- 1National Institute of Hygiene and Epidemiology, Hanoi, Vietnam
- 2Oxford University Clinical Research Unit, Hanoi, Vietnam
- 3Quadram Institute Bioscience, Norwich Research Park, Norwich, United Kingdom
- 4Hanoi Medical University, Hanoi, Vietnam
- 5MIVEGEC (IRD-CNRS-Université de Montpellier), LMI DRISA, Center IRD, Montpellier, France
- 6Oxford University Clinical Research Unit, Ho Chi Minh City, Vietnam
- 7Centre for Tropical Medicine and Global Health, Nuffield Department of Clinical Medicine, University of Oxford, Oxford, United Kingdom
- 8National Institute of Infectious Diseases, Tokyo, Japan
Livestock has been implicated as a reservoir for antimicrobial resistance (AMR) genes that can spread to humans when antimicrobials are used in animals for food production to treat clinical diseases and prevent and control common disease events. In Vietnam, mcr-1-harboring Escherichia coli (MCRPEC) strains have been isolated from humans, animals (chickens, pigs, and dogs) feces, flies, foods, and the environment (rainwater, well water, and irrigation water) in communities and from clinical specimens in hospitals. The relationship between levels of AMR in livestock and its occurrence in humans is complex and is driven by many factors. We conducted whole genome sequencing of MCRPEC to analyze the molecular epidemiological characteristics, history, and relatedness of 50 isolates obtained in 2019 from different reservoirs in farms and markets in Ha Nam province, Vietnam. 34 sequence types (STs) with 3 new STs were identified in multilocus sequence typing analysis: ST12945 and ST12946 from chicken feces, and ST12947 from flies. The AMR phenotypes of 50 MCRPEC isolates were as follows: ampicillin (100%, 50/50), cefotaxime (10%, 5/50), gentamicin (60%, 30/50), amikacin (8%, 4/50), meropenem (6%, 3/50), ceftazidime (18%, 9/50), colistin (24%, 12/50) and ciprofloxacin (80%, 40/50). All 50 MCRPEC isolates were identified as MDR. 100% (50/50) isolates carried AMR genes, ranging from 5 to 22 genes. The most prevalent plasmid replicon types carrying mcr-1 were IncP-1 (17/37, 45.9%), IncX4 (7/37, 18.9%), and IncHI2/IncHI2A (6/37, 16.2%). These data suggest that the epidemiology of the mcr-1 gene is mostly determined by plasmid spreading instead of clonal dissemination of MCRPE strains. The co-occurrence of several STs such as ST10, ST48, ST155, ST206, ST2705 in various sample types, joined to the higher prevalence of a few types of Inc plasmids, confirms the dissemination of the mcr-1 carrying plasmids in E. coli clones established in livestock. 5 over 8 STs identified in flies (ST206, ST2705, ST155, ST10, and ST48) suggested the fly contribution in the transmission of AMR bacteria in environments. These popular STs also occur in human samples and 100% of the human samples were positive for the mcr-1 gene.
Introduction
Antimicrobial resistance (AMR) is a global public health and development threat. In 2019, WHO declared that AMR has been one of the top ten global health threats facing humanity that caused least 700,000 deaths globally per year, which could potentially increase to 10 million deaths globally per year by 2050 (1, 2). The WHO Global Antimicrobial Resistance and Use Surveillance System (GLASS), 2021 emphasized high proportions of AMR in the bloodstream, and urinary and gastroenteric infections in most countries, including in last resort antimicrobials such as carbapenem, or first-line drugs such as co-trimoxazole (3). Livestock has been implicated as a reservoir for AMR that can spread to humans when antimicrobials are used in food animals to treat clinical diseases, prevent common disease events, and enhance animal growth, and many of these antimicrobials are identical to or closely resemble drugs used in humans (4, 5).
Carbapenem-resistant Enterobacterales (CRE) have spread worldwide and present a serious public health threat with high mortality rates as high resistance also occurs to other clinically important antimicrobials, such as cephalosporins, fluoroquinolones, and aminoglycosides (6). Colistin (Polymyxin E) is an old antimicrobial with cationic detergent properties that is considered to be a last therapeutic option to treat infections caused by carbapenem-resistant Gram-negative bacteria that are also resistant to all other first and second line drugs, because (i) the treatment options for first and second line drug resistant CRE remain very limited and (ii) colistin has proved its function in killing Gram-negative bacteria in severe infections (7–9). Colistin also has been used in veterinary medicine for decades, mainly for the prevention and treatment of Enterobacterales infection (10). While the spread of carbapenemase genes has been mainly recognized in humans but rarely evidenced in animals, colistin resistance in Escherichia coli seems to be related to the use of colistin in veterinary medicine on a global scale (11). The cases of colistin-resistant bacteria were reported from various parts of the world and became a major public health threat with the variation of genes encoding colistin resistance (mcr-1 to mcr-10), especially the mcr-1 gene that spread rapidly in animals, travelers, foods, and environment around the world (12, 13).
In Vietnam, MCRPEC strains have been isolated from humans, animals (chickens, pigs, and dogs), flies, foods, and the environment (rain, well, and irrigation water) in a community and from clinical specimens in referral hospitals (14–16). However, the relationship between levels of AMR in livestock and its occurrence in humans is complex and multi-factorial and includes the combinations of antimicrobials, bacterial strains, mobile genetic elements (MGEs), and livestock species, each with their dynamics (4, 17). The application of whole genome sequencing (WGS) as a potential powerful tool facilitates our baseline understanding of these pathways. Therefore, we conducted WGS to analyze molecular epidemiological characteristics, history, and relatedness of isolates obtained from different reservoirs in livestock settings.
Materials and methods
Bacterial isolates
Samples were obtained in 2019 from various sources in Ha Nam, a province in Northern Vietnam including poultry and swine farms, farmers and markets. Samples including farmer feces (20), chicken feces (61), pigs feces (31), dogs feces (18), farm flies (36), wastewater (21), and market surface (15) were collected and kept at – 80°C in the Antimicrobial Resistance Laboratory of the National Institute of Hygiene and Epidemiology (NIHE) in Vietnam. All samples were pre-processed as previously described in 2022 (16).
To screen for samples containing mcr-1 genes, the samples were kept overnight in tryptic soy broth at 37°C without shaking. We used 1.5 ml of enriched broth for total DNA extraction and detected mcr-1 by using PCR. Among mcr-1 positive samples, we randomly selected 50% of the samples for further analysis.
The selected samples were cultured on MacConkey agar containing 0.5 mg/L colistin. We then picked up one E. coli-like colony represent for each sample for identification by MALDI-TOF MS (Bruker, Berlin, Germany). All confirmed E. coli isolates were selected for further screening for mcr-1. Next, DNA from E. coli isolates was subjected to confirm the presence of mcr-1 by PCR.
Farm-scale is computed and classified by Decree 13/2020/ND-CP Detail Guideline of Livestock Law issued by the Vietnamese Government. Number of all types of food animals raised, including pigs, chickens, ducks, geese, quails, doves, ostriches, etc., is converted to “livestock unit” for each type of food animals, “total farm livestock unit” is sum of livestock units of all food animal types raised and is used to categorize farm-scale (House-hold: < 10 units, small: from 10 to under 30 units, medium: from 30 to under 300 units, large: ≥ 300 units) (18).
Antimicrobial resistance phenotyping of MCRPEC isolates
The minimum inhibitory concentrations (MIC) to antimicrobials commonly used in treatment were determined on 50 MCRPEC isolates to identify susceptible (S) or resistant (R) phenotypes. The MCRPECs were tested for resistance against 8 drugs of 6 class of antimicrobials. MICs were determined using the agar dilution method for ampicillin (AMP), cefotaxime (CTX), gentamicin (GEN), amikacin (AMK), meropenem (MER), ceftazidime (CAZ), ciprofloxacin (CIP) and the microdilution method for colistin (CST) (Sigma-Aldrich) according to CLSI 2018 and European Committee on Antimicrobial Susceptibility Testing (EUCAST) 2018 (19, 20). E. coli ATCC 25922 and Pseudomonas aeruginosa ATCC 27853 were used as controls.
Whole genome sequencing and genetic environment characterization of mcr-1 genes
The procedure was performed as previously reported (14). Whole genome sequencing was performed on DNA from all mcr-1-positive E. coli isolates. Genomic DNA libraries of selected strains were prepared for WGS using the Nextera XT DNA Library Preparation Kit (Illumina), following the manufacturer's instructions. 300 bp paired-end sequencing was performed on an Illumina MiSeq platform (MiSeq Reagent Kit v3; 600 cycles). Raw sequence reads were de novo assembled into contigs using Shovill v1.1.0 with SPAdes v3.14.1 as the assembler. NCBI Prokaryotic Genome Annotation Pipeline (PGAP) was used to annotate genes. AMR genes from ResFinder databases of the Center for Genomic Epidemiology were identified using ABricate and Staramr (scans genome contigs against ResFinder. PlasmidFinder databases, https://libraries.io/pypi/staramr) were used to identify replicon typess, and MLST following Achtman sequence definitions database (https://pubmlst.org/bigsdb?db=pubmlst_ecoli_achtman_seqdef) was used to identify sequence type for the isolates. A maximum-likelihood phylogenetic trees based on the core genome single nucleotide polymorphisms (SNPs) were constructed from WGS data of the 50 MCRPEC isolates utilizing Prokka and Roary and IQ-TREE v1.6.11.
Phylogenetic groups
All isolates were assigned to phylogenetic groups (A, B1, C, B2, D, E and F) based on Clermont phylogenetic typing schemes (21).
Genes of virulence factors
Virulence genes were identified by VirulenceFinder V2.0 (https://cge.cbs.dtu.dk/services/VirulenceFinder/).
Statistical methods
Isolates data were analyzed in MS Excel 2017 (Microsoft Corp., USA) using descriptive statistics as appropriate.
Institutional review board statement
The study was conducted according to the guidelines of the Declaration of Helsinki and approved by the Institutional Review Board of the National Institute of Hygiene and Epidemiology, Vietnam (IRB approval number: HDDD−06/2019).
Informed consent statement
Informed consent was obtained from all human subjects involved in the study.
Results
Samples containing mcr-1 gene in livestock setting and E. coli isolates for whole genome sequencing
We found that 51.5% (104/202) of samples were mcr-1 positive: 100% (20/20) of farmer feces samples, 62.3% (38/61) of chicken feces samples, 41.9% (13/31) of pig feces samples, 11.1% (2/18) of dog feces samples, 55.6% (20/36) of farm fly samples, 22.6% (7/31) of wastewater samples, and 26.7% (4/15) of market surface samples. We selected 50% (n = 52) from 104 mcr-1 positive samples for further analysis. Among 52 selected samples, 50 samples appeared E. coli like colonies and all of them were confirmed E. coli by MALDI-TOF-Biotyper. Next, DNA of E. coli isolates was subjected to confirm the presence of mcr-1 by PCR. Whole genome sequencing was performed on DNA from all mcr-1-positive E. coli isolates (n = 50) which included feces from farmer feces (n = 17) chicken feces (14), pig feces (4), dog feces (1), farm flies (11), wastewater (2), and market surface (1).
Antimicrobial resistance phenotyping of MCRPEC isolates
Examining antimicrobial resistance of MCRPEC isolates, per drug and sample type, we observed that the resistance proportions to tested antimicrobials in the isolates were as follows: 100% (50/50) resistance against ampicillin, 10% (5/50) against cefotaxime, 60% (30/50) against gentamicin, 8% (4/50) against amikacin, 18% (9/50) against ceftazidime, 24% (12/50) against colistin, and 80% (40/50) against ciprofloxacin (Table 1). In particular, 6% (3/50) of isolates were at intermediate level with meropenem at MIC of 2 mg/L in comparison to breakpoint (CLSI 2019), indicating meropenem susceptibility reduction.
Households which provided 78% (39/50) MCRPECs have resistance to more antimicrobials with 8 chosen antimicrobials than 18% (9/50) strains from medium-scale farms, 2% (1/50) strains from small-scale farms, and 2% (1/50) strains from market. 46% (23/50) and 44% (22/20) MCRPECs in this study belonged to poultry and mixed farms. Notably, there was one MCRPEC strain collected on the surface market but resistant to 8/8 antimicrobials tested in this study.
Based on the PCR screening results, WGS was conducted to analyze AMR genes for further characterization of these species. The results of the MIC tests and WGS analysis are presented in Supplementary Table 1 and Figure 1. The AMR isolates showed strong resistance with MIC values higher than the breakpoint of resistance in recommendation of CLSI, such as 98% of strains were resistant to ampicillin at the MIC value >256 mg/L, 80% to cefotaxim (>64mg/L), and 75% to amikacin (>256 mg/L), respectively.
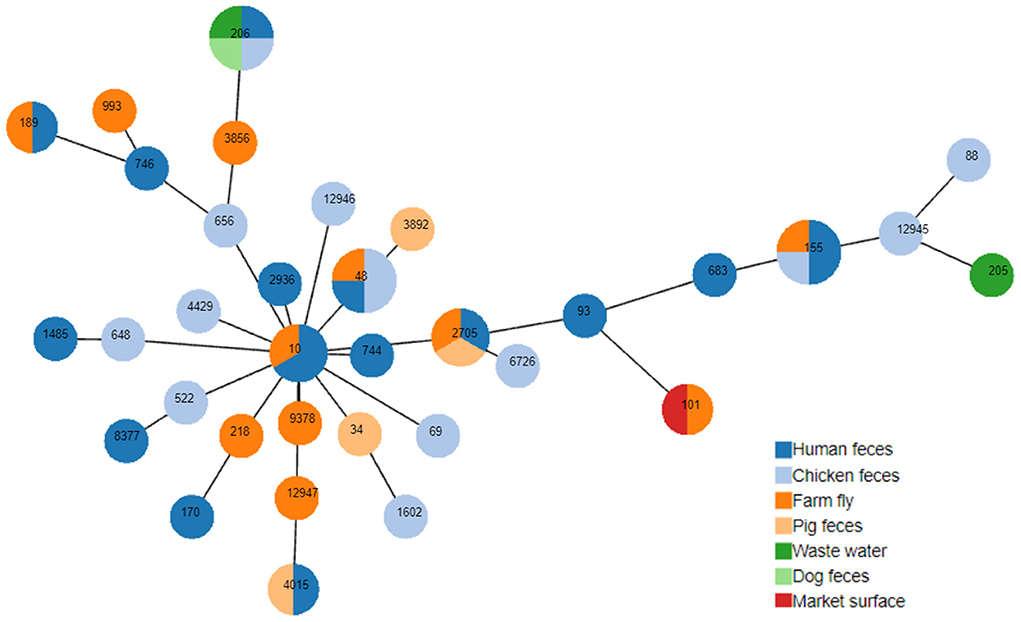
Figure 1. Minimum spanning tree based on multi-locus sequence types and seven housekeeping gene alleles of MCRPEC. Each circle corresponds to an individual sequence type (ST), the circle size indicated the number of isolates assigned to the same ST; The color of the circle denotes the sample type. The length of the branch is equal to the number of different alleles (calculated using seven MLST genes) between two linked nodes/STs.
In our study, all isolates bearing mcr-1 but 76% (38/50 of MCRPECs isolates showed susceptibility to colistin at MIC values ≤2 mg/L, of which 74% (37/50) strains had MIC value at 2 mg/L. Among which 24% (12/50) of isolates were resistant to colistin, of which 33% (4/12) were resistant at an MIC value >16 mg/L. Especially, three isolates showed reduced susceptibility to meropenem (3/50) at 2 mg/L.
Results from whole genome sequencing showed that 100% (50/50) of isolates harbored antimicrobial resistance genes (ARGs) with the range from 5 to 22 genes responsibility for resistance to colistin (mcr-1), aminoglycosides (aac(3)-, aac(6')-, ant(3”)-IIa, aph(3”)-Ib, aph(3')-Ia, aph(6)-Id, aadA), fosfomycin (fosA), β-lactams (blaCARB − 3, blaCARB − 2, blaCTX − M, Escherichia_coli_ampC, Escherichia_coli_ampC1_beta−lactamase, blaOXA, and blaTEM − 1), sulfonamides (sul1, sul2, sul3), fluoroquinolones (qnrD1, qnrS), tetracyclins [tet(A)], trimethoprim (dfrA), phenicols (floR, catA4, catB3), and macrolides (emrE, mphA, mphB) (Supplementary Table 1).
MLST analysis and phylogenic tree
Thirty four sequence types (ST) of 50 selected MCRPECs were identified (Figure 1) and classified in 4 phylogroups (A, B1, C, and F) (Supplementary Table 1). Phylotyping analysis revealed that most of these strains belonged to the A group 38/50 (76%) with 26 different STs (ST10, ST34, ST48, ST69, ST93, ST101, ST155, ST170, ST189, ST205, ST206, ST648, ST656, ST683, ST744, ST746, ST1485, ST1602, ST2705, ST2936, ST3856, ST4015, ST6726, ST8377, ST12945, ST12946, ST12947, ST218, ST3892, ST4429, ST522, ST88, ST9378, and ST993). 9 / 50 strains (18%) were assigned to group B1 with 5 STs (ST101, ST155, ST205, ST683, and ST12945), 2/50 (4%) to group F with 2 STs (ST648, and ST1485) and 1 strain, with ST88, to group C. The eight most common STs were ST155 (n = 4; 8%); ST206 (n = 4; 8%), ST48 (n = 4; 8%), ST2705 (n = 3; 6%), ST10 (n = 3; 6%), ST 4015 (n = 2; 4%), ST101 (n = 2; 4%) and ST189 (n = 2; 4%). The minimum spanning tree based on multi-locus sequence types (Figure 1) showed the most of the STs found in this study belonged to clonal complex 10 (CC10). Three new STs were identified in this study, including ST12945, ST12946, and ST12947 with ST12945 collected from chicken feces and belonged to the phylogroup B1, ST12946 from chicken feces and ST12947 from flies belonged to the phylogroup A. There were several STs isolated from different sample types such as ST155 appeared on human feces, chicken feces, and farm flies; and ST2705 were obtained from human, pig feces, and farm flies. In addition, 5/8 STs from farm flies (ST206, ST2705, ST155, ST10, and ST48) and belonged to the A and B1 groups were also recorded on other sample types including humans. The co-occurrence of several STs in various sample types, joined to the higher prevalence of a few types of Inc plasmids, confirms the dissemination of the mcr-1 carrying plasmids in E. coli clones established in livestock.
The phylogenetic tree indicated two major clades: one clade (Figure 2) contained 47 isolates and 31 STs, including common STs like ST155, ST2705, ST48, ST4015, ST10, and ST206; the other clade (Figure 2) contained 3 isolates and 3 STs including ST69, ST648, and ST1485.
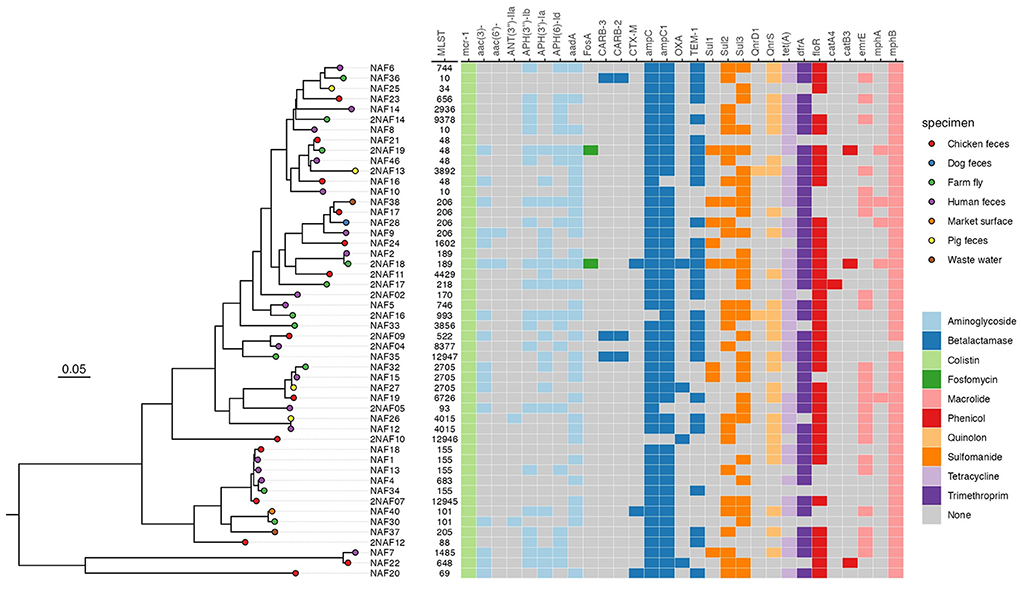
Figure 2. Maximum likelihood phylogenic analysis of 50 MCRPEC isolates from farms in Ha Nam. The heat map represents the AMR genotype of 50 MCRPECs.
The core gene phylogeny revealed the close relation among MCRPECs isolated from different sample types and sources: ST2705, ST155, ST683, and ST206 have the close relation; ST2705 isolated from human, pig feces and flies, ST155 and ST683 from human, chicken feces, flies, ST206 from human, dog feces, market, wastewater.
All 50 MCRPECs harbored genes conferring resistance to various other antimicrobials: aminoglycosides, β-lactams, fosfomycin, macrolides, phenicols, fluoroquinolones, sulfonamide, tetracyclin, trimethoprim also presented on the Figure 2. Only three isolates (ST189, ST101, and ST69) harbored CTX-M gene encoding extended-spectrum beta-lactamase. Two of these isolates were collected from farm fly samples.
Virulence factors genes
A total of 59 different virulence genes were detected from the MCRPECs. The virulence genes; csgG, entA, entE, entS, fepC, fepD, fes and ompA were detected in ≥ 98% the MCRPEC isolates. Other virulent genes that were commonly prevalent include; aslA, csgB, csgD, csgF, entB, entC, entD, entF, fdeC, fepA, fepB, fepG, fimA, fimB, fimC, fimD, fimE, fimF, fimG, fimH, fimI, gspC, gspD, gspE, gspF, gspG, gspH, gspI, gspJ, gspK, gspL, gspM, yagV/ecpE, yagW/ecpD, yagX/ecpC, yagY/ecpB, yagZ/ecpA, and ykgk/ecpR. The less prevalent (≤ 30%) were astA, chuS, chuT, chuU, chuV, chuW, chuY, fyuA, iroN, iutA, kpsD, kpsM, and PapC. The proportion of virulence genes from humans, animals, flies and environment was presented in Supplementary Tables 2, 3.
Plasmid genotyping
To classify the locations of mcr-1 in the genomes, mcr-1-containing contigs were extracted from the complete genomes of MCRPEC isolates. We detected that mcr-1 is located on both the chromosome (n = 7) and on plasmids (n = 43) isolates (Figure 3). Among plasmids carrying mcr-1, we identified 13 plasmid replicon types, the predominant plasmid replicon type was IncP-1 (17/37, 45.9%) followed by IncX4 (7/37, 18.9%), and IncHI2/IncHI2A (6/37, 16.2%). Other replicons were IncHI2/IncHI2A/IncN/p0111 (2/37, 5.4%), IncFIA(HI1)/IncHI1A/IncHI1B(R27) (1/37, 2.7%), IncHI2/IncHI2A/IncN (1/37, 2.7%), IncFIB(AP001918)/IncHI2/IncHI2A (1/37, 2.7%), p0111 (1/37, 2.7%), and IncY (1/37, 2.7%). Plasmid replicons of six isolates were unable to identify.
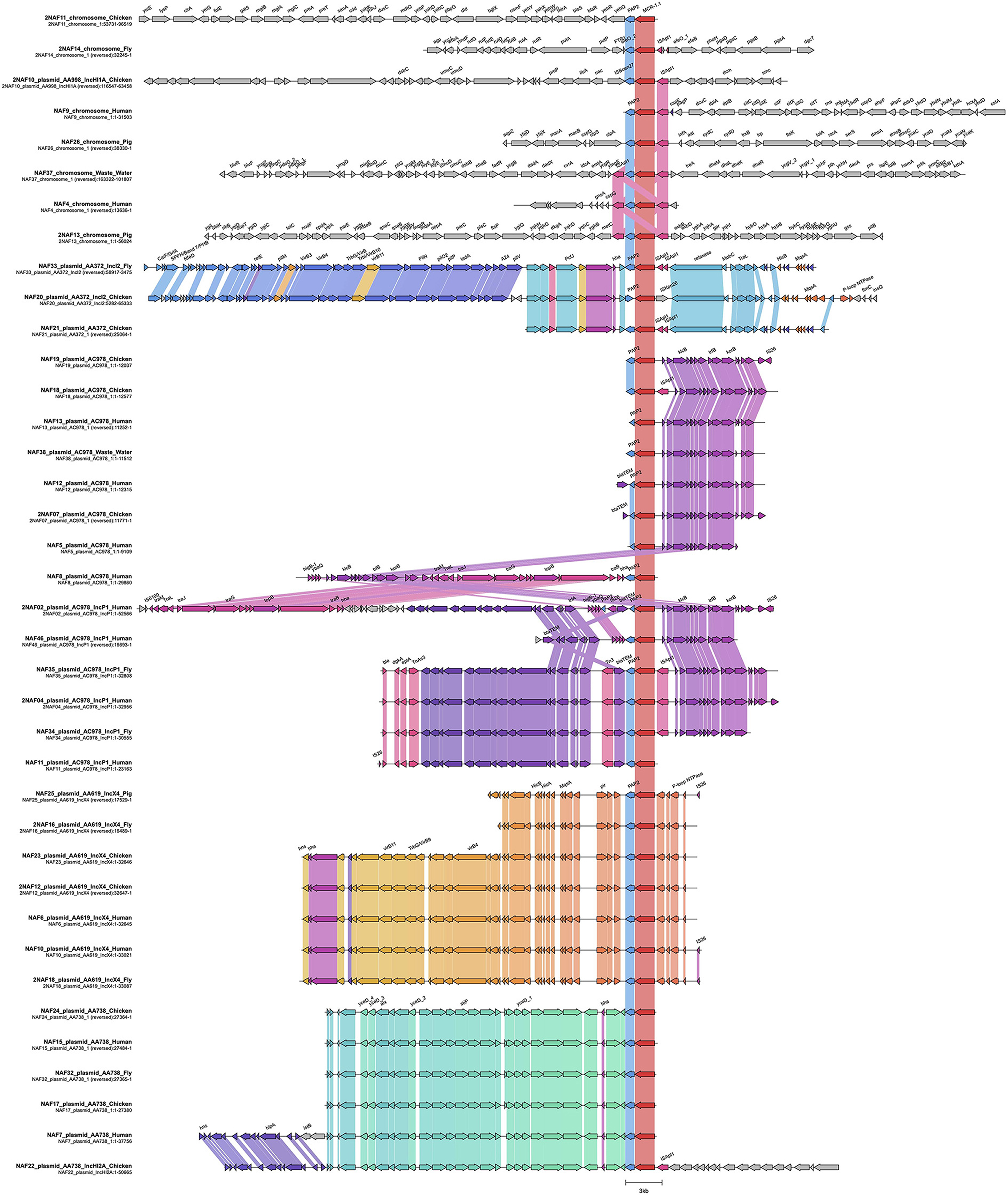
Figure 3. Representative of sequence alignment of mcr-1-containing cassettes. Arrow indicates an open reading frame and the direction of transcription. ISApl1 is indicated by the pink arrow, mcr-1 is indicated by the red arrow, the hypothetical gene is indicated by the gray arrow, and intrinsic genes of the plasmid backbone or chromosome are indicated by orange arrows. Color shadings connect regions of >99% similarity.
We observed 15 different genetic contexts of mcr-1 with the presence of insertion sequence ISApl1 on both chromosomes (n = 7) and plasmids (n = 8). Among 7 isolates carrying mcr-1 on their chromosome, 3 contained the full Tn6330 transposon sequence ISApl1-pap2-mcr-1-ISApl1. These strains were collected from human feces, pig feces and wastewater. Although, no full transposon was found on plasmids, 5 plasmids contained indication of the presence of ISApl1 with a full copy downstream and 2 plasmids with a partial downstream ISApl1 element. Among plasmids carrying the full copy of downstream ISApl1, 3 plasmids were IncP1 replicon, which has been known as an important plasmid type in antimicrobial transmission between gram negative bacteria.
Discussion
Farms with high positive rate of mcr-1 selected for sampling in this study had a high diversity of specimen types with MCRPECs; including fecal samples of humans and farm animals, such as chickens, dogs, fly samples, wastewater samples from farms and market surface samples. A study conducted in more than 25 nations on four continents also indicate the existence of mcr-1 gene in animals, food, human, and the environment (22). Particularly, a study documented the rapid spread and proliferation of E. coli strains carrying the mcr-1 gene in 2009, the first detection of mcr-1, in a retrospective study on E. coli strains isolated from chickens in the 1980s (23). In Vietnam, the prevalence of samples contain MCRPECs in this study was 51.5% (104/202), higher than the previous study in Ha Nam community in the Northern region between 2014 and 2015 with37.6% samples contain MCRPECsE. coli overall (14). A study conducted on chicken and pig farms in the Southern region showed that E. coli isolates had high levels of resistance (>20%) to important antimicrobials such as colistin (24). Meanwhile, another study in Thai Binh province reported that colistin-based drugs (CBD) were used in more than 50% of veterinary drugs purchased by chicken farms suggesting that CBD use could be responsible for increased resistance to colistin in extended spectrum β-lactamase-producing E. coli strains (25).
In 2017, the Vietnamese government issued the Circular 20/2017/TT-BNNPTNT that includes the instruction for controlling antimicrobials use in animal growth (26). However, the comparison between two studies illustrated that it will take more time for the rule to promote the efficiency in the Vietnamese livestock system by controlling and using antimicrobial properly.
In our study, MCRPECs isolates in our study originated from various sample resources, most of them were highly resistant to common antibiotics used in humans and agriculture, such as penicillin, cephalosporins, and aminoglycosides. However, most of them still showed susceptibility to meropenem, an antibiotic in the carbapenem family that is not permitted for use in livestock. There are three isolates that showed a reduced susceptibility to meropenem with a MIC value of 2 mg/L but no gene encoding carbapenemase enzyme [such as VIM-type, NDM-type, OXA-type and the serine carbapenemase genes (27)] was found in the whole genome sequencing analysis. It could be the result of an unknown gene conferring meropemen resistance, which will be revealed in future research.
The results of WGS confirmed this statement when they observed various AMR genes with a minimum of 5 resistance genes and a maximum of 22 AMR genes. E. coli is intrinsically susceptible to almost all clinically relevant antimicrobials, but this bacterium has a great capacity to accumulate resistance genes, primarily through horizontal gene transfer (11). 22 AMR genes found in 50 MCRPECs included colistin (mcr-1) and genes conferring resistant to aminoglycosides, fosfomycin, β-lactams, sulfonamides, fluoroquinolones, tetracyclins, trimethoprim, phenicols and macrolides, suggesting that the impact of MCRPECs on the transmission of AMR genes should not be underestimated.
Recent studies reported phenotypically susceptible or colistin-resistant MCRPECs strains at low MIC value (<6 mg/L) (28, 29). In this study, 76% (38/50 of MCRPECs strains showed susceptibility to colistin at MIC values ≤2 mg/L, 24% (12/50) showed colistin resistant phenotype, of which 33% (4/12) were resistant to colistin at high concentration (MIC >16 mg /L). In line with previous study conducted in Ha Nam in 2014–2015, the percentage of colistin-resistant MCRPECs with MIC value >6 mg /L in this study (4/50,8.0%) was <10% overall (14). However, our study reported similar prevalence of colistin-resistant E. coli strains as the study conducted in the Southern region of Vietnam, with colistin resistance rates in chickens and pigs of 22.2 and 24.4%, respectively (24). A recent study demonstrated that the acquisition of mcr-1 by E. coli is a “poisoned chalice”. The high-level expression of mcr-1 can reduce the growth rate of E. coli by compromising the bacterium's normal physiology, which has been observed in presence of selection pressure of colistin (30). Possibly the low proportion of high-level colistin resitance in MCRPECs indicates a low use of colistin in livestock setting but it permit for the omnipresence of mcr-1 in various reservoirs. ADDIN EN.CITE (31, 32)Although it is insufficient evidence on the mechanism of interaction between the mcr-1 gene and the colistin resistance of E. coli, the prevalence of MCRPECs with high MIC values in the agricultural farm environment exchangeability of genetic factors raise the alarm on the potential spread of drug-resistant strains in the community (31). This can lead to major challenges for public health systems in controlling the transmission of AMR strains of bacteria in the community.
Most of MCRPECs (74%) in this study had the MIC value at 2 mg/L coresponding with multiple reports of mcr-1 positive Enterobacteriaceae with MIC values of ≤ 2 mg/L (32). Recent data on polymyxin pharmacokinetics (PK), pharmacodynamics (PD) have shown that a steady state concentration at 2 mg/L is required for killing bacteria with MICs of 2 mg/L. However, only nearly 50% of patients with normal renal function achieve this exposure and it is of limited clinical efficacy and is associated with substantial risk of nephrotoxicity (33). The omnipresence of mcr-1 within Enterobacterales particularly in E. coli, which have been known opportunistic pathogens, thus could be a concern in clinical practice in future due to the effectiveness and safety at higher dose.
Previous study results suggested that there might be fly-mediated transmission of mcr-1 from animals and the environment to humans, but there was no specific genetic evidence to verify this hypothesis (16). By sequencing technique, analyzing family trees, and analyzing multi-locus sequence types and seven housekeeping gene alleles of 50 strains of MCRPECs, our study brings support for this hypothesis through the presence of several clusters of STs on different reservoirs and the genetic similarity of STs isolated from flies to STs isolated from other reservoirs (ST206, ST2705, ST155, ST10, and ST48). Thus, it reflects that flies are vectors that are in the constant contact with various reservoirs of E. coli, and flies as such do not have a characteristic ST because they are transient hosts of E. coli by contact with other reservoirs (34). Alarmingly, 4/11 fly samples in this study carried colistin-resistant MCRPECs at MIC values >16 mg/L. There is not enough evidence to make the assertion about the role of flies in spreading of AMR genes in livestock environments but constant contact with various reservoirs of colistin-resistant MCRPECs should be consideration.
The study also showed a diversity of STs with 50 strains of MCRPECs showing 34 different STs from 4 phylo-groups (A, B1, C, F). Phylogenetic groups A (76%) and B1 (18%) were predominant among the tested isolates while group C and group F had low prevalence 2 and 4%, respectively. This result is in agreement with those reports that indicated E. coli from animal, human, environment, and food, mainly belonged to the A and B1 phylogenetic groups (35–37). Common STs found in this study, including ST48, ST2705, ST4015, ST206, ST10, ST189 (6STs belonging to group A), ST101, and ST155 (2 STs belonging to group B1) also appeared in many studies on food, livestock farms, and the environment in other countries, such as China, Poland, Korea, Laos, Thailand, France, Algeria (28, 38–41). Furthermore, the most of the STs found in this study belonged to clonal complex 10 (CC10), which has been known as the most dominant clonal group colonized in vertebrate hosts as a commensal in the gastrointestinal tract and aquatic environments, and carries diverse antimicrobial-resistance (AMR) genes and is known to cause extra-intestinal infections (42). It is also consistent with the major prevalence of E. coli ST10 worldwide (43). Three new STs discovered in this study were isolated from chicken feces samples (ST12495, ST12946) and fly samples (ST12947), the latter one being resistant to colistin at MIC value >16 mg/L. Among them, two STs (ST12946 and ST12947) belonged to CC10. The occurrence of STs in CC10 is likely result of genetic exchange among bacterial strains from animals, humans and the environment. This is also the model that contributes to the spread of resistance genes. In many livestock farms in Vietnam, antimicrobials are commonly used in the treatment or prevention of livestock diseases, or as growth promoters, including aminoglycosides, tetracyclines, phenicols, β-lactams, colistin, sulfonamides and fluoroquinolones groups (44, 45). This is considered a cause of increased AMR in the community including in humans, animals, and environment. A retrospective study conducted in Ha Nam, Vietnam, showed high prevalence of AMR genes with varied resistance data depending on the type of sample in humans, animals, and the environment (15). MCRPECs strains isolated in this study have a high proportion of carrying resistance genes in the groups of colistin, aminoglycosides, fosfomycin, β-lactams, sulfonamides, fluoroquinolones, tetracyclines, trimethoprim, phenicols, and macrolides, which is consistent with previous reports on AMR gene-carrying data in rural Vietnamese communities (15).
E. coli strains can possess different virulence markers to invade and colonize inner layers of the intestinal lining causing infections with different clinical symptoms (46). VFs include five main groups: (1) adhesions; (2) toxins; (3) siderophores; (4) capsule production and (5) protections and invasions (47). There was a strong link between the virulence and phylogeny in E. coli infections. Commensal E. coli, with no pathogenic features and occurring, among other places, on the gastrointestinal tract mucosa, most often belong to group A or B1. Pathogenic E. coli responsible for intestinal infections belong to phylogenetic groups A, B1 or D. E. coli responsible for extra-intestinal infections belong to groups B2 and D, group F is related to the main group B2 (21, 48). These isolates in our study harbor a total of 59 different virulence genes and classified in 4 phylogroups (A, B1, C, F). Our results show that MCRPEC strains isolated from livestock, farmers, flies, environment disposed of various virulence genes. Frequently recorded virulence genes comprised genes related to invasion and iron-uptake in E. coli (invasion-aslA, ompA, kpsD, kpsM, ompA; iron-uptake chuS, chuT, chuU, chuV, chuW, chuY, entA, entB, entC, entE, entF, fepA, fepB, fepC, fepD, fepG …), the less prevalent virulence genes was reported the close related to ESBL genes such as iutA; fmH, papC encode the adhesion subunit of type 1 fimbriae and related to colonization. Overal, the presence of MCRPECs bearing different virulence genes which are reported in extraintestinal pathogenic E. coli (ExPEC) (49). This can be considered a warning for the risk of infection by pathogenic strain from communities.
Studies on the mcr-1 gene showed that there were many types of plasmids that could carry mcr-1 belonging to incompatibility groups IncX4, IncI2, InHI2, IncF, IncFIB, IncY and IncP-1 (11, 50). In this research, up to 10 plasmids carrying mcr-1 gene are reported, including common plasmids reported worldwide, in which the most prevalent is IncP-1 (17/37, 45.9%), followed by IncX4 (7/37, 18.9%). IncP-1 plasmid group is widely distributed in feces, soil, wastewater treatment plants and in both pathogenic and opportunistic species of bacteria, playing an important role in encoding multidrug resistance and bacterial adaptation by horizontal gene transfer (51). 45.9% of MCRPEC strains in this study have IncP-1 plasmid, which is a serious concern as IncP-1 plasmids can encode multidrug resistance, carry adaptive genes, AMR genes, virulence genes to migrate to new host through conjugated transfer and remains stable in bacterial cells (51). Therefore, we believe that in the future, deeper studies to determine the extent and risk of spreading resistance genes located on these plasmids are highly needed. Two major plasmids in our study, IncX4 (18.9%) and IncHI2/IncHI2A (16.2%) were also common in clinical intestinal bacteria strains associated with the prevalence of AMR genes (50, 52, 53). Thus, the AMR gene diversity and AMR phenotype of MCRPECs strains in this study could be the result of horizontal gene transfer from different strains on different hosts. These strains could become the next transmission threat due to broad-host range plasmids. A report from Americas also suggest that the epidemiology of the mcr-1 gene in the Americas is mostly determined by plasmid spreading instead of clonal dissemination of MCRPE strains (43).
Recent studies have proposed that the mcr-1 is mobilized by a composite transposon (Tn6330) flanked by two ISApl1 insertion sequences. In this study, we found full genetic context of the Tn6330 transposon, which was found on only chromosome of three E. coli isolates. In line with previous studies, these findings suggested that the insertion of mcr-1 into the chromosome of E. coli occurred recently. However, we also detected a loosening of ISApl1 insertion sequences on both chromosome and plasmid harboring mcr-1, indicating that the mcr-1 is being stabilized on the core genetic backbone in the ecological niches (54).
This study revealed the high possibility of spreading drug resistance genes through the vector mediated by flies in livestock farms in Ha Nam. This process is occurring alarmingly in livestock environments with clear evidence, in which samples from humans, livestock, wastewater, and traditional markets obtained strains of MCRPEC that were resistant to many antimicrobials. In the studied strains, the appearance of new STs is a sign of continuous recombination process between bacterial strains from the community and the environment. The occurrence of mcr-1 in different plasmids indicates high mobility of this gene in E. coli as a host. One limitation in this study is the lack of access to information on the use of antimicrobials in farms to be able to evaluate the impact of selective pressure of livestock antimicrobials on collected strains.
Data availability statement
The data of this study have been deposited in the European Nucleotide Archive (ENA) at EMBL-EBI under accession number PRJEB55625 (https://www.ebi.ac.uk/ena/browser/view/PRJEB55625).
Author contributions
HHT, HRvD, A-LB, DAD, and NDT design the study and directed study implementation. HHT, TMHT, PTLN, DTP, TTTN, and TPLN managed the fieldwork. HHT, HTN, and HAT performed laboratory work. PTLN, DTP, HTN, DLT, and THHN managed data. HHT, PTLN, VTL, THHN, TNBV, MC, and MS analyzed data. HHT, PTLN, TNBV, and THHN developed the first draft of the paper. All the authors reviewed and edited drafts of the manuscript and approved the final version.
Funding
This study is funded by Vietnam National Foundation for Science and Technology Development (NAFOSTED) under grant number 108.02-2017.320, GCRF OneHealth Poultry Hub (Royal Veterinary College, UK) under grant number BB/S001269/1, the Japan Agency for Medical Research and Development (AMED) under grants numbers JP22gm1610003, JP22fk0108133, JP22fk0108139, JP22fk0108642, JP22wm0225004, JP22wm0225008, JP22wm0225022, JP22wm0325022, JP22wm0325003, and JP22wm0325037, and from IRD and LMI DRISA.
Acknowledgments
We are grateful to colleagues in Ha Nam province for assistance with collecting samples in the field. We express our appreciation to Dr. Pham Thi Ngoc from National Institute of Veterinary Research Vietnam for her scientific advisory during this research.
Conflict of interest
The authors declare that the research was conducted in the absence of any commercial or financial relationships that could be construed as a potential conflict of interest.
Publisher's note
All claims expressed in this article are solely those of the authors and do not necessarily represent those of their affiliated organizations, or those of the publisher, the editors and the reviewers. Any product that may be evaluated in this article, or claim that may be made by its manufacturer, is not guaranteed or endorsed by the publisher.
Supplementary material
The Supplementary Material for this article can be found online at: https://www.frontiersin.org/articles/10.3389/fvets.2022.1034610/full#supplementary-material
References
1. WHO. Antimicrobial resistance. (2021). Available online at: https://www.who.int/news-room/fact-sheets/detail/antimicrobial-resistance (accessed January 10, 2022).
2. EclinicalMedicine. Antimicrobial resistance: a top ten global public health threat. EClinicalMedicine. (2021) 41:101221. doi: 10.1016/j.eclinm.2021.101221
4. Bryan AW, Dishon MM, Bram ADVB. Quantifying the transmission of antimicrobial resistance at the human and livestock interface with genomics. Clin Microbiol Infect. (2020) 26:1612–6. doi: 10.1016/j.cmi.2020.09.019
5. Scott AM, Paula JFC. Antimicrobial use and resistance in animals. Clinical Infect Dis. (2002) 34:S93-S106. doi: 10.1086/340246
6. Audrey W, Violeta C, Richard SPH, Amer W, Jeffrey A, Amitava D. Antibiotics, antimicrobial resistance, antibiotic susceptibility testing, and therapeutic drug monitoring for selected drugs. In: Microbiology and Molecular Diagnosis in Pathology. (2017). p. 119–53. doi: 10.1016/B978-0-12-805351-5.00007-7
7. Dror M, Teena C, Jason MP, Federico P, Andrea MH, Susan R, et al. Outbreak of colistin-resistant, carbapenem-resistant Klebsiella pneumoniae in metropolitan Detroit, Michigan. Antimicrob Agents Chemother. (2011) 55:593–9. doi: 10.1128/AAC.01020-10
8. Haley JM, Jason MP, Keith SK, Kerry LLP. Treatment options for carbapenem-resistant enterobacteriaceae infections. Open Forum Infect Dis. (2015) 2:ofv050. doi: 10.1093/ofid/ofv050
9. Michele Y, Aurora EPV. Treatment for infections with carbapenemresistant Enterobacteriaceae: what options do we still have? Critical Care. (2014) 18:229. doi: 10.1186/cc13949
10. Isabella Kempf, Eric J, Claire C. Colistin use and colistin resistance in bacteria from animals. Int J Antimicrob Agents. (2016) 48:598–606. doi: 10.1016/j.ijantimicag.2016.09.016
11. Lauren P, Jean-Yves M, Agnese L, Anne-Kathrin S, Nicolas K, Patrice N, et al. Antimicrobial resistance in Escherichia coli. Microbiol Spectr. (2018) 6:6–4. doi: 10.1128/microbiolspec.ARBA-0026-2017
12. Keith SK, Jason MP, Thien BT, Roger LN, Jian L. Agents of last resort: polymyxin resistance. Infect Dis Clin North Am. (2016) 30:391–414. doi: 10.1016/j.idc.2016.02.005
13. George V, Ioanna K. Colistin resistant mcr genes prevalence in livestock animals (Swine, Bovine, Poultry) from a multinational perspective. A systematic review. Vet Sci. (2021). 8:265. doi: 10.3390/vetsci8110265
14. Vu Thi Ngoc B, Le Viet T, Nguyen Thi Tuyet M, Nguyen Thi Hong T, Nguyen Thi Ngoc D, Le Van D, et al. Characterization of genetic elements carrying mcr-1 gene in Escherichia coli from the community and hospital settings in Vietnam. MicrobiolSpectrum. (2022) 10:e01356-21. doi: 10.1128/spectrum.01356-21
15. Bich VTN, Thanh LV, Mai NTT, Thuong NTH, Diep NTN, Duyet LV, et al. An exploration of the gut and environmental resistome in a community in northern Vietnam in relation to antibiotic use. Antimicrob Resist Infect Control. (2019) 8:194. doi: 10.1186/s13756-019-0645-9
16. Phuong NTL, Hung TTM, Anh TH, Thai PD, Tan LM, Thanh NH, et al. Carriage of plasmid-mediated colistin resistance-1-positive escherichia coli in humans, animals, and environment on farms in Vietnam. Am J Trop Med Hygiene. (2021) 107:65–71. doi: 10.4269/ajtmh.21-1203
17. Akiyo N, Ryuichi N, Ryuji N, Yuki S, Saori H, Takane KU, et al. Prevalence and relatedness of mcr-1-mediated colistin-resistant Escherichia coli isolated from livestock and farmers in Japan. Front Microbiol. (2021) 12:664931. doi: 10.3389/fmicb.2021.664931
19. CLSI. M100-Performance Standards for Antimicrobial Susceptibility Testing. 2018, Clinical and Laboratory Standards Institute.
20. EUCAST. Clinical breakpoints—breakpoints and guidance. (2018). Available online at: https://www.eucast.org/clinical_breakpoints/ (accessed January 10, 2022).
21. Olivier Clermont J, Erick D, David MG The clermont Escherichia coliphylo-typing method revisited: improvement of specificity and detection of new phylo-groups. Environ Microbiol Rep. (2013) 5:58–65. doi: 10.1111/1758-2229.12019
22. Yang W, Rongmin Z, Jiyun L, Zuowei Y, Wenjuan Y, Stefan S, et al. Comprehensive resistome analysis reveals the prevalence of NDM and MCR-1 in Chinese poultry production. Nat Microbiol. (2017) 2:16260. doi: 10.21037/jlpm.2017.06.02
23. Takahiro Y, Ryuji K, Kazuo H, Shihono T, Tatsuya N, Daisuke M, et al. The presence of colistin resistance gene mcr-1 and−3 in ESBL producing Escherichia coli isolated from food in Ho Chi Minh City, Vietnam. FEMS Microbiol Lett. (2018). 365:fny100. doi: 10.1093/femsle/fny100
24. Nhung NT, Hoa NM, Cuong NV, Trung NV, Men NT, Hieu TQ, et al. Use of colistin and other critical antimicrobials on pig and chicken farms in Southern Vietnam and its association with resistance in commensal Escherichia coli bacteria. Appl Environ Microbiol. (2016) 82:3727–35. doi: 10.1128/AEM.00337-16
25. Tatsuya N, Michio J, Ryuji K, Diep KT, Thang NM, Hoa TT, et al. Frequent use of colistin-based drug treatment to eliminate extended-spectrum beta-lactamase-producing Escherichia coli in backyard chicken farms in Thai Binh Province, Vietnam. Trop Anim Health Prod. (2017) 49:31–7. doi: 10.1007/s11250-016-1154-y
26. Vietnamese Ministry of Agriculture and Rural Development. Circular 20/2017/TT-BNNPTNT Guidelines for the implementation of Decree No. 39/2017/ND-CP dated April 4, 2017 of the Government on the management of animal culture and aquaculture. (2017).
27. Seydina MD, Jean-Marc R. Carbapenemase genes and genetic platforms in Gram-negative bacilli: Enterobacteriaceae, Pseudomonas and Acinetobacter species. Clin Microbiol Infect. (2014) 20:831–8. doi: 10.1111/1469-0691.12655
28. Linda H, Toihata R, Yan Z, Jian L, Seydina MD, Jean-Marc R. Study of mcr-1 gene-mediated colistin resistance in enterobacteriaceae isolated from humans and animals in different countries. Genes. (2017) 8:394. doi: 10.3390/genes8120394
29. Xianhui H, Linfeng Y, Xiaojie C, Chanping Z, Xu Y, Yiyun L, et al. High prevalence of colistin resistance and mcr-1 gene in Escherichia coli isolated from food animals in China. Front Microbiol. (2017) 8:562. doi: 10.3389/fmicb.2017.00562
30. Qiue Y, Mei L, Owen BS, Diego OA, Philip H, Hui L, et al. Balancing mcr-1 expression and bacterial survival is a delicate equilibrium between essential cellular defence mechanisms. Nat Commun. (2017) 8:2054. doi: 10.1038/s41467-017-02149-0
31. Nordmann P, Poirel L. Plasmid-mediated colistin resistance: an additional antibiotic resistance menace. Clin Microbiol Infect. (2016) 22:398–400. doi: 10.1016/j.cmi.2016.03.009
32. Jason MP, Ronald NJ, John SB, David RA, Sujata MB, George LD, et al. Polymyxin susceptibility testing and interpretive breakpoints: recommendations from the United States committee on antimicrobial susceptibility testing (USCAST). Antimicrob Agents Chemother. (2020) 64:e01495–19. doi: 10.1128/AAC.01495-19
33. Michael JS, James SL, Melvin PW, Jean P, Romney MH, Gunnar K, et al. Clinical and laboratory standards institute and european committee on antimicrobial susceptibility testing position statements on polymyxin b and colistin clinical breakpoints. Clin Infect Dis. (2020) 71:e523–9.
34. Ji-Hang J, Patrick JK, Chengming W. Flies as vectors and potential sentinels for bacterial pathogens and antimicrobial resistance: a review. Vet Sci. (2022). 9:300. doi: 10.3390/vetsci9060300
35. Mabel KA, Jacob KPK, Rene SH, Emmanuel CO, Siddhartha T. Genetic relatedness of multidrug resistant Escherichia coli isolated from humans, chickens and poultry environments. Antimicrob Resist Infect Control. (2021) 10:58. doi: 10.1186/s13756-021-00930-x
36. Rabee AO, Atsushi H, Sharda PA, Atsushi I, Ayaka S, Abdel-Rahman ME, et al. Prevalence and pathogenic potential of Escherichia coli isolates from raw milk and raw milk cheese in Egypt. Int J Food Microbiol. (2016) 221:69–76. doi: 10.1016/j.ijfoodmicro.2016.01.009
37. Ping C, Yuqi Y, Sai C, Haibin L, Xiaoting L, Jichao S, et al. Prevalence and characteristic of swine-origin mcr-1-positive Escherichia coli in Northeastern China. Front Microbiol. (2021) 12:712707. doi: 10.3389/fmicb.2021.712707
38. Yong-Qiang Y, Yun-Xia L, Tao S, Yan-Xian Y, Wei J, An-Yun Z, et al. Colistin resistance gene mcr-1 and its variant in Escherichia coli isolates from chickens in China. Antimicrob Agents Chemother. (2017) 61:e01204–16. doi: 10.1128/AAC.01204-16
39. Sung-Suck Oh, Jihyun S, Junghee K, Jinwook S. Increasing prevalence of multidrug-resistant mcr-1-positive Escherichia coli isolates from fresh vegetables and healthy food animals in South Korea. Int J Infect Dis. (2020) 92:53–55. doi: 10.1016/j.ijid.2019.12.025
40. Magdalena Z, Pawel S, Valeria B, Pimlapas L, Lina MC, Anna ZB, et al. Occurrence and characterization of mcr-1-positive Escherichia coli isolated from food-producing animals in Poland, 2011–2016. Front Microbiol. (2019) 10:1753. doi: 10.3389/fmicb.2019.02816
41. Cong S, Lan-Lan Z, Yongqiang Y, Yohei D, David LP, Nicole S, et al. Dynamics of mcr-1 prevalence and mcr-1-positive Escherichia coli after the cessation of colistin use as a feed additive for animals in China: a prospective cross-sectional and whole genome sequencing-based molecular epidemiological study. The Lancet Microbe. (2020) 1:e34–43. doi: 10.1016/S2666-5247(20)30005-7
42. Cameron JR, Matthew ZD, Steven PD. Australian porcine clonal complex 10 (CC10) Escherichia coli belong to multiple sublineages of a highly diverse global CC10 phylogeny. Microb Genom. (2019) 5:e000225. doi: 10.1099/mgen.0.000225
43. Rodríguez-Santiago J, Cornejo-Juárez P, Silva-Sánchez J, Garza-Ramos U. Polymyxin resistance in Enterobacterales: overview and epidemiology in the Americas. Int J Antimicrob Agents. (2021) 58:106426. doi: 10.1016/j.ijantimicag.2021.106426
44. Cuong NV, Nhung NT, Nghia NH, Hoa NTM, Trung NV, Guy T, et al. Antimicrobial consumption in medicated feeds in vietnamese pig and poultry production. Ecohealth. (2016) 13:490–8. doi: 10.1007/s10393-016-1130-z
45. Dang PK, Claude S, Caroline D, Ton VD, Bo HX, Binh DV, et al. First survey on the use of antibiotics in pig and poultry production in the red river delta region of Vietnam. Food Public Health. (2013) 3:10. doi: 10.5923/j.fph.20130305.03
46. Olukayode OO, Salihu MG, Victor SF. Characteristics of virulence factors and prevalence of virulence markers in resistant escherichia coli from patients with gut and urinary infections in Lafia, Nigeria. Microbiol Insights. (2022). 15:11786361221106993. doi: 10.1177/11786361221106993
47. Qing-wen H, Xiao-hong X, Fang-jun L, Zhi-chang Z, Zhi-yun W, Ying-ping C, et al. Molecular characteristic of mcr-1 producing Escherichia coli in a Chinese university hospital. Ann Clin Microbiol Antimicrob. (2017) 16:32. doi: 10.1186/s12941-017-0207-z
48. Dobroslava B, Lívia K, Vladimír K. Escherichia coli specific virulence-gene markers analysis for quality control of ovine cheese in Slovakia. Microorganisms. (2021) 9:1808. doi: 10.3390/microorganisms9091808
49. Jolanta S, Bozena FK, Agnieszka JK, Magdalena FM, Marta K, Marta K, et al. Virulence factors, prevalence and potential transmission of extraintestinal pathogenic Escherichia coli isolated from different sources: recent reports. Gut Pathog. (2019) 11:10. doi: 10.1186/s13099-019-0290-0
50. Laurent P, Nicolas K, Patrice N. In vitro study of ISApl1-mediated mobilization of the colistin resistance gene mcr-1. Antimicrob Agents Chemother. (2017) 61:e00127–17. doi: 10.1128/AAC.00127-17
51. Magdalena P, Agata KB. Broad-host-range IncP-1 plasmids and their resistance potential. Front Microbiol. (2013) 4:44. doi: 10.3389/fmicb.2013.00044
52. Aurora GF, Alessandra C. Plasmid double locus sequence typing for IncHI2 plasmids, a subtyping scheme for the characterization of IncHI2 plasmids carrying extended-spectrum beta-lactamase and quinolone resistance genes. J Antimicrob Chemother. (2010) 65:1155–61. doi: 10.1093/jac/dkq101
53. Jian S, Liang-Xing F, Zuowei W, Hui D, Run-Shi Y, Xing-Ping L, et al. Genetic analysis of the IncX4 plasmids: implications for a unique pattern in the mcr-1 acquisition. Sci Rep. (2017) 7:424. doi: 10.1038/s41598-017-00095-x
Keywords: mcr-1, E. coli, resistance, livestock, farm, plasmid harboring mcr-1, colistin resistance, antimicrobial resistance
Citation: Nguyen PTL, Ngo THH, Tran TMH, Vu TNB, Le VT, Tran HA, Pham DT, Nguyen HT, Tran DL, Nguyen TPL, Nguyen TTT, Tran ND, Dang DA, Bañuls A-L, Choisy M, van Doorn HR, Suzuki M and Tran HH (2022) Genomic epidemiological analysis of mcr-1-harboring Escherichia coli collected from livestock settings in Vietnam. Front. Vet. Sci. 9:1034610. doi: 10.3389/fvets.2022.1034610
Received: 01 September 2022; Accepted: 05 October 2022;
Published: 26 October 2022.
Edited by:
Ulises Garza-Ramos, National Institute of Public Health, MexicoReviewed by:
Jonathan Rodriguez-Santiago, Center for Research on Infectious Diseases (CISEI), MexicoRigoberto Hernandez-Castro, Hospital General Dr. Manuel Gea Gonzalez, Mexico
Copyright © 2022 Nguyen, Ngo, Tran, Vu, Le, Tran, Pham, Nguyen, Tran, Nguyen, Nguyen, Tran, Dang, Bañuls, Choisy, van Doorn, Suzuki and Tran. This is an open-access article distributed under the terms of the Creative Commons Attribution License (CC BY). The use, distribution or reproduction in other forums is permitted, provided the original author(s) and the copyright owner(s) are credited and that the original publication in this journal is cited, in accordance with accepted academic practice. No use, distribution or reproduction is permitted which does not comply with these terms.
*Correspondence: Huy Hoang Tran, thh@nihe.org.vn
†These authors have contributed equally to this work and share first authorship