- 1College of Veterinary Medicine, Inner Mongolia Agricultural University, Hohhot, China
- 2Key Laboratory of Clinical Diagnosis and Treatment Technology in Animal Disease, Ministry of Agriculture and Rural Affairs, Hohhot, China
- 3Animal Disease Control Center of Ordos, Ordos City, China
- 4Inner Mongolia Saikexing Reproductive Biotechnology (Group) Co., Ltd., Hohhot, China
- 5Shanghai Origingene Bio-pharm Technology Co. Ltd., Shanghai, China
Ticks were identified as arthropods that are pathogenic vectors. Dermacentor nuttalli is one of the dominant tick species in Inner Mongolia, and it carries and transmits a wide range of pathogenic microorganisms. However, at present, only the detection of D. nuttalli adult ticks and D. nuttalli different developmental stages carrying one specific pathogen, or the next-generation sequencing of D. nuttalli adult ticks were available. In this study, we investigated the microbial community structures of D. nuttalli in different growth stages under laboratory artificial feeding conditions. Total DNA was extracted from seven growth stages (female adult ticks, eggs, larval ticks, engorged larval ticks, nymphal ticks, engorged nymphal ticks, and second-generation adult ticks) obtained from laboratory artificial feeding of engorged D. nuttalli female ticks in Inner Mongolia. Then, the 16S rDNA V3–V4 hypervariable region was amplified to construct an Illumina PE250 library. Finally, 16S rRNA sequencing was performed on Illumina Novaseq 6000 platform. The sequencing data were analyzed using molecular biology software and platforms. The Illumina PE250 sequencing results showed that the egg stage had the highest diversity and number of species (28.74%, 98/341), while the engorged nymph stage had the lowest diversity and number of species (9.72%, 21/216). A total of 387 genera of 22 phyla were annotated in D. nuttalli, with 9 phyla and 57 genera found throughout all 7 growth stages. The dominant phylum was Proteobacteria; the dominant genera were Arsenophonus and Rickettsia; and the genera with the highest relative abundance in the 7 growth stages were Pseudomonas, Paenalcaligenes, Arsenophonus, Arsenophonus, Pseudomonas, Arsenophonus, and Rickettsia, respectively. Among the 23 exact species annotated, Brucella melitensis exhibits pathogeny that poses a serious threat to humans and animals. In this study, the microbial community composition at different growth stages of D. nuttalli was comprehensively analyzed for the first time.
Introduction
Ticks are bloodsucking ectoparasites found on mammalian, avian, reptilian, and amphibian body surfaces (1). Ticks are classified into 4 families (Ixodidae, Argasidae, Nuttalliellidae, and Deinocrotonidae), with 949 species of ticks worldwide, including 731 species of Ixodidae (2). China recorded 2 families of 124 tick species, of which 113 were hard-tick species. Ticks are the most common in Central China, South China, and Inner Mongolia-Xinjiang, with 56, 54, and 46 hard-tick species, respectively (3). Ticks from the family Ixodidae are the most common and harmful, with four developmental stages: egg, larva, nymph, and adult, with the larva, nymph, and adult stages requiring host blood to complete their development (4). Ticks can directly harm the host by feeding on their blood; Additionally, they can carry and transmit pathogenic microorganisms such as bacteria, viruses, protozoan parasites, and spirochetes (5–8). They are the world's second largest group of vectors, after mosquitoes (9, 10).
Dermacentor nuttalli belongs to the family Ixodidae and genus Dermacentor, and it is one of the dominant tick species in the Inner Mongolia region (11). In addition to the Inner Mongolia region, D. nuttalli individuals carrying multiple microorganisms have been identified in the Xinjiang, Yunnan, Gansu, Shaanxi, Shanxi, Heilongjiang, and Jilin Province, etc. Moreover, D. nuttalli individuals carrying microorganisms have been found in the neighboring countries of Mongolia and Russia (Table 1). The above studies are sufficient to prove that D. nuttalli has a strong ability to carry and transmit pathogenic microorganisms. Different species of ticks prefer distinct biotopes or environments, which determines the geographic distribution of ticks (10). This is coupled with the fact that different tick species carry and transmit different pathogens (12). Hence, a comprehensive analysis of microbial communities transmitted by D. nuttalli in Inner Mongolia is important for assessing the risk of local tick-borne diseases.
High-throughput sequencing technology is quick and efficient for analyzing tick microbial communities, thereby eliminating the need for bacterial culture techniques and allowing the identification of non-culturable bacteria and unknown pathogens (13). The study of 16S rDNA regions can be used to identify species and as indicators in microbial systematics, classification, and identification. The V3–V4 hypervariable regions are the most accurate for identifying organisms at the genus level (14). Previous studies have mainly focused on detecting D. nuttalli adult ticks (15–33) and D. nuttalli different developmental stages (34–40) carrying one specific pathogen or on next-generation sequencing of D. nuttalli adult ticks (41–44). The microbial community structure and diversity of D. nuttalli at different developmental stages have not been comprehensively compared. In this study, we used different growth stages of D. nuttalli as part of the study objectives under laboratory artificial feeding conditions and sequenced the V3–V4 hypervariable regions based on the 16S rDNA region using the Illumina Novaseq 6000 platform to determine the microbial community structure and diversity of D. nuttalli at various growth stages and to provide a scientific basis for risk assessment and prevention of D. nuttalli and its tick-borne diseases.
Materials and methods
Study areas and sample collection
Ticks used in this study were from a colony of D. nuttalli maintained at the Tick Research Laboratory, Inner Mongolia Agricultural University, College of Veterinary Medicine, Hohhot, using mice as hosts for all growth stages. This colony originated from field collections of ticks from the same sheep in New Barag Left Banner, Hulunbeier, Inner Mongolia (619 m above sea level; 48°48′N, 118°24′E), who had no other obvious abnormalities except emaciation. Ticks were manually pulled from the sheep using forceps and placed directly into a 50 mL centrifuge tube, after which the nozzle was plugged close with damp cotton and transported to the laboratory. Engorged adult female D. nuttalli ticks were identified in the laboratory using morphological and molecular biological methods. The remaining six growth stages (egg, larval ticks, engorged larval ticks, nymphal ticks, engorged nymphal ticks, and second-generation adult ticks) were obtained from the colony in the laboratory (Figure 1), and three replicates of each stage were set up (each sample was mixed), yielding a total of 21 samples stored at −80°C.
DNA extraction
Each sample was washed three times in 70% ethanol and sterile deionized water, followed by extraction using the TaKaRa MiniBEST Universal Genomic DNA extraction kit in the biosafety cabinet. The 5.0 (Takara, Beijing, China, Code No. 9765) kit was used to extract total DNA from samples based on the manufacturer's instructions, and the DNA was stored temporarily at −20°C.
Polymerase chain reaction amplification and high-throughput sequencing
The 16S rDNA V3–V4 region was amplified using the TransStart® FastPfu DNA polymerase (TransStart, Beijing, China, Code No. AP221-02) kit, using sample DNA as a template and specific barcode primers. The primer was 338F: 5'-ACTCCTACGGGAGGCAGCA-3′, 806R: 5′-GGACTACHVGGGTWTCTAAT-3′. The annealing temperature was 55°C. The PCR products were recovered by gel extraction using the AXYPREP DNA gel extraction kit (AXYGEN, Suzhou, China, Code No. AP-GX-50G), which were tested for conformance using 2% agarose gel electrophoresis, and then the VAHTS® ssDNA Library Prep kit (Illumina, San Diago, USA, Code No. ND6201) was used to construct the Illumina PE250 library, followed by high-throughput sequencing of 16S rDNA on the Illumina Novaseq 6000 platform (San Diego, CA, USA).
Data analysis
For Illumina PE250 sequencing sequences, we first obtained valid sequences for all samples based on the barcode. Then, paired-end reads from the original DNA fragments were merged using FLASH (version 1.2.11). Sequence analysis was performed using the UPARSE software package (version 7.0.1090 http://drive5.com/uparse/) (45). Based on sequences with ≥97% similarity, all sequences were categorized and operational taxonomic units (OTUs) were generated. The RDP Classifier version 2.2 (http://sourceforge.net/projects/rdp-classifier/) (46) bayesian algorithm was used to perform taxonomic analysis of OTU representative sequences from domain to species. For single-sample diversity analysis (alpha diversity), we calculated the number of unique OTUs in each sample, the Chao index of community richness [the Chao1 estimator (http://www.mothur.org/wiki/Chao)], the Shannon index for community diversity (http://www.mothur.org/wiki/Shannon), Simpson index (http://www.mothur.org/wiki/Simpson), and the sequencing depth coverage [the Good's coverage (http://www.mothur.org/wiki/Coverage)]. Species accumulation curves were combined to determine whether the sample size was sufficient to assess and predict species richness (47). The number of common and unique OTUs in multiple samples was counted using the R language and displayed in Venn diagrams (48). The composition of microbial communities was presented using histograms based on OTU data using the R language (49).
Sequencing and sequence analysis were performed in collaboration with Origin-gene biology Co., Ltd. (Shanghai, China).
Results
General statistics
The sequencing results of all samples were corrected, and chimeras were removed to obtain 3,437,890 optimized sequences, including 499,595 from female adult ticks; 518,688 from eggs; 534,255 from larval ticks; 492,368 from engorged larval ticks; 519,116 from nymphal ticks; 472,277 from engorged nymphal ticks; and 401,591 from second-generation adult ticks. The base number of these optimized data was 1,452,643,023 bp. The average sequence length of these optimized data was 422.54 bp, and 99.92% of the sequences were mapped to the 401–440 bp regions. There were 3,305,190 high-quality, effective, where clean reads were generated for the analysis using OTU selection and taxonomic assignments (Table 2).
Alpha-diversity analysis
The alpha-diversity indices (Shannon, Simpson, Chao, ACE, and Good's coverage) were calculated based on a 97% similarity level, which showed that the Shannon index values were eggs > nymphal ticks > larval ticks > second-generation adult ticks > female adult ticks > engorged larval ticks > engorged nymphal ticks; the Simpson index values were eggs < nymphal ticks < larval ticks < second-generation adult ticks < female adult ticks < engorged larval ticks < engorged nymphal ticks; and the Chao and ACE indices were eggs > larval ticks > nymphal ticks > engorged larval ticks > female adult ticks > second-generation adult ticks > engorged nymphal ticks. The Good's coverage of all samples exceeded 99.9% (Table 3). The species accumulation curves (Figure 2) showed that as sample size increased, the curves first increased sharply and then plateaued.
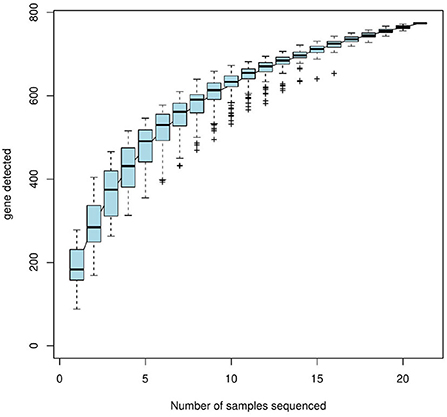
Figure 2. Species accumulation curves (horizontal coordinate, sample size, vertical coordinate, and number of OTUs after sampling).
OTU cluster analysis
The Venn diagram (Figure 3) shows that 221, 341, 350, 265, 353, 216, and 259 OTUs were obtained for female adult ticks, eggs, larval ticks, engorged larval ticks, nymphal ticks, engorged nymphal ticks, and second-generation adult ticks, respectively. Of these, 53 OTUs showed high similarity in the 7 growth stage samples of D. nuttalli, and the unique OTUs for the 7 growth stage samples were 25 (11.31%, 25/221), 98 (28.74%, 98/341), 45 (12.86%, 45/ 350), 33 (12.45%, 33/265), 49 (13.88%, 49/353), 21 (9.72%, 21/216), and 28 (10.81%, 28/259), respectively. In all samples, most tags were classified and >87.07% of tags were assigned to the genus level. Only a small percentage of tags could be accurately classified at the species level.
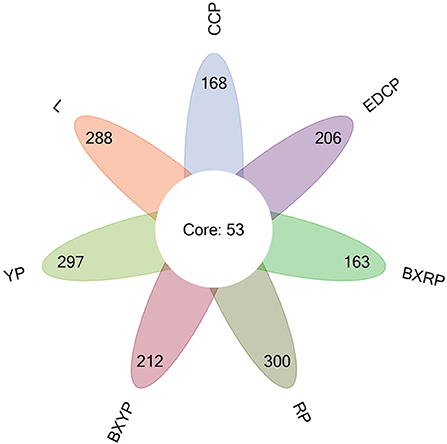
Figure 3. Venn diagram of seven samples based on OTUs (CCP, female adult ticks; L, eggs, YP, larval ticks; BXYP, engorged larval ticks; RP, nymphal ticks; BXRP, engorged nymphal ticks; EDCP, second-generation adult ticks).
Microbial population
Microbial community composition at the phylum level
A total of 22 bacterial phyla were identified in D. nuttalli, with 9 phyla found in 7 growth stages, only 4 phyla (Proteobacteria, Firmicutes, Bacteroidetes, and Actinobacteria) with an abundance of >1% in every growth stage. Proteobacteria had the highest relative abundance, with a total abundance of 85.10% in all samples and relative abundances of 52.27–98.93% in different growth stages, indicating a marked predominance. Firmicutes had a total abundance of 8.12% in all samples and the highest relative abundances in eggs (32.76%) but only 0.33% in engorged larval ticks. In addition, the total abundances of Bacteroidetes and Actinobacteria in all samples were 4.31 and 2.43%, respectively, and the relative abundances of these families in different growth stages were 0.04–13.03% and 0.21–9.04%, respectively (Figure 4; Table 4).
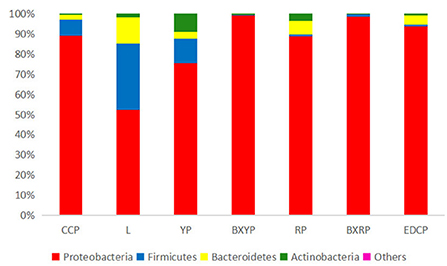
Figure 4. Microbial community bar plot of bacterial phylum of seven samples (for the best view, relative abundances of <0.05% are merged into other).
Microbial community composition at the genus level
A total of 387 bacterial genera were identified in D. nuttalli, and 57 genera were present in every growth stage of D. nuttalli. There were 30 bacterial genera with high relative abundances in all samples of D. nuttalli (16 genera with relative abundances of >1%), 17 of which belonged to Proteobacteria. The top six bacterial genus in terms of relative abundance belonged to Proteobacteria (Figure 5). The bacterial genus with the highest relative abundance was Arsenophonus, with a total abundance of 23.70% in all samples. There were large differences in abundances in different growth stages as well as a high abundance in engorged ticks and larval ticks, wherein the relative abundances were 64.94 and 54.90%, respectively, and the lowest relative abundance was found in female adult ticks (0.15%). Rickettsia was the second most abundant bacterial genus in D. nuttalli (total abundance of 19.12%) and was found in all growth stages, with the highest relative abundance being the engorged larva stage (38.79%), followed by the engorged nymph stage (29.06%), and lower relative abundances in eggs and nymphal ticks (3.04 and 4.69%, respectively; the abundances in both adult stages were approximately the same (23.22% for female adult ticks and 23.38% for second-generation adult ticks). Pseudomonas was found primarily in female adult ticks (31.30%) and nymphal ticks (27.76%), with low relative abundances in engorged larval ticks (0.06%) and engorged nymphal ticks (0.01%). Alcaligenes had the highest relative abundance in second-generation adult ticks (17.36%), but abundance was only 0.005% in female adult ticks. Brevundimonas had the highest relative abundance in nymphal ticks (14.99%) but disappeared completely in the engorged nymph stage (0.008%). Stenotrophomonas was mainly found in female adult ticks (15.28%) and in low abundance in engorged larval ticks and engorged nymphal ticks (0.01 and 0.003%, respectively). Some common zoonotic pathogenic bacterial genus such as Staphylococcus, Coxiella, and Brucella were also detected, with total abundances of 3.14, 1.58, and 1.32% in all samples of D. nuttalli, respectively (Table 5).
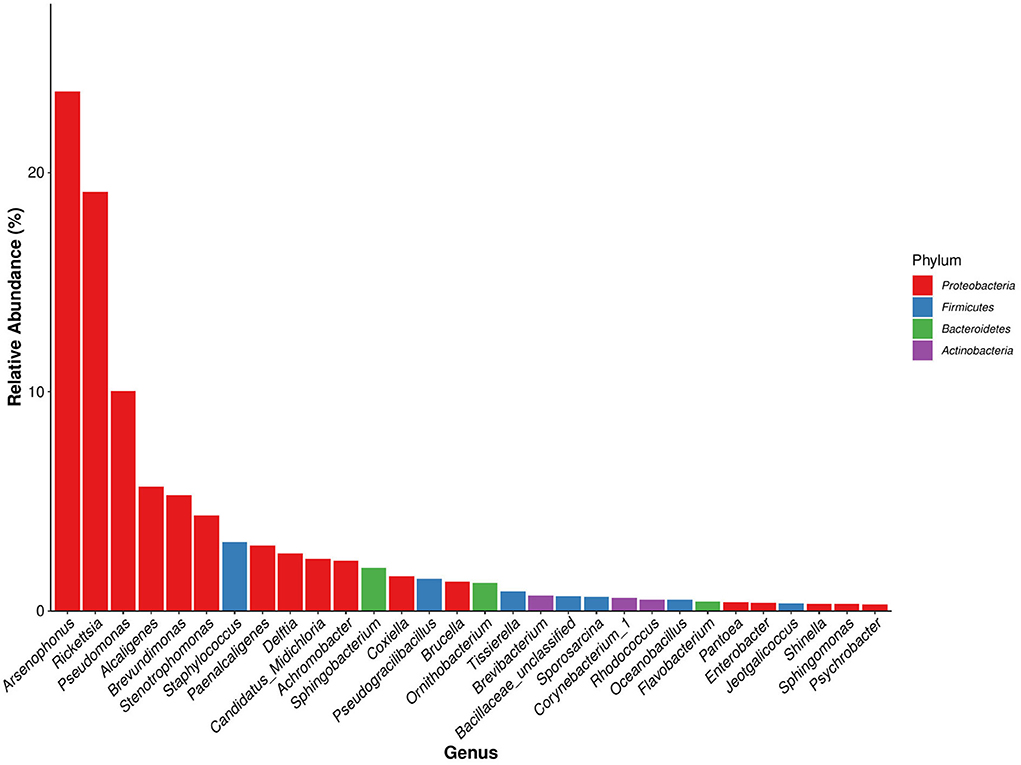
Figure 5. Dominant species bar plot of bacterial genus of Dermacentor nuttalli (a bacterial genus of the same color indicates that it is derived from the same bacterial phylum).
Some bacterial genera were present in specific growth stages, e.g., Stenotrophomonas was found in all six growth stages but not in eggs; Paenalcaligenes was detected in eggs and nymphal ticks but not in other stages; Pseudogracilibacillus was detected in eggs, larval ticks, engorged larval ticks, and nymphal ticks but not in female adult ticks, engorged nymphal ticks, and second-generation adult ticks; and Ornithobacterium was found only in eggs. Some bacterial genera with total abundances of <1% were also present in some growth stages, including Tissierella, Bacillaceae unclassified, Sporosarcina, Oceanobacillus, Flavobacterium, Pantoea, and Psychrobacter (Table 5).
The dominant genera of D. nuttalli at different growth stages differed from the overall dominant genera of all samples of D. nuttalli. The genera with higher relative abundances among female adult ticks were Pseudomonas (31.30%), Rickettsia (23.22%), Stenotrophomonas (15.28%), Staphylococcus (7.72%), and Candidatus Midichloria (7.31%). The genera with the highest relative abundances of eggs were Paenalcaligenes (20.63%), Pseudogracilibacillus (10.06%), Ornithobacterium (9.03%), and Coxiella (8.01%). Arsenophonus had the highest relative abundances of both larval ticks and engorged larval ticks (38.05 and 54.90%, respectively). The relative abundance of Rickettsia increased from 11.64% in the larval tick stage to 38.79% in the engorged larval tick stage. The relative abundance of Staphylococcus decreased from 9.65% in the larval tick stage to 0.18% in the engorged larval tick stage. Pseudomonas (27.76%), Brevundimonas (14.99%), Delftia (11.29%), and Stenotrophomonas (8.27%) were the genera with higher relative abundances among the nymphal ticks. The dominant genera in the engorged nymph stage were Arsenophonus (64.94%) and Rickettsia (29.07%). In the second generation of adult ticks, the genus with the highest relative abundance was Rickettsia (23.38%), Alcaligenes (17.37%), Brevundimonas (9.63%), Achromobacter (9.01%), and Pseudomonas (8.07%) (Figure 6).
Microbial community composition at the species level
Dermacentor nuttalli was associated with 23 bacterial species, with Alcaligenes faecalis, Brevundimonas diminuta, Delftia tsuruhatensis, Achromobacter xylosoxidans subsp. xylosoxidans, Staphylococcus xylosus, Brucella melitensis, Sphingobacterium mizutaii, Staphylococcus sciuri subsp. sciuri accounting for more than 1% of the total. Total abundances were higher in Alcaligenes faecalis and Brevundimonas diminuta, where the abundances in the larval tick and nymphal tick stages were significantly higher in their engorged stages and were also significantly higher in the second generation of adult ticks. The abundances of Delftia tsuruhatensis and Achromobacter xylosoxidans subsp. xylosoxidans were higher in the nymph and second-generation adult tick stages. Staphylococcus xylosus and Brucella melitensis were more abundant in female adult and larval tick stages than in any other stages (Table 6).
Discussion
China reported 124 tick species, 103 tick-borne agents, and 29 tick-borne pathogens infecting humans from 1950 to 2018 (3). In the 33 years since 1982, Mainland China has identified 33 emerging tick-associated agents, of which 20 have been identified to cause human diseases (10). Different tick species prefer different biotopes or environments, which influences their geographical distribution and, as a result, the risk area for human tick-borne infections. D. nuttalli is ubiquitous in northern China (11, 50), one of the dominant tick species in Inner Mongolia and is one of the most dangerous tick species in Inner Mongolia in terms of public health (11). It spreads multiple pathogens and causes multiple infectious diseases (10, 34). Current research on the carriage and transmission of pathogens by D. nuttalli focuses primarily on the detection of one specific pathogen carried by D. nuttalli adult ticks (15–33) and different developmental stages of D. nuttalli (34–40); however, a few studies have employed next-generation sequencing on D. nuttalli adult ticks (41–44). The 16S rDNA amplicon sequencing has advantages, such as the identification of low-abundance and non-culturable bacteria (44). The microbial community structure and diversity of different growth stages of D. nuttalli have not yet been comprehensively studied. As the Hulunbuir League is one of the most important pastoral regions in Inner Mongolia, hard ticks thrive there (41). In this study, we investigated the microbial community structures of D. nuttalli at seven growth stages in the Hulunbuir League region of China using high-throughput sequencing.
In the alpha diversity of community ecology, a higher diversity in the sample microbial community was indicated by higher Shannon Index and lower Simpson Index values. Higher ACE values indicate a greater total number of species (51). The sequencing results showed that the egg stage had the greatest diversity and the highest number of species, while the engorged nymph stage had the least diversity and number of species. The sequencing depth index (Good's coverage) of all 7 growth stage samples exceeded 99.9%, indicating that the sequencing depth is sufficient to demonstrate the microbial diversity of the samples and can reflect the actual situation of microorganisms carried by the samples. The species accumulation curves also reflected the abundance of the D. nuttalli microbial community as the sample size required to detect a large number of species in the community. OTU cluster analysis showed that some microbial populations were identical among the seven growth stages of D. nuttalli. On the basis of the above estimators, analysis of the sequencing data from this study showed that 387 genera of 22 phyla were associated with D. nuttalli, of which 57 genera of 9 phyla were found in all 7 growth stages. Proteobacteria are the dominant phylum of D. nuttalli and are consistent with the dominant phylum of Haemaphysalis flava (13), Amblyomma maculatum (52), Amblyomma tuberculatum (53), Haemaphysalis longicornis (54), and D. nuttalli in Guertu County, Wusu City (44), which may be related to the widespread distribution of Proteobacteria in nature and suggests that Proteobacteria are viable and abundant in different tick species. At the level of bacterial genera, the dominant genera of D. nuttalli in this study were Arsenophonus and Rickettsia. It has also been reported that Rickettsia was detected in Haemaphysalis flava (Xinyang city, Henan Province, China), Amblyomma americanum (Edwards and Sutton Counties, USA), Rhipicephalus turanicus (Kibbutz Hulda, Israel) and Rhipicephalus sanguineus (Caesarea) (13). This is not consistent with the dominant genera (Pseudomonas and Coxiella) reported for D. nuttalli in Guertu County (44) and may suggest regional differences.
In this study, Arsenophonus has the highest total abundance in D. nuttalli and the lowest abundance in the first-generation female adult tick stage (0.15%); its abundance increased from 4.46% for eggs and 38.05% for larval ticks to 54.90% for engorged larval ticks but then dropped to 1.36% in the nymph stage and increased again to a peak of 64.94% in engorged nymphal ticks before decreasing to 2.01% in the second generation of adult ticks. According to the literature, blood-feeding behavior alters the composition and abundance of the microbiome in insects (12). Studies have shown that the community diversity of Anopheles gambiae significantly decreased (55) and the midgut microbiota of Aedes aegypti increased after blood meals (56); blood feeding reduces the microbial richness of the sand fly midgut and stimulates the proliferation (57, 58) and diversification of colonizing female midge intestinal bacterial populations (59); and blood sucking increases the relative abundance of Bartonella while decreasing Wolbachia and most other bacterial genera after bloodsucking Melophagus ovinus (51). According to the literature, the process of imbibing blood appears to affect the tick microbiome (60), and blood meal feeding usually reduces the diversity of internal tick microbiota (44). The results of this study showed that the act of blood sucking significantly increased the relative abundance of Arsenophonus. Arsenophonus is an intracellular symbiotic bacterium that infects a variety of arthropods and plays an important role in killing male host insects while also providing vitamins and other nutrients to host insects (61). The role of Arsenophonus in ticks is not well understood (62). Further studies are needed to determine the effect of bloodsucking behavior on Arsenophonus in D. nuttalli and the role of Arsenophonus in D. nuttalli.
In this study, Rickettsia was the second most abundant bacterial genus of D. nuttalli in terms of total abundance. Rickettsia is an obligate, intracellular, parasitic bacterium that is spread mainly by arthropods (61). Rickettsia is also considered a symbiont in the broad sense and has been found in high concentrations in a number of ticks, including Rhipicephalus microplus (63), Ixodes scapularis (64), Rhipicephalus turanicus, and Rhipicephalus sanguineus. In previous studies, spotted fever group Rickettsiae were detected in up to 67.4% of D. nuttalli and potential novel Rickettsia species were found (65). Furthermore, Rickettsia raoultii may be the predominant Rickettsia in D. nuttalli. Additionally, Rickettsia raoultii and Rickettsia sibirica subsp. Sibirica causes Siberian tick typhus, which has been found in nymphal ticks as well as in both unfed and engorged adults of D. nuttalli (23, 27, 39, 66). The pathogen of Q fever was also detected in unfed D. nuttalli ticks and in D. nuttalli ticks collected from livestock (26). Rickettsia sibirica, Rickettsia slovaca (23), Rickettsia sp. 10CYF, Candidatus Rickettsia tibetani (27), Rickettsia tarasevichiae (41), Rickettsia aeschlimannii (18), Rickettsia heilongjiangensis (10), and others were also detected in D. nuttalli. The above study showed that D. nuttalli carried a high abundance and a variety of Rickettsia. The results of this study showed an increased abundance of Rickettsia after a blood meal, which is consistent with the abundance of Rickettsia in unfed ticks (D. nuttalli) in Guertu County being <5% (44). The difference in Rickettsia abundance in D. nuttalli in different reports may also be related to the geographical location of the samples. The ability of another arthropod, M. ovinus carrying Rickettsia, also reflects geographic regional differences (61). Many Rickettsia are tick-borne pathogens that cause tick-borne rickettsial diseases, which pose a serious threat to human and animal health worldwide. However, in ticks, these Rickettsia are not pathogenic and exist only as maternally inherited symbionts with an intimate (but not necessarily beneficial) relationship with the host (67), which is consistent with the present study, in which Rickettsia were found in all seven different growth stages. It has also been reported that Rickettsia switching between pathogenic and non-pathogenic forms and between hosts is a common feature of Rickettsia evolution, which may lead to the emergence of new infectious diseases (68). In summary, arthropod Rickettsia may require more of our attention.
Additionally, in the present study, there were many other bacterial genera that were presented in various stages, including eggs, indicating that these microorganisms may be transovarially transmitted and may be in a symbiotic relationship with D. nuttalli, despite the fact that these bacteria do not appear to be pathogenic in ticks, as reported in the literature (69). Similarly, the pathogenicity of these microorganisms to humans and animals cannot be overlooked. After engorgement in larval ticks and nymphal ticks, the abundance of Pseudomonas, Alcaligenes, Brevundimonas, and Stenotrophomonas decreased to varying degrees. This coincides with the highest abundance of Pseudomonas in the unfed ticks (D. nuttalli) in Guertu County (44). It has been previously reported that sheep sera appears to lyse Borrelia garinii and Borrelia valaisiana (70), but the relationship of blood to the decreased abundance of these microorganisms in D. nuttalli is unknown. In this study, a large number of microorganisms were annotated in different growth stages of D. nuttalli, but the dominant genera in each growth stage were different, e.g., the dominant genus in eggs was Paenalcaligenes, but only a low abundance of Paenalcaligenes was found in the nymph stage, whereas the abundance of Paenalcaligenes in houseflies increased with increased larval development (71). All of this evidence suggests that the microbial community composition and diversity in ticks are dynamic and are influenced by a variety of factors, including tick developmental stage (72), geographical location (73), blood sucking (74), engorgement status (75, 76), sex (77), host, and tick species (12, 78). In conclusion, the microbial community structure of D. nuttalli exhibits differential and stage-specific characteristics.
Finally, we would like to discuss a few species with higher abundance in the 23 specific bacterial species that were only annotated to D. nuttalli. Alcaligenes faecalis is an obligate aerobic, gram-negative rod that causes skin and soft tissue infections, meningitis, endocarditis, chronic otitis, bacteremia, pyelonephritis, endophthalmitis, peritonitis, abscesses (79), urinary tract infections, and pneumonia (80). Most infections caused by this organism are nosocomial, occur in immunocompromised hosts, and have a high level of resistance to commonly used antibiotics (79). Brevundimonas diminuta is a gram-negative aerobic bacillus. It causes bacteremia, urinary tract infection, pleuritis, empyema, peritonitis, keratitis, bloodstream infection, nephrotic syndrome, post-traumatic abscess, Leg ulcer (81), and pyogenic liver abscesses and is typically resistant to a wide range of antimicrobials (82). Delftia tsuruhatensis is a gram-negative bacillus discovered in Japan in 2003. These bacteria cause bacteremia (83), catheter-related infection (84), respiratory infection in a premature infant (85), and are resistant to a variety of antibiotics (83, 84). Achromobacter xylosoxidans, formerly known as Alcaligenes denitrificans subsp. xylosoxidans, is a gram-negative bacillus that is intrinsically resistant to most antibiotics and was first found in the purulent ear drainage of patients with chronic otitis media. It was later identified as an etiologic agent of meningitis, pneumonia, surgical wound infections, bacteremia, septicemia, urinary tract infections, peritonitis, pharyngitis, etiologic agent (86), and endocarditis (87). Patients with cystic fibrosis and patients with non-cystic fibrosis (patients with bronchiectasis and patients with cancer) are at risk of infection with Achromobacter xylosoxidans (88), and there is evidence of patient-to-patient transmission (87). Brucella melitensis is a major pathogen of brucellosis in goats and sheep and is the most common pathogen in human brucellosis (89). In animals, Brucella infection has a negative impact on fetal development and reproductive organs, leading to reproductive failure, abortions, and infertility (90). The Food and Agriculture Organization of the United Nations, the World Health Organization, and the Office International des Epizootics consider brucellosis to be one of the world's most serious neglected zoonotic diseases (90). Ticks are reservoir vectors for brucellosis and genes specific to the Brucella genus have been found in developmental stages and anatomical regions of ticks. B. melitensis was isolated from eggs and engorged adults of D. nuttalli. Dermacentor nuttalli is a potent, long-term carrier of Brucella spp. and shows transovarial transmission potential (34), indicating the potential risk of transmission of brucellosis between animals and humans via tick bites. Staphylococcus xylosus is a commensal gram-positive bacterium found on human and animal skin and is a clinically relevant pathogen in veterinary medicine (91). Sphingobacterium mizutaii is a gram-negative organism that is very similar to Sphingobacterium mizutaii, which has been isolated from patients with cellulitis (92). Staphylococcus sciuri is thought to be a commensal animal-associated species, but it has been isolated from bovine mastitis, goats with ovine rinderpest, canine dermatitis (93), and piglets with fatal exudative epidermitis. These bacteria cause lesions of the lungs and endocarditis in piglets; are fatal to mice and newly born piglets (94); may be related to human endocarditis, endophthalmitis, skin wound infection, peritonitis, pelvic inflammatory disease, septic shock, urinary tract infections, and wound infections; and are multidrug-resistant bacteria (95). These microorganisms that we identified in D. nuttalli deserve further investigation, and it is necessary to conduct surveillance of some highly abundant bacteria in D. nuttalli that require close monitoring because of their potential to cause disease in humans or animals.
Conclusions
The egg stage of D. nuttalli in Inner Mongolia, China, had the most diversity and species, with 387 genera and 23 species of 22 phyla, of which 9 phyla, 57 genera, and 23 species were found in all 7 growth stages of D. nuttalli. The dominant phylum was Proteobacteria, and the dominant bacterial genera were Arsenophonus and Rickettsia. The dominant genera differ at different growth stages. In this study, the microbial community composition of different growth stages of D. nuttalli was comprehensively analyzed for the first time.
Data availability statement
The raw tags have been deposited in Sequence Read Archive (SRA) from the NCBI under BioProject accession number PRJNA848250. The individual run files received the accession numbers SRR19668431 ~ SRR19668451.
Ethics statement
The Biomedical Research Ethics Committee of Inner Mongolia Agricultural University specifically approved this study [No. 2020(081)]. Written informed consent was obtained from the owners for the participation of their animals in this study.
Author contributions
LZ, Y-MM, and Y-HL conceived and designed the study and critically revised the manuscript. BY, W-XH, LZ, and Y-MM performed the sample collection. LZ, Y-MM, W-HZ, H-LC, Z-SZ, Y-JZ, J-LW, Y-LD, and Y-HL conducted the laboratory experiments. Y-HL, LZ, Y-MM, YX, and L-FY conducted sequencing and participated in sequence analysis. All authors read and approved the final manuscript.
Funding
This study was funded by the Inner Mongolia Agricultural University High-level Talents Research Initiation Fund Project (NDYB2019-3 and NDYB2018-5), National Natural Science Foundation of China (32260887 and 31860698), National Natural Science Foundation of Inner Mongolia (2022MS03023), and supported by State Key Laboratory of Veterinary Biotechnology Foundation (SKLVBF202204).
Conflict of interest
Author W-XH was employed by Inner Mongolia Saikexing Reproductive Biotechnology (Group) Co., Ltd., Hohhot, China. Authors YX and L-FY were employed by Shanghai Origingene Bio-pharm Technology Co. Ltd., Shanghai, China.
The remaining authors declare that the research was conducted in the absence of any commercial or financial relationships that could be construed as a potential conflict of interest.
Publisher's note
All claims expressed in this article are solely those of the authors and do not necessarily represent those of their affiliated organizations, or those of the publisher, the editors and the reviewers. Any product that may be evaluated in this article, or claim that may be made by its manufacturer, is not guaranteed or endorsed by the publisher.
References
1. Lesley B-S, Houssam A. Endogenous tick viruses and modulation of tick-borne pathogen growth. Front Cell Infect Microbiol. (2013) 25:1–10. doi: 10.3389/fcimb.2013.00025
2. Filipe D-T. Species concepts: what about ticks? Trends Parasitol. (2018) 34:1017–26. doi: 10.1016/j.pt.2018.09.009
3. Zhao GP, Wang YX, Fan ZW, Ji Y, Liu MJ, Zhang WH, et al. Mapping ticks and tick-borne pathogens in china. Nat Commun. (2021) 12:1075. doi: 10.1038/s41467-021-21375-1
4. Rochlin I, Toledo A. Emerging tick-borne pathogens of public health importance: a mini-review. J Med Microbiol. (2020) 69:781–91. doi: 10.1099/jmm.0.001206
5. Jongejan F, Uilenberg G. The global importance of ticks. Parasitology. (2004) 129 (Suppl):S3–14. doi: 10.1017/S0031182004005967
6. Madison-Antenucci S, Kramer LD, Gebhardt LL, Kauffman E. Emerging tick-borne diseases. Clin Microbiol Rev. (2020) 33:e00083–e00018. doi: 10.1128/CMR.00083-18
7. Parola P. Tick-borne rickettsial diseases: emerging risks in Europe. Comp Immunol Microbiol Infect Dis. (2004) 27:297–304. doi: 10.1016/j.cimid.2004.03.006
8. Parola P, Raoult D. Ticks and tickborne bacterial diseases in humans: an emerging infectious threat. Clin Infect Dis. (2001) 32:897–928. doi: 10.1086/319347
9. de la Fuente J, Estrada-Pena A, Venzal JM, Kocan KM, Sonenshine DE. Overview: ticks as vectors of pathogens that cause disease in humans and animals. Front Biosci. (2008) 13:6938–46. doi: 10.2741/3200
10. Fang LQ, Liu K, Li XL, Liang S, Yang Y, Yao HW, et al. Emerging tick-borne infections in mainland China: an increasing public health threat. Lancet Infect Dis. (2015) 15:1467–79. doi: 10.1016/S1473-3099(15)00177-2
11. Gui Z, Wu L, Cai H, Mu L, Yu JF, Fu SY. Genetic diversity analysis of Dermacentor nuttalli within Inner Mongolia, China. Parasit Vectors. (2021) 14:131. doi: 10.1186/s13071-021-04625-5
12. Yadav KK, Datta S, Naglot A, Bora A, Hmuaka V, Bhagyawant S, et al. Diversity of cultivable midgut microbiota at different stages of the asian tiger mosquito, aedes albopictus from tezpur, India. PLoS ONE. (2016) 11:e0167409. doi: 10.1371/journal.pone.0167409
13. Duan DY, Cheng TY. Determination of the microbial community features of Haemaphysalis flava in different developmental stages by high-throughput sequencing. J Basic Microbiol. (2016) 57:302–8. doi: 10.1002/jobm.201600557
14. Ahn J, Yang LY, Paster BJ, Ganly I, Morris L, Pei ZH, et al. Oral microbiome profiles: 16S rRNA pyrosequencing and microarray assay comparison. PLoS ONE. (2011) 6:e22788. doi: 10.1371/journal.pone.0022788
15. Voorhees MA, Padilla SL, Jamsransuren D, Koehler JW, Delp KL, Adiyadorj D, et al. Crimean-Congo hemorrhagic fever virus, Mongolia, 2013-2014. Emerg Infect Dis. (2018) 24:2202–9. doi: 10.3201/eid2412.180175
16. Wang Q, Pan YS, Jiang BG, Ye RZ, Chang QC, Shao HZ, et al. Prevalence of multiple tick-borne pathogens in various tick vectors in Northeastern China. Vector Borne Zoonotic Dis. (2021) 21:162–71. doi: 10.1089/vbz.2020.2712
17. Enkhtaivan B, Narantsatsral S, Davaasuren B, Otgonsuren D, Amgalanbaatar T, Uuganbayar E, et al. Molecular detection of Anaplasma ovis in small ruminants and ixodid ticks from Mongolia. Parasitol Int. (2019) 69:47–53. doi: 10.1016/j.parint.2018.11.004
18. Gaowa W, Yin XH, Guo SC, Ding CL, Cao MZ, et al. Spotted fever group rickettsiae in inner Mongolia, China, 2015-2016. Emerg Infect Dis. (2018) 24:2105–7. doi: 10.3201/eid2411.162094
19. von Fricken ME, Qurollo BA, Boldbaatar B, Wang YW, Jiang RR, Lkhagvatseren S, et al. Genetic diversity of anaplasma and ehrlichia bacteria found in dermacentor and ixodes ticks in Mongolia. Ticks Tick Borne Dis. (2020) 11:101316. doi: 10.1016/j.ttbdis.2019.101316
20. von Fricken ME, Voorhees MA, Koehler JW, Asbun C, Lam B, Qurollo B, et al. Molecular characteristics of rickettsia in ticks collected along the Southern Border of Mongolia. Pathogens. (2020) 9:943. doi: 10.3390/pathogens9110943
21. Gao Y, Lv XL, Han SZ, Wang W, Liu Q, Song MX, et al. First detection of Borrelia miyamotoi infections in ticks and humans from the northeast of Inner Mongolia, China. Acta Trop. (2021) 217:105857. doi: 10.1016/j.actatropica.2021.105857
22. Li YC, Wen XX, Li M, Moumouni PFA, Galon EM, Guo QY, et al. Molecular detection of tick-borne pathogens harbored by ticks collected from livestock in the Xinjiang Uygur Autonomous Region, China. Ticks Tick Borne Dis. (2020) 11:101478. doi: 10.1016/j.ttbdis.2020.101478
23. Song SN, Chen CF, Yang MH, Zhao SS, Wang BJ, Hornok S, et al. Diversity of Rickettsia species in border regions of northwestern China. Parasit Vectors. (2018) 11:634. doi: 10.1186/s13071-018-3233-6
24. Liu H, Liang XT, Wang HJ, Sun XT, Bai X, Hu B, et al. Molecular evidence of the spotted fever group Rickettsiae in ticks from Yunnan Province, Southwest China. Exp Appl Acarol. (2020) 80:339–48. doi: 10.1007/s10493-020-00467-5
25. Shao JW, Zhang XL, Li WJ, Huang HL, Yan J. Distribution and molecular characterization of rickettsiae in ticks in Harbin area of Northeastern China. PLoS Negl Trop Dis. (2020) 14:e0008342. doi: 10.1371/journal.pntd.0008342
26. Ni J, Lin HL, Xu XF, Ren QY, Aizezi M, Luo J, et al. Coxiella burnetii is widespread in ticks (Ixodidae) in the Xinjiang areas of China. BMC Vet Res. (2020) 16:317. doi: 10.1186/s12917-020-02538-6
27. Han R, Yang JF, Niu QL, Liu ZJ, Chen Z, Kan W, et al. Molecular prevalence of spotted fever group rickettsiae in ticks from Qinghai Province, northwestern China. Infect Genet Evol. (2018) 57:1–7. doi: 10.1016/j.meegid.2017.10.025
28. Wang YC, Li S, Wang ZD, Zhang L, Cai YN, Liu Q. Prevalence and Identification of Borrelia burgdorferi Sensu Lato Genospecies in Ticks from Northeastern China. Vector Borne Zoonotic Dis. (2019) 19:309–15. doi: 10.1089/vbz.2018.2316
29. Zhang L, Li S, Huang SJ, Wang ZD, Wei F, Feng XM, et al. Isolation and genomic characterization of lymphocytic choriomeningitis virus in ticks from northeastern China. Transbound Emerg Dis. (2018) 65:1733–9. doi: 10.1111/tbed.12946
30. Song R, Wang Q, Guo F, Liu X, Song S, Chen C, et al. Detection of Babesia spp. Theileria spp. and Anaplasma ovis in Border Regions, northwestern China. Transbound Emerg Dis. (2018) 65:1537–44. doi: 10.1111/tbed.12894
31. Liu XF, Zhang X, Wang ZD, Dong ZH, Xie SS, Jiang MM, et al. A tentative tamdy orthonairovirus related to febrile illness in Northwestern China. Clin Infect Dis. (2020) 70:2155–60. doi: 10.1093/cid/ciz602
32. Narankhajid M, Yeruult C, Gurbadam A, Battsetseg J, Aberle SW, Bayartogtokh B, et al. Some aspects on tick species in Mongolia and their potential role in the transmission of equine piroplasms, Anaplasma phagocytophilum and Borrelia burgdorferi L. Parasitol Res. (2018) 117:3557–66. doi: 10.1007/s00436-018-6053-x
33. Shen S, Duan XM, Wang B, Zhu LY, Zhang YF, Zhang JY, et al. A novel tick-borne phlebovirus, closely related to severe fever with thrombocytopenia syndrome virus and Heartland virus, is a potential pathogen. Emerg Microbes Infect. (2018) 7:95. doi: 10.1038/s41426-018-0093-2
34. Huang TP, Zhang JB, Sun CY, Liu ZC, He HY, Wu J, et al. A novel arthropod host of brucellosis in the arid steppe ecosystem. Front Vet Sci. (2020) 7:566253. doi: 10.3389/fvets.2020.566253
35. Kholodilov I, Belova O, Burenkova L, Korotkov Y, Romanova L, Morozova L, et al. Ixodid ticks and tick-borne encephalitis virus prevalence in the South Asian part of Russia (Republic of Tuva). Ticks Tick Borne Dis. (2019) 10:959–69. doi: 10.1016/j.ttbdis.2019.04.019
36. Kholodilov IS, Belova OA, Morozkin ES, Litov AG, Ivannikova AY, Makenov MT, et al. Geographical and tick-dependent distribution of flavi-like Alongshan and Yanggou Tick viruses in Russia. Viruses. (2021) 13:458. doi: 10.3390/v13030458
37. Fischer T, Myalkhaa M, Krücken J, Battsetseg G, Batsukh Z, Baumann MPO, et al. Molecular detection of tick-borne pathogens in bovine blood and ticks from Khentii, Mongolia. Transbound Emerg Dis. (2020) 67 Suppl 2:111–8. doi: 10.1111/tbed.13315
38. Guo WP, Wang YH, Lu QZ, Xu G, Luo YH, Ni XB, et al. Molecular detection of spotted fever group rickettsiae in hard ticks, northern China. Transbound Emerg Dis. (2019) 66:1587–96. doi: 10.1111/tbed.13184
39. Zhao S, Yang MH, Jiang MM, Yan B, Zhao SS, Yuan WM, et al. Rickettsia raoultii and Rickettsia sibirica in ticks from the long-tailed ground squirrel near the China-Kazakhstan border. Exp Appl Acarol. (2019) 77:425–33. doi: 10.1007/s10493-019-00349-5
40. Moore TC, Pulscher LA, Caddell L, von Fricken ME, Anderson BD, Gonchigoo B, et al. Evidence for transovarial transmission of tick-borne rickettsiae circulating in Northern Mongolia. PLoS Negl Trop Dis. (2018) 12:e0006696. doi: 10.1371/journal.pntd.0006696
41. Jiao J, Lu ZY, Yu YH, Ou YX, Fu MJ, Zhao YE, et al. Identification of tick-borne pathogens by metagenomic next-generation sequencing in Dermacentor nuttalli and Ixodes persulcatus in Inner Mongolia, China. Parasit Vectors. (2021) 14:287. doi: 10.1186/s13071-021-04740-3
42. Dedkov VG, Dolgova AS, Safonova MV, Samoilov AE, Belova OA, Kholodilov IS, et al. Isolation and characterization of Wad Medani virus obtained in the tuva Republic of Russia. Ticks Tick Borne Dis. (2021) 12:101612. doi: 10.1016/j.ttbdis.2020.101612
43. Meng F, Ding MM, Tan ZZ, Zhao ZH, Xu L, Wu JM, et al. Virome analysis of tick-borne viruses in Heilongjiang Province, China. Ticks Tick Borne Dis. (2019) 10:412–20. doi: 10.1016/j.ttbdis.2018.12.002
44. Song K, Ji YX, Sun SR, Yue XH, Wang C, Luo T, et al. Bacterial microbiota in unfed ticks (Dermacentor nuttalli) from Xinjiang detected through 16S rDNA amplicon sequencing and culturomics. Zoonoses. (2021) 12:1–18. doi: 10.15212/ZOONOSES-2021-0007
45. Edgar RC. UPARSE: highly accurate OTU sequences from microbial amplicon reads. Nat Methods. (2013) 10:996–8. doi: 10.1038/nmeth.2604
46. Wang Q, Garrity GM, Tiedje JM, Cole JR. Naive Bayesian classifier for rapid assignment of rRNA sequences into the new bacterial taxonomy. Appl Environ Microbiol. (2007) 73:5261–7. doi: 10.1128/AEM.00062-07
47. Maughan H, Wang PW, Diaz Caballero J, Fung P, Gong YC, Donaldson SL, et al. Analysis of the cystic fibrosis lung microbiota via serial Illumina sequencing of bacterial 16S rRNA hypervariable regions. PLoS ONE. (2012) 7:e45791. doi: 10.1371/journal.pone.0045791
48. Fouts DE, Szpakowski S, Purushe J, Torralba M, Waterman RC, MacNeil MD, et al. Next generation sequencing to define prokaryotic and fungal diversity in the bovine rumen. PLoS ONE. (2012) 7:e48289. doi: 10.1371/journal.pone.0048289
49. Oberauner L, Zachow C, Lackner S, Högenauer C, Smolle KH, Berg G. The ignored diversity: complex bacterial communities in intensive care units revealed by 16S pyrosequencing. Sci Rep. (2013) 3:1413. doi: 10.1038/srep01413
50. Chen Z, Yang XJ, Bu FJ, Yang XH, Yang XL, Liu JZ. Ticks (acari: ixodoidea: argasidae, ixodidae) of China. Exp Appl Acarol. (2010) 51:393–404. doi: 10.1007/s10493-010-9335-2
51. Duan DY, Zhou HM, Cheng TY. Comparative analysis of microbial community in the whole body and midgut from fully engorged and unfed female adult Melophagus ovinus. Med Vet Entomol. (2020) 34:215–24. doi: 10.1111/mve.12424
52. Budachetri K, Browning RE, Adamson SW, Dowd SE, Chao CC, Ching WM, et al. An insight into the microbiome of the Amblyomma maculatum (Acari: Ixodidae). J Med Entomol. (2014) 51:119–29. doi: 10.1603/ME12223
53. Budachetri K, Gaillard D, Williams J, Mukherjee N, Karim S. A snapshot of the microbiome of Amblyomma tuberculatum ticks infesting the gopher tortoise, an endangered species. Ticks Tick Borne Dis. (2016) 7:1225–9. doi: 10.1016/j.ttbdis.2016.07.010
54. Zhang RL, Huang ZD, Yu GF, Zhang Z. Characterization of microbiota diversity of field-collected Haemaphysalis longicornis (Acari: Ixodidae) with regard to sex and blood meals. J Basic Microbiol. (2019) 59:215–23. doi: 10.1002/jobm.201800372
55. Wang Y, Gilbreath TM III, Kukutla P, Yan GY, Xu JN. Dynamic gut microbiome across life history of the malaria mosquito Anopheles gambiae in Kenya. PLoS ONE. (2011) 6:e24767. doi: 10.1371/journal.pone.0024767
56. Oliveira JH, Gonçalves RL, Lara FA, Dias FA, Gandara AC, Menna-Barreto RF, et al. Blood meal-derived heme decreases ROS levels in the midgut of Aedes aegypti and allows proliferation of intestinal microbiota. PLoS Pathog. (2011) 7:e1001320. doi: 10.1371/journal.ppat.1001320
57. Kelly PH, Bahr SM, Serafim TD, Ajami NJ, Petrosino JF, Meneses C, et al. The gut microbiome of the vector Lutzomyia longipalpis is essential for survival of Leishmania infantum. MBio. (2017) 8:e01121–e01116. doi: 10.1128/mBio.01121-16
58. Telleria EL, Martins-da-Silva A, Tempone AJ, Traub-Csekö YM. Leishmania, microbiota and sand fly immunity. Parasitology. (2018) 145:1336–53. doi: 10.1017/S0031182018001014
59. Nayduch D, Erram D, Lee MB, Zurek L, Saski CA. Impact of the blood meal on humoral immunity and microbiota in the gut of female Culicoides sonorensis. Vet Ital. (2015) 51:385–92. doi: 10.12834/VetIt.495.2397.2
60. Varela-Stokes AS, Park SH, Kim SA, Ricke SC. Microbial communities in North American ixodid ticks of veterinary and medical importance. Front Vet Sci. (2017) 4:179. doi: 10.3389/fvets.2017.00179
61. Duan DY, Liu GH, Cheng TY, Wang YQ. Microbial population analysis of the midgut of Melophagus ovinus via high-throughput sequencing. Parasit Vectors. (2017) 10:382. doi: 10.1186/s13071-017-2323-1
62. Ahantarig A, Trinachartvanit W, Baimai V, Grubhoffer L. Hard ticks and their bacterial endosymbionts (or would be pathogens). Folia Microbiol. (2013) 58:419–28. doi: 10.1007/s12223-013-0222-1
63. Xiang LL, Pozniak B, Cheng TY. Bacteriological analysis of saliva from partially or fully engorged female adult Rhipicephalus microplus by next-generation sequencing. Antonie Van Leeuwenhoek. (2017) 110:105–13. doi: 10.1007/s10482-016-0780-8
64. Benson MJ, Gawronski JD, Eveleigh DE, Benson DR. Intracellular symbionts and other bacteria associated with deer ticks (Ixodes scapularis) from Nantucket and Wellfleet, Cape Cod, Massachusetts. Appl Environ Microbiol. (2004) 70:616–20. doi: 10.1128/AEM.70.1.616-620.2004
65. Liu HH, Li QH, Zhang XZ, Li ZY, Wang ZD, Song MX, et al. Characterization of rickettsiae in ticks in northeastern China. Parasit Vectors. (2016) 9:498. doi: 10.1186/s13071-016-1764-2
66. Speck S, Derschum H, Damdindorj T, Dashdavaa O, Jiang J, Kaysser P, et al. Rickettsia raoultii, the predominant Rickettsia found in Mongolian Dermacentor nuttalli. Ticks Tick Borne Dis. (2012) 3:227–31. doi: 10.1016/j.ttbdis.2012.04.001
67. Perlman SJ, Hunter MS, Zchori-Fein E. The emerging diversity of Rickettsia. Proc Biol Sci. (2006) 273:2097–106. doi: 10.1098/rspb.2006.3541
68. Bonnet SI, Binetruy F, Hernández-Jarguín AM, Duron O. The tick microbiome: why non-pathogenic microorganisms matter in tick biology and pathogen transmission. Front Cell Infect Microbiol. (2017) 7:236. doi: 10.3389/fcimb.2017.00236
69. Noda H, Munderloh UG, Kurtti TJ. Endosymbionts of ticks and their relationship to Wolbachia spp. and tick-borne pathogens of humans and animals. Appl Environ Microbiol. (1997) 63:3926–32. doi: 10.1128/aem.63.10.3926-3932.1997
70. Chu CY, Jiang BG, Qiu EC, Zhang F, Zuo SQ, Yang H, et al. Borrelia burgdorferi sensu lato in sheep keds (Melophagus ovinus), Tibet, China. Vet Microbiol. (2011) 149:526–9. doi: 10.1016/j.vetmic.2010.11.031
71. Zhang Q, Wang SM, Zhang XY, Zhang KX, Liu WJ, Zhang RL, et al. Enterobacter hormaechei in the intestines of housefly larvae promotes host growth by inhibiting harmful intestinal bacteria. Parasit Vectors. (2021) 14:598. doi: 10.1186/s13071-021-05053-1
72. Clay K, Klyachko O, Grindle N, Civitello D, Oleske D, Fuqua C. Microbial communities and interactions in the lone star tick, Amblyomma americanum. Mol Ecol. (2008) 17:4371–81. doi: 10.1111/j.1365-294X.2008.03914.x
73. Carpi G, Cagnacci F, Wittekindt NE, Zhao FQ, Qi J, Tomsho LP, et al. Metagenomic profile of the bacterial communities associated with Ixodes ricinus ticks. PLoS ONE. (2011) 6:e25604. doi: 10.1371/journal.pone.0025604
74. Swei A, Kwan JY. Tick microbiome and pathogen acquisition altered by host blood meal. ISME J. (2017) 11:813–6. doi: 10.1038/ismej.2016.152
75. Heise SR, Elshahed MS, Little SE. Bacterial diversity in Amblyomma americanum (Acari: Ixodidae) with a focus on members of the genus Rickettsia. J Med Entomol. (2010) 47:258–68. doi: 10.1093/jmedent/47.2.258
76. Zhang XC, Yang ZN, Lu B, Ma XF, Zhang CX, Xu HJ. The composition and transmission of microbiome in hard tick, Ixodes persulcatus, during blood meal. Ticks Tick Borne Dis. (2014) 5:864–70. doi: 10.1016/j.ttbdis.2014.07.009
77. Williams-Newkirk AJ, Rowe LA, Mixson-Hayden TR, Dasch GA. Characterization of the bacterial communities of life stages of free living lone star ticks (Amblyomma americanum). PLoS ONE. (2014) 9:e102130. doi: 10.1371/journal.pone.0102130
78. Xu XL, Cheng TY, Yang H, Yan F. Identification of intestinal bacterial flora in Rhipicephalus microplus ticks by conventional methods and PCR-DGGE analysis. Exp Appl Acarol. (2015) 66:257–68. doi: 10.1007/s10493-015-9896-1
79. Tena D, Fernández C, Lago MR. Alcaligenes faecalis: an unusual cause of skin and soft tissue infection. Jpn J Infect Dis. (2015) 68:128–30. doi: 10.7883/yoken.JJID.2014.164
80. Huang C. Extensively drug-resistant Alcaligenes faecalis infection. BMC Infect Dis. (2020) 20:833. doi: 10.1186/s12879-020-05557-8
81. Ryan MP, Pembroke JT. Brevundimonas spp: emerging global opportunistic pathogens. Virulence. (2018) 9:480–93. doi: 10.1080/21505594.2017.1419116
82. Burch J, Tatineni S, Enofe I, Laird-Fick H. Brevundimonas diminuta coinfection as source of pyogenic liver abscess. BMJ Case Rep. (2021) 14:e236235. doi: 10.1136/bcr-2020-236235
83. Yin ZQ, Liu XB, Qian CQ, Sun L, Pang SQ, Liu JN, et al. Pan-genome analysis of Delftia tsuruhatensis reveals important traits concerning the genetic diversity, pathogenicity, and biotechnological properties of the species. Microbiol Spectr. (2022) 10:e0207221. doi: 10.1128/spectrum.02072-21
84. Cheng C, Zhou WX, Dong X, Zhang PY, Zhou KX, Zhou DY, et al. Genomic analysis of Delftia tsuruhatensis strain TR1180 isolated from a patient from china with in4-like integron-associated antimicrobial resistance. Front Cell Infect Microbiol. (2021) 11:663933. doi: 10.3389/fcimb.2021.663933
85. Ranc A, Dubourg G, Fournier PE, Raoult D, Fenollar F. Delftia tsuruhatensis, an emergent opportunistic healthcare-associated pathogen. Emerg Infect Dis. (2018) 24:594–6. doi: 10.3201/eid2403.160939
86. Spear JB, Fuhrer J, Kirby BD. Achromobacter xylosoxidans (Alcaligenes xylosoxidans subsp. xylosoxidans) bacteremia associated with a well-water source: case report and review of the literature. J Clin Microbiol. (1988) 26:598–9. doi: 10.1128/jcm.26.3.598-599.1988
87. Pickrum AM, DeLeon O, Dirck A, Tessmer MH, Riegert MO, Biller JA, et al. Achromobacter xylosoxidans cellular pathology is correlated with activation of a type III secretion system. Infect Immun. (2020) 88:e00136–e00120. doi: 10.1128/IAI.00136-20
88. Warner NC, Bartelt LA, Lachiewicz AM, Tompkins KM, Miller MB, Alby K, et al. Cefiderocol for the treatment of adult and pediatric patients with cystic fibrosis and Achromobacter xylosoxidans infections. Clin Infect Dis. (2021) 73:e1754–7. doi: 10.1093/cid/ciaa1847
89. Lignereux L, Chaber AL, Fretin D, Godfroid J, Saegerman C. Brucella melitensis biovar 1 isolation in a captive wildlife population in the United Arab Emirates. First isolation in the scimitar-horned Oryx (Oryx dammah). Vet Microbiol. (2022) 266:109360. doi: 10.1016/j.vetmic.2022.109360
90. Dadar M, Shahali Y, Fakhri Y, Godfroid J. The global epidemiology of Brucella infections in terrestrial wildlife: a meta-analysis. Transbound Emerg Dis. (2021) 68:715–29. doi: 10.1111/tbed.13735
91. Brand YE, Rufer B. Late prosthetic knee joint infection with Staphylococcus xylosus. IDCases. (2021) 24:e01160. doi: 10.1016/j.idcr.2021.e01160
92. Huys G, Purohit P, Tan CH, Snauwaert C, Vos P, Saffar HA, et al. Sphingobacterium cellulitidis sp. nov, isolated from clinical and environmental sources. Int J Syst Evol Microbiol. (2017) 67:1415–21. doi: 10.1099/ijsem.0.001832
93. Nemeghaire S, Argudín MA, Feßler AT, Hauschild T, Schwarz S, Butaye P. The ecological importance of the Staphylococcus sciuri species group as a reservoir for resistance and virulence genes. Vet Microbiol. (2014) 171:342–56. doi: 10.1016/j.vetmic.2014.02.005
94. Lu LX, He KW, Ni YX, Yu ZY, Mao AH. Exudative epidermitis of piglets caused by non-toxigenic Staphylococcus sciuri. Vet Microbiol. (2017) 199:79–84. doi: 10.1016/j.vetmic.2016.12.016
95. Hu XJ, Zheng BW, Jiang HY, Kang Y, Cao Q, Ning HB, et al. Draft genome sequence of Staphylococcus sciuri subsp. sciuri strain Z8, isolated from human skin. Genome Announc. (2015) 3:e00714–15. doi: 10.1128/genomeA.00714-15
Keywords: tick, Dermacentor nuttalli, growth stage, microbial population, high-throughput sequencing
Citation: Zhao L, Ma Y-M, Yang B, Han W-X, Zhao W-H, Chai H-L, Zhang Z-S, Zhan Y-J, Wang L-F, Xing Y, Yu L-F, Wang J-L, Ding Y-L and Liu Y-H (2022) Comparative analysis of microbial communities in different growth stages of Dermacentor nuttalli. Front. Vet. Sci. 9:1021426. doi: 10.3389/fvets.2022.1021426
Received: 17 August 2022; Accepted: 21 September 2022;
Published: 14 October 2022.
Edited by:
Yulong Gao, Harbin Veterinary Research Institute (CAAS), ChinaReviewed by:
Zhijun Hou, Northeast Forestry University, ChinaGuanghui Zhao, Northwest A&F University, China
Copyright © 2022 Zhao, Ma, Yang, Han, Zhao, Chai, Zhang, Zhan, Wang, Xing, Yu, Wang, Ding and Liu. This is an open-access article distributed under the terms of the Creative Commons Attribution License (CC BY). The use, distribution or reproduction in other forums is permitted, provided the original author(s) and the copyright owner(s) are credited and that the original publication in this journal is cited, in accordance with accepted academic practice. No use, distribution or reproduction is permitted which does not comply with these terms.
*Correspondence: Yong-Hong Liu, bHloZGt5QDEyNi5jb20=
†These authors have contributed equally to this work
‡ORCID: Li Zhao orcid.org/0000-0001-8538-5605
Yong-Hong Liu orcid.org/0000-0003-4432-9433