- 1State Key Laboratory of Livestock and Poultry Breeding, Ministry of Agriculture Key Laboratory of Animal Nutrition and Feed Science in South China, Guangdong Public Laboratory of Animal Breeding and Nutrition, Guangdong Provincial Key Laboratory of Animal Breeding and Nutrition, Maoming Branch, Guangdong Laboratory for Lingnan Modern Agriculture, Institute of Animal Science, Guangdong Academy of Agricultural Sciences, Guangzhou, China
- 2Guangdong Provincial Key Laboratory of Animal Nutrition Control, Institute of Subtropical Animal Nutrition and Feed, College of Animal Science, South China Agricultural University, Guangzhou, China
Deoxynivalenol (DON) reduces growth performance and damage intestinal function, and resveratrol (RES) has positive effects on growth performance and intestinal function. The purpose of this study was to investigate the protective mechanism of RES in vitro and vivo challenged with DON. The results showed that dietary supplementation with DON significantly increase the mRNA expression levels of mitophagy- related genes, and protein level for PINK1, Parkin, Beclin-1, Lamp, Atg5, Map1lc, Bnip3, Fundc1, Bcl2l1 and SQSTMS1 (P < 0.05), while supplementation with both RES and DON decreased those indexes in the ileum. Besides DON significantly decreased protein level for Pyruvate Dehydrogenase, Cytochrome c, MFN1, OPA1, and PHB1 (P < 0.05), while supplementation with both RES and DON increased protein level for PHB1, SDHA, and VDAC in the ileum. Moreover, in vitro, we found that DON significantly decreased mitochondrial respiration (P < 0.05), while RES + DON increased the rate of spare respiratory capacity. Also, DON significantly decreased total NAD and ATP (P < 0.05), while RES + DON increased the total NAD and ATP. These results indicate that RES may ameliorates the intestinal damage challenged with deoxynivalenol through mitophagy in weaning piglets.
Introduction
Deoxynivalenol (DON) or vomiting toxin, is the most common trichothecenes toxin produced by Fusarium, which mainly contaminates cereal crops (1). DON could reduce animal feed intake, vomiting, fever, diarrhea, and anorexia, even death. However, different animals have different sensitivity to DON. Pigs are the most sensitive animals among monogastric animals and ruminants (2–5). Recent study has shown that DON could reduce the growth performance of pigs, affect immune system, antioxidant system, cell signal transduction, gene expression and protein synthesis of livestock (6). Our previous studies have found that DON decreased the growth performance weaned piglets, destroyed intestinal function and structural integrity, weakened antioxidant capacity and protein synthesis levels of weaning piglets (2, 3). The mechanism by which DON exerts its toxicological effects is through binding to the phthaloyltransferase on the 60 s subunit of eukaryotic ribosomes, causing MAPK phosphorylation, inducing inflammatory process in the organism, and leading to lipid peroxidation damage in cell membranes, which in turn inhibition of protein and genetic material synthesis. However, the traditional chemical detoxification of DON pollution is unable to meet the low-energy, high-efficiency, and green environmental protection in modern farming. In recent years, the control of piglet stress damage through nutrition has received ever greater attention. Therefore, it is one of the hot spots in animal nutrition research to effectively mitigate DON damage to piglets through nutritional regulation to improve piglet growth performance.
Studies have shown that the addition of arginine, glutamic acid and antibacterial peptides to feed can effectively mitigate the toxic effect of DON on the intestinal damage in weaned piglets (4). RES is a bioactive material, naturally occurring polyphenolic plant antitoxin with anti-inflammatory, anti-aging, anti-cancer and cardioprotective properties. Found mainly in wine, blueberries, peanuts and other nuts, it is a potential additive to mitigate the toxic effects of DON (7). RES was shown to improve mitochondrial respiratory metabolism and lipid oxidation through Sirt1, and to target mitochondria to ameliorate stress damage (8). Mitochondria are the main target organelles for oxidative damage. Excessive ROS produced by damaged mitochondria will activate proteins such as p53 and Caspase to initiate apoptosis. Effective identification and removal of damaged mitochondria from the cells is therefore essential to ameliorate stress damage. Study shows RES attenuates the oxidative damage via mitochondrial autophagy in Parkinson's patients (9), and mitigate mitochondrial damage, and improve the intestinal function in diquat-challenge piglets (10). Thus, RES could mitigate organismal damage through mitochondrial autophagy, however, its mitigating effect on DON-induced intestinal damage are not well understood.
Therefore, in this study, by using DON-induced model in vitro and vivo, we want to know the protective regulatory role of resveratrol on intestinal function challenged with DON. This study provides a theoretical basis for the nutritional regulation of early weaned piglets.
Materials and Methods
Animals and Diets
All animal procedures used in the present study were approved by the Animal Care and Use Committee of Guangdong Academy of Agricultural Sciences and followed the Guidelines for the Care and Use of Animals for Research and Teaching. A total of 64 weaned piglets [Duroc × (Landrace × Yorkshire), 21 days old, barrow] with an initial weaning weight of 6.97 ± 0.10 kg were randomly allocated to four dietary treatments. The piglets fed a basal diet were considered the control group (CON), and the other groups were fed the basal diet supplemented with 300 mg RES/kg diet (RES), 3.8 mg DON/kg diet (DON) or 3.8 mg DON plus 300 mg RES per kg diet (DON+RES group) for a 28-days feeding trial. RES (> 99.0%) was obtained commercially from Shaanxi Ciyuan Biotechnology Co., Ltd. (Xian, China). Each treatment consisted of eight replicate pens, with two piglets per pen (n = 16 piglets per treatment). The basal diet was formulated to meet the nutrient recommendations of the National Research Council (NRC) 2012.
Sample Collection and Processing
At the end of the experiment, eight piglets from each group were anesthetized and bled, the abdominal cavity was quickly dissected and the viscera removed. The intestinal segments were ligated and 1 cm sections of intestine were taken from the middle of the whole jejunum and ileum respectively, washed with pre-cooled PBS, collected in centrifuge tubes and snap frozen in liquid nitrogen, then stored at −80°C for further study.
Cell Culture and Treatment
The cell culture refers to our previous study (11). High-glucose (25 mM) Dulbecco's modified Eagle's (DMEM-H), fetal bovine serum (FBS), and antibiotics were procured from Invitrogen (Grand Island, NY, USA). Plastic culture plates were manufactured by Corning Inc. (Corning, NY, USA). Unless indicated, all other chemicals were purchased from Sigma-Aldrich (St. Louis, MO, USA). IPEC-J2 cells were seeded and cultured with DMEM-H medium containing 10% FBS, 5mM l-glutamine, 100 U/mL penicillin, and 100 μg/ml streptomycin at 37°C in a 5% CO2 incubator. After an overnight incubation, the cells were changed to culture in 15 μmol/L RES for 24 h and then exposed to 0.5 μmol/L DON for another 24 h. Cells were treated or collected for the analysis of extracellular flux, and GC-MS.
Real-Time PCR
The protocol of total RNA extraction, quantification, cDNA synthesis and real-time PCR was adapted from the method of (12). Briefly, total RNA was isolated from intestinal samples by using the Trizol method. Real time PCR was carried out by using forward and reverse primers (Supplementary Table 1) to amplify the target genes. For quantification, amplification efficiencies curves were constructed from serial 1:2 dilutions, and the 2−ΔΔCT method was used to calculate the mRNA expression of the target genes relative to housekeeping gene (β-actin).
Western Blotting Analysis
Frozen intestinal samples were collected as described by Tan et al. (13). Protein concentrations of tissue homogenates were measured by using the BCA method and bovine serum albumin as standard. All samples were adjusted to an equal concentration (50 μg protein). The western blotting was conducted based on previous description. The primary antibodies are LC-3B (1: 1,000; Cell Signaling Technology), P62 (1: 1,000; Cell Signaling Technology), Parkin (1: 1,000; Cell Signaling Technology), BNIP3/Nix (1: 1,000; Cell Signaling Technology), BNIP3 (1: 1,000; Cell Signaling Technology), Pyruvate Dehydrogen (1: 1,000; Cell Signaling Technology), COX IV(1: 1,000; Cell Signaling Technology), Cytochrome c(1: 1,000; Cell Signaling Technology), HSP 60(1: 1,000; Cell Signaling Technology), Mitofusin 1(1: 1,000; Cell Signaling Technology), Mitofusin 2(1: 1,000; Cell Signaling Technology), OPA1(1: 1,000; Cell Signaling Technology), PHB1(1: 1,000; Cell Signaling Technology), SDHA(1: 1,000; Cell Signaling Technology), SOD1(1: 1,000; Cell Signaling Technology), TOM20(1: 1,000; Cell Signaling Technology), VDAC(1: 1,000; Cell Signaling Technology) or β-actin(1: 1,000; Cell Signaling Technology). All protein measurements were normalized to β-actin.
Extracellular Flux Assays
The XF-24 Extracellular Flux Analyzer and Cell Mito Stress Test Kit from Seahorse Biosciences were used to examine the effects of addition of different treated with 0 (NC) or 0.5 μmol/L or 1 μmol/L DON and 0 or 15 μM RES, respectively on mitochondrial respiration in IPEC-J2 cells. Cells in four replicates per group. Owing to the effects of DON on IPEC-J2 cell proliferation, total cellular protein was determined and used to normalize mitochondrial respiration rates.
Gene Knockout With CRISPR-Cas9
Thanks to Yulong Yin lab for providing ATG5 plasmid (13). Guide RNAs were designed using the online CRISPR design tool (http://crispr.mit.edu/) and then cloned into the BbsI-digested plasmids (pSpCas9n) containing the entire guide RNA scaffold. The genomic region flanking the ATG5 target site was amplified using polymerase chain reaction (PCR). The products underwent a reannealing process to facilitate heteroduplex formation. After re-annealing, the products were treated with T7 Endonuclease I (NEB) following the manufacturer's recommended protocol. Then used lentiviral transfection, the viral solution was added to the cell culture medium and co-incubated with the cells.
Statistical Analysis
Results are expressed as Mean ± SEM. The statistical analysis was performed by one-way ANOVA using SPSS 17.0 (SPSS Inc., Chicago, IL, USA). Probability values <0.05 and <0.01 were considered statistically significant. P-values were calculated using a two-tailed paired Student's t-test.
Results
Dietary Supplementation With RES Alleviated the Negative Effects on mRNA Expression Levels of Mitophagy-Related Genes Challenged With DON
To determine the molecular mechanism of RES on DON-fed piglets, we analyzed the mRNA expressions of mitophagy-related genes in the ileum and jejunum of weaning piglets (Figure 1). Dietary supplementation with DON increased (P < 0.05) the mRNA expressions of PINK1, Parkin, Beclin-1, Lamp, Atg5, Map1lc, Bnip3, Fundc1, Bcl2l1 and SQSTM1 in the ileum, while there were no differences (P > 0.05) in those indexes among the Control, RES, and RES+ DON treatments in the ileum. However, there were no differences (P > 0.05) in the mRNA expressions of PINK1, Parkin, Beclin-1, Lamp, Atg5, Map1lc, Bnip3, Fundc1, Bcl2l1 and SQSTM1 expressions among the four treatments in the jejunum.
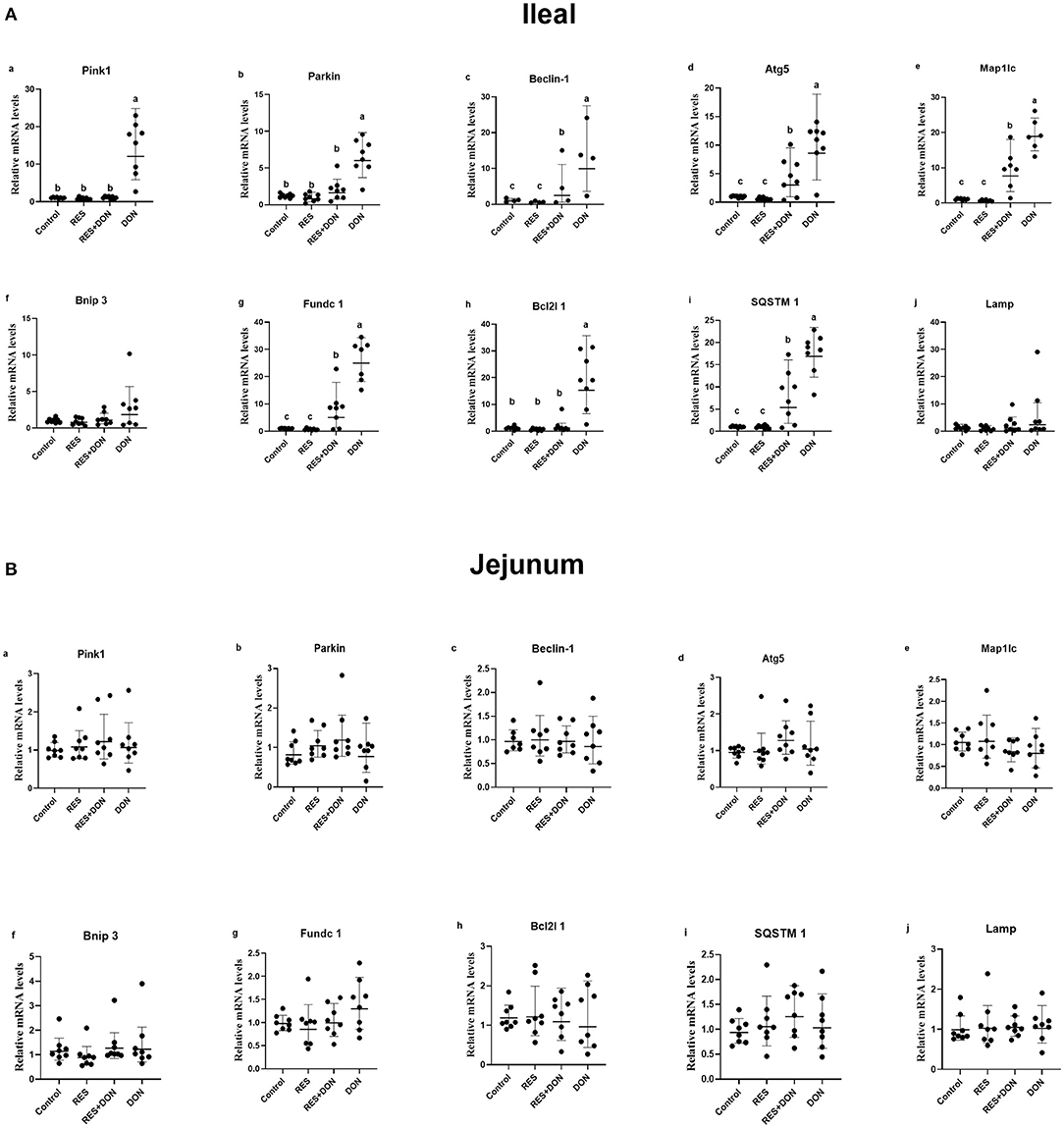
Figure 1. Dietary supplementation with RES alleviated the negative effects on mRNA expression levels of mitophagy-related genes challenged with DON. Data were expressed as means ± SEM of at least three independent experiments. (A,B) Values with different letters are significantly different (P < 0.05).
Dietary Supplementation With RES Increases the Expression of Mitophagy-Related Genes
Since we know that there are significant differences in mitophagy-related genes in the ileum, and then we analyzed the protein expressions of autophagy genes in ileum. The relative expression levels of LC3B, p62, Parkin, Binp3/Nix, and BINP3 are shown in Figure 2. Dietary supplementation with 3.8 mg DON/kg diet (DON) increased (P < 0.05) protein levels for BNIP3, while there were no differences (P > 0.05) in those indexes among the Control, RES, and RES+ DON treatments in the ileum.
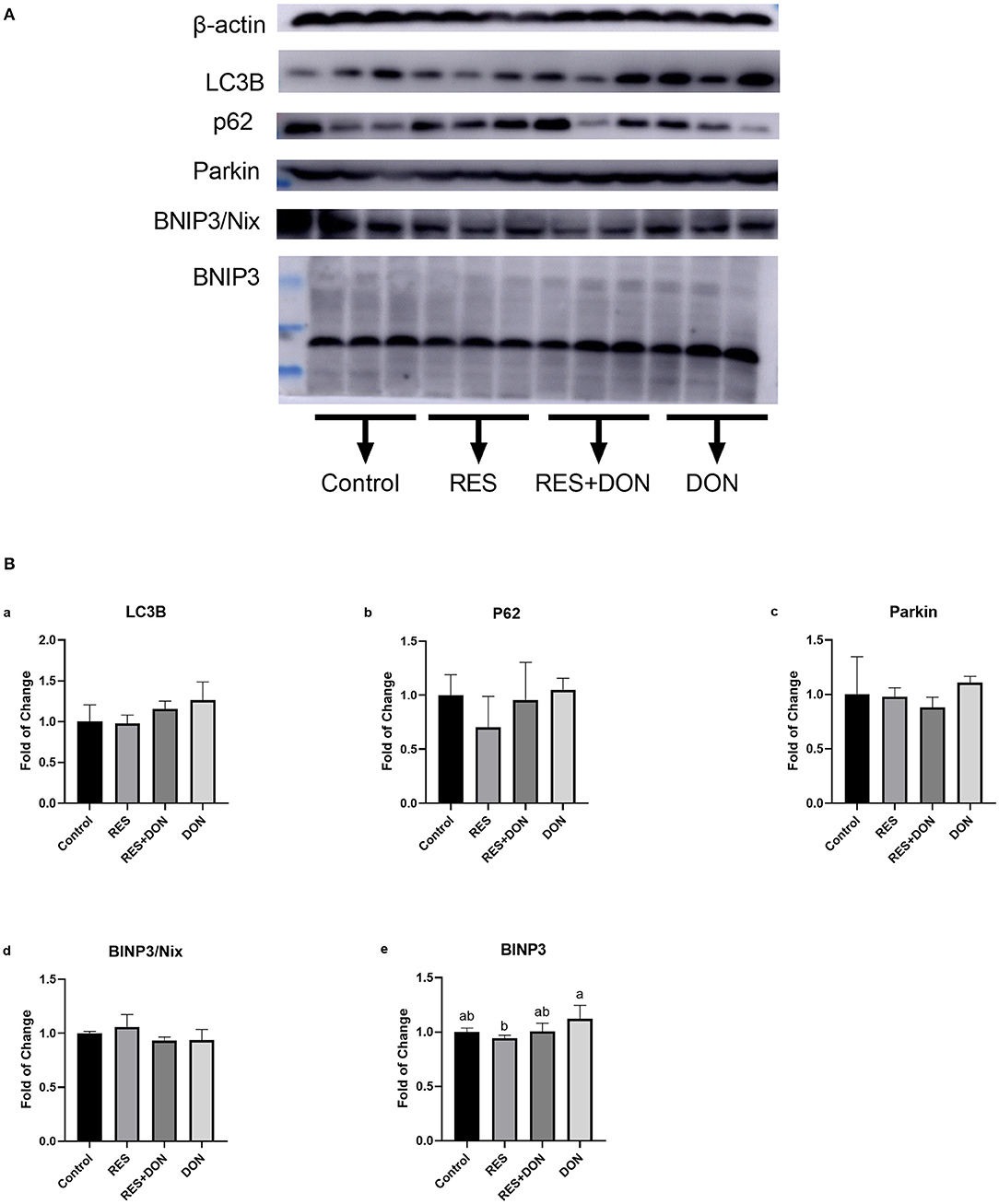
Figure 2. Dietary supplementation with RES increases the expression of mitophagy-related genes. Cells were treated with 0 (NC) or 0.5 μmol/L DON and 0 or 15 μM RES, respectively. Data were expressed as means ± SEM of at least three independent experiments. (A,B) Values with different letters are significantly different (P < 0.05).
Dietary Supplementation With RES Affects the Expression of Mitochondrial Related Genes
In order to know whether the mitochondrial is related to this study, we analyzed the protein expressions of mitochondrial related genes in ileum. The relative expression levels of C54G1, COX IV, Cyt c, HSP60, Mitofusin1, Mitofusin2, OPA1, PHB1, SDHA, SOD1, TOM20, and VDAC are shown in Figure 3. Dietary supplementation with 3.8 mg DON/kg diet (DON) decreased (P < 0.05) protein levels for Pyruvate Dehydrogenase, Cytochrome c, MFN1, OPA1, and PHB1 (P < 0.05), while supplementation with 300 mg RES increased (P < 0.05) protein levels for PHB1, SDHA, and VDAC. Supplementation with RES decreased (P < 0.05) protein levels for MFN2 and OPA1, compared with control group.
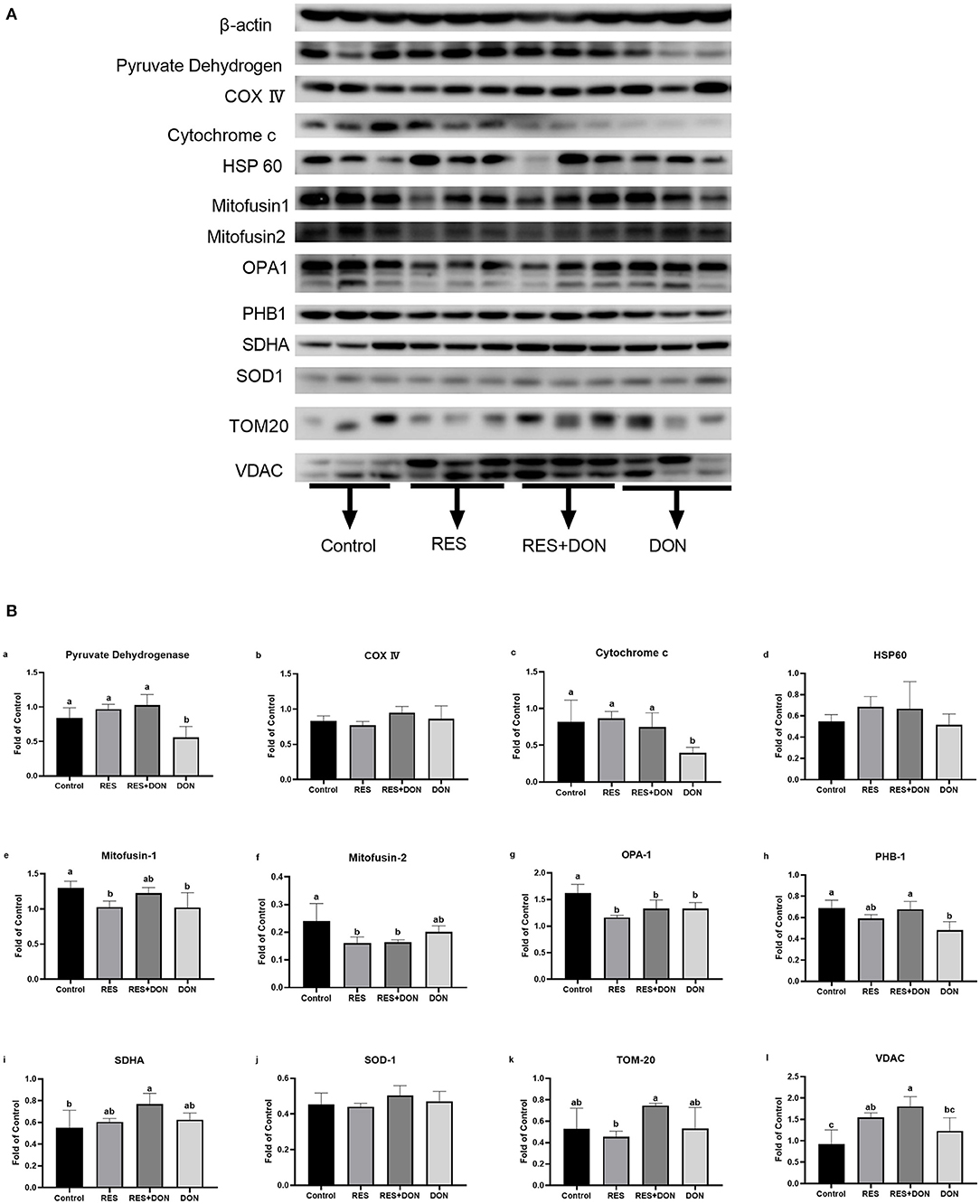
Figure 3. Dietary supplementation with RES affects the expression of mitochondrial related genes. Cells were treated with 0 (NC) or 0.5 μmol/L DON and 0 or 15 μM RES, respectively. Data were expressed as means ± SEM of at least three independent experiments. (A,B) Values with different letters are significantly different (P < 0.05).
RES Improved the Negative Effect on Mitochondrial Respiration by DON in vitro
Our results have shown that RES ameliorates the damage challenged with DON through mitophagy, then we want to find out whether RES and DON affect the mitochondrial respiration in vitro. We found that supplementation with 0.5 μmol/L or 1 μmol/L DON gradually decreased (P < 0.05) individual parameters for basal respiration, proton leak, maximal respiration, spare respiratory capacity, non-mitochondrial respiration, and ATP production in cells. While supplementation with 15 μM RES elevated the rate of spare respiratory capacity in 0.5 μmol/L DON-treated cells (P < 0.05) but not normal cells (Figure 4).
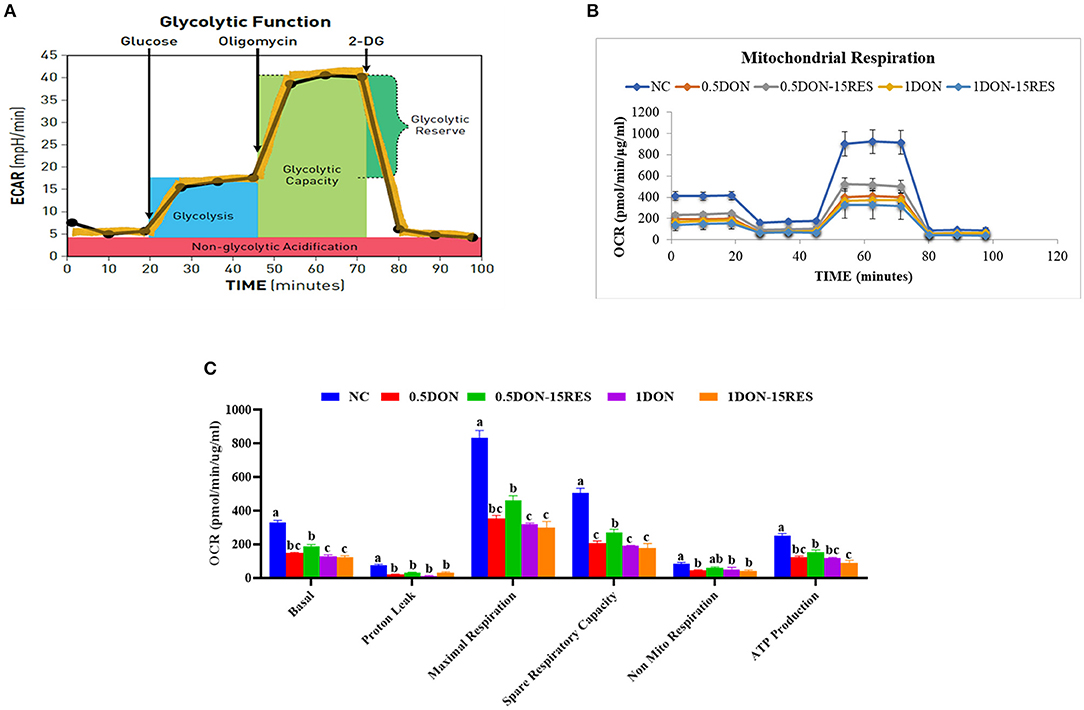
Figure 4. RES improved the negative effect on mitochondrial respiration by DON in vitro. (A) Schematic and (B) oxygen consumption rate (OCR) assessed by extracellular flux analysis. OCR was measured under basal conditions followed by the sequential addition of oligomycin (0.5 μM), FCCP (1 μM), rotenone (1 μM), or antimycin A (1 μM). Each data point represents an OCR measurement. (C) Individual parameters for basal respiration, proton leak, maximal respiration, spare respiratory capacity, nonmitochondrial respiration, and ATP production were determined. Cells were treated with 0 (NC) or 0.5μmol/L or 1μmol/L DON and 0 or 15μM RES, respectively. Data were expressed as means ± SEM of at least three independent experiments. (A–C) Values with different letters are significantly different (P < 0.05).
The results of total NAD and ATP in IPEC-J2 cells are shown in Figure 5. supplementation with 0.5 μmol/L DON and 15 μM RES increased (P < 0.05) for total NAD, while supplementation with DON or RES alone decreased (P < 0.05) for ATP. However, addition of both 0.5 μmol/L DON and 20 μM RES increased (P < 0.05) the content of ATP.
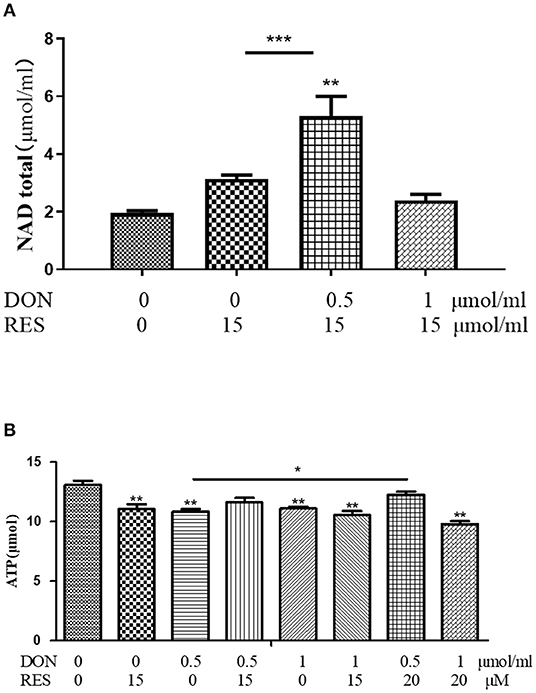
Figure 5. (A,B) Effect of DON and RES on Total NAD and ATP. Cells were treated with 0 (NC) or 0.5 μmol/L or 1 μmol/L DON and 0 or 15 μM or 20 μM RES, respectively. Data were expressed as means ± SEM of at least three independent experiments. *Values with different letters are significantly different (P < 0.05), *P < 0.05, **P < 0.01, ***P < 0.001.
RES Did Not Relieve Injury on Mitochondrial Respiration Caused by DON When Knockout Atg5
The results of mitochondrial respiration in IPEC-J2 cells are shown in Figure 6. Supplementation with 0.5 μmol/L and 1 μmol/L DON gradually decreased (P < 0.05) individual parameters for basal respiration, proton leak, maximal respiration, spare respiratory capacity, non-mitochondrial respiration, and ATP production in cells. While supplementation with 15 μM RES there is no significant difference between the mitochondrial respiration.
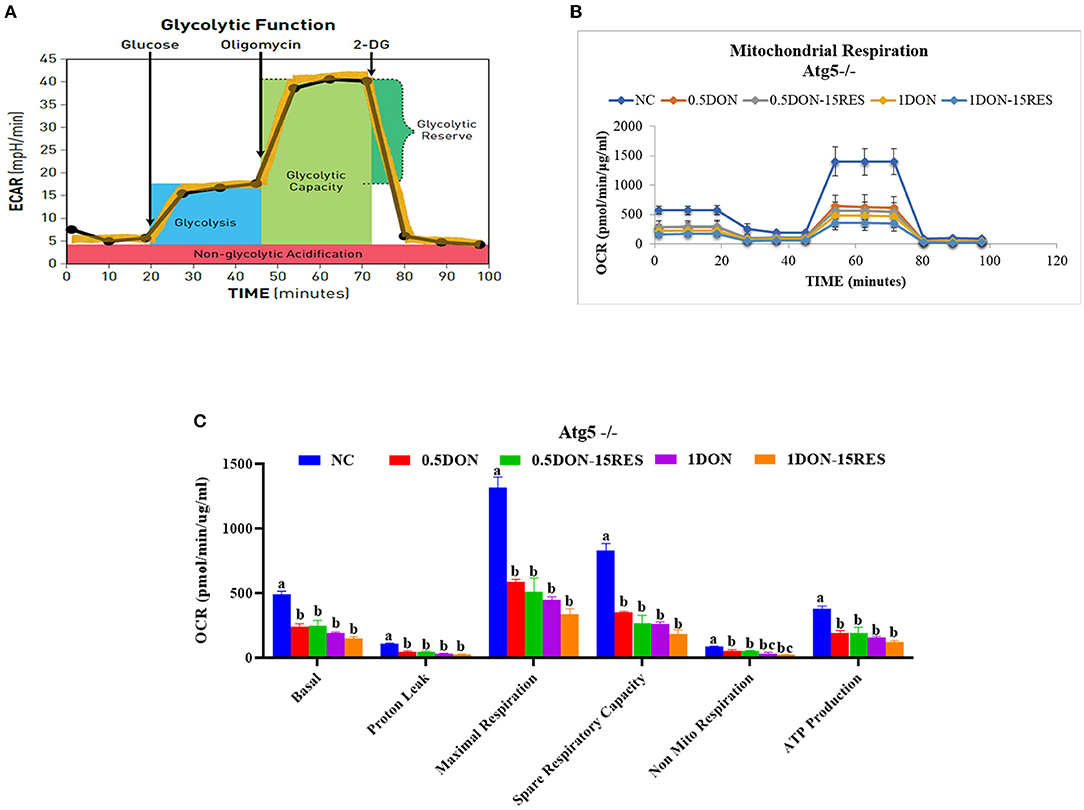
Figure 6. RES did not relieve injury on mitochondrial respiration caused by DON when knockout Atg5. (A) Schematic and (B) oxygen consumption rate (OCR) assessed by extracellular flux analysis. OCR was measured under basal conditions followed by the sequential addition of oligomycin (0.5 μM), FCCP (1 μM), rotenone (1 μM), or antimycin A (1 μM). Each data point represents an OCR measurement. (C) Individual parameters for basal respiration, proton leak, maximal respiration, spare respiratory capacity, nonmitochondrial respiration, and ATP production were determined. Cells were treated with 0 (NC) or 0.5 μmol/L or 1 μmol/L DON and 0 or 15 μM RES, respectively. Data were expressed as means ± SEM of at least three independent experiments. (A–C) Values with different letters are significantly different (P < 0.05).
Discussion
DON is a common source of grain pollution, and has a negative impact on intestinal function and reduce growth performance for animals (14). Supplementation with 1 mg kg/DON damaged the intestinal morphology and impaired intestinal mucosa and permeability, accompanied by an inflammation response (15). 0.5 μg/ml DON cultured for 6 h in IPEC-J2 cells induced oxidative stress, inflammation and apoptosis. It has been reported that RES, as an effective antioxidant, can significantly increase cellular antioxidant enzyme activity, reduce intracellular ROS content, and decrease oxidative stress in intestinal epithelial cells, indicating that RES can be used as an effective feed additive to prevent intestinal damage in livestock production (16). Consistent with their research, the results of our study suggests that RES could improve the intestinal damage by DON. It shows RES plays an important role in protecting animal health.
RES regulates biological functions, such as anti-oxidative stress, anti-inflammatory, and antibacterial through molecular regulatory mechanisms (SIRT1, Nrf2, and NF-κB, etc). Moreover, RES is effective in preventing diseases like cardiovascular disease, diabetes, neurodegeneration, and cancer (17). In addition, RES can be used as an alternative to antibacterial feed additives to regulate piglets' intestinal flora, enhance antioxidant capacity in piglets' serum, reduce oxidative stress on piglets at weaning, and significantly improve growth performance of weaned piglets (18, 19). Recent study has shown that RES could be able to mitigate diquat-induced intestinal oxidative stress in piglets through mitochondrial autophagy (10). Interestingly, RES also enhances the transcription of BNIP3, a mitochondrial autophagy-related gene, through HIF1α and AMPK, thereby maintaining mitochondrial homeostasis and alleviating high-fat-induced endothelial function impairment (20).
VDAC is a class of pore-protein ion channels located in the outer mitochondrial membrane and plays a key role in regulating metabolism and energy fluxes across the outer mitochondrial membrane: it is involved in the transport of ATP, ADP, pyruvate, malate and other metabolites (21). VDAC expression was decreased in the DON group in our experiments, and there was an imbalance in the functions regulating mitochondrial outer membrane metabolism and energy. This situation was alleviated by the addition of RES. Cytochrome c plays a role in the electron transport chain and apoptosis, and also acts as an antioxidant enzyme in mitochondria to remove superoxide and hydrogen peroxide from mitochondria (22). Our results are consistent with previous studies, in that the expression of Cytochrome c protein was significantly reduced in the DON group, suggesting that the addition of DON caused oxidative damage to the cells. The damage was alleviated by the addition of RES. PHB1 has an important role in mitochondrial function and morphology and promotes cell proliferation in mice (23, 24). Consistent with the results in this experiment, PHB1 protein expression was decreased and cell proliferation was impaired in the DON group, and the addition of RES was associated with a recovery in PHB1 expression. All these proteins are closely associated with autophagy, and because the previous RT-PCR results showed that RES alleviates intestinal damage in relation to autophagy genes, we further validated the role of RES by testing the levels of these proteins. To sum up, our results have suggested that RES might alleviate DON-induced intestinal damage by improving mitochondrial autophagy.
Cellular respiration results in the conversion of nutrients into, for example, ATP, and then the release of a series of metabolic reaction products. In eukaryotic cells, mitochondria are important organelles for cellular respiration and are involved in the process of aerobic respiration. The nutrients protein, fat and carbohydrates in aerobic respiration are degraded by pyruvate to enter the tricarboxylic acid cycle to produce energy. In this paper, we examined the changes in the oxygen consumption rate of cells under RES treatment by Seahorse and found that 15 μM RES elevated the rate of spare respiratory capacity in 0.5 μmol/L DON-treated cells (P < 0.05) but not normal cells, however there is no significant change in basal respiration, proton leak, maximal respiration, non-mitochondrial respiration. When the autophagy-related gene ATG-5 was knocked out, there were no significant changes in the indicators of mitochondrial respiratory metabolism. In this experiment, the total intracellular ATP and NAD were also tested, and it was found that the addition of 0.5 μmol/L DON and 20 μM RES significantly increase for ATP, while addition of DON or RES alone significantly decrease. This indicates that RES could relieve DON-induced mitochondrial damage through mitophagy.
Conclusions
RES alleviated the negative effects on mRNA and protein expression levels of mitophagy-related genes challenged with DON in piglets, elevated the rate of spare respiratory capacity, increased for ATP, and improved DON-induced mitochondrial damage in vitro. In conclusion, we have suggested that resveratrol would ameliorate the intestinal damage challenged with deoxynivalenol may through mitophagy in weaning piglets.
Data Availability Statement
The raw data supporting the conclusions of this article will be made available by the authors, without undue reservation.
Ethics Statement
The animal study was reviewed and approved by the Animal Care and Use Committee of Guangdong Academy of Agricultural Sciences and followed the Guidelines for the Care and Use of Animals for Research and Teaching. Written informed consent was obtained from the owners for the participation of their animals in this study.
Author Contributions
YH and HX performed experiments, analyzed data, and wrote the manuscript. LW wrote and edited the manuscript. HX supervised the project, developed the study concept, and wrote and edited the manuscript. All authors contributed to the article and approved the submitted version.
Funding
This study was jointly supported by the National Natural Science Foundation of China (31902172), Special fund for scientific innovation strategy-construction of high levelhigh-level Academy of Agriculture Science (R2020PY-JG009, R2017YJ-YB1004, R2018PY-JC001, and R2018PY-QF001), China Agriculture Research System of MOF and MARA; the Project of Swine Innovation Team in Guangdong Modern Agricultural Research System (2021KJ126), China.
Conflict of Interest
The authors declare that the research was conducted in the absence of any commercial or financial relationships that could be construed as a potential conflict of interest.
Publisher's Note
All claims expressed in this article are solely those of the authors and do not necessarily represent those of their affiliated organizations, or those of the publisher, the editors and the reviewers. Any product that may be evaluated in this article, or claim that may be made by its manufacturer, is not guaranteed or endorsed by the publisher.
Supplementary Material
The Supplementary Material for this article can be found online at: https://www.frontiersin.org/articles/10.3389/fvets.2021.807301/full#supplementary-material
References
1. Tutelyan VA. Deoxynivalenol in cereals in Russia. Toxicol Lett. (2004) 153:173–9. doi: 10.1016/j.toxlet.2004.04.042
2. Xiao H, Wu MM, Tan BE, Yin YL Li TJ, Xiao DF Li L. Effects of composite antimicrobial peptides in weanling piglets challenged with deoxynivalenol: I. Growth performance, immune function, and antioxidation capacity. J Anim Sci. (2013) 91:4772–80. doi: 10.2527/jas.2013-6426
3. Xiao H, Tan BE, Wu MM, Yin YL Li TJ, Yuan DX Li L. Effects of composite antimicrobial peptides in weanling piglets challenged with deoxynivalenol: II. Intestinal morphology and function. J Anim Sci. (2013) 91:4750–6. doi: 10.2527/jas.2013-6427
4. Wu L, Wang W, Yao K, Zhou T, Yin J, Li T, et al. Effects of dietary arginine and glutamine on alleviating the impairment induced by deoxynivalenol stress and immune relevant cytokines in growing pigs. PLoS ONE. (2013) 8:e69502. doi: 10.1371/journal.pone.0069502
5. Wu M, Xiao H, Ren W, Yin J, Hu J, Duan J, et al. An NMR-based metabolomic approach to investigate the effects of supplementation with glutamic acid in piglets challenged with deoxynivalenol. PLoS ONE. (2014) 9:e113687. doi: 10.1371/journal.pone.0113687
6. Pinton A, Faraut T, Yerle M, Gruand J, Pellestor F. Ducos A. Comparison of male and female meiotic segregation patterns in translocation heterozygotes: a case study in an animal model (Sus scrofa domestica L). Hum Reprod. (2005) 20:2476–82. doi: 10.1093/humrep/dei067
7. Fremont L. Biological effects of resveratrol. Life Sci. (2000) 66:663–73. doi: 10.1016/S0024-3205(99)00410-5
8. Rodgers JT, Lerin C, Haas W, Gygi SP, Spiegelman BM, Puigserver P. Nutrient control of glucose homeostasis through a complex of PGC-1alpha and SIRT1. Nature. (2005) 434:113–8. doi: 10.1038/nature03354
9. Wang H, Jiang T, Li W, Gao N, Zhang T. Resveratrol attenuates oxidative damage through activating mitophagy in an in vitro model of Alzheimer's disease. Toxicol Lett. (2018) 282:100–8. doi: 10.1016/j.toxlet.2017.10.021
10. Cao S, Shen Z, Wang C, Zhang Q, Hong Q, He Y, et al. Resveratrol improves intestinal barrier function, alleviates mitochondrial dysfunction and induces mitophagy in diquat challenged piglets(1). Food Funct. (2019) 10:344–54. doi: 10.1039/C8FO02091D
11. Yang J, Zhu C, Ye J, Lv Y, Wang L, Chen Z, et al. Protection of Porcine Intestinal-Epithelial Cells from Deoxynivalenol-Induced Damage by Resveratrol via the Nrf2 Signaling Pathway. J Agric Food Chem. (2019) 67:1726–35. doi: 10.1021/acs.jafc.8b03662
12. Li G, Li J, Tan B, Wang J, Kong X, Guan G, et al. Characterization and regulation of the amino acid transporter SNAT2 in the small intestine of piglets. PLoS One. (2015) 10:e0128207. doi: 10.1371/journal.pone.0128207
13. Tang Y, Li J, Li F, Hu CA, Liao P, Tan K, et al. Autophagy protects intestinal epithelial cells against deoxynivalenol toxicity by alleviating oxidative stress via IKK signaling pathway. Free Radic Biol Med. (2015) 89:944–51. doi: 10.1016/j.freeradbiomed.2015.09.012
14. Prelusky DB, Gerdes RG, Underhill KL, Rotter BA, Jui PY, Trenholm HL. Effects of low-level dietary deoxynivalenol on haematological and clinical parameters of the pig. Nat Toxins. (1994) 2:97–104. doi: 10.1002/nt.2620020302
15. Xu X, Yan G, Chang J, Wang P, Yin Q, Liu C, et al. Astilbin ameliorates deoxynivalenol-induced oxidative stress and apoptosis in intestinal porcine epithelial cells (IPEC-J2). J Appl Toxicol. (2020) 40:1362–72. doi: 10.1002/jat.3989
16. Zhuang Y, Wu H, Wang X, He J, He S, Yin Y. Resveratrol Attenuates Oxidative Stress-Induced Intestinal Barrier Injury through PI3K/Akt-Mediated Nrf2 Signaling Pathway. Oxid Med Cell Longev. (2019) 2019:7591840. doi: 10.1155/2019/7591840
17. Jang M, Cai L, Udeani GO, Slowing KV, Thomas CF, Beecher CW, et al. Cancer chemopreventive activity of resveratrol, a natural product derived from grapes. Science. (1997) 275:218–20. doi: 10.1126/science.275.5297.218
18. Ahmed ST, Hossain ME, Kim GM, Hwang JA Ji H, Yang CJ. Effects of resveratrol and essential oils on growth performance, immunity, digestibility and fecal microbial shedding in challenged piglets. Asian-Australas J Anim Sci. (2013) 26:683–90. doi: 10.5713/ajas.2012.12683
19. Zhang H, Chen Y, Chen Y, Ji S, Jia P, Li Y, et al. Comparison of the protective effects of resveratrol and pterostilbene against intestinal damage and redox imbalance in weanling piglets. J Anim Sci Biotechnol. (2020) 11:52. doi: 10.1186/s40104-020-00460-3
20. Li C, Tan Y, Wu J, Ma Q, Bai S, Xia Z, et al. Resveratrol Improves Bnip3-Related Mitophagy and Attenuates High-Fat-Induced Endothelial Dysfunction. Front Cell Dev Biol. (2020) 8:796. doi: 10.3389/fcell.2020.00796
21. Blachly-Dyson E, Forte M VDAC. channels. IUBMB Life. (2001) 52:113–8. doi: 10.1080/15216540152845902
22. Bowman SE, Bren KL. The chemistry and biochemistry of heme c: functional bases for covalent attachment. Nat Prod Rep. (2008) 25:1118–30. doi: 10.1039/b717196j
23. Tatsuta T, Model K, Langer T. Formation of membrane-bound ring complexes by prohibitins in mitochondria. Mol Biol Cell. (2005) 16:248–59. doi: 10.1091/mbc.e04-09-0807
Keywords: piglets, resveratrol, deoxynivalenol, mitophagy, intestinal function
Citation: Huang Y, Zheng C, Song B, Wang L, Xiao H and Jiang Z (2022) Resveratrol Ameliorates Intestinal Damage Challenged With Deoxynivalenol Through Mitophagy in vitro and in vivo. Front. Vet. Sci. 8:807301. doi: 10.3389/fvets.2021.807301
Received: 02 November 2021; Accepted: 09 December 2021;
Published: 13 January 2022.
Edited by:
Tarique Hussain, Nuclear Institute for Agriculture and Biology, PakistanReviewed by:
Jiashun Chen, Hunan Agricultural University, ChinaXin Wu, Chinese Academy of Sciences (CAS), China
Elsayed Metwally, Suez Canal University, Egypt
Muhammad Saleem Kalhoro, Sindh Agriculture University, Pakistan
Copyright © 2022 Huang, Zheng, Song, Wang, Xiao and Jiang. This is an open-access article distributed under the terms of the Creative Commons Attribution License (CC BY). The use, distribution or reproduction in other forums is permitted, provided the original author(s) and the copyright owner(s) are credited and that the original publication in this journal is cited, in accordance with accepted academic practice. No use, distribution or reproduction is permitted which does not comply with these terms.
*Correspondence: Hao Xiao, xiaohao@gdaas.cn
†These authors have contributed equally to this work