-positive). The proportion of spermatozoa with abnormal tail morphology was already determined before HOST and was subtracted from the proportion of HOST-positive spermatozoa to obtain the true percentage of HOST-positive spermatozoa.
Fluorescein isothiocyanate-conjugated peanut agglutinin (FITC-PNA) staining technique was used to determine acrosomal membrane integrity as described in our previous research (22). Briefly, an aliquot (10 μL) of semen was fixed with 4% paraformaldehyde (Sigma Chemical Company, USA) at room temperature for 30 min and then diluted (1:10) with phosphate-buffered saline (PBS) containing 0.1% polyvinyl alcohol (Sigma Chemical Company, USA) and 0.1% polyethylene glycol (Sigma Chemical Company, USA) before being (5 μL) smeared onto a glass slide and dried on a warmed plate at 38.5°C. Spermatozoa were permeabilized by using 200 μL of 1% (v/v) Triton X-100 for 5 min at room temperature and then allowed to dry and stained with FITC-PNA (20 μg/mL; Sigma Chemical Company, USA) for 30 min in a humidified chamber in a dark place.
After incubation, stained smears were rinsed with PBS to remove unbound probes, allowed to dry, and covered with 0.22 M 1,4-diazabicyclo [2,2,2] octane (Sigma–Aldrich, Germany) dissolved in glycerol-PBS mixture (9:1) (DABCO). Stained smears were covered with a coverslip (24 mm × 50 mm) before examination with a phase-contrast microscope with fluorescence illumination (mirror unit U-MWB2: excitation filter, BP460–490, dichroic mirror DM500, and emission filter BA520IF; Olympus, Tokyo, Japan). Sperm cells with uniform, intense, and well-demarcated green fluorescent acrosomes were graded as intact-acrosomes while those exhibiting fluorescence in the anterior region only, no fluorescence in the head, or fluorescence only along the outline or acrosomal fringe were graded as damaged-acrosomes. At least 200 spermatozoa were examined in each smear to calculate the intact-acrosomes percentage.
The remaining volume of ejaculate was centrifuged at 12,000 × g in a cooling centrifuge at 4°C for 30 min to collect SP. The supernatant (SP) was re-centrifuged at 12,000 × g for 10 min in a microfuge (Mittelsachsen, Saxony, Germany) to obtain clear SP. Total protein concentration (g/dL) in SP was determined with a hand-held refractometer [ATAGO, Brix 0–32%, Japan, (23)], and then SP was frozen stored at −80°C until hormonal, biochemical, and proteomic analyses. In parallel, throughout the experiment, blood samples have been drawn from the jugular vein of all buffalo bulls before ejaculation. The collected blood samples were centrifuged at 2,500 × g for 20 min at 4°C to separate serum, and the collected serum was also frozen stored at −80°C until hormonal and biochemical analyses.
Serum and seminal assays of LH and FSH were performed by chemiluminescence through the immune-enzymatic technique using the commercial kits Beckman Coulter® (Beckman Coulter, USA) and the Access® equipment (Beckman Coulter, USA) according to the manufacturer's instructions. Serum and seminal total testosterone levels were measured using commercial ELISA kits (Bio Check, Foster City, CA, USA) in duplicate with a sensitivity of 0.5 ng/mL, while the intra- and inter-assay coefficients of variation (CV) were 7.7 and 9.0%, respectively. The immuno-radiometric assay kit (Immunotech SAS, Marseille Cedex, France) was used to measure serum and seminal IGF-1 levels (24), with a 2 ng/mL sensitivity and an intra-assay CV of 3.26%.
Total antioxidant capacity (TAC) was estimated using an antioxidant assay kit provided by Cayman chemical company (Michigan, USA) as designated by Lone et al. (25). Seminal levels of Catalase [CAT; (26)], Glutathione peroxidase [GPx; (3)], Nitric oxide [NO; (27)], and Malondialdehyde [MDA; (3)] were calculated. Seminal fructose was measured with the colorimetric method (28), whereas the Cobas c 311/501 Analyzer (Roche Diagnostics GmbH, Mannheim, Germany) was utilized to determine the serum glucose level. Colorimetric methods were used to assess the serum and seminal amounts of total protein (29) and albumin (30). Also, serum and seminal contents of triglycerides, cholesterol, and high-density lipoproteins (HDL) were calorimetrically determined using commercial kits (Bio-Diagnosis Co., Cairo, Egypt).
This experiment was run in four repetitions, utilizing the SP of both fertile and subfertile buffalo bulls in the same separating gel in the presence of a known standard to evaluate the relative content of different SP proteins and their molecular weight. Frozen SP was thawed and adjusted to a total protein concentration of 500 μg/25 μL before being subjected to SDS-PAGE (31). Briefly, electrophoresis sample buffer [4% (w/v) SDS, 20% (v/v) glycerol, 10% (v/v) β-mercaptoethanol, and pH 6.8] was used to dilute (1:1) SP samples then boiled for exactly 5 min, followed by centrifugation at 10,000 × g at 4°C for 5 min to collect the supernatants containing SP proteins.
Extracted SP proteins were separated on a 15% (w/v) polyacrylamide gel containing 0.1% (w/v) SDS at room temperature and 20 mA/gel using a mini protean III vertical slab gel electrophoresis apparatus (Bio-Rad Laboratories, Hercules, CA, USA). The separating gels were immersed for 5 min in a freshly prepared pre-fixative solution containing 20% (v/v) methanol and 7.5% (v/v) acetic acid in H2O before being stained with 0.1% (w/v) Coomassie brilliant blue R-250 (Oxford, Mumbai, India) at room temperature with gentle shaking for 1 h (32). Stained gels were destained in a solution of 30% (v/v) methanol and 10% (v/v) acetic acid in H2O overnight with gentle shaking. Finally, the gel was scanned with a scanner (HP Scanjet G3110, Hong Kong, China) and the apparent molecular weight was calculated with a broad-way dual prestained protein marker (6.5–212 kDa, New England, BioLabs, UK). The proportions of different protein fractions were also determined using an analytical system (Gel-Doc. Model-Alpha Imager TM1220; Alpha Innotech Corporation, Santa Clara, CA, USA).
The results are tabulated as mean ± SEM. A GraphPad Prism computer program version 7.0 (GraphPad Software, San Diego, CA, USA) was used to perform all statistical analyses, and a Student's t-test was employed to compare fertile and subfertile groups. Differences with P < 0.05 were considered statistically significant. Using the gel-doc system, gel images were processed to quantify the molecular weights of protein bands as well as the relative protein fractions (protein %).
As shown in Table 1, the values of sperm characteristics (total motility, progressive motility, viability, intact-plasma membrane, and intact-acrosome) and sperm kinematics variables except LIN and WOB were higher (P < 0.01) in the fertile group than in the subfertile group.
Both seminal and serum levels of FSH, LH, testosterone, and IGF-1 were greater (P < 0.01) in the fertile group than in the subfertile group, as shown in Tables 2, 3. Also, seminal TAC, CAT, GPx, and NO were higher (P < 0.05) in the fertile group. Likewise, seminal fructose, total protein, albumin, triglycerides, cholesterol, and HDL were greater (P < 0.01) in the fertile group compared with the subfertile group (Table 2). On the contrary, seminal MDA was greater (P < 0.001) in the subfertile (1.56 ± 0.08 μM/mL) group than in the fertile (0.96 ± 0.05 μM/mL) group as presented in Table 2. Serum levels of glucose, total protein, albumin, triglycerides, cholesterol, and HDL were comparable (P ≥ 0.05) between the two fertility groups as given in Table 3.
The SP protein profile (kDa) and their protein fractions (%) of fertile and subfertile buffalo bulls are represented in Table 4 and Figure 1. Gel analysis revealed 10 (70, 55, 42, 30, 26, 24, 19, 16, 15, and 14 kDa) protein bands with a molecular weight ranging from 14 to 70 kDa were identified in both fertile and subfertile buffalo bulls. Almost all the detected bands were larger and denser in the fertile group compared with the subfertile group as illustrated in Figure 1. It is worth mentioning that protein bands of 14, 15, 26, 30, and 55 kDa were more predominant in the fertile group compared to the subfertile one. Additionally, the protein % of 55, 30, and 26 kDa protein bands was considerably greater (P < 0.001) in the fertile group, whereas, the protein % of the 16 kDa protein band was considerably greater (P < 0.001) in the subfertile group as presented in Table 4.
Table 4. Seminal plasma proteins profiles (kDa) and protein fractions (%) of fertile and subfertile buffalo bulls.
Figure 1. SDS-PAGE of SP proteins in fertile and subfertile buffalo bulls. Bulls were classified into fertile and subfertile groups based on their conception rates. SP was collected by centrifugation (12,000 × g for 30 min) of the collected semen samples. Reduction, denaturation, and separation of SP proteins on 15% polyacrylamide gel were performed in the presence of a broad-way (6.5–212 kDa) prestained protein marker. Separated protein bands were detected by Coomassie brilliant blue staining. Std, Standards; kDa, Kilo Dalton; Mr. Wt, Molecular Weight.
Male fertility is a multifactorial trait depending upon several factors, including animal behavior and physical fitness, as well as semen quality and biochemical and/or hormonal components of SP (33). As we expected, in the present study, the proportion of sperm motility and viability, and almost all sperm kinematics were significantly higher in fertile buffalo bulls compared with subfertile animals. This is likely attributed to normal testicular anatomy, histology, and function in fertile animals, which backed the findings of Singh et al. (34), who found that the proportion of motile spermatozoa in highly fertile bulls was higher (P < 0.001) than that of low fertile bulls. Further, our findings indicated that the proportions of intact-acrosome and intact-plasma membrane were greater in the fertile animals compared to the subfertile ones, which increased oocyte penetration and enhanced the fertilization process (35).
Oxidative stress is among the most implicated causes of low semen quality and has a prolonged history of being linked to male subfertility (36). This oxidative stress increases sperm cell abnormalities, loss of membrane integrity, DNA damage, and enzyme inactivation, leading to subfertility and/or infertility (37). Antioxidants in the SP or on spermatozoa are the major defense mechanisms against oxidative stress by minimizing the liberation of free radicals. As a result, they enhance sperm motility and might be beneficial in the treatment of male subfertility and/or infertility (38). Herein, fertile buffalo bulls had greater seminal TAC, CAT, GPx, and NO and lower MDA content than subfertile buffalo bulls, emphasizing the notion that MDA is a cytotoxic aldehyde and its elevation in SP has negative impacts on the quality and fertility of sperm cells due to its action on lipid membrane structures (39). In line with our results, Barranco et al. (40) reported that antioxidants have a favorable correlation with the fertility index, which may prevent oxidative damage to the sperm plasma membrane and might be used as a strong fertility estimate in boars. This could explain the higher seminal values of TAC, CAT, GPx, and NO of fertile buffalo bulls obtained in the current trial.
IGF-1 plays an important role in energy metabolism and improves the metabolic activity of buffalo bull spermatozoa through increasing intracellular calcium ion concentration, which results in increased progressive sperm motility (41). Our results indicated that fertile buffalo bulls had higher seminal and serum IGF-1 concentrations, which is partially consistent with Kumar et al. (42), who found that serum IGF-1 concentrations in fertile buffalo bulls were higher than those of subfertile animals and were positively correlated with sperm concentration, mass motility, and fertility. Our findings, On the other hand, are inconsistent with Kumar et al. (42) who found no difference in seminal IGF-1 levels between fertile and subfertile buffalo bulls. This might be due to our study having a higher number of fertile (10 vs. 5) and subfertile (4 vs. 2) buffalo bulls than Kumar et al. (42). Thus, quantification of seminal and/or serum IGF-1 concentration is recommended to predict sperm cell functions (43, 44).
In our trial, fertile buffalo bulls had greater levels of serum and seminal testosterone compared to subfertile animals. This is likely due to testosterone regulating testicular function, particularly Sertoli cell function, and also has a crucial role in the process of spermatogenesis (45). Recently, it has been proven that seminal testosterone level is positively associated with sperm cell concentration and the proportion of motile spermatozoa in fertile bulls (46). Both FSH and LH regulate testosterone synthesis and sustain proper spermatogenesis, sperm vitality, and density (47). This might explain why the fertile group had greater seminal and serum FSH and LH levels than the subfertile group. The low LH concentration in the subfertile group may not activate the Leydig cells, resulting in low serum and seminal testosterone levels. Moreover, low FSH levels impair Sertoli cell activity, leading to subfertility or even infertility (48). Based on these findings, it is plausible to obtain greater levels of FSH and LH in either the serum or SP of fertile buffalo bulls.
Seminal fructose is produced via the conversion of glucose with aldose reductase into sorbitol in the vesicular glands, which is testosterone-dependent, and eventually, sorbitol dehydrogenase reduces sorbitol into fructose (49). Our results indicated that seminal fructose was greater in the fertile group than in the subfertile one; this supports the fact that seminal fructose plays an important role in the metabolic activity of sperm cells, where sperm cells consume fructose to produce ATP to maintain their motility (50).
Our findings reveal that the fertile group has higher seminal total protein and albumin content in comparison with the subfertile group. It is well-known that the seminal protein fraction constitutes the amphoteric characteristic of SP and represents the main component of its buffering activity (51). Also, seminal albumin plays a crucial role in sperm motility, capacitation, and acrosome reaction by acting as a cholesterol acceptor, leading to cholesterol efflux from the sperm plasma membrane (52), which is an initial and essential step in the fertilization cascade. These aforementioned findings might be the reason for the low semen quality and/or low fertility of subfertile buffalo bulls.
Cholesterol has a pivotal role in sperm motility, capacitation, acrosome reaction, and fertility (53). In the current study, the fertile group had a higher seminal cholesterol level (117.70 ± 2.38 mg/dL) than the subfertile (78.17 ± 1.89 mg/dL) group, which is consistent with the findings of El-Sayed et al. (54), who reported that seminal cholesterol level was positively correlated with ejaculate volume, total sperm cell count per ejaculate, sperm motility, viability, as well as the integrity of plasma and acrosomal membranes in buffalo bulls. Similarly, seminal triglyceride content was greater in the fertile (58.83 ± 1.47 mg/dL) buffalo bulls because they are one of the main energy substrates available for sperm cell metabolism; thus, low triglyceride levels might lead to insufficient energy, low sperm motility, and subfertility (55). Moreover, seminal triglycerides and HDL levels in buffalo bulls were also positively associated with sperm concentration, the total number of sperm cells per ejaculate, and acrosomal integrity (54).
In our study, 10 protein bands were detected in SP collected from either fertile or subfertile buffalo bulls but those of 14, 15, 26, 30, 42, and 55 kDa were predominant in the fertile animals. Protein bands of 70, 55, 42, 26, and 24 kDa may correspond to albumin or clusterin precursor, osteopontin, clusterin, or ecto-ADP-ribosyltransferase 5, prostaglandin D synthase, tissue inhibitor metalloproteinase-2 (TIMP-2), respectively as summarized in Table 4 (56, 57). Protein bands of 30, 16, 15, and 14 kDa may correspond to bovine seminal plasma protein-30 kDa (BSP-30 kDa), BSP-A3, BSP-A2, and BSP-A1 or spermadhesin, respectively (58).
Notably, the protein % of 55, 30, and 26 kDa protein bands was higher in the fertile group (Table 4), indicating that these protein bands are related to in vivo fertility at least in Egyptian buffalo bulls. In accord with Killian et al. (59), we found that the protein % of the 16 KDa protein band was higher in subfertile animals, indicating that this protein band might be used as a diagnostic for male subfertility. Based on the findings of Gwathmey et al. (60) who reported that all BSPA1/A2, BSP-A3, and BSP-30 kDa are the major proteins in bovine SP which play substantial roles in animal fertility, and also results of the current study revealed that protein bands of 14, 15, 26 and 30 kDa were denser and larger in fertile buffalo bulls, suggesting that these proteins are fertility-associated at least in buffalo bulls.
It is well-known that osteopontin is a 55 kDa acidic SP protein that has a critical role in sperm oocyte encounter and the early stages of embryo development (61) which might be one of the possible reasons for the difference in the in vivo fertility of the two animal groups (fertile vs. subfertile). Furthermore, a protein band of 26 kDa might be prostaglandin D synthase that is localized in the apical ridge of the sperm acrosome (62) and indirectly helps the male genital organs in uptaking retinoids, essential for growth, differentiation, and spermatogenesis (63).
Seminal TAC, CAT, GPx, NO, and fructose, as well as seminal and/or serum FSH, LH, testosterone, and IGF-1, are all fertility-associated biomarkers. Seminal levels of total protein, albumin, triglycerides, cholesterol, and HDL can also be used to distinguish fertile buffalo bulls from subfertile buffalo bulls. Furthermore, protein bands of 26, 30, and 55 kDa in buffalo bull SP may represent fertility-associated proteins that require further investigation in the future.
The datasets presented in this study can be found in online repositories. The names of the repository/repositories and accession number(s) can be found in the article/supplementary material.
The animal study was reviewed and approved by the ARRIVE guidelines (https://arriveguidelines.org) and approved by the Committee for Ethics in Research, Faculty of Veterinary Medicine, Kafrelsheikh University, Egypt.
Conceptualization, methodology, investigation, data curation, writing, review, and editing of the manuscript, supervision, formal analysis, and visualization were performed by EA, AA, FS, KK, TA, and WE. AA, FS, and WE drafted the manuscript, performed the statistical analysis, and supervised by EA. All authors read and approved the final manuscript.
The unlimited support of the animal production crew of the Mahallet-Mousa buffalo farm is highly appreciated.
The authors declare that the research was conducted in the absence of any commercial or financial relationships that could be construed as a potential conflict of interest.
All claims expressed in this article are solely those of the authors and do not necessarily represent those of their affiliated organizations, or those of the publisher, the editors and the reviewers. Any product that may be evaluated in this article, or claim that may be made by its manufacturer, is not guaranteed or endorsed by the publisher.
1. Rodriguez-Martinez H, Martinez EA, Calvete JJ, Peña Vega FJ, Roca J. seminal plasma: relevant for fertility? Int J Mol Sci. (2021) 22:4368–96. doi: 10.3390/ijms22094368
2. Garg A, Kumaresan A, Ansari MR. Effects of hydrogen peroxide (H2O2) on fresh and cryopreserved buffalo sperm functions during incubation at 37 degrees C in vitro. Reprod Domest Anim. (2009) 44:907–12. doi: 10.1111/j.1439-0531.2008.01115.x
3. Kumar P, Kumar D, Sikka P, Singh P. Sericin supplementation improves semen freezability of buffalo bulls by minimizing oxidative stress during cryopreservation. Anim Reprod Sci. (2015) 152:26–31. doi: 10.1016/j.anireprosci.2014.11.015
4. Gonzales GF. The function of seminal vesicles and their role on male fertility. Asian J Androl. (2001) 3:251–8. Available online at: https://pubmed.ncbi.nlm.nih.gov/11753468
5. Williams AC, Ford WC. The role of glucose in supporting motility and capacitation in human spermatozoa. J Androl. (2001) 22:680–95.
6. Velho ALC, Menezes E, Dinh T, Kaya A, Topper E, Moura AA, et al. Metabolomic markers of fertility in bull seminal plasma. PLoS ONE. (2018) 13:e0195279. doi: 10.1371/journal.pone.0195279
7. Almadaly EA, Ashour MA, El-Kon II, Heleil BA. Traditional and non-traditional methods are used for discrimination among Ossimi rams with different field fertility. Small Rumin Res. (2019) 179:30–8. doi: 10.1016/j.smallrumres.2019.09.003
8. Almadaly EA, Ashour MA, Elfeky MS, Gewaily MS, Assar DH, Gamal IM. Seminal plasma and serum fertility biomarkers in Ossimi rams and their relationship with functional membrane integrity and morphology of spermatozoa. Small Rumin Res. (2021) 196:106318. doi: 10.1016/j.smallrumres.2021.106318
9. Hafez ESE, Hafez B. Reproduction in Farm Animals. 7th ed. London, Blackwell Publishing (2013). p. 293–397. Available online at: https://www.wiley.com/enus/Reproduction±in±Farm±Animals%2C±7th±Edition-p-9781118710289 (accessed January 5, 2023).
10. Macpherson ML, Simmen RCM, Simmen FA, Hernandez J, Sheerin BR, Varner DD. Insulin-like growth factor-I and insulin-like growth factor binding protein-2 and−5 in equine seminal plasma: association with sperm characteristics and fertility. Biol Reprod. (2002) 67:648–54. doi: 10.1095/biolreprod67.2.648
11. Kumar A, Singh G, Jerome A, Kumar P, Arjun V, Bala R, et al. IGF-1 supplementation in semen affects mitochondrial function and calcium status of buffalo sperm following cryopreservation. Anim Reprod Sci. (2021) 231:106783. doi: 10.1016/j.anireprosci.2021.106783
12. Dixit S, Pandey V, Swain DK, Nigam R, Sharma A, Sharma D, et al. Seminal plasma and sperm membrane proteins of buffalo and cattle bulls: a comparative study. Buffalo Bull. (2016) 35:437–43. Available online at: https://www.cabdirect.org/cabdirect/abstract/20163340558
13. Lu J-C, Jing J, Yao Q, Fan K, Wang G-H, Feng R-X, et al. Relationship between lipids levels of serum and seminal plasma and semen parameters in 631 Chinese subfertile men. PLoS ONE. (2016) 11:e0146304. doi: 10.1371/journal.pone.0146304
14. Divyashree BC, Roy SC. Species-specific and differential expression of BSP-5 and other BSP variants in normozoospermic and asthenozoospermic buffalo (Bubalus bubalis) and cattle (Bos taurus) seminal plasma. Theriogenology. (2018) 106:279–86. doi: 10.1016/j.theriogenology.2017.10.014
15. Willforss J, Morrell JM, Resjö S, Hallap T, Padrik P, Siino V, et al. Stable bull fertility protein markers in seminal plasma. J Proteomics. (2021) 236:104135. doi: 10.1016/j.jprot.2021.104135
16. Rolim Filho ST, Ribeiro HFL, de Camargo GMF, Cardoso DF, Aspilcueta- Borquis RR, Tonhati H, et al. Identification of polymorphisms in the osteopontin gene and their associations with certain semen production traits of water buffaloes in the Brazilian Amazon. Reprod Domest Anim. (2013) 48:705–9. doi: 10.1111/rda.12144
17. Fu Q, Pan L, Huang D, Wang Z, Hou Z, Zhang M. Proteomic profiles of buffalo spermatozoa and seminal plasma. Theriogenology. (2019) 134:74–82. doi: 10.1016/j.theriogenology.2019.05.013
18. Kumar D, Kumar P, Singh P, Yadav SP, Yadav PS. 66 buffalo bull semen-fertility evaluation with motility and integrity of acrosome, plasma membrane, and spermatozoa DNA. Reprod Fertil Dev. (2012) 25:180–180. doi: 10.1071/RDv25n1Ab66
19. NRC. Nutrient Requirements of Dairy Cattle. 7th ed. Washington, DC. USA: National Academies Press (2001).
20. Mortimer D. Sperm recovery techniques to maximize fertilizing capacity. Reprod Fertil Dev. (1994) 6:25–31. doi: 10.1071/RD9940025
21. Fukui Y, Togawa M, Abe N, Takano Y, Asada M, Okada A, et al. Validation of the sperm quality analyzer and the hypo-osmotic swelling test for frozen-thawed ram and minke whale (Balanenoptera bonarensis) spermatozoa. J Reprod Dev. (2004) 50:147–54. doi: 10.1262/jrd.50.147
22. Almadaly E, El-Kon I, Heleil B, Fattouh E-S, Mukoujima K, Ueda T, et al. Methodological factors affecting the results of staining frozen-thawed fertile and subfertile Japanese Black bull spermatozoa for acrosomal status. Anim Reprod Sci. (2012) 136:23–32. doi: 10.1016/j.anireprosci.2012.10.016
23. Marsh WH, Fingerhut B. Serum protein determination by automatic recording refractometry. Clin Chem. (1962) 8:640–6. doi: 10.1093/clinchem/8.6.640
24. Francis GL, Upton FM, Ballard FJ, McNeil KA, Wallace JC. Insulin-like growth factors II and I in bovine colostrums. Sequences and biological activities compared with those of a potent truncated form. Biochem J. (1988) 251:95–103. doi: 10.1042/bj2510095
25. Lone SA, Prasad JK, Ghosh SK, Das GK, Balamurugan B, Sheikh AA, et al. The activity of enzymatic antioxidants and total antioxidant capacity in seminal plasma of Murrah bulls during cryopreservation. J Anim Res. (2016) 63:405–10. doi: 10.5958/2277-940X.2016.00038.3
26. Sinha AK. Determination of catalase activity in white blood. Adv Enzymol Relat Areas Mol Biol. (1971) 27:380–90.
27. Wo D, Zhuang P, Xu ZG, Xu S, Lu Y, Mao HM. A novel spectrophotometric method for indirect determination of nitric oxide (NO) in serum. Clin Chim Acta. (2013) 424:187–90. doi: 10.1016/j.cca.2013.06.008
28. Foreman D, Gaylor L, Evans E, Trella C. A modification of the Roe procedure for determination of fructose in tissues with increased specificity. Anal Biochem. (1973) 56:584–90. doi: 10.1016/0003-2697(73)90225-X
29. Henry RJ. Colorimetric determination of total protein. In: Clinical Chemistry. New York, NY USA: Harper and Row Publishing (1964). p. 181–220. Available online at: https://refhub.elsevier.com/S1382-6689(18)30079-6/sbref0155 (accessed January 5, 2023).
30. Doumas BT, Biggs HG, Arends RL, Pinto PVC. Determination of serum albumin In: Standard Methods of Clinical Chemistry. New York, NY, USA: Academic Press (1972). p. 7:175–88. doi: 10.1016/B978-0-12-609107-6.50022-2
31. Laemmli VK. Cleavage of structural proteins during the assembly of the head of bacteriophage T4. Nature. (1970) 227:680–5. doi: 10.1038/227680a0
32. Bürk RR, Eschenbruch M, Leuthard P, Steck G. Sensitive detection of proteins and peptides in polyacrylamide gels after formaldehyde fixation. Methods Enzymol. (1983) 91:247–54. doi: 10.1016/S0076-6879(83)91021-2
33. Assumpcao TI, Fontes W, Sousa MV, Ricart CA. Proteome analysis of Nelore bull (Bos taurus indicus) seminal plasma. Prot Peptide Lett. (2005) 12:813–7. doi: 10.2174/0929866054864292
34. Singh RK, Kumaresan A, Chhillar S, Rajak SK, Tripathi UK, Nayak S, et al. Identification of suitable combinations of in vitro sperm-function test for the prediction of fertility in buffalo bull. Theriogenology. (2016) 86:2263–71. doi: 10.1016/j.theriogenology.2016.07.022
35. Minervini F, Guastamacchia R, Pizzi F, Dell'Aquila ME, Barile VL. Assessment of different functional parameters of frozen–thawed buffalo spermatozoa by using cytofluorimetric determinations. Reprod Domest Anim. (2013) 48:317–24. doi: 10.1111/j.1439-0531.2012.02152.x
36. Dutta S, Ahmad M, Ashok A. Oxidative stress and sperm function: a systematic review on evaluation and management. Arab J Urol. (2019) 17:87–97. doi: 10.1080/2090598X.2019.1599624
37. Agarwal A, Virk G, Ong C, Plessis SS. Effect of oxidative stress on male reproduction. World J Mens Health. (2014) 32:1–7. doi: 10.5534/wjmh.2014.32.1.1
38. Bansal AK, Bilaspuri GS. Effect of manganese on bovine sperm motility, viability, and lipid peroxidation in vitro. Anim Reprod. (2008) 5:90–6. Available online at: https://www.animal-reproduction.org/article/5b5a6076f7783717068b4795
39. Guthrie HD, Welch GR, Theisen DD, Woods LC. Effects of hypothermic storage on intracellular calcium, reactive oxygen species formation, mitochondrial function, motility, and plasma membrane integrity in striped bass (Morone saxatilis) sperm. Theriogenology. (2011) 75:951–61. doi: 10.1016/j.theriogenology.2010.10.037
40. Barranco I, Padilla L, Tvarijonaviciute A, Parrilla I, Martínez EA, Rodriguez-Martinez H, et al. Levels of activity of superoxide dismutase in seminal plasma do not predict fertility of pig AI-semen doses. Theriogenology. (2019) 140:18–24. doi: 10.1016/j.theriogenology.2019.08.010
41. Miah AG, Salma U, Takagi Y, Kohsaka T, Hamano KI, Tsujii H. Effects of relaxin and IGF-I on capacitation, acrosome reaction, cholesterol efflux and utilization of labeled and unlabeled glucose in porcine spermatozoa. Reprod Med Biol. (2008) 7:29–36. doi: 10.1111/j.1447-0578.2007.00198.x
42. Kumar P, Suman, Pawaria S, Dalal J, Bhardwaj S, Patil S, et al. Serum and seminal plasma IGF-1 associations with semen variables and effect of IGF-1 supplementation on semen freezing capacity in buffalo bulls. Anim Reprod Sci. (2019) 204:101–10. doi: 10.1016/j.anireprosci.2019.03.010
43. Lee HS, Park Y-S, Lee JS, Seo JT. Serum and seminal plasma insulin-like growth factor-1 in male infertility. Clin Exp Reprod Med. (2016) 43:97–101. doi: 10.5653/cerm.2016.43.2.97
44. Souza FA, Martins JA-M, Emerick LL, Laskoski LM, Osorio JP, Souza FF, et al. Seminal plasma insulin-like growth factor I and total protein concentration in peripubertal period of the Gyr bulls. Reprod Domest Anim. (2021) 56:1279–85. doi: 10.1111/rda.13987
45. Griswold MD. Perspective on the function of Sertoli cells. In: Griswold MD, editor. Sertoli Cell Biology. San Diego, CA: Elsevier Science USA (2005). p. 15–8. doi: 10.1016/B978-012647751-1/50003-9
46. Dasrul D, Wahyuni S, Sugito S, Abdulah Hamzah A, Zaini Z, Haris A, et al. Correlation between testosterone concentrations with scrotal circumference, and semen characteristics in Aceh bulls. E3S Web Conf 151. (2020) 01015. doi: 10.1051/e3sconf/202015101015
47. Kavoussi P, Costabile RA, Salonia A. Clinical Urologic Endocrinology: Principles for Men's Health. London, UK: Springer (2012). Available online at: https://link.springer.com/book/10.1007/978-1-4471-4405-2 (accessed January 5, 2023).
48. Shimon I, Lubina A, Gorfine M, Ilany J. Feedback inhibition of gonadotropins by testosterone in men with hypogonadotropic hypogonadism: comparison to the intact pituitary-testicular axis in primary hypogonadism. J Androl. (2006) 27:358–64. doi: 10.2164/jandrol.05140
49. Pruneda A, Pinart E, Bonet S, Yeung CH, Cooper TG. Study of the polyol pathway in the porcine epididymis. Mol Reprod Dev. (2006) 73:859–65. doi: 10.1002/mrd.20481
50. Ford WC. Glycolysis and sperm motility: does a spoonful of sugar help the flagellum go round? Hum. Reprod Update. (2006) 12:269–74. doi: 10.1093/humupd/dmi053
51. Mahsud T, Jamil H, Qureshi ZI, Asi MN, Lodhi LA, Waqas MS, et al. Semen quality parameters and selected bio-chemical constituents level in plasma of Lohi rams. Small Rumin Res. (2013) 113:175–8. doi: 10.1016/j.smallrumres.2013.04.004
52. Elzanaty S, Erenpreiss J, Becker C. Seminal plasma albumin: origin and relation to the male reproductive parameters. Andrologia. (2007) 39:60–5. doi: 10.1111/j.1439-0272.2007.00764.x
53. Srivastava N, Srivastava SK, Ghosh A, Kumar P, Perumal P, Jerome A. Acrosome membrane integrity and cryocapacitation are related to cholesterol content of bull spermatozoa. Asian Pac J Reprod. (2013) 2:126–31. doi: 10.1016/S2305-0500(13)60132-3
54. El-Sayed RA, El-Badry DA, Abou-Ahmed MM, Ghallab AM. Studying the lipids, antioxidants, and enzymatic profiles in seminal plasma and blood serum of buffalo bulls in relation to fresh semen quality. J Egypt Vet Med Assoc. (2014) 74:147–61.
55. Lahnsteiner F, Patzner R, Weismann T. The testicular main duct and the spermatic duct in motility. II Composition of the seminal fluid. J Fish Biol. (1994) 44:459–67. doi: 10.1111/j.1095-8649.1994.tb01226.x
56. Moura AA, Koc H, Chapman DA, Killian GJ. Identification of accessory sex gland fluid proteins as related to fertility indexes of dairy bulls: a proteomic approach. J Androl. (2006) 27:201–12. doi: 10.2164/jandrol.05089
57. Moura AA, Chapman DA, Koc H, Killian GJ. A comprehensive proteomic analysis of the accessory sex gland fluid from mature Holstein bulls. Anim Reprod Sci. (2007) 98:169–88. doi: 10.1016/j.anireprosci.2006.03.012
58. Desnoyers L, Thêrien I, Manjunath P. Characterization of the major proteins of bovine seminal fluid by two dimensional polyacrylamide gel electrophoresis. Mol Reprod Dev. (1994) 37:425–35. doi: 10.1002/mrd.1080370409
59. Killian GJ, Chapman DA, Rogowski LA. Fertility-associated proteins in Holstein bull seminal plasma. Biol Reprod. (1993) 49:1202–7. doi: 10.1095/biolreprod49.6.1202
60. Gwathmey TM, Ignotz GG, Mueller JL, Manjunath P, Suarez SS. Bovine seminal plasma proteins PDC-109, BSP-A3, and BSP-30-kDa share functional roles in storing sperm in the oviduct. Biol Reprod. (2006) 75:501–7. doi: 10.1095/biolreprod.106.053306
61. Erikson WD, Way LA, Chapman AD, Killian JG. Detection of osteopontin on Holstein bull spermatozoa, in cauda epididymal fluid and testis homogenates, and its potential role in bovine fertilization. Reproduction. (2007) 133:909–17. doi: 10.1530/REP-06-0228
62. Gerena RL, Eguchi N, Urade Y, Killian GJ. Stage and region-specific localization of lipocalin-type prostaglandin D synthase in the adult murine testis and epididymis. J Androl. (2000) 21:848–54. Available online at: https://pubmed.ncbi.nlm.nih.gov/11105911/
Keywords: antioxidants, buffalo bulls, fertility biomarkers, hormones, proteomic, sperm kinematics
Citation: Almadaly EA, Abdel-Salam A-BS, Sahwan FM, Kahilo KA, Abouzed TK and El-Domany WB (2023) Fertility-associated biochemical components in seminal plasma and serum of buffalo (Bubalus bubalis) bulls. Front. Vet. Sci. 9:1043379. doi: 10.3389/fvets.2022.1043379
Received: 13 September 2022; Accepted: 23 December 2022;
Published: 17 January 2023.
Edited by:
Abouzar Najafi, University of Tehran, IranReviewed by:
Syed Aftab Hussain Shah, Pakistan Scientific and Technological Information Center (PASTIC), PakistanCopyright © 2023 Almadaly, Abdel-Salam, Sahwan, Kahilo, Abouzed and El-Domany. This is an open-access article distributed under the terms of the Creative Commons Attribution License (CC BY). The use, distribution or reproduction in other forums is permitted, provided the original author(s) and the copyright owner(s) are credited and that the original publication in this journal is cited, in accordance with accepted academic practice. No use, distribution or reproduction is permitted which does not comply with these terms.
*Correspondence: Essam A. Almadaly, ZHJfZXNzYW1fMjAwNUB5YWhvby5jb20=;
ZXNzYW0ubW9zdGFmYUB2ZXQua2ZzLmVkdS5lZw==
Disclaimer: All claims expressed in this article are solely those of the authors and do not necessarily represent those of their affiliated organizations, or those of the publisher, the editors and the reviewers. Any product that may be evaluated in this article or claim that may be made by its manufacturer is not guaranteed or endorsed by the publisher.
Research integrity at Frontiers
Learn more about the work of our research integrity team to safeguard the quality of each article we publish.