- 1Department of Food Control, Faculty of Veterinary Medicine, Zagazig University, Zagazig, Egypt
- 2Department of Anatomy and Embryology, Faculty of Veterinary Medicine, Menoufia University, Shebin Alkom, Egypt
- 3Department of Nutrition and Animal Husbandry, University of Veterinary Medicine and Pharmacy, Košice, Slovakia
- 4Department of Husbandry and Development of Animal Wealth, Faculty of Veterinary Medicine, Menoufia University, Shebin Alkom, Egypt
- 5Department of Pathobiochemistry, Faculty of Pharmacy, Meijo University Yagotoyama, Nagoya-shi, Japan
Fish is one of the most valuable foods with high-quality animal protein. However, aquaculture, or ingesting contaminated food, allows organochlorine pesticides (OCPs) to enter the fish's body, and therefore, it negatively impacted public health. One-hundred and twenty random samples of Clupea harengus (C. harengus), Mugil cephalus (M. cephalus), Sardinella aurita (S. aurita), Oreochromis niloticus (O. niloticus), Neptunus pelagicus (N. pelagicus) and Sepia savigngi (S. savigngi) (n = 20 each) were collected from local markets in Mansoura city, Egypt. Samples were checked to see whether any residues of OCPs with the application of risk assessment due to their consumption by Mansoura citizens. The findings indicated that summation hexachlorocyclohexane (∑HCH) in examined seafood samples ranged from 0.27 ± 0.13 in N. pelagicus to 61.61 ± 52.03 μg.kg−1 in S. aurita. Also, the γ-HCH isomer was considered the more prominent among isomers. Hexachlorobenzene (HCB) was found in five different species, with mean values of 2.03 ± 1.85, 1.5.7 ± 1.17, 0.94 ± 0.87, 0.35 ± 0.06, and 0.18 ± 0.06 μg.kg−1 in C. harengus, S. aurita, M. cephlaus, O. niloticus, and S. savigngi. Moreover, summation of Heptachlors (∑HPTs) was 10.19 ± 7.63, 1.27 ± 0.26, 2.58 ± 0.11, 0.95 ± 0.12, 0.21 ± 0.11 and 0.32 ± 0.03 μg.kg−1 of wet weight in examined C. harengus, M. cephlaus, S. aurita, O. niloticus, N. pelagicus, and S. savigngi. Aldrin and dieldrin residues were 3.75 ± 1.31 and 4.86 ± 1.33 μg.kg−1 in C. harengu, meanwhile they were 1.61 ± 0.77 and 0.78 ± 0.04 μg.kg−1in M. cephalus. Dichlorodiphenyldichloroethylene (pp-DDE) was dominant in all examined species within different concentrations 5.08 ± 4.12, 0.98 ± 0.10, 3.07 ± 0.91, 0.93 ± 0.27, 0.08 ± 0.01 and 0.35 ± 0.02 μg.kg−1 in C. harengus, M. cephlaus, S. aurita, O. niloticus, N. pelagicus and S. savigngi, respectively. We concluded that all examined seafood samples were lower than the recommended maximum residue limit. Also, the estimated daily intake was less than the permitted daily intake. Non-carcinogenic indices of target hazard quotient and hazard index for OCPs in all examined species were less than 1.
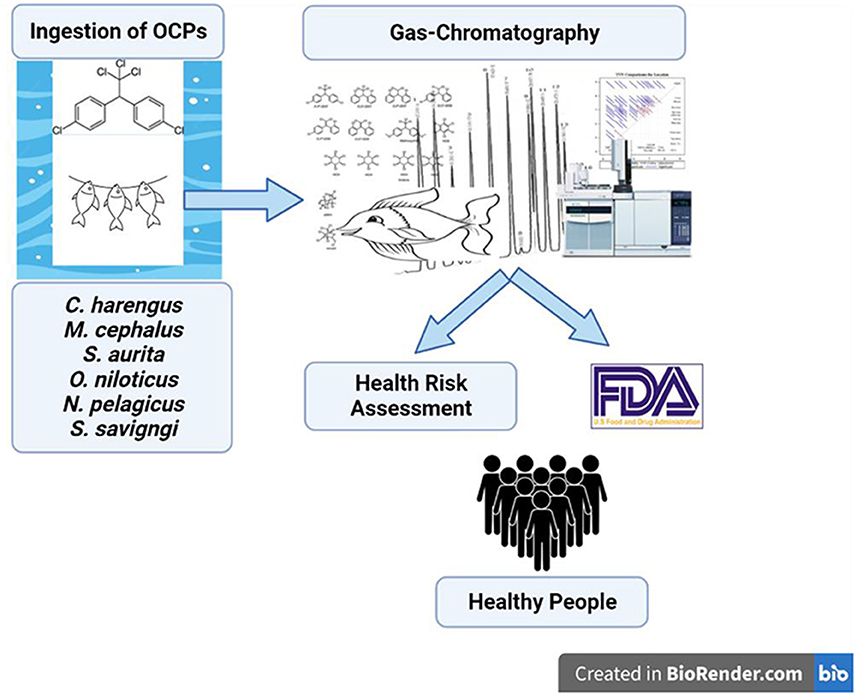
Graphical Abstract Schematic cartoon of the experimental protocol – Created on BioRender.com.
Introduction
Fish is considered one of the most valuable foods because it provides Egyptian consumers with high biological value animal protein while also addressing the issue of animal meat scarcity (1). The total fish production in Egypt was 2.2 MMT (million metric tons) in 2021 with aquaculture share at 1.7 MMT of total production. Egypt has a promising plan to raise fish production to 3 MMT by 2025 (2). Moreover, fish consumption is strongly advised for children's nutrition, and therefore, the safety and quality of the product should always be checked to safeguard public health (3). The unpredictable and flighty utilization of pesticides in horticulture creates ecological issues; particularly for the aquatic environment by changing the nature of water and influencing the biochemical and physiological merits of non-target fish (4). Different routes, such as direct contact with water or ingesting contaminated food, allow organochlorine pesticides (OCPs) to enter the fish's body (5). The pollution load in fish is affected by the level of pollution in the water. As a result, fish are the most important bioindicators of pesticide contamination in water bodies (6). OCPs, that remains in the environment, bioaccumulate in organisms, and are hazardous to both humans and wildlife, becoming a major worry for the world in recent years. OCP bioaccumulation in the biota can have negative consequences for immunity, the neurological system, and reproduction (7). The OCPs have lipophilic nature, hydrophobicity, and low chemical and biological degradation rates that prompt their bioaccumulation and subsequent magnification in beings of the food chain (8). The primary cause of pesticide pollution is food contaminated with pesticides. It was assumed that because fish is the final animal in the food chain, it is responsible for more than 80% of all pesticide residues consumed by humans. It is the one most affected by it, and society as a whole has subsequently grown more worried about the potential damage to human health posed by eating such contaminated biota (9). Furthermore, several recent studies in Japan and China have observed a correlation between eating fish and serum organochlorine levels in humans (10, 11). Since late 1990, the importation and use of OCPs have been outlawed in Egypt after more than 50 years of use in agriculture and public health. However, some OCPs, including DDT and -HCH (lindane), are still used illegally by farmers due to their low cost, simplicity of use, and ability to effectively eradicate pests (12, 13). Egypt reportedly utilized 45,000 MT of toxaphene (1955–1961), 10,500 MT of endrin (1961–1981), 13,500 MT of DDT (1952–1971), and 11,300 MT of lindane (1952–1978) over 30 years, switching between the various chemicals regularly (14). Therefore, the objective of this study was to assess the presence of OCPs and public health hazards associated with the consumption of C. harengus, M. cephalus, S. aurita, O. niloticus, N. pelagicus, and S. savigngi marketed in Mansoura city, Egypt.
Materials and methods
Collection and preparation of samples
One-hundred and twenty samples of C. harengus, M. cephalus, S. aurita, O. niloticus, N. pelagicus, and S. savigngi (n = 20 each) were collected randomly from local markets in Mansoura, Egypt. C. harengus is usually imported from Holland. However, O. niloticus and M. cephalus are usually farmed in Egypt, S. pilchards from the Mediterranean Sea, and S. savigngi and N. pelagicus from the Red Sea. The fish samples were collected from the Mansoura City fish market in Egypt (marketed fish for human consumption). We study the safety of fish for consumers – The fish sample collection site is not the same place for fishing so we are of not need a map. Those samples were maintained in an ice box and directly shipped to the Department of Food Control, Faculty of Veterinary Medicine, Zagazig University. To get rid of dirt and other entrapped materials before being distinguished and given remarkably recognizable proof codes, the samples were washed many times with deionized water. Using a stainless-steel knife, gut each fish sample to remove the intestines, scales, head, tails, fins, and bones. Remove the edible portion of the samples (50 g) for extraction and cleaning procedures.
Chemicals
Sigma-Aldrich Chemie GmbH provided standard OCPs such as pp-DDT, pp-DDD, pp-DDE, HCH, heptachlor, heptachlor epoxide, aldrin, endrin, chlordane, methoxychlor, and HCB (Kappelweg, Schnelldor, Germany). Other chemicals were purchased from Merck and were of the highest quality available (Darmstadt, Germany). Dichloromethane (DCM) was purchased from J.T. Baker (Phillipsburg, NJ, USA). Acetonitrile (ACN, GC-MS SupraSolv®) and n-hexane (HEX, Ultra Resi-Analyzed) were purchased from Merck KGaA (Darmstadt, Germany).
Sample extraction, preparation, partitioning, and cleaning
In Egypt, the consumer eats the muscle of fish only. Also, the regulation and risk analysis adopted for muscle. Each muscle sample (50 g) was mixed with anhydrous sodium sulfate (100 g) and petroleum ether for 2 min for three successive extraction steps (15). Samples were dried by solvent evaporation in a rotary evaporator at 40°C after filtration. During the portioning of the extracted samples, according to the previous report (16). The extracted samples were separated using an 80:20 n-hexanes and acetonitrile solution in a volume of 100 mL. The acetonitrile layer was collected and evaporated on a rotary evaporator to a 10 mL volume for use in Florisil cleanup after three partitioning cycles. The Florisil was provided by Silica (Silica Co., USA). The solvents were all of pesticide quality. Florisil was activated at 130°C for 24 h and then cooled at 25°C. A chromatographic column containing 20 g of activated Florisil was used to clean the extracted samples. The eluent was cleaned up, evaporated on a rotary evaporator, and then dissolved in 10 mL of hexane. An aliquot of each extract was transferred to 2 mL injection vials in order to get it ready for the electron capture gas chromatography examination (17).
Determination of OCPs concentrations
The maximum residue limit (MRL) of OCPs is proposed by the Food and Drug Administration (FDA) since the MRL has not been established by the WHO yet (12, 13). Organochlorine pesticide residues were evaluated using electron capture gas chromatography (Hewlett Packard GC Model 6890) equipped with a Ni63-electron capture detector. An HP- 5MS capillary column (30 m in length, 0.32 mm inner diameter, and 0.25 μm film thickness, with N2 as the carrier gas and a flow rate of 4 mL/min, respectively) was used in the gas chromatograph (GC). The extract was injected into a single input that was split into two columns. The instrumental settings were as follows: The temperature program for the gas chromatography oven began at 150°C for 5 min, increased to 170°C at a rate of 5 °C/min and held for 10 min, then increased to 220°C at a rate of 10°C/min and held for 20 min (with a total run time of 44 min); the injection volume was 1 ll and the flow rate of nitrogen gas was 20 mL/min. Analytical process quality control was carried out based on a comparison with the developed standard curves and injector and detector temperatures of 230°C. To verify the analytical procedures, the standard reference material, SRM 1947 (Lake Michigan Fish Tissue), was handled and inspected comparably to the samples. Recovery rates for the examined OCPs ranged from 88 to 106%.
Health risk assessment
Estimated daily intake (EDI) was determined by factoring in data on organochlorine analyses, fish intake patterns, and adult Egyptian body weight. (μg/kg/day) was obtained following the subsequent equation: EDI = (Cm x FIR)/BW. Where, Cm = organochlorine concentration in fish (μg.kg−1), FIR = the food ingestion rate, estimated at 48.57g/day (18), and BW = the average body weight of an adult is 70 kg, then compared to acceptable daily intakes (ADIs).
The target hazard quotient (THQ) was employed to describe the health risk that the consumption of seafood poses to the Egyptian population. The exposure to reference dosages (RfD) ratio is shown below, refers to the amount of a contaminant that a person could be exposed to every day for the rest of their life without significantly increasing the probability that it would cause harm. Rfd was 0.0003, 0.0008, 0.0005, 0.00003, 0.0005, and 0.0005 (g/g bw/day), respectively, for HCH, HCB, HPTs, Drins, CHLs, and DDTs (19, 20). If the ratio is less than 1, the population would not be at risk; if it's equal to or higher than 1, the population will be at risk of health problems. It uses the equation shown below (21):
THQ stands for target hazard quotient, EF for exposure frequency (365 days annually), and ED for exposure duration (average lifetime of 70 years); FIR stands for food ingestion rate (g/day); C stands for organochlorine concentration in fish (g/g); RfD stands for oral reference dose (mg/kg/day); BW stands for average adult body weight (70 kg); and AT stands for the average exposure time (365 days /year, assuming a lifespan of 70 years).
The hazard index (HI) has been performed to assess the probable human health hazard of more than one organochlorine. The HI refers to the sum of all THQ for various organochlorine exposures as shown in the equation below: HI = ΣTTHQs = THQ HCH + THQ HCB + THQ HPTs +THQ Drins +THQ CHLs +THQ DDTs where Σ TTHQs is the target hazard quotients of all organochlorines; while, the hazard index becomes over 1, the possible human health risk is expected (22).
Statistical analysis
A completely randomized design (CRD) was used to statistically evaluate the data with a significance level of P ≤ 0.05. SAS 9.3 was used for all statistical analyses (SAS Institute Inc., Cary, NC, USA). The information was displayed as means and relative standard deviation (RSD).
Results and discussion
Organochlorine pesticide residues
There are five main isomeric forms of hexachlorocyclohexane (HCH): α-HCH, β-HCH, γ-HCH, δ-HCH, and ε-HCH. The most common isomer of HCH is called α-HCH (23). Among these isomers, only the γ-HCH isomer, which is sold as Lindane, has insecticidal properties (24). The main component of several commercial medications used to treat scabies and head lice in children is lindane, which is manufactured as a shampoo or lotion (25). Also, the γ-HCH has been used to treat soil, animals, pets, forestry products, seeds, and seedlings, according to the previous report (26). Because HCHs are particularly poisonous to aquatic organisms, they are highly susceptible to bioaccumulation (27). The downward concentrations of ∑HCH in seafood samples were determined, S. aurita (61.61 ± 52.03 μg.kg 1) > C. harengus (19.7 ± 11.64 μg.kg−1) > O. niloticus (2.32 ± 0.83 μg.kg−1) > M. cephlaus (1.33 ± 0.74 μg.kg−1) > S. savigngi (1.43 ± 0.70 μg.kg−1) > N. pelagicus (0.27 ± 0.13 μg.kg−1) as shown in Table 1. The ∑HCH was detected previously in seafoods worldwide at concentrations 38.82 μg.kg−1 in Kenya (from twelve fish species; Lethrinus “harak, ribulose”, Siganus rivulatus, Litianus russeli, Tilapia zilli, Mondaclilus argentus, Epinephelus Caeruleopantantus, Carcharhinus macloti, Valamugil buchsrhinus, Sardinella fimbrita, Penaeus sp., Apolectus niger, and Pampus argenteus) (28). Moreover, ∑HCH was 62.95 μg.kg−1 in Cote d'Ivoire (from two species of fish, Oreochromis niloticus, and Chrysichthys nigrodigitatus) (29). Also, ∑HCH was 6910 μg.kg−1 in Nigeria (from three species of fish, Tilapia zilli, Ethmalosa fimbriata, and Chrysichthys nigrodigitatus) (30). Additionally, ∑HCH was 23.8 μg.kg−1 in Turkey (from eighteen fish species (trout, red mullet, bluefish, gilt-headbream, sea bass, horse mackerel, goby, gray mullet, gurnard, greater amberjack, common sea bream, whiting, bonito, pilchard, native mackerel, import mackerel, pike perch, and garfish) (31). In Africa, ∑HCH was 15.44 μg.kg−1 in Ghana (from six fish species, Heterotis niloticus, Channa obscura, Hepsetus odoe, Tilapia zilli, Clarias gariepinus, and Chrysichthys nigrodigitatus) (32), 1.1 μg.kg−1 in Ethiopia (from six fish species, Oreochromis niloticus, Clarias gariepinus, Barbus intermedius, Barbus paludinosus, Garra quadrimaculata, and Aplocheilichthyes antinorii) (17) and 0.88 μg.kg−1 in Egypt (from Tilapia nilotica) (33). Regarding HCH isomer concentration in this study, γ-HCH isomer was considered the more prominent between isomers. Nearly similar findings for α-HCH, β-HCH, γ-HCH and δ-HCH were 0.22, 0.17, 0.52, and 0.19 μg.kg−1 in Ethiopian fish (17). Also, β-HCH and γ-HCH were 5.5 and 25.38 μg.kg−1 in fish samples collected from Ghana (34). Regarding the obtained results, all examined samples were below the MRL 300 μg.kg−1 (35).
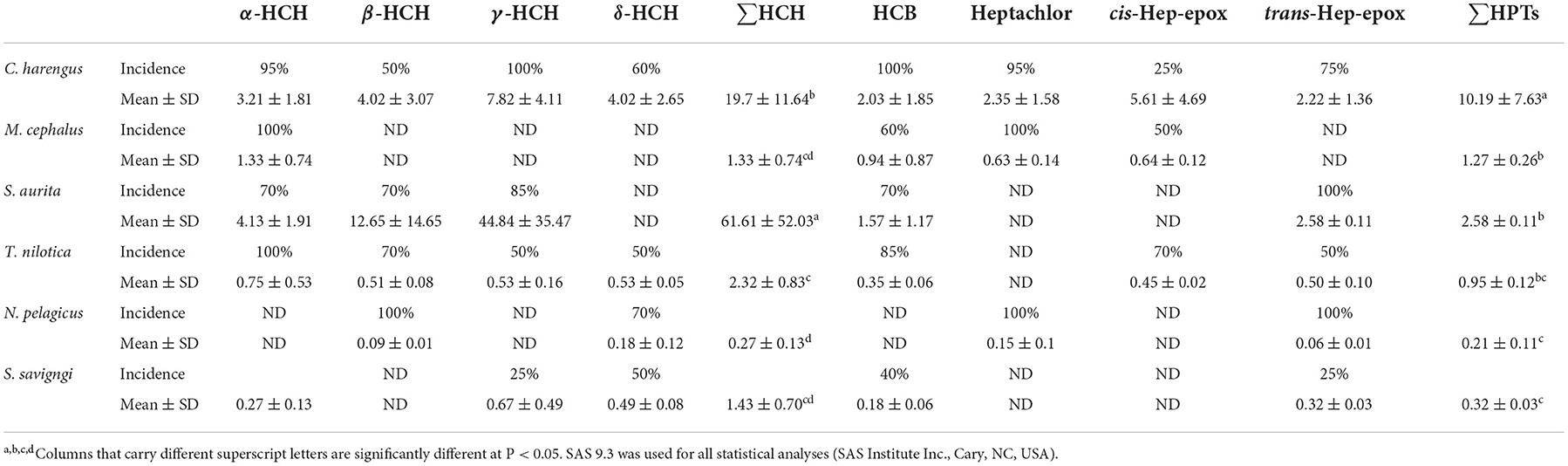
Table 1. Incidence and residual level (mean ± SD) of Hexachlorocyclohexane (HCH), Hexachlorobenzene (HCB), and Heptachlor (HPTs) in examined seafood species (μg.kg−1) of wet weight (n = 20 each).
Hexachlorobenzene (HCB), chlorinated benzene, has been applied to wheat seeds as a fungicide to prevent fungal infections. Since its introduction as a fungicide in 1945, HCB has been detected in all forms of food as a result of the accumulation of this substance in high-fat foods, such as dairy products, eggs, animal fats, and some fish (36). There has been a continuous decrease in the amount of HCB in the environment during the last 20–25 years. However, there is still a lot of this chemical in the environment (37). The HCB residues were detected in 100, 70, 60, 85, and 40% with mean values of 2.03 ± 1.85, 1.5.7 ± 1.17, 0.94 ± 0.87, 0.35 ± 0.06 and 0.18 ± 0.06 μg.kg−1 in examined C. harengus, S. aurita, M. cephlaus, O. niloticus, and S. savigngi, respectively. Meanwhile, it was not detected in N. pelagicus (Table 1). All examined samples were below the MRL 200 μg.kg−1 (38). C. harengus significantly contained higher HCB residues than other species (P < 0.05). The findings show that species-specific ecological traits, such as eating patterns and habitat, have a role in the bioaccumulation of OCPs in fish. HCB residues of 0.80–1.68 μg.kg−1 were in Spain (39) and 1.78 μg.kg−1 was detected in fish caught off the coast of South Africa (40). Meanwhile, higher HCB residues of 175.10 μg.kg−1 were detected in fish samples from Cote d'Ivoire (29), and 22.93 μg.kg−1 was detected in Nigerian fish samples (41). There have been reports of lower HCB levels in Italian and European markets (mean: 0.06; range: 0.03–0.15 g.kg−1 of wet weight) (42).
Heptachlor is a cyclodiene pesticide used to control termites and as an insecticide on food and seed crops (24). The primary metabolite of heptachlor, heptachlor epoxide, is quite persistent in soil. 14–16 years after treatment, traces of heptachlor epoxide have occasionally been detected in soil. Heptachlor epoxide can be directly absorbed by plants from the soil, and it bioaccumulates in mammals. In more than 60 nations, it has been outlawed or regulated (43). Three isomers heptachlor, cis-Heptachor-epoxide, and trans-Heptachlor-epoxide were detected only in C. harengus examined samples at concentrations 2.35 ± 1.58, 5.61 ± 4.69 and 2.22 ± 1.36 μg.kg−1of wet weight, respectively indicate the past and recent exposure to heptachlor. While trans-Heptachlor-epoxide S. aurati and S. savigngi at concentrations 2.58 ± 0.11 and 0.32 ± 0.03 μg.kg−1 of wet weight indicate the past exposure. The summation of heptachlor (∑HPTs) were 10.19 ± 7.63, 1.27 ± 0.26, 2.58 ± 0.11, 0.95 ± 0.12, 0.21 ± 0.11 and 0.32 ± 0.03 μg.kg−1 of wet weight in examined C. harengus, M. cephlaus, S. aurita, O. niloticus, N. pelagicus, and S. savigngi, respectively (Table 1). The difference between C. harengus and the other investigated species was significant (P < 0.05), which may be related to its ongoing exposure to heptachlor in the environment. All examined species were below the permissible limits for heptachlor in edible seafood at 300 μg.kg−1of wet weight (35). The herein ∑HPTs in this study were lower than 35.33 μg.kg−1 in fish collected from Cote d'Ivoire (29) and 40.18 μg.kg−1 in those from Nigeria (41). The lower ∑HPTs were reported in Ethiopia and Egypt at 0.57 and 0.65 μg.kg−1 (17, 33).
Aldrin and dieldrin are substances that have been extensively utilized in public health applications to stop mosquito-borne diseases including malaria and sleeping sickness as well as to control insects in agriculture (24). Aldrin changes into dieldrin in living systems, while dieldrin is resistant to bacterial and chemical destruction outside of living systems (32). Dieldrin's stereoisomer, endrin, exists. It tends to be stationary, adsorbs soil particles, and is hydrophobic. Endrin has largely been utilized as an avicide, rodenticide, and insecticide (44). The aldrin residues were detected in 20%, 85%, 50% and 100% with mean values of 3.75 ± 1.31, 1.61 ± 0.77, 0.88 ± 0.31 and 0.09 ± 0.01 μg.kg−1 in C. harengus, M. cephalus, O. niloticus, and N. pelagicus, respectively. Aldrin residues were found in Turkey, Nigeria, and Ghana at quantities ranging from 3.3 to 26.1, 18.47, and 1.02 μg.kg−1 (31, 34, 41). The dieldrin mean values were 4.86 ± 1.33, 0.78 ± 0.04 and 0.55 ± 0.02 μg.kg−1 in C. harengus, M. cephalus, and O. niloticus, respectively. On the contrary, Table 2 demonstrates that not all samples had endrin. The results can be explained by the previous report (24), which reported that endrin was extremely tenacious, but when exposed to sunshine, it partially breaks down into endrin ketone and endrin aldehyde. Higher dieldrin residues were obtained at 109.38 and 3,098 and 67.96 μg.kg−1 in Kenya and Nigeria (28, 45). Meanwhile, lower values 0.04 and 0.02 μg.kg−1 in Ghana and Ethiopia (46, 47). All obtained values were lower than the permissible according to the previous report (35), Aldrin, dieldrin, and endrin in fish did not exceed 300 μg.kg−1.
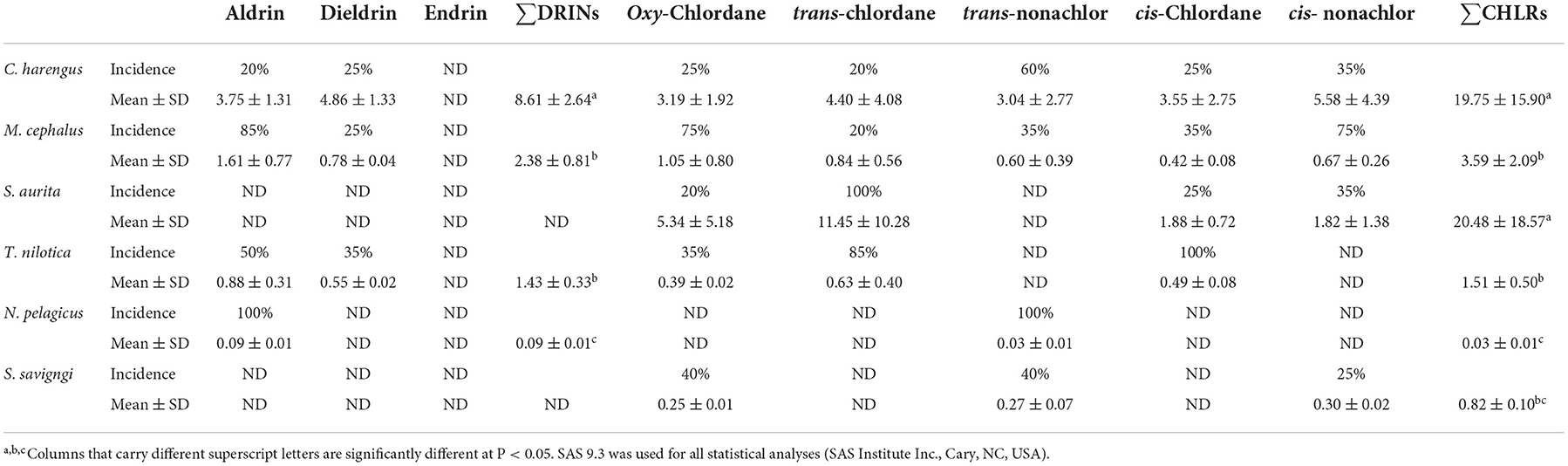
Table 2. Incidence and residual level (mean ± SD) of Drins and Chlordane in examined seafood species (μg.kg−1) of wet weight (n = 20 each).
A popular brand of cyclodiene insecticides known as chlordane has been used extensively as termiticides and pesticides on residential properties and agricultural food crops (48). It can keep a strong bond with soil particles for up to 20 years and is extremely hydrophobic (49). The recorded data in Table 2, showed that the summation of chlordane residues (∑CHLRs) was 19.75 ± 15.90, 3.59 ± 2.09, 20.48 ± 18.57, 1.51 ± 0.50, 0.03 ± 0.01 and 0.82 ± 0.10 μg.kg−1 in examined C. harengus, M. cephlaus, S. aurita, O. niloticus, N. pelagicus, and S. savigngi, respectively. Chlordane residues were detected in fish samples from Cote d'Ivoire, Ghana, and Iran at concentrations 43.73, 3.44, and 0.083 μg.kg−1 (29, 32, 50). S. savigngi and N. pelagicus had much lower levels of chlordane residues than the other species under study (P < 0.05), which is likely due to their lower trophic status compared to fish (51). Regarding the acceptability of fish samples, all obtained chlordane values were lower than the permissible 300 μg.kg−1 according to the previous report (35).
Dichlorodiphenyltrichloroethane (DDT) was extensively used since its registration in 1946. DDT was primarily employed in agriculture to control pests that affected crop and livestock output, as well as in public health to combating the spread of the bubonic plague and malaria (52). According to reports from the WHO, DDT usage in global malaria prevention programs spared the deaths of more than five million people (53). The pp-DDE was dominant in all examined species within different concentrations of 5.08 ± 4.12, 0.98 ± 0.10, 3.07 ± 0.91, 0.93 ± 0.27, 0.08 ± 0.01 and 0.35 ± 0.02 μg.kg−1 in C. harengus, M. cephlaus, S. aurita, O. niloticus, N. pelagicus, and S. savigngi, respectively. Meanwhile, PP-DDT was detected only in three species 3.35 ± 2.84, 0.85 ± 0.05, and 6.53 ± 4.33 μg.kg−1 in C. harengus, M. cephlaus, and S. aurita, respectively (Table 3). The obtained results coincided with the authors (54) noted that epidemiological studies have used DDE as a biomarker for DDT exposure, also, agrees with the authors (55) who found pp-DDE in every fish sample from Ghana's Volta Lake. PP-DDE has also been found in fish muscle tissues from Lake Parishan in Iran (56).
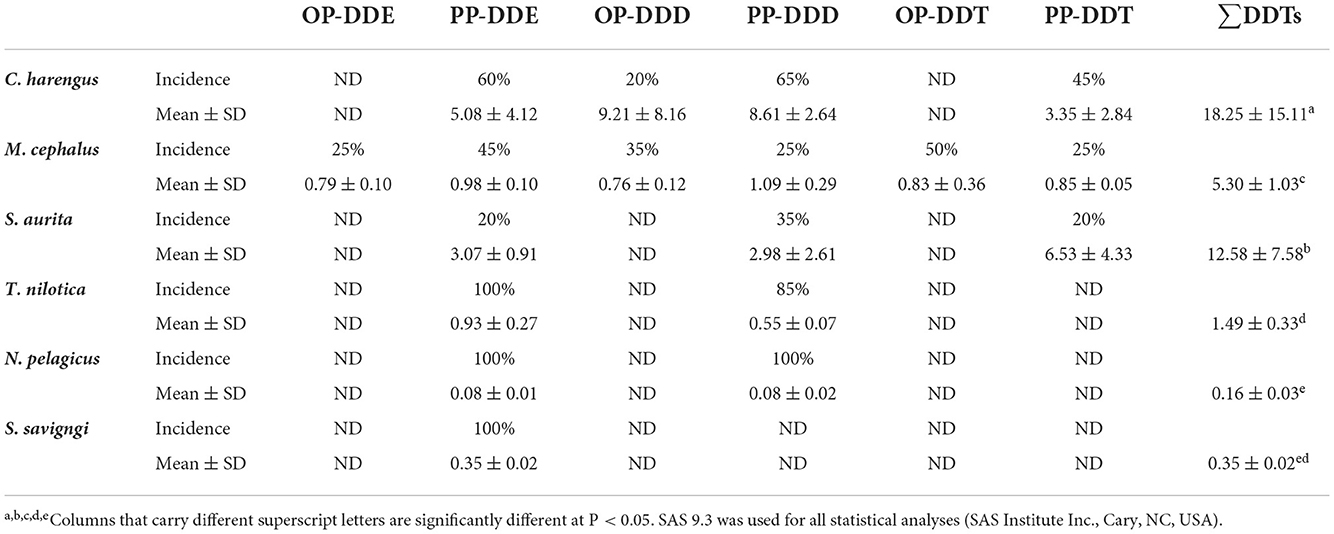
Table 3. Incidence and mean residual level (Mean ± SD) of Dichlorodiphenyltrichloroethane (DDT) in examined seafood species (μg.kg−1) of wet weight (n = 20 each).
The recorded results in Table 3 revealed that ∑DDTs metabolites in descending form were 18.25 ± 15.11, 12.58 ± 7.58, 5.30 ± 1.03, 1.49 ± 0.33, 0.16 ± 0.03 and 0.35 ± 0.02 μg.kg−1 in examined C. harengus, S. aurita, M. cephlaus, O. niloticus, N. pelagicus, and S. savigngi, respectively. The ∑DDTs in fish samples from different countries were 140.63, 109.35, 0.8–82.9, 743, 8.97, and 6 in Kenya, Cote d'Ivoire, Turkey, Ethiopia and Nigeria (28, 29, 31, 45, 47, 57). The ∑DDTs in all examined species were below the MRL 1000 ppb (58).
The discrepancies between the tested species could often be attributed to variations in environment and feeding strategies. It was also found that higher trophic species had a considerable increase in OCPs in their muscle compared to lower trophic species (59). Herein, in descending order, the contribution percentages of each OCP category collectively among species arranged as 34.485, 20.43 19.88, 12.32, 9.44, and 3.45 % for HCH, DDTs, CHLRs, HPTs, DRINs, and HCB, respectively (Figure 1). More information on the concentration percentages of the OCPs categories of each fish species is represented in Supplementary Figure 1. The obtained results in this study, like the results obtained previously (60) have revealed that Stizostedion lucioperca in Beysehir Lake has the highest pesticide residues overall for HCH derivatives. Another study in Turkey revealed that DDTs are the highest contaminant among OCPs (31).
Risk assessment of detected organochlorine pesticide residues on human health
Fish consumption is thought to be a significant source of OCPs in humans. The potential for human exposure to OCPs was calculated using EDIs, the mean concentration of the target pollutants in fish. The data in Table 4 demonstrated that the EDIs of OCPs through eating various species of fish were significantly lower than the ADIs (61). The EDIs are low or on par with those in seafood (62, 63). The EDIs for HCHs in N. pelagicus and S. savigngi were nearly similar to seafood collected from Quanzhou and Xinghua Bay, Southeast China 0.00013 and 0.00024 (μg/kg/day), respectively. Also, herein EDIs of DDTs in C. harengus were comparable to the levels found in Nigeria, which were 0.012 and 0.013 (μg/kg/day), respectively (64). Meanwhile, the EDI of OCPs due to the consumption of fish collected from the Owan River in Nigeria exceeded the allowable daily average intake as a result of eating fish from the Owan River (65).
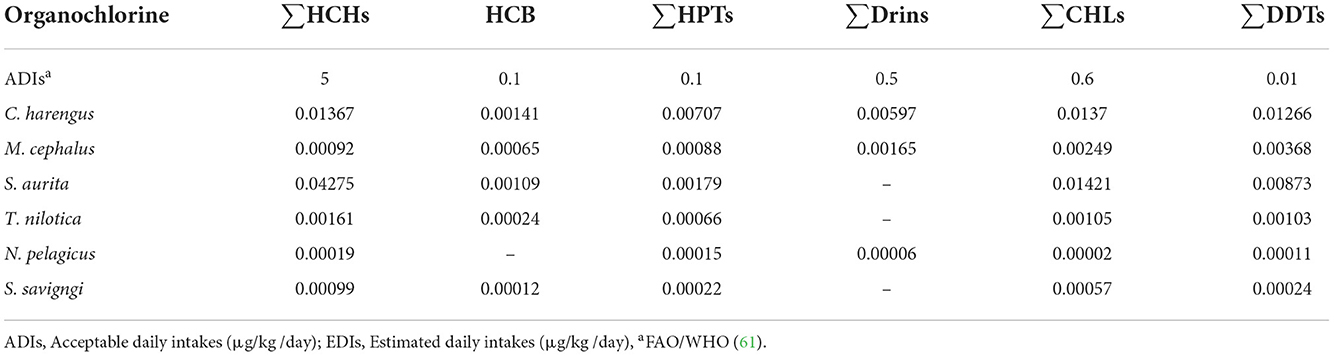
Table 4. Estimated daily intake (EDI μg/kg /day) of Organochlorine pesticides for Mansoura citizens, Egypt, due to seafood consumption in comparison to recommended acceptable daily intake (ADIs μg/kg /day).
The non-carcinogenic indices of THQ and HI for OCPs in all examined species below or more than 1, are given in Table 5 and Figure 2, respectively, which indicate that consuming some fish species has no major damage to one's health. Nearly similar results from Wuhan, China, and South China revealed that THQ and HI were below 1 (66, 67). On contrary, THQ and HI exceeded 1 in many African countries, Nigeria's Lake Chad HI was 34.34 (45), Lagos Lagoon HI was 12.01 (30), and Lake Geriyo HI was 16.53 (68).
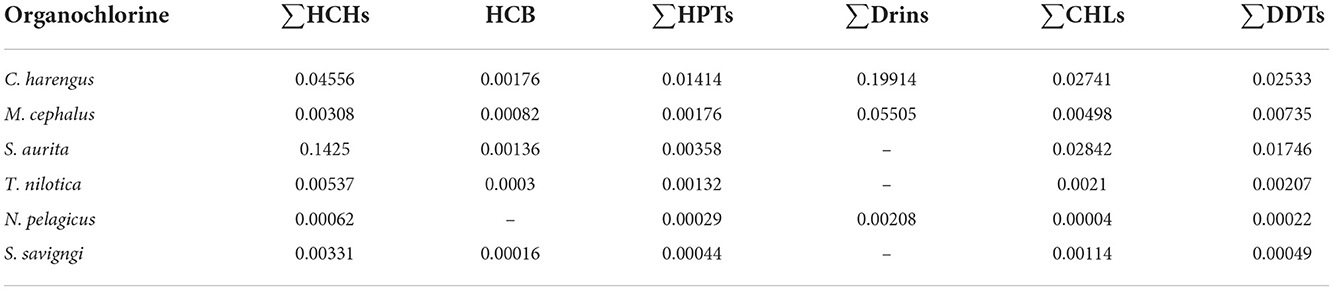
Table 5. Target hazard quotient (THQ) of organochlorine pesticides for Mansoura citizens, Egypt, due to seafood consumption.
Conclusion
The level of OCPs in the edible tissue of seafood was lower than the regulatory level. Overall, the health risk associated with OCP exposure through seafood consumption was negligible. The findings demonstrated that organochlorines were under the FAO's permissible range.
Data availability statement
The original contributions presented in the study are included in the article/Supplementary material, further inquiries can be directed to the corresponding authors.
Ethics statement
The animal study was reviewed and approved by Institutional Review Board Statement: Animal Experimental Guidelines were followed, and the Animal Care and Use Committee of the Animal Health Research Institute, Zagazig University, and Egypt approved the experimental procedures.
Author contributions
MH and WD jointly developed the hypothesis and concept of the study and contributed to the chemicals and materials preparations, as well as the techniques performed. For this research and scientific paper, OH, AT, IR, AS-A, FZ, and ZF are involved in the experimental procedures and analyses for this study and scientific paper. Each author contributed to the rewriting of the paper and the experimental analysis. All authors have read and approved the final manuscript.
Funding
This study was supported by grant KEGA no. 009UVLF-4/2021: Innovation and implementation of new knowledge of scientific research and breeding practice to improve the teaching of foreign students on the subject of Animal Husbandry.
Acknowledgments
The manuscript was edited by SpringEdit Inc. 103 Woodstream Blvd, Suite 02, Woodbridge/Vaughan, ON 141 7Z1 Toronto, Ontario, Canada. Moreover, we thank the staff at the Department of Food Control, the Faculty of Veterinary Medicine at Zagazig University, and the Faculty of Veterinary Medicine at Menoufia University for supporting us. Also, the efforts of the referees in evaluating this article are greatly appreciated.
Conflict of interest
The authors declare that the research was conducted in the absence of any commercial or financial relationships that could be construed as a potential conflict of interest.
Publisher's note
All claims expressed in this article are solely those of the authors and do not necessarily represent those of their affiliated organizations, or those of the publisher, the editors and the reviewers. Any product that may be evaluated in this article, or claim that may be made by its manufacturer, is not guaranteed or endorsed by the publisher.
Supplementary material
The Supplementary Material for this article can be found online at: https://www.frontiersin.org/articles/10.3389/fvets.2022.1042956/full#supplementary-material
Supplementary Figure 1. The concentration of OCPs categories among six species of fish samples.
References
1. Hussein MA, Merwad AM, Elabbasy MT, Suelam II, Abdelwahab AM. Taha MA. Prevalence of Enterotoxigenic Staphylococcus aureus and Shiga toxin-producing Escherichia coli in fish in Egypt: quality parameters and public health hazard. Vect-Borne Zoo Dis. (2019) 19:255–64. doi: 10.1089/vbz.2018.2346
2. Ahmed W, Olutayo OA. An overview of the aquaculture industry in Egypt. United States Department of Agriculture (USDA) – Foreign Agricultural Service. GAIN. (2022). Available online at: https://apps.fas.usda.gov/newgainapi/api/Report/DownloadReportByFileName?fileName=An%20Overview%20of%20the%20Aquaculture%20Industry%20in%20Egypt%20_Cairo_Egypt_02-11-2022.pdf (accessed November 2, 2022).
3. Maulu S, Nawanzi K, Abdel-Tawwab M. Khalil, HS. Fish nutritional value as an approach to children's nutrition. Front Nutr. (2021) 8:780844. doi: 10.3389/fnut.2021.780844
4. Murty AS. Toxicity of pesticides to fish, Boca Raton, FL; Mwevura, H; Othman, OC; Mhehe, G L Organochlorine pesticide residues in sediments and biota from the coastal area of Dar es Salaam city. Tanzania Mar Pollut Bull. (2002) 45:262–7. doi: 10.1016/S0025-326X(01)00331-9
5. Monirith I, Nakata H, Watanabe M, Takahashi S, Tanabe S. Organochlorine contamination in fish and mussels from Cambodia and other Asian Countries. Water Sci Technol. (2000) 42:241–52. doi: 10.2166/wst.2000.0575
6. Ntow JW. Pesticide residues in Volta Lake, Ghana. Lakes Reser Res Manage. (2005) 10:243–8. doi: 10.1111/j.1440-1770.2005.00278.x
7. Misumi I, Vella AT, Leong JAC, Nakanishi T, Schreck CB. p, p′-DDE depresses the immune competence of chinook salmon (Oncorhynchus tshawytscha) leukocytes. Fish Shellfish Immunol. (2005) 19:97–114. doi: 10.1016/j.fsi.2004.11.005
8. Vassilopoulou V, Gregoriades GE. Factors influencing the uptake of organochlorines in red mullet (Mullus barbatus) from a gulf of Central Greece. Mar Pollut Bull. (1993) 26:285–7. doi: 10.1016/0025-326X(93)90071-Q
9. Mwevurah H, Othman OC, Mhehe GL. Organochlorine pesticide residues in waters from the coastal area of Dar ES salaam and their effect on aquatic biota. Tanz J Sci. (2002) 28:117–30. doi: 10.4314/tjs.v28i2.18360
10. Tsukino H, Hanaoka T. Sasaki, H, Motoyama H, Hiroshima M, Tanaka T, Kabuto M, Turner W, Patterson DG, Needham, L, Tsugane S. Fish intake and serum levels of organochlorines among Japanese women. Sci Total Environ. (2006) 359:90–100. doi: 10.1016/j.scitotenv.2005.04.014
11. Li JY, Zhou WD, Lei FM, Zeng HY, Li SF, Wu DS. Study on serum organochlorine pesticides (DDTs) level, CYP1A1 genetic polymorphism, and risk of breast cancer: a case-control study. Zhonghua Liu Xing Bing Xue Za Zhi. (2007) 28:123–6. Available online at: https://pubmed.ncbi.nlm.nih.gov/16792888/
12. Morshdy AEMA, Darwish WS, Daoud JR, Hussein MA, Sebak MA. Monitoring of organochlorine pesticide residues in Oreochromis Niloticus collected from some localities in Egypt. Slov Vet Res. (2018) 553:303–11. doi: 10.26873/SVR-658-2018
13. Mohamed AA, Tharwat AE, Khalifa HA. Estimation of organochlorine pesticides and heavy metal residues in two species of mostly consumed fish in Sharkia Governorate, Egypt. Japan J Vet Res. (2016) 64:S233–41. Available online at: http://hdl.handle.net/2115/61999
14. El-Sebae AH, Abou Zeid M, Saleh MA. Status and environmental impact of toxaphene in the third world. A case study of African agriculture. Chemosphere. (1993) 27:2063–72. doi: 10.1016/0045-6535(93)90401-P
15. Yohannes YB, Ikenaka Y, Saengtienchai A, Watanabe KP, Nakayama SM, Ishizuka M. Concentrations and human health risk assessment of organochlorine pesticides in edible fish species from a Rift Valley lake—Lake Ziway, Ethiopia. Ecotoxicol Environ Saf. (2014) 106:95–101. doi: 10.1016/j.ecoenv.2014.04.014
16. Association Association of Official Agricultural Chemists (AOAC) International In Cunnif p., editor. Official Methods of Analysis of AOAC International (Gaithersburg, MD: AOAC International) (1999).
17. Yohannes YB, Ikenaka Y, Nakayama SM, Saengtienchai A, Watanabe K, Ishizuka M. Organochlorine pesticides and heavy metals in fish from Lake Awassa, Ethiopia: insights from stable isotope analysis. Chemosphere. (2013) 91:857–63. doi: 10.1016/j.chemosphere.2013.01.047
18. Food and Agricultural Organization (FAO). The State of World Fisheries and Aquaculture Rome (2010).
19. Akoto O, Bismark EF, Darko G, Adei E. Concentrations and health risk assessments of heavy metals in fish from the Fosu Lagoon. Int J Environ Res. (2014) 8:403–10. doi: 10.22059/IJER.2014.731
20. WHO. Agents Classified by the IARC Monographs, Volumes 1e101. IARC. (2013). Available online at: http://monographs.iarc.fr/ENG/Classification/index.php (accessed August 12, 2022).
21. Chien LC, Hung TC, Choang KY, Yeh CY, Meng PJ, Shieh MJ, et al. Daily intake of TBT, Cu, Zn, Cd and as for fishermen in Taiwan. Sci Total Environ. (2002) 285:177–85. doi: 10.1016/S0048-9697(01)00916-0
22. Huang ML, Zhou SL, Sun B, Zhao QG. Heavy metals in wheat grains: assessment of potential health risk for inhabitants in Khunshan, China. Sci Total Environ. (2008) 405:54–61. doi: 10.1016/j.scitotenv.2008.07.004
23. Kaur M, Sharma JK, Gill JP, Aulakh RS, Bedi JS, Joia BS. Determination of organochlorine pesticide residues in freshwater fish species in Punjab, India. Bullet Environ Contam Toxicol. (2008) 80:154–7. doi: 10.1007/s00128-007-9335-z
25. USEPA (United States Environmental Protection Agency). Integrated risk information system (IRIS) database. National Center for Environmental Assessment, Washington DC (2002). Available online at: https://www.epa.gov/iris (accessed April 14, 2022).
26. Łozowicka B, Kaczyński P, Wolejko E, Piekutin J, Sagitov A, Toleubayev K, et al. Evaluation of organochlorine pesticide residues in soil and plants from 869 East Europe and Central Asia. Desalin Wat Treat. (2016) 57:1310–21. doi: 10.1080/19443994.2014.996008
27. Liu M, Feng J, Kang B, Chen Y, Liu Q, Sun J. Ecological and health risks of DDTs and HCHs in surface water from the upper reaches of the Huaihe River. Toxicol Environ Chem. (2015) 97:1124–36. doi: 10.1080/02772248.2015.1091891
28. Wandiga SO, Yugi PO, Barasa MW, Jumba IO, Lalah JO. The distribution of organochlorine pesticides in marine samples along the Indian Ocean coast of Kenya. Environ Technol. (2002) 23:1235–46. doi: 10.1080/09593332308618328
29. Roche H, Tidou A, Persic A. Organochlorine pesticides and biomarker responses in two fishes Oreochromis niloticus (Linnaeus, 1758) and Chrysichthys nigrodigitatus (Lacepede, 1803) and an Invertebrate, Macrobrachium vollenhovenii (Herklot, 1857), from the Lake Taabo (Cote d'ivoire). J Appl Sci. (2007) 7:3860–9. doi: 10.3923/jas.2007.3860.3869
30. Adeyemi D, Ukpo G, Anyakora C, Unyimadu J. Organochlorine pesticide residues in fish samples from Lagos Lagoon, Nigeria. Ame J Environ Sci. (2008) 4:649–6. doi: 10.3844/ajessp.2008.649.653
31. Kalyoncu L, Agca I, Aktumsek A. Some organochlorine pesticide residues in fish species in Konya, Turkey. Chemosphere. (2009) 74:885–9. doi: 10.1016/j.chemosphere.2008.11.020
32. Afful S, Anim AK, Serfor-Armah Y. Spectrum of organochlorine pesticide residues in fish samples from the Densu Basin. Res J Environ Earth Sci. (2010) 2:133–8. Available online at: https://maxwellsci.com/print/rjees/v2-133-138.pdf
33. Kamel E, Moussa S, Abonorag MA, Konuk M. Occurrence and the possible fate of organochlorine pesticide residues at Manzala Lake in Egypt as a model study. Environ Monit Assess. (2015) 187:1–10. doi: 10.1007/s10661-014-4161-3
34. Gbeddy G, Glover E, Doyi I, Frimpong S, Doamekpor L. Assessment of organochlorine pesticides in water, sediment, African catfish and nile tilapia, consumer exposure and human health implications, Volta Lake, Ghana. J Environ Anal Toxicol. (2015) 5:1–8. doi: 10.4172/2161-0525.1000297
35. Food and Drug Administration (FDA). Environmental chemical contaminant and pesticide tolerances, action levels and guidance levels for fish and fishery products, compliance policy guide Sec.575.100. Pesticide chemical residues in food – Enforcement criteria, CPG (2001) 7141.01. Available online at: http://agrilife.org/fisheries2/files/2013/09/SRAC-Publication-No.-4900-The-HACCP-Seafood-Program-and-Aquaculture.pdf (accessed February, 2004).
36. Kumar JM, Kumar SD, Kubendran D, Kalaichelvan PT. Hexachlorobenzene sources, remediation, and future prospects. Int J Curr Res Rev. (2013) 5:1–12. Available online at: https://www.researchgate.net/publication/316167999_Hexachlorobenzene-sources_remediation_and_future_prospects/link/5d6e028992851c8538889959/download
37. Barber JL, Sweetman AJ, van Wijk D. Jones, KC. Hexachlorobenzene in the global environment: emissions, levels, distribution, trends, and processes. Sci Total Environ. (2005) 349:1–44. doi: 10.1016/j.scitotenv.2005.03.014
38. Food and Drug Administration (FDA). CPG Sec. 575.100 Pesticide residues in food and feed - enforcement criteria, (2011). Available online at: http://www.fda.gov/ICECI/ComplianceManuals/CompliancePolicyGuidanceManual/ucm123236.htm (accessed September 12, 2011).
39. Falcó G, Llobet JM, Bocio A, Domingo JL. Exposure to hexachlorobenzene through fish and seafood consumption in Catalonia, Spain. Sci Total Environ. (2008) 389:289–95. doi: 10.1016/j.scitotenv.2007.09.025
40. Chukwumalume RC, Louwrens HC, Umezurike OL, Bernadette O, Marietjie SA. Polycyclic aromatic hydrocarbons (PAHs) and organochlorinated pesticides (OCPs) in yellowtail (Seriola lalandi) from three spatially distinct locations along the coast of south africa: levels, sources and fish size effect. J Food Proc Technol. (2017) 8:1–8. doi: 10.4172/2157-7110.1000644
41. Ibigbami OA, Aiyesanmi AF, Adeyeye EI, Aladesanwa A. Assessment of organochlorine and organophosphorus pesticide residue in water and sediments from Ero river in southwestern Nigeria. J Chem Biol Phys Sci. (2015) 5:4679–90. doi: 10.5539/enrr.v5n3p28
42. Naso B, Perrone D, Ferrante MC, Bilancione M. Lucisano A. Persistent organic pollutants in edible marine species from the Gulf of Naples, Southern Italy. Sci Total Environ. (2005) 343:83–95. doi: 10.1016/j.scitotenv.2004.10.007
43. WHO. Health risks of persistent organic pollutants from long-range transboundary air pollution. http://www.euro.who.int/en/health-topics/environment-and-health/air-quality/publications/pre2009/health-risks-of-persistent-organic-pollutants-from-long-range transboundary-air-pollution (2003). Available online at: https://apps.who.int/iris/handle/10665/107471 (accessed January 7, 2003).
44. ATSDR (Agency for Toxic Substances Disease Registry). Toxicological profile for chlordane. U.S. Department of Health and Human Services. U.S. Public Health Service. Agency for Toxic Substances and Disease Registry. (1994). Available online at: https://www.atsdr.cdc.gov/ (accessed January 5, 1994).
45. Akan JC, Mohammed Z, Jafiya L, Ogugbuaja VO. Organochlorine pesticide residues in fish samples from Alau Dam, Borno State, North Eastern Nigeria. Environ Anal Toxicol. (2013) 3:1–7. doi: 10.11648/j.ijema.20130102.14
46. Darko G, Akoto O, Oppong C. Persistent organochlorine pesticide residues in fish, sediments, and water from Lake Bos- omtwi, Ghana. Chemosphere. (2008) 72:21–4. doi: 10.1016/j.chemosphere.2008.02.052
47. Teklit G. Residues analysis of organochlorine pesticides in fish, sediment, and water samples from Tekeze Dam, Tigray, Ethiopia. J Environ Anal Toxicol. (2016) 6:1.
48. ATSDR (Agency for Toxic Substances and Disease Registry). Toxicological profile for heptachlor and heptachlor epoxide. Atlanta, GA: U.S. Department of Health and Human Services. Public Health Service. Agency for Toxic Substances and Disease Registry. ATSDR (2007). Available online at: https://www.atsdr.cdc.gov/toxprofiles/tp12.pdf (accessed November, 2007).
49. USEPA (United States Environmental Protection Agency). National primary drinking water regulations. (2017). Available online at: https://www.epa.gov/ground-water-and-drinking-water/national-primary-drinking-water-regulations (accessed January 11, 2017).
50. Kafilzadeh F. Assessment of organochlorine pesticide residues in water, sediments, and fish from Lake Tashk, Iran. Achiev Life Sci. (2015) 9:107–11. doi: 10.1016/j.als.2015.12.003
51. Fuhrmann MM. The role of the invasive red king crab in the food web of a high-latitude fjord Studying macrobenthic communities and trophic control in Porsangerfjord northern. Norway J Sea Res. (2016) 106:1–13. doi: 10.1016/j.seares.2015.09.003
52. Snow RW, Amratia P, Kabaria CW, Noor AM, Marsh K. The changing limits and incidence of malaria in Africa: 1939–2009. Adv Parasitol. (2012) 78:169–262. doi: 10.1016/B978-0-12-394303-3.00010-4
53. Bachelet D, Truong T, Verner M-A, Arveux P, Kerbrat P, Charlier C. Guihenneuc- Jouyaux C, Guénel P. Determinants of serum concentrations of 1,1-dichloro 2,2- bis (p-chlorophenyl) ethylene and polychlorinated biphenyls among French women in the CECILE. study Environ Res. (2011) 111:861–70. doi: 10.1016/j.envres.2011.06.001
54. Cohn BA, Wolff MS, Cirillo PM, Sholtz RI, DDT. and breast cancer in young women: new data on the significance of age at exposure. Environ Health Perspect. (2007) 115:1406. doi: 10.1289/ehp.10260
55. Kuranchie-Mensah H, Atemo SM, Maud LNP, Blankson-Arthur S, Tutu AO, Fosu P. Determination of organochlorine pesticide residue in sediment and water from the Densu river basin, Ghana. Chemosphere. (2011) 86:286–92. doi: 10.1016/j.chemosphere.2011.10.031
56. Kafilzadeh F. Amir, HS, Malekpour R, Azad HN. Determination of organochlorine pesticide residues in water, sediments, and fish from Lake Parishan, Iran. World J Fish Mar Sci. (2012) 4:150–4. doi: 10.5829/idosi.wjfms.2012.04.02.56399
57. Adeyemi MM, Abdulmalik H. Determination of organochlorine pesticides in some imported frozen fish species consumed within Kaduna Metropolis. IOSR. J Environ Sci Toxicol. Food Technol. (2017) 11:1–5. doi: 10.9790/2402-1104020105
58. FAO/WHO. Evaluation of certain food additives. Twenty-first report of the joint FAO/WHO Expert Committee on Food Additives, WHO Technical Series No 617. (1978). Available online at: https://apps.who.int/iris/handle/10665/41293 (accessed April 18–27, 1977).
59. Zhou H, Cheung R, Wong M. Bioaccumulation of organochlorines in freshwater fish with different feeding modes cultured in treated wastewater. Water Res. (1999) 33:2747–56. doi: 10.1016/S0043-1354(98)00506-5
60. Aktumsek A, Kara H, Nizamlioglu F, Dinc I. Monitoring of organochlorine pesticide residues in pikeperch, Stizostedion lucioperca L in Beysehir lake (Central Anatolia). Environ Technol. (2002) 23:391–4. doi: 10.1080/09593332508618402
61. FAO/WHO. Pesticide residues in food. Joint FAO/WHO meeting on pesticide residues. Report of the joint meeting of the FAO panel of Experts on pesticide residues in food and the environment and the WHO core assessment group on pesticide residues. World Health Organization, food, and agriculture organization of the United Nations. Rome (2009). Available online at: https://inchem.org/documents/jmpr/jmpmono/v2003pr08.htm (accessed September 16–25, 2009).
62. Yang N, Matsuda M, Kawano M, Wakimoto T. PCBs and organochlorine pesticides (OCPs) in edible fish and shellfish from China. Chemosphere. (2002) 63:1342–52. doi: 10.1016/j.chemosphere.2005.09.029
63. Guo JY, Zeng EY, Wu FC, Meng XZ, Mai BX, Luo XJ. Organochlorine pesticides in seafood products from southern China and health risk assessment. Environ Toxicol Chem. (2007) 26:1109–15. doi: 10.1897/06-446R.1
64. Yatawara M, Qi S, Owago OJ, Zhang Y, Yang D, Zhang J, Burnet JE. Organochlorine pesticide and heavy metal residues in some edible biota collected from Quanzhou Bay and Xinghua Bay, Southeast China. J. Environ Sci. (2010) 22:314–20. doi: 10.1016/S1001-0742(09)60110-8
65. Ogbeide O, Tongo I, Ezemonye L. Risk assessment of agricultural pesticides in water, sediment, and fish from Owan River, Edo State, Nigeria. Environ Monit Assess. (2015) 187:654. doi: 10.1007/s10661-015-4840-8
66. Cui L, Ge J, Zhu Y, Yang Y, Wang J. Concentrations, bioaccumulation, and human health risk assessment of organochlorine pesticides and heavy metals in edible fish from Wuhan, China. ESPR. (2015) 22:15866–79. doi: 10.1007/s11356-015-4752-8
67. Zhao P, Sanganyado E, Wang T, Sun Z, Jiang Z, Zeng M, Huang Z, Li Y, Li P, Bi R, Liu W. Accumulation of nutrients and potentially toxic elements in plants and fishes in restored mangrove ecosystems in South China. Sci Total Environ. (2022) 16:155964. doi: 10.1016/j.scitotenv.2022.155964
Keywords: target hazard quotient, seafood, health risk assessment, hazard index, organochlorine pesticides (OCPs)
Citation: Hussein MA, Hammad OS, Tharwat AE, Darwish WS, Sayed-Ahmed A, Zigo F, Farkašová Z and Rehan IF (2022) Health risk assessment of organochlorine pesticide residues in edible tissue of seafood. Front. Vet. Sci. 9:1042956. doi: 10.3389/fvets.2022.1042956
Received: 13 September 2022; Accepted: 04 November 2022;
Published: 28 November 2022.
Edited by:
Hany M. R. Abdel-Latif, Alexandria University, EgyptReviewed by:
Hazuki Mizukawa, Ehime University, JapanAnna Zielak-Steciwko, Wroclaw University of Environmental and Life Sciences, Poland
Beata Holečková, University of Veterinary Medicine in Kosice, Slovakia
Mahmoud M. Alagawany, Zagazig University, Egypt
Hala Saber Khalil, WorldFish, Malaysia
Copyright © 2022 Hussein, Hammad, Tharwat, Darwish, Sayed-Ahmed, Zigo, Farkašová and Rehan. This is an open-access article distributed under the terms of the Creative Commons Attribution License (CC BY). The use, distribution or reproduction in other forums is permitted, provided the original author(s) and the copyright owner(s) are credited and that the original publication in this journal is cited, in accordance with accepted academic practice. No use, distribution or reproduction is permitted which does not comply with these terms.
*Correspondence: Ibrahim F. Rehan, ibrahim.rehan@vet.menofia.edu.eg; František Zigo, frantisek.zigo@uvlf.sk