- 1College of Veterinary Medicine, Henan Agricultural University, Zhengzhou, China
- 2Department of Veterinary Medicine, Centre for Infection Medicine, Institute of Microbiology and Epizootics, Freie Universität Berlin, Berlin, Germany
- 3Veterinary Centre of Resistance Research (TZR), Freie Universität Berlin, Berlin, Germany
Tigecycline and carbapenems are last-resort antimicrobial agents to treat serious infections caused by multi-drug resistant bacterial pathogens. However, the co-occurrence of tigecycline and carbapenem resistance determinants challenges the clinical efficacy of these antimicrobial agents. In this study, we report the co-existence of tet(X4), blaNDM−1 and blaOXA−58 genes in the porcine Acinetobacter towneri isolate 19110F47. Sequence analysis revealed that tet(X4) gene, along with the florfenicol resistance gene floR, was flanked by three copies of IS91-like elements, which can form three different translocatable units (TUs), and were located in a 41,098-bp multidrug resistance region (MDRR) within a novel 100,354-bp genomic island (GI) region. TUs comprising floR-virD2-ISVsa3, hp-abh-tet(X4)-ISVsa3 and virD2-floR-ISVsa3-hp-abh-tet(X4)-ISVsa3 can be looped out from the chromosomal DNA and facilitate the transfer of the TU-based resistance genes into other plasmidic or chromosomal sites. In addition, the carbapenemase genes blaNDM−1 and blaOXA−58 were found on different non-conjugative multiresistance plasmids in this isolate, with the genetic contexts ISAba125-blaNDM−1-bleMBL-tnpR and ΔISAba3-blaOXA−58-ISAba3, respectively. The simultaneous occurrence of tet(X4), blaNDM−1 and blaOXA−58 in the same porcine A. towneri isolate emphasizes the importance of antimicrobial resistance surveillance in food-producing animals.
Introduction
Antimicrobial resistance poses a significant threat to public health globally. The presence of extensively drug-resistant (XDR) Gram-negative bacteria, in particular carbapenem-resistant Enterobacteriaceae and Acinetobacter spp., compromises the efficacy of carbapenems. Moreover, the choices of effective antimicrobial agents against carbapenem-resistant bacteria are very limited (1). Tigecycline has been recognized as a last-resort antimicrobial agent to treat infections caused by XDR Gram-negative bacteria (2). However, a variety of plasmid-borne tet(X) variants genes, which confer high-level resistance to tigecycline, have been reported in Acinetobacter spp. and Enterobacteriaceae from China (3, 4). The gene tet(X4) was detected in bacteria from food-producing animals, meat for human consumption, migratory birds, humans and environmental samples (5–7).
Acinetobacter spp. are considered as ubiquitous in the nature and have emerged as a major cause of nosocomial infections globally in recent decades (8). In addition, Acinetobacter spp. are not only associated with hospital-acquired infections, but also responsible for community-acquired infections (9). XDR Acinetobacter isolates, especially when they are carbapenem-resistant, are recognized as one of the most troublesome pathogens worldwide (10). Food-producing animals have been regarded as a potential reservoir for Acinetobacter spp. in many countries (11). To date, the tet(X4) gene was mostly reported in E. coli, and sometimes described in other bacterial species, such as Acinetobacter spp. (4, 12, 13). Currently, the genetic basis for co-resistance against tigecycline and carbapenems has been investigated in Acinetobacter spp. The tigecycline resistance genes tet(X) have been reported with carbapenem resistance gene blaNDM in A. baumannii, A. indicus, A. schindleri, A. lwoffii, and other Acinetobacter (14–16).
In this study, we investigated a tigecycline- and carbapenem-resistant A. towneri isolate collected from a pig in China for the tigecycline- and carbapenem resistance genes present, their association with mobile genetic elements and their transfer potential.
Materials and methods
Sample collection and bacterial isolation
A total of 1,146 non-duplicate anal swab samples were collected from three unrelated and geographically distant pig farms and one pig slaughterhouse located in the Henan Province of China in 2019. Brain heart infusion (BHI) broth was used as transport medium for the anal swabs. The swabs were streaked on BHI agar plates supplemented with tigecycline (2 mg/L) and meropenem (2 mg/L) and incubated at 37°C for 24 h. Bacteria growing on these double-selective plates were identified to the species level by 16S rRNA amplification and sequencing of the amplicons (17).
Antimicrobial susceptibility testing
The minimal inhibitory concentrations (MICs) of the A. towneri isolate to meropenem, tigecycline, ceftazidime, florfenicol, tetracycline, colistin and gentamicin were determined and evaluated using broth microdilution according to the recommendations of the Clinical and Laboratory Standards Institute (CLSI) (18). E. coli ATCC25922 was used as quality control strain.
Whole-genome sequencing analysis
The A. towneri isolate 19110F47, was sequenced by using the PacBio RS and Illumina MiSeq platforms (Shanghai Personal Biotechnology Co., Ltd, China). The PacBio long reads were assembled with HGAP4 and CANU (Version 1.6) and corrected by the Illumina MiSeq short reads with pilon (Version 1.22). The prediction of ORFs and their annotations were performed using Glimmer 3.0. The blast software is used following the procedures at https://blast.ncbi.nlm.nih.gov.
PCR analysis
The presence of translocatable units (TUs) was detected by PCR using the primers shown in Table 1. All the PCR products were subjected to Sanger sequencing. The obtained sequences were analyzed by BLAST comparison with the NCBI database (https://blast.ncbi.nlm.nih.gov/Blast.cgi).
Transfer experiments
Conjugation assays were performed to assess the transferability of carbapenem and tigecycline resistance genes from A. towneri isolate to the azide-resistant recipient E. coli J53 and the rifampicin-resistant recipient E. coli EC600 according to a previously described method with a minor modification (1). Briefly, the donor and recipient strains were mixed at a ratio of 1:4 and incubated on LB agar for 5 h. The mixtures were collected and then plated on LB agar containing two selective markers, including azide (128 μg/mL) and meropenem (2 μg/mL), rifampicin (64 μg/mL) and meropenem (2 μg/mL), azide (128 μg/mL) and tigecycline (2 μg/mL), rifampicin (64 μg/mL) and tigecycline (2 μg/mL), respectively. The transconjugants were confirmed by PCR analysis.
Results
Identification of a carbapenem- and tigecycline-resistant A. towneri isolate
An isolate conferring resistance to both meropenem and tigecycline, designated 19110F47, was identified from swine origin in 2019. 16S rRNA sequence analysis suggested that it was assigned to the species A. towneri, which is involved in nosocomial infections as described in a previous study (16). Antimicrobial susceptibility testing results revealed that it had an expanded resistance profile, including resistances to meropenem (MIC, 16 μg/mL), tigecycline (8 μg/mL), ceftazidime (256 μg/mL), tetracycline (32 μg/mL), gentamicin (64 μg/mL) and florfenicol (128 μg/mL). However, it was classified by the CLSI breakpoints as colistin-intermediate (< 0.5 μg/mL). It is noteworthy that CLSI classifies all isolates with colistin MICs of ≤ 2 μg/mL as intermediate and does not provide a breakpoint for the category susceptible. Instead, when applying the breakpoints of the European Committee on Antimicrobial Susceptibility Testing (EUCAST) (http://www.eucast.org), this isolate would have been classified as colistin-susceptible.
tet(X4) was located in the chromosomal DNA in A. towneri and is part of TUs
The tet(X4) gene was found to be a part of a multidrug resistance region (MDRR) located on a 100,354-bp genomic island (GI) that was present in the chromosomal DNA of A. towneri 19110F47. The complete GI shared only a low query coverage (16–37%) with the sequences deposited in GenBank. It was inserted between the DUF3375 and AAA family ATPase encoding genes in the chromosomal DNA of A. towneri 19110F47. Database searches identified a distinctly smaller GI without an MDR region being present at the same location in A. towneri CIP_107472_V1 (accession number GCA_000368785.1) from an activated sludge plant in Australia. A total of 94 ORFs were identified in the GI of the present study. These ORFs encoded proteins responsible for several functions, such as gene regulation, transfer, efflux and antimicrobial resistance (Figure 1). The 41,098-bp MDRR consists of 33 ORFs [from IS26 to aph(6)-Ib] (Figure 1). Among them, a total of 12 resistance genes were identified, including tet(X4), the aminoglycoside resistance genes aadA1, aadA2b, aph(3”)-Ib, aph(3')-Ia and aph(6)-Id, the sulfonamide resistance genes sul1 and sul2, the phenicol resistance genes cmlA1 and floR, the β-lactam resistance gene blaCARB−2 and the trimethoprim resistance gene dfrA16 (Figure 1). In the close vicinity of floR and tet(X4), one truncated and two complete copies of ISVsa3, all in the same orientation, were found (Figure 1), which was similar to the corresponding region on p47EC from a porcine E. coli (MK134376).
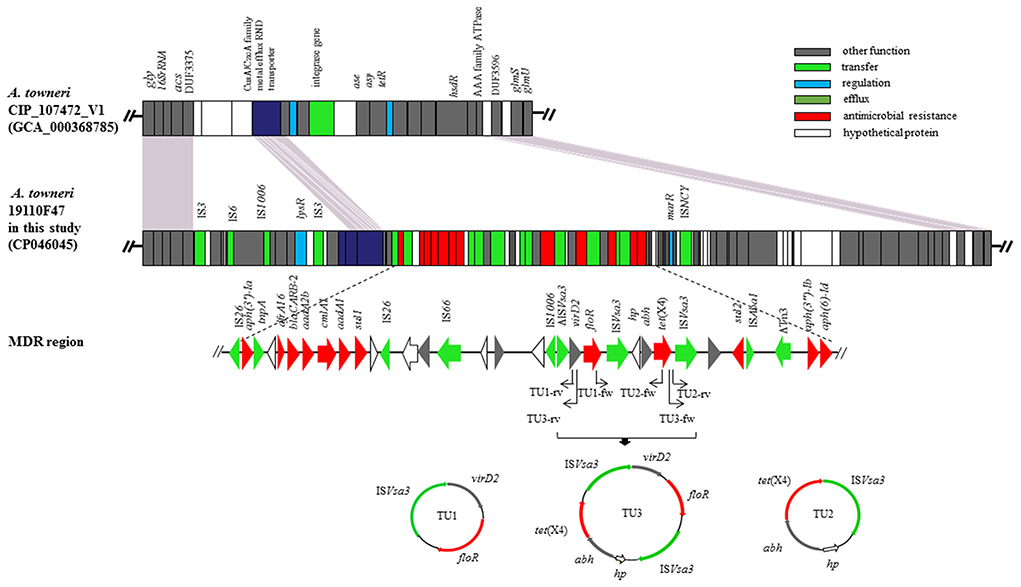
Figure 1. The genomic island carrying of the tet(X4) gene in the chromosomal DNA of A. towneri 19110F47 in this study. Genes are indicated by different colors. Δ indicates that the gene is truncated. The primers used for PCR were shown by arrows. Three TUs, including TU1 (virD2-floR-ISVsa3, 4,274 bp), TU2 [hp-abh-tet(X4)-ISVsa3, 4,608 bp] and TU3 [virD2-floR-ISVsa3-hp-abh-tet(X4)-ISVsa3, 8,882 bp] were formed.
Three PCR assays were developed to detect ISVsa3-mediated rolling-circle transposition of tet(X4) and/or floR. The results revealed that three TUs, including TU1 (virD2-floR-ISVsa3, 4,274 bp), TU2 [hp-abh-tet(X4)-ISVsa3, 4,608 bp] and TU3 [virD2-floR-ISCR2-hp-abh-tet(X4)-ISVsa3, 8,882 bp] were formed in A. towneri 19110F47 (Figure 1).
blaNDM−1 and blaOXA−58 were located on novel non-conjugative plasmids
The blaNDM−1 gene was located on a plasmid with a size of 47,094 bp, designated p19110F47-1 (Figure 2A). BLAST analysis revealed that p19110F47-1 showed similiarities with two other plasmids, pAT232 (GN014838) and pGX7 (CP071772), present in the GenBank database. However, these similarities did neither include the regions covering the antimicrobial resistance genes, nor those with the plasmid replication gene. Overall, a low query coverage (with the highest of 48%) (Supplementary Figure 1) was detected, suggesting that p19110F47-1 is a novel carbapenem resistance plasmid. Further analysis of the flanking regions of blaNDM−1 revealed that the insertion sequence ISAba125 was located upstream of blaNDM−1. Moreover, the blaNDM−1 gene was part of a region that contained also the resistance genes aac(3)-IId, aphA6 and bleMBL (Figure 2A).
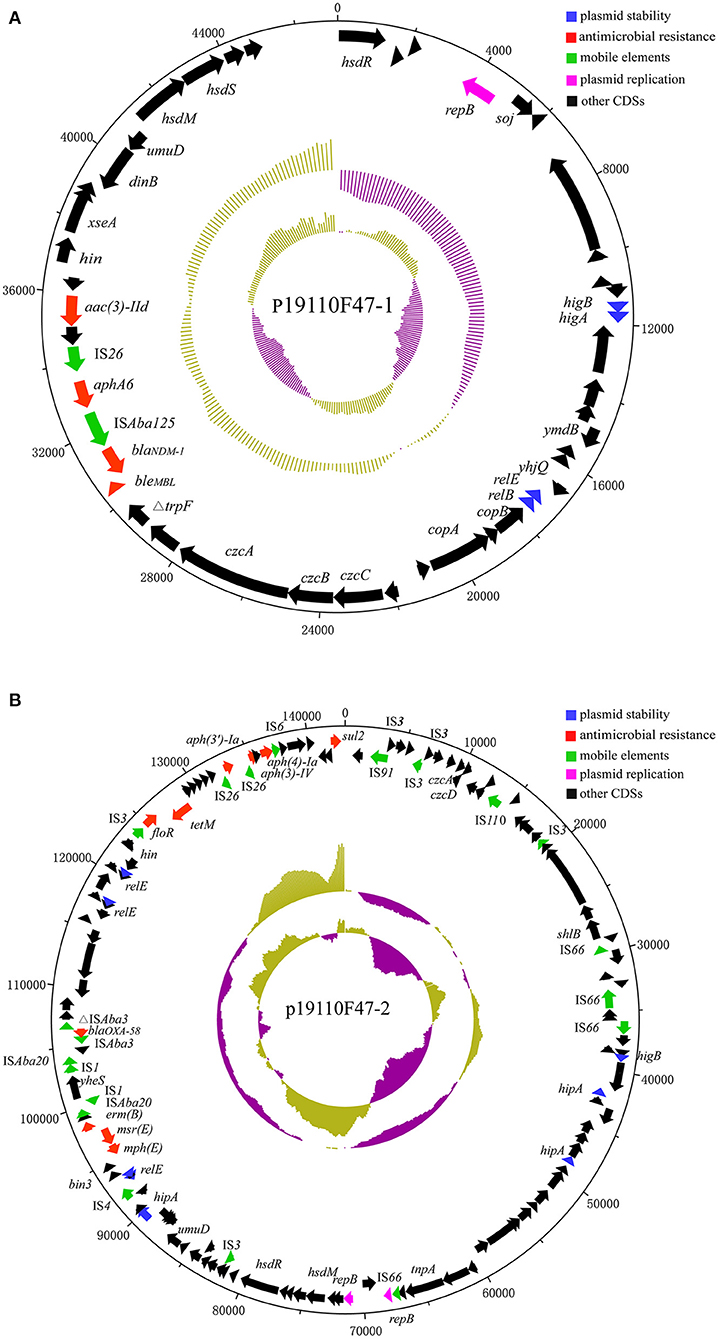
Figure 2. Structure of plasmids p19110F47-1 (A) and p19110F47-2 (B) from A. towneri 19110F47. The size scales are given in bp; genes are color-coded, depending on functional annotations: red, antimicrobial resistance; blue, plasmid stability; green, mobile elements; purple, plasmid replication; and black, other coding sequences (CDS).
The blaOXA−58-carrying plasmid p19110F47-2 was 143,035 bp in size, also carried the aminoglycoside resistance genes aac(3)-IV, aph(3')-Ia and aph(4)-Ia, floR, sul2, the tetracycline resistance gene tet(M), the macrolide-lincosamide-streptogramin B resistance gene erm(B), and the macrolide resistance genes mph(E) and msr(E) (Figure 2B). GenBank database searches identified the complete sequences of seven plasmids from Acinetobacter spp. with p19110F47-2 query coverage ranges from 40 to 87% (Supplementary Figure 2), and most of these plasmids harbored both blaOXA−58 and tet(X) orthologs (19, 20).
Conjugation experiments were performed using A. towneri 19110F47 as donor and two different E. coli strains as recipients. No transconjugants were obtained, which might be explained by the fact that the blaNDM−1-, but also the blaOXA−58-carrying plasmids lack a conjugative transfer region (Figure 2).
Discussion
Acinetobacter spp. are ubiquitous in the natural environment, and have become important opportunistic pathogens, e.g., A. towneri, A. baumannii, A. indicus, and A. lwoffii. Acinetobacter spp. strains are widely distributed in a variety of environmental sources, including water, soil, foods, and livestock (15, 16, 21). In this study, we report, the identification of an Acinetobacter spp. strain co-harboring blaNDM−1, blaOXA−58 and tet(X4) collected from food-producing animals in China. It belonged to Acinetobacter towneri, which is involved in nosocomial infections (16). Carbapenem-resistant Acinetobacter spp. is one of the most dangerous pathogens in the world (21). And Acinetobacter spp. was the major reservoir of tigecycline-resistant tet(X) genes (12). The co-location of tet(X) and carbapenem resistance gene blaNDM−1 was previously described in Acinetobacter isolates from animals and the environment (14, 22). The strain 19110F47 had an expanded resistance profile. More attention should be paid to multidrug resistant (MDR) A. towneri isolates, because they have been reported increasingly in recent years, especially in hospital sewage and from livestock (23–26).
The tet(X4) gene conferring resistance to tigecycline was found to be on the chromosome of 19110F47. The floR and tet(X4) were found to be flanked by one truncated and two complete copies of ISVsa3. ISVsa3 is 977 bp in size and represents a member of the IS91 family. This family of insertion sequences differs from others in that they transpose by rolling circle transposition (27). Members of the IS91 family can mobilize genes, including resistance genes that are located in their close vicinity. For this, only a single copy of the IS91 element—not two copies as with most other insertion sequences—is necessary (27). The tet(X4) is the part of active TUs in the chromosomal DNA, may excise from the chromosomal DNA and transfer to other plasmidic or chromosomal sites. Of three TUs identified in A. towneri 19110F47, a sequence indistinguishable from that of TU2 was also found in p47EC (MK134376) from E. coli in a previous study (3), suggesting its mobility across genus boundaries. Of note, TU3 contained both tet(X4) and floR, implying that tigecycline resistance can be co-selected by florfenicol, a veterinary antimicrobial agent commonly used in cattle, pigs, poultry and fish (28).
The blaNDM−1 located on the plasmid p19110F47-1. Insertion of blaNDM−1 together with ISAba125 have been found within the chromosomal DNA and plasmids of Acinetobacter isolates (29, 30), suggesting that ISAba125 might be involved in the dissemination of blaNDM−1 among Acinetobacter spp. Numerous studies have documented that the blaNDM−1 was frequently present adjacent to truncated or intact ISAba125 as well as in other Enterobacteriaceae, including E. coli, Klebsiella pneumoniae, Proteus mirabilis and Salmonella enterica (31–33), indicating that ISAba125 plays a vital role in the spread of blaNDM−1 among different species of bacteria.
The blaOXA−58 was located on another plasmid p19110F47-2. An analysis for the genetic environments of blaOXA−58 showed that a truncated ISAba3 element was located upstream of blaOXA−58 and a complete ISAba3 element downstream of it. The genetic context found in the present study was consistent with that in plasmid pLHC22-2-tetX-162k (CP084298) in a previous study (19). In addition, the presence of two intact ISAba3 copies in opposite orientation up-stream and down-stream of blaOXA−58 was described in a series of studies and led to the assumption that ISAba3 might play a role in the transfer of blaOXA−58 (22, 34). It has been documented that transformation of a cloned blaOXA−58 gene on a low-copy-number vector into a susceptible A. baumannii strain increased the MICs of carbapenems, but not to levels considered as resistant (35, 36). For clinical carbapenem resistance in Acinetobacter spp., other carbapenem resistance mechanisms in addition to OXA-58-like β-lactamases are required (35). In the present study, the A. towneri isolate 19110F47 showed resistance to meropenem with a MIC of 16 μg/mL. It was speculated that blaNDM−1 played a dominant role in conferring the meropenem resistance phenotype.
The tet(X4), blaNDM−1 and blaOXA−58 genes have been documented in multiple studies solely or any two of them (14, 16, 20, 22, 37). Only one study by Zheng et al. described that a tet(X6) variant and the two carbapenemase genes blaNDM−1 and blaOXA−58 were located on the same plasmid in an A. baumannii isolate of chicken origin (15). In the current study, we identified the occurrence of tet(X4), blaNDM−1 and blaOXA−58 in the same A. baumannii isolate of swine origin, with tet(X4) being located on a novel chromosomal GI and blaNDM−1 and blaOXA−58 carried by two novel plasmids.
Conclusions
In conclusion, we described the co-existence of tet(X4), blaNDM−1 and blaOXA58 in a porcine A. towneri isolate that displays resistance to carbapenems and tigecycline among numeous other antimicrobial agents. The tet(X4) is part of active translocatable units in the chromosomal DNA, facilitating its transfer into other plasmidic or chromosomal sites. The emergence of chromosomal tet(X) genes combined with plasmid-mediated carbapenem resistance genes in the same isolate, as shown in this study, will further compromise the treatment options of severe infections caused by Acinetobacter spp.
Data availability statement
The datasets presented in this study can be found in online repositories. The names of the repository/repositories and accession number(s) can be found in the article/supplementary material.
Author contributions
X-DD and HY designed the research and supervised the study. AL, WZ, RY, and CL performed the experiments and analyzed the data. AL and HY wrote the manuscript. SS and X-DD reviewed and edited the manuscript. All authors revised the manuscript and approved the final version for submission.
Funding
This work was supported by Henan Outstanding Foreign Scientist Studio (No. GZS2022010), High-end Foreign Experts Project (G2022026023L), the China Postdoctoral Science Foundation (No. 2021M690923), and the Federal Ministry of Education and Research (BMBF) under project number 01KI2009D as part of the Research Network Zoonotic Infectious Diseases.
Conflict of interest
The authors declare that the research was conducted in the absence of any commercial or financial relationships that could be construed as a potential conflict of interest.
Publisher's note
All claims expressed in this article are solely those of the authors and do not necessarily represent those of their affiliated organizations, or those of the publisher, the editors and the reviewers. Any product that may be evaluated in this article, or claim that may be made by its manufacturer, is not guaranteed or endorsed by the publisher.
Supplementary material
The Supplementary Material for this article can be found online at: https://www.frontiersin.org/articles/10.3389/fvets.2022.1002149/full#supplementary-material
Supplementary Figure 1. Structure analysis of blaNDM−1-bearing plasmid. Comparison analysis of the plasmid p19110F47-1 with other similar plasmids submitted in GenBank database.
Supplementary Figure 2. Structure analysis of blaOXA−58-bearing plasmid. Comparison analysis of the plasmid p19110F47-2 with other similar plasmids submitted in GenBank database.
References
1. Borgia S, Lastovetska O, Richardson D, Eshaghi A, Xiong J, Chung C, et al. Outbreak of carbapenem-resistant Enterobacteriaceae containing blaNDM-1, Ontario, Canada. Clin Infect Dis. (2012) 55:e109–17. doi: 10.1093/cid/cis737
2. Seifert H, Blondeau J, Dowzicky MJ. In vitro activity of tigecycline and comparators (2014-2016) among key WHO 'priority pathogens' and longitudinal assessment (2004-2016) of antimicrobial resistance: a report from the T.E.S.T. study Int J Antimicrob Agents. (2018) 52:474–84. doi: 10.1016/j.ijantimicag.2018.07.003
3. He T, Wang R, Liu D, Walsh TR, Zhang R, Lv Y, et al. Emergence of plasmid-mediated high-level tigecycline resistance genes in animals and humans. Nat Microbiol. (2019) 4:1450–6. doi: 10.1038/s41564-019-0445-2
4. Sun J, Chen C, Cui CY, Zhang Y, Liu X, Cui ZH, et al. Plasmid-encoded tet(X) genes that confer high-level tigecycline resistance in Escherichia coli. Nat Microbiol. (2019) 4:1457–64. doi: 10.1038/s41564-019-0496-4
5. Sun C, Cui M, Zhang S, Wang H, Song L, Zhang C, et al. Plasmid-mediated tigecycline-resistant gene tet(X4) in Escherichia coli from food-producing animals, China, 2008-2018. Emerg Microbes Infect. (2019) 8:1524–7. doi: 10.1080/22221751.2019.1678367
6. Mohsin M, Hassan B, Martins W, Li R, Abdullah S, Sands K, et al. Emergence of plasmid-mediated tigecycline resistance tet(X4) gene in Escherichia coli isolated from poultry, food and the environment in South Asia. Sci Total Environ. (2021) 787:147613. doi: 10.1016/j.scitotenv.2021.147613
7. Chen C, Cui CY, Zhang Y, He Q, Wu XT Li G, et al. Emergence of mobile tigecycline resistance mechanism in Escherichia coli strains from migratory birds in China. Emerg Microbes Infect. (2019) 8:1219–22. doi: 10.1080/22221751.2019.1653795
8. Dijkshoorn L, Nemec A, Seifert H. An increasing threat in hospitals: multidrug-resistant Acinetobacter baumannii. Nat Rev Microbiol. (2007) 5:939–51. doi: 10.1038/nrmicro1789
9. Chen CT, Wang YC, Kuo SC, Shih FH, Chen TL, How CK, et al. Community-acquired bloodstream infections caused by Acinetobacter baumannii: a matched case-control study. J Microbiol Immunol Infect. (2018) 51:629–35. doi: 10.1016/j.jmii.2017.02.004
10. Wong D, Nielsen TB, Bonomo RA, Pantapalangkoor P, Luna B, Spellberg B. Clinical and pathophysiological overview of Acinetobacter infections: a century of challenges. Clin Microbiol Rev. (2017) 30:409–47. doi: 10.1128/cmr.00058-16
11. Carvalheira A, Silva J, Teixeira P. Acinetobacter spp. in food and drinking water - A review. Food Microbiol. (2021) 95:103675. doi: 10.1016/j.fm.2020.103675
12. Chen C, Cui CY Yu JJ, He Q, Wu XT, He YZ, Cui ZH Li C, et al. Genetic diversity and characteristics of high-level tigecycline resistance tet(X) in Acinetobacter species. Genome Med. (2020) 12:111. doi: 10.1186/s13073-020-00807-5
13. Zhang R, Dong N, Zeng Y, Shen Z, Lu J, Liu C, et al. Chromosomal and plasmid-borne tigecycline resistance genes tet(X3) and tet(X4) in Dairy Cows on a chinese farm. Antimicrob Agents Chemother. (2020) 64:e00674–20. doi: 10.1128/aac.00674-20
14. He T, Li R, Wei R, Liu D, Bai L, Zhang L, et al. Characterization of Acinetobacter indicus co-harbouring tet(X3) and blaNDM-1 of dairy cow origin. J Antimicrob Chemother. (2020) 75:2693–6. doi: 10.1093/jac/dkaa182
15. Zheng XR, Zhu JH, Zhang J, Cai P, Sun YH, Chang MX, et al. novel plasmid-borne tet(X6) variant co-existing with blaNDM-1 and blaOXA-58 in a chicken Acinetobacter baumannii isolate. J Antimicrob Chemother. (2020) 75:3397–9. doi: 10.1093/jac/dkaa342
16. Cui CY, Chen C, Liu BT, He Q, Wu XT, Sun RY, et al. Co-occurrence of plasmid-mediated tigecycline and carbapenem resistance in Acinetobacter spp. from waterfowls and their neighboring environment. Antimicrob Agents Chemother. (2020) 64:e02502–19. doi: 10.1128/aac.02502-19
17. Kim TW, Kim YH, Kim SE, Lee JH, Park CS, Kim HY. Identification and distribution of Bacillus species in doenjang by whole-cell protein patterns and 16S rRNA gene sequence analysis. J Microbiol Biotechnol. (2010) 20:1210–4. doi: 10.4014/jmb.1002.02008
18. Clinical and Laboratory Standards Institute (CLSI). Performance Standards for Sntimicrobial Susceptibility Testing. 32nd ed. Wayne, PA: CLSI supplement M100 (2022).
19. Li Y, Peng K, Yin Y, Sun X, Zhang W, Li R, et al. Occurrence and Molecular Characterization of abundant tet(X) variants among diverse bacterial species of chicken origin in Jiangsu, China. Front Microbiol. (2021) 12:751006. doi: 10.3389/fmicb.2021.751006
20. Ma J, Wang J, Feng J, Liu Y, Yang B, Li R, et al. Characterization of three porcine Acinetobacter towneri strains co-harboring tet(X3) and blaOXA-58. Front Cell Infect Microbiol. (2020) 10:586507. doi: 10.3389/fcimb.2020.586507
21. Asif M, Alvi IA, Rehman SU. Insight into Acinetobacter baumannii: pathogenesis, global resistance, mechanisms of resistance, treatment options, and alternative modalities. Infect Drug Resist. (2018) 11:1249–60. doi: 10.2147/idr.S166750
22. Wang J, Wang Y, Wu H, Wang ZY, Shen PC, Tian YQ, et al. Coexistence of bla (OXA-58) and tet(X) on a novel plasmid in Acinetobacter sp. from pig in Shanghai, China. Front Microbiol. (2020) 11:578020. doi: 10.3389/fmicb.2020.578020
23. Cheng Y, Chen Y, Liu Y, Song J, Chen Y, Shan T, et al. Detection of a new tet(X6)-encoding plasmid in Acinetobacter towneri. J Glob Antimicrob Resist. (2021) 25:132–6. doi: 10.1016/j.jgar.2021.03.004
24. Jiang N, Zhang X, Zhou Y, Zhang Z, Zheng X. Whole-genome sequencing of an NDM-1- and OXA-58-producing Acinetobacter towneri isolate from hospital sewage in Sichuan Province, China. J Glob Antimicrob Resist. (2019) 16:4–5. doi: 10.1016/j.jgar.2018.11.015
25. Li Y, Qiu Y, Fang C, Dai X, Zhang L. Coexistence of blaOXA-58 and blaNDM-1 on a novel plasmid of GR59 from an Acinetobacter towneri isolate. Antimicrob Agents Chemother. (2022) 12:e0020622. doi: 10.1128/aac.00206-22
26. Wang K, Li P, Li J, Hu X, Lin Y, Yang L, et al. An NDM-1-producing Acinetobacter towneri isolate from hospital sewage in China. Infect Drug Resist. (2020) 13:1105–10. doi: 10.2147/idr.S246697
27. Toleman MA, Bennett PM, Walsh TR, ISCR. elements: novel gene-capturing systems of the 21st century? Microbiol Mol Biol Rev. (2006) 70:296–316. doi: 10.1128/mmbr.00048-05
28. Li B, Zhang Y, Wei J, Shao D, Liu K, Shi Y, et al. Characterization of a novel small plasmid carrying the florfenicol resistance gene floR in Haemophilus parasuis. J Antimicrob Chemother. (2015) 70:3159–61. doi: 10.1093/jac/dkv230
29. Jones LS, Carvalho MJ, Toleman MA, White PL, Connor TR, Mushtaq A, et al. Characterization of plasmids in extensively drug-resistant acinetobacter strains isolated in India and Pakistan. Antimicrob Agents Chemother. (2015) 59:923–9. doi: 10.1128/aac.03242-14
30. Zhu Y, Liu W, Schwarz S, Wang C, Yang Q, Luan T, et al. Characterization of a blaNDM-1-carrying IncHI5 plasmid from Enterobacter cloacae complex of food-producing animal origin. J Antimicrob Chemother. (2020) 75:1140–5. doi: 10.1093/jac/dkaa010
31. Li R, Xie M, Liu L, Huang Y, Wu X, Wang Z, et al. Characterisation of a cointegrate plasmid harbouring blaNDM-1 in a clinical Salmonella Lomita strain. Int J Antimicrob Agents. (2020) 55:105817. doi: 10.1016/j.ijantimicag.2019.09.021
32. Wang Y, Zhang R, Li J, Wu Z, Yin W, Schwarz S, et al. Comprehensive resistome analysis reveals the prevalence of NDM and MCR-1 in Chinese poultry production. Nat Microbiol. (2017) 2:16260. doi: 10.1038/nmicrobiol.2016.260
33. Wu W, Feng Y, Tang G, Qiao F, McNally A, Zong Z, et al. metallo-β-lactamases and their bacterial producers in health care settings. Clin Microbiol Rev. (2019) 32:e00115–18. doi: 10.1128/cmr.00115-18
34. Chen Y, Guo P, Huang H, Huang Y, Wu Z, Liao K. Detection of co-harboring OXA-58 and NDM-1 carbapenemase producing genes resided on a same plasmid from an Acinetobacter pittii clinical isolate in China. Iran J Basic Med Sci. (2019) 22:106–11. doi: 10.22038/ijbms.2018.28934.6994
35. Evans BA, Amyes SG, OXA. β-lactamases. Clin Microbiol Rev. (2014) 27:241–63. doi: 10.1128/cmr.00117-13
36. Héritier C, Poirel L, Lambert T, Nordmann P. Contribution of acquired carbapenem-hydrolyzing oxacillinases to carbapenem resistance in Acinetobacter baumannii. Antimicrob Agents Chemother. (2005) 49:3198–202. doi: 10.1128/aac.49.8.3198-3202.2005
Keywords: tigecycline, carbapenem, resistance, tet(X4), blaNDM−1, blaOXA−58, Acinetobacter towneri
Citation: Li A, Yu R, Zhao W, Schwarz S, Li C, Yao H and Du X-D (2022) Characterization of a genomic Island carrying the tet(X4) gene in porcine Acinetobacter towneri co-harboring plasmid-borne blaNDM−1 and blaOXA−58 genes. Front. Vet. Sci. 9:1002149. doi: 10.3389/fvets.2022.1002149
Received: 24 July 2022; Accepted: 12 September 2022;
Published: 29 September 2022.
Edited by:
Patrick Rik Butaye, Ghent University, BelgiumReviewed by:
Chengming Wang, Auburn University, United StatesChong Chen, Yangzhou University, China
Xinglong Wang, Northwest University, China
Copyright © 2022 Li, Yu, Zhao, Schwarz, Li, Yao and Du. This is an open-access article distributed under the terms of the Creative Commons Attribution License (CC BY). The use, distribution or reproduction in other forums is permitted, provided the original author(s) and the copyright owner(s) are credited and that the original publication in this journal is cited, in accordance with accepted academic practice. No use, distribution or reproduction is permitted which does not comply with these terms.
*Correspondence: Hong Yao, eWFvaDA5MTNAaGVuYXUuZWR1LmNu; Xiang-Dang Du, eGRkdUBoZW5hdS5lZHUuY24=