- 1College of Animal Science, Inner Mongolia Agricultural University, Hohhot, China
- 2Key Laboratory of Animal Genetics, Breeding and Reproduction, Hohhot, China
- 3Key Laboratory of Mutton Sheep Genetics and Breeding, Ministry of Agriculture and Rural Affairs, Hohhot, China
- 4Research Center for Animal Genetic Resources of Mongolian Plateau, College of Life Sciences, Inner Mongolia University, Hohhot, China
- 5Inner Mongolia Jinlai Animal Husbandry Technology Co., Ltd., Hohhot, China
The current study aimed to detect the relationship between the spermatozoa cryotolerance and the post-thawed sperm lipidome. Ejaculates from 20 goats, and performed a uniform frozen-thawed procedure in this study. According to the total motility of thawed sperm of goats, semen samples were classified into HF group (High Freezers, n = 8) with >60% total motility and LF group (Low Freezers, n = 8) with < 45% total motility. The lipidomic analysis based on UHPLC-MS/MS was utilized to investigate the relationship between sperm cryotolerance and their lipid metabolites expression. The results showed that the cryotolerance of sperm from different individual goats were in great variation. The total motility of post-thawed sperm in HF group (60.93 ± 2.43%) is significantly higher than that in LF group (34.04 ± 3.41%, P < 0.01). And the post-thawed sperm in HF group exhibited significantly higher plasma membrane (59.06 ± 2.34%) and acrosome integrity (62.93 ± 1.15%) than that in LF group (34.06 ± 4.85%, 44.92 ± 2.19% respectively, P < 0.01). The total of 29 lipid subclasses and 1,133 lipid molecules in the post-thawed goat sperm were identified by lipidomics analysis. The lipid content of thawed sperm in HF group was higher than that in LF group, the lipid profile in HF group was significantly separated from LF group, which indicated that the difference in lipid composition and lipid metabolism mode of sperm between the two groups was existed, especially the expression of phosphatidylcholine and triglyceride molecules. In conclusion, the cryotolerance of sperm from different individual goats were in great variation. Sperm with high cryotolerance may be able to uptake more lipids during cryopreservation. The increase in phosphatidylcholine and triglyceride content of thawed. Sperm may relate to more active lipid anabolic processes.
Introduction
Artificial insemination can utilize the male animals with fine genetic properties effectively, and rapidly increase the proportion of excellent individuals in population (1, 2). Semen cryopreservation can prolong the life of sperm, and is frequently used in artificial insemination (1–4). However, sperm undergo cold shock, ice crystal formation, oxidative stress, and other molecular changes during cryopreservation, resulting in significant freezing damage on sperm motility, viability, and structural integrity (5–7). Compared with fresh semen, the fertilization capacity of sperm after cryopreservation was reduced. Therefore, it will limit the applications of frozen-thawed semen for artificial insemination in goats (6, 8–10).
However, variations have been observed in the quality of semen from different individuals after frozen-thawed procedures, these were influenced by the lipid composition of the sperm plasma membrane, the freezing protocol, and species-specific characteristics (11–16). The plasma membrane is an extremely important structure for sperm physiology as it regulates the critical events of sperm cholesterol efflux and calcium influx during oocyte-sperm interactions (17–19). Injury to the plasma membrane can trigger cell death and loss of homeostasis (20, 21). Thus, the biochemical composition of the plasma membrane is an important topic in sperm physiology and cryopreservation. The sperm plasma membrane is assumed to be the main structure in which sperm freezing damage occurs (22). Such damage results in membrane instability caused by lateral lipid rearrangement and membrane lipid loss (23, 24), and has potential impacts on sperm motility, osmotic stress response, and capacitation (25–28). Goat sperm are more sensitive to the cryopreservation process than those of other domestic species, possibly owing to lipid changes in the plasma membrane of goat sperm during cryopreservation (29). In addition, some studies have reported that sperm cells take up lipids or fatty acids from their environment during in vitro incubation (30–32).
Generally, there has been a lack of consistent cryopreservation protocols for different individual. The sperm motility of goats after thawed is still not good. Thus, it is necessary to further address the limitations of the goat semen cryopreservation technique. In this study, we performed uniform freeze-thawed process to goat sperm from different individuals, and the lipidome variation among semen samples with different quality after thawed were revealed by lipidomic analysis. All of these will provide a research basis and possible future directions for improving the goat semen cryopreservation technology.
Materials and methods
Semen collection, cryopreservation and thawing
Semen samples were provided by the Inner Mongolia Jinlai Livestock Technology Co., Ltd. (TuZuo country, Hohhot, Inner Mongolia, China). Twenty male Inner Mongolian cashmere goats at ages of 2 years old were selected, and the semen in each individual was collected three times a week during the reproductive season. The semen volumes in each sample ranged from 0.75 to 1.5 mL. The semen concentration was measured by A Bovine Accuread Photometer (IMV, France). The criteria for semen samples used for subsequent analysis are as follows: concentration of 20–30 × 108 sperm/mL, and percentage of sperm total motility ≥75%.
Except for antibiotics, all chemicals used in this study were purchased from Sigma-Aldrich (St. Louis, MO, USA). The collected fresh semen was diluted with preheated a Tris-citric-glucose base solution at the volume ratio of 1:5 at 37°C, and centrifugally washed at 600 g at 4°C for 3 min, this process was repeated twice to remove the seminal plasma. Then, the washed sperm were extended with freezing diluent at 37°C, semen concentration was adjusted to 2 × 108 sperm/mL, equilibrated at 4°C for 3 h. The freezing diluent were composed of 300 mM Tris, 95 mM citric acid, 56 mM glucose, 10% (v/v) egg yolk, 5% (v/v) glycerol, and 1% (v/v) antibiotics (Gibco). The chilled semen was loaded into 0.25 mL straws, and exposed to liquid nitrogen vapor at a height of 4 cm for 7 min. The straws were then placed into liquid nitrogen for storage. After 2 days of storage, straws were thawed in a water bath at 37°C for 30 s. The thawed semen was centrifuged at 10,000 g for 3 min, and the pellet was washed twice before being subjected lipidomic analysis.
CASA-motility evaluation
The sperm motility was assessed soon after thawing. A 3 μL thawed semen was dropped into a prewarmed Leja slide analysis chamber. A computer-assisted semen analyzed system (CASA, IVOS II, IMV, France) was applied to measure sperm motion parameters at 250 × magnification. A minimum of five fields were evaluated per sample, and a minimum of 1,000 sperm were counted. The main sperm motion parameters measurements included total motility (TM; %), progressive motility (PM; %), curvilinear velocity (VCL; μm/s), straight line velocity (VSL; μ/s), average path velocity (VAP; μ/s), linearity (LIN, %) and the amplitude of lateral head displacement (ALH; μ). The definitions of these sperm motility parameters can be found in Dorado (33), and the customized settings used for goat sperm are shown in Table 1.
Flow cytometric analysis
The fluorescence signals of marked sperm were analyzed by using a flow cytometer (ACEA NovoCyteTM, Agilent, USA) equipped with a 488 nm blue all-solid-state laser, a 640 nm red all-solid-state laser, and a photomultiplier tube. The applied fluorescence channels (FITC, PE, PerCP, and APC) were monitored simultaneously. The recorded parameters included the area of all channels, width, height, and time. These parameters were used to adherent cells, cell debris and single cells to be effectively distinguished. A total of 20,000 events were analyzed for each semen sample.
The plasma membrane integrity status of the frozen-thawed sperm was evaluated using Annexin V (AV) and propidium iodide (PI, BD Biosciences, USA) following the protocol described by Peña (34). Each semen aliquot was diluted in 1 × binding buffer, supplied with the kit, to a concentration of 1.2 × 106 cells/mL. Fluorescent dyes (5 μL AV and 5 μL PI; 50 μg/mL) were added to the sperm suspension to enable identification of cell events, following incubation in the dark, and sperm were washed in PBS. The AV/PI results revealed four sperm subpopulations: live sperm (AV–/PI–), live sperm with damaged plasma membrane (AV+/PI–), dead cells (AV+/ PI+), and dead late-necrotic cells (AV–/PI+).
The acrosome status of the frozen-thawed sperm was assessed using FITC-peanut (Arachis hypogaea) agglutinin (GENMED SCIENTIFICS, INC. USA). Briefly, frozen-thawed semen was diluted to 1.2 × 107 cells/mL, and mixed with 150 μL FITC-PNA and 200 μL PI (0.4 μg/mL). The mixed suspensions were incubated in a darkened area for 15 min (35). After staining, the FITC-PNA signal (green) was read as an intact acrosome marker, and dead sperm were indicated by the PI signal (red). Readings were performed on each sample, and four sperm populations were identified using FITC/ PerCP filters: sperm particles, dead sperm, intact sperm and sperm with damaged acrosome.
Sample preparation and lipid extraction
The methyl tert-butyl ether (MTBE) technique was used to extract lipids. In brief, samples were homogenized using 240 μL methanol and 200 μL water. Following the addition of 800 L MTBE, the mixture was subjected to ultrasonication for 20 min at 4°C before being left undisturbed remaining still for 30 min at room temperature. The solution was centrifuged at 14,000 g for 15 min, at 10°C, and the upper organic solvent layer was collected and dried under nitrogen.
LC-MS/MS method for lipid analysis
A CSH C18 column (1.7 μm, 2.1 mm × 100 mm, Waters) was used for LC separation utilizing reversed-phase chromatography. The lipid extracts were re-diluted in 200 μL of a 90/10 mixture of isopropanol and acetonitrile and centrifuged at 14,000 g for 15 min, after which 3 μL of the sample was injected later. Acetonitrile-water (6:4) and acetonitrile-isopropanol (1:9), both with formic acid and ammonium formate contents of 0.1% and 0.1 Mm, served as solvents A and B, respectively. At a flow rate of 300 L/min, the initial mobile phase of solvent B contained 30% of its volume. It was maintained for 2 min, increased linearly to 100% solvent B over 23 min, and then equilibrated in 5% solvent B for 10 min.
Q-Exactive Plus was used to acquire positive and negative mode mass spectra. All measurements used the following optimized preset ESI parameters: ion spray voltage 3,000 V; S-Lens RF level 50%; source temperature 300°C, and capillary temperature was 350°C. The scan range of the instruments was 200–1,800 m/z.
Data processing and analysis
“Lipid Search” is a search engine for identifying lipid species based on MS/MS math. In the LipidSearch database, there are more than 1,500,000 fragment ions and more than 30 lipid classes. The precursor and fragment mass tolerances were both set to 5 ppm. Peak identification, peak extraction, and lipid identification were all performed using LipidSearch (secondary identification). The precursor tolerance is set at 5 ppm, the product tolerance is set at 5 ppm, and the product ion threshold is set at 5%.
Univariate statistical analysis, multivariate statistical analysis, and hierarchical cluster analysis were performed on the data retrieved by LipidSearch. Student's t-test, a non-parametric test, and multiplicity of variation analysis were included in the univariate statistical analysis. The multivariate statistical analysis included orthogonal partial least square discriminant analysis (OPLS-DA). The variable importance in projection (VIP) scores were evaluated, and the metabolites with VIP >1 were chosen as class discriminants.
Statistical analysis
The difference significance of kinetics and flow cytometry variables of all samples between the HF and LF groups were analyzed using Student's t-test in SPSS statistical software (version 22.0; Chicago, IL, USA). And the data are presented as mean ± SEM.
Results
Identification of goats with HF or LF and sperm evaluation
The quality of frozen-thawed sperm varied among the individuals. The frozen-thawed sperm samples from different goat individuals were sorted based on total motility (Figure 1), the eight samples with the higher total motility (>60%) were as HF group, and the another eight samples with the lower motility (< 45%) were as LF group, and the motion characteristics showed significant differences between HF and LF (P < 0.01, Table 2), all of the motion parameters in HF group was significant higher than that in LF group. The results showed that sperm plasma membrane integrity and acrosome integrity were higher in the HF group than that in the LF group (P < 0.01, Figures 2A,B).
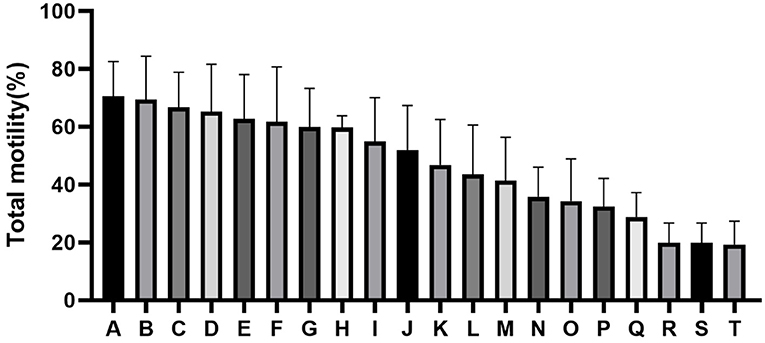
Figure 1. Difference in percentage total motility as determined by computer-aided sperm analysis (CASA) assessment of spermatozoa from all goats (A-T).
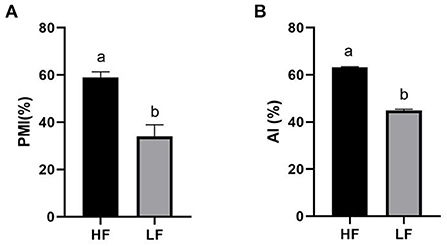
Figure 2. Differences of sperm parameters. Percent differences in the levels of (A) PMI, plasma membrane integrity, (B) AI, acrosome integrity. The data are presented as mean±SEM. (P < 0.05, calculated using Student's t-test).
Identification of lipid class and species quantity
LipidSearch was used to analyze the data in both positive and negative modes in this study. The lipid classes and the number of lipids identified in each class are shown in Figure 3. It was found that 29 lipid classes and the number of 1,133 lipids species were identified. Phosphatidylcholine, phosphatidylethanolamine, triglyceride, ceramides, sphingomyelin were observed as the major lipid classes in the goat sperm. The total lipid content in HF sperm was higher than that in LF sperm, and the relative abundance of each lipid subclass in HF and LF groups is shown in Figure 4.
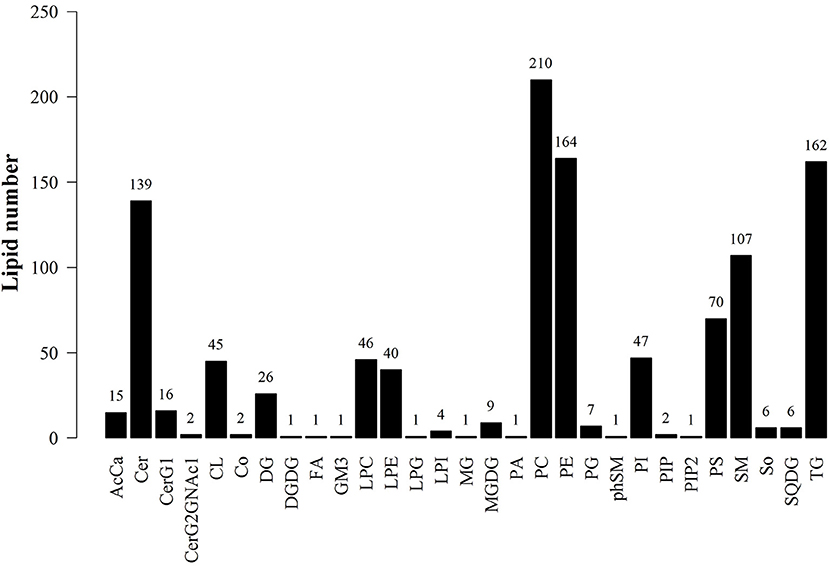
Figure 3. Statistics of lipid subclasses and lipid molecules. AcCa (Acyl Carnitine), Cer (ceramides), CerG1 (glucocerebroside), CerG2GNAc1 (Simple Glc series), CL (cardiolipins), Co (Coenzyme), DG (Triacylglycerol), DGDG (digalacyosyl diacylglycerols), EPA (Eicosapentaenoic acid), FA (fatty acid), GM3 (Gangliosides), LPC (lyso-Phosphatidylcholine), LPE (lyso-Phosphatidylethanolamine), LPG(lyso-phosphatidylglycerols), LPI (lyso-phosphatidylethanola), MG (monoacylglycerol), MGDG (monogalactosyldiacylglycerols), PA (phosphatidic acids), PE (phosphatidylethanola mines), PG (phosphatidylglycerols), PhSM (phytosphingosine), PI (phosphatidylethanola), PIP (phosphatidylinositol (4)phosphate), PIP2 (phosphatidylinositol (4,5)bisphosphate), PS (phosphatidylserines), SM (sphingomyelin), So (Sphingosine), SQDG (Sulfoquinovosyldiacylglycerol), TG (triglyceride).
Multivariate statistical analysis
The clear segregation of lipidomic profiles between HF and LF was not observed using principal component analysis (PCA). Further supervised analysis, PLS-DA and OPLS-DA were conducted using the training set (Figure 5). The performance characteristics of the PLS-DA and OPLS-DA models were R2(X) = 0.575; R2(Y) = 0.557; and Q2(Y) = −0.723. The permutation test (200 random permutations) validated the model, and no overfitting of the data was observed. According to PLS-DA score plots, the HF and LF groups were separated into two distinct clusters.
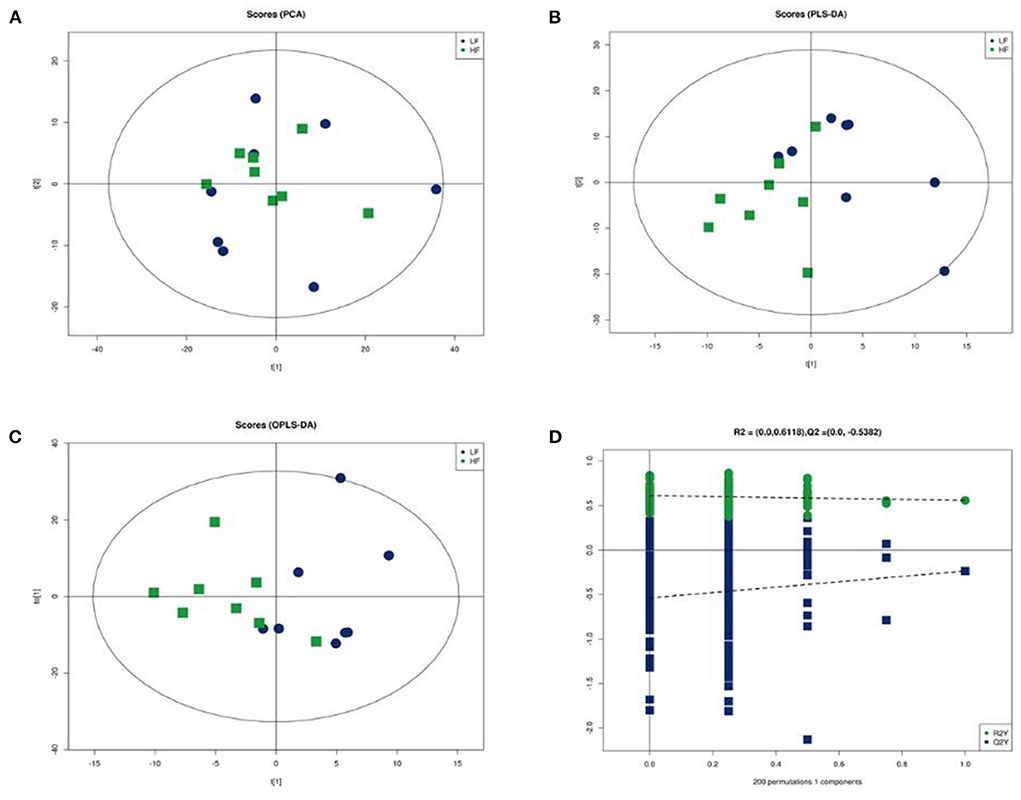
Figure 5. Multivariate statistical analysis of seminal plasma metabolic profiling between HF and LF. (A) Principal component analysis score plot. (B) Partial least squares-discriminant analysis score plot. (C) Orthogonal partial least squares-discriminant analysis (OPLS-DA) score plot. (D) Statistical validation of the established OPLS-DA model with permutation analysis (200 random permutations).
The potential of the lipid species as a marker was assessed by the VIP score for the PLS-DA model. Variables with VIP scores >1 was regarded as significant for the classification model. The phosphatidylcholine and triglyceride lipid species showed significant difference between the HF and LF groups (Table 3), and they showed a higher abundance in the HF group.
Identification and cluster analysis of potential lipid molecule markers
Univariate statistical analyses, including fold change analysis and t-test, were used to visualize the significance of lipid changes between the HF and LF sperm after cryopreservation, which can screen for potential lipid molecular markers. A volcano plot was drawn using an FC > 1.5 or FC < 0.67 and P < 0.05 as the screening criteria (Figure 6), to assess the abundance of differential lipids and illustrate the connection between samples and variations in lipid expression patterns. To evaluate the rationality of differential lipids and visualize the relationship between samples and the differences in lipid expression patterns, the samples were hierarchically clustered based on the expression of significantly differential lipids molecular (VIP>1, P < 0.05). It was observed that the lipid expression profiles could be clearly divided into two categories (Figure 7). Therefore, it was indicated that sperm from HF and LF groups in goats showed significantly differential lipid expression patterns after cryopreservation.
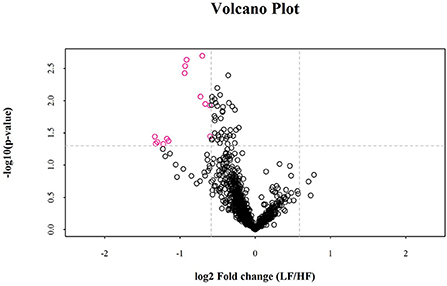
Figure 6. Volcano plots for the distribution of significantly differences in lipid molecules. The rosy dots are significantly differential lipid molecules screened for univariate statistical analysis.
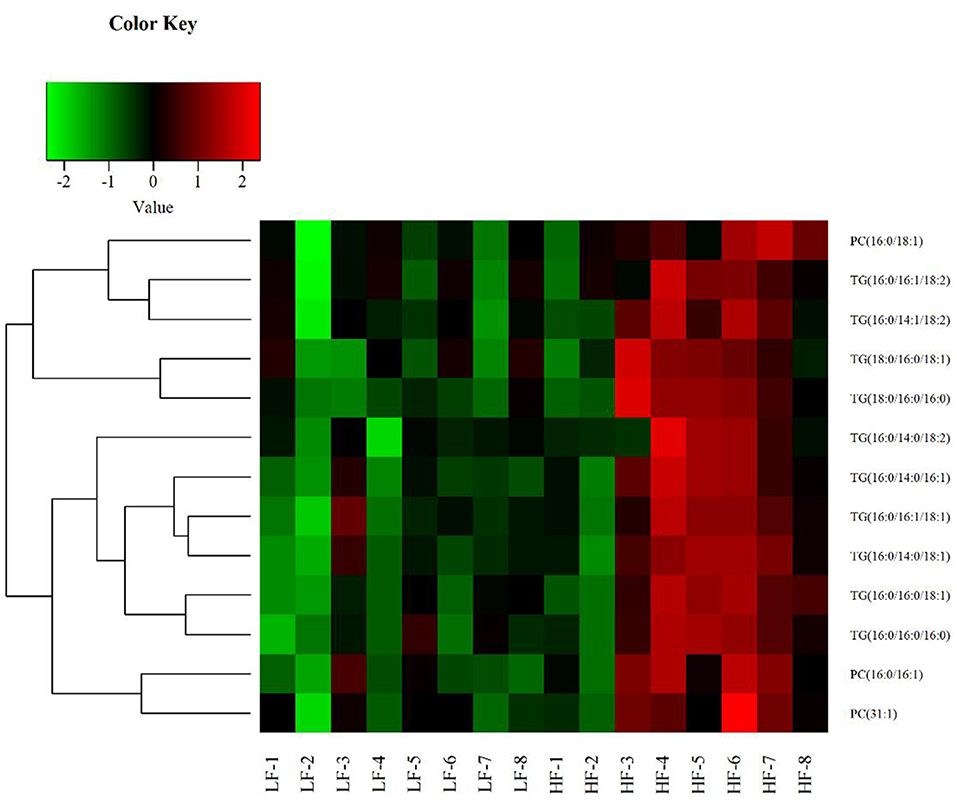
Figure 7. Hierarchical Clustering results of significant differential lipid molecules. The greenpart represents down regulation of expression. The red part represents upregulation.
Discussion
Semen cryopreservation causes the sperm to undergo considerable biophysical and biochemical changes, including alterations to membranes and their constituent parts, resulting in decreased post-thaw sperm motility and fertilization ability (36, 37). However, semen from different individuals had the various capacity to protect against freezing damage (38–41). In this study, the extent of cryopreservation-induced changes in motility to characterize variation in sperm cryotolerance across different individuals were measured. It was found that the quality of post-thawed sperm from different individuals varies greatly in motion parameter and structural integrity after a unified cryopreservation procedure. For 20 goats studied, eight goats were classified as high freezers group, and the sperm quality after thawed were better. The sperm from another eight goats showed very low quality after cryopreservation. However, the mechanism of the sperm cryotolerance among different individuals was not explained from now on. Such differences could be due to genetic and non-genetic factors. In origin, and making genetic selection of males as an important factor for successful sperm freezing (42). In previous studies, it attempted to elucidate differences in sperm cryotolerance through investigating marker prediction, management system and cryopreservation technology processes (43, 44). Thus, it is the first time to characterize the lipidomic profiles of post-thawed sperm with high and low freezing resistance, and investigate the relationship between the lipids of the sperm and the post-thawing sperm characteristics of goats.
As the main components of sperm plasma membranes, the lipids play an important role in regulating plasma membrane function (17, 45). The lipid composition of the sperm plasma membrane was susceptible to alteration during cryopreservation, affecting sperm motility and fertilization potential (45). Lipidomics is a rapid and sensitive tool for systematically resolving changes in lipid composition and expression in sperm (46). It was found that the lipids of sperm after freeze-thawed contained a large amount of the lipid subclass, such as phosphatidylcholine (PC), phosphatidethanolamine (PE), triglycerides (TG), ceramides (CER), sphingolipids (SM). Compared with LF group, the total lipids in the thawed sperm from HF was higher. Sperm with high and low cryotolerance had different heritability, transcripts, proteins, and metabolites associated with sperm function (47–50). The lipidomic analysis of post-thawed sperm in this study revealed that the lipid metabolism patterns with different cryotolerance were various, and the lipid molecules of PC and TG were significantly different between the two groups.
The higher relative abundances of phosphatidylcholines was observed in high Freezer group, represented by ions of m/z 718.54, 732.55, and 760.59. According to previous research, a positive correlation between phosphatidylcholines abundance and the sperm motility in thawed sperm had been found (12, 51). As the biomarkers related to sperm freezability, PC may affect the motility, membrane integrity and lipid peroxidation (13, 52). As the primary component of the plasma membrane, phosphatidylcholines are crucial for controlling the function of the membrane, particularly membrane fusion, fluidity and protein binding (53). During the cryopreservation of goat semen, the addition of a substance rich in phosphatidylcholines prevents sperm from suffering from decreased motility caused by cold shock as well as structural damage (54–56). When adding sperm freezing solution, isolated soybean phosphatidylcholine displays cryoprotective properties comparable to those of egg yolk. Compared to phosphatidylcholine or egg yolk alone, egg yolk and soy-phosphatidylcholine are added in combination have been found to support better post-thaw outcomes (57). The PC molecules were amphipathic. It could be incorporated into sperm membrane for preventing mechanical damage to membrane structures during cryopreservation processes, also be taken up and utilized during lipid metabolism (58). However, the PC supplement interacts with sperm membrane influencing the lipid profile of the post-thawed sperm is still unclear, it needed to be further studied.
In our study, the thawed HF sperm had a higher triglyceride content. However, triglycerides do not act as lipid components in plasma membranes, and the adverse effects of high levels of triglycerides on sperm have been described. The study showed that rats with diabetes exhibit decreased sperm motility and antioxidant enzyme activity and increased triglyceride levels in sperm (59). In infertility mice subjected to Slc22a14 (riboflavin transporter) knockout, triglycerides accumulate in sperm, and significant fatty acid β-oxidation deficiencies are observed. Additionally, levels of in acylcarnitines and metabolites from the TCA cycle were significantly reduced (60). In this study, the thawed sperm in HF group showed better quality before freezing. Therefore, the increase of the relative abundance of triglyceride lipid molecules after thawing may be due to that the sperm uptake lipids in the freezing diluent during cryopreservation. The previous studies reported that exogenous lipids can bind to the surface of the sperm plasma membrane, and provide a physical barrier to cryogenic damage by changing the lipid composition of the membrane (61, 62). Exogenous lipids are exchanged between the medium and the membrane, replenishing the lipids lost and stabilizing cold shock (24, 63). The thawed boar sperm with better quality may have a stronger affinity for yolk lipoprotein. The lipid content of the post-thawed sperm was increased, and was rich in triglycerides and phosphatidylcholine (64). The lipid synthesis capacity of mammalian sperm had been described in previous studies (65), and describing the synthesis of triglycerides have not been elaborated.
Conclusions
In summary, the differences in the cryotolerance of sperm among individual goats were found. And it was observed that the total lipid content in the high freezer sperm after thawed is higher than that in the LF sperm, especially phosphatidylcholine and triglycerides subclass molecules. It may be that the high freezer sperm were able to take up more lipids in freezing diluent and may have the active lipid anabolic processes. But more research is needed to further understand the mechanism of lipid uptake in goat sperm and its relationship with the quality of goat sperm after thawed to improve preservation methods.
Data availability statement
The datasets presented in this study can be found in online repositories. The names of the repository/repositories and accession number(s) can be found in the article/supplementary material.
Ethics statement
The animal study was reviewed and approved by the Animal Ethics Committee of the Inner Mongolia Academy of Agriculture and Animal Husbandry Sciences. Written informed consent was obtained from the owners for the participation of their animals in this study.
Author contributions
BX, RW, and ZhiW conceptualized the study. BX, RW, ZhiW, JL, and JZ conceptualized, designed and carried out the investigations. BX, HL, ZheW, and WZ performed the experiments. BX, YZ, RS, YL, and ZL analyzed the data. BX wrote the paper. JZ and JL critically revised the manuscript. All authors contributed to the article and approved the submitted version.
Funding
This work was supported by the Major Projects of the Inner Mongolia Autonomous Region of China (No. 2020ZD0003), Inner Mongolia Autonomous Region Science and Technology Research Project (No. 2021GG0086), and China Agriculture Research System of MOF and MARA (No. CARS-39-06).
Acknowledgments
The authors are grateful to the staff of Inner Mongolia Jinlai Livestock Technology Co., Ltd for providing assistance.
Conflict of interest
Author JL was employed by the company Inner Mongolia Jinlai Livestock Technology Co., Ltd.
The remaining authors declare that the research was conducted in the absence of any commercial or financial relationships that could be construed as a potential conflict of interest.
Publisher's note
All claims expressed in this article are solely those of the authors and do not necessarily represent those of their affiliated organizations, or those of the publisher, the editors and the reviewers. Any product that may be evaluated in this article, or claim that may be made by its manufacturer, is not guaranteed or endorsed by the publisher.
References
1. Cardoso Consentini CE, Wiltbank MC, Sartori R. Factors that optimize reproductive efficiency in dairy herds with an emphasis on timed artificial insemination programs. Animals. (2021) 11:301. doi: 10.3390/ani11020301
2. Amoah EA, Gelaye S. Biotechnological advances in goat reproduction. J Anim Sci. (1997) 75:578–85. doi: 10.2527/1997.752578x
3. Leboeuf B, Restall B, Salamon S. Production and storage of goat semen for artificial insemination. Anim Reprod Sci. (2000) 62:113–41. doi: 10.1016/S0378-43200000156-1
4. Cseh S, Faigl V, Amiridis GS. Semen processing and artificial insemination in health management of small ruminants. Anim Reprod Sci. (2012) 130:187–92. doi: 10.1016/j.anireprosci.2012.01.014
5. Sung-Jae Y, Woo-Sung K, Saidur RM, June-Sub L, Pang MG, Wei Y. A Novel approach to identifying physical markers of cryo-damage in bull spermatozoa. Plos ONE. (2015) 10:e0126232-e. doi: 10.1371/journal.pone.0126232
6. Sung-Jae Y, Saidur RM, Woo-Sung K, Yoo-Jin P, Pang MG, Guo X. Addition of cryoprotectant significantly alters the epididymal sperm proteome. PLoS ONE. (2016) 11:e0152690-e. doi: 10.1371/journal.pone.0152690
7. Peris-Frau P, Soler AJ, Iniesta-Cuerda M, Martin-Maestro A, Sanchez-Ajofrin I, Medina-Chavez DA, et al. Sperm cryodamage in ruminants: understanding the molecular changes induced by the cryopreservation process to optimize sperm quality. Int J Mol Sci. (2020) 21:2781. doi: 10.3390/ijms21082781
8. Watson PF. The Causes of Reduced Fertility with Cryopreserved Semen. Anim Reprod Sci. (2000) 60:481-92. doi: 10.1016/S0378-4320(00)00099-3
9. Squires E, Barbacini S, Matthews P, Byers W, Schwenzer K, Steiner J, et al. Retrospective study of factors affecting fertility of fresh, cooled and frozen semen. Equine Vet Educ. (2006) 18:96-9. doi: 10.1111/j.2042-3292.2006.tb00425.x
10. Masoudi R, Zare Shahneh A, Towhidi A, Kohram H, Akbarisharif A, Sharafi M. Fertility response of artificial insemination methods in sheep with fresh and frozen-thawed semen. Cryobiology. (2017) 74:77-80. doi: 10.1016/j.cryobiol.2016.11.012
11. Lv C, Wu G, Hong Q, Quan G. Spermatozoa cryopreservation: state of art and future in small ruminants. Biopreserv Biobank. (2019) 17:171–82. doi: 10.1089/bio.2018.0113
12. Lucio CF, Brito MM, Angrimani D, Belaz K, Morais D, Zampieri D, et al. Lipid composition of the canine sperm plasma membrane as markers of sperm motility. Reprod Domest Anim. (2017) 52 (Suppl 2):208–13. doi: 10.1111/rda.12860
13. Cabrera T, Ramires-Neto C, Belaz KRA, Freitas-Dell'aqua CP, Zampieri D, Tata A, et al. Influence of spermatozoal lipidomic profile on the cryoresistance of frozen spermatozoa from stallions. Theriogenology. (2018) 108:161–6. doi: 10.1016/j.theriogenology.2017.11.025
14. Wood PL, Scoggin K, Ball BA, Troedsson MH, Squires EL. Lipidomics of equine sperm and seminal plasma: identification of amphiphilic (O-acyl)-ω-hydroxy-fatty acids. Theriogenology. (2016) 86:1212–21. doi: 10.1016/j.theriogenology.2016.04.012
15. Zerbinati C, Caponecchia L, Rago R, Leoncini E, Bottaccioli AG, Ciacciarelli M, et al. Fatty acids profiling reveals potential candidate markers of semen quality. Andrology. (2016) 4:1094–101. doi: 10.1111/andr.12236
16. Braga LT. Jr., Bravo N, Belaz K, Zampieri D, Eberlin MN, Conforti VA. Lipid profiles of canine spermatozoa as revealed via matrix-assisted laser desorption/ionization mass spectrometry. Reprod Domest Anim. (2016) 51:1055–8. doi: 10.1111/rda.12792
17. Flesch FM, Gadella BM. Dynamics of the mammalian sperm plasma membrane in the process of fertilization. Biochim Biophys Acta. (2000) 1469:197–235. doi: 10.1016/S0304-4157(00)00018-6
18. Felix R. Molecular physiology and pathology of Ca2+-conducting channels in the plasma membrane of mammalian sperm. Reproduction. (2005) 129:251–62. doi: 10.1530/rep.1.00478
19. Töpfer-Petersen E, Petrounkina AM, Ekhlasi-Hundrieser M. Oocyte-sperm interactions. Anim Reprod Sci. (2000) 60-61:653-62. doi: 10.1016/S0378-4320(00)00128-7
20. Guthrie HD, Welch GR. Impact of storage prior to cryopreservation on plasma membrane function and fertility of boar sperm. Theriogenology. (2005) 63:396–410. doi: 10.1016/j.theriogenology.2004.09.020
21. Sieme H, Oldenhof H, Wolkers WF. Sperm membrane behaviour during cooling and cryopreservation. Reprod Domest Anim. (2015) 50 (Suppl 3):20–6. doi: 10.1111/rda.12594
22. Parks JE, Lynch DV. Lipid composition and thermotropic phase behavior of boar, bull, stallion, and rooster sperm membranes. Cryobiology. (1992) 29:255–66. doi: 10.1016/0011-2240(92)90024-V
23. Quinn PJ, A. Lipid-phase separation model of low-temperature damage to biological membranes. Cryobiology. (1985) 22:128–46. doi: 10.1016/0011-2240(85)90167-1
24. Buhr MM, Curtis EF, Kakuda NS. Composition and behavior of head membrane lipids of fresh and cryopreserved boar sperm. Cryobiology. (1994) 31:224–38. doi: 10.1006/cryo.1994.1028
25. Holt WV. Fundamental aspects of sperm cryobiology: the importance of species and individual differences. Theriogenology. (2000) 53:47–58. doi: 10.1016/S0093-691X(99)00239-3
26. Fukami AK, Ken-ichi S, Keiichi S, Zhize O, Tetsushi I, Yasushi U, et al. Uroplakin III, a novel src substrate in xenopus egg rafts, is a target for sperm protease essential for fertilization. Dev Biol. (2005) 286:483–92. doi: 10.1016/j.ydbio.2005.08.020
27. Rajamanickam GD, Kastelic JP, Thundathil JC. Na/K-ATPase regulates bovine sperm capacitation through raft- and non-raft-mediated signaling mechanisms. Mol Reprod Dev. (2017) 84:1168–82. doi: 10.1002/mrd.22879
28. Tapia JA, Macias-Garcia B, Miro-Moran A, Ortega-Ferrusola C, Salido GM, Pena FJ, et al. The membrane of the mammalian spermatozoa: much more than an inert envelope. Reprod Domest Anim. (2012) 47 (Suppl s3):65-75. doi: 10.1111/j.1439-0531.2012.02046.x
29. Darin-Bennett A, White IG. Influence of the cholesterol content of mammalian spermatozoa on susceptibility to cold-shock. Cryobiology. (1977) 14:466–70. doi: 10.1016/0011-2240(77)90008-6
30. Vazquez JM, Roldan ER. Phospholipid metabolism in boar spermatozoa and role of diacylglycerol species in the De Novo formation of phosphatidylcholine. Mol Reprod Dev. (2015) 47:105–12. doi: 10.1002/(SICI)1098-2795(199705)47:1<105::AID-MRD14>3.0.CO;2-0
31. Islam MM, Umehara T, Tsujita N, Shimada M. Saturated fatty acids accelerate linear motility through mitochondrial atp production in bull sperm. Reprod. Med. Biol. (2021) 20:289–98. doi: 10.1002/rmb2.12381
32. Kaka A, Haron W, Yusoff R, Yimer N, Khumran AM, Sarsaifi K, et al. Effect of docosahexanoic acid on quality of frozen-thawed bull semen in bioxcell extender. Reprod. Fertil. Dev. (2017) 29:490–5. doi: 10.1071/rd15089
33. Dorado J, Rodriguez I, Hidalgo M. Cryopreservation of goat spermatozoa: comparison of two freezing extenders based on post-thaw sperm quality and fertility rates after artificial insemination. Theriogenology. (2007) 68:168–77. doi: 10.1016/j.theriogenology.2007.04.048
34. Peña FJ, Johannisson A, Wallgren M, Rodríguez-Martínez H. Assessment of fresh and frozen-thawed boar semen using an annexin-v assay: a new method of evaluating sperm membrane integrity. Theriogenology. (2003) 60:677–89. doi: 10.1016/S0093-691X(03)00081-5
35. Mocé E, Grasseau I, Blesbois E. Cryoprotectant and freezing-process alter the ability of chicken sperm to acrosome react. Anim Reprod Sci. (2010) 122:359–66. doi: 10.1016/j.anireprosci.2010.10.010
36. Watson PF. The responses of boar sperm membranes to cold shock and cooling. In: International Conference on Deep Freezing of Boar Semen, Uppsala (Sweden). (1985). pp 25-7.
37. Upadhyay VR, Ramesh V, Dewry RK, Kumar G, Raval K, Patoliya P. Implications of cryopreservation on structural and functional attributes of bovine spermatozoa: an overview. Andrologia. (2021) 53:e14154. doi: 10.1111/and.14154
38. Rickard JP, Schmidt RE, Maddison JW, Bathgate R, Lynch GW, Druart X, et al. Variation in seminal plasma alters the ability of ram spermatozoa to survive cryopreservation. Reprod Fertil Dev. (2016) 28:516–23. doi: 10.1071/RD14123
39. Ryu DY, Song WH, Pang WK, Yoon SJ, Rahman MS, Pang MG. Freezability biomarkers in bull epididymal spermatozoa. Sci Rep. (2019) 9:12797. doi: 10.1038/s41598-019-49378-5
40. Evans HC, Dinh TTN, Ugur MR, Hitit M, Sajeev D, Kaya A, et al. Lipidomic markers of sperm cryotolerance in cattle. Sci Rep. (2020) 10:20192. doi: 10.1038/s41598-020-77089-9
41. Ugur MR, Dinh T, Hitit M, Kaya A, Topper E, Didion B, et al. Amino acids of seminal plasma associated with freezability of bull sperm. Front Cell Dev Biol. (2019) 7:347. doi: 10.3389/fcell.2019.00347
42. Sieme H, Harrison RA, Petrunkina AM. Cryobiological determinants of frozen semen quality, with special reference to stallion. Anim Reprod Sci. (2008) 107:276–92. doi: 10.1016/j.anireprosci.2008.05.001
43. Yeste M. Sperm cryopreservation update: cryodamage, markers, and factors affecting the sperm freezability in pigs. Theriogenology. (2016) 85:47–64. doi: 10.1016/j.theriogenology.2015.09.047
44. Kumar N, Rai B, Bhat SA, Kharche SD, Gangwar C, Jindal SK, et al. Effect of management system and season on semen freezability in Jakhrana Bucks. Vet World. (2016) 9:199–202. doi: 10.14202/vetworld.2016.199-202
45. Hinkovska-Galcheva V, Petkova D, Koumanov K. Changes in the phospholipid composition and phospholipid asymmetry of ram sperm plasma membranes after cryopreservation. Cryobiology. (1989) 26:70–5. doi: 10.1016/0011-2240(89)90034-5
46. Hu T, Zhang JL. Mass-Spectrometry-Based Lipidomics. J Sep Sci. (2018) 41:351–72. doi: 10.1002/jssc.201700709
47. Lavara R, Moce E, Baselga M, Vicente JS. Freezability genetics in rabbit semen. Theriogenology. (2017) 102:54–8. doi: 10.1016/j.theriogenology.2017.07.013
48. Rickard JP, Leahy T, Soleilhavoup C, Tsikis G, Labas V, Harichaux G, et al. The identification of proteomic markers of sperm freezing resilience in ram seminal plasma. J Proteomics. (2015) 126:303–11. doi: 10.1016/j.jprot.2015.05.017
49. Longobardi V, Kosior MA, Pagano N, Fatone G, Staropoli A, Vassetti A, et al. Changes in bull semen metabolome in relation to cryopreservation and fertility. Animals. (2020) 10:1065. doi: 10.3390/ani10061065
50. Fraser L, Brym P, Pareek CS, Mogielnicka-Brzozowska M, Paukszto Ł, Jastrzebski JP, et al. Transcriptome analysis of boar spermatozoa with different freezability using RNA-seq. Theriogenology. (2020) 142:400–13. doi: 10.1016/j.theriogenology.2019.11.001
51. Simpson AM, Swan MA, White IG. Susceptibility of epididymal boar sperm to cold shock and protective action of phosphatidylcholine. Gamete Res. (1987) 17:355. doi: 10.1002/mrd.1120170408
52. Yu J, Wang Z, An X, Liu H, Wang F, Ji C, et al. Metabolomic profiling of dezhou donkey sperm associated with freezability. Theriogenology. (2022) 181:131–9. doi: 10.1016/j.theriogenology.2022.01.020
53. Fhaner CJ, Liu S, Zhou X, Reid GE. Functional group selective derivatization and gas-phase fragmentation reactions of plasmalogen glycerophospholipids. J. Mass Spectrom. (2013) 2:S0015. doi: 10.5702/massspectrometry.S0015
54. Najafi A, Daghigh Kia H, Mehdipour M, Shamsollahi M, Miller DJ. Does Fennel extract ameliorate oxidative stress frozen-thawed ram sperm? Cryobiology. (2019). doi: 10.1016/j.cryobiol.2019.02.006
55. Singh A, Bhakat M, Mohanty TK, Mondal S, Yadav SK, Kumar P, et al. Effect of tris-egg yolk, soya milk, and liposome-based extenders on Sahiwal (Bos Indicus) sperm quality during pre- and post-cryopreservation stages. Cryo Lett. (2019) 40:94-102
56. Tabarez A, García W, Palomo MJ. Soy lecithin as a potential alternative to powdered egg yolk for buck sperm cryopreservation does not protect them from mitochondrial damage. Anim Reprod Sci. (2020). doi: 10.1016/j.anireprosci.2020.106473
57. Vireque AA, Tata A, Silva OF, LoTurco EG, Azzolini A, Ferreira CR, et al. Effects of N-6 and N-3 polyunsaturated acid-rich soybean phosphatidylcholine on membrane lipid profile and cryotolerance of human sperm. Fertil Steril. (2016) 106 :273-83 e6. doi: 10.1016/j.fertnstert.2016.03.044
58. Gadella BM, Miller NG, Colenbrander B, van Golde LM, Harrison RA. Flow cytometric detection of transbilayer movement of fluorescent phospholipid analogues across the boar sperm plasma membrane: elimination of labeling artifacts. Mol Reprod Dev. (1999) 53:108–25. doi: 10.1002/(SICI)1098-2795(199905)53:1<108::AID-MRD13>3.0.CO;2-K
59. Belhan S, Yildirim S, Huyut Z, Özdek U, Oto G, Algül S. Effects of curcumin on sperm quality, lipid profile, antioxidant activity and histopathological changes in streptozotocin-induced diabetes in rats. Andrologia. (2020) 52:e13584. doi: 10.1111/and.13584
60. Kuang W, Zhang J, Lan Z, Deepak R, Liu C, Ma Z, et al. Slc22a14 is a mitochondrial riboflavin transporter required for sperm oxidative phosphorylation and male fertility. Cell Rep. (2021) 35:109025. doi: 10.1016/j.celrep.2021.109025
61. Simpson AM, Swan MA, White IG. Action of Phosphatidylcholine in Protecting Ram Sperm from Cold Shock. Mol Reprod Dev. (1986) 15:43–56. doi: 10.1002/mrd.1120150106
62. Zeron Y, Tomczak M, Crowe J, Arav A. The effect of liposomes on thermotropic membrane phase transitions of bovine spermatozoa and oocytes: implications for reducing chilling sensitivity. Cryobiology. (2002) 45:143–52. doi: 10.1016/S0011-2240(02)00123-2
63. Buhr MM, He L, Kasimanickam V. Lipids in extenders affect boar sperm function during cryopreservation. In: International Conference Boar Semen Preservation (2000). pp. 61–69.
64. Cerolini S, Maldjian A, Pizzi F, Gliozzi T. Changes in sperm quality and lipid composition during cryopreservation of boar semen. Theriogenology. (2005) 63:411–21. doi: 10.1016/j.theriogenology.2004.09.021
Keywords: lipidomic, buck spermatozoa, cryotolerance, phosphatidylcholine, triglyceride
Citation: Xu B, Wang R, Wang Z, Liu H, Wang Z, Zhang W, Zhang Y, Su R, Liu Z, Liu Y, Li J and Zhang J (2022) Evaluation of lipidomic change in goat sperm after cryopreservation. Front. Vet. Sci. 9:1004683. doi: 10.3389/fvets.2022.1004683
Received: 27 July 2022; Accepted: 12 September 2022;
Published: 20 October 2022.
Edited by:
Guobo Quan, Yunnan Animal Science and Veterinary Institute, ChinaReviewed by:
Larbi Allai, Université Chouaib Doukkali, MoroccoYi Fang, Northeast Institute of Geography and Agroecology (CAS), China
Copyright © 2022 Xu, Wang, Wang, Liu, Wang, Zhang, Zhang, Su, Liu, Liu, Li and Zhang. This is an open-access article distributed under the terms of the Creative Commons Attribution License (CC BY). The use, distribution or reproduction in other forums is permitted, provided the original author(s) and the copyright owner(s) are credited and that the original publication in this journal is cited, in accordance with accepted academic practice. No use, distribution or reproduction is permitted which does not comply with these terms.
*Correspondence: Jinquan Li, bGlqaW5xdWFuX25kQDEyNi5jb20=; Jiaxin Zhang, emp4Y2F1QDE2My5jb20=