- State Key Laboratory of Animal Nutrition, College of Animal Science and Technology, China Agricultural University, Beijing, China
Tea tree oil (TTO) exerts key roles in improving growth performance of pigs. However, knowledge is limited regarding comparative effects of Encp TTO and Un-encp TTO supplementation on growth performance of pigs. A study determined the effects of TTO or its capsulation on growth performance, antioxidant capacity, and intestinal microbiome of weaned pigs. A total of 144 healthy pigs (8.5 ± 0.24 kg) were subjected to four treatments for a 28-d trial with six replicates per treatment and six pigs per pen: negative control, NC; positive control, PC (antibiotic supplemented); Un-encp TTO (supplemented with unencapsulated TTO); Encp TTO (supplemented with encapsulated TTO). NC, TTO, and PC treatments were compared with regard to improved average daily gain (ADG), average daily feed intake (ADFI), feed conversion rate, nutrient digestibility, and intestinal morphology (p < 0.05) and decreased diarrhea rate. TTO- and PC-treated pigs had higher levels of serum superoxide dismutase, glutathione peroxidase, and immunoglobulin G; lower levels of liver aspartate aminotransferase and alanine aminotransferase; and improved concentrations of interleukin 10 (IL-10), tumor necrosis factor α, and IL-1β (p < 0.05). TTO- and PC-treated pigs had higher abundance of beneficial bacterial species Subdoligranulum and lower abundance of diarrhea associated species Escherichia–Shigella in cecal and colonic digesta (p < 0.05). Encapsulation of TTO preserved more activities of TTO than its unencapsulated counterpart by showing higher ADG, ADFI, and feed conversion rate during day 1 (d1) to d14 (p < 0.05) and tended to lower diarrhea rate (p = 0.083) and improve villous height/crypt depth (VH/CD) ratio (p = 0.089) in jejunum. Encapsulation of TTO also improved antioxidant indexes and decreased liver injury and inflammation accordingly (p < 0.05). Encapsulated TTO-treated pigs had higher abundance of beneficial Clostridium_sensu_stricto_1 and lower the abundance of harmful Escherichia–Shigella in cecal and colonic digesta (p < 0.05). Our results demonstrated TTO benefits on improving growth performance of weaned pigs and further proved that encapsulation of TTO was superior to its unencapsulated counterpart at multiples. Encapsulated TTO was similar to the PC group and could be potentially an alternative of feed antibiotics for weaned pigs.
Introduction
Under weaning stress, piglets often experience diarrhea, digestion dysfunction, low utilization rate of feed, suppressed immune response, and changed composition of intestinal microbes (1). Supplementing diets with antibiotics is one of the main means to reduce diarrhea and promote growth in newly weaned piglets. However, routine use of antibiotics may pose a significant threat to human health (2). Consequently, many scientists seek to develop novel alternatives to antibiotics. Essential oils are a potential alternative as they are residue-free and broad-spectrum and are generally recognized as safe and beneficial (3, 4).
Tea tree oil (TTO) is an essential oil steam-distilled from Melaleuca alternifolia (5). Tea is widely planted in tropical and subtropical areas of China (6). Tea oil production reached 2.61 million metric tons in 2019 in China (7). TTO contains more than 100 compounds, including α-pinene, terpene-4-ol, linalool, and α-terpenol, which have antibacterial activities (8). Dietary TTO exhibited prophylactic effects on weaned pigs by improving the immune response and growth performance (9–11).
Use of TTO as a feed additive presents many challenges. TTO is unstable and can be damaged by environmental factors such as oxygen, heat, light, and humidity when stored (12). It has unstable functional groups in which oxygen acts as an oxidizing agent for alcohols and aldehydes, light radiation changes unsaturated monoterpenic and sesquiterpenic hydrocarbons, and water plays a role in ether and ester hydrolysis (13). For example, Rudback et al. (14) demonstrated that α-terpinene, an antioxidant in TTO, autoxidizes rapidly to allergens on exposure to air. TTO is a lipophilic liquid that contains many volatile compounds, such as alcohols, ethers, and esters in TTO, which produce a smell that may affect the palatability of diets for piglets (8). However, the effects of TTO on feed intake of weaned pigs were inconsistent, ranging from 3% depression to 19% increase (15).
New encapsulation of TTO is able to overcome above challenges by coating particles or droplets in a matrix to give a physical barrier between the core compound and the surroundings. Therefore, encapsulation protects them from environmental damage and controls their release (16–18). We hypothesized that supplemental encapsulation of TTO might exert positive effects on feed intake, and may have better effects on growth performance, immune response, and intestinal microflora of weaned pigs compared with unencapsulated TTO.
To the best of our knowledge, no studies have specifically reported the effective difference introduced by encapsulation process on TTO activity in weaned pigs. Therefore, the aim of the present study was to (1) evaluate the effects of TTO (regardless of encapsulation) on growth performance, diarrhea rate, immune response, antioxidative activity, and bacteria composition in gastrointestinal tract of weaned pigs; (2) compare the different effect between encapsulated and unencapsulated TTO on the above indexes in weaned pigs.
Materials and Methods
This experiment was carried out at the National Feed Engineering Technology Research Center at the Ministry of Agriculture Feed Industry Center Animal Testing Base (Hebei, China). All procedures used in this study were conducted in accordance with Chinese Guidelines for Animal Welfare and approved by the China Agricultural University Institutional Animal Care and Use Committee (201905610420678).
Preparation of Encapsulated TTO
Commercial TTO (1 kg) was mixed with phosphatidyl choline (1 kg) isolated from soybean, and an antioxidant (0.2 kg vitamin E) in n-hexane solution (2 L; Figure 1; Hebei Chenguang Biotech Group Co., Ltd.). The solution was heated to 50°C and stirred for 3 h followed by vacuum drying to form 1 layer on TTO particles. Carrier material was prepared by mixing lactose (4 kg) and gum arabic (3.75 kg) in water (25 L, 50°C). This carrier solution was emulsified at 9,600 g. Dried 1-layer TTO particles were mixed with the carrier solution and further emulsified at 9,600 for 30 min and homogenized at a pressure of 20 to 60 MPa for two to three times. To this second step, two-layer encapsulated TTO was produced. Finally, another carrier, mixture of maltodextrin and cornstarch (1:2 wt/wt), was spray-dried on two-layer TTO and sieved (60-mesh screen) forming encapsulated three-layer TTO particles. The diameter of the one-layer particle composed of TTO and phosphatides was from 2 to 20 μm, and the diameter of the three-layer encapsulated TTO was from 75 to 250 μm.
Gas Chromatography–Mass Spectrometry Analysis of TTO
Commercial TTO was obtained from Hebei Chenguang Biotech Group Co., Ltd. (Handan, China), and extraction was performed by the steam distillation technique, utilizing leaves of tea trees. The analysis was conducted using gas chromatography–mass spectrometry (QP2010, Shimadzu Company, Japan) according to Adams (19). The ionization voltage, mass range, and the condition of GC condition were set according to previously described methods (20). Peaks were identified that corresponded to the data from mass spectra library (National Institute of Standard and Technology). Finally, quantitative determination was conducted based on the peak area integration.
Animals, Diets, and Experimental Design
Piglets (n = 144, Duroc × Landrace × Large white, weaned at 28 days after birth) with an average initial weight of 8.5 ± 0.24 kg were used in this experiment. This study designed six replicates per treatment with six piglets (three male and three female piglets) per pen, and the experiment was separated into phase 1 (days 1–14) and phase 2 (days 15–28). Dietary treatments included basal diet (NC), basal diet supplemented with 75 mg/kg aureomycin (PC), basal diet supplemented with 0.4% unencapsulated TTO (Un-encp TTO; unencapsulated TTO was sprayed on porous starch and dried at 20°C for 30–50 min), and basal diet supplemented with 0.4% encapsulated TTO (Encp TTO; the encapsulation strategy was described in “preparation of encapsulated TTO”). The actual content of TTO in each product was 11% (Hebei Chenguang Biotech Group Co., Ltd.). We added 0.4% encapsulated or unencapsulated TTO to the basic diet, so the actual value of TTO among treatments was 0.04%. All diets were formulated to meet or exceed the requirements suggested by National Research Council (NRC, 2012) estimates of the nutrient requirements of weaned piglets (Table 1). Chromic oxide (0.3%) was added to each treatment diet as an indigestible marker to calculate the apparent total tract digestibility (ATTD) of nutrients. Pigs were housed in pens that measured 1.2 ×2.1 m and were equipped with stainless-steel feeder and one nipple waterer with slatted plastic floors. The environmental temperature was controlled and maintained at 24–26°C. Pigs had ad libitum access to feed and water.
Each piglet was weighed on days 0, 14, and 28, and feed consumption was also recorded on a pen basis to determine average daily gain (ADG), average daily feed intake (ADFI) and the feed to gain ratio (F:G). Fecal consistency was assessed visually three times per day by observers blinded to treatments according to previously described methods (21). The scoring system was applied to determine the rate of diarrhea as following: 0 = firm and normally shaped, 1 = soft (retained some shape), 2 = brown liquid, 3 = frequent passage of watery feces (22). The occurrence of diarrhea was defined as a continuous 2-day fecal score at level 2 or 3. Diarrhea rate was calculated using the following formula. Diarrhea rate (%) = (number of piglets with diarrhea × diarrhea days) / (number of piglets × total observational days) ×100%.
Sample Collection
On the morning of days 14 and 28, one pig closest to the average body weight in each pen was selected to collect blood samples (8 mL) via the anterior vena cava puncture into vacuum tubes following a 12-h fasting. Serum samples were separated by centrifugation at 3,000 g at 4°C for 15 min and stored at −20°C until further analysis.
On the morning of day 28, six pigs per treatment group were randomly selected and were euthanized by electric shock and then bled. The selected pigs were from different pens, and their body weights were close to the average body weight of pigs in each pen. The small intestine was dissected from the mesentery and immediately placed on ice. Segments (2 cm in length) of the middle parts of the duodenum, jejunum, and ileum without any digesta were fixed in 10% neutral-buffered formalin for subsequent morphological examination. Liver tissues were collected and quickly washed with ice-cold saline solution and then homogenized in ice-cold phosphate buffer (phosphate-buffered saline: 1:9 wt/vol, pH 7.4) and centrifuged at 2,000 g for 20 min. The supernatant was collected and stored at −80°C for inflammatory cytokine analysis. Liver microsomes were prepared by differential centrifugation (11,000 and 2 ×100,000 g) in a Tris/KCl buffer (20 mM, pH 7.4) and stored at −80°C for cytochrome P450 (P450) analysis (23).
Fecal samples for determination of nutrient digestibility were collected from each pen during the last 3 days (days 25–27) and were immediately stored at −20°C. At the end of the experiment, the 3-day collection of fecal samples was pooled by pen and then dried in a forced-air drying oven at 65°C for 72 h. Feed and dried fecal samples were ground finely to pass through a 1-mm screen (40-mesh) before chemical analysis. For analysis of gut microbiota, digesta in the cecum and colon of slaughter piglets were sampled (each sample from one pig per pen) in sterile tubes on day 28 and immediately immersed in liquid nitrogen and then stored at −80°C (24).
Apparent Total Tract Digestibility of Feed Nutrients
Dry matter (DM; method 934.01), crude protein (CP; method 990.03), and ether extract (EE; method 920.39) of feed and fecal samples were determined according to the Association of Official Analytical Chemists (25). Neutral detergent fiber (NDF) concentration of these samples were determined according to the method of McCarthy et al. (26). The content of chromium in feed and fecal samples was determined by atomic absorption spectrophotometer (Hitachi Z-5000, Tokyo, Japan) (27).
Serum Antioxidant, Immune, and Biochemical Parameter Analysis
In the serum, concentrations of glutathione peroxidase (GSH-Px) and superoxide dismutase (SOD) were determined according to the manufacturer's instructions using commercial kits (Jiancheng Biochemical Reagent Co., Nanjing, China). The content of serum immunoglobulins A, M, and G (IgA, IgM, and IgG) were measured using commercially available enzyme-linked immunosorbent assay (ELISA) kits following manufacturer's instructions (Nanjing Jiancheng Bioengineering Institute, Nanjing, China). Serum biochemical parameters including aspartate aminotransferase (AST), alanine aminotransferase (ALT), and alkaline phosphatase (ALP) were measured using corresponding commercially available kits (BioSino Bio-technology and Science Inc., Beijing, China).
Cytokine Measurement and Enzyme Assays
In serum and liver, concentrations of interleukin 10 (IL-10), tumor necrosis factor α (TNF-α), and IL-1β were determined using the corresponding commercially available ELISA kits (Beijing Sino-UK Institute of Biological Technology, Beijing, China). Concentrations of the microsomal cytochromes P450 were determined using the spectrophotometric method of Omura and Sato (28).
Intestinal Morphology
Intestinal morphology was characterized according to the methods of our previous study (22). Briefly, fixed intestinal samples were dehydrated and embedded in paraffin. Embedded samples were cut into slices (6 μm thick) and stained with hematoxylin-eosin. Then six villous height (VH, determined as the distance between the crypt opening to the end of the villous), crypt depth (CD, measured from the crypt–villous junction to the base of the crypt), and VH/CD (VH/CD) ratio were measured under a light microscope (Nikon, Tokyo, Japan) at a 40 × magnification and were analyzed using Image-Pro Plus 6.0 software.
Microbiota Analysis
Microbial DNA was extracted from cecal and colonic digesta using the E.Z.N.A.® stool DNA kit (Omega Bio-tek, Norcross, GA, USA) according to the manufacturer's instructions. Markers and adaptors-linked universal primers 338F (ACTCCTACGGGAGGCAGCAG) and 806R (GGACTACHVGGGTWTCTAAT) targeting the V3–V4 region were used to amplify microbial 16S rRNA. The polymerase chain reaction (PCR) amplification of 16S rRNA gene was performed as follows: 95°C for 3 min, followed by 25 cycles at 95°C for 30 s, 55°C for 30 s, and 72°C for 45 s and a final extension at 72°C for 10 min (29). The PCR product was extracted with 2% agarose gel and purified using the AxyPrep DNA Gel Extraction Kit (Axygen Biosciences, Union City, CA, USA). Purified amplicons were pooled in equimolar proportions and paired-end sequenced (2 ×300) on an Illumina MiSeq platform (Illumina, San Diego, CA, USA). The processing of sequencing data was conducted as previously described (30). The data presented in the study are deposited in the NCBI repository, accession number PRJNA677843. Operational taxonomic units (OTUs) with a 97% similarity cutoff (31) were clustered using UPARSE (version 7.1, http://drive5.com/uparse/), and chimeric sequences were identified and removed. Taxonomy of each OTU representative sequence was analyzed by RDP Classifier (http://rdp.cme.msu.edu/) against the 16S rRNA database (Silva SSU128) using confidence threshold of 0.7.
Statistical Analysis
GLM procedure of SAS (SAS Institute Inc., Cary, NC, USA) was used for data analysis. Each pen of pigs was considered as an experimental unit. Diarrhea rates were analyzed by χ2 test (SAS Institute Inc., Cary, NC, USA). Planned orthogonal contrasts were used to evaluate the overall effect of TTO ([NC] vs. [Un-encp TTO, Encp TTO]) or encapsulation effect ([Un-encp TTO] vs. [Encp TTO]). All data were checked for normal distribution and homogeneity of variance using the Shapiro–Wilk normality test and Bartlett test, respectively. The differences and composition of bacterial abundance were analyzed by Kruskal–Wallis rank-sum test (29). p < 0.05 was considered statistically significant, and 0.05 ≤ p < 0.10 was indicative of a differential trend.
Results
Composition of TTO
The total yield of volatile compounds from encapsulated TTO was 2.74% ± 0.22%. Fourteen compounds from the encapsulated TTO were detected by gas chromatography–mass spectrometry (Table 2). Two volatile compounds, terpinen-4-ol (50.31%) and γ-terpinene (20.06%), accounted for the majority of volatiles measured.
Effect of TTO on Growth Performance and Diarrhea Rate
Regardless of encapsulation, compared with the negative control treatment, TTO and positive control treatment increased (p < 0.05) ADG at phase 1 (days 0–14), phase 2 (days 15–28), and overall (days 0–28) and increased (p < 0.05) ADFI at phase 2 and overall (Table 3). TTO and PC treatment decreased F:G (p < 0.01) and diarrhea rate (p < 0.01) compared with the NC treatment. In addition, there were no differences in growth performance between TTO treatment and PC treatment. Concerning the encapsulation effect, compared with the Un-encp TTO treatment, the treatment of Encp TTO had higher ADG at phase 1, phase 2, and overall phases (p < 0.05) and higher ADFI in phase 2 and overall phases (p < 0.05). Encp TTO treatment had lower F:G at phase 1 (p < 0.05) and tended to have lower diarrhea rate compared with Un-encp TTO treatment (p = 0.083).
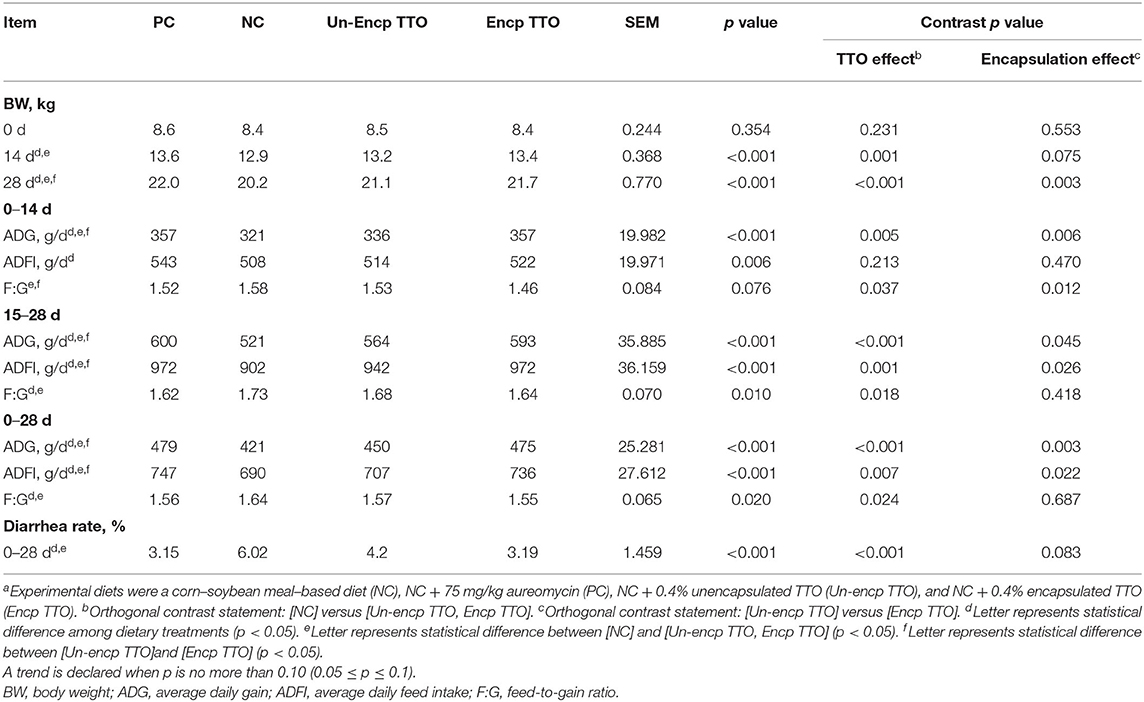
Table 3. Effects of TTO and encapsulation on growth performance and diarrhea rate of weaned pigs (n = 6)a.
Effect of TTO on Apparent Total Tract Digestibility of Nutrient
TTO treatment (Un-encp TTO and Encp TTO) increased ATTD of CP, EE, and DM compared with the negative control treatment (p < 0.05, Table 4). TTO treatment tended to increase ATTD of EE (p = 0.089) compared with the PC treatment. There were no differences in ATTD of DM, CP, and NDF between TTO treatment and PC treatment. Encapsulation of TTO (Encp TTO vs. Un-encp TTO) had no significant effect on ATTD of these nutrients.
Effect of TTO on Small Intestinal Morphology
Pigs fed the TTO and positive control diets had higher VH and VH/CD ratio in the jejunum than those fed NC diet (p < 0.05, Table 5). The VH, CD, and VH/CD ratio in the duodenum and ileum did not differ among NC, PC, and TTO treatments (Un-encp TTO and Encp TTO). Encp TTO tended to increase the VH/CD ratio (p = 0.089) in the jejunum compared with the Un-encp TTO treatment.
Effect of TTO on Antioxidant and Immunoglobulin Indexes in Serum
Serum SOD, GSH-Px, and IgG in piglets fed TTO and PC diets were higher than the NC treatment (p < 0.05, Table 6). Serum IgG was greater in pigs fed the TTO diet than those fed the PC diet. Compared with the Un-encp TTO and PC treatment, piglets fed with Encp TTO showed higher serum SOD and GSH-Px activities (p < 0.05).
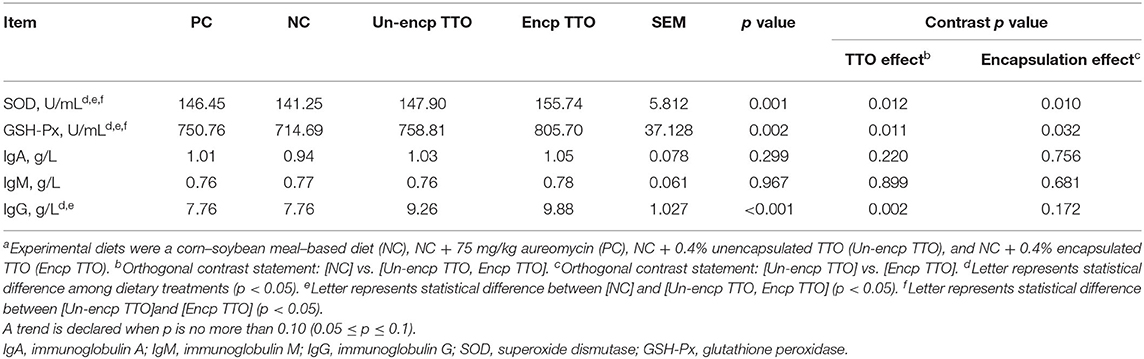
Table 6. Effects of TTO and encapsulation on serum antioxidant and immune indexes of weaned pigs (n = 6)a.
Effect of TTO on Serum Enzyme Activities and Inflammatory Cytokines of Weaned Pigs
Pigs fed TTO exhibited lower serum concentrations of ALT and AST compared with pigs fed the NC and PC treatments (p < 0.05, Table 7). In addition, supplementation with TTO increased serum level of IL-10 (p < 0.05) and tended to decrease the level of TNF-α (p > 0.05) compared with NC and PC treatments. Compared with pigs fed Un-encp TTO and PC, Encp TTO decreased concentrations of AST and increased concentration higher level of IL-10 (p < 0.05).
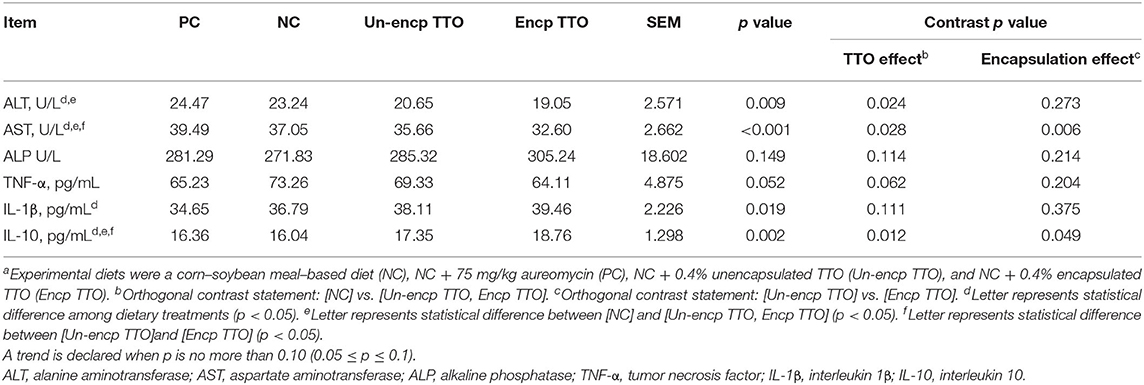
Table 7. Effects of TTO and encapsulation on serum enzyme activities and inflammatory cytokines of weaned pigs (n = 6)a.
Effect of TTO on Inflammatory Cytokines and P450 in Liver of Weaned Pigs
Pigs fed TTO treatments had lower levels of IL-1β (p = 0.004) in the liver than those in the negative control treatment (Figures 2B,C). Compared with the Un-encp TTO and positive control treatments, lower level of IL-1β and higher level of IL-10 were observed in pigs fed Encp TTO (p < 0.05). There were no significant differences in concentration of TNF-α and P450 in liver among experimental treatments (Figures 2A,D).
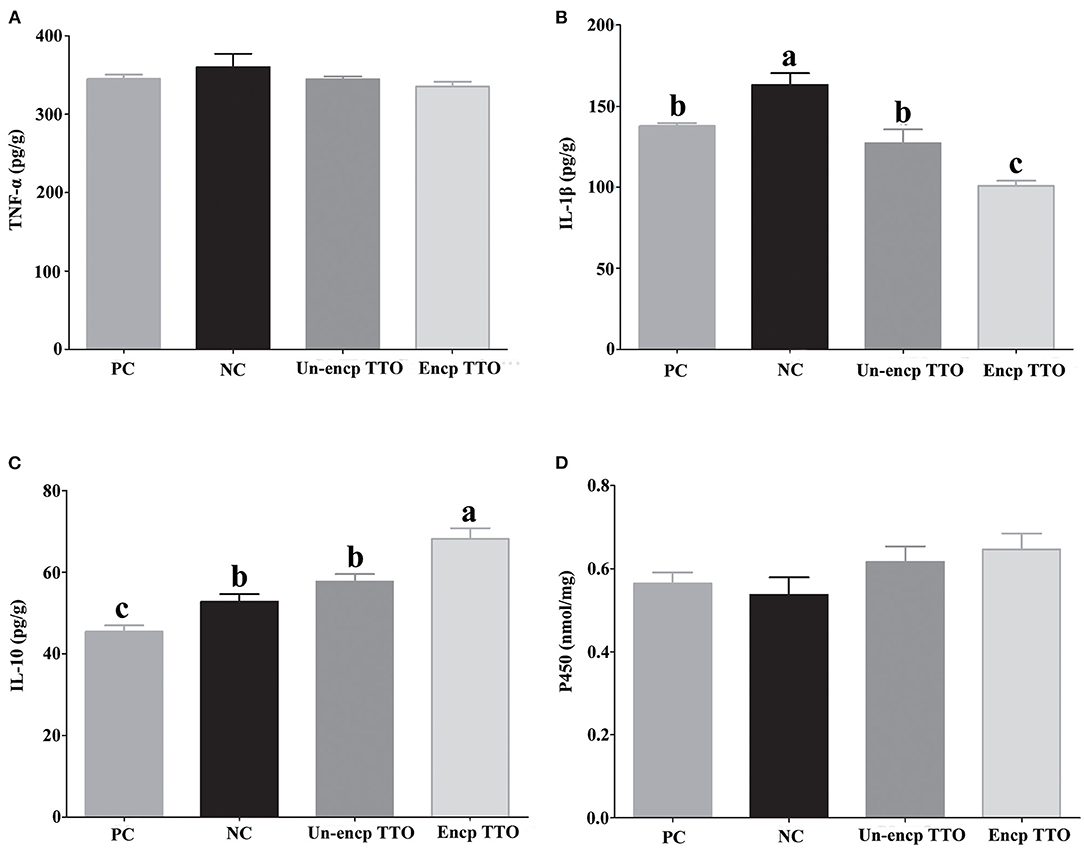
Figure 2. Expression of tumor necrosis factor α (TNF-α), interleukin 1β (IL-1β), and interleukin 10 (IL-10), contents of cytochrome P450 (P450) in livers of weaned pigs (n = 6). Experimental diets were control diet (NC), NC + 75 mg/kg aureomycin (PC), NC + NC + 0.4% unencapsulated TTO (Un-encp TTO), and NC + 0.4% encapsulated TTO (Encp TTO). Values are means, error bars indicate SEM, and different letters above a bar denote the significant difference between treatments (p < 0.05). (A) Relative expression of TNF-α. (B) Relative expression of IL-1β. (C) Relative expression of IL-10. (D) Contents of P450. Statistical analysis was performed using one-way ANOVA.
Effect of TTO on Intestinal Microbiota Composition
We observed a shift in the microbial composition of cecal and colonic digesta (Figure 3). The dominant bacteria phyla across treatments were mainly Firmicutes, Bacteroidetes, and Proteobacteria. At the genus level, abundance of Subdoligranulum in cecal and colonic microbial communities in the TTO (Un-encp TTO and Encp TTO) and PC treatments was higher than that in the NC treatment (Figures 3C,D), whereas Escherichia–Shigella were lower (p < 0.05, Figures 4B,D). Compared with the Un-encp TTO and PC treatments, pigs fed the Encp TTO diets had higher relative abundance of Clostridium_sensu_stricto_1 in the colonic digesta (Figure 3D) and lower relative abundance of Escherichia–Shigella (Figure 4B) in the cecal and colonic digesta (p < 0.05). Moreover, the relative abundance of Streptococcus in cecum and colon was not altered among all treatments (Figures 4A,C).
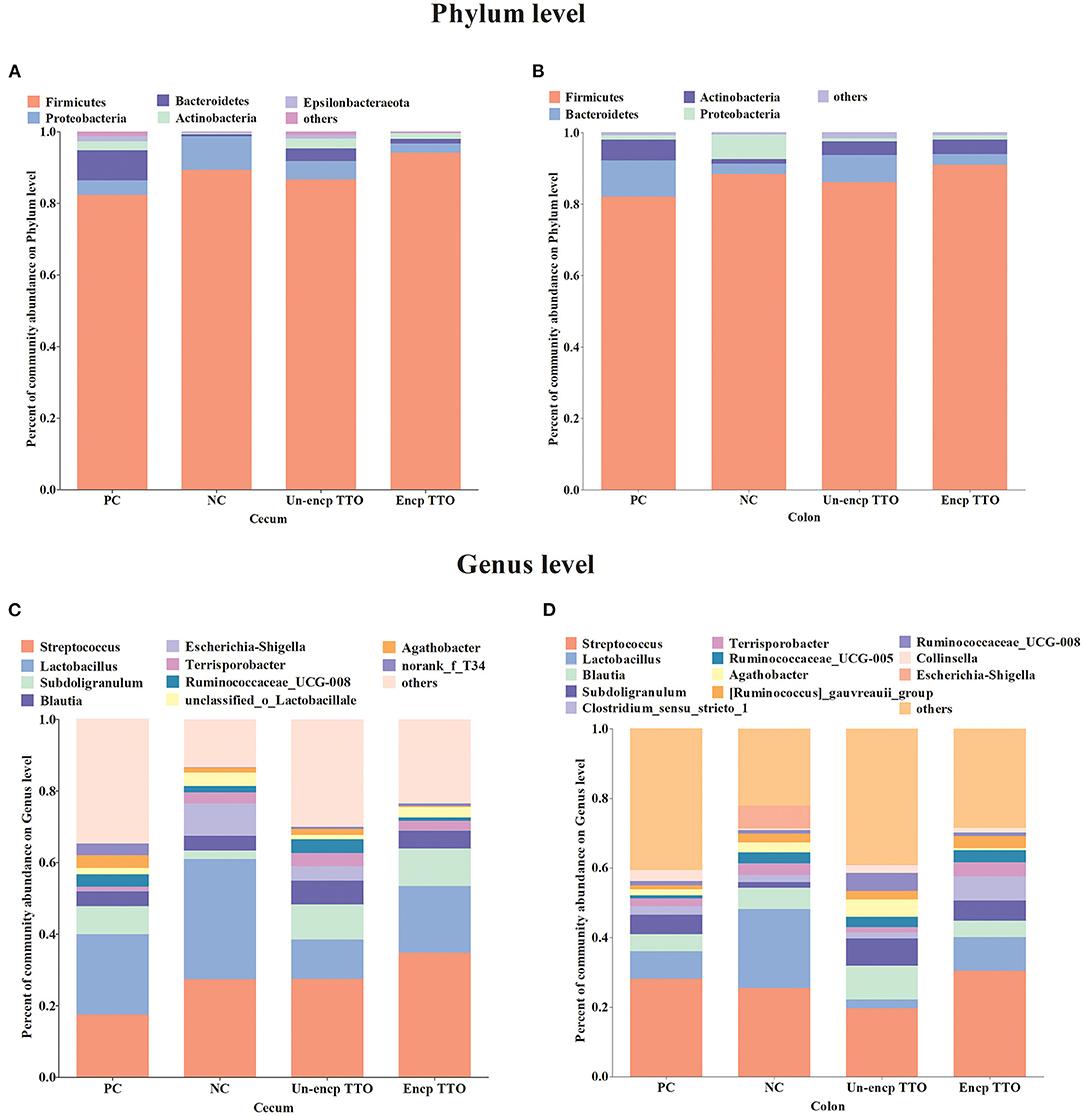
Figure 3. Effects of dietary treatment on intestinal bacterial communities of weaned pigs (n = 5). Bacterial taxa present at relative abundances of >0.01% and the proportion of other bacteria is <0.01% at the phylum level, whereas at the genus level, it was 0.03%. Experimental diets were control diet (NC), NC + 75 mg/kg aureomycin (PC), NC + 0.4% unencapsulated TTO (Un-encp TTO), and NC + 0.4% encapsulated TTO (Encp TTO). (A) Community barplot analysis for the cecal bacterial communities at the phylum level. (B) Community barplot analysis for the colonic bacterial communities at the phylum level. (C) Community barplot analysis for the cecal bacterial communities at the genus level. (D) Community barplot analysis for the colonic bacterial communities at the genus level.
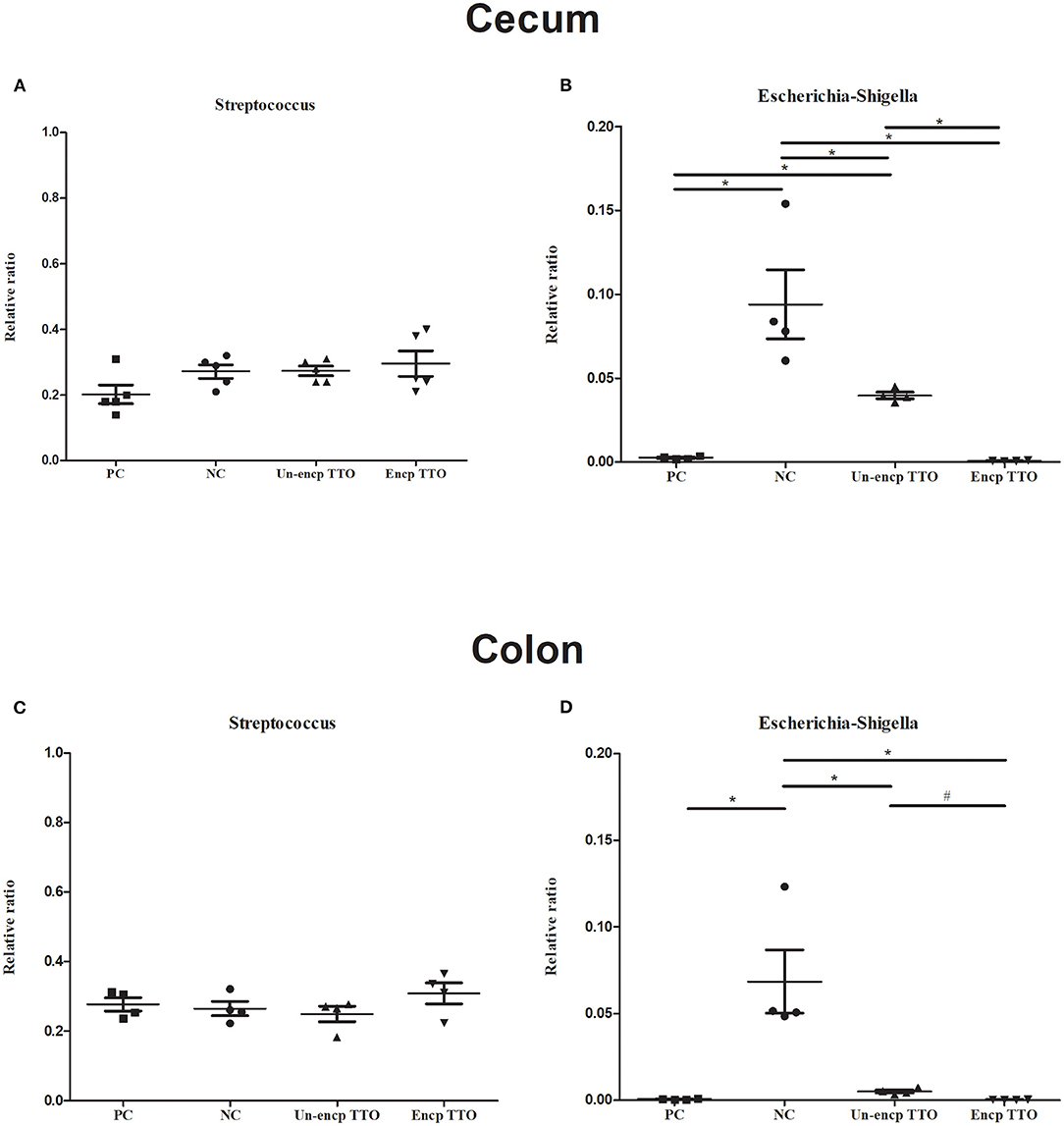
Figure 4. Effects of diet treatment on the relative abundance of Escherichia–Shigella and Streptococcus of weaned pigs at the genus level (n = 5). Experimental diets were control diet (NC), NC + 75 mg/kg aureomycin (PC), NC + 0.4% unencapsulated TTO (Un-encp TTO), and NC + 0.4% encapsulated TTO (Encp TTO). (A) The relative abundance of cecal Streptococcus. (B) The relative abundance of cecal Escherichia–Shigella. (C) The relative abundance of colonic Streptococcus. (D) The relative abundance of colonic Escherichia–Shigella. Error bars represent standard error of the mean. The p values are based on Kruskal–Wallis tests. *,#Differences were considered significant at p < 0.05 when compared with NC, Un-encp TTO groups, respectively.
Discussion
TTO comprises multiple identified constituents including terpinene, terpinolene, and terpineol, which account for more than 85% of the total mixture. Terpinen-4-ol accounts for nearly 60% of the major components of TTO. Because of the antimicrobial activity of its components (32–34), TTO exhibits in vitro antimicrobial activity (35). In the current study, we revealed TTO in vivo may be a potential alternative to antibiotics in weanling pigs. In addition, we further investigated the mechanisms of TTO function.
Our observation that TTO increased ADG and improved feed conversion rate is consistent with previously reported studies of TTO (9, 10) and terpinene in an essential oil blend (36). The positive effect on ADG and feed conversion rate may be related to the increase in feed intake promoted by TTO. TTO likely contributed to elevated feed intake by two mechanisms. Many types of essential oils emit appealing smells to arouse pigs' interest in feed, whereas other essential oils emit aversive smells that may deter piglets from feed intake [reviewed by Zeng (15)]. TTO appeared to positively stimulate pig appetite as evidenced by the increased feed intake of pigs over the experimental period. Encapsulated TTO induced even higher feed intake, which previously hid TTO smells. This phenomenon indicated the proper coating process of TTO that favored animal sensory. Feed intake is regulated by postingestional signals such as released gastrointestinal hormones or stimulus from gastrointestinal nerves (37). Higher feed intake indicates an expressed well-being process of digestion (38). Encapsulation process of TTO preserved its activity from being oxidized and volatizing to air. Higher activity of encapsulated TTO during digestion by improving nutrient utilization contributed to benefit feed intake.
TTO increased digestibility of protein, fat, and DM in diets. These results are consistent with feeding other essential oils to pigs (9, 39–41) and broilers (42). The above essential oils are similar to TTO and contain numerous monoterpenes and sesquiterpene compounds. Essential oils stimulate secretion of saliva and bile, leading to elevated activity of digestive enzymes (43). High monoterpene present in essential oils has spasmolytic effect to increase the interaction between feed and digestive enzymes (44). Improved VH and villous-to-crypt ratio in the jejunum may have led to higher absorption of digested nutrients, which contributed to increased ADG of weanling piglets. Improved morphology was associated with higher nutrient digestibility in previously reported studies (45).
In the current study, TTO showed increased antioxidant potential reflected by higher levels of serum SOD and GSH-Px compared with unsupplemented control and positive control pigs. TTO also enhanced the anti-inflammatory effect represented by higher levels of IL-10 in serum compared with NC- and PC-fed pigs. In addition, TTO treatment enhanced IgG level in serum compared with NC- and PC-fed pigs. For many types of essential oils, enhanced antioxidant potential often occurs concurrently with greater anti-inflammation and greater immunoglobulin levels in serum (46–48). The systemically beneficial effects of TTO helped protect the liver from injury, which was marked by lower levels of serum ALT and ASP. In a study of uninfected animals, TTO did not stimulate immune responses (49). When rats and sheep were infected by bacteria or gastrointestinal parasites, TTO helped protect the animals by activating cellular and humoral immune responses and decreasing levels of ALT and ASP (50, 51). Previous study observed that Escherichia coli challenge caused oxidative injury when compared with the unchallenged pigs (52). In the current study, TTO impressively inhibited abundance of E. coli–Shigella in cecum and colon. Meanwhile, we found three mycotoxins (DON, ZEA, and AFB1) present in the experimental diets of this study. Animals that ingest mycotoxin-contaminated diets exhibit reduced feed intake and growth rate (53, 54). Mycotoxins also promoted inflammatory injury of livers in piglets (55–57). Mycotoxins are produced by fungi such as Aspergillus, Fusarium, Penicillium, and Alternaria (58, 59). These fungal genera are found commonly in crops and cereals, especially when storage conditions are humid and temperature is not well controlled (60), leading to contaminated feed [reviewed by Pitt et al. (61)]. In China, mycotoxins in feed are widely present (62). Terpinene-4-ol and gamma-terpinene, active components in TTO, can inhibit growth of toxic fungi in vitro and thus inhibit production of mycotoxins (35, 63). In this study, Encp TTO was prepared with antioxidant, which helped it maintain more antioxidant activity of TTO and explain why Encp TTO exhibited better effects on antioxidation and anti-inflammation compared with its unencapsulated counterpart.
Diarrhea in weaned piglets causes severe economic loss globally. Infections are mainly colibacillosis diarrhea (64). In China, especially in warm and rural areas, the infection rate in weaned piglets was 25%, and the death rate reached 21% (65). Weaning is a critical period for piglets when piglets encounter multiple stressors such as changes of diets, social interactions, and environment (66). These stressors usually damage intestinal structures, making pigs more susceptible to infection, and induce unbalanced gut microbiota (67). In vitro, TTO has antibacterial activity by disrupting the microbial cell membrane which leads to loss of chemiosmotic control (68, 69). In the current in vivo study, TTO impressively inhibited abundance of E. coli–Shigella in cecum and colon, and lowered diarrhea rate of weanling piglets was comparable to that of PC supplementation in diets. Shigella are taxonomically part of E. coli species characterized by invasion of colonic and rectal mucosa, which provokes a strong inflammatory response and destruction of colonic epithelium (70). Signs of shigellosis include severe, bloody diarrhea and mucous in feces. Shigella are the principal bacterial cause of sustained endemic diarrhea (71). Shigella species have developed antibiotic resistance in the last 20 years (72–74). In this respect, TTO has potential to control Shigella-caused diarrhea in weanling piglets. When comparing the effective difference between unencapsulated TTO and encapsulated TTO, we found that encapsulated TTO reduced Shigella abundance in the cecum and in the colon, where Shigella specifically invade. This is another example that supports the notion that encapsulation preserves more activities of TTO from loss of function in digestion and cross-link reactions in gastrointestinal tract.
On the phylum level, TTO treatment led to higher counts of dominant phyla (Firmicutes and Bacteroidetes) and fewer Proteobacteria than in NC treatment. The bacteria of Firmicutes and Bacteroidetes in gut microbiota can ferment carbohydrates to a variety of short-chain fatty acids (SCFAs), and strengthen intestinal barrier functions (75, 76). An increased proportion of Proteobacteria is a potential signature of microbial dysbiosis and risk of intestinal diseases (77). On the genus level, TTO treatment caused higher relative abundance of Subdoligranulum and Clostridium_sensu_stricto_1 in digesta than NC treatment. Clostridium_sensu_stricto is reported to produce SCFAs and promote the intestinal mucus barrier (78, 79). Subdoligranulum has anti-inflammatory activity and plays a key role in human colon health as a butyrate-producing bacterium (80–82). These results demonstrate that TTO (especially encapsulated TTO) reduces diarrhea rate by reducing pathogenic E. coli and improving the abundance of beneficial bacteria in weanling pigs.
Conclusions
TTO (regardless of encapsulation) improved growth performance, diarrhea rate, immune responses, antioxidative activity, and bacterial composition in gastrointestinal tract of weaned piglets. Encapsulation of TTO enhanced TTO effects on the above indexes. Besides, encapsulated TTO significantly reduced diarrhea rate and reduced the abundance of E. coli–Shigella in colon and cecum, which were similar to the antibiotic treatment. This result indicated encapsulated TTO as a potential alternative of feed antibiotics for weaned pigs.
Data Availability Statement
The datasets presented in this study can be found in online repositories. The names of the repository/repositories and accession number(s) can be found below: PRJNA677843.
Ethics Statement
The animal study was reviewed and approved by Chinese Guidelines for Animal Welfare and approved by the China Agricultural University Institutional Animal Care and Use Committee. Written informed consent was obtained from the owners for the participation of their animals in this study.
Author Contributions
LW and BD designed and wrote the manuscript. YZ and LL critically edited the text. LW, LL, and BD finalized the manuscript. All authors read and approved the final manuscript.
Conflict of Interest
The authors declare that the research was conducted in the absence of any commercial or financial relationships that could be construed as a potential conflict of interest.
Publisher's Note
All claims expressed in this article are solely those of the authors and do not necessarily represent those of their affiliated organizations, or those of the publisher, the editors and the reviewers. Any product that may be evaluated in this article, or claim that may be made by its manufacturer, is not guaranteed or endorsed by the publisher.
References
1. Bomba L, Minuti A, Moisa SJ, Trevisi E, Eufemi E, Lizier M, et al. Gut response induced by weaning in piglet features marked changes in immune and inflammatory response. Funct Integr Genomics. (2014) 14:657–71. doi: 10.1007/s10142-014-0396-x
2. Hedegaard CJ, Lauridsen C, Heegaard PMH. Purified natural pig immunoglobulins can substitute dietary zinc in reducing piglet post weaning diarrhoea. Vet Immunol Immunopathol. (2017) 186:9–14. doi: 10.1016/j.vetimm.2017.02.001
3. Zeng Y, Tan X, Zhang L, Jiang N, Cao H. Identification and expression of fructose-1,6-bisphosphate aldolase genes and their relations to oil content in developing seeds of tea oil tree (Camellia oleifera). PLoS ONE. (2014) 9:e107422. doi: 10.1371/journal.pone.0107422
4. Yan L, Wang JP, Kim HJ, Meng QW, Ao X, Hong SM, et al. Influence of essential oil supplementation and diets with different nutrient densities on growth performance, nutrient digestibility, blood characteristics, meat quality and fecal noxious gas content in grower–finisher pigs. Livest Sci. (2010) 128:115–22. doi: 10.1016/j.livsci.2009.11.008
5. Pazyar N, Yaghoobi R, Bagherani N, Kazerouni A A. review of applications of tea tree oil in dermatology. Int J Dermatol. (2013) 52:784–90. doi: 10.1111/j.1365-4632.2012.05654.x
6. Xie S, Feng H, Yang F, Zhao Z, Hu X, Wei C, et al. Does dual reduction in chemical fertilizer and pesticides improve nutrient loss and tea yield and quality? A pilot study in a green tea garden in Shaoxing, Zhejiang Province, China. Environ Sci Pollut Res Int. (2019) 26:2464–76. doi: 10.1007/s11356-018-3732-1
8. Carson CF, Riley TV. Antimicrobial activity of the essential oil of Melaleuca alternifolia. Lett Appl Microbiol. (1993) 16:49–55. doi: 10.1111/j.1472-765X.1993.tb00340.x
9. Zhang G, Zhao J, Dong W, Song X, Zang J, Ni S, et al. Effects of tea tree oil supplementation on growth performance, antioxidant capacity, immune status and microbial community in weaned pigs. Arch Anim Nutr. (2021) 1–16. doi: 10.1080/1745039X.2021.1877074
10. Dong L, Liu J, Zhong Z, Wang S, Wang H, Huo Y, et al. Dietary tea tree oil supplementation improves the intestinal mucosal immunity of weanling piglets. Anim Feed Sci Technol. (2019) 255. doi: 10.1016/j.anifeedsci.2019.114209
11. Shunan W, Li D, Zhenwu W, Yongjiu H, Wei F, Feifei F, et al. Effects of tea tree oil on growth performance, serum biochemical and viscera indexes of weaned piglets. China Anim Husb Vet Med. (2018) 45:86–92. doi: 10.16431/j.cnki.1671-7236.2018.01.011
12. Martín Á, Varona S, Navarrete A, Cocero MJ. Encapsulation and Co-Precipitation Processes with Supercritical Fluids: Applications with Essential Oils. Open Chem Eng. (2010) 4:31–41. doi: 10.2174/1874123101004020031
13. Grumezescu AM, Chifiriuc MC, Saviuc C, Grumezescu V, Hristu R, Mihaiescu DE, et al. Hybrid nanomaterial for stabilizing the antibiofilm activity of Eugenia carryophyllata essential oil. IEEE Trans Nanobioscience. (2012) 11:360–5. doi: 10.1109/tnb.2012.2208474
14. Rudback J, Bergstrom MA, Borje A, Nilsson U, Karlberg AT. alpha-Terpinene, an antioxidant in tea tree oil, autoxidizes rapidly to skin allergens on air exposure. Chem Res Toxicol. (2012) 25:713–21. doi: 10.1021/tx200486f
15. Zeng Z, Zhang S, Wang H, Piao X. Essential oil and aromatic plants as feed additives in non-ruminant nutrition: a review. J Anim Sci Biotechnol. (2015) 6:7. doi: 10.1186/s40104-015-0004-5
16. Das S, Singh VK, Dwivedy AK, Chaudhari AK, Dubey NK. Anethum graveolens essential oil encapsulation in chitosan nanomatrix: investigations on in vitro release behavior, organoleptic attributes, and efficacy as potential delivery vehicles against biodeterioration of rice (Oryza sativa L) food and bioprocess technology. (2021) 14:831–53. doi: 10.1007/s11947-021-02589-z
17. Alven S, Aderibigbe BA. Nanoparticles formulations of artemisinin and derivatives as potential therapeutics for the treatment of cancer, leishmaniasis and malaria. Pharmaceutics. (2020) 12:748. doi: 10.3390/pharmaceutics12080748
18. Shim TS, Kim S-H, Yang S-M. Elaborate design strategies toward novel microcarriers for controlled encapsulation and release. Particle & Particle Systems Characterization. (2013) 30:9–45. doi: 10.1002/ppsc.201200044
19. Adams RP. Identification of essential oil components by gas chromatography/ mass spectrometry. Carol Stream: Allured. (2001).
20. Abdel-Wahhab MA, El-Nekeety AA, Hassan NS, Gibriel AAY, Abdel-Wahhab KG. Encapsulation of cinnamon essential oil in whey protein enhances the protective effect against single or combined sub-chronic toxicity of fumonisin B(1) and/or aflatoxin B(1) in rats. Environ Sci Pollut Res Int. (2018) 25:29144–61. doi: 10.1007/s11356-018-2921-2
21. Hart GK, Dobb GJ. Effect of a fecal bulking agent on diarrhea during enteral feeding in the critically Ill. J Parenter Enteral Nutr. (1988) 12:465–8. doi: 10.1177/0148607188012005465
22. Wang L, Gong L, Zhu L, Peng C, Liao J, Ke L, et al. Effects of activated charcoal-herb extractum complex on the growth performance, immunological indices, intestinal morphology and microflora in weaning piglets. RSC Adv. (2019) 9:5948–57. doi: 10.1039/c8ra10283j
23. Daniel WA, Haduch A, Wojcikowski J. Inhibition and possible induction of rat CYP2D after short- and long-term treatment with antidepressants. J Pharm Pharmacol. (2002) 54:1545–52. doi: 10.1211/002235702162
24. Wang J, Liu Y, Yang Y, Bao C, Cao Y. High-level expression of an acidic thermostable xylanase in Pichia pastoris and its application in weaned piglets. J Anim Sci. (2020) 98:skz364. doi: 10.1093/jas/skz364
26. McCarthy JF, Aherne FX. Okai. DB Use of HCl insoluble ash as an index material for determining apparent digestibility with pigs. Can J Anim Sci. (1974) 54:107–9.
27. Murthy GK, Rhea U, Peeler JT. Levels of antimony, cadmium, chromium, cobalt, manganese, and zinc in institutional total diets. Environ. Sci. Technol. (1971) 5:436–42. doi: 10.1021/es60052a009
28. Omura T, Sato R. The carbon monoxide-binding pigment ofliver microsomes. J Biol Chem. (1964) 239:2379–85. doi: 10.1016/s0021-9258(20)82245-5
29. Zhang W, Bao C, Wang J, Zang J, Cao Y. Administration of Saccharomyces boulardii mafic-1701 improves feed conversion ratio, promotes antioxidant capacity, alleviates intestinal inflammation and modulates gut microbiota in weaned piglets. J Anim Sci Biotechnol. (2020) 11:112. doi: 10.1186/s40104-020-00516-4
30. Liu S, Ma C, Liu L, Ning D, Liu Y, Dong B. Beta-xylosidase and beta-mannosidase in combination improved growth performance and altered microbial profiles in weanling pigs fed a corn-soybean meal-based diet. Asian-Australas J Anim Sci. (2019) 1734–44. doi: 10.5713/ajas.18.0873
31. Liu T, Zhang AN, Wang J, Liu S, Jiang X, Dang C, et al. Integrated biogeography of planktonic and sedimentary bacterial communities in the Yangtze River. Microbiome. (2018) 6:16. doi: 10.1186/s40168-017-0388-x
32. Fahim M, Ibrahim M, Zahiruddin S, Parveen R, Khan W, Ahmad S, et al. TLC-bioautography identification and GC-MS analysis of antimicrobial and antioxidant active compounds in Musa × paradisiaca L fruit pulp essential oil. Phytochem Anal. (2019) 30:332–45. doi: 10.1002/pca.2816
33. Wróblewska A, Retajczyk M, Kadziołka D, Markowska-Szczupak A. Microbiological tests of natural limonene and the compounds obtained afte isomerization of limonene in the presence of Ti-SBA-15 Catalyst-α-Terpinene, γ-Terpinene, Terpinolene, and p-Cymene. J Cosmet Sci. (2019) 70:137–47.r
34. Yang TS, Chao LK, Liu TT. Antimicrobial activity of the essential oil of Glossogyne tenuifolia against selected pathogens. J Sci Food Agric. (2014) 94:2965–71. doi: 10.1002/jsfa.6641
35. Carson CF, Hammer KA, Riley TV. Melaleuca alternifolia (Tea Tree) oil: a review of antimicrobial and other medicinal properties. Clin Microbiol Rev. (2006) 19:50–62. doi: 10.1128/CMR.19.1.50-62.2006
36. Zhang S, Jung JH, Kim HS, Kim BY, Kim IH. Influences of phytoncide supplementation on growth performance, nutrient digestibility, blood profiles, diarrhea scores and fecal microflora shedding in weaning pigs. Asian-Australas J Anim Sci. (2012) 25:1309–15. doi: 10.5713/ajas.2012.12170
37. Rayner DV. Gastrointestinal satiety in animals other than man. Proc Nutr Soc. (1992) 51:1–6. doi: 10.1079/pns19920003
38. Wilson ME. Jr. DCL Considerations When Using Physiological Data in Assessing Animal Well-Being. J Anim Vet Adv. (2004) 3:613–25.
39. Maenner K, Vahjen W, Simon O. Studies on the effects of essential-oil-based feed additives on performance, ileal nutrient digestibility, and selected bacterial groups in the gastrointestinal tract of piglets. J Anim Sci. (2011) 89:2106–12. doi: 10.2527/jas.2010-2950
40. Li P, Piao X, Ru Y, Han X, Xue L, Zhang H. Effects of adding essential oil to the diet of weaned pigs on performance, nutrient utilization, immune response and intestinal health. Asian-Australas J Anim Sci. (2012) 25:1617–26. doi: 10.5713/ajas.2012.12292
41. Ahmed ST, Hossain ME, Kim GM, Hwang JA, Ji H, Yang CJ. Effects of resveratrol and essential oils on growth performance, immunity, digestibility and fecal microbial shedding in challenged piglets. Asian-Australas J Anim Sci. (2013) 26:683–90. doi: 10.5713/ajas.2012.12683
42. Mohebodini H, Jazi V, Ashayerizadeh A, Toghyani M, Tellez-Isaias G. Productive parameters, cecal microflora, nutrient digestibility, antioxidant status, and thigh muscle fatty acid profile in broiler chickens fed with Eucalyptus globulus essential oil. Poult Sci. (2021) 100:100922. doi: 10.1016/j.psj.2020.12.020
43. Xu YT, Liu L, Long SF, Pan L, Piao XS. Effect of organic acids and essential oils on performance, intestinal health and digestive enzyme activities of weaned pigs. Anim Feed Sci Technol. (2018) 235:110–9.
44. Magalhaes PJC, Criddle DN, Tavares RA, Melo EM, Mota TL, Leal-Cardoso JH. Intestinal myorelaxant and antispasmodic effects of the essential oil of Croton nepetaefolius and its constituents cineole, methyl-eugenol and terpineol. Phytotherapy Research. (1998) 12:172–7. doi: 10.1002/(sici)1099-1573(199805)12:3<172::Aid-ptr212>3.0.Co;2-e
45. Wang L, Yan S, Li J, Li Y, Ding X, Yin J, et al. Rapid Communication: The relationship of enterocyte proliferation with intestinal morphology and nutrient digestibility in weaning piglets. J Anim Sci. (2019) 97:353–8. doi: 10.1093/jas/sky388
46. Su G, Zhou X, Wang Y, Chen D, Chen G, Li Y, et al. Effects of plant essential oil supplementation on growth performance, immune function and antioxidant activities in weaned pigs. Lipids Health Dis. (2018) 17:139. doi: 10.1186/s12944-018-0788-3
47. Zeng Z, Xu X, Zhang Q, Li P, Zhao P, Li Q, et al. Effects of essential oil supplementation of a low-energy diet on performance, intestinal morphology and microflora, immune properties and antioxidant activities in weaned pigs. Anim Sci J. (2015) 86:279–85. doi: 10.1111/asj.12277
48. Park Y, Jung SM, Yoo SA, Kim WU, Cho CS, Park BJ, et al. Antinociceptive and anti-inflammatory effects of essential oil extracted from Chamaecyparis obtusa in mice. Int Immunopharmacol. (2015) 29:320–5. doi: 10.1016/j.intimp.2015.10.034
49. Baldissera MD, Da Silva AS, Oliveira CB, Vaucher RA, Santos RC, Duarte T, et al. Effect of tea tree oil (Melaleuca alternifolia) on the longevity and immune response of rats infected by Trypanosoma evansi. Res Vet Sci. (2014) 96:501–6. doi: 10.1016/j.rvsc.2014.03.013
50. Nachman RJ. Introduction: Invertebrate neuropeptides XIV. Peptides. (2014) 53:1–2. doi: 10.1016/j.peptides.2014.03.002
51. Grando TH, Baldissera MD, Gressler LT, de Sá MF, Bortoluzzi BN, Schafer AS, et al. Melaleuca alternifolia anthelmintic activity in gerbils experimentally infected by Haemonchus contortus. Exp Parasitol. (2016) 170:177–83. doi: 10.1016/j.exppara.2016.09.004
52. Jiménez MJ, Berrios R, Stelzhammer S, Bracarense A. Ingestion of organic acids and cinnamaldehyde improves tissue homeostasis of piglets exposed to enterotoxic Escherichia coli (ETEC). J Anim Sci. (2020) 98:skaa012. doi: 10.1093/jas/skaa012
53. Dersjant-Li Y, Verstegen MW, Gerrits WJ. The impact of low concentrations of aflatoxin, deoxynivalenol or fumonisin in diets on growing pigs and poultry. Nutr Res Rev. (2003) 16:223–39. doi: 10.1079/nrr200368
54. Nguyen-Ba H, Taghipoor M, van Milgen J. Modelling the feed intake response of growing pigs to diets contaminated with mycotoxins. Animal. (2020) 14:s303–s12. doi: 10.1017/s175173112000083x
55. Taranu I, Hermenean A, Bulgaru C, Pistol GC, Ciceu A, Grosu IA, et al. Diet containing grape seed meal by-product counteracts AFB1 toxicity in liver of pig after weaning. Ecotoxicol Environ Saf. (2020) 203:110899. doi: 10.1016/j.ecoenv.2020.110899
56. Skiepko N, Przybylska-Gornowicz B, Gajecka M, Gajecki M, Lewczuk B. Effects of deoxynivalenol and zearalenone on the histology and ultrastructure of pig liver. Toxins (Basel). (2020) 12:463. doi: 10.3390/toxins12070463
57. Haschek WM, Motelin G, Ness DK, Harlin KS, Hall WF, Vesonder RF, et al. Characterization of fumonisin toxicity in orally and intravenously dosed swine. Mycopathologia. (1992) 117:83–96. doi: 10.1007/bf00497283
58. Ozcan MM. Chalchat JC. Chemical composition and antifungal activity of rosemary (Rosmarinus officinalis L) oil from Turkey. Int J Food Sci Nutr. (2008) 59:691–8. doi: 10.1080/09637480701777944
59. Morcia C, Malnati M, Terzi V. In vitro antifungal activity of terpinen-4-ol, eugenol, carvone, 1,8-cineole (eucalyptol) and thymol against mycotoxigenic plant pathogens. Food Addit Contam Part A Chem Anal Control Expo Risk Assess. (2012) 29:415–22. doi: 10.1080/19440049.2011.643458
60. Peraica M, Radić B, Lucić A, Pavlović M. Toxic effects of mycotoxins in humans. Bull World Health Organ. (1999) 77:754–66.
61. Pitt JI, Basílico JC, Abarca ML, López C. Mycotoxins and toxigenic fungi. Med Mycol. (2000) 38 Suppl 1:41–6.
62. Zhao L, Zhang L, Xu Z, Liu X, Chen L, Dai J, et al. Occurrence of Aflatoxin B(1), deoxynivalenol and zearalenone in feeds in China during 2018-2020. J Anim Sci Biotechnol. (2021) 12:74. doi: 10.1186/s40104-021-00603-0
63. Hammer KA, Carson CF, Riley TV. Antifungal effects of Melaleuca alternifolia (tea tree) oil and its components on Candida albicans, Candida glabrata and Saccharomyces cerevisiae. J Antimicrob Chemother. (2004) 53:1081–5.
64. Fairbrother JM, Nadeau E, Gyles CL. Escherichia coli in postweaning diarrhea in pigs: an update on bacterial types, pathogenesis, and prevention strategies. Anim Health Res Rev. (2005) 6:17–39. doi: 10.1079/ahr2005105
65. Ding L, Zhang Y. Investigation on the incidence of diarrhea in rural piglets and its prevention and control measures. (Chinese) Heilongjiang Animal Sceince and Veterinary Medicine. (2016) (08):116–9.
66. Lallès JP, Bosi P, Smidt H, Stokes CR. Nutritional management of gut health in pigs around weaning. Proc Nutr Soc. (2007) 66:260–8. doi: 10.1017/s0029665107005484
67. Gresse R, Chaucheyras-Durand F, Fleury MA, Van de Wiele T, Forano E, Blanquet-Diot S. Gut Microbiota Dysbiosis in Postweaning Piglets: Understanding the Keys to Health. Trends Microbiol. (2017) 25:851–73. doi: 10.1016/j.tim.2017.05.004
68. Cox SD, Mann CM, Markham JL, Bell HC, Gustafson JE, Warmington JR, et al. The mode of antimicrobial action of the essential oil of Melaleuca alternifolia (tea tree oil). J Appl Microbiol. (2000) 88:170–5. doi: 10.1046/j.1365-2672.2000.00943.x
69. Li M, Zhu L, Liu B, Du L, Jia X, Han L, et al. Tea tree oil nanoemulsions for inhalation therapies of bacterial and fungal pneumonia. Colloids Surf B Biointerfaces. (2016) 141:408–16. doi: 10.1016/j.colsurfb.2016.02.017
70. Belotserkovsky I, Sansonetti PJ. Shigella and Enteroinvasive Escherichia Coli. Curr Top Microbiol Immunol. (2018) 416:1–26. doi: 10.1007/82_2018_104
71. Baker S, The HC. Recent insights into Shigella. Curr Opin Infect Dis. (2018) 31:449–54. doi: 10.1097/qco.0000000000000475
72. Chung The H, Baker S. Out of Asia: the independent rise and global spread of fluoroquinolone-resistant Shigella. Microb Genom. (2018) 4. doi: 10.1099/mgen.0.000171
73. Chung The H, Rabaa MA, Pham Thanh D, De Lappe N, Cormican M, Valcanis M. South Asia as a reservoir for the global spread of ciprofloxacin-resistant Shigella Sonnei: a cross-sectional study. PLoS Med. (2016) 13:e1002055. doi: 10.1371/journal.pmed.1002055
74. Baker KS, Dallman TJ, Ashton PM, Day M, Hughes G, Crook PD, et al. Intercontinental dissemination of azithromycin-resistant shigellosis through sexual transmission: a cross-sectional study. Lancet Infect Dis. (2015) 15:913–21. doi: 10.1016/s1473-3099(15)00002-x
75. Huang Y, Shi X, Li Z, Shen Y, Shi X, Wang L, et al. Possible association of Firmicutes in the gut microbiota of patients with major depressive disorder. Neuropsychiatr Dis Treat. (2018) 14:3329–37. doi: 10.2147/NDT.S188340
76. Duncan SH, Louis P, Flint HJ. Cultivable bacterial diversity from the human colon. Lett Appl Microbiol. (2007) 44:343–50. doi: 10.1111/j.1472-765X.2007.02129.x
77. Shin NR, Whon TW, Bae JW. Proteobacteria: microbial signature of dysbiosis in gut microbiota. Trends Biotechnol. (2015) 33:496–503. doi: 10.1016/j.tibtech.2015.06.011
78. Cheng, Kong, Renyuan, Gao, Xuebing, Yan. Probiotics improve gut microbiota dysbiosis in obese mice fed a high-fat or high-sucrose diet. Nutrition. (2019) 60:175–84. doi: 10.1016/j.nut.2018.10.002
79. Wlodarska M, Willing BP, Bravo DM, Finlay BB. Phytonutrient diet supplementation promotes beneficial Clostridia species and intestinal mucus secretion resulting in protection against enteric infection. Sci Rep. (2015) 5:9253. doi: 10.1038/srep09253
80. Song R, Yao J, Shi Q, Wei R. Nanocomposite of half-fin anchovy hydrolysates/zinc oxide nanoparticles exhibits actual non-toxicity and regulates intestinal microbiota, short-chain fatty acids production and oxidative status in mice. Mar Drugs. (2018) 16:23. doi: 10.3390/md16010023
81. Ferreira-Halder CV, Faria AVS, Andrade SS. Action and function of Faecalibacterium prausnitzii in health and disease. Best Pract Res Clin Gastroenterol. (2017) 31:643–8. doi: 10.1016/j.bpg.2017.09.011
Keywords: tea tree oil, encapsulated, weaned pig, growth performance, antioxidant, intestinal microbiota
Citation: Wang L, Zhang Y, Liu L, Huang F and Dong B (2021) Effects of Three-Layer Encapsulated Tea Tree Oil on Growth Performance, Antioxidant Capacity, and Intestinal Microbiota of Weaned Pigs. Front. Vet. Sci. 8:789225. doi: 10.3389/fvets.2021.789225
Received: 04 October 2021; Accepted: 29 October 2021;
Published: 02 December 2021.
Edited by:
Manuel Gonzalez Ronquillo, Universidad Autónoma del Estado de México, MexicoReviewed by:
Marcos Elias Duarte, North Carolina State University, United StatesAyhan Filazi, Ankara University, Turkey
Copyright © 2021 Wang, Zhang, Liu, Huang and Dong. This is an open-access article distributed under the terms of the Creative Commons Attribution License (CC BY). The use, distribution or reproduction in other forums is permitted, provided the original author(s) and the copyright owner(s) are credited and that the original publication in this journal is cited, in accordance with accepted academic practice. No use, distribution or reproduction is permitted which does not comply with these terms.
*Correspondence: Bing Dong, ZG9uZ2JpbmcmI3gwMDA0MDtjYXUuZWR1LmNu