- Department of Reproductive Immunology and Pathology, Institute of Animal Reproduction and Food Research, Polish Academy of Sciences, Olsztyn, Poland
We investigated the effects of different doses of dinoprost injected directly into the bovine corpus luteum (CL) on (i) concentrations of progesterone (P4) and oxytocin (OT) in peripheral blood and (ii) mRNA levels of steroidogenic acute regulatory protein (STAR), cytochrome P450 family 11 subfamily A member 1 (P450scc), hydroxy-delta-5-steroid dehydrogenase, 3 β- and steroid delta-isomerase 1 (HSD3B), and receptor-interacting protein kinases 1 and 3 (RIPK1, RIPK3) in CL tissue. Moreover, we examined the effects of dinoprost, injected intra-CL or administered intramuscularly (IM), on CL secretory function and on indicators of CL vascular network status: luteal tissue area (LTA), CL blood flow (CLBF), and the CLBF:LTA ratio (Adj. CLBF), in cows at the early and mid-luteal phases. In the Experiment 1, cows (day 10 of the cycle) were allocated to (i) an intra-CL injection of saline (control; n = 3); (ii) an intra-CL injection of dinoprost (1.25 mg; 2.5 mg, or 5 mg; n = 3 for each dose); (iii) an IM administration of saline (control; n = 3); or (iv) an IM administration of dinoprost (25 mg; positive control; n = 3). Concentrations of OT and P4 were measured in plasma samples. The mRNA expression of steroidogenesis- or necroptosis-related factors was determined in CL tissue 4 h after treatments. In Experiment 2, cows on day 4 (n = 12) or day 10 (n = 12) were allocated to (i) an intra-CL injection of dinoprost (2.5 mg/0.5 ml; n = 6), or (ii) IM administration of dinoprost (25 mg/5 ml; n = 6). Concentrations of P4 were measured in plasma samples. Luteal tissue area, CLBF, and Adj. CLBF were assessed based on color Doppler ultrasonography. An intra-CL injection of dinoprost increased OT and decreased P4 levels in the jugular vein (JV) in a dose-dependent manner in cows at the mid-luteal phase. Increased CLBF and Adj. CLBF, accompanied by reduced P4 levels, were observed 2 h after intra-CL dinoprost injection in middle-stage CL. Decreased STAR and increased RIPK1 and RIPK3 mRNA levels confirmed that 2.5 mg dinoprost injected directly into CL is the minimum dose that induces luteolytic cascade. Injection of dinoprost directly into the CL (at a dosage lower than recommended for peripheral application) results in a pattern similar to IM dinoprost administration.
Introduction
The corpus luteum (CL) plays the main role in regulation of the ovarian cycle and maintenance of pregnancy in mammalian species, because of progesterone (P4) production (1, 2). Many luteotrophic and luteolytic factors are involved in the life span of CL (1, 3–7). Development of the bovine CL is associated with intensive angiogenesis, which is crucial for its steroidogenic activity (8–10). Corpus luteum regression, on the other hand, consists of interruption of steroidogenesis (functional luteolysis) and demise of CL tissue due to cell death (structural luteolysis) (11, 12). Although apoptosis had been considered the most potent mechanism of cell death during luteolysis, a caspase-independent cell death pathway, necroptosis, was found recently to represent an alternative CL regression process in cows (13, 14).
Prostaglandin (PG) F2α exerts stage-specific actions on bovine CL development, maintenance, and regression (15–18). During early luteal phase, PGF2α may act as a luteo-supporting factor (18–20) and play a luteoprotective role (21–23). Uterine PGF2α is necessary to induce luteolysis on days 17–18 of the estrous cycle in cattle, and initiates a new reproductive cycle (1, 24–27). Additionally, CL regression can be pharmacologically induced by the administration of exogenous PGF2α in the middle stage of the estrous cycle, when CL is sensitive to PGF2α (24, 28). During the early luteal phase, bovine CL is resistant to the luteolytic action of PGF2α until day 5 of the estrous cycle (28–30).
Blood flow is a key regulatory component of CL function in cows (31). One method used to monitor luteal function during the estrous cycle of dairy cows is color Doppler sonography (32, 33). Changes in blood flow and P4 secretion in response to exogenous PGF2α were previously examined in both early- and middle-stage CL (32, 34). On one hand, prior to the luteolytic cascade, PGF2α increases luteal blood flow in cows (29). On the other hand, the lack of change in blood flow in the early-stage CL seems to be directly correlated with the resistance of CL to PGF2α action during this period of the estrous cycle (31, 32).
The response of luteal steroidogenic cells may depend on local, direct effects of PGF2α (autocrine/paracrine modes of action), or on indirect effects, including several regulatory mechanisms within the female reproductive tract (e.g., endocrine action, blood flow regulation, the contribution of the immune system, etc.) (35–37). Moreover, our previous study has demonstrated that intra-CL vs. intramuscular (IM) administration of PGF2α differentially affects P4 secretion, modulating the main genes involved in angiogenic, steroidogenic, and cell death pathways in bovine CL (22, 23).
Prostaglandin F2α analogs (e.g., dinoprost, cloprostenol, luprostiol, oestrophan) are well known as luteolytic drugs and are widely used in many synchronization protocols and manipulations of the estrous cycle in cows (38–41). Until now, IM has been the main route of administration used in veterinary practice. It was previously suggested that action of PGF2α analogs on function of the middle-stage CL is dose-dependent (42). Therefore, in the present study, effects of different doses of dinoprost on bovine CL functions were examined at the mid-luteal phase. To the best of our knowledge, the effects of an intra-CL PGF2α analog (dinoprost) on CL function as well as on luteal blood flow during the estrous cycle remain poorly understood. Furthermore, the intra-CL vs. peripheral actions of dinoprost on P4 output and local blood flow in bovine CL may depend on the phase of the estrous cycle. Therefore, the objective of the present study was to investigate the effects of different doses of dinoprost injected directly into bovine middle-stage CL on (i) concentrations of P4 and oxytocin (OT) in peripheral blood plasma samples, and (ii) mRNA levels of steroidogenic acute regulatory protein (STAR), cytochrome P450 family 11 subfamily A member 1 (P450scc), hydroxy-delta-5-steroid dehydrogenase, 3 β- and steroid delta-isomerase 1 (HSD3B) and receptor-interacting protein kinases 1 and 3 (RIPK1, RIPK3) in CL tissue. Moreover, we examined the effect of dinoprost either injected directly into CL or administered IM on CL secretory function and on indicators of the CL vascular network luteal tissue area (LTA), CL blood flow (CLBF), and the CLBF:LTA ratio (Adj. CLBF), in cows at the early (PGF2α-resistant) and mid-luteal (PGF2α-responsive) phases of the estrous cycle.
Materials and Methods
Animals and Surgical Procedures
For the present study, 42 healthy, cycling Polish Holstein-Friesian cows from a local commercial dairy farm (Smietki, Poland) were used. The herd calves all year and is monitored by trained veterinary and nutrition consultants. The history of the cows and the structure of the farms were investigated by a questionnaire for the owners. Written owner consent was obtained through the farm manager. The experiment was performed in a group of non-pregnant cows (650 ± 86 kg; 3–5 lactations; ages 5–7 years) that were considered for culling because of their low milk production. A tie-up system was use to contain the experimental cows. The animals were milked two times daily, and fed maize/grass-based full total mix ratio (TMR). Cows had ad libitum access to water and a salt-based mineral supplement. The absence of reproductive disorders was determined and general clinical examinations were performed in cows enrolled in the experiment. The estrous cycle was synchronized in all experimental cows by two IM injections of PGF2α analog (dinoprost, 25 mg/5 ml; Dinolytic; Zoetis, Poland) over an 11-day interval, as reported previously (38). Follicular development and morphological changes of the CL during the estrous cycle were monitored with transrectal ultrasonographic visualization, and confirmed by observing visual signs of estrus (e.g., standing heat behavior and vaginal mucus discharge). The onset of estrus was taken as day 0 of the estrous cycle. Additionally, the stage of the estrous cycle was confirmed by radioimmunoassay (RIA) of P4 concentrations in blood plasma samples collected from the coccygeal vessels. The concentration of P4 in samples collected on day 0 of the estrous cycle was 0.41 ± 0.12 ng/ml (mean ± SEM).
Polyvinyl Catheter Insertion
The animals were pre-medicated with xylazine (25–30 mg/animal; Xylavet 2%, ScanVet, Poland) followed by insertion of a polyvinyl catheter (outside diameter = 2.1 mm; inside diameter = 1.6 mm Tomel Sp, Poland) into the jugular vein (JV) to permit frequent blood sample collection, as described previously by Skarzynski et al. (43).
Intra-CL Injection
Each cow was treated with 4 ml of 2% procaine hydrochloride (Polocainum Hydrochloricum; Biowet Drwalew, Poland) between the first and second coccygeal vertebrae for local epidural anesthesia. Intra-CL injections were administered under ultrasound guidance through a sterile needle 1.25 × 50 mm (18 G × 2″). The transducer and needle guide were coated with a sterile lubricant (Medicum, Poland) and positioned within the vagina. The ovary bearing the CL was positioned rectally to visualize it. The needle was then passed through the vaginal wall, and the injection of dinoprost or sterile saline was made directly into the CL. The intra-CL injection model was validated and described by Jonczyk et al. (22).
Ovary Collection
Ovaries with CL were collected by colpotomy using a Hauptner's effeminator (Hauptner & Herberholz GmbH & Co. KG, Solingen, Germany), as described previously by Piotrowska et al. (44).
Design of in vivo Experiments
Experiment 1
Figure 1 shows the in vivo Experiment 1 study design. For frequent blood collection, a polyvinyl catheter was inserted into the JV of cows (n = 18) on day 9 of the estrous cycle, as described above. On day 10 of the estrous cycle, animals were randomly allocated to (i) an intra-CL injection of sterile saline (control; n = 3), (ii) an intra-CL injection of three different doses of dinoprost (1.25 mg/0.5 ml; 2.5 mg/0.5 ml, and 5 mg/0.5 ml; n = 3 per dose), (iii) an IM administration of sterile saline (control; n = 3), or (iv) an IM administration of dinoprost (25 mg/5 ml; positive control; n = 3). The time point of dinoprost or saline injection was defined as hour 0 of the experiment.
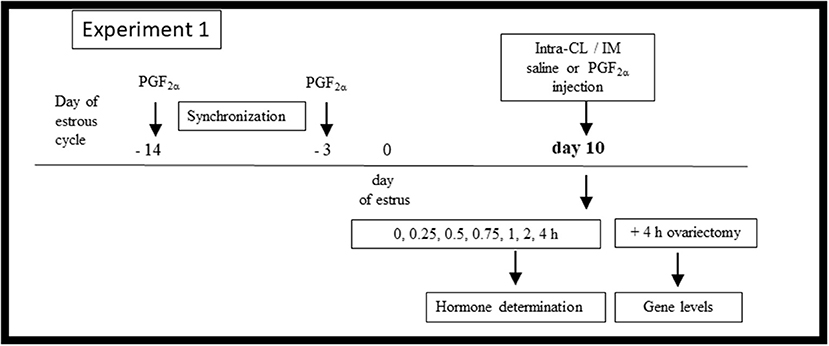
Figure 1. Schematic diagram of the Experiment 1 in vivo study design. Cows were synchronized via two injections of prostaglandin (PG) F2α administered over an 11-day interval, starting on day −14 of the estrous cycle, with the onset of estrus considered day 0. On day 10 of the estrous cycle, the cows (n = 18) were allocated to (i) an intra-CL injection of sterile saline (control; n = 3), (ii) an intra-CL injection of three different doses of dinoprost (1.25 mg/0.5 ml, 2.5 mg/0.5 ml, and 5 mg/0.5 ml; n = 3 per dose), (iii) an IM administration of sterile saline (control; n = 3), (iv) an IM administration of dinoprost (25 mg/5 ml; positive control; n = 3). After treatment (time 0 h), blood plasma was collected over a 4-h period for hormone determination. At 4 h after treatment, the cows were ovariectomized. CLs were collected for evaluation of mRNA levels of specific genes.
Dose-Dependent Effects of Intra-CL Injection of Dinoprost on Oxytocin and Progesterone Concentrations in the Jugular Vein of Cows in the Mid-Luteal Phase of the Estrous Cycle
Blood samples were collected from the JV of cows at 0, 0.25, 0.5, 0.75, 1, 2, and 4 h for determination of OT and P4 concentrations. Blood was collected into sterile 10-ml spray-coated K2EDTA tubes (BD Vacutainer, BD Diagnostic, Warsaw, Poland). After centrifugation (1,500 × g, 15 min at 4°C), the plasma was stored at −20°C until hormone concentrations were analyzed.
Dose-Dependent Effect of Intra-CL Injection of Dinoprost on mRNA Levels of Steroidogenesis- and Necroptosis-Related Factors in the Middle-Stage CL
The CL was collected by ovariectomy 4 h after treatments, to examine mRNA expression of steroidogenesis- or necroptosis-related factors in CL tissues. Each CL tissue was immediately placed into a 1.5 ml microcentrifuge tube containing 1 ml RNALater (#R0901, Sigma-Aldrich, Germany), homogenized, and stored at −80°C until quantitative real-time polymerase chain reaction (RT-qPCR) analysis was carried out.
Experiment 2
Effects of Intra-CL Injection of Dinoprost on Progesterone Concentration and Blood Flow in Cows at the Early and Mid-Luteal Phases of the Estrous Cycle, Compared to IM Dinoprost Administration
Figure 2 shows the in vivo Experiment 2 study design. Cows (n = 24) were separated into two cohorts according to the phase of the estrous cycle: group I (early luteal phase; n = 12) and group II (mid-luteal phase; n = 12). A polyvinyl catheter was inserted into the JV for frequent blood collection on day 3 of the estrous cycle (group I) or on day 9 (group II), as described above. Afterwards, cows on day 4 (group I) or on day 10 (group II) were randomly allocated to (i) intra-CL injection of dinoprost (2.5 mg/0.5 ml; n = 6) or (ii) IM injection of dinoprost (25 mg/5 ml; n = 6).
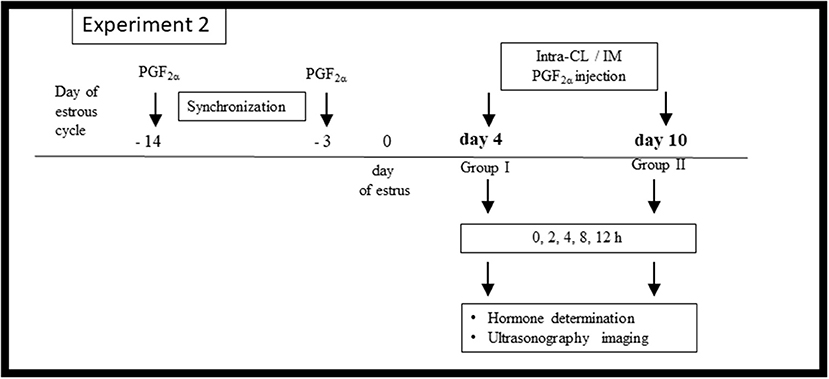
Figure 2. Schematic diagram of the Experiment 2 in vivo study design. Cows were synchronized via two injections of prostaglandin (PG) F2α administered over an 11-day interval, starting on protocol day −14 of the estrous cycle, with the onset of estrus considered day 0. On day 4 (n = 12) or day 10 (n = 12) of the estrous cycle, the cows were allocated to (i) an intra-CL injection of dinoprost (2.5 mg/0.5 ml; n = 6 at each time point), (ii) IM administration of dinoprost (25 mg/5 ml; n = 6 at each time point). After treatment (time 0 h), blood plasma was collected over a 12-h period for hormone determination, and ultrasonographic imaging was performed.
Blood samples were collected from the JV 0, 2, 4, 8, and 12 h after dinoprost injection, for determination of P4 concentrations.
Bovine ovaries with active CL were examined 0, 2, 4, 8, and 12 h after dinoprost injection, by transrectal ultrasonography using an ultrasound scanner (MyLab 30VET Gold Color Doppler Diagnostic Ultrasound System, ESOATE Pie Medica, Genoa, Italy) equipped with a 7.5 MHz convex transducer. Ultrasonographic examination was carried out for blood flow mapping. Imaging settings (gain, depth, frequency, power, focus position, and frame rate) were standardized and remained unchanged for the duration of the experiment. Color-flow Doppler mapping modes were used to generate short video clips in which blood flow was detectable within the CL. Then videos were stored in the internal memory of the ultrasound machine for further computer-assisted image analyses.
Hormone Determination
The OT concentrations in blood plasma were measured in duplicate via ELISA, as reported earlier by Skarzynski and Okuda (19), and using ice-chilled acetone for extraction (45). Non-commercial anti-OT serum was characterized previously (46), and used at a final dilution of 1:70,000. The standard curve ranged from 7.8 to 2,000 pg/ml. The intra- and inter-assay coefficients of variation (CV) were 9.5 and 11.3%, respectively.
The P4 concentration in blood plasma was measured in duplicate via direct RIA (P4 125IRIA kit IM1188, Immunotech, Prague, Czech Republic) according to the manufacturer's instructions. The standard curve ranged from 0.1 to 100 ng/ml, and the effective dose for 50% inhibition (ED50) was 0.05 ng/ml. The intra- and inter-assay CVs were 6.2 and 8.8%, respectively.
Computer-Assisted Image Analyses
Analyses of ultrasonographic images were adapted from Siqueira et al. (47). Briefly, LTA, CLBF, and Adj. CLBF were obtained from CL image characteristics. Areas of regions with CL or of the cavity (if present) were calculated, and the area of the cavity was subtracted from the CL area. For the quantitative assessment of vascularization of the CL, the area of colored pixels within the CL was determined, for indirect estimation of CLBF. An Adj. CLBF was calculated by dividing CLBF by LTA (ratio CLBF: LTA). All computer-assisted image analyses were performed using ZEN 2.6 (Carl-Zeiss, Germany), supported by ImageJ software.
Quantitative Real-Time PCR
Total RNA was extracted from bovine CL tissues (40 ± 5 mg) using the Total RNA Mini Kit (#031-100, A&A Biotechnology, Gdansk, Poland) according to the manufacturer's instructions. The amount and purity of RNA were assessed using a NanoDrop 1000 (Thermo Fisher Scientific, ND-1000, Wilmington, DE, USA). The 260/280 nm absorbance ratio for all samples was approximately 2.0, and the 260/230 nm absorbance ratio ranged between 1.8 and 2.2. Then, 1 μg of each sample of total RNA was reverse-transcribed into cDNA using a QuantiTect Reverse Transcription Kit (#205311, Qiagen, Düsseldorf, Germany) according to the manufacturer's instructions.
Quantitative real-time PCR (RT-qPCR) assays were performed in an ABI 7900 HT sequence detection system using SYBR Green PCR master mix (Applied Biosystems, Foster City, CA, USA). The sequences for bovine glyceraldehyde-3-phosphate dehydrogenase (GAPDH), β-actin (ACTB), 18S ribosomal RNA (RN18S1), STAR, P450scc, HSD3B, RIPK1, and RIPK3 were reported previously by Jonczyk et al. (22, 23). All primers were synthesized by Sigma-Aldrich (Custom Oligos Sigma-Aldrich, Haverhill, United Kingdom). Reference gene stability was determined using the NormFinder software program (MOMA, Aarhus University Hospital, Denmark), as previously described by Andersen et al. (48). Gene expression data are expressed relative to the best combination of two housekeeping genes (ACTB/RN18S1), and are presented as arbitrary units. The RT-qPCR results were analyzed using the method described by Zhao and Fernald (49). The total reaction volume was 10 μl, and contained 3 μl cDNA (10 ng), 1 μl each of forward and reverse primers (250 nM), and 5 μl SYBR Green PCR master mix (Applied Biosystems, Foster City, California, USA, #4309155). The RT-qPCR protocol was carried out as follows: initial denaturation (10 min at 95°C), followed by 45 cycles of denaturation (15 s at 95°C) and annealing (1 min at 60°C). After PCR amplification, melting curves were obtained by stepwise increases in temperature from 60 to 95°C, to ensure single product amplification. Control reactions without template or without primers were performed to confirm that products were free of primer dimers and genomic DNA contamination, respectively.
Statistical Analysis
All experimental data are shown as means ± standard errors of the mean (SEM). The statistical analyses of the results of mRNA levels were performed using a non-parametric one-way ANOVA Kruskal-Wallis test followed by Dunnett's multiple comparison test (GraphPad Prism ver. 8.3.0; Graph Pad Software, San Diego, CA). The differences in P4 and OT concentrations in the venous blood plasma samples and analyses of indicators of vascular network status of the CL (LTA, CLBF, and Adj. CLBF) were analyzed using a repeated measures approach, in which treatments and time of sample collection (h) were treated as fixed effects, and all interactions were included (Supplementary Tables 1–3, two-way ANOVA test followed by the Sidak multiple comparison test; ANOVA; GraphPad Prism), as described previously (43).
Results
Experiment 1
Dose-Dependent Effects of Intra-CL Injection of Dinoprost on JV Oxytocin and Progesterone Concentrations in Cows in the Mid-Luteal Phase of the Estrous Cycle
Table 1 shows changes in OT concentrations in the peripheral blood plasma of cows after intra-CL injection of dinoprost at different doses, or IM administration (positive control) of dinoprost or saline solution, in the mid-luteal phase of the estrous cycle. Intra-CL injection of 1.25 mg dinoprost increased OT concentrations between 0.25 and 0.5 h after its injection, compared to the control group (P < 0.05; Table 1). After intra-CL injection of dinoprost at doses of 2.5 and 5 mg, increased OT concentrations were observed between 0.25 and 0.75 h after injection, compared to the control groups (P < 0.05; Table 1). In comparison, IM administration of 25 mg dinoprost enhanced OT concentrations between 0.25 and 0.75 h after treatment, compared to the control group (P < 0.05; Table 1).
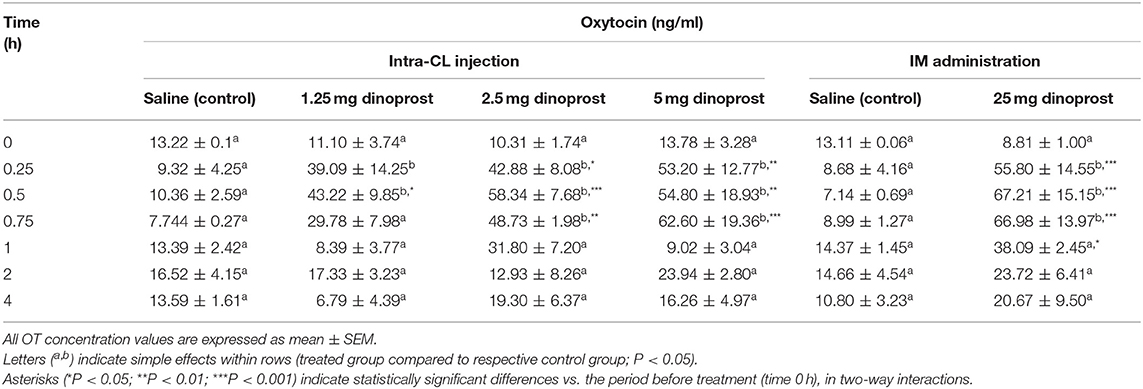
Table 1. Effect of intra-CL injection or IM administration (positive control) of dinoprost or saline solution on oxytocin (OT) concentrations in blood plasma of cows (n = 3 per dose), on day 10 of the estrous cycle.
Table 2 shows changes in P4 concentrations in the peripheral blood plasma of cows after intra-CL injection at different doses, or IM administration (positive control) of dinoprost or saline solution, in the mid-luteal phase of the estrous cycle. Intra-CL injection of 2.5 mg dinoprost decreased P4 concentrations at 0.5 h and between 1 and 4 h after its injection, compared to the control group (P < 0.05; Table 2). Intra-CL injection of 5 mg dinoprost increased P4 concentrations at 0.25 h, then decreased its concentrations between 0.5 and 0.75 h and 2 and 4 h, respectively (P < 0.05; Table 2). An increase in P4 concentration was observed between 0.25 and 0.5 h, with a decline at 4 h after IM administration of dinoprost (P < 0.05; Table 2).
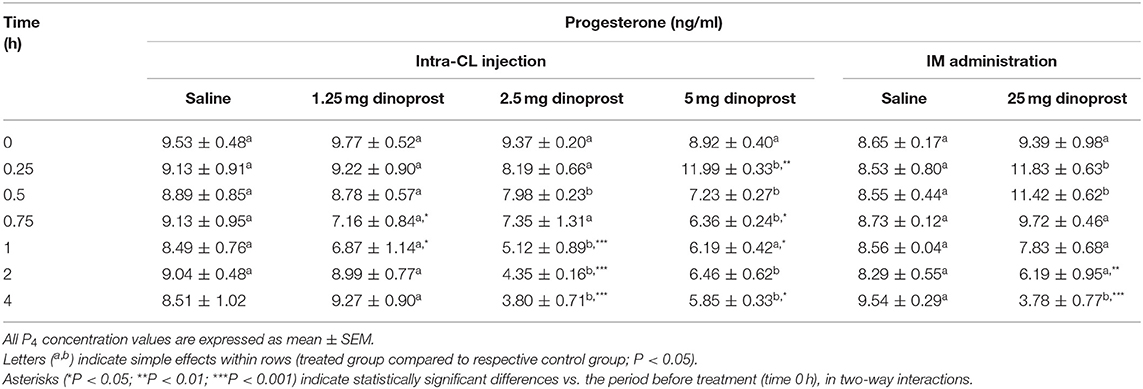
Table 2. Effect of intra-CL injection or IM administration (positive control) of dinoprost or saline solution on progesterone (P4) concentrations in blood plasma of cows (n = 3 per dose), on day 10 of the estrous cycle.
Dose-Dependent Effects of Intra-CL Injection of Dinoprost on mRNA Levels of Steroidogenesis-and Necroptosis-Related Factors in the Middle-Stage CL
Figure 3 shows the results for quantitative analysis of mRNA levels of STAR, P450scc, and HSD3B in bovine middle-stage CL at 4 h after intra-CL injection of dinoprost at different doses, or IM administration (positive control) of dinoprost or saline solution. The intra-CL injection of dinoprost at doses of 2.5 and 5 mg downregulated STAR mRNA levels, compared to the control group (P < 0.05; Figure 3A). Furthermore, IM administration of dinoprost decreased STAR mRNA levels, compared to the control group (P < 0.05; Figure 3A). Additionally, lower STAR mRNA levels were observed after IM administration of dinoprost, compared to intra-CL injection of dinoprost at doses of 2.5 and 5 mg (P < 0.05; Figure 3A). Intramuscular administration of dinoprost downregulated HSD3B mRNA levels, compared to the control group, and also compared to all groups receiving dinoprost by intra-CL injection (P < 0.05; Figure 3C).
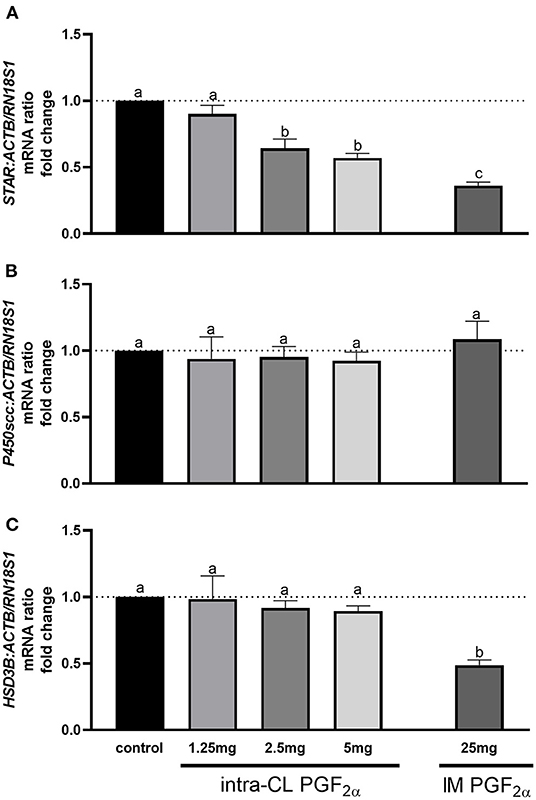
Figure 3. Effect of intra-CL injection or IM administration of dinoprost or saline solution on the mRNA levels of (A) steroidogenic acute regulatory protein (STAR), (B) cytochrome P450 family 11 subfamily A member 1 (P450scc), and (C) hydroxy-delta-5-steroid dehydrogenase, 3β- and steroid delta-isomerase 1 (HSD3B) in middle-stage corpora lutea (CL). Data are the mean ± SEM for three samples per treatment. Letters (a−c) indicate statistical differences between treatment groups (P < 0.05).
Figure 4 shows the results for quantitative analysis of mRNA levels of RIPK1 and RIPK3 in bovine middle-stage CL 4 h after intra-CL injection of dinoprost at different doses, or IM administration (positive control) of dinoprost or saline solution. The intra-CL injection of dinoprost at doses of 2.5 or 5 mg upregulated RIPK1 and RIPK3 mRNA, compared to the control group (P < 0.05; Figures 4A,B). Furthermore, RIPK1 and RIPK3 mRNA levels were enhanced after IM administration of dinoprost, compared to the control group (P < 0.05; Figures 4A,B). Additionally, greater RIPK3 mRNA levels were observed after IM administration of dinoprost, compared to the mRNA levels after intra-CL injection of dinoprost at any of the doses tested (P < 0.05; Figure 4B). A dose of 2.5 mg dinoprost injected directly into CL was chosen as the luteolytic dose for further study.
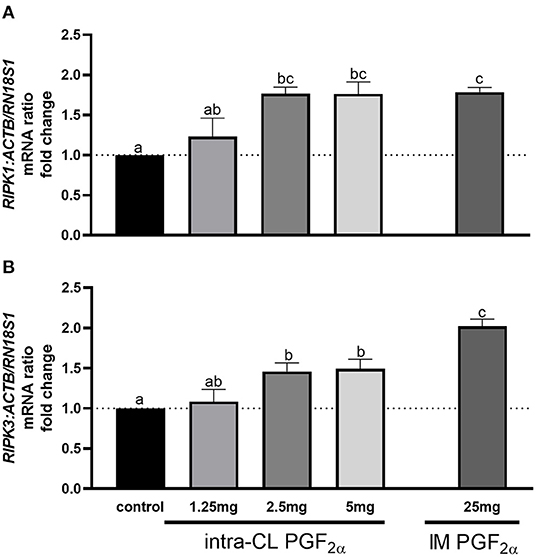
Figure 4. Effect of intra-CL injection of different doses of dinoprost on the mRNA levels of (A) receptor-interacting protein kinase 1 (RIPK1) and (B) receptor-interacting protein kinase 3 (RIPK3) in middle-stage corpora lutea (CL). Data are the mean ± SEM for three samples per treatment. Letters (a−c) indicate statistically significant differences between treatment groups (P < 0.05).
Experiment 2
Effects of Intra-CL Injection of Dinoprost on Progesterone Concentration and Blood Flow in Cows at the Early and Mid-Luteal Phases of the Estrous Cycle, Compared to IM Dinoprost Administration
Figure 5 shows changes in P4 concentrations in cows after intra-CL injection or IM administration of luteolytic doses of dinoprost. Blood plasma P4 concentrations decreased in cows at mid-luteal phase of the estrous cycle between 2 and 12 h after intra-CL injection of dinoprost, while its IM administration reduced P4 concentrations between 8 and 12 h, compared to the pre-treatment period (0 h) (P < 0.001; Figure 5B). Interestingly, P4 concentrations were lower 2 h after intra-CL injection of dinoprost than after the IM administration of dinoprost (P < 0.05; Figure 5B).
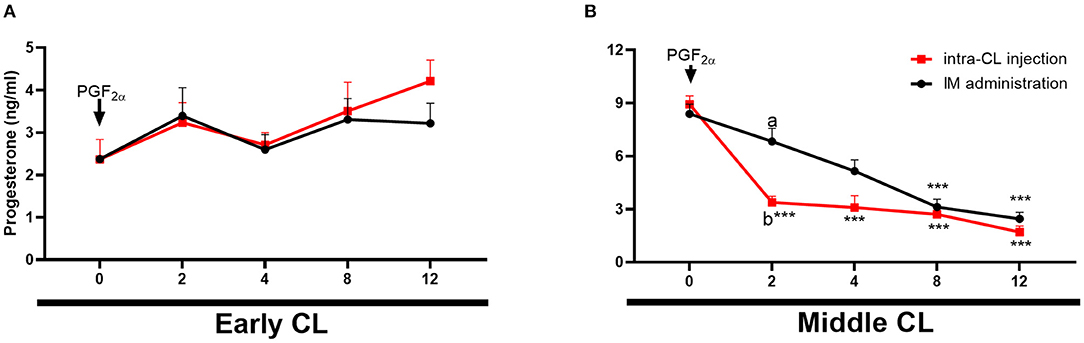
Figure 5. Effects of intra-CL injection (red lines) or IM administration (black lines) of dinoprost on progesterone (P4) concentrations in the blood in cows during the early (A) and middle (B) stages of the estrous cycle. Data are the mean ± SEM for six cows per treatment. Asterisks (***P < 0.001) indicate statistically significant differences vs. the period before treatment (time 0 h), in two-way interactions. Different subscript letters (a,b) indicate statistically significant differences (P < 0.05) between groups (intra-CL group vs. IM group) at the same time point, in three-way interactions.
Figure 6 shows temporal analysis of early- and middle-stage CL morphology and blood flow in cows after intra-CL injection or IM administration of luteolytic doses of dinoprost. In the early-stage CL, CLBF increased 12 h after either intra-CL injection of dinoprost or its IM administration, compared to the pre-treatment period (0 h) (P < 0.05; Figure 6C). Additionally, an increase in Adj. CLBF was observed 12 h after intra-CL injection of dinoprost, compared to the pre-treatment period (P < 0.05; Figure 6E). In the middle-stage CL, intra-CL injection of dinoprost reduced LTA between 8 and 12 h, compared to the pre-treatment period (0 h) (P < 0.01; Figure 6B). Both intra-CL injection of dinoprost and its IM administration increased CLBF and Adj. CLBF at 2 h, while both treatments decreased CLBF and Adj. CLBF between 8 and 12 h, compared to the pre-treatment period (0 h) (P < 0.05; Figures 6D,F). Interestingly, values of CLBF and Adj. CLBF were lower 2 h after intra-CL injection of dinoprost, compared to the IM administration of dinoprost (P < 0.05; Figures 5B,F,H). Moreover, LTA was lower 12 h after intra-CL injection of dinoprost vs. IM administration of dinoprost (P < 0.05; Figure 5D).
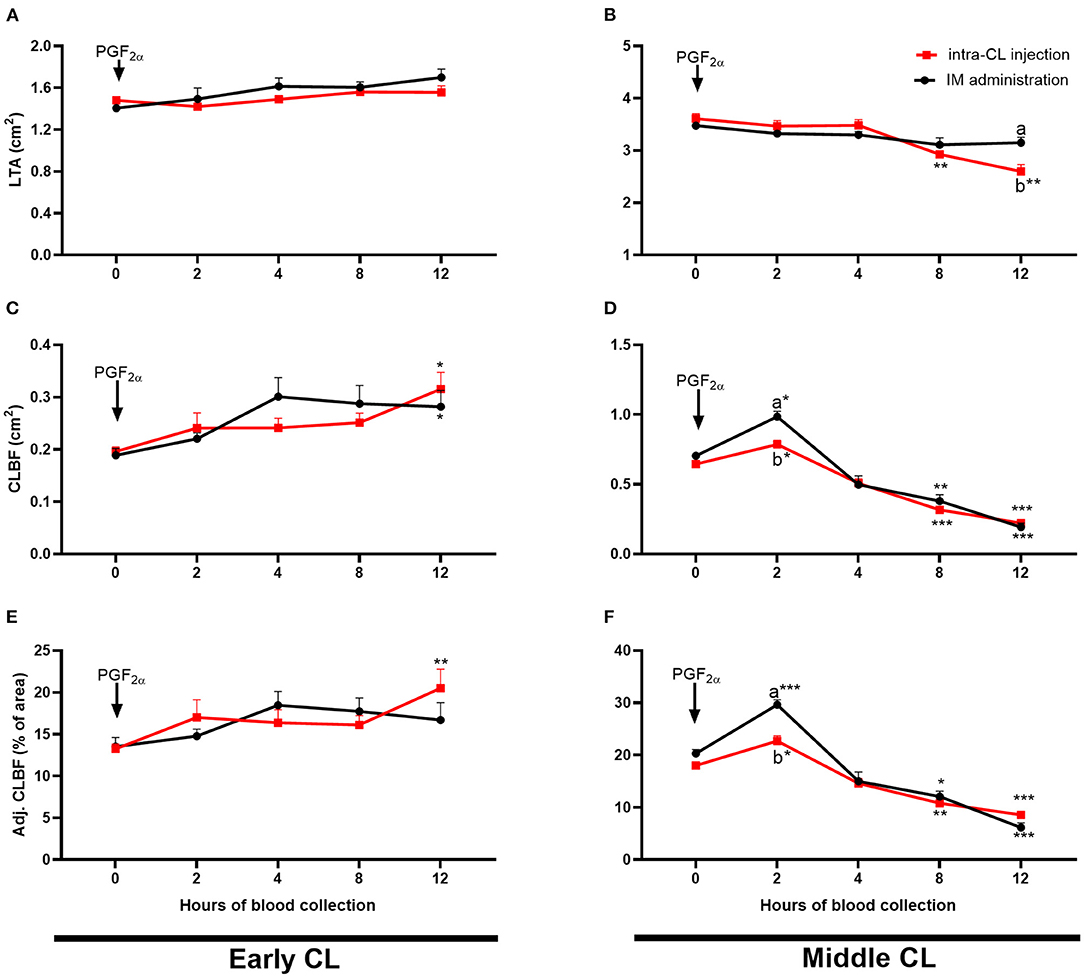
Figure 6. Effect of intra-CL injection (red lines) or IM administration (black lines) of dinoprost on luteal tissue area [LTA; (A,B)], amount of blood flow to the corpus luteum (CL) [CLBF; (C,D)], and CLBF:LTA ratio [Adj. CLBF in %; (E,F)] in cows during the early (A,C,E) and middle (B,D,F) stages of the estrous cycle. Data are the mean ± SEM for six cows per treatment. Asterisks (*P < 0.05; **P < 0.01; ***P < 0.001) indicate statistically significant differences vs. the period before treatment (time 0 h), in two-way interactions. Different subscript letters (a,b) indicate statistically significant differences (P < 0.05) between groups (intra-CL group vs. IM group) at the same time point, in three-way interactions.
Discussion
Regulation of the estrous cycle is widely applied in veterinary practice for estrus synchronization. The most effective routes and sites for administration of PGF2α analogs, used for manipulation of the reproductive processes in breeding cows, have been the subject of numerous studies (40, 50, 51). Besides IM administration of PGF2α analogs, their subcutaneous injection (SC) in the cervical area, or SC injection in the ischio-rectal fossa have also produced declines in P4 concentration, inducing luteolysis in the cow (40, 50, 51). Recently, we have noted that intra-CL injection of dinoprost reduced P4 levels at 4 h in the middle-stage CL, whereas it did not change P4 profiles in the early-stage CL (22). Moreover, peripheral application of PGF2α analogs may be subject to side effects, and may have actions that differ from natural PGF2α (38, 52–54).
The present study expands knowledge about luteolytic action of dinoprost applied directly into CL or IM, on PGF2α-resistant or PGF2α-responsive CL. It is well known that IM PGF2α analogs induce the luteolytic process in cows. Therefore, our study used IM administration of dinoprost as a positive control. Interestingly, we showed that intra-CL injection of dinoprost affected not only secretory function of the bovine CL but also modulated luteal blood flow, depending on the phase of the estrous cycle. Moreover, to the best of our knowledge, we have provided the first evidence of dose-dependent effects of intra-CL injection of dinoprost on OT concentrations in JV blood plasma in the mid-luteal phase of the bovine estrous cycle.
It is well known that PGF2α analogs stimulate rapid OT release from the CL in cattle (55, 56). Therefore, measurement of OT concentration in plasma after IM PGF2α analog treatment is a good indicator of CL sensitivity to PGF2α in cattle (42). Moreover, Skarzynski et al. (42) showed that the effect of IM administration of oestrophan on OT secretion was different on days 12 and 18 of the cycle, and it depended on the drug dosage. Therefore, to confirm the sensitivity of CL to intra-CL injection of different doses of dinoprost, OT concentrations were assessed in blood plasma in the middle-stage CL. Interestingly, in our study we observed that all doses of dinoprost injected directly into CL enhanced OT concentrations in blood plasma during the period of observation. Our results suggest that a rapid increase in OT concentration after intra-CL injection of dinoprost is the hallmark feature of the responsive CL. Therefore, we suspect that intra-CL injection is an adequate method to study the mechanism of PGF2α actions on refractory and responsive bovine CL.
To determine luteolytic activity of different doses of dinoprost injected directly into CL, P4 concentrations were measured in blood plasma in cows at mid-luteal phase of the estrous cycle. Recently, Lopez-Gatius and Hunter (57) examined the action of different dinoprost doses (0.5 mg, 1.5 mg, and 2.5 mg) on CL function, reporting that intra-CL application of 2.5 mg dinoprost resulted in spontaneous twin reduction in pregnant cows by regression of CL. Moreover, Andrlikova et al. (58) studied effects of different doses of cloprostenol (5, 25, 50, and 100 μg) on the luteolytic process, showing that the minimal luteolytic dose of cloprostenol in cows, when injected directly into CL, is 10% of the recommended IM dose of the PGF2α analog. In our study, 1.25 mg of dinoprost was ineffective when injected directly into middle-stage CL during 4 h of observation, while intra-CL injection of 2.5 or 5 mg of dinoprost induced luteolysis by reduction of P4 levels. Our above results suggest that 2.5 mg of dinoprost injected directly into the CL is a minimum luteolytic dose, which is 10% of the recommended IM dose of dinoprost.
To better understand the luteolytic action of PGF2α, we analyzed luteal blood flow and secretory function of the refractory or responsive CL after intra-CL injection of dinoprost, compared to the known actions of IM dinoprost. On one hand, in our study, IM administration of the luteolytic dose of dinoprost into middle-stage CL induced an increase, followed by a decrease, of indicators of the CL vascular network, evidence of certain early events of CL luteolysis in cows, a finding consistent with previous studies (32, 37). It had been previously shown that treatment with various doses of cloprostenol induced an acute increase in luteal blood flow 1 h after treatment, without dose-dependence, in the reduction of luteal volume in the mid-luteal phase (59). Our study is the first to compare the influence of intra-CL injection of dinoprost on blood flow in the early and mid-luteal phases of the estrous cycle. Interestingly, intra-CL injection of dinoprost showed parallel reductions in luteal blood flow and plasma concentrations of P4, relative to IM injection of a luteolytic dose of dinoprost. We should take into account that a drop in the level of P4 began 2 h after intra-CL injection of dinoprost, while the reduction in P4 concentration after dinoprost was administered IM occurred 8 h after treatment. Therefore, we assume that dinoprost injected directly into the CL may be more effective during PGF2α-induced luteolysis. We should highlighted that above results are relevant since a rapid decrease in the functionality of the CL is a better indicator of luteolysis than luteal tissue reduction. For instance, this may imply better results in synchronization protocols when PGF2α is used in combination with other hormones. The above results indicate that the method of intra-CL injection of PGF2α analog may be successfully applied to breeding protocols in dairy cows. Our results are also in agreement with the previous report of Acosta et al. (32) showing that dinoprost administered IM was unable to cause CL regression when given in the early stage of the estrous cycle. Also, the study of Minela and Pursley (59) indicated that different doses of cloprostenol administered IM did not impair luteal development of the early-stage CL. Interestingly, in our study, increases in CLBF and Adj. CLBF were observed 12 h after intra-CL injection of dinoprost, compared to the pre-treatment period, in the early luteal phase of the estrous cycle. The above result confirmed that dinoprost did not induce luteolytic cascade, and did not disturb CL supply and its growth, when the drug was injected directly into early-stage CL. However, to clarify the effect of luteolytic PGF2α on refractory CL, an additional in vivo study should be conducted that explores the mechanisms involved in early-stage CL secretory function in response to intra-CL injection of PGF2α analog.
To better understand the molecular mechanisms underlying luteolysis, we examined the expression of genes involved in steroidogenesis and cell death (necroptosis) in response to different doses of dinoprost injected directly into the CL. It is important to know that acute changes in steroidogenesis appear to be primarily associated with changes in active STAR protein, without changes in activities of other steroidogenic enzymes (60). Moreover, Shirasuna et al. (15) demonstrated that IM administration of PGF2α analog decreased STAR mRNA transcription 0.5 h after treatment in the center and periphery of the middle-stage CL. Recently, we have shown that dinoprost, injected directly into CL or administered IM, downregulated STAR mRNA levels 4 h after treatment in the middle-stage CL (22). Importantly, our study found that intra-CL injection of 1.25 mg dinoprost was not effective in reducing the steroidogenesis pathway, while doses of 2.5 and 5 mg of dinoprost decreased STAR mRNA levels. Thus, early stages of the steroidogenic pathway were blocked. The latest investigations have demonstrated necroptosis (in addition to apoptosis) as a mechanism of the cell death during CL regression (13, 14, 23). Hojo et al. (13) showed that RIPK1 and RIPK3 (the main genes playing roles in necroptotic processes) are strongly elevated in the bovine CL during both spontaneous and IM PGF2α-induced luteolysis. Our previous study reported that dinoprost, injected directly into CL or administered IM, enhanced indicators of necroptosis and apoptosis 4 h after the treatment in the middle-stage CL (23). Interestingly, in our present study, we observed dose-dependent effects of intra-CL injection of dinoprost on genes involved in necroptosis, i.e., intra-CL injection of 1.25 mg dinoprost did not affect the necroptosis pathway, while 2.5 and 5 mg doses of dinoprost up-regulated the relative mRNA levels of RIPK1 and RIPK3. Analyses of expression of genes involved in steroidogenesis or necroptosis showed that the smallest dose of dinoprost injected directly into CL was not related to their effects. Additionally, 2.5 mg of dinoprost injected into the CL was confirmed as the minimum effective dose to induce CL regression, and may be used in veterinary practice to manipulate the estrous cycle.
The method of intra-CL application of drugs/hormones could be a prevalent tool in bovine reproduction. It is important to know that the dairy and beef cow industry economies depend on fertility, and this has been the subject of numerous studies (40, 50, 51, 61). Quality assurance of beef in particular seeks to minimize meat damage to preserve its value, by minimizing the use of hormones in beef production (40). The previous studies of Chebel et al. (50) and Mezera et al. (51), which focused on differences between SC and IM administration, indicated no differences in cows that underwent complete luteolysis. These authors suggested that the SC method of dinoprost administration is acceptable for CL regression. Our results highlight that injection of dinoprost directly into the CL (at lower dose than would be recommended for IM administration) results in a similar physiological response to that produced by IM dinoprost administration. Therefore, we suspect that administration of PGF2α analogs directly into the CL to induce luteolysis may be incorporated into synchronization protocols. This method might have a huge economic value in the dairy and beef cow industries. However, further studies are still needed to understand the influence of intra-CL injection of dinoprost on the duration of the estrous cycle.
In conclusion, the present study demonstrated that intra-CL injection of dinoprost increases OT concentrations and decreases JV P4 levels, in a dose-dependent manner, in cows at the mid-luteal phase of the estrous cycle. An increase in indicators of vascularization of CL (CLBF and Adj. CLBF), accompanied by a drop in P4 level, was observed 2 h after intra-CL dinoprost injection in the middle-stage CL. Moreover, the lack of changes in blood flow and P4 concentration at the early luteal phase of the estrous cycle appeared to be directly correlated with the resistance of CL to the action of dinoprost injected directly into the early-stage CL. Furthermore, the decrease in STAR mRNA and increases in RIPK1 and RIPK3 mRNA levels confirmed that 2.5 mg of dinoprost injected directly into CL is a minimum dose that will induce the luteolytic cascade. We expect that our results could provide new knowledge to optimize breeding protocols in cows.
Data Availability Statement
The original contributions presented in the study are included in the article/Supplementary Materials, further inquiries can be directed to the corresponding author/s.
Ethics Statement
The animal study was reviewed and approved by University of Warmia and Mazury in Olsztyn, Poland (Agreement No. 23/2012/N). Written informed consent was obtained from the owners for the participation of their animals in this study.
Author Contributions
AWJ, KKP-T, and DJS: investigation and writing—review and editing. AWJ and KKP-T: methodology, formal analysis, data curation, visualization, and writing—original draft. DJS: conceptualization, supervision, and funding acquisition. All authors have read, critically revised, and approved the final version of the manuscript.
Funding
The research was supported by a grant from the National Science Center in Poland (DEC-2011/03/B/NZ9/01634).
Conflict of Interest
The authors declare that the research was conducted in the absence of any commercial or financial relationships that could be construed as a potential conflict of interest.
Publisher's Note
All claims expressed in this article are solely those of the authors and do not necessarily represent those of their affiliated organizations, or those of the publisher, the editors and the reviewers. Any product that may be evaluated in this article, or claim that may be made by its manufacturer, is not guaranteed or endorsed by the publisher.
Acknowledgments
The authors thank Drs. G. Kotwica (University of Warmia and Mazury, Olsztyn, Poland) for the antibodies against OT. We are grateful to Dr. M. M. Bah (Institute of Animal Reproduction and Food Research, Polish Academy of Science) for his assistance and cooperation in providing animals for the study from the farm in Research Station in Popielno. The authors wish to thank K. Jankowska, A. Bacławska, W. Krzywiec, P. Kordowitzki, and B. Czarkowska (Institute of Animal Reproduction and Food Research, Polish Academy of Science) for technical support in the experiments. The authors wish to thank K. Witek for Computer-assisted image analyses (Institute of Animal Reproduction and Food Research, Polish Academy of Science). This research is a part of a Ph.D. thesis conducted by AWJ.
Supplementary Material
The Supplementary Material for this article can be found online at: https://www.frontiersin.org/articles/10.3389/fvets.2021.811809/full#supplementary-material
Abbreviations
CL, corpus luteum; P4, progesterone; PGF2α, prostaglandin F2α; IM, intramuscular; OT, oxytocin; LTA, luteal tissue area; CLBF, corpus luteum blood blow; Adj. CLBF, adjusted corpus luteum blood flow; RIA, radioimmunoassay; JV, jugular vein; RT-qPCR, quantitative real-time polymerase chain reaction; GAPDH, glyceraldehyde-3-phosphate dehydrogenase; ACTB, β-actin; RN18S1, 18S ribosomal RNA; STAR, steroidogenic acute regulatory protein; P450scc, cytochrome P450 family 11 subfamily A member; HSD3B, hydroxy-delta-5-steroid dehydrogenase, 3 β- and steroid delta-isomerase 1; RIPK1, receptor-interacting protein kinases 1; RIPK3, receptor-interacting protein kinases 3; ELISA, enzyme-linked immunosorbent assay; SC, subcutaneous injection.
References
1. Niswender GD, Juengel JL, Silva PJ, Rollyson MK, McIntush EW. Mechanisms controlling the function and life span of the corpus luteum. Physiol Rev. (2000) 80:1–29. doi: 10.1152/physrev.2000.80.1.1
2. Miyamoto A, Shirasuna K, Shimizu T, Bollwein H, Schams D. Regulation of corpus luteum development and maintenance: specific roles of angiogenesis and action of prostaglandin F2alpha. Soc Reprod Fertil Suppl. (2010) 67:289–304. doi: 10.7313/upo9781907284991.024
3. Skarzynski DJ, Jaroszewski JJ, Bah MM, Deptuła KM, Barszczewska B, Gawronska B, et al. Administration of a nitric oxide synthase inhibitor counteracts prostaglandin F2-induced luteolysis in cattle. Biol Reprod. (2003) 68:1674–81. doi: 10.1095/biolreprod.102.008573
4. Skarzynski DJ, Bah MM, Deptula KM, Woclawek-Potocka I, Korzekwa A, Shibaya M, et al. Roles of tumor necrosis factor of the estrous cycle in cattle: an in vivo study. Biol Reprod. (2003) 69:1907–13. doi: 10.1095/biolreprod.103.016212
5. Arosh JA, Banu SK, Chapdelaine P, Madore E, Sirois J, Fortier MA. Prostaglandin biosynthesis, transport, and signaling in corpus luteum: a basis for autoregulation of luteal function. Endocrinology. (2004) 145:2551–60. doi: 10.1210/en.2003-1607
6. Zerani M, Catone G, Maranesi M, Gobbetti A, Boiti C, Parillo F. Gonadotropin-releasing hormone 1 directly affects corpora lutea lifespan in mediterranean buffalo (Bubalus bubalis) during diestrus: presence and in vitro effects on enzymatic and hormonal activities. Biol Reprod. (2012) 87:45. doi: 10.1095/biolreprod.112.099598
7. Parillo F, Catone G, Maranesi M, Gobbetti A, Gasparrini B, Russo M, et al. Immunolocalization, gene expression, and enzymatic activity of cyclooxygenases, prostaglandin e2-9-ketoreductase, and nitric oxide synthases in Mediterranean buffalo (Bubalus bubalis) corpora lutea during diestrus. Microsc Res Tech. (2012) 75:1682–90. doi: 10.1002/jemt.22116
8. Shirasuna K, Akabane Y, Beindorff N, Nagai K, Sasaki M, Shimizu T, et al. Expression of prostaglandin F2α (PGF2α) receptor and its isoforms in the bovine corpus luteum during the estrous cycle and PGF2α-induced luteolysis. Domest Anim Endocrinol. (2012) 43:227–38. doi: 10.1016/j.domaniend.2012.03.003
9. Miyamoto A, Shirasuna K, Shimizu T, Matsui M. Impact of angiogenic and innate immune systems on the corpus luteum function during its formation and maintenance in ruminants. Reprod Biol. (2013) 13:272–8. doi: 10.1016/j.repbio.2013.09.006
10. Skarzynski DJ, Piotrowska-Tomala KK, Lukasik K, Galvão A, Farberov S, Zalman Y, et al. Growth and regression in bovine corpora lutea: regulation by local survival and death pathways. Reprod Domest Anim. (2013) 48:25–37. doi: 10.1111/rda.12203
11. Juengel JL, Garverick HA, Johnson AL, Youngquist RS, Smith MF. Apoptosis during luteal regression in cattle. Endocrinology. (1993) 132:249–54. doi: 10.1210/endo.132.1.8419126
12. Yadav VK, Lakshmi G, Medhamurthy R. Prostaglandin F2α-mediated activation of apoptotic signaling cascades in the corpus luteum during apoptosis: involvement of caspase-activated DNase. J Biol Chem. (2005) 280:10357–67. doi: 10.1074/jbc.M409596200
13. Hojo T, Siemieniuch MJ, Lukasik K, Piotrowska-Tomala KK, Jonczyk AW, Okuda K, et al. Programmed necrosis - a new mechanism of steroidogenic luteal cell death and elimination during luteolysis in cows. Sci Rep. (2016) 6:3821. doi: 10.1038/srep38211
14. Hojo T, Piotrowska-Tomala KK, Jonczyk AW, Lukasik K, Jankowska K, Okuda K, et al. Receptor interacting protein kinases-dependent necroptosis as a new, potent mechanism for elimination of the endothelial cells during luteolysis in cow. Theriogenology. (2019) 128:193–200. doi: 10.1016/j.theriogenology.2019.01.035
15. Shirasuna K, Sasahara K, Matsui M, Shimizu T, Miyamoto A. Prostaglandin F2alpha differentially affects mRNA expression relating to angiogenesis, vasoactivation and prostaglandins in the early and mid corpus luteum in the cow. J Reprod Dev. (2010) 56:428–36. doi: 10.1262/jrd.10-004o
16. Mondal M, Schilling B, Folger J, Steibel JP, Buchnick H, Zalman Y, et al. Deciphering the luteal transcriptome: potential mechanisms mediating stage-specific luteolytic response of the corpus luteum to prostaglandin F2α. Physiol Genomics. (2011) 43:447–56. doi: 10.1152/physiolgenomics.00155.2010
17. Atli MO, Bender RW, Mehta V, Bastos MR, Luo W, Vezina CM, et al. Patterns of gene expression in the bovine corpus luteum following repeated intrauterine infusions of low doses of prostaglandin F2alpha. Biol Reprod. (2012) 86:130. doi: 10.1095/biolreprod.111.094870
18. Zalman Y, Klipper E, Farberov S, Mondal M, Wee G, Folger JK, et al. Regulation of angiogenesis-related prostaglandin f2alpha-induced genes in the bovine corpus luteum. Biol Reprod. (2012) 86:92. doi: 10.1095/biolreprod.111.095067
19. Skarzynski DJ, Okuda K. Sensitivity of bovine corpora lutea to prostaglandin F2alpha is dependent on progesterone, oxytocin, and prostaglandins. Biol Reprod. (1999) 60:1292–8. doi: 10.1095/biolreprod60.6.1292
20. Skarzynski DJ, Jaroszewski JJ, Okuda K. Luteotropic mechanisms in the bovine corpus luteum: role of oxytocin, PGF2α, progesterone and noradrenaline. J Reprod Dev. (2001) 47:125–37. doi: 10.1262/jrd.47.125
21. Bowolaksono A, Nishimura R, Hojo T, Sakumoto R, Acosta TJ, Okuda K. Antiapoptotic roles of prostaglandin E2 and F2alpha in bovine luteal steroidogenic cells. Biol Reprod. (2008) 79:310–7. doi: 10.1095/biolreprod.107.066084
22. Jonczyk AW, Piotrowska-Tomala KK, Kordowitzki P, Skarzynski DJ. Effects of prostaglandin F2α on angiogenic and steroidogenic pathways in the bovine corpus luteum may depend on its route of administration. J Dairy Sci. (2019) 102:10573–86. doi: 10.3168/jds.2019-16644
23. Jonczyk AW, Piotrowska-Tomala KK, Skarzynski DJ. Effects of prostaglandin F2α (PGF2α) on cell-death pathways in the bovine corpus luteum. BMC Vet Res. (2019) 15:416. doi: 10.1186/s12917-019-2167-3
24. McCracken JA, Custer EE, Lamsa JC. Luteolysis: a neuroendocrine-mediated event. Physiol Rev. (1999) 79:263–323. doi: 10.1152/physrev.1999.79.2.263
25. Schams D, Berisha B. Regulation of CL function in cattle-an overview. Reprod Domest Anim. (2004) 39:241–51. doi: 10.1111/j.1439-0531.2004.00509.x
26. Ginther OJ, Araujo RR, Palhao MP, Rodrigues BL, Beg MA. Necessity of sequential pulses of prostaglandin F2a for complete physiologic luteolysis in cattle. Biol Reprod. (2009) 80:641–8. doi: 10.1095/biolreprod.108.072769
27. Ginther OJ, Araujo RR, Rodrigues BL, Beg MA. Luteal function and blood flow during intravenous infusion of prostaglandin F2α in heifers. Anim Reprod. (2009) 6:400–8. doi: 10.1095/biolreprod11.2.191
28. Wenzinger B, Bleul U. Effect of a prostaglandin F2α analogue on the cyclic corpus luteum during its refractory period in cows. BMC Vet Res. (2012) 8:220. doi: 10.1186/1746-6148-8-220
29. Tsai SJ, Wiltbank MC. Prostaglandin F2alpha regulates distinct physiological changes in early and mid- cycle bovine corpora lutea. Biol Reprod. (1998) 58:346–52. doi: 10.1095/biolreprod58.2.346
30. Levy N, Kobayashi S, Roth Z, Wolfenson D, Miyamoto A, Meidan R. Administration of prostaglandin F2α during the early bovine luteal phase does not alter the expression of ET-1 and of its type A receptor: A possible cause for corpus luteum refractoriness. Biol Reprod. (2000) 63:377–82. doi: 10.1095/biolreprod63.2.377
31. Miyamoto A, Shirasuna K, Wijayagunawardane MPB, Watanabe S, Hayashi M, Yamamoto D, et al. Blood flow: a key regulatory component of corpus luteum function in the cow. Domest Anim Endocrinol. (2005) 29:329–39. doi: 10.1016/j.domaniend.2005.03.011
32. Acosta TJ, Yoshizawa N, Ohtani M, Miyamoto A. Local changes in blood flow within the early and midcycle corpus luteum after prostaglandin F(2alpha) injection in the cow. Biol Reprod. (2002) 66:651–8. doi: 10.1095/biolreprod66.3.651
33. Herzog K, Brockhan-Lüdemann M, Kaske M, Beindorff N, Paul V, Niemann H, et al. Luteal blood flow is a more appropriate indicator for luteal function during the bovine estrous cycle than luteal size. Theriogenology. (2010) 73:691–7. doi: 10.1016/j.theriogenology.2009.11.016
34. Ginther OJ, Silva LA, Araujo RR, Beg MA. Temporal associations among pulses of 13,14-dihydro-15-keto-PGF2α, luteal blood flow, and luteolysis in cattle. Biol Reprod. (2007) 76:506–13. doi: 10.1095/biolreprod.106.057653
35. Pate JL. Involvement of immune cells in regulation of ovarian function. J Reprod Fertil Suppl. (1995) 49:365–77.
36. Korzekwa AJ, Okuda K, Woclawek-Potocka I, Murakami S, Skarzynski DJ. Nitric oxide induces apoptosis in bovine luteal cells. J Reprod Dev. (2006) 52:353–61. doi: 10.1262/jrd.17092
37. Acosta TJ, Bah MB, Korzekwa A, Woclawek-Potocka I, Markiewicz W, Jaroszewski JJ, et al. Acute changes in circulating concentrations of progesterone and nitric oxide and partial pressure of oxygen during prostaglandin F2alpha-induced luteolysis in cattle. J Reprod Dev. (2009) 55:149–55. doi: 10.1262/jrd.20133
38. Skarzynski DJ, Siemieniuch MJ, Pilawski W, Woclawek-Potocka I, Bah MM, Majewska M, et al. In vitro assessment of progesterone and prostaglandin e(2) production by the corpus luteum in cattle following pharmacological synchronization of estrus. J Reprod Dev. (2009) 55:170–6. doi: 10.1262/jrd.20121
39. Thomas JM, Bishop BE, Abel JM, Ellersieck MR, Smith MF, Patterson DJ. The 9-day CIDR-PG protocol: incorporation of PGF2α pretreatment into a long-term progestin-based estrus synchronization protocol for postpartum beef cows. Theriogenology. (2016) 85:1555–61. doi: 10.1016/j.theriogenology.2016.01.010
40. Holland SC, Whittier WD, Clark SG, Hafez SA, Swecker WSJr. Comparison of luteolysis and timed artificial insemination pregnancy rates after administration of PGF2α in the muscle or the ischiorectal fossa in cattle. Anim Reprod Sci. (2018) 198:11–9. doi: 10.1016/j.anireprosci.2018.07.003
41. Bandai K, Kusaka H, Miura H, Kikuchi M, Sakaguchi M. A simple and practical short-term timed artificial insemination protocol using estradiol benzoate with prostaglandin F2α in lactating dairy cows. Theriogenology. (2020) 141:197–201. doi: 10.1016/j.theriogenology.2019.09.024
42. Skarzynski DJ, Bogacki M, Kotwica J. Changes in ovarian oxytocin secretion as an indicator of corpus luteum response to prostaglandin F2α treatment in cattle. Theriogenology. (1997) 48:733–42. doi: 10.1016/s0093-691x(97)00297-5
43. Skarzynski DJ, Piotrowska KK, Bah MM, Korzekwa A, Woclawek-Potocka I, Sawai K, et al. Effects of exogenous tumour necrosis factor-alpha on the secretory function of the bovine reproductive tract depend on tumour necrosis factor-alpha concentrations. Reprod Domest Anim. (2009) 44:371–9. doi: 10.1111/j.1439-0531.2007.01016.x
44. Piotrowska KK, Woclawek-Potocka I, Bah MM, Piskula M, Pilawski W, Bober A, et al. Phytoestrogens and their metabolites inhibit the sensitivity of bovine CL on luteotropic factors. J Reprod Dev. (2006) 52:33–41. doi: 10.1262/jrd.17054
45. Schams D, Schmidt-Polex B, Kruse V. Oxytocin determination by radioimmunoassay in cattle. I Method and preliminary physiological data. Acta Endocrinol. (1979) 92:258–70. doi: 10.1530/acta.0.0920258
46. Kotwica J, Skarzynski D. Influence of oxytocin removal from the corpus luteum on secretory function and duration of the oestrous cycle in cattle. J Reprod Fertil. (1993) 97:411–7. doi: 10.1530/jrf.0.0970411
47. Siqueira LG, Arashiro EK, Ghetti AM, Souza ED, Feres LF, Pfeifer LF, et al. Vascular and morphological features of the corpus luteum 12 to 20 days after timed artificial insemination in dairy cattle. J Dairy Sci. (2019) 102:5612–22. doi: 10.3168/jds.2018-15853
48. Andersen CL, Jensen JL, Orntoft TF. Normalization of real-time quantitative reverse transcription-PCR data: a model-based variance estimation approach to identify genes suited for normalization, applied to bladder and colon cancer data sets. Cancer Res. (2004) 64:5245–50. doi: 10.1158/0008-5472.CAN-04-0496
49. Zhao S, Fernald RD. Comprehensive algorithm for quantitative real-time polymerase chain reaction. J Comput Biol. (2005) 12:1047–64. doi: 10.1089/cmb.2005.12.1047
50. Chebel RC, Santos JE, Rutigliano HM, Cerri RL. Efficacy of an injection of dinoprost tromethamine when given subcutaneously on luteal regression in lactating Holstein cows. Theriogenology. (2007) 67:590–7. doi: 10.1016/j.theriogenology.2006.09.007
51. Mezera MA, Lauber MR, Beard AD, Cabrera EM, Wiltbank MC, Fricke PM. Effect of route of administration of dinoprost tromethamine on plasma profiles of 13,14-dihydro-15-keto-prostaglandin F2α and progesterone in lactating Holstein cows. JDS Commun. (2021) 2:421–5. doi: 10.3168/jdsc.2021-0142
52. MacMillan KL, Day AM. Prostaglandin F2α-fertility drug in dairy cattle. Theriogenology. (1982) 16:245–53. doi: 10.1016/0093-691x(82)90001-2
53. Hansen TR, Randel RD, Segerson ECJr, Rutter LM, Harms PG. Corpus luteum function following spontaneous or PGF-induced estrus in Brahman cows and heifers. J Anim Sci. (1987) 65:524–33. doi: 10.2527/jas1987.652524x
54. Diskin MG, Austin EJ, Roche JF. Exogenous hormonal manipulation of ovarian activity in cattle. Dom Animal Reprod. (2002) 23:211–28. doi: 10.1016/s0739-7240(02)00158-3
55. Schallenberger E, Schams D, Bullermann B, Walters DL. Pulsatile secretion of gonadotrophins, ovarian steroids and ovarian oxytocin during prostaglandin-induced regression of the corpus luteum in the cow. J Reprod Fertil. (1984) 71:493–501. doi: 10.1530/jrf.0.0710493
56. Ohtani M, Kobayashi S, Miyamoto A, Hayashi K, Fukui Y. Real-time relationships between intraluteal and plasma concentrations of endothelin, oxytocin, and progesterone during prostaglandin F2alpha-induced luteolysis in the cow. Biol Reprod. (1998) 58:103–8. doi: 10.1095/biolreprod58.1.103
57. López-Gatius F, Hunter R. Twin reduction by PGF2α intraluteal instillation in dairy cows. Reprod Domest Anim. (2016) 51:940–4. doi: 10.1111/rda.12765
58. Andrlikova M, Andrlova P, Barbato O, Tabery J, Bina V, Vostry L, et al. Responses to intra-luteal administration of cloprostenol in dairy cows. Anim Reprod Sci. (2020) 219:106512. doi: 10.1016/j.anireprosci.2020.106512
59. Minela T, Pursley JR. Effect of cloprostenol sodium dose on luteal blood flow and volume measurements in Holstein heifers with both day-4 and day-10 corpora lutea. J Dairy Sci. (2021) 104:9327–39. doi: 10.3168/jds.2020-19933
60. Stocco DM, Clark BJ. Regulation of the acute production of steroidsin steroidogenic cells. Endocr Rev. (1996) 17:221–44. doi: 10.1210/edrv-17-3-221
Keywords: prostaglandin F2α, corpus luteum, bovine, progesterone, oxytocin, blood flow, luteolysis
Citation: Jonczyk AW, Piotrowska-Tomala KK and Skarzynski DJ (2022) Comparison of Intra-CL Injection and Peripheral Application of Prostaglandin F2α Analog on Luteal Blood Flow and Secretory Function of the Bovine Corpus Luteum. Front. Vet. Sci. 8:811809. doi: 10.3389/fvets.2021.811809
Received: 09 November 2021; Accepted: 13 December 2021;
Published: 11 January 2022.
Edited by:
Arumugam Kumaresan, National Dairy Research Institute (ICAR), IndiaReviewed by:
Mauricio Silva, Temuco Catholic University, ChileMargherita Maranesi, University of Perugia, Italy
Copyright © 2022 Jonczyk, Piotrowska-Tomala and Skarzynski. This is an open-access article distributed under the terms of the Creative Commons Attribution License (CC BY). The use, distribution or reproduction in other forums is permitted, provided the original author(s) and the copyright owner(s) are credited and that the original publication in this journal is cited, in accordance with accepted academic practice. No use, distribution or reproduction is permitted which does not comply with these terms.
*Correspondence: Katarzyna K. Piotrowska-Tomala, k.piotrowska-tomala@pan.olsztyn.pl
†ORCID: Agnieszka W. Jonczyk orcid.org/0000-0002-6867-0823
Katarzyna K. Piotrowska-Tomala orcid.org/0000-0001-6989-9243
Dariusz J. Skarzynski orcid.org/0000-0001-9537-3560